Intravascular Ultrasound in the Endovascular Treatment of Patients With Peripheral Arterial Disease: Current Role and Future Perspectives
- Department of Vascular Medicine and Interventional Radiology, François-Mitterrand University Hospital, Dijon, France
Over the last decade, intravascular ultrasound (IVUS) has emerged as a useful adjunctive tool to angiography in an increasing number of catheter-based procedures for peripheral arterial disease (PAD). IVUS catheters offer accurate cross-sectional imaging of arterial vessels with high dimensional accuracy and provide accurate information about lesion morphology. IVUS enables assessment of the plaque morphology, vessel diameter, and the presence of arterial dissections. Furthermore, IVUS is able to properly guide the best choice of appropriate percutaneous transluminal angioplasty (PTA) technique, guide the delivery of different devices, and assess the immediate result of any endovascular intervention. In the present review, the role of IVUS for PAD will be discussed, specifically the applications of IVUS technology during interventional procedures including PTA, stent sizing, crossing total occlusion, assessing residual narrowing and stent apposition and expansion, and atherectomy. Future perspectives of IVUS-guided treatments and cost-effectiveness of the systematic use of IVUS during endovascular interventions will be also discussed.
Introduction
Advances in endovascular techniques and devices have led to the increased use of percutaneous procedures to treat peripheral arterial disease (PAD) over the last decade (1). Smaller delivery devices, better quality materials for balloons and stents, more predictable delivery mechanisms, increased familiarity among vascular specialists using microguide wires, and the ever-aging population at risk have led to an important increase in the number of vascular interventions being performed (2). Because of this increase in the volume of procedures performed, more emphasis should be placed on precise imaging modalities to guide such procedures. Digital subtraction angiography (DSA) remains a widely used imaging modality during endovascular peripheral procedures and is still the gold standard (2). However, traditional DSA has its limitations by underestimating several morphological aspects of atherosclerotic lesions and by its ability to only display the outline of the vessel lumen.
Intravascular ultrasound (IVUS) has emerged as an important adjunctive modality to DSA in the guidance of coronary percutaneous transluminal angioplasty (PTA) (3). However, its use for peripheral applications is less popular (4–6). IVUS is the ideal imaging tool to help guide peripheral endovascular procedures because of its ease and accuracy at determining different imaging parameters, including luminal cross-sectional measurements and accurate information about lesion morphology such as true vessel diameters, wall thickness/layers, length, shape, and volume of lesion, position of lesion within the lumen (concentric or eccentric), type of lesion (fibrous, necrotic, calcified and mixed), any presence and extent of intimal flap, arterial dissection, plaque ulceration, presence and volume of thrombus (5). IVUS does not only provide diagnostic information per-procedure, but it also may guide the choice of the appropriate PTA method, assist in the accurate deployment of an endovascular device, and check the efficiency of the procedure. In this article, we will discuss the current role and future perspectives of IVUS in the endovascular management of PAD from a practical point of view.
Technical Aspects
By coupling IVUS catheters with real-time computerized processing devices, this technology has been transformed over the last decade into a user-friendly tool that may give three-dimensional information in order to facilitate endovascular arterial procedures (1, 5). IVUS during vascular interventions offers valuable information about plaque morphology, vessel diameter and lesion severity, lumen area, detection of calcium severity and thrombus, detection of dissections, stent apposition and expansion. IVUS catheters are currently provided by two companies, Philips and Boston Scientific. The catheter sizes range from 2- to 4-French (Fr) and can be easily guided through a 5- or 6-Fr sheath. Larger IVUS catheters are also used for larger peripheral vessel applications and require larger sheaths. The larger IVUS catheters come over 0.035-inch guidewires, and the smaller ones that are more often used for infrainguinal procedures require 0.018-inch or 0.014-inch guidewires, similar to the small-profile balloons or stents used most often in this anatomical location (Figure 1). The length of IVUS catheters ranges from 90 to 150 cm, allowing imaging of infra-popliteal arteries via contralateral approach (5, 6).

Figure 1. Commonly used peripheral vascular intravascular ultrasound catheter. It comes on a 0.014- or 0.035-inch guidewire with monorail system.
IVUS uses a piezoelectric transducer generating sound waves after electrical stimulation at the tip of the catheter. Propagation of the waves into different tissues produces a reflection image based on the acoustic properties of each tissue. First images provided by IVUS technology were in gray-scale and plaque morphology was visually classified based on the echogenicity of the plaque compared to its surrounding adventitia. Thus, 20–40 MHz transducers were routinely used (1, 5). The classification of plaques was then as follows: (1) soft, (2) fibrous, (3) calcified, and (4) mixed plaques. Later, virtual histology-IVUS (VH-IVUS) came up to the market, providing better evaluation and characterization of the histological composition of the arterial plaque thanks to the analysis of an additional low radiofrequency (RF) content. In addition to the gray-scale, this RF signal is processed with an autoregressive model before being matched to color coded histological databases in order to classify plaques based on their morphological composition. Using VH-IVUS plaques may be classified as follows: (1) fibrous tissue, (2) fibro fatty plaque, (3) necrotic core and (4) calcification (1, 6). New RF-based IVUS modalities have evolved including integrated backscattered IVUS (IB-IVUS). They use more sophisticated software algorithms allowing better tissue characterization.
Current Role of IVUS in Peripheral Arterial Disease
Main data regarding the utility of IVUS in the guidance of lower limb revascularization procedures in patients with PAD and its potential contribution in prolonging the durability of this therapeutic approach are coming mainly from retrospective studies (7). In a systematic review conducted in 2017, Makris et al. identified 13 clinical studies, which evaluated IVUS as an adjunct tool to DSA during endovascular interventions in patients with PAD in iliac, femoral or popliteal arteries (7). The majority were retrospective cohorts with a total number of 2,258 patients having had IVUS as part of their treatment. Out of 13 studies, 7 studies investigated the role of IVUS for PTA and stenting of PAD, 5 studies used IVUS to guide true-lumen reentry and one study tested IVUS during atherectomy. Some more recent studies also investigated the role of IVUS in the management of patients with PAD. Tables 1, 2 summarize characteristics and outcomes of the main studies evaluating IVUS in the endovascular management of PAD.
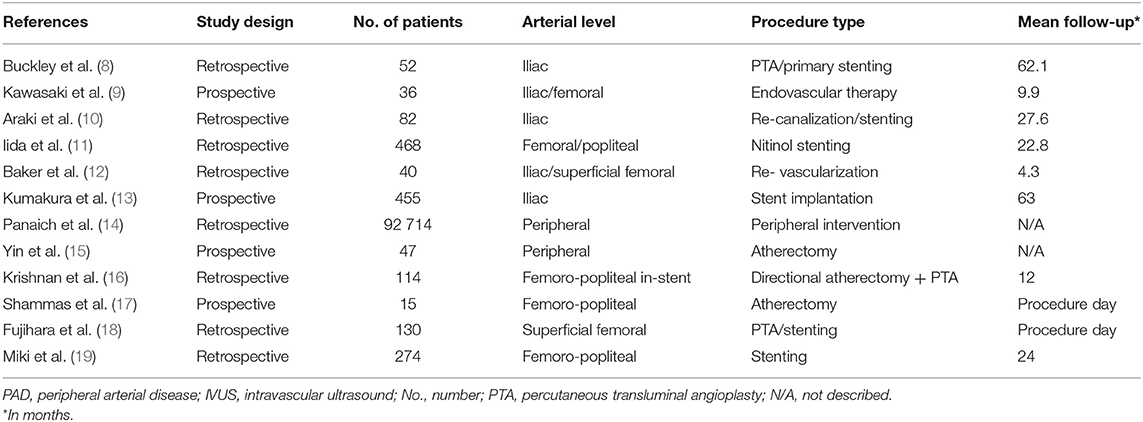
Table 1. Summary of characteristics the main studies assessing IVUS in the endovascular management of PAD.
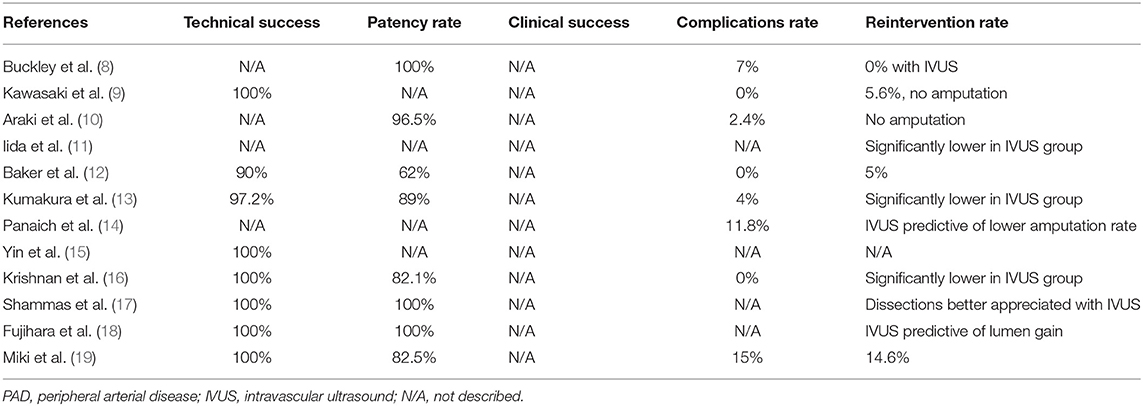
Table 2. Summary of outcomes of the main studies assessing IVUS in the endovascular management of PAD.
IVUS and PTA/Stenting
Seven studies reported results regarding the role of IVUS for PTA and stenting of PAD (8–14). Four of them were retrospective studies, investigating a total number of 2,258 patients, and comparing PTA and stenting in such a setting with or without the use of IVUS. In total, 1,589 patients were in the IVUS group (8, 11, 12, 14). Long-term patency rates ranged from 62 to 100% in the IVUS group vs. 69 to 83.4% in the non-IVUS group. Follow-up ranged from 4 to 62 months (8, 11, 12, 14). Three of these studies reported free from re-intervention and event free survival data (8, 11, 14). Iida et al. investigated the efficacy of IVUS in femoro-popliteal stenting for PAD with TASC II class A to C lesions (11). The author found a statistically significant difference in favor of the IVUS group in a total of 468 patients. Indeed, IVUS use was associated with a significantly higher 5-year primary patency (65 ± 6% vs. 35 ± 6%, P < 0.001) rate, better freedom from any adverse limb event rate (P < 0.001) and better event-free survival rate (P < 0.001).
Panaich et al. analyzed data from the Healthcare Cost and Utilization Project Nationwide Inpatient Sample on peripheral endovascular procedures performed between 2006 and 2011 (n = 92,714; 55% men; mean age, 60 years) (14). Overall, IVUS was used in 1.4% of cases analyzed. IVUS use during lower limb arterial interventions was predictive of lower post-procedural complication and amputation (OR = 0.59; 95%CI, 0.45–0.77; P < 0.001) rates with a non-significant increase in hospitalization costs. The overall rate of amputation was 9.7%, with a lower rate in the IVUS group (5.3%) compared to the group without IVUS (9.8%, P < 0.001). Baker et al. was the only to report three events of post-procedural complications in the IVUS group (12).
One explanation regarding these results may be the possible superiority and higher accuracy of IVUS compared to DSA alone. Indeed, the evaluation of vessel plaque morphology and vessel size is a major component of peripheral endovascular procedures. Historically, DSA has been considered the gold standard for assessment of vessel size and endovascular treatment. However, DSA has some limitations such as providing a two-dimensional image of a three-dimensional luminal structure (2). It mainly focuses on the lumen and confounding artifacts that derive from the arterial wall motion can be created (4, 20, 21). On the other hand, due to the direct view of the arterial wall, IVUS permits detailed information not only about the lumen, but also about plaque morphology, composition and vessel structure. Furthermore, while DSA underestimates, IVUS evaluates accurately the degree of stenosis and may contribute to the detection of the cause of technical failure (5–7). IVUS does not only provide diagnostic information per-procedure, but it also may guide the choice of the appropriate PTA method, assist in the accurate deployment of an endovascular device, and check the efficiency of the procedure more accurately than DSA.
Pliagas et al. recently conducted a retrospective study on a population of 43 patients who underwent an endovascular treatment using DSA and IVUS imaging modality (22). In total, measurements estimated from DSA images were significantly smaller than those obtained with IVUS imaging analysis. The author concluded that IVUS appears to give a higher degree of accuracy in measuring vessel lumen size. As measurements obtained from DSA under-estimated vessel diameter, the use of IVUS may help in determining the treatment algorithms and lead to improve the endovascular outcomes. IVUS also allows mandatory measurements regarding stent expansion. As a result, it is a powerful tool for the recognition of under deployment, which is the main cause of restenosis (7, 22).
In the three other studies of the meta-analysis, all lower limb revascularization interventions were guided by IVUS (9, 10, 13). Overall, 573 patients were included and follow-up ranged from 10 to 63 months. According to data from the first 5 years after PTA or stenting, long-term patency ranged from 89 to 96.5% and overall survival was from 82 to 100%. Periprocedural complications were reported only by Kumakura et al. in 18 (4%) of the 455 patients (13). Amputation rate was nil in the 3 studies. In addition, Kumakura et al. reported a 71% freedom from MACLE (Major Adverse Cardiovascular and Limb Events) during a 5-year post-procedural period. The favorable long-term patency results from these 3 studies that evaluated only IVUS-guided PTA or stenting also support the efficacy, durability and superiority of this technique, despite the absence of comparative groups. Specifically, Kumakura et al. demonstrated a 5-, 10-, and 15-year patency of 89, 83, and 75%, respectively, with no statistically significant differences among TASC-II categories (13).
IVUS and Atherectomy
One prospective study assessed the use of IVUS in the guidance of atherectomy in a population of 30 patients and showed an encouraging 100% technical success rate (23). At follow-up, long-term patency was 90% and clinical success was 100%. There were no periprocedural complications or major amputations. However, a revascularization rate of 10% was reported.
More recently, Krishnan et al. retrospectively compared 1-year outcomes for patients with femoro-popliteal in-stent restenosis using directional atherectomy guided by IVUS vs. directional atherectomy guided by DSA (16). Directional atherectomy guided by IVUS reduced clinically driven target lesion revascularization (CD-TLR) for patients with femoro-popliteal in-stent restenosis. Indeed, IVUS in conjunction with directional atherectomy may improve CD-TLR rates for femoro-popliteal in-stent restenosis patients by allowing the operator the ability to more accurately visualize the lesion than with DSA, and thereby minimize residual stenosis post-directional atherectomy treatment through aggressive debulking.
Interestingly, results from the CliRpath Excimer Laser System to Enlarge Lumen Openings (CELLO) registry including patients treated with specific excimer laser systems for the vascular management of PAD affecting the superficial femoral and proximal popliteal arteries were recently reported (24). The goal of this study was to evaluate, via IVUS, the dissections in the arterial wall after treatment with the laser devices. Treatments using the specific Excimer laser catheters resulted in a significant increase in lumen area of 5.5 ± 3.2 mm2 (95%CI, 4.3–6.8, P < 0.0001) and reduction in the volume of plaque plus media of −10.6 ± 36.0 mm3 (95%CI, −25.8 to 4.6, P = 0.1619) whilst giving rise to intramural hematoma after Turbo-Booster laser therapy in 55% of frames evaluated and 24% medial dissections with <1% of adventitial disruption. The Excimer laser-based Turbo-Booster management of PAD resulted in significant plaque removal and increased lumen size with almost no adventitial layer injury (24). Figures 2, 3 illustrate the usefulness of IVUS after atherectomy.
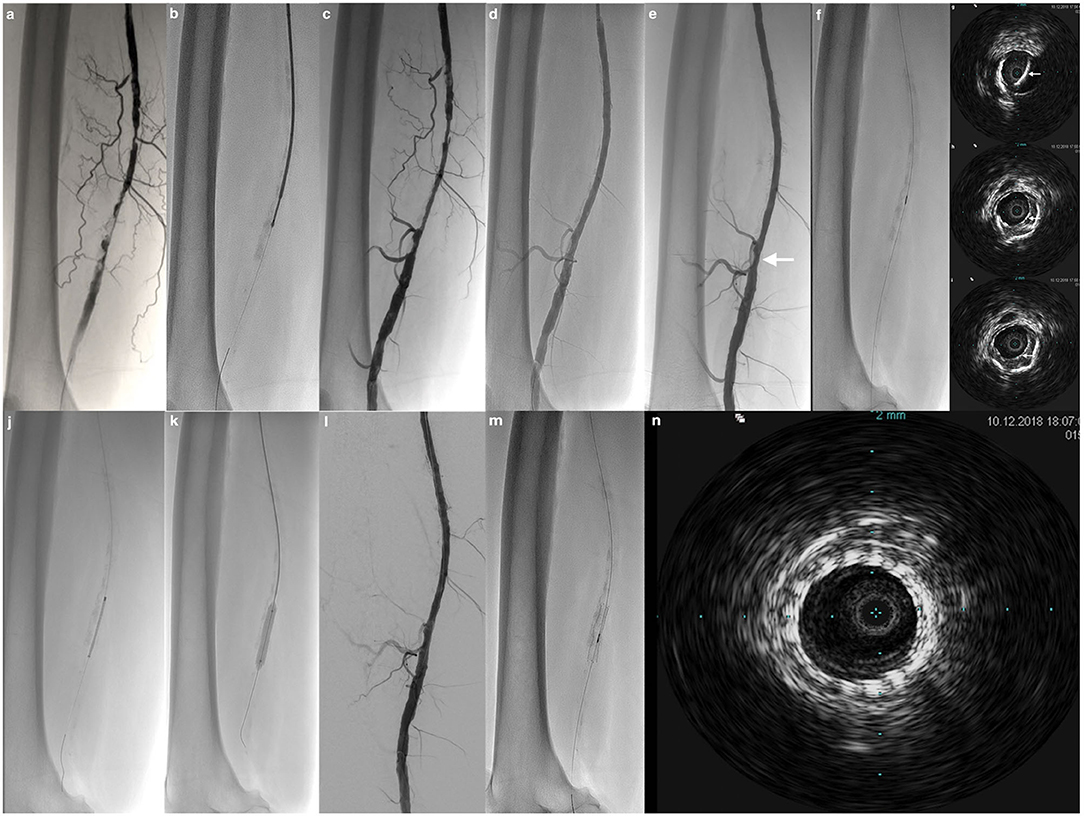
Figure 2. Limping patient of 67-year old. (a) Antegrade angiography by common femoral approach shows pre-occlusive calcified stenotic lesion of the mid and distal part of the right superficial femoral artery. (b) Atherectomy with the Phoenix device. (c) Control after atherectomy shows lumen gain. (d) Control after conventional PTA demonstrates no residual stenosis. (e) Result after application of drug-coated balloon shows no significant focal residual stenosis (arrow). (f) Checking with a 0.014-inch IVUS catheter. (g–i) IVUS demonstrates very well a focal intimal dissection post-angioplasty at the level of the focal residual stenosis, not visible at angiogram (arrows). (j–l) Result after spot stenting. (m,n) Final IVUS control shows normal arterial lumen with stent patency.
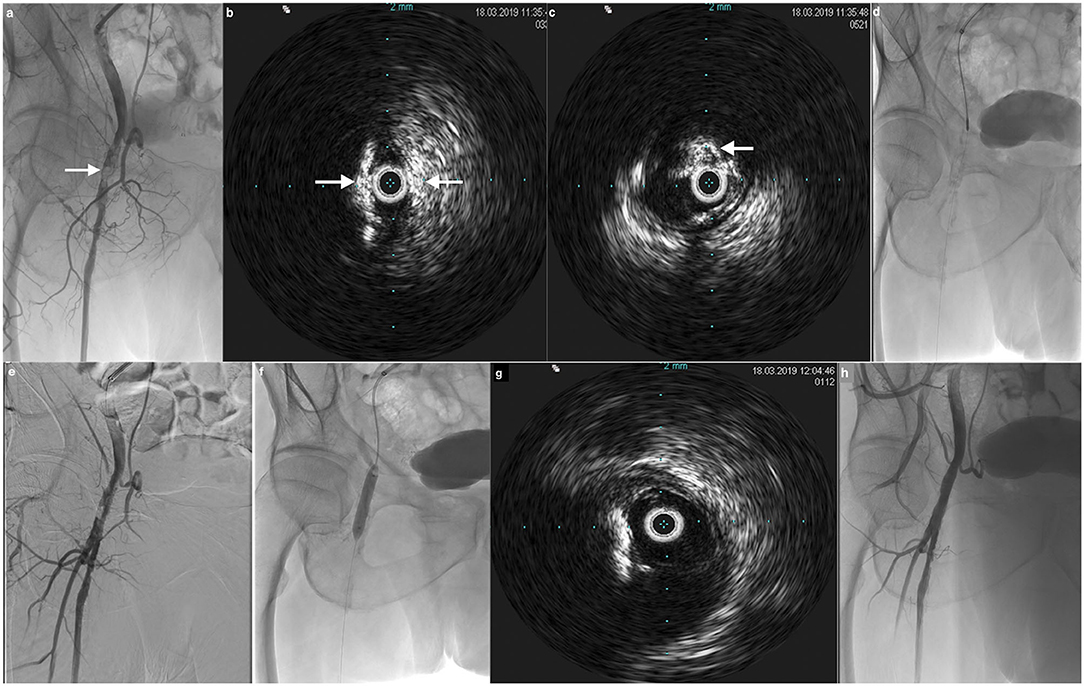
Figure 3. 72-year old patient with right claudication. (a) Angiogram by crossover shows important calcified stenotic lesions of the right common femoral artery (arrow). (b,c) IVUS confirms the large circonferential hyperechogenic calcifications (arrows). (d) Use of JetStream atherectomy device for debulking. (e) Result after debulking shows lumen gain. (f) Conventional PTA and drug-coated balloon angioplasty. (g) IVUS control confirms the excellent debulking result with removal of calcifications and lumen gain. No additional stenting was needed. (h) Final result at angiogram demonstrates normal lumen size with no residual stenosis.
IVUS and True-Lumen Re-entry
Five retrospective studies, that evaluated IVUS for true-lumen reentry during subintimal PTA, showed very promising technical success rates ranging from 97 to 100%, whatever the catheter used (9, 25–29). Follow-up demonstrated a clinical success rate of 100% in 3 of the studies (25, 27, 29). The goal of one of these 5 studies was to perform comparisons between true lumen re-entry with and without the use of IVUS (26). Technical success was higher in the IVUS group (97 vs. 81%). The real time imaging of IVUS enables subintimal tract creation and directed needle deployment. IVUS scanner adds information not only about the intima and the lumen, but also confirms vessel patency at the point of the needle due to its color flow capabilities. The accuracy and controlled reentry offered by IVUS catheters reduce the risk of dissection-related complications, such as perforations caused by guidewire or catheter malpositions, even for thick calcified plaques (6, 7, 25). Another advantage of IVUS reentry catheters is that they shorten intervention time between 3 and 10 min. This limits the radiation exposure for the patient and operator (27, 29). In addition, the use of these devices decreases the risk of periprocedural complications that are related to prolonged operation times. As a result, in the 5 studies that investigated the use of IVUS reentry devices, no significant periprocedural or post-procedural complications were reported.
IVUS and Contrast Exposure
In 2 studies, it was proposed that with IVUS guidance contrast injection could be reduced or completely avoided (9, 26). Kawasaki et al. performed comparisons between IVUS and non IVUS guided true-lumen re-entry and demonstrated a significantly lower contrast exposure in favor of the IVUS group (104 vs. 201 mL, P < 0.001) (26). Another benefit of IVUS is that injection of contrast medium can be reduced or even completely avoided, as it has been reported in many studies (9, 26, 30, 31). Contrast induced acute kidney injury may be associated with higher morbidity and mortality rates as well as prolonged hospitalization. Therefore, this contrast-free alternative seems to be very promising for high risk patients suffering from diabetes, chronic renal insufficiency or contrast allergies, but also for all other patients due to the contrast overload reduction.
IVUS and Cost-Effectiveness
Two studies suggested that IVUS leads to additional cost to a revascularization procedure, with an increase that ranges from $1,080 to $1,333 (8, 14). According to Panaich et al. the IVUS derived increase of procedural costs was non-significant ($1,333, 95%CI,–$167 to + $2,833, P = 0.082) (14).
Buckley et al. reported that the medical costs were higher with IVUS guidance vs. DSA alone during PAD interventions (8). According to Schiele et al. acute procedural costs can be 18% higher with IVUS compared to non IVUS use (32). However, the cumulative costs are only slightly higher ranging from 1 to 7.8% due to the lower number of re-interventions and the decreased length of hospital stay in the IVUS guided endovascular procedures, meaning that the use of IVUS can be cost effective when properly used.
Future Perspectives
Although retrospective studies report a high rate of patency and freedom from revascularization with the utilization of IVUS imaging as an adjunctive tool to standard DSA, data from randomized controlled trials are still lacking. Evaluation and improvement of stent placement in PAD and the identification of post-procedural dissections that are missed by DSA alone can be obtained with the use of IVUS (7, 33). Despite a significant improvement in immediate technical outcomes and short-term results, wide adoption of this technology prior to peripheral interventions requires universal algorithm to optimize long-term outcomes. In addition, IVUS enables to diminish the amount of contrast medium used and its associated complications in peripheral endovascular interventions (26, 31). Last, despite cost-effective analyses in favor of the use of IVUS by decreasing the rate of complications in lower limb interventions, further studies are needed to definitely demonstrate the cost-effectiveness of the systematic and routine use of IVUS during endovascular interventions. Indeed, the use of disposable catheter-delivered transducers, the expensive equipment and an experienced technician who may be necessary for the operation of IVUS system are the main factors responsible for the increased procedural costs of this technique (6, 8). However, there is no doubt that IVUS is likely to be more often used in the near future for modern endovascular therapy in PAD.
Summary and Conclusions
IVUS has improved fast from a purely diagnostic imaging modality to a very useful adjunctive tool to DSA in the evaluation of the vasculature. It may provide a more precise visualization to what happens into the blood vessel and play an increasing role in peripheral arterial occlusive procedures. Miniaturization of the components has allowed the system and catheter to be as small-profile as new technologies, and has made IVUS a mandatory adjunct tool for the best outcomes. As endovascular procedures become increasingly complex, technical, and clinical successes will be related to the degree of accuracy of the guidance device used during the procedure. IVUS is relatively easy to use and can be widely available despite cost but requires some expertise in image interpretation which might limit its systematic use. However, IVUS is an important element of current and future vascular interventions and should be part of training programs and the routine practice of all vascular specialists.
Author Contributions
RL, NF, CG, LF, FL, MM, and OC the conception and design of the study, or acquisition of data, or analysis and interpretation of data, drafting the article or revising it critically for important intellectual content, and final approval of the version to be submitted. Each author has participated sufficiently in this article to take public responsibility for its content.
Conflict of Interest
The authors declare that the research was conducted in the absence of any commercial or financial relationships that could be construed as a potential conflict of interest.
References
1. Diethrich EB, Irshad K, Reid DB. Virtual histology and color flow intravascular ultrasound in peripheral interventions. Semin Vasc Surg. (2006) 19:155–62. doi: 10.1053/j.semvascsurg.2006.06.001
2. Duran C, Bismuth J. Advanced imaging in limb salvage. Methodist Debakey Cardiovasc J. (2012) 8:28–32. doi: 10.14797/mdcj-8-4-28
3. Nakatani S, Proniewska K, Pociask E, Paoletti G, de Winter S, Muramatsu T, et al. How clinically effective is intravascular ultrasound in interventional cardiology? Present and future perspectives. Exp Rev Med Devices. (2013) 10:735–49. doi: 10.1586/17434440.2013.841353
4. Arthurs ZM, Bishop PD, Feiten LE, Eagleton MJ, Clair DG, Kashyap VS. Evaluation of peripheral atherosclerosis: a comparative analysis of angiography and intravascular ultrasound. J Vasc Surg. (2010) 51:933–9. doi: 10.1016/j.jvs.2009.11.034
5. Lee JT, Fang TD, White RA. Applications of intravascular ultrasound in the treatment of peripheral occlusive disease. Semin Vasc Surg. (2006) 19:139–44. doi: 10.1053/j.semvascsurg.2006.06.004
6. Shammas NW, Radaideh Q, Shammas WJ, Daher GE, Rachwan RJ, Radaideh Y. The role of precise imaging with intravascular ultrasound in coronary and peripheral interventions. Vasc Health Risk Manag. (2019) 15:283–90. doi: 10.2147/VHRM.S210928
7. Makris GC, Chrysafi P, Little M, Patel R, Bratby M, Wigham A, et al. The role of intravascular ultrasound in lower limb revascularization in patients with peripheral arterial disease. Int Angiol. (2017) 36:505–16. doi: 10.23736/S0392-9590.17.03866-4
8. Buckley CJ, Arko FR, Lee S, Mettauer M, Little D, Atkins M, et al. Intravascular ultrasound scanning improves long-term patency of iliac lesions treated with balloon angioplasty and primary stenting. J Vasc Surg. (2002) 35:316–23. doi: 10.1067/mva.2002.119755
9. Kawasaki D, Fujii K, Fukunaga M, Fujii N, Masutani M, Kawabata ML, et al. Preprocedural evaluation and endovascular treatment of iliofemoral artery disease without contrast media for patients with pre-existing renal insufficiency. Circ J. (2011) 75:179–84. doi: 10.1253/circj.CJ-10-0438
10. Araki M, Hirano K, Nakano M, Ito Y, Ishimori H, Yamawaki M, et al. Two-year outcome of the self-expandable stent for chronic total occlusion of the iliac artery. Cardiovasc Interv Ther. (2014) 29:40–6. doi: 10.1007/s12928-013-0210-z
11. Iida O, Takahara M, Soga Y, Suzuki K, Hirano K, Kawasaki D, et al. Efficacy of intravascular ultrasound in femoropopliteal stenting for peripheral artery disease with TASC II class A to C lesions. J Endovasc Ther. (2014) 21:485–92. doi: 10.1583/14-4721R.1
12. Baker AC, Humphries MD, Noll RE Jr, Salhan N, Armstrong EJ, Williams TK, et al. Technical and early outcomes using ultrasound-guided reentry for chronic total occlusions. Ann Vasc Surg. (2015) 29:55–62. doi: 10.1016/j.avsg.2014.10.011
13. Kumakura H, Kanai H, Araki Y, Hojo Y, Iwasaki T, Ichikawa S. 15-year patency and life expectancy after primary stenting guided by intravascular ultrasound for iliac artery lesions in peripheral arterial disease. JACC Cardiovasc Interv. (2015) 8:1893–901. doi: 10.1016/j.jcin.2015.08.020
14. Panaich SS, Arora S, Patel N, Patel NJ, Savani C, Patel A, et al. Intravascular ultrasound in lower extremity peripheral vascular interventions: variation in utilization and impact on in-hospital outcomes. From the nationwide inpatient sample (2006-2011). J Endovasc Ther. (2016) 23:65–75. doi: 10.1177/1526602815620780
15. Yin D, Maehara A, Shimshak TM, Ricotta JJ 2nd, Ramaiah V, Foster MT 3rd, et al. Intravascular ultrasound validation of contemporary angiographic scores evaluating the severity of calcification in peripheral arteries. J Endovasc Ther. (2017) 24:478–87. doi: 10.1177/1526602817708796
16. Krishnan P, Tarricone A, K-Raman P, Majeed F, Kapur V, Gujja K, et al. Intravascular ultrasound guided directional atherectomy versus directional atherectomy guided by angiography for the treatment of femoropopliteal in-stent restenosis. Ther Adv Cardiovasc Dis. (2018) 12:17–22. doi: 10.1177/1753944717745509
17. Shammas NW, Torey JT, Shammas WJ, Jones-Miller S, Shammas GA. Intravascular ultrasound assessment and correlation with angiographic findings demonstrating femoropopliteal arterial dissections post atherectomy: results from the iDissection study. J Invasive Cardiol. (2018) 30:240–4.
18. Fujihara M, Kozuki A, Tsubakimoto Y, Takahara M, Shintani Y, Fukunaga M, et al. Lumen gain after endovascular therapy in calcified superficial femoral artery occlusive disease assessed by intravascular ultrasound (CODE study). J Endovasc Ther. (2019) 26:322–30. doi: 10.1177/1526602819836095
19. Miki K, Fujii K, Tanaka T, Yanaka K, Yoshihara N, Nishimura M, et al. Impact of IVUS-derived vessel size on midterm outcomes after stent implantation in femoropopliteal lesions. J Endovasc Ther. (2020) 27:77–85. doi: 10.1177/1526602819896293
20. Nissen SE, Yock P. Intravascular ultrasound. Novel pathophysiological insights and current clinical applications. Circulation. (2001) 103:604–16. doi: 10.1161/01.CIR.103.4.604
21. Kashyap VS, Pavkov ML, Bishop PD, Nassoiy SP, Eagleton MJ, Clair DG, et al. Angiography underestimates peripheral atherosclerosis: lumenography revisited. J Endovasc Ther. (2008) 15:117–25. doi: 10.1583/07-2249R.1
22. Pliagas G, Saab F, Stavroulakis K, Bisdas T, Finton S, Heaney C, et al. Intravascular ultrasound imaging versus digital subtraction angiography in patients with peripheral vascular disease. J Invasive Cardiol. (2020) 32:99–103.
23. Cioppa A, Stabile E, Popusoi G, Salemme L, Cota L, Pucciarelli A, et al. Combined treatment of heavy calcified femoro-popliteal lesions using directional atherectomy and a paclitaxel coated balloon: One-year single centre clinical results. Cardiovasc Revasc Med. (2012) 13:219–23. doi: 10.1016/j.carrev.2012.04.007
24. Kuku KO, Garcia-Garcia HM, Koifman E, Kajita AH, Desale S, Azizi V, et al. Intravascular ultrasound assessment of the effect of laser energy on the arterial wall during the treatment of femoro-popliteal lesions: a CliRpath excimer laser system to enlarge lumen openings (CELLO) registry study. Int J Cardiovasc Imaging. (2018) 34:345–52. doi: 10.1007/s10554-017-1248-2
25. Krishnamurthy VN, Eliason JL, Henke PK, Rectenwald JE. Intravascular ultrasound-guided true lumen reentry device for recanalization of unilateral chronic total occlusion of iliac arteries: technique and follow-up. Ann Vasc Surg. (2010) 24:487–97. doi: 10.1016/j.avsg.2009.12.002
26. Kawasaki D, Tsujino T, Fujii K, Masutani M, Ohyanagi M, Masuyama T. Novel use of ultrasound guidance for recanalization of iliac, femoral, and popliteal arteries. Catheter Cardiovasc Interv. (2008) 71:727–33. doi: 10.1002/ccd.21503
27. Jacobs DL, Motaganahalli RL, Cox DE, Wittgen CM, Peterson GJ. True lumen re-entry devices facilitate subintimal angioplasty and stenting of total chronic occlusions: initial report. J Vasc Surg. (2006) 43:1291–6. doi: 10.1016/j.jvs.2006.02.051
28. Saket RR, Razavi MK, Padidar A, Kee ST, Sze DY, Dake MD. Novel intravascular ultrasound-guided method to create transintimal arterial communications: initial experience in peripheral occlusive disease and aortic dissection. J Endovasc Ther. (2004) 11:274–80. doi: 10.1583/03-1133.1
29. Saketkhoo RR, Razavi MK, Padidar A, Kee ST, Sze DY, Dake MD. Percutaneous bypass: subintimal recanalization of peripheral occlusive disease with IVUS guided luminal re-entry. Tech Vasc Interv Radiol. (2004) 7:23–7. doi: 10.1053/j.tvir.2004.01.006
30. Mariani J Jr, Guedes C, Soares P, Zalc S, Campos CM, Lopes AC, et al. Intravascular ultrasound guidance to minimize the use of iodine contrast in percutaneous coronary intervention: the MOZART (Minimizing cOntrast utiliZAtion with IVUS guidance in coRonary angioplasTy) randomized controlled trial. JACC Cardiovasc Interv. (2014) 7:1287–93. doi: 10.1016/j.jcin.2014.05.024
31. Ali ZA, Karimi Galougahi K, Nazif T, Maehara A, Hardy MA, Cohen DJ, et al. Imaging- and physiology-guided percutaneous coronary intervention without contrast administration in advanced renal failure: a feasibility, safety, and outcome study. Eur Heart J. (2016) 37:3090–5. doi: 10.1093/eurheartj/ehw078
32. Schiele F, Meneveau N, Seronde MF, Caulfield F, Pisa B, Arveux P, et al. Medical costs of intravascular ultrasound optimization of stent deployment. Results of the multicenter randomized ‘REStenosis after Intravascular ultrasound STenting’ (RESIST) study. Int J Cardiovasc Intervent. (2000) 3:207–13. doi: 10.1080/14628840050515957
Keywords: intravascular ultrasound (IVUS), peripheral arterial disease, percutaneous transluminal angioplasty (PTA), stent placement, atherectomy
Citation: Loffroy R, Falvo N, Galland C, Fréchier L, Ledan F, Midulla M and Chevallier O (2020) Intravascular Ultrasound in the Endovascular Treatment of Patients With Peripheral Arterial Disease: Current Role and Future Perspectives. Front. Cardiovasc. Med. 7:551861. doi: 10.3389/fcvm.2020.551861
Received: 14 April 2020; Accepted: 16 November 2020;
Published: 02 December 2020.
Edited by:
Matteo Cameli, University of Siena, ItalyReviewed by:
Tomas Lapinskas, Lithuanian University of Health Sciences, LithuaniaErhan Tenekecioglu, University of Health Sciences, Turkey
Copyright © 2020 Loffroy, Falvo, Galland, Fréchier, Ledan, Midulla and Chevallier. This is an open-access article distributed under the terms of the Creative Commons Attribution License (CC BY). The use, distribution or reproduction in other forums is permitted, provided the original author(s) and the copyright owner(s) are credited and that the original publication in this journal is cited, in accordance with accepted academic practice. No use, distribution or reproduction is permitted which does not comply with these terms.
*Correspondence: Romaric Loffroy, romaric.loffroy@chu-dijon.fr