The Role of Four-Dimensional Automatic Right Ventricular Quantification Technology to Determine RV Function and Hemodynamics in Patients With Pulmonary Hypertension Compared With Right Heart Catheterization
- 1State Key Laboratory of Cardiovascular Disease, Department of Echocardiography, National Center for Cardiovascular Diseases, Fuwai Hospital, Chinese Academy of Medical Sciences and Peking Union Medical College, Beijing, China
- 2State Key Laboratory of Cardiovascular Disease, Department of Cardiology, Pulmonary Vascular Disease Center, National Center for Cardiovascular Diseases, Fuwai Hospital, Chinese Academy of Medical Sciences and Peking Union Medical College, Beijing, China
- 3Department of Ultrasound, Meishan People's Hospital, Meishan, China
- 4Heart and Vascular Institute, Stamford Hospital, Stamford, CT, United States
- 5General Electric Healthcare, Beijing, China
- 6Department of Cardiology, Capital Institute of Pediatrics, Beijing, China
Background: Four-dimensional automatic right ventricular quantification technology (4D auto-RVQ) is a new method that can simultaneously measure right ventricular (RV) structure and strain. The role of 4D auto-RVQ in determining RV function and hemodynamics is not clear. The role of 4D auto-RVQ in determining RV function and hemodynamics is not clear. We assessed the 4D auto-RVQ to measure right heart structure, function, and hemodynamics in patients with pulmonary hypertension (PHTN) correlated with right heart catheterization (RHC).
Methods: We enrolled a prospective cohort of 103 patients with PHTN and 25 healthy controls between September 2017 and December 2018. All patients with PHTN underwent echocardiography and RHC. Patients were included if they underwent two-dimensional (2D) and 4D auto-RVQ echocardiographic sequences on the same day as RHC. We analyzed RV functional indices using 2D and 4D auto-RVQ analyses. We divided patients with PHTN into three groups according to echocardiographic image quality as follows: high (n = 24), average (n = 48), and poor (n = 4). Hemodynamic parameters were measured using RHC, including mean right atrial pressure, mean pulmonary arterial pressure, RV cardiac index (RV-CI), and pulmonary vascular resistance.
Results: There were significant differences in most 2D and 4D auto-RVQ parameters between patients with PHTN and healthy controls. Interobserver variability showed significant agreement with 4D auto-RVQ for most measurements except for 4D end-diastolic volume. Indices measured by auto 4D-RVQ in the high-quality image group had a good correlation with RHC but not in the average- and poor-quality image group. Mid-RV diameter showed the best predictive power for the right RV-CI [area under the curve (AUC) 0.935; 95% confidence interval (CI), 0.714–0.997; p < 0.001]. RV end-systolic volume >121.50 mL had a 71.43% sensitivity and a 100% specificity to predict right RV-CI (AUC, 0.890; 95% CI, 0.654–0.986; p < 0.001).
Conclusions: 4D auto-RVQ may be used to estimate RV function and some hemodynamic changes compared with RHC in PHTN patients with high image quality. Furthermore, a large sample of the study is needed to evaluate RV function by 4D auto-RVQ in PHTN patients with average image quality.
Introduction
Echocardiography is the most commonly used imaging technique for the study of right ventricular (RV) morphology, volume, function, and tissue characterization (1, 2). The accuracy of 2DE is inferior to cardiac magnetic resonance imaging (3). Because of the complex RV geometry, 2DE cannot capture RV inflow and outflow in the same image acquisition. Real-time three-dimensional echocardiography (3DE), also named four-dimensional echocardiography (4DE), is a more accurate and quicker method to assess RV volume and function than 2DE, but in some cases, this approach still poses some technical difficulties (4).
The right ventricle has a unique crescent shape and complex muscle moment, which influences the accurate evaluation of RV function. In patients with pulmonary hypertension (PHTN), the right heart chambers are significantly enlarged, and the scanning width of the echocardiography is insufficient to cover the entire right ventricle. The walls of the right ventricle can be difficult to identify. RV strain has been extensively used to evaluate the myocardial function and has predictive potential in patients with PHTN. RV strain is also recommended in European guidelines as part of the echocardiographic assessment of the right heart in adults (5, 6).
Right heart catheterization (RHC) is recommended to confirm the diagnosis of pulmonary arterial hypertension (PAH) and to support treatment decisions (7). RV hemodynamic deterioration, which can be measured by RHC, such as changes in mean pulmonary arterial pressure (PAP), pulmonary vascular resistance (PVR), and decrease in RV cardiac index (CI), is associated with poor clinical outcomes in patients with PHTN (8). PHTN is a progressive and life-threatening disease leading to RV pressure overload, dysfunction, and ultimately death. RHC can reliably measure indices of right heart function, such as cardiac output (CO) and RV-CI.
Recently, 4D automatic RV quantification (4D auto-RVQ) has emerged as a new technology for comprehensive RV assessment. This technique can simultaneously measure RV volume, tricuspid annular plane systolic exertion (TAPSE), RV diameter, 4D ejection fraction (4D-EF), and longitudinal strain of the RV free wall and septum (9). In our study, we attempted to use this technology to estimate right heart function and important hemodynamic indices, as well as to obtain other indirect information on right heart structure and function in patients with PHTN.
Materials and Methods
Study Population
Our study was a prospective cross-sectional project by design. Adult inpatients with PHTN and healthy volunteers were enrolled from September 2017 to December 2018. All patients with PHTN underwent echocardiography and RHC. Patients with PHTN were included if they had a diagnosis of idiopathic PAH, chronic thromboembolic PAH, connective tissue disease PAH, or residual PAH after surgery for congenital heart disease by RHC with a mean PAP of >25 mm Hg (7). Patients were included if they underwent 2D and 4D auto-RVQ on the same day. Patients were excluded if they were <18 years of age, if echocardiographic images were inadequate for tracing. The exclusion criteria included congenital heart disease before the operation, serious valvular heart disease, significant coronary heart disease, atrial fibrillation, acute heart failure, renal or hepatic failure, and chronic obstructive pulmonary disease. Healthy adult volunteers served as healthy controls. These volunteers had no cardiac defects or family history of cardiac disease.
Our primary endpoint is the role of 4D auto-RVQ to determine RV function and hemodynamics in PHTN patients compared with RHC data. The secondary endpoint is the accuracy and characteristic of 4D auto-RVQ indices in PHTN patients compared to normal people.
Written informed consent was obtained from all participants or their legal representatives. The present study was approved by the ethics committee of Fuwai Hospital (no. 2018-1063). All procedures were performed in accordance with the 1964 Helsinki Declaration and its later amendments.
2DE Acquisition and Analysis
All patients underwent standard transthoracic echocardiography using a GE Vivid E9 (GE Healthcare, Milwaukee, WI, USA) with a 3.5-MHz phased-array transducer. Consecutive cardiac cycles were recorded during breath-holding with stable electrocardiography tracing. All patients underwent standard 2DE and Doppler echocardiography examinations with detailed evaluation of right heart function. A comprehensive evaluation of the right ventricle by 2DE obtained six standardized views, including the parasternal long-axis, RV inflow, parasternal short-axis, apical four-chamber, and subcostal views.
Offline analysis was then performed on digitally stored images. Indices of 2DE included TAPSE and RV fractional area change. Right heart size was quantified as RV end-diastolic area at the end of the electrocardiogram (ECG) T-wave. RV chamber size was assessed at the apex of the ECG R-wave in the apical four-chamber view with a focus on the right ventricle. We measured pulmonary systolic pressure by Doppler tricuspid regurgitation peak velocity plus estimated right atrial pressure.
4D Auto-RVQ Acquisition and Analysis
Digital data were analyzed offline (EchoPAC 7 Workstation version 201, GE Healthcare; TomTec 4D RV Function 2.0). Measurements using 4D auto-RVQ were acquired using the 4-V matrix-array transducer on the cardiac ultrasound GE system. Images were acquired using single beats with frame rates of ≥12 FPS. Apical RV-focused four-chamber views were acquired with patients in the lateral decubitus position. The transducer position was modified for optimal simultaneous visualization of the tricuspid valve, cardiac apex, and RV outflow tract. We visualized left ventricular apex, mid–mitral valve, aortic annulus, RV apex, mid–tricuspid valve, and RV diameter in the short-axis view (Figure 1) and performed an automatic calculation to determine 4D end-diastolic volume (4D-EDV), 4D end-systolic volume (4D-ESV), 4D stroke volume (4D-SV), and 4D-EF. The longitudinal strain of RV septum and free wall and TAPSE, RV diameter (middle/basal/longitudinal RV diameter). All the measurements were performed by trained technicians blinded to clinical data, according to the guidelines of the American Society of Echocardiography (10).
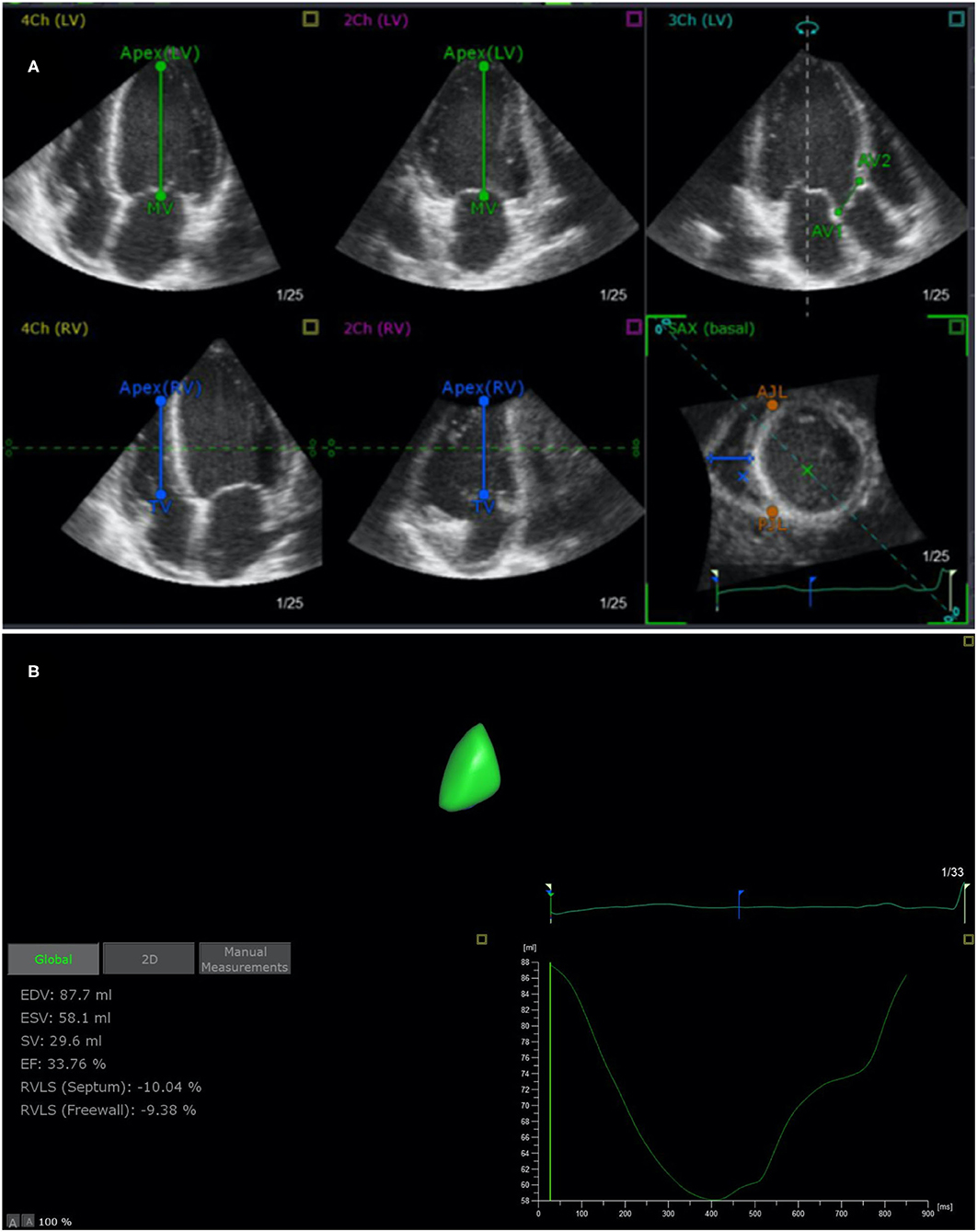
Figure 1. End-systolic verification and editing of endocardial RV borders using TomTec 4D RV Function 2.0 (A) and 4D auto-RVQ measurement (B). RV, right ventricular; EDV, end-diastolic volume; ESV, end-systolic volume; EF, ejection fraction; RVLS (septum), right ventricular longitudinal strain of the septum; RVLS (free wall), right ventricular longitudinal strain of the free wall; TAPSE, tricuspid annular plane systolic excursion.
Right Heart Catheterization
Hemodynamic parameters, including mean right atrial pressure, mean PAP, and mixed venous oxygen saturation, were recorded by RHC. CO was measured using the thermodilution method. RV-CI was calculated as CO ÷ body surface area. PVR was calculated as (mean PAP – PAWP) ÷ CO [PAWP (pulmonary artery wedge pressure)]. CI <2.5 L/min/m2 was defined as reduced right heart function (7). The interval between echocardiography and RHC was <24 h in 76 of 103 participants. For the other 27 patients, RHC was performed >24 h after echocardiography; therefore, their hemodynamic indices were not included in the analysis.
Statistical Analysis
Analyses were performed using SPSS version 20.0 (SPSS, Inc., Chicago, IL, USA) and MedCalc 19.0.5. Continuous data are presented as mean ± standard deviation. Categorical data are presented as absolute numbers or percentages. Differences between groups were analyzed using the χ2 test. Pearson correlation analysis was performed to evaluate the relationship between 4D auto-RVQ and strain, as well as those parameters measured using the reference method. Interobserver agreement for qualitative analysis score using 4D auto-RVQ manual and software assessments was calculated using the intraclass correlation coefficient. Receiver operating characteristic (ROC) curves were used to investigate and compare the predictive ability of 4D auto-RVQ parameters to evaluate right heart function. A p-value of <0.05 was considered statistically significant.
Results
There were 103 adult patients with PHTN and 25 healthy controls from September 2017 to December 2018. We excluded 27 patients on whom RHC was not performed on the same day as echocardiography. Finally, 76 patients diagnosed with PHTN by RHC were recruited. We divided patients with PHTN (n = 76) into three groups according to echocardiographic image quality as follows: high image quality (n = 24), average image quality (n = 48), and poor image quality (n = 4). Clinical and conventional echocardiographic and 4D auto-RVQ characteristics of adult patients with PHTN are described in Table 1.
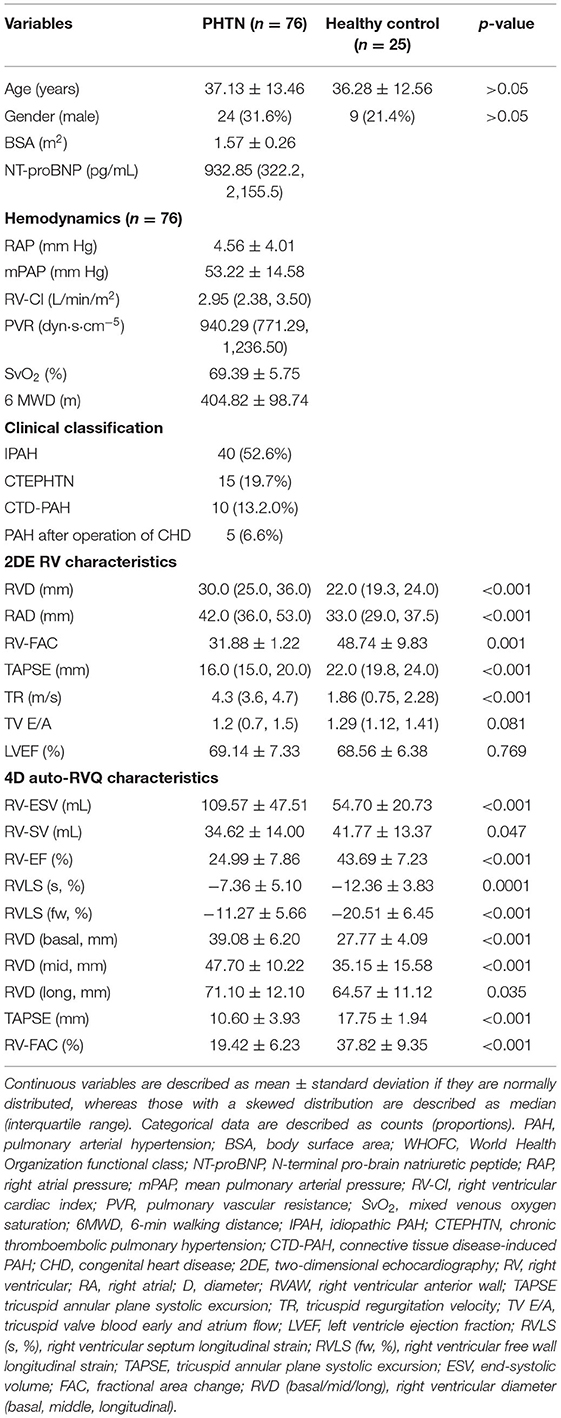
Table 1. Clinical, 2DE, and 4D auto-RVQ characteristics of 76 patients with pulmonary arterial hypertension.
There were no significant differences in age and sex between the PHTN and control groups. Patients with PHTN were predominantly in World Health Organization functional class II or III, with a markedly decreased 6-min walking distance, increased mean PAP, decreased CO, and increased PVR.
The intraclass correlation coefficient is listed in Table 2. Interobserver variability showed significant agreement with a 4D auto-RVQ method for most of the measurements except 4D-EDV.
We performed a correlation analysis of the 4D auto-RVQ indices and RHC indices in all PHTN cases (n = 76). Table 3 shows that there was no significant correlation between the RHC and most of the 4D indices in all PHTN patients.
In 24 patients with high image quality, 4D auto-RVQ indices had a very good correlation with RV-CI, and 4D-ESV had a good correlation with all important RHC indices (Table 4).
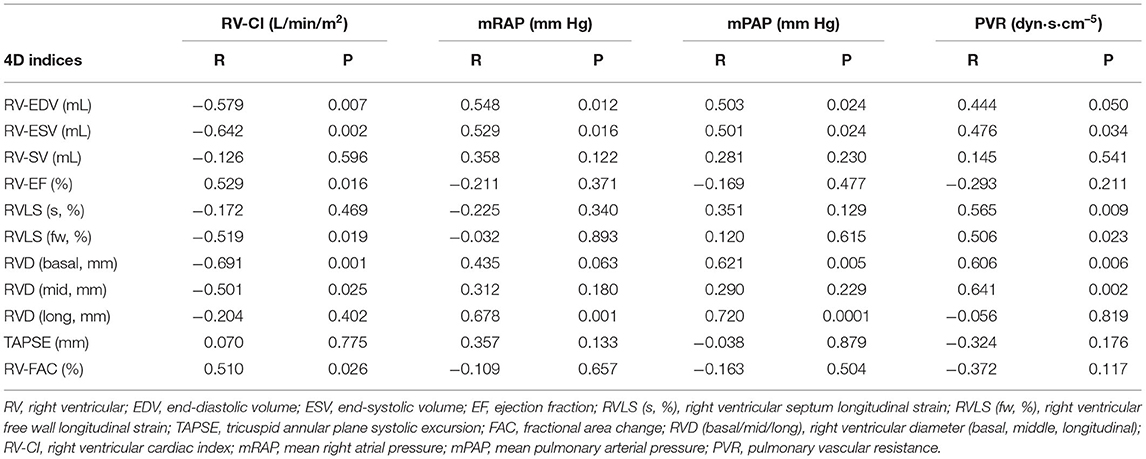
Table 4. Correlation between RHC and 4D auto-RVQ indices in the high-quality image PHTN group (n = 24).
When all PHTN patients with high, average, and poor image quality data combined, the 4D auto-RVQ indices were not clinically meaningful based on the ROC curve analysis (Table 5).
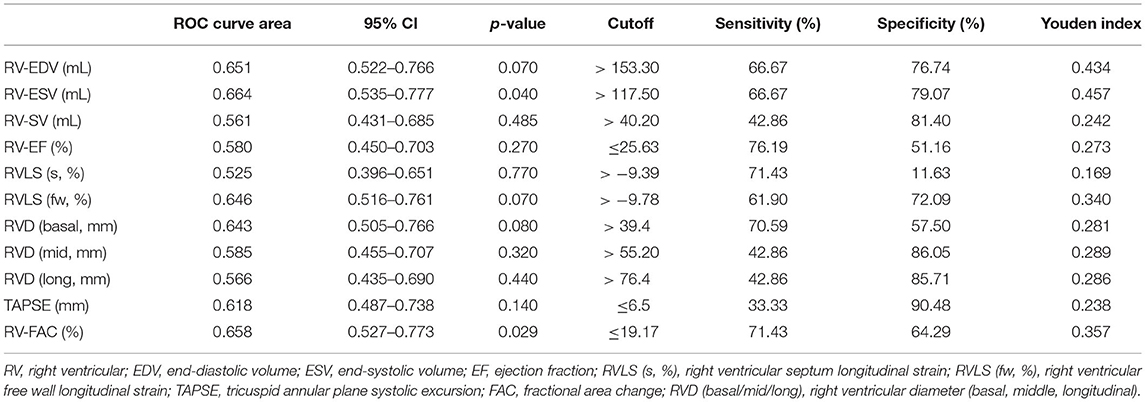
Table 5. All PHTN cases (n = 76) of 4D auto-RVQ areas of receiver operating characteristic (ROC) curves of various right ventricular function parameters.
ROC curves (Figure 2, Table 6) illustrated that all six 4D auto-RVQ parameters could predict right heart function in the subgroup of high-quality images, especially mid-RV diameter [area under the curve (AUC) 0.935; 95% confidence interval (CI), 0.714–0.997; p < 0.001]. A mid-RV diameter of >50.8 mm had an 85.7% sensitivity and a 90.9% specificity. A RV-ESV of >121.5 mL had a 71.4% sensitivity and a 100% specificity, as well as a Youden index of 0.714 to predict RV-CI (AUC, 0.890; 95% CI, 0.654–0.986; p < 0.001).
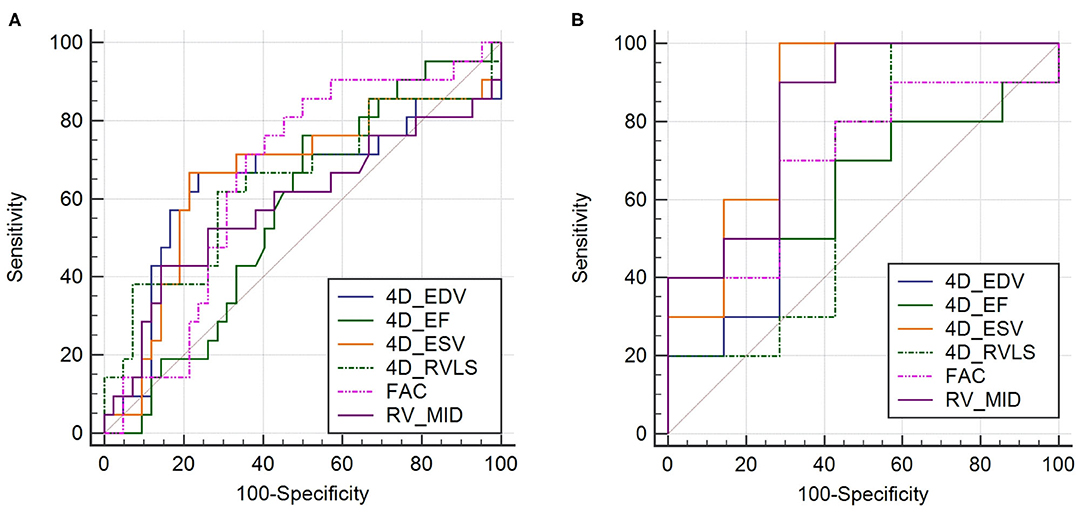
Figure 2. Receiver operating characteristic curves in all patient groups of PHTN (n = 76) of 4D auto-RVQ areas of (A) and in the high-quality image group of PHTN (n = 24) (B). 4D_EDV, four-dimensional end-diastolic volume; 4D_ESV, four-dimensional end-systolic volume; 4D_EF, four-dimensional ejection fraction; 4D-RVLS, right ventricular longitudinal strain of the free wall; FAC, fractional area change; RV_MID, mid–right ventricular diameter.
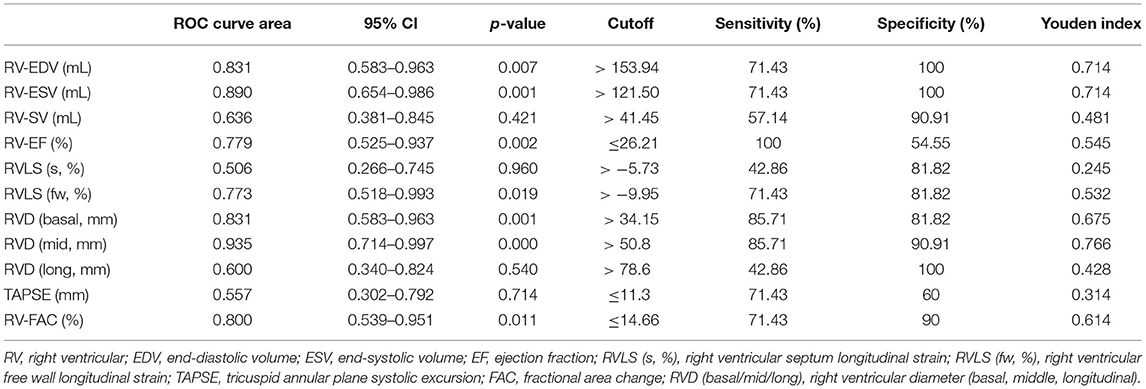
Table 6. High-quality image group of PHTNs (n = 24) of 4D auto-RVQ areas of receiver operating characteristic (ROC) curves of various right ventricular function parameters.
Discussion
Despite its clinical importance, the study of RV function is technically challenging, in patients with PHTN. It is usually very difficult to evaluate RV morphology and function using 2DE. Recently, 3DE and 4DE have been introduced and are reportedly feasible and clinically applicable for RV volumetric quantification in patients with acquired RV pressure or volume overload (11). At present, the advantages of 4DE could better reflect RV size and function. As a new technology, 4D auto-RVQ can combine the 3D echocardiographic morphological, functional, and strain characteristics for comprehensive RV evaluation. It has the potential to reflect changes in RV function and pulmonary artery hemodynamics (9, 12).
Our results showed that 4D auto-RVQ, like 3D echocardiography, can accurately detect RV volume measurements in both PHTN patients and healthy control with good interobserver reproducibility (13, 14). However, the intraclass correlation coefficient of 4D-EDV was suboptimal. This may be due to the large RV volume in patients with PHTN so the right ventricle could not be completely included in some cases. Furthermore, 4D auto-RVQ can possibly detect differences in RV diameter, volume, and function. RV volume and basal and middle diameters were significantly enlarged in PHTN, but the longitudinal diameter was not significantly changed. This may be due to lateral expansion of the RV in patients with PHTN. In terms of the strain indices measured by 4D auto-RVQ, the longitudinal strains of the septum and free wall were significantly lower in patients with PHTN compared with the control group (15, 16). Similar to our findings, some reports found that the longitudinal strain of the septum and free wall had the potential to independently predict intermediate- to high-risk features in PAH patients (17). Smith also showed that RV longitudinal strain of the free wall (hazard ratio, 7.63; 95% CI, 1.76–10.27; p < 0.001) was a significant determinant of all-cause mortality (18).
When analyzing the data from RHC and 4D auto-RVQ indices, we observed a good correlation between 4D quantification and RHC in patients with high-quality images, but not in the other two groups with suboptimal imaging quality. RV-ESV by 4D auto-RVQ tracked well with RV-CI, mean right atrial pressure, mean PAP, and PVR measurements with RHC. We propose that RV-ESV may be an effective indicator of right heart function and hemodynamic changes in patients with PHTN. RV function analysis needs to take into consideration of its afterload including PVR. Measurement of 4D-ESV, 4D-RV strain, and RV basal and middle diameters had a good correlation with PVR. In the high-quality image group, all six 4DE parameters predicted right heart function. The indices with the best predictive power were RV middle diameter and 4D-ESV. We did notice that some authors previously used RV-EF as an index compared with cardiac magnetic resonance imaging (19, 20).
In summary, 4D auto-RVQ is a possibly useful quantitative tool to measure RV function with validation by RHC and provide meaningful data reflecting RV function. It is important to obtain high-quality RV images and to include the entire RV within the scanning sector. Previous studies have shown that in patients with chronic PHTN, 3D and 3D speckle-tracking echocardiography parameters for global and regional RV dysfunction were better than conventional echocardiographic indices (21, 22). The software for 4D auto-RVQ generates functional parameters from 4D datasets, making it an easy tool to implement in clinical practice and reducing the amount of time spent using multiple software parameters to simultaneously analyze 4D datasets and speckle-tracking echocardiography.
RHC remains the gold standard for RV hemodynamic evaluation, but 4D auto-RVQ has the potential to be included in routine clinical applications because it provides a relatively quantitative evaluation method for RV function in PHTN patients if high-quality echocardiographic images were acquired.
Limitations
There are several limitations to our data and results. As a single-center study in a cardiac referral center, we might have had selection bias. Among 103 patients, 27 patients did not simultaneously undergo ultrasonography and RHC and were excluded from the final analysis. World Health Organization functional class IV patients were not enrolled in the study. Our data are mainly applicable to patients with high-quality echocardiographic images. Our sample size was relatively small for an ROC analysis. Finally, we collected only the RHC data of PHTN without the results of cardiac magnetic resonance imaging. Thus, it is difficult to evaluate changes in cardiac volume in real time.
Conclusion
In conclusion, 4D auto-RVQ is a new method to estimate RV function and hemodynamic changes compared with gold-standard RHC in patients with PHTN only if high-quality echocardiographic images were acquired.
Data Availability Statement
The raw data supporting the conclusions of this article will be made available by the authors, without undue reservation.
Ethics Statement
The studies involving human participants were reviewed and approved by the Ethics Committee of Fuwai Hospital (No. 2018-1063). Written informed consent to participate in this study was provided by the participants' legal guardian/next of kin.
Author Contributions
WW, BL, and MH drafted the manuscript. LN, YT, JL, JW, SY, and HL contributed to data collection and statistical analysis. HW, DH, CX, and ZZ provided supervision and revised the manuscript. CX contributed to the conception, design, and supervision of the study. All authors contributed to the article and approved the submitted version.
Funding
The present study was supported by a grant from Capital Health Development and Scientific Research Projects (Grant No. 2016-2-4036). Fund from Construction of Key Laboratory (Cultivation) of Chinese Academy of Medical Sciences (2019PT310025).
Conflict of Interest
The authors declare that the research was conducted in the absence of any commercial or financial relationships that could be construed as a potential conflict of interest.
Acknowledgments
We thank Emily Woodhouse, Ph.D., from Liwen Bianji, Edanz Editing China (www.liwenbianji.cn/ac), for editing the English text of a draft of this manuscript.
References
1. Dandel M, Hetzer R. Evaluation of the right ventricle by echocardiography: particularities and major challenges. Expert Rev Cardiovasc Ther. (2018) 16:259–75. doi: 10.1080/14779072.2018.1449646
2. Venkatachalam S, Wu G, Ahmad M. Echocardiographic assessment of the right ventricle in the current era: application in clinical practice. Echocardiography. (2017) 34:1930–47. doi: 10.1111/echo.13651
3. Gürdogan M, Ustabaşioglu FE, Kula O, Korkmaz S. Cardiac magnetic resonance imaging and transthoracic echocardiography: investigation of concordance between the two methods for measurement of the cardiac chamber. Medicina. (2019) 55:260. doi: 10.3390/medicina55060260
4. Kjaergaard J, Petersen CL, Kjaer A, Schaadt BK, Oh JK, Hassager C. Evaluation of right ventricular volume and function by 2D and 3D echocardiography compared to MRI. Eur J Echocardiogr. (2006) 7:430–8. doi: 10.1016/j.euje.2005.10.009
5. Lang RM, Badano LP, Mor-Avi V, Afilalo J, Armstrong A, Ernande L, et al. Recommendations for cardiac chamber quantification by echocardiography in adults: an update from the American Society of Echocardiography and the European Association of Cardiovascular Imaging. Eur Heart J Cardiovasc Imaging. (2015) 16:233–70. doi: 10.1093/ehjci/jev014
6. Zaidi A, Knight DS, Augustine DX, Harkness A, Oxborough D, Pearce K, et al. Echocardiographic assessment of the right heart in adults: a practical guideline from the British Society of Echocardiography. Echo Res Pract. (2020) 7:G19–41. doi: 10.1530/ERP-19-0051
7. Galiè N, Humbert M, Vachiery J-L, Gibbs S, Lang I, Torbicki A, et al. 2015 ESC/ERS Guidelines for the diagnosis and treatment of pulmonary hypertension: The Joint Task Force for the Diagnosis and Treatment of Pulmonary Hypertension of the European Society of Cardiology (ESC) and the European Respiratory Society (ERS): Endorsed by: Association for European Paediatric and Congenital Cardiology (AEPC), International Society for Heart and Lung Transplantation (ISHLT). Eur Respir J. (2015) 46:903–75. doi: 10.1183/13993003.01032-2015
8. Miller WL, Grill DE, Borlaug BA. Clinical features, hemodynamics, and outcomes of pulmonary hypertension due to chronic heart failure with reduced ejection fraction: pulmonary hypertension and heart failure. JACC Heart Fail. (2013) 1:290–9. doi: 10.1016/j.jchf.2013.05.001
9. Aubert R, Venner C, Huttin O, Haine D, Filippetti L, Guillaumot A, et al. Three-dimensional echocardiography for the assessment of right ventriculo-arterial coupling. J Am Soc Echocardiogr. (2018) 31:905–15. doi: 10.1016/j.echo.2018.04.013
10. Rudski LG, Lai WW, Afilalo J, Hua L, Handschumacher MD, Chandrasekaran K, et al. Guidelines for the echocardiographic assessment of the right heart in adults: a report from the American Society of Echocardiography endorsed by the European Association of Echocardiography, a registered branch of the European Society of Cardiology, and the Canadian Society of Echocardiography. J Am Soc Echocardiogr. (2010) 23:685–713. doi: 10.1016/j.echo.2010.05.010
11. Knight DS, Grasso AE, Quail MA, Muthurangu V, Taylor AM, Toumpanakis C, et al. Accuracy and reproducibility of right ventricular quantification in patients with pressure and volume overload using single-beat three-dimensional echocardiography. J Am Soc Echocardiogr. (2015) 28:363–74. doi: 10.1016/j.echo.2014.10.012
12. Zhang XM, Zhuang Q, Yang MH, Wang W, Zheng Y, Qiao ZQ, et al. [Value of four-dimensional echocardiography combined with speckle tracking technique on the assessment of right heart function and prognosis in patients with pulmonary arterial hypertension]. Zhonghua Xin Xue Guan Bing Za Zhi. (2018) 46:965–71. doi: 10.3760/cma.j.issn.0253-3758.2018.12.007
13. Nagata Y, Wu VC-C, Kado Y, Otani K, Lin F-C, Otsuji Y, et al. Prognostic value of right ventricular ejection fraction assessed by transthoracic 3D echocardiography. Circ Cardiovasc Imaging. (2017) 10:e005384. doi: 10.1161/CIRCIMAGING.116.005384
14. Moceri P, Duchateau N, Baudouy D, Schouver E-D, Leroy S, Squara F, et al. Three-dimensional right-ventricular regional deformation and survival in pulmonary hypertension. Eur Heart J Cardiovasc Imaging. (2018) 19:450–8. doi: 10.1093/ehjci/jex163
15. Jone P-N, Schäfer M, Pan Z, Bremen C, Ivy DD. 3D echocardiographic evaluation of right ventricular function and strain: a prognostic study in paediatric pulmonary hypertension. Eur Heart J Cardiovasc Imaging. (2018) 19:1026–33. doi: 10.1093/ehjci/jex205
16. Leong DP, Grover S, Molaee P, Chakrabarty A, Shirazi M, Cheng YH, et al. Nonvolumetric echocardiographic indices of right ventricular systolic function: validation with cardiovascular magnetic resonance and relationship with functional capacity. Echocardiography. (2012) 29:455–63. doi: 10.1111/j.1540-8175.2011.01594.x
17. Liu B-Y, Wu W-C, Zeng Q-X, Liu Z-H, Niu L-L, Tian Y, et al. The value of three-dimensional echocardiography in risk stratification in pulmonary arterial hypertension: a cross-sectional study. Int J Cardiovasc Imaging. (2020) 36:577–84. doi: 10.1007/s10554-019-01743-1
18. Smith BCF, Dobson G, Dawson D, Charalampopoulos A, Grapsa J, Nihoyannopoulos P. Three-dimensional speckle tracking of the right ventricle: toward optimal quantification of right ventricular dysfunction in pulmonary hypertension. J Am Coll Cardiol. (2014) 64:41–51. doi: 10.1016/j.jacc.2014.01.084
19. Kidawa M, Chizyński K, Zielińska M, Kasprzak JD, Krzeminska-Pakula M. Real-time 3D echocardiography and tissue Doppler echocardiography in the assessment of right ventricle systolic function in patients with right ventricular myocardial infarction. Eur Heart J Cardiovasc Imaging. (2013) 14:1002–9. doi: 10.1093/ehjci/jes321
20. Gopal AS, Chukwu EO, Iwuchukwu CJ, Katz AS, Toole RS, Schapiro W, et al. Normal values of right ventricular size and function by real-time 3-dimensional echocardiography: comparison with cardiac magnetic resonance imaging. J Am Soc Echocardiogr. (2007) 20:445–55. doi: 10.1016/j.echo.2006.10.027
21. Vitarelli A, Mangieri E, Terzano C, Gaudio C, Salsano F, Rosato E, et al. Three-dimensional echocardiography and 2D-3D speckle-tracking imaging in chronic pulmonary hypertension: diagnostic accuracy in detecting hemodynamic signs of right ventricular (RV) failure. J Am Heart Assoc. (2015) 4:e001584. doi: 10.1161/JAHA.114.001584
22. Lu KJ, Chen JXC, Profitis K, Kearney LG, DeSilva D, Smith G, et al. Right ventricular global longitudinal strain is an independent predictor of right ventricular function: a multimodality study of cardiac magnetic resonance imaging, real time three-dimensional echocardiography and speckle tracking echocardiography. Echocardiography. (2015) 32:966–74. doi: 10.1111/echo.12783
Keywords: pulmonary hypertension, 4D-echocardiography, strain, right heart catheterization, right heart function
Citation: Wu W, Liu B, Huang M, Hsi DH, Niu L, Tian Y, Lin J, Wang J, Yang S, Lu H, Xiong C, Zhu Z and Wang H (2021) The Role of Four-Dimensional Automatic Right Ventricular Quantification Technology to Determine RV Function and Hemodynamics in Patients With Pulmonary Hypertension Compared With Right Heart Catheterization. Front. Cardiovasc. Med. 8:628610. doi: 10.3389/fcvm.2021.628610
Received: 12 November 2020; Accepted: 10 June 2021;
Published: 14 July 2021.
Edited by:
Patrick W. Serruys, Imperial College London, United KingdomCopyright © 2021 Wu, Liu, Huang, Hsi, Niu, Tian, Lin, Wang, Yang, Lu, Xiong, Zhu and Wang. This is an open-access article distributed under the terms of the Creative Commons Attribution License (CC BY). The use, distribution or reproduction in other forums is permitted, provided the original author(s) and the copyright owner(s) are credited and that the original publication in this journal is cited, in accordance with accepted academic practice. No use, distribution or reproduction is permitted which does not comply with these terms.
*Correspondence: Hao Wang, amystamford@163.com; Zhenhui Zhu, dr.zhu@139.com; Changming Xiong, xiongcmfw@163.com
†These authors have contributed equally to this work and share first authorship