Roles of Ferroptosis in Cardiovascular Diseases
- 1The Second School of Clinical Medicine, Southern Medical University, Guangzhou, China
- 2Department of Cardiology, Hainan Hospital of Chinese PLA General Hosptial, Hainan Geriatric Disease Clinical Medical Research Center, Hainan Branch of China Geriatric Disease Clinical Research Center, Hainan, China
- 3Department of Cardiology, Second Medical Center, PLA General Hospital, Beijing, China
- 4Department of Cardiology and Laboratory of Heart Center, Zhujiang Hospital, Southern Medical University, Guangzhou, China
- 5Department of Cell Biology, National Translational Science Center for Molecular Medicine, Fourth Military Medical University, Xi'an, China
Ferroptosis is an iron-dependent regulated cell death characterized by lipid peroxidation and iron overload, which is different from other types of programmed cell death, including apoptosis, necroptosis, autophagy, and pyroptosis. Over the past years, emerging studies have shown a close relation between ferroptosis and various cardiovascular diseases such as atherosclerosis, acute myocardial infarction, ischemia/reperfusion injury, cardiomyopathy, and heart failure. Herein, we will review the contributions of ferroptosis to multiple cardiovascular diseases and the related targets. Further, we discuss the potential ferroptosis-targeting strategies for treating different cardiovascular diseases.
Introduction
Cardiovascular diseases include hypertension, atherosclerosis, acute myocardial infarction (AMI), arrhythmia, cardiomyopathy, valvular heart diseases, congenital cardiovascular diseases and heart failure (1), which are the leading causes of disability and death in the world (2). Cardiomyocyte death is a basic pathological process in the progression of cardiovascular diseases. Understanding the mechanism of cardiomyocyte death can provide support for protecting cardiac function.
Ferroptosis, which was proposed by Dixon et al. (3), is a non-apoptotic form of cell death. Ferroptosis is characterized by lipid peroxidation and iron overload. Its morphological features mainly involve mitochondrial changes encompassing mitochondria shrinkage, increased mitochondria membrane density, crista destruction, and outer membrane rupture, but not nucleus morphological changes. Ferroptosis is a new pattern of programmed cell death that differs from several other forms of regulated cell death in various aspects, including morphology, biochemistry, and immune status (Table 1).
Recently, several studies have found various significant factors of ferroptosis and revealed a range of complex regulatory mechanisms in the progression of ferroptosis involving iron metabolism, lipid metabolism, and amino acid metabolism (Figure 1). In the iron metabolism pathways, transferrin receptor 1 (TfR1) transport extracellular Fe3+ to the nucleus and convert it into Fe 2+, which is released from the nucleus through divalent metal transporter 1 (DMT1), triggering the Fenton reaction, activating lipoxygenases, and promoting the generation of lipid peroxides, resulting in ferroptosis (4, 5). Amino acid metabolism involves vital regulatory factors, including system (consisting of two subunits SLC3A2 and SLC7A11) (6, 7) and glutathione peroxidase 4 (GPX4). Inhibitors of system decrease the uptake of cystine and reduce cysteine and suppress glutathione (GSH) production, further inactivating GPX4 (8) and reducing the conversion of GSH to glutathione disulfide (GSSG) (9), which will result in lipid peroxidation and ferroptosis in amino acid metabolism. By activating acyl-CoA synthetase long-chain family member four (ACSL4) and lysophosphatidylcholine acyltransferase three (LPCAT3), polyunsaturated fatty acids (PuFAs) induce lipid peroxidation and promote ferroptosis (10).
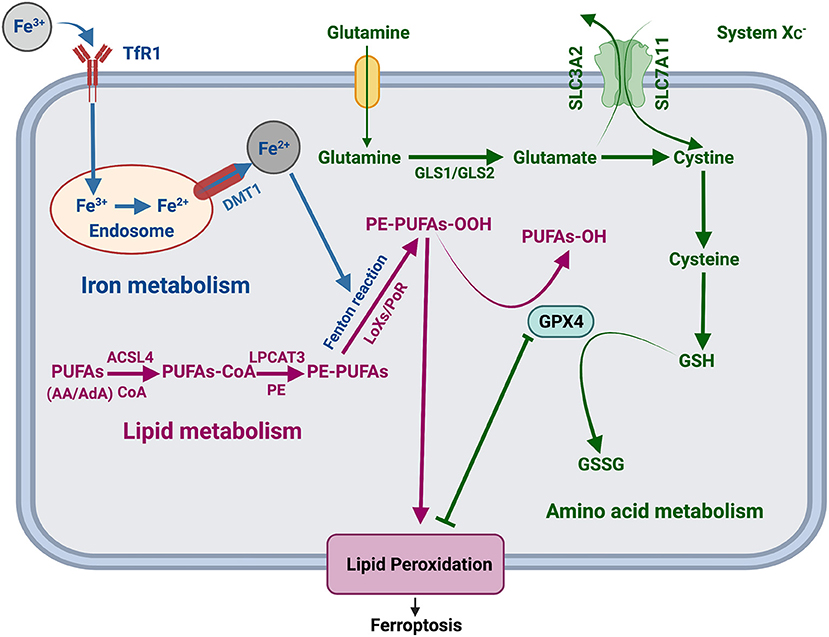
Figure 1. Regulatory mechanism of ferroptosis. TfR1, transferrin receptor 1; DMT1, divalent metal transporter 1; LOXs, lipoxygenases; POR, cytochrome P450 oxidoreductase; PUFAs, polyunsaturated fatty acids; AA, arachidonic acid; AdA, adrenal acid; CoA, coenzyme A; PE, phosphatidylethanolamine; ACSL4, acyl-CoA synthetase long-chain family member 4; LPCAT3, lysophosphatidylcholine acyltransferase 3; SLC7A11, solute carrier family 7 member 11; SLC3A2, solute carrier family 3 member 2; GSH, glutathione; GSSG, glutathione disulfide.
Over the years, researches on the link between ferroptosis and clinical diseases have been gradually improved, with cancer and neurodegenerative diseases being the focus (11–19). Recent studies have demonstrated ferroptosis participates in the genesis and development of cardiovascular diseases. We discuss the roles and potential mechanisms of ferroptosis in cardiovascular diseases in this article and hopefully provide an effective strategy for the treatment of cardiovascular diseases.
Ferroptosis and Cardiovascular Diseases
Ferroptosis and Cardiomyopathy
Cardiomyopathy is a group of myocardial diseases caused by heterogeneous factors, leading to myocardial and/or cardiac electrical dysfunction, with high mortality (20).
Doxorubicin (DOX), also known as adriamycin, is the second-generation anthracycline chemotherapy drug, a commonly used antitumor agent with fatal cardiotoxicity. Its most serious side effect is cardiomyopathy, called doxorubicin-induced cardiomyopathy (DIC) (21). Tadokoro et al. (22) found that mitochondria-dependent ferroptosis plays an essential role in DIC. DOX down-regulated GPX4 and caused excessive lipid peroxides production in mitochondria through the DOX-Fe2+ complex, resulting in mitochondria-dependent ferroptosis. GPX4 overexpression in mitochondria or iron chelates targeting Fe2+ can ameliorate doxorubicin-induced ferroptosis. Furthermore, this study showed that apoptosis is also a major form of doxorubicin-induced cardiomyocyte death. And two death forms are independent of each other. The combination of ferrostatin-1 (Fer-1) and zVAD-FMK to inhibit ferroptosis and apoptosis could completely prevent doxorubicin-induced cardiomyocyte death in rats. In addition, Fang et al. (23) showed that DOX significantly up-regulated heme oxygenase-1 (Hmox1) through NF-E2-related factor 2 (NRF2), induced local heme degradation, leading to the release of free iron, and further inducing ferroptosis in mouse myocardial tissue. Zinc protoporphyrin IX (ZnPP), a competitive inhibitor of Hmox1, reduced DOX-induced ferroptosis. These results suggest that Hmox1 plays an important role in doxorubicin-induced ferroptosis and cardiomyopathy. This study also found that ferroptosis inhibitor Fer-1 or dexrazoxane (DXZ) prevented lipid peroxidation and DIC by maintaining mitochondrial function. However, MitoTEMPO, a mitochondria-targeted antioxidant, can alleviate DIC by specifically clearing lipid peroxidation in mitochondria. These studies show that DOX-induced cardiotoxicity is closed with mitochondrial iron overload and subsequent ferroptosis. In 2021, He et al. (24) proved in vitro and in vivo that ferroptosis, autophagy, and apoptosis are related to DOX-induced cardiotoxicity. Epigallocatechin-3-gallate(EGCG)is a polyphenol compound in green tea and is also a natural antioxidant. EGCG up-regulated AMP-activated protein kinaseα2 (AMPKα2), activated adaptive autophagy, reduced iron deposition, inhibited reactive oxygen species (ROS) overproduction and rectified abnormal lipid metabolism, thereby reversing ferroptosis in DIC. Similarly, in a recent article, Sun et al. (25) demonstrated potent antioxidant melatonin inhibited mitochondrial lipid peroxidation and ameliorated doxorubicin-induced cardiac ferroptosis. In summary, we know that many forms of cell death are involved in DIC, among which ferroptosis is a pivotal one. Thus, targeting ferroptosis might be an effective treatment for DIC in cancer patients.
Diabetic cardiomyopathy (DCM) is defined as a disorder of cardiac structure and function in patients with diabetes in the absence of coronary artery disease, hypertension, valvular heart diseases, and other conventional cardiovascular risk factors (26). Excessive overproduction of ROS is regarded as an essential mechanism for the occurrence and development of diabetic cardiomyopathy (27), and the accumulation of lipid ROS induced ferroptosis (28). Therefore, ferroptosis is more likely to be involved in DCM. Some studies have supported that administration of ferroptotic inhibitors coenzyme Q10 and Vitamin E in diabetic animals might protect the myocardium by suppressing oxidative stress (29, 30). GPX4 is one of the crucial regulators of ferroptosis, and GPX4 deficiency induced lipid peroxidation and resulted in myocardial metabolic disturbance in high-fat, high-sucrose diet mice (31). Conversely, GPX4 overexpression could alleviate mitochondrial dysfunction and protect the hearts from diabetic damage (32). A recent study has identified that ferroptosis exerts a pivotal effect on the pathogenesis of DCM. NRF2 agonist sulforaphane inhibited lipid peroxidation via AMPK/NRF2 pathways, which suppressed ferroptosis and prevented DCM (33). These findings suggest that ferroptosis has a substantial impact on DCM.
Sepsis cardiomyopathy is a severe life-threatening complication caused by sepsis (34). Li et al. (35) found ferroptosis is involved in the progression of sepsis cardiomyopathy. Their experiments showed that ferroptotic inhibitor Fer-1 or iron chelates DXZ mitigated lipopolysaccharide (LPS)-induced ferroptotic cell death in sepsis cardiomyopathy model, while ferroptosis inducers sorafenib and erastin exacerbated LPS-induced myocardial injury.
In conclusion, ferroptosis plays a crucial role in the pathogenesis of cardiomyopathy, and ferroptosis inhibitors are expected to be a novel therapeutic strategy for cardiomyopathy.
Ferroptosis and Atherosclerosis
Atherosclerosis is a chronic inflammatory disease involving the main and middle arteries (36). Martinet et al. (37) suggested that intraplaque hemorrhage, iron deposition, and lipid peroxidation are common pathological features of an advanced stage of human atherosclerotic plaque. Guo et al. (38) have found that overexpression of GPX4 inhibited lipid peroxidation and delayed the pathological process of atherosclerosis in ApoE−/−mouse. And lipid peroxidation accumulation is one of the characteristics of ferroptosis, so we speculate that ferroptosis plays an essential role in the initiation and development of atherosclerosis. CD98 heavy chain (CD98hc), also named solute carrier family 3 member 2 (SLC3A2), is a component of the antiporter system . Inhibitor of system triggered endoplasmic reticulum stress and resulted in ferroptosis, while the expression of CD98hc in vascular smooth muscle cells contributed to the stable formation of atherosclerotic plaque (39, 40). There is direct evidence that ferroptosis occurs in the development of atherosclerosis. Ferroptotic inhibitor Fer-1 delayed the progression of atherosclerosis by reducing endothelial dysfunction, lipid peroxidation and iron content in mouse aortic endothelial cells (41). It is well known that diabetes can be complicated with vascular diseases, which include atherosclerosis. A study by Meng et al. (42) indicated that ferroptosis is involved in the occurrence and development of atherosclerosis in diabetes mellitus. In the cell models treated with high glucose, and high lipids, Hmox1 deficiency reduced iron overload, ROS production and lipid peroxidation to inhibit ferroptosis in endothelial cells. Hmox1 may be a therapeutic target for diabetic atherosclerosis. Based on those studies, we know that ferroptosis has an essential effect on atherosclerosis. Targeting ferroptosis may provide new ideas for the treatment of atherosclerosis.
Ferroptosis and Acute Myocardial Infarction
The clinical definition of AMI refers to myocardial injury with abnormal cardiac biomarkers detected in the condition of acute myocardial ischemia (43). Park et al. (44) found that the down-regulation of GPX4 induced ferroptosis during AMI, resulting in cardiomyocyte death and myocardial injury. Baba et al. (45) showed that mechanistic target of rapamycin (mTOR) suppressed cell death, ferroptosis and improved left ventricular remodeling by reducing the production of ROS. MiR-23a-3p is a kind of enriched miRNAs in exosomes derived from mesenchymal stem cells (MSCs) (46). It was reported that DMT1 is a miR-23a-3p target gene. Ferroptosis occurred in the hypoxic cardiomyocytes and infarcted myocardium. MSCs exosomes derived from human umbilical cord blood inhibited ferroptosis via miR-23a-3p/DMT1 axis and mediated myocardial repair in AMI mice (47). In the above studies, ferroptosis has been implicated in the initiation and development of AMI. Inhibition of ferroptosis has been provide novel tactics for the precise treatment of myocardial infarction. Meanwhile, Through machine learning, Huang et al. (48) filtered out ferroptosis-related genes (FRGs) specifically expressed in the peripheral blood of AMI patients. In this study, they also proposed a diagnostic model composed of mitogen-activated protein kinase 3 (MAPK3), WD repeat domain phosphoinositide-interacting protein 2 (WIPI2) and voltage-dependent anion channel three (VDAC3) and provided a new direction for early diagnosis of AMI.
Since diabetes mellitus significantly inhibits the establishment of collateral circulation of ischemic myocardium, aggravating myocardial injury, patients with diabetes comorbiditied with AMI have higher incidence and mortality of coronary heart disease (49). Diabetes increases ROS production in the infarcted myocardium (50), and ROS are considered as essential signals of ferroptosis (51). We hypothesize that ferroptosis might be involved in the pathological process of diabetes comorbiditied with AMI. However, it has not been reported explicitly whether ferroptosis participates in diabetes comorbiditied with AMI, and further studies are needed.
Ferroptosis and Myocardial Ischemia/Reperfusion Injury
Myocardial ischemia/reperfusion injury (I/RI) refers to the pathological process of aggravated myocardial damage caused by reperfusion within a certain period of time after partial or complete acute occlusion of coronary artery. Tang et al. (52) proposed that up-regulation of ubiquitin-specific protease 7 (USP7) activated the protein 53 (p53)/TfR1 pathway to promote ferroptosis in the I/RI rat model. Increased oxidized phosphatidylcholines (OxPCs) caused mitochondrial dysfunction and disrupted calcium transients and resulted in extensive cardiomyocyte death via ferroptosis during myocardial I/RI. Intervention to OxPCs could prevent ferroptosis in I/RI patients (53). These findings supported that ferroptosis might play a significant role in the pathogenesis of myocardial I/RI. Pretreating mice with ferroptotic inhibitor Fer-1, DXZ or liproxstatin-1 (Lip-1) could alleviate myocardial injury after ischemia/reperfusion (23, 54). The latter was mainly achieved by reducing mitochondrial ROS production, increasing GPX4 level, and decreasing voltage-dependent anion channel 1 (VDAC1) level (54). Anthocyanins can be found in most plants and cyanidin-3-glucoside (C3G) is a major type of anthocyanins. Anthocyanins have strong antioxidant activity, which can effectively scavenge free-radical and protect the heart (55). C3G suppressed the promotion of ras synthetic lethal 3 (RSL3) on ferroptosis. C3G reduced the Fe2+content, down-regulated TfR1 and up-regulated ferritin heavy chain1 (FTH1), inhibited ferroptosis and alleviated myocarial injury in I/RI models (56). Likewise, Xanthohumol (XN) isolated from Humulus lupulus had also been shown to protect ischemic/reperfusion myocardium from ferroptosis (57). Besides, exosomal long noncoding RNA (lncRNA) MIR9-3 host gene (Mir9-3hg) derived from bone MSCs mitigated ferroptosis in I/RI mice by regulating pumilio RNA binding family member two (Pum2)/peroxiredoxin 6 (PRDX6) axis and showed cardioprotective effects both in vitro and in vivo (58). These exciting findings have further broadened therapeutic approaches for ferroptosis in I/RI.
Recent studies have demonstrated the pathological process of diabetic I/RI is relevant to ferroptosis. Wang et al. (59) discovered that diabetes exacerbated I/RI via decreasing AMPK, inducing oxidative stress associated with NADPH oxidase 2 (NOX2) and programmed cell death including ferroptosis. Meanwhile, Li et al. (60) found that restraining ferroptosis could reduce endoplasmic reticulum stress and oxidative stress damage and delay the progression of diabetic I/RI. Nevertheless, the role of ferroptosis in diabetes I/RI needs to be better elucidated.
Ferroptosis also participates in I/RI related to heart transplantation. Ferroptosis mediated I/RI after heart transplantation by recruiting neutrophils to the transplanted heart. Inhibition of ferroptosis before transplantation can alleviate reperfusion injury, reduce left ventricular remodeling, and improve the prognosis of heart transplant recipients (61).
Ferroptosis and Heart Failure
Heart failure is a set of clinical syndromes in which cardiac output is inadequate due to various structural and functional abnormalities of the heart (62). The loss of cardiomyocytes plays a crucial part in the development of heart failure. Programmed cell death, such as autophagy and ferroptosis, occurs in the heart failure stage. Knockdown of toll-like receptor 4 (TLR4) or NADPH oxidase 4 (NOX4) restrained ferroptosis and autophagy, which attenuated the loss of cardiomyocytes and delayed the progression of heart failure (63). Moreover, ferroptosis has been observed in heart failure resulted from pressure overload. The model of heart failure was established by aortic coarctation in this research. Antioxidant puerarin could inhibit ferroptosis via increasing GPX4 and ferritin heavy chain 1 (FTH1), and down-regulating expression of NOX4, which could improve cell viability in rats, reduce death of H9C2 cardiomyocytes treated with erastin or isoproterenol (ISO) and retard the development of heart failure (64). Nitenberg et al. (65) demonstrated abnormal myocardial iron probably exists in diabetic heart failure. Iron chelator deferoxamine can improve coronary microcirculation in patients with type two diabetes by suppressing the increase of oxygen radicals, which may be a novel target for reversing deterioration of cardiac function in patients with diabetic heart failure. Nevertheless, the toxicity and short half-life of deferoxamine affect its application in improving cardiac function for clinical patients with diabetic heart failure. Thus, the role of ferroptosis in heart failure remains to be further studied.
Ferroptosis and Other Cardiovascular Diseases
Hypertension is a common cardiovascular disease. Currently, there are few works on the relationship between hypertension and ferroptosis. A research by Yang et al. (66) showed that reductions of GPX4 and GSH in the brains of hypertensive rats led to lipid peroxidation and iron overload, inducing hypertensive brain injury. Elabela is an endogenous ligand for apelin receptor, which is primarily expressed in the cardiac microvascular endothelial cells (CMVECs). Zhang et al. (67) studied the effect of elabela on hypertension. They found that elabela inhibited cardiac oxidative stress, inflammation, fibrosis, and ferroptosis in Angiotensin II (Ang-II) treated CMVECs and hypertensive mice to suppress hypertensive ventricular remodeling. Hence, we guess that ferroptosis might be involved in hypertension and result in the damage to hypertensive target organs.
Aortic dissection (AD), also known as aortic dissecting aneurysm (ADA), is a type of cardiovascular diseases with high mortality (68). Zou et al. (69) revealed that ferroptosis is an important pathological mechanism of Stanford type A aortic dissection (TAAD). Some ferroptosis-related genes mediated ferrptosis in cells and influenced the development of TAAD. Smooth muscle cell (SMC) loss is an important mechanism of aortic dissection. Ferroptosis participated in SMC loss and AD progression. BRD4770 is a new ferroptosis inhibitor, which suppressed inflammatory response, reduced lipid peroxidation and inhibited ferroptosis in SMC of AD mice to prevent the formation of aortic dissection (70, 71).
In addition, recent studies have indicated a possible link between ferroptotic death and arrhythmia. Iron overload caused the occurrence of arrhythmia via promoting mitochondrial ROS generation and membrane potential depolarization, and mitochondrial dysfunction is one of the main characteristics of ferroptosis (72). Frequent alcohol consumption is known to increase the risk of atrial fibrillation (73). Regular drinking promoted ferroptosis via iron overload and increased the incidence of atrial fibrillation. Ferroptosis inhibitor Fer-one, reduced the susceptibility to atrial fibrillation induced by frequent drinking in mice (74). Hence, we supposed that ferroptotic cell death might be a latent target for arrhythmia therapy in the future.
Discussion
Ferroptosis is a novel regulated cell death, which has received much attention in recent years. We discuss the roles of ferroptosis in cardiomyopathy, atherosclerosis, acute myocardial infarction, ischemia, and reperfusion injury, heart failure, hypertension, arrhythmia and aortic dissection in this review (Table 2). But the roles of ferroptosis in other cardiovascular diseases, including valvular heart disease, have been rarely studied, which require further researches. Besides, except for iron chelators DXZ and deferiprone (DFP) authorized by FDA are used in treating DIC and AMI (75, 76), a majority of researches of ferroptosis in cardiovascular diseases have only been confirmed in the cell and animal models, with relatively limited clinical evidence. Thus, clinical investigations are essential for the application of ferroptosis in cardiovascular diseases. Furthermore, ferroptotic inhibitors are greatly limited in the human body due to their toxicity, instability and short half-life. And it is urgent to develop non-toxic and long-acting inhibitors targeting ferroptosis.
A series of researches showed that ferroptosis and other types of programmed cell death take part in cardiovascular diseases together (22, 24, 59, 63). Whether there is a crosstalk between ferroptosis and other cell death forms in various cardiovascular diseases is unclear and needs further researches, which is crucial for reducing cardiomyocyte death and broadening the treatment models of cardiovascular diseases. Liu et al. (77) found that self-assembly indocyanine green-Lecithin (ICG/LECI) can be used to enhance magnetic resonance/ photoacoustic (MR/PA) imaging and reduce iron toxicity, opening the way for personalized diagnosis and treatment for iron overload patients. FRGs specifically expressed in the peripheral blood of AMI patients also provided a new direction for early diagnosis of AMI. However, more attention needs to be paid to the development of testing methods suitable for routine clinical diagnosis of ferroptosis, and the introduction of biomarkers of ferroptosis characteristics is expected to provide helps for the early identification and diagnosis of cardiovascular disease.
In conclusion, ferroptosis plays a key role in the progression of cardiovascular diseases, and the roles of ferroptosis in cardiovascular diseases remain to be further studied. We can anticipate that diagnostic tools and therapeutic drugs based on ferroptosis will greatly help in the diagnosis and treatment of cardiovascular diseases in the future.
Author Contributions
MS: conceived and designed the review. YuG, WZ, XZ, SZ, JWa, YiG, YL, HL, and JL: collected the literatures. YuG, WZ, and MS: wrote the manuscript. MS, JWu, and YC: reviewed and edited the manuscript. JWu: revised the manuscript and the language. All authors contributed to the article and approved the submitted version.
Funding
This study was supported by Hainan Science and Technology Project (ZDYF2020123, ZDYF2020027, and ZDKJ2019012), Hainan Province Clinical Medical Center, National Key R & D Plan (2020YFC2004706), and National Natural Science Foundation of China (Fund No. 81500202).
Conflict of Interest
The authors declare that the research was conducted in the absence of any commercial or financial relationships that could be construed as a potential conflict of interest.
Publisher's Note
All claims expressed in this article are solely those of the authors and do not necessarily represent those of their affiliated organizations, or those of the publisher, the editors and the reviewers. Any product that may be evaluated in this article, or claim that may be made by its manufacturer, is not guaranteed or endorsed by the publisher.
References
1. Mozaffarian D, Benjamin EJ, Go AS, Arnett DK, Blaha MJ, Cushman M, et al. Heart disease and stroke statistics-2016 update: a report from the American Heart Association. Circulation. (2016) 133:e38–60. doi: 10.1161/cir.0000000000000350
2. Joseph P, Leong D, McKee M, Anand SS, Schwalm JD, Teo K, et al. Reducing the global burden of cardiovascular disease, part 1: the epidemiology and risk factors. Circ Res. (2017) 121:677-94. doi: 10.1161/CIRCRESAHA.117.308903
3. Dixon SJ, Lemberg KM, Lamprecht MR, Skouta R, Zaitsev EM, Gleason CE, et al. Ferroptosis: an iron-dependent form of nonapoptotic cell death. Cell. (2012) 149:1060-72. doi: 10.1016/j.cell.2012.03.042
4. Wang J, Pantopoulos K. Regulation of cellular iron metabolism. Biochem J. (2011) 434:365-81. doi: 10.1042/BJ20101825
5. Xie Y, Hou W, Song X, Yu Y, Huang J, Sun X, et al. Ferroptosis: process and function. Cell Death Differ. (2016) 23:369-79. doi: 10.1038/cdd.2015.158
6. Bentea E, Villers A, Moore C, Funk AJ, O'Donovan SM, Verbruggen L, et al. Corticostriatal dysfunction and social interaction deficits in mice lacking the cystine/glutamate antiporter. Mol Psychiatry. (2021) 26:4754-69. doi: 10.1038/s41380-020-0751-3
7. Kim DH, Kim WD, Kim SK, Moon DH, Lee SJ. TGF-beta1-mediated repression of SLC7A11 drives vulnerability to GPX4 inhibition in hepatocellular carcinoma cells. Cell Death Dis. (2020) 11:406. doi: 10.1038/s41419-020-2618-6
8. Yang WS, SriRamaratnam R, Welsch ME, Shimada K, Skouta R, Viswanathan VS, et al. Regulation of ferroptotic cancer cell death by GPX4. Cell. (2014) 156:317–31. doi: 10.1016/j.cell.2013.12.010
9. Lv H, Zhen C, Liu J, Yang P, Hu L, Shang P. Unraveling the potential role of glutathione in multiple forms of cell death in cancer therapy. Oxid Med Cell Longev. (2019) 2019:3150145. doi: 10.1155/2019/3150145
10. Dixon SJ, Winter GE, Musavi LS, Lee ED, Snijder B, Rebsamen M, et al. Human haploid cell genetics reveals roles for lipid metabolism genes in nonapoptotic cell death. ACS Chem Biol. (2015) 10:1604–9. doi: 10.1021/acschembio.5b00245
11. Alvarez SW, Sviderskiy VO, Terzi EM, Papagiannakopoulos T, Moreira AL, Adams S, et al. NFS1 undergoes positive selection in lung tumors and protects cells from ferroptosis. Nature. (2017) 551:639–43. doi: 10.1038/nature24637
12. Do Van B, Gouel F, Jonneaux A, Timmerman K, Gele P, Petrault M, et al. Ferroptosis, a newly characterized form of cell death in Parkinson's disease that is regulated by PKC. Neurobiol Dis. (2016) 94:169–78. doi: 10.1016/j.nbd.2016.05.011
13. Hao S, Yu J, He W, Huang Q, Zhao Y, Liang B, et al. Cysteine dioxygenase 1 mediates erastin-induced ferroptosis in human gastric cancer cells. Neoplasia. (2017) 19:1022–32. doi: 10.1016/j.neo.2017.10.005
14. Lane DJR, Ayton S, Bush AI. Iron and Alzheimer's disease: an update on emerging mechanisms. J Alzheimers Dis. (2018) 64:S379-95. doi: 10.3233/JAD-179944
15. Yuan L, Li S, Chen Q, Xia T, Luo D, Li L, et al. EBV infection-induced GPX4 promotes chemoresistance and tumor progression in nasopharyngeal carcinoma. Cell Death Differ. (2022). doi: 10.1038/s41418-022-00939-8
16. Tao W, Wang N, Ruan J, Cheng X, Fan L, Zhang P, et al. Enhanced ROS-Boosted phototherapy against pancreatic cancer via Nrf2-mediated stress-defense pathway suppression and ferroptosis induction. ACS Appl Mater Interfaces. (2022) 14:6404–16. doi: 10.1021/acsami.1c22861
17. Wang C, Chen S, Guo H, Jiang H, Liu H, Fu H, et al. Forsythoside a mitigates Alzheimer's-like pathology by inhibiting ferroptosis-mediated neuroinflammation via Nrf2/GPX4 axis activation. Int J Biol Sci. (2022) 18:2075–90. doi: 10.7150/ijbs.69714
18. La Rosa P, Petrillo S, Turchi R, Berardinelli F, Schirinzi T, Vasco G, et al. The Nrf2 induction prevents ferroptosis in Friedreich's Ataxia. Redox Biol. (2021) 38:101791. doi: 10.1016/j.redox.2020.101791
19. Lou JS, Zhao LP, Huang ZH, Chen XY, Xu JT, Tai WC, et al. Ginkgetin derived from Ginkgo biloba leaves enhances the therapeutic effect of cisplatin via ferroptosis-mediated disruption of the Nrf2/HO-1 axis in EGFR wild-type non-small-cell lung cancer. Phytomedicine. (2021) 80:153370. doi: 10.1016/j.phymed.2020.153370
20. McKenna WJ, Maron BJ, Thiene G. Classification, epidemiology, and global burden of cardiomyopathies. Circ Res. (2017) 121:722–30. doi: 10.1161/CIRCRESAHA.117.309711
21. Singal PK, Iliskovic N. Doxorubicin-induced cardiomyopathy. N Engl J Med. (1998). 339:900–5. doi: 10.1056/NEJM199809243391307
22. Tadokoro T, Ikeda M, Ide T, Deguchi H, Ikeda S, Okabe K, et al. Mitochondria-dependent ferroptosis plays a pivotal role in doxorubicin cardiotoxicity. JCI Insight. (2020) 5:e132747. doi: 10.1172/jci.insight.132747
23. Fang X, Wang H, Han D, Xie E, Yang X, Wei J, et al. Ferroptosis as a target for protection against cardiomyopathy. Proc Natl Acad Sci USA. (2019) 116:2672–80. doi: 10.1073/pnas.1821022116
24. He H, Wang L, Qiao Y, Yang B, Yin D, He M. Epigallocatechin-3-gallate pretreatment alleviates doxorubicin-induced ferroptosis and cardiotoxicity by upregulating AMPKalpha2 and activating adaptive autophagy. Redox Biol. (2021) 48:102185. doi: 10.1016/j.redox.2021.102185
25. Sun X, Sun P, Zhen D, Xu X, Yang L, Fu D, et al. Melatonin alleviates doxorubicin-induced mitochondrial oxidative damage and ferroptosis in cardiomyocytes by regulating YAP expression. Toxicol Appl Pharmacol. (2022) 437:115902. doi: 10.1016/j.taap.2022.115902
26. Jia G, Hill MA, Sowers JR. Diabetic cardiomyopathy: an update of mechanisms contributing to this clinical entity. Circ Res. (2018) 122:624–38. doi: 10.1161/CIRCRESAHA.117.311586
27. Wilson AJ, Gill EK, Abudalo RA, Edgar KS, Watson CJ, Grieve DJ. Reactive oxygen species signaling in the diabetic heart: emerging prospect for therapeutic targeting. Heart. (2018) 104:293–9. doi: 10.1136/heartjnl-2017-311448
28. Yang WS, Stockwell BR. Ferroptosis: death by lipid peroxidation. Trends Cell Biol. (2016) 26:165–76. doi: 10.1016/j.tcb.2015.10.014
29. Shirpoor A, Salami S, Khadem-Ansari MH, Ilkhanizadeh B, Pakdel FG, Khademvatani K. Cardioprotective effect of vitamin E: rescues of diabetes-induced cardiac malfunction, oxidative stress, and apoptosis in rat. J Diabet Complic. (2009) 23:310–6. doi: 10.1016/j.jdiacomp.2008.02.009
30. Huynh K, Kiriazis H, Du XJ, Love JE, Jandeleit-Dahm KA, Forbes JM, et al. Coenzyme Q10 attenuates diastolic dysfunction, cardiomyocyte hypertrophy and cardiac fibrosis in the db/db mouse model of type 2 diabetes. Diabetologia. (2012) 55:1544–53. doi: 10.1007/s00125-012-2495-3
31. Katunga LA, Gudimella P, Efird JT, Abernathy S, Mattox TA, Beatty C, et al. Obesity in a model of gpx4 haploinsufficiency uncovers a causal role for lipid-derived aldehydes in human metabolic disease and cardiomyopathy. Mol Metab. (2015) 4:493–506. doi: 10.1016/j.molmet.2015.04.001
32. Baseler WA, Dabkowski ER, Jagannathan R, Thapa D, Nichols CE, Shepherd DL, et al. Reversal of mitochondrial proteomic loss in Type 1 diabetic heart with overexpression of phospholipid hydroperoxide glutathione peroxidase. Am J Physiol Regul Integr Comp Physiol. (2013) 304:R553-65. doi: 10.1152/ajpregu.00249.2012
33. Wang X, Chen X, Zhou W, Men H, Bao T, Sun Y, et al. Ferroptosis is essential for diabetic cardiomyopathy and is prevented by sulforaphane via AMPK/NRF2 pathways. Acta Pharm Sin B. (2022) 12:708–22. doi: 10.1016/j.apsb.2021.10.005
34. Zechendorf E, O'Riordan CE, Stiehler L, Wischmeyer N, Chiazza F, Collotta D, et al. Ribonuclease 1 attenuates septic cardiomyopathy and cardiac apoptosis in a murine model of polymicrobial sepsis. JCI Insight. (2020) 5:e131571. doi: 10.1172/jci.insight.131571
35. Li N, Wang W, Zhou H, Wu Q, Duan M, Liu C, et al. Ferritinophagy-mediated ferroptosis is involved in sepsis-induced cardiac injury. Free Radic Biol Med. (2020) 160:303–18. doi: 10.1016/j.freeradbiomed.2020.08.009
36. Wolf D, Ley K. Immunity and inflammation in atherosclerosis. Circ Res. (2019) 124:315–27. doi: 10.1161/CIRCRESAHA.118.313591
37. Martinet W, Coornaert I, Puylaert P, De Meyer GRY. Macrophage death as a pharmacological target in atherosclerosis. Front Pharmacol. (2019) 10:306. doi: 10.3389/fphar.2019.00306
38. Guo Z, Ran Q, Roberts LJ, Zhou L, Richardson A, Sharan C, et al. Suppression of atherogenesis by overexpression of glutathione peroxidase-4 in apolipoprotein E-deficient mice. Free Radic Biol Med. (2008) 44:343–52. doi: 10.1016/j.freeradbiomed.2007.09.009
39. Dixon SJ, Patel DN, Welsch M, Skouta R, Lee ED, Hayano M, et al. Pharmacological inhibition of cystine-glutamate exchange induces endoplasmic reticulum stress and ferroptosis. Elife. (2014) 3:e02523. doi: 10.7554/eLife.02523
40. Baumer Y, McCurdy S, Alcala M, Mehta N, Lee BH, Ginsberg MH, et al. CD98 regulates vascular smooth muscle cell proliferation in atherosclerosis. Atherosclerosis. (2017) 256:105–14. doi: 10.1016/j.atherosclerosis.2016.11.017
41. Bai T, Li M, Liu Y, Qiao Z, Wang Z. Inhibition of ferroptosis alleviates atherosclerosis through attenuating lipid peroxidation and endothelial dysfunction in mouse aortic endothelial cell. Free Radic Biol Med. (2020) 160:92–102. doi: 10.1016/j.freeradbiomed.2020.07.026
42. Meng Z, Liang H, Zhao J, Gao J, Liu C, Ma X, et al. HMOX1 upregulation promotes ferroptosis in diabetic atherosclerosis. Life Sci. (2021) 284:119935. doi: 10.1016/j.lfs.2021.119935
43. Thygesen K, Alpert JS, Jaffe AS, Chaitman BR, Bax JJ, Morrow DA, et al. Fourth Universal Definition of Myocardial Infarction (2018). J Am Coll Cardiol. (2018) 72:2231–64. doi: 10.1016/j.jacc.2018.08.1038
44. Park TJ, Park JH, Lee GS, Lee JY, Shin JH, Kim MW, et al. Quantitative proteomic analyses reveal that GPX4 downregulation during myocardial infarction contributes to ferroptosis in cardiomyocytes. Cell Death Dis. (2019) 10:835. doi: 10.1038/s41419-019-2061-8
45. Baba Y, Higa JK, Shimada BK, Horiuchi KM, Suhara T, Kobayashi M, et al. Protective effects of the mechanistic target of rapamycin against excess iron and ferroptosis in cardiomyocytes. Am J Physiol Heart Circ Physiol. (2018) 314:H659-68. doi: 10.1152/ajpheart.00452.2017
46. Ferguson SW, Wang J, Lee CJ, Liu M, Neelamegham S, Canty JM, et al. The microRNA regulatory landscape of MSC-derived exosomes: a systems view. Sci Rep. (2018) 8:1419. doi: 10.1038/s41598-018-19581-x
47. Song Y, Wang B, Zhu X, Hu J, Sun J, Xuan J, et al. Human umbilical cord blood-derived MSCs exosome attenuate myocardial injury by inhibiting ferroptosis in acute myocardial infarction mice. Cell Biol Toxicol. (2021) 37:51–64. doi: 10.1007/s10565-020-09530-8
48. Huang D, Zheng S, Liu Z, Zhu K, Zhi H, Ma G. Machine learning revealed ferroptosis features and a novel ferroptosis-based classification for diagnosis in acute myocardial infarction. Front Genet. (2022) 13:813438. doi: 10.3389/fgene.2022.813438
49. Weihrauch D, Lohr NL, Mraovic B, Ludwig LM, Chilian WM, Pagel PS, et al. Chronic hyperglycemia attenuates coronary collateral development and impairs proliferative properties of myocardial interstitial fluid by production of angiostatin. Circulation. (2004) 109:2343–48. doi: 10.1161/01.CIR.0000129225.67353.1F
50. Shen M, Bai D, Liu B, Lu X, Hou R, Zeng C, et al. Dysregulated Txnip-ROS-Wnt axis contributes to the impaired ischemic heart repair in diabetic mice. Biochim Biophys Acta Mol Basis Dis. (2018) 1864:3735–45. doi: 10.1016/j.bbadis.2018.09.029
51. Tang D, Chen X, Kang R, Kroemer G. Ferroptosis: molecular mechanisms and health implications. Cell Res. (2021) 31:107–25. doi: 10.1038/s41422-020-00441-1
52. Tang LJ, Zhou YJ, Xiong XM, Li NS, Zhang JJ, Luo XJ, et al. Ubiquitin-specific protease 7 promotes ferroptosis via activation of the p53/TfR1 pathway in the rat hearts after ischemia/reperfusion. Free Radic Biol Med. (2021) 162:339–52. doi: 10.1016/j.freeradbiomed.2020.10.307
53. Stamenkovic A, O'Hara KA, Nelson DC, Maddaford TG, Edel AL, Maddaford G, et al. Oxidized phosphatidylcholines trigger ferroptosis in cardiomyocytes during ischemia-reperfusion injury. Am J Physiol Heart Circ Physiol. (2021) 320:H1170-84. doi: 10.1152/ajpheart.00237.2020
54. Feng Y, Madungwe NB, Imam Aliagan AD, Tombo N, Bopassa JC. Liproxstatin-1 protects the mouse myocardium against ischemia/reperfusion injury by decreasing VDAC1 levels and restoring GPX4 levels. Biochem Biophys Res Commun. (2019) 520:606–11. doi: 10.1016/j.bbrc.2019.10.006
55. Eng QY, Thanikachalam PV, Ramamurthy S. Molecular understanding of Epigallocatechin gallate (EGCG) in cardiovascular and metabolic diseases. J Ethnopharmacol. (2018) 210:296–310. doi: 10.1016/j.jep.2017.08.035
56. Shan X, Lv ZY, Yin MJ, Chen J, Wang J, Wu QN. The protective effect of cyanidin-3-glucoside on myocardial ischemia-reperfusion injury through ferroptosis. Oxid Med Cell Longev. (2021) 2021:8880141. doi: 10.1155/2021/8880141
57. Lin JH, Yang KT, Lee WS, Ting PC, Luo YP, Lin DJ, et al. Xanthohumol protects the rat myocardium against ischemia/reperfusion injury-induced ferroptosis. Oxid Med Cell Longev. (2022) 2022:9523491. doi: 10.1155/2022/9523491
58. Zhang JK, Zhang Z, Guo ZA, Fu Y, Chen XJ, Chen WJ, et al. The BMSC-derived exosomal lncRNA Mir9-3hg suppresses cardiomyocyte ferroptosis in ischemia-reperfusion mice via the Pum2/PRDX6 axis. Nutr Metab Cardiovasc Dis. (2022) 32:515–27. doi: 10.1016/j.numecd.2021.10.017
59. Wang C, Zhu L, Yuan W, Sun L, Xia Z, Zhang Z, et al. Diabetes aggravates myocardial ischaemia reperfusion injury via activating Nox2-related programmed cell death in an AMPK-dependent manner. J Cell Mol Med. (2020) 24:6670–79. doi: 10.1111/jcmm.15318
60. Li W, Li W, Leng Y, Xiong Y, Xia Z. Ferroptosis is involved in diabetes myocardial ischemia/reperfusion injury through endoplasmic reticulum stress. DNA Cell Biol. (2020) 39:210–25. doi: 10.1089/dna.2019.5097
61. Li W, Feng G, Gauthier JM, Lokshina I, Higashikubo R, Evans S, et al. Ferroptotic cell death and TLR4/Trif signaling initiate neutrophil recruitment after heart transplantation. J Clin Invest. (2019) 129:2293–304. doi: 10.1172/JCI126428
62. Ponikowski P, Voors AA, Anker SD, Bueno H, Cleland JGF, Coats AJS, et al. 2016 ESC Guidelines for the diagnosis and treatment of acute and chronic heart failure: the task force for the diagnosis and treatment of acute and chronic heart failure of the European Society of Cardiology (ESC) Developed with the special contribution of the Heart Failure Association (HFA) of the ESC. Eur Heart J. (2016) 37:2129–200. doi: 10.1093/eurheartj/ehw128
63. Chen X, Xu S, Zhao C, Liu B. Role of TLR4/NADPH oxidase 4 pathway in promoting cell death through autophagy and ferroptosis during heart failure. Biochem Biophys Res Commun. (2019) 516:37–43. doi: 10.1016/j.bbrc.2019.06.015
64. Liu B, Zhao C, Li H, Chen X, Ding Y, Xu S. Puerarin protects against heart failure induced by pressure overload through mitigation of ferroptosis. Biochem Biophys Res Commun. (2018) 497:233–40. doi: 10.1016/j.bbrc.2018.02.061
65. Nitenberg A, Ledoux S, Valensi P, Sachs R, Antony I. Coronary microvascular adaptation to myocardial metabolic demand can be restored by inhibition of iron-catalyzed formation of oxygen free radicals in type 2 diabetic patients. Diabetes. (2002) 51:813–8. doi: 10.2337/diabetes.51.3.813
66. Yang J, Wang M, Wang S, Li G, Gao Y. Study on ferroptosis pathway that operates in hypertensive brain damage. Clin Exp Hypertens. (2020) 42:748–52. doi: 10.1080/10641963.2020.1783545
67. Zhang Z, Tang J, Song J, Xie M, Liu Y, Dong Z, et al. Elabela alleviates ferroptosis, myocardial remodeling, fibrosis and heart dysfunction in hypertensive mice by modulating the IL-6/STAT3/GPX4 signaling. Free Radic Biol Med. (2022) 181:130–42. doi: 10.1016/j.freeradbiomed.2022.01.020
68. Nienaber CA, Clough RE. Management of acute aortic dissection. Lancet. (2015) 385:800–11. doi: 10.1016/s0140-6736(14)61005-9
69. Zou HX, Qiu BQ, Lai SQ, Huang H, Zhou XL, Gong CW, et al. Role of ferroptosis-related genes in Stanford type a aortic dissection and identification of key genes: new insights from bioinformatic analysis. Bioengineered. (2021) 12:9976–90. doi: 10.1080/21655979.2021.1988840
70. Wu D, Shen YH, Russell L, Coselli JS, LeMaire SA. Molecular mechanisms of thoracic aortic dissection. J Surg Res. (2013) 184:907–24. doi: 10.1016/j.jss.2013.06.007
71. Chen Y, Yi X, Huo B, He Y, Guo X, Zhang Z, et al. BRD4770 functions as a novel ferroptosis inhibitor to protect against aortic dissection. Pharmacol Res. (2022) 177:106122. doi: 10.1016/j.phrs.2022.106122
72. Gordan R, Fefelova N, Gwathmey JK, Xie LH. Iron overload, oxidative stress and calcium mishandling in cardiomyocytes: role of the mitochondrial permeability transition pore. Antioxidants. (2020) 9:758. doi: 10.3390/antiox9080758
73. Kim YG, Han KD, Choi JI, Boo KY, Kim DY, Lee KN, et al. Frequent drinking is a more important risk factor for new-onset atrial fibrillation than binge drinking: a nationwide population-based study. Europace. (2020) 22:216–24. doi: 10.1093/europace/euz256
74. Dai C, Kong B, Qin T, Xiao Z, Fang J, Gong Y, et al. Inhibition of ferroptosis reduces susceptibility to frequent excessive alcohol consumption-induced atrial fibrillation. Toxicology. (2022) 465:153055. doi: 10.1016/j.tox.2021.153055
75. Behrouzi B, Weyers JJ, Qi X, Barry J, Rabadia V, Manca D, et al. Action of iron chelator on intramyocardial hemorrhage and cardiac remodeling following acute myocardial infarction. Basic Res Cardiol. (2020) 115:24. doi: 10.1007/s00395-020-0782-6
76. Li N, Jiang W, Wang W, Xiong R, Wu X, Geng Q. Ferroptosis and its emerging roles in cardiovascular diseases. Pharmacol Res. (2021) 166:105466. doi: 10.1016/j.phrs.2021.105466
Keywords: ferroptosis, atherosclerosis, acute myocardial infarction, cardiomyopathy, heart failure
Citation: Guo YT, Zhang W, Zhou X, Zhao S, Wang J, Guo Y, Liao Y, Lu H, Liu J, Cai Y, Wu J and Shen M (2022) Roles of Ferroptosis in Cardiovascular Diseases. Front. Cardiovasc. Med. 9:911564. doi: 10.3389/fcvm.2022.911564
Received: 02 April 2022; Accepted: 02 May 2022;
Published: 23 May 2022.
Edited by:
Yuli Huang, Southern Medical University, ChinaCopyright © 2022 Guo, Zhang, Zhou, Zhao, Wang, Guo, Liao, Lu, Liu, Cai, Wu and Shen. This is an open-access article distributed under the terms of the Creative Commons Attribution License (CC BY). The use, distribution or reproduction in other forums is permitted, provided the original author(s) and the copyright owner(s) are credited and that the original publication in this journal is cited, in accordance with accepted academic practice. No use, distribution or reproduction is permitted which does not comply with these terms.
*Correspondence: Mingzhi Shen, shenmz301@163.com; Jiao Wu, jiaowubio@hotmail.com
†These authors have contributed equally to this work and share first authorship