Alternative pacing strategies for optimal cardiac resynchronization therapy
- Department of Cardiology, The Second Affiliated Hospital of Nanchang University, Nanchang, China
Cardiac resynchronization therapy (CRT) via biventricular pacing (BVP) improves morbidity, mortality, and quality of life, especially in subsets of patients with impaired cardiac function and wide QRS. However, the rate of unsuccessful or complicated left ventricular (LV) lead placement through coronary sinus is 5–7%, and the rate of “CRT non-response” is approximately 30%. These reasons have pushed physicians and engineers to collaborate to overcome the challenges of LV lead implantation. Thus, various alternatives to BVP have been proposed to improve CRT effectiveness. His bundle pacing (HBP) has been increasingly used by activating the His–Purkinje system but is constrained by challenging implantation, low success rates, high and often unstable thresholds, and low perception. Therefore, the concept of pacing a specialized conduction system distal to the His bundle to bypass the block region was proposed. Multiple clinical studies have demonstrated that left bundle branch area pacing (LBBAP) has comparable electrical resynchronization with HBP but is superior in terms of simpler operation, higher success rates, lower and stable capture thresholds, and higher perception. Despite their well-demonstrated effectiveness, the transvenous lead-related complications remain major limitations. Recently, leadless LV pacing has been developed and demonstrated effective for these challenging patient cohorts. This article focuses on the current state and latest progress in HBP, LBBAP, and leadless LV pacing as alternatives for failed or non-responsive conventional CRT as well as their limits and prospects.
Introduction
Heart failure (HF) is a cardiovascular epidemic, with high morbidity and mortality and poor quality of life, especially in patients with HF and reduced ejection fraction (HFrEF) (1). According to the 2021 European HF guidelines, sodium–glucose cotransporter 2 inhibitors (SGLT2i) are used as a first-line therapy along with angiotensin-converting enzyme inhibitors (ACEi)/angiotensin receptor–neprilysin inhibitors (ARNI), β-blockers, and mineralocorticoid receptor antagonists (MRA) (2). However, the HF symptoms of some patients cannot be resolved, despite optimized medical treatments (OMT).
Cardiac resynchronization therapy (CRT) is a well-established modality that offers remarkable clinical benefits for patients with medically refractory HF (3). It has a class IA indication for symptomatic HF patients with sinus rhythm (SR), a QRS ≥ 150 ms, left bundle branch block (LBBB) QRS morphology, and a left ventricular ejection fraction (LVEF) ≤ 35%, despite OMT according to the 2021 European Society of Cardiology (ESC) guidelines on CRT (4). Conventional CRT via biventricular pacing (BVP) is non-physiological with the fusion of the epicardial LV wavefront and the endocardial wavefront from the right ventricular (RV) apex, leaving some degree of dyssynchrony. However, conventional CRT is precluded in a proportion of eligible candidates due to anatomic or technical constraints such as occluded venous access, an inappropriate coronary sinus (CS) anatomy, or a high threshold in regions of fibrosis (5, 6). In addition, approximately 30% of recipients are non-responsive to CRT due to the inability to effectively stimulate diseased tissue, or suboptimal LV lead placement (7, 8).
For these reasons, physicians and engineers have been working together to overcome the challenges of LV lead implantation but have also shown increased interest in developing physiological pacing techniques to improve CRT effectiveness. His bundle pacing (HBP) has increased in use by activating the His bundle but is restricted by implant challenges, low success rates, and a high and often unstable pacing threshold (9–12). Therefore, the concept of pacing the specialized conduction system distal to the His bundle to bypass the block region has been introduced (13). Multiple clinical studies reported that left bundle branch area pacing (LBBAP) has electrical resynchronization that is comparable with that of HBP but superior due to its simpler operation, higher success rate, and low and stable pacing threshold (13–17). Despite their well-demonstrated effectiveness, the resulting complications of transvenous leads and typical pocket infections remain a non-negligible limitation (18, 19). Thus, leadless cardiac pacing has been engineered and demonstrated as having potential efficacy for treating those challenging patient cohorts (20, 21).
From CS epicardial pacing to leadless endocardial stimulation, various LV pacing alternatives reportedly improve CRT effectiveness (Figure 1). This review focuses on the current state and latest progress of HBP, LBBAP, and leadless LV pacing as alternatives for impossible or failed conventional CRT as well as their limits and future areas of improvement.
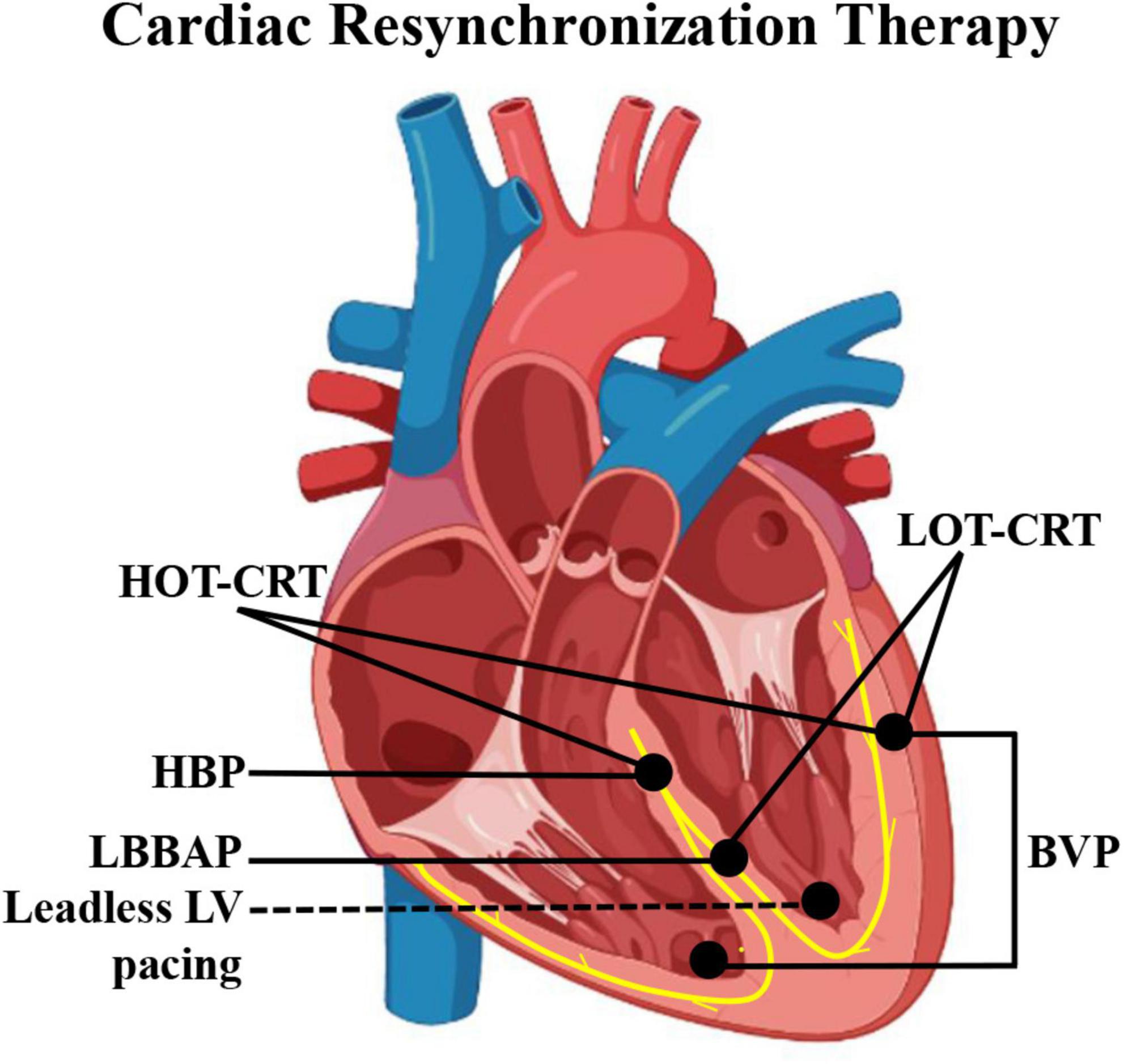
Figure 1. Schematic diagram of pacing electrode positions of different CRT modalities. BVP, biventricular pacing; CRT, cardiac resynchronization therapy; HBP, His bundle pacing; HOT-CRT, His-optimized CRT; LBBAP, left bundle branch area pacing; LOT-CRT, LBBAP-optimized CRT.
Benefits and limits of biventricular pacing-cardiac resynchronization therapy
The efficacy of CRT in patients with HF has been demonstrated in numerous trials. The Multisite Stimulation in Cardiomyopathies (MUSTIC) study was the first to assess the clinical outcomes of CRT in 67 patients with severe HF (22). Finally, 48 patients completed both phases of the study. The quality-of-life score improved by 32%, while hospitalizations decreased by 67.7%. Similarly, the Multicenter InSync Randomized Clinical Evaluation (MIRACLE) trial was the first double-blind trial to assess the CRT outcomes in 453 patients with moderate to severe HF with an LVEF ≤ 35% and QRS ≥ 130 ms (23). CRT improved the New York Heart Association (NYHA) class, quality of life, and LVEF and reduced hospitalization and intravenous interventions. The Cardiac Resynchronization–Heart Failure (CARE-HF) trial was mainly conducted to assess the morbidity and mortality of CRT in 813 patients with HF (NYHA class III/IV) (24). The results indicated that CRT reduced mortality of any cause, increased the LVEF, and improved symptoms and quality of life. Subsequently, the REVERSE, MADIT-CRT, and RAFT trials assessed the efficacy of CRT in mildly symptomatic HF (25–27). These results showed that CRT significantly delayed the time to the first HF hospital stay or death, reduced the risk of HF events, and improved LV reverse remodeling.
However, approximately 30% of candidates respond unfavorably to CRT or even worsen (23, 24, 28). In fact, the optimal LV pacing site is not always consistent with the right branch of the CS. In addition, phrenic stimulation, occlusion of the CS anatomy, or other anatomical constraints would hamper the procedure. Thus, several approaches based on BVP-CRT, such as the adaptive CRT algorithm (29), the SyncAV algorithm (30), and multipoint pacing (MPP) (31), have improved the effectiveness of BVP-CRT. Notably, the optimal electrode position was not equal to that of the optimal treatment. In addition, little experience has been gained regarding the optimal programming of pacemakers after MPP. Furthermore, the higher battery use of MPP also prevents its recommendation.
Alternative pacing strategies for conventional cardiac resynchronization therapy
Despite great advances in multipolar LV electrodes and MPP, non-response due to suboptimal lead position remains a critical problem. To overcome the challenges of the CS approach, physicians have strived for alternative solutions, such as surgical LV epicardial lead placement, LV endocardial pacing, or HBP to most recently leadless LV pacing. However, surgical LV epicardial lead placement does not always deliver additional improvement in the LVEF versus conventional CRT, and it is inherently more invasive and challenging in patients with a previous history of heart surgery (32). Moreover, the greatest concern for LV endocardial pacing is the risk of thromboembolic complications and the need for lifelong anticoagulation (33). By contrast, physiological pacing modalities have advantages over conventional LV epicardial and endocardial pacing. Next, this review focuses on the progress, limits, and prospects of HBP, LBBAP, and leadless LV pacing used for CRT.
His bundle pacing for cardiac resynchronization therapy
His bundle pacing activates the His–Purkinje system (HPS) and restores physiological activation of the ventricles. HBP is defined as the presence or absence of His–Purkinje conduction disease (HPCD) according to four basic criteria (34): (1) relationship between the His-QRS (H-QRS) and stimulus-QRS (S-QRS) intervals; (2) the presence or absence of direct capture of the local ventricular electrogram (EGM) on the pacing lead; (3) QRS duration (QRSd) and morphology; and (4) capture thresholds. Broadly, there are two forms of HBP: selective HBP (S-HBP), in which the His bundle is exclusively captured; and non-selective HBP (NS-HBP), in which both the His bundle and its surrounding ventricular tissues are captured. The form of S-HBP or NS-HBP is usually dependent on the location of the pacing electrode in relation to the His bundle and the surrounding tissue (35). S-HBP can be differentiated from NS-HBP by review of the His bundle EGM on the pacing lead (34, 36): the local ventricular EGM is discrete and separate from the pacing stimulus on S-HBP, whereas it is fused with the pacing stimulus on NS-HBP. The specific criteria for S-HBP or NS-HBP are as follows (9, 34):
S-HBP: (1) S-QRS = H-QRS with an isoelectric interval. In patients with HPCD, S-QRS ≤ H-QRS with BBB correction and S-QRS ≤ or > H-QRS without BBB correction; (2) discrete local ventricular EGM in HBP leads with the stimulus to the local ventricle (S-V) = His to the local ventricle (H-V); (3) paced QRS = native QRS. In patients with HPCD, paced QRS < native QRS with BBB correction; paced QRS = native QRS without BBB correction; and 4) a single capture threshold (His capture) was observed. In patients with HPCD, two distinct His capture thresholds (with and without BBB correction) may be observed.
NS-HBP: (1) S-QRS < H-QRS (usually 0, S-QRSend = H-QRSend) with or without an isoelectric interval. In patients with HPCD, S-QRSend < H-QRSend with BBB correction; (2) direct capture of local ventricular EGM in HBP lead by a stimulus artifact; (3) paced QRS > native QRS. In patients with HPCD, paced QRS ≤ native QRS with BBB correction and paced QRS > native QRS without BBB correction; (4) usually, two distinct capture thresholds (His bundle capture and RV capture) are observed. In patients with HPCD, three distinct capture thresholds (with or without correction for BBB and RV capture) may be observed.
Theoretically, S-HBP may be advantageous over NS-HBP in terms of clinical outcomes. Instead, few hemodynamic and clinical differences were observed between these two forms of capture, probably owing to the rapid conduction of the HPS relative to the ventricular myocardial conduction (37, 38).
Evidence for resynchronization using His bundle pacing
In 2000, HBP was first described in 12 HF patients with atrial fibrillation, cardiomyopathy, and improvements in LV function after HBP and atrioventricular node ablation (39). In 2012, a series of direct HBP (DHBP) was reported in 16 patients after CS approach failure (40). Of these, LBBB was corrected in 13 of 16 patients, and permanent HBP (pHBP) was performed successfully in nine of 13 patients. LBBB was corrected using pHBP, with a mean QRS reduction (166 ± 8 to 97 ± 9 ms; P < 0.01). Subsequently, a prospective study assessed the HBP outcomes of patients with HF and LBBB (18). A total of 74 patients were enrolled, and pHBP was successful in 56 of them (75.7%). Of them, 30 had completed a 3-year follow-up with an increased mean LVEF (32.4 ± 8.9% to 55.9 ± 10.7%, P < 0.001) and decreased LV end-systolic volume (LVESV; 137.9 ± 64.1 to 52.4 ± 32.6 mL, P < 0.001). Similarly, the outcomes of HBP were explored in 106 CRT-eligible or -failed patients (41). Among them, HBP was successful in 95 patients (89.6%). During an average follow-up of 14 months, it also delivered significant QRS narrowing, increased LVEF, and improved NYHA class. Lead-related complications were observed in seven patients. These studies demonstrated that HBP may be a promising treatment for failed BVP.
His bundle pacing versus biventricular pacing for cardiac resynchronization therapy
Multiple studies demonstrated that HBP may be an effective alternative to BVP; however, whether it is equal to or better than BVP requires further evaluation. The first crossover study to compare the outcomes of HBP and BVP enrolled 29 patients for HBP as an alternative to BVP (10). Finally, 21 of 29 patients achieved narrow-paced QRSd. The baseline LVEF was 26% with improvements at 6 months in the HBP (32%) and BVP (31%). A similar result of HBP delivering a greater reduction in QRSd, LV activation time (LVAT), and LV dyssynchrony index (LVDI) than BVP was reported (42). The His-SYNC trial was the first randomized comparison of HBP and BVP using treatment-received (TR) and per-protocol (PP) analyses (43). A total of 41 patients were enrolled and randomized into HBP (n = 21) and BVP (n = 20) groups. Compared with BVP, HBP achieved a narrower mean QRSd, regardless of TR or PP analyses (TR: 125 ± 22 vs. 164 ± 25 ms, P < 0.001; PP: 124 ± 19 vs. 162 ± 24 ms, P < 0.001). Furthermore, a non-significant trend toward a higher echocardiographic response was observed. There were also no significant intergroup differences in CV hospitalization and mortality. Another randomized trial of HBP versus BVP (His-Alternative) was performed in patients with symptomatic HF and LBBB (44). The pacing thresholds of HBP were higher than those of BVP, both at implantation and at the 6-month follow-up. Using PP analysis, the LVEF was significantly increased, and the 6-month LVESV was lower in patients with HBP than in those with BVP. These data revealed that HBP was equivalent to, or even better than, BVP in some cases; however, further investigations are required to confirm these findings.
His-optimized cardiac resynchronization therapy
The use of HBP alone may not always be optimal for obtaining QRS narrowing. Several studies have explored whether CRT could maximize electrical resynchronization by HBP fused with sequential LV pacing, termed His-optimized CRT (HOT-CRT) (45–48). HOT-CRT was attempted in 27 patients with LBBB/intraventricular conduction defect (IVCD) partially corrected by HBP alone (45). HOT-CRT produced a greater narrowing of the mean QRSd to 120 ± 16 ms (vs. baseline, BVP, or HBP; P < 0.0001). LVEF improved significantly (from 24 ± 7% to 38 ± 10%, P = 0.001) after a mean follow-up of 14 ± 10 months. Similar results with a narrower QRSd, increased LVEF, and improved NYHA were reported in other studies (46–48). In addition, HOT-CRT versus HBP resulted in significant QRS narrowing, thus achieving electrical resynchronization in four of five patients with IVCD (45). These data indicate that HOT-CRT produced more pronounced QRS narrowing and improved clinical outcomes than HBP alone. Particularly, HOT-CRT could further optimize electrical resynchronization in patients with advanced cardiomyopathy and conduction disease; however, this finding requires further verification.
Limits of His bundle pacing
These trials indicated that HBP generates a narrow paced QRS and improves clinical outcomes, which seem potential for CRT. However, HBP has some limitations. First, the major limit is the inability to map the precise location of the His bundle, which is approximately 1–2 mm in diameter (49). The mean success rate of HBP was approximately 79.8% (mostly were performed with the SelectSecure 3830 lead; Medtronic, Minneapolis, MN, United States), while the lead-related complication rate was 6% (50). Second, 30–40% of LBBB cannot be corrected by HBP because of the presence of lesions distal or more extensive to the conduction tract during implantation (51). Third, the HBP threshold increased over time. About 53.6% of patients had a significant increase in the His capture threshold after a mean follow-up of 3 years (18). A progressive increase in the pacing threshold implies a shortened battery longevity. HBP may also undersense the ventricle and oversense the atrium, thus resulting in crosstalk. Finally, most of the current research conclusions on the application of HBP in HF with LBBB were derived from the data of HBP with failed BVP, while large-scale randomized controlled clinical trials of HBP and BVP are lacking. Furthermore, the number of patients who responded to HBP and did not respond to BVP was small, and similarly, the number of patients who included HOT-CRT with IVCD HF and were refractory to BVP or HBP was small.
Left bundle branch area pacing for cardiac resynchronization therapy
In 2017, Huang et al. (13) first introduced left bundle branch pacing (LBBP) in a patient with HF and LBBB and confirmed its feasibility and safety. LBBP captures the proximal LBB or its branches with or without the LV septal myocardium. LV septal pacing (LVSP) exclusively captures the LV septal myocardium. However, during the early stage of LBBP, the criteria for LBB capture are not well defined and uniform. With the increased use and further research on LBBP, the definition of LBB capture is gradually becoming definitive (52). Broadly, there are two forms of LBBP: selective LBBP (S-LBBP) exclusively captures the LBB, whereas non-selective LBBP (NS-LBBP) captures the LBB along with the surrounding local myocardium. The detailed characteristics of LBBP are defined as follows: (1) RBBB pattern, (2) LBB potential, (3) S-LBBP with specific ECG changes and a discrete component on EGM, and (4) a constant and shortest stimulus to the LVAT, regardless of high or low pacing outputs. LBBP is differentiated from LVSP based on the mentioned characteristics of the indirect criteria for LBB capture. Thus, LVSP is mistakenly considered LBBP in some cases. Wu et al. (53) proposed retrograde His bundle potential or anterograde left conduction system potentials to directly confirm LBB capture, which can more accurately distinguish between LBBP and LVSP. However, this method is complicated and unsuitable for routine clinical use. In this context, the concept of LBB area pacing (LBBAP) has been proposed, that is, LBBP or LVSP, without clear evidence for LBB capture (4). During LBBAP, the QRS morphologies in lead V1 are typically demonstrated as Qr (60.7%), qR (19.6%), rSR’ (7.1%), or QS (12.5%) patterns, and the duration of the terminal R’ wave was significantly shorter than that of native RBBB (54).
Evidence for resynchronization using left bundle branch area pacing
Several single-center studies with short follow-up periods have confirmed the potential of LBBAP in patients with HF and wide QRS (13, 55). A prospective multicenter medium-term study assessed LBBP in patients with LBBB and non-ischemic cardiomyopathy (56). LBBP was successful in 61 of 63 patients (97%). It produced a shortened mean QRSd (169 ± 16 to 118 ± 12 ms, P < 0.001), increased LVEF (33 ± 8% to 55 ± 10%, P < 0.001), decreased LVESV (123 ± 61 to 67 ± 39 mL, P < 0.001), and improved NYHA class (2.8 ± 0.6 to 1.4 ± 0.6, P < 0.001). A subsequent long-term trial with a larger sample size (N = 632) assessed LBBP feasibility and safety (57). LBBP was successful in 618 (97.8%) patients, and the mean follow-up was 18.6 ± 6.7 months. A significant decrease in QRSd was observed in patients with LBBB. LVEF after LBBP improved in patients with QRS ≥ 120 ms (N = 88). No serious complications occurred during the procedure or follow-up. A similar result was reported by another large study (N = 325) (19) in which LBBAP was successfully achieved in 277 (85%) patients. During a mean follow-up period of 6 ± 5 months, LBBAP also resulted in significant QRS narrowing and improved LVEF. In a current meta-analysis, LBBP for CRT resulted in a narrower QRSd and an increased LVEF than baseline (58). Nonetheless, relatively few studies have examined LVSP for CRT. To date, LVSP has been demonstrated to generate short-term hemodynamic improvement and electrical resynchronization equal to that of BVP and possibly HBP (59). These data demonstrated that LBBAP may be a promising rescue strategy for failed BVP; however, further investigations are needed.
Left bundle branch area pacing versus biventricular pacing for cardiac resynchronization therapy
Several clinical trials have explored whether LBBAP is equal or superior to conventional CRT (15–17, 60, 61). In these studies, LBBAP/LBBP produced a narrower paced QRSd than did BVP as expected. Accordingly, LBBAP/LBBP resulted in an increased LVEF and improved NYHA class. LBBAP improved the LVEF more than BVP in this study (16). By contrast, LBBAP/LBBP was equivalent to BVP in other studies (15, 17, 61). LBBP, HBP, and BVP were compared in 137 non-randomized patients with an LVEF ≤ 40% and typical LBBB (60). Finally, HBP and LBBP delivered similar improvement in the LVEF and NYHA class after the 1-year follow-up, which was significantly higher than that in BVP. Furthermore, some meta-analyses of LBBAP for CRT have been reported (62, 63). A meta-analysis compared LBBAP and BVP for CRT (62). Compared with BVP, LBBAP produced significantly narrower QRSd with a mean difference (MD) 29.18 ms, LVEF improvement of 6.93%, LVEDD reduction of 2.96 mm, and NYHA class improvement of 0.54. Similarly, a network meta-analysis compared LBBAP, HBP, and BVP for patients requiring CRT (63). Compared with BVP, LBBAP produced greater LVEF improvement with an MD of 7.17%, followed by an HBP of 4.06%. In addition, HBP produced a narrower QRSd with an MD of 31.58 ms, followed by an LBBAP of 27.40 ms. There were no differences in LVEF improvement and QRS narrowing for LBBAP versus HBP. These data indicated that LBBAP, comparable with HBP, may be superior to BVP, but further evaluations are needed.
Left bundle branch area pacing-optimized cardiac resynchronization therapy
To our knowledge, proximal LBB pacing is inherently limited by its inability to restore physiological activation of the lateral wall of the LV in patients with a distal conduction delay (19). Thus, it may not always be optimal for QRS narrowing by LBBAP alone. Whether LBBAP-optimized CRT (LOT-CRT), LBBAP combined with CS LV pacing, would be advantageous over LBBAP or BVP is unknown. Thus, the LOT-CRT was assessed in an international multicenter study of non-consecutive patients who were indicated for CRT or non-responders (64). LOT-CRT was successful in 91 of 112 patients (81%). The average follow-up was 7.8 ± 2.3 months. LOT-CRT generated significantly greater narrowing of QRSd to 144 ± 22 ms (vs. baseline, BVP, and LBBAP, P < 0.0001), increased LVEF (28.5 ± 9.9% to 37.2 ± 12%, P < 0.0001), and decreased LVEDD (62.0 ± 8.9 to 59.1 ± 9.1 mm, P < 0.0442) and N-terminal pro-hormone B-type natriuretic peptide (5,668 ± 8,249 to 2,561 ± 3,555 pg/mL, P < 0.0001). These results indicated that LOT-CRT provided greater electrical resynchronization and clinical benefits than BVP or LBBAP alone, but further research is needed to confirm this finding.
Limits of left bundle branch area pacing
Multiple studies demonstrated the technical advantages and clinical potential of LBBAP. It has comparable LV synchrony with HBP but a high success rate of 81.1–97% (15, 16, 19, 56, 65) and a low lead-related complication rate of 1.5% (65) with the SelectSecure 3830 lead (Medtronic, Minneapolis, MN, United States). Currently, other stylet-driven conventional active fixation pacing leads can also effectively obtain LBBAP, such as the Solia S60 lead (Biotronik, SE & Co., KG, Germany) (66–68), the Ingevity pacing lead (Boston Scientific Inc., Marlborough, MA, United States) (68), and the Tendril 2088TC lead (Abbott, Inc., United States) (68, 69). In addition, LBBAP has a lower and stable threshold and high perception, and it is preferred for patients with a block far beyond the His bundle branch. Furthermore, the broad and expansive nature of LBB makes LBBAP implantation simpler and faster than that of HBP (70). However, some issues should still be noted, including its acute and long-term safety. Several complications may occur during the procedure, such as LV perforation as the lead advances into the deep interventricular septum (IVS) (71). Thus, a pre-procedural IVS thickness evaluation would be safer. Furthermore, the lead should be rapidly rotated until it penetrated deep into IVS, and fluoroscopic image and pacing parameters and morphologies should be monitored to avoid the perforation of IVS during the process (13, 19). In addition, the safety of postoperative lead extraction after a long duration has been the focus of much attention. Chen et al. (72) reported that three of 612 patients repositioned the lead during the follow-up (one postoperative septum perforation and one postoperative lead dislodgement at 1 month, and one postoperative lead dislodgement at 1 month after repositioned for 5 months). These leads were extracted and repositioned at different sites, and the parameters were stable at an additional 1-year follow-up. Similarly, Su et al. (57) reported a septal perforation during the follow-up in one patient, and the lead was removed and reimplanted without serious complications. Collectively, further research is needed to firmly establish the safety of LBBAP for CRT, particularly for lead extraction over a long duration after the procedure.
Leadless left ventricular pacing: New direction for patients after coronary sinus approach failure
Despite the well-demonstrated effects of HBP and LBBAP, transvenous leads and typical pocket infections remain a non-negligible limitation (18, 19). Leadless cardiac pacing has been proposed to address these complications. The WiSE-CRT system (EBR Systems Inc., Sunnyvale, CA, United States) is the only currently available leadless LV pacing system that comprises a subcutaneous pulse generator transmitter and LV endocardial receiver electrode (73). In this system, acoustic energy is converted from the pulse generator transmitter, located subcutaneously at the fourth, fifth, or sixth intercostal space, to electrical stimulation of a receiver electrode implanted into the LV cavity. The system works in conjunction with a co-implant of RV pacing, which could be a conventional device such as a pacemaker or implantable cardioverter defibrillator (ICD) or a leadless pacemaker such as Micra (Medtronic, Minneapolis, MN, United States). Biventricular pacing is accomplished by perceiving the RV pacing output of the co-implant, followed by the system immediately transducing acoustic energy to electrical stimulation of the LV electrode, thus achieving near-synchronous RV and LV pacing.
Evidence for leadless pacing
In 2014, the Wireless Stimulation Endocardially for CRT (WiSE-CRT) study (20) included 17 HF patients, two-thirds of whom showed ≥ 1 NYHA class improvement at the 6-month follow-up. The Safety and Performance of Electrodes implanted in the Left Ventricle (SELECT-LV), a prospective multicenter non-randomized trial, enrolled 35 CRT-indicated patients who “failed” conventional CRT and underwent implantation of leadless pacing (21). The procedure was successfully performed in 34 (97.1%) patients. Of them, 84.8% (N = 28) showed an improved clinical composite score and 66% (N = 21) gained a ≥ 5% absolute increase in the LVEF at 6 months. Of note, serious procedure/device-related complications were observed in 8.6% of patients (N = 3) within 24 h and 22.9% of patients (N = 8) between 24 h and 1 month. A real-world experience with the WiSE-CRT system was shared in an international trial (ClinicalTrials.gov identifier: NCT02610673) (74) in which procedural success and the delivery of biventricular endo-pacing occurred in 85 of 90 patients (94.4%). The acute (within 24 h), 1- to 30-day, and 1- to 6-month complication rates were 4.4% (N = 4), 18.8% (N = 17), and 6.7% (N = 6), respectively. A total of five deaths (5.6%) occurred within 6 months. HF symptoms improved in 70% of patients. Subsequently, the Stimulation of the Left Ventricular Endocardium for Cardiac Resynchronization Therapy (SOLVE-CRT) trial assessed the short-term outcomes of the WiSE-CRT system in cases without prior implant experience (75). WiSE-CRT was successful in all 31 patients. Of them, 30 completed the 6-month follow-up. In total, 14 (46.7%) patients achieved ≥ 1 NYHA class improvements and an improved LVEF, decreased LVESV, and increased LV end-diastolic volume (LVEDV); three (9.7%) device-related complications occurred: insufficient LV pacing (N = 1), embolization of an unanchored LV electrode (N = 1), and skin infection (N = 1). These results indicated that biventricular endo-pacing from the WiSE-CRT system was effective in cases of failed conventional CRT or non-response, but complications must be noted.
Totally leadless cardiac resynchronization therapy
The aforementioned trials of leadless LV endocardial pacing were combined with a traditional pacemaker or ICD instead of a totally leadless CRT. The successful coexistence of Micra and the WISE-CRT system was first reported in 2019 (76). Also, two other cases were published in the same year or later (77, 78). The patients in these case reports have a common characteristic, that is, they have a complex history including old age, infection, valvular replacement surgery, or venous occlusion. These patients achieved a narrower QRSd and satisfactory clinical outcomes without serious complications after leadless CRT. These cases raised the possibility of completely leadless CRT. Subsequently, multiple European centers shared their experiences with totally leadless CRT (79). A total of eight patients from six centers underwent combination treatment with Micra and WiSE-CRT systems. The QRSd reduction immediately after WiSE-CRT implantation was significant (204.38 ± 30.26, 137.5 ± 24.75 ms, P = 0.012), and it was maintained at the 6-month follow-up. Only a significant improvement in the LVEF was achieved after WiSE-CRT implantation (28.43 ± 8.01% vs. 39.71 ± 11.89%, P = 0.018) without evidence of LV reverse remodeling and improved NYHA class. A current meta-analysis of leadless LV pacing for CRT (80) included five studies (four with RV leads of conventional devices and one with Micra) involving 181 total patients in the final analysis. The success rate of the procedure was 90.6%. It generated a mean increase in the LVEF with an MD of 6.3% and NYHA class improvement of 0.43. Notably, the procedure-related complications and mortality rates were 23.8% and 2.8%, respectively. However, this new pacing modality was used in only a small number of patients, and further studies are needed to confirm its feasibility and safety.
Limits of leadless left ventricular pacing
Taken together, these data support the efficacy of leadless LV pacing as an alternative in patients in whom CRT is impossible or ineffective. It significantly reduces diaphragm stimulation, avoids mitral regurgitation, and can be performed at multiple physiological pacing positions. In addition, the receiver electrode was completely endothelialized for approximately 4 weeks; therefore, long-term anticoagulation was not required (74). However, leadless LV pacing has several limitations. First, it is challenging to choose a suitable acoustic window (distance < 10 cm and angulation < 30°) to effectively transmit ultrasound. Second, some regions of the left lateral free wall of the enlarged LV may be difficult to reach owing to the current delivery sheath. Third, the battery life projections averaged 18 months (range, 9–42 months) (20), which is often overestimated and should be improved. Moreover, the procedure is complex and has a relatively high complication rate. However, security issues are a common problem in the early stages of any novel technique. Improvements in the safety profile, such as different delivery sheaths, increased operator experience, and practice modifications, would reduce its complication rates and increase its widespread use. Additionally, pre-procedural cardiac computed tomography can be used to identify the optimal positioning of the receiver electrode based on indicators such as scar burden, simulated latest activation (81), and hemodynamic assessment (82).
Conclusion
In summary, HBP, LBBAP, and leadless LV pacing have been demonstrated as potential alternatives for optimal CRT when conventional CRT fails. Each technique has its advantages and disadvantages (Table 1). HBP and LBBAP have shown more effective electrical resynchronization than conventional BVP. Accordingly, they provided equivalent or even superior clinical outcomes in some challenging cohorts. However, transvenous leads remain a major limitation of these pacing modalities. Thus, leadless LV pacing has been developed and demonstrated to provide more physiological LV endocardial activation coupled with clinical benefits. Furthermore, the advantage of leadless LV pacing would become more pronounced in cases of venous occlusion or lead infection. With a better understanding of HBP, LBBAP, leadless LV pacing, and their appropriate candidates, it is more likely that the most suitable alternative will be chosen when conventional CRT is impossible or ineffective.
Author contributions
JH conducted the reference analysis and wrote the manuscript. QK contributed to the reference collection and manuscript revision. QC contributed to the topic conception, manuscript revision, and decided to submit for publication. All authors contributed to the article and approved the submitted version.
Funding
This study was supported by the National Natural Science Foundation of China (grant no. 81360025).
Conflict of interest
The authors declare that the research was conducted in the absence of any commercial or financial relationships that could be construed as a potential conflict of interest.
Publisher’s note
All claims expressed in this article are solely those of the authors and do not necessarily represent those of their affiliated organizations, or those of the publisher, the editors and the reviewers. Any product that may be evaluated in this article, or claim that may be made by its manufacturer, is not guaranteed or endorsed by the publisher.
References
1. Tomasoni D, Adamo M, Lombardi CM, Metra M. Highlights in heart failure. ESC Heart Fail. (2019) 6:1105–27. doi: 10.1002/ehf2.12555
2. McDonagh TA, Metra M, Adamo M, Gardner RS, Baumbach A, Böhm M, et al. 2021 ESC guidelines for the diagnosis and treatment of acute and chronic heart failure. Eur Heart J. (2021) 42:3599–726.
3. St John Sutton MG, Plappert T, Abraham WT, Smith AL, DeLurgio DB, Leon AR, et al. Effect of cardiac resynchronization therapy on left ventricular size and function in chronic heart failure. Circulation. (2003) 107:1985–90. doi: 10.1161/01.CIR.0000065226.24159.E9
4. Glikson M, Nielsen JC, Kronborg MB, Michowitz Y, Auricchio A, Barbash IM, et al. 2021 ESC guidelines on cardiac pacing and cardiac resynchronization therapy. Eur Heart J. (2021) 42:3427–520. doi: 10.1093/eurheartj/ehab699
5. Chung ES, Leon AR, Tavazzi L, Sun JP, Nihoyannopoulos P, Merlino J, et al. Results of the predictors of response to CRT (PROSPECT) trial. Circulation. (2008) 117:2608–16. doi: 10.1161/CIRCULATIONAHA.107.743120
6. Prinzen FW, Vernooy K, Auricchio A. Cardiac resynchronization therapy: state-of-the-art of current applications, guidelines, ongoing trials, and areas of controversy. Circulation. (2013) 128:2407–18. doi: 10.1161/CIRCULATIONAHA.112.000112
7. Daubert C, Behar N, Martins RP, Mabo P, Leclercq C. Avoiding non-responders to cardiac resynchronization therapy: a practical guide. Eur Heart J. (2017) 38:1463–72.
8. Auricchio A, Prinzen FW. Non-responders to cardiac resynchronization therapy: the magnitude of the problem and the issues. Circ J. (2011) 75:521–7. doi: 10.1253/circj.CJ-10-1268
9. Vijayaraman P, Chung MK, Dandamudi G, Upadhyay GA, Krishnan K, Crossley G, et al. His bundle pacing. J Am Coll Cardiol. (2018) 72:927–47. doi: 10.1016/j.jacc.2018.06.017
10. Lustgarten DL, Crespo EM, Arkhipova-Jenkins I, Lobel R, Winget J, Koehler J, et al. His-bundle pacing versus biventricular pacing in cardiac resynchronization therapy patients: a crossover design comparison. Heart Rhythm. (2015) 12:1548–57. doi: 10.1016/j.hrthm.2015.03.048
11. Subzposh FA, Vijayaraman P. Long-term results of his bundle pacing. Card Electrophysiol Clin. (2018) 10:537–42.
12. Zanon F, Ellenbogen KA, Dandamudi G, Sharma PS, Huang W, Lustgarten DL, et al. Permanent his-bundle pacing: a systematic literature review and meta-analysis. Europace. (2018) 20:1819–26. doi: 10.1093/europace/euy058
13. Huang W, Su L, Wu S, Xu L, Xiao F, Zhou X, et al. A novel pacing strategy with low and stable output: pacing the left bundle branch immediately beyond the conduction block. Can J Cardiol. (2017) 33:1736. doi: 10.1016/j.cjca.2017.09.013
14. Zhang W, Huang J, Qi Y, Wang F, Guo L, Shi X, et al. Cardiac resynchronization therapy by left bundle branch area pacing in patients with heart failure and left bundle branch block. Heart Rhythm. (2019) 16:1783–90. doi: 10.1016/j.hrthm.2019.09.006
15. Guo J, Li L, Xiao G, Ye T, Huang X, Meng F, et al. Remarkable response to cardiac resynchronization therapy via left bundle branch pacing in patients with true left bundle branch block. Clin Cardiol. (2020) 43:1460–8. doi: 10.1002/clc.23462
16. Li X, Qiu C, Xie R, Ma W, Wang Z, Li H, et al. Left bundle branch area pacing delivery of cardiac resynchronization therapy and comparison with biventricular pacing. ESC Heart Fail. (2020) 7:1711–22. doi: 10.1002/ehf2.12731
17. Wang Y, Gu K, Qian Z, Hou X, Chen X, Qiu Y, et al. The efficacy of left bundle branch area pacing compared with biventricular pacing in patients with heart failure: a matched case-control study. J Cardiovasc Electrophysiol. (2020) 31:2068–77. doi: 10.1111/jce.14628
18. Huang W, Su L, Wu S, Xu L, Xiao F, Zhou X, et al. Long-term outcomes of his bundle pacing in patients with heart failure with left bundle branch block. Heart. (2019) 105:137–43. doi: 10.1136/heartjnl-2018-313415
19. Vijayaraman P, Ponnusamy S, Cano Ó, Sharma PS, Naperkowski A, Subsposh FA, et al. Left bundle branch area pacing for cardiac resynchronization therapy: results from the international LBBAP collaborative study group. JACC Clin Electrophysiol. (2021) 7:135–47. doi: 10.1016/j.jacep.2020.08.015
20. Auricchio A, Delnoy PP, Butter C, Brachmann J, Van Erven L, Spitzer S, et al. Feasibility, safety, and short-term outcome of leadless ultrasound-based endocardial left ventricular resynchronization in heart failure patients: results of the wireless stimulation endocardially for CRT (WiSE-CRT) study. Europace. (2014) 16:681–8. doi: 10.1093/europace/eut435
21. Reddy VY, Miller MA, Neuzil P, Søgaard P, Butter C, Seifert M, et al. Cardiac resynchronization therapy with wireless left ventricular endocardial pacing: the SELECT-LV study. J Am Coll Cardiol. (2017) 69:2119–29. doi: 10.1016/j.jacc.2017.02.059
22. Cazeau S, Leclercq C, Lavergne T, Walker S, Varma C, Linde C, et al. Effects of multisite biventricular pacing in patients with heart failure and intraventricular conduction delay. N Engl J Med. (2001) 344:873–80. doi: 10.1056/NEJM200103223441202
23. Abraham WT, Fisher WG, Smith AL, Delurgio DB, Leon AR, Loh E, et al. Cardiac resynchronization in chronic heart failure. N Engl J Med. (2002) 346:1845–53. doi: 10.1056/NEJMoa013168
24. Cleland JG, Daubert JC, Erdmann E, Freemantle N, Gras D, Kappenberger L, et al. The effect of cardiac resynchronization on morbidity and mortality in heart failure. N Engl J Med. (2005) 352:1539–49. doi: 10.1056/NEJMoa050496
25. Daubert C, Gold MR, Abraham WT, Ghio S, Hassager C, Goode G, et al. Prevention of disease progression by cardiac resynchronization therapy in patients with asymptomatic or mildly symptomatic left ventricular dysfunction: insights from the European cohort of the REVERSE (resynchronization reverses remodeling in systolic left ventricular dysfunction) trial. J Am Coll Cardiol. (2009) 54:1837–46.
26. Moss AJ, Hall WJ, Cannom DS, Klein H, Brown MW, Daubert JP, et al. Cardiac-resynchronization therapy for the prevention of heart-failure events. N Engl J Med. (2009) 361:1329–38. doi: 10.1056/NEJMoa0906431
27. Birnie DH, Ha A, Higginson L, Sidhu K, Green M, Philippon F, et al. Impact of QRS morphology and duration on outcomes after cardiac resynchronization therapy: results from the resynchronization-defibrillation for ambulatory heart failure trial (RAFT). Circ Heart Fail. (2013) 6:1190–8. doi: 10.1161/CIRCHEARTFAILURE.113.000380
28. Singh JP, Klein HU, Huang DT, Reek S, Kuniss M, Quesada A, et al. Left ventricular lead position and clinical outcome in the multicenter automatic defibrillator implantation trial-cardiac resynchronization therapy (MADIT-CRT) trial. Circulation. (2011) 123:1159–66. doi: 10.1161/CIRCULATIONAHA.110.000646
29. Martin DO, Lemke B, Birnie D, Krum H, Lee KL, Aonuma K, et al. Investigation of a novel algorithm for synchronized left-ventricular pacing and ambulatory optimization of cardiac resynchronization therapy: results of the adaptive CRT trial. Heart Rhythm. (2012) 9:1807–14. doi: 10.1016/j.hrthm.2012.07.009
30. Wang J, Liang Y, Chen H, Wang W, Bai J, Chen X, et al. Patient-tailored SyncAV algorithm: a novel strategy to improve synchrony and acute hemodynamic response in heart failure patients treated by cardiac resynchronization therapy. J Cardiovasc Electrophysiol. (2020) 31:512–20. doi: 10.1111/jce.14315
31. Forleo GB, Santini L, Giammaria M, Potenza D, Curnis A, Calabrese V, et al. Multipoint pacing via a quadripolar left-ventricular lead: preliminary results from the Italian registry on multipoint left-ventricular pacing in cardiac resynchronization therapy (IRON-MPP). Europace. (2017) 19:1170–7. doi: 10.1093/europace/euw094
32. van Dijk VF, Fanggiday J, Balt JC, Wijffels M, Daeter EJ, Kelder JC, et al. Effects of epicardial versus transvenous left ventricular lead placement on left ventricular function and cardiac perfusion in cardiac resynchronization therapy: a randomized clinical trial. J Cardiovasc Electrophysiol. (2017) 28:917–23. doi: 10.1111/jce.13242
33. Gamble JHP, Herring N, Ginks MR, Rajappan K, Bashir Y, Betts TR. Endocardial left ventricular pacing across the interventricular septum for cardiac resynchronization therapy: clinical results of a pilot study. Heart Rhythm. (2018) 15:1017–22. doi: 10.1016/j.hrthm.2018.02.032
34. Vijayaraman P, Dandamudi G, Zanon F, Sharma PS, Tung R, Huang W, et al. Permanent his bundle pacing: recommendations from a multicenter his bundle pacing collaborative working group for standardization of definitions, implant measurements, and follow-up. Heart Rhythm. (2018) 15:460–8.
35. Vijayaraman P, Dandamudi G, Bauch T, Ellenbogen KA. Imaging evaluation of implantation site of permanent direct his bundle pacing lead. Heart Rhythm. (2014) 11:529–30. doi: 10.1016/j.hrthm.2013.01.027
36. Keene D, Arnold A, Shun-Shin MJ, Howard JP, Sohaib SA, Moore P, et al. Rationale and design of the randomized multicentre his optimized pacing evaluated for heart failure (HOPE-HF) trial. ESC Heart Fail. (2018) 5:965–76. doi: 10.1002/ehf2.12315
37. Zhang J, Guo J, Hou X, Wang Y, Qian Z, Li K, et al. Comparison of the effects of selective and non-selective his bundle pacing on cardiac electrical and mechanical synchrony. Europace. (2018) 20:1010–7. doi: 10.1093/europace/eux120
38. Upadhyay GA, Tung R. Selective versus non-selective his bundle pacing for cardiac resynchronization therapy. J Electrocardiol. (2017) 50:191–4. doi: 10.1016/j.jelectrocard.2016.10.003
39. Deshmukh P, Casavant DA, Romanyshyn M, Anderson K. Permanent, direct his-bundle pacing: a novel approach to cardiac pacing in patients with normal his-purkinje activation. Circulation. (2000) 101:869–77. doi: 10.1161/01.CIR.101.8.869
40. Barba-Pichardo R, Manovel Sánchez A, Fernández-Gómez JM, Moriña-Vázquez P, Venegas-Gamero J, Herrera-Carranza M. Ventricular resynchronization therapy by direct his-bundle pacing using an internal cardioverter defibrillator. Europace. (2013) 15:83–8. doi: 10.1093/europace/eus228
41. Sharma PS, Dandamudi G, Herweg B, Wilson D, Singh R, Naperkowski A, et al. Permanent his-bundle pacing as an alternative to biventricular pacing for cardiac resynchronization therapy: a multicenter experience. Heart Rhythm. (2018) 15:413–20. doi: 10.1016/j.hrthm.2017.10.014
42. Arnold AD, Shun-Shin MJ, Keene D, Howard JP, Sohaib SMA, Wright IJ, et al. His resynchronization versus biventricular pacing in patients with heart failure and left bundle branch block. J Am Coll Cardiol. (2018) 72:3112–22. doi: 10.1016/j.jacc.2018.09.073
43. Upadhyay GA, Vijayaraman P, Nayak HM, Verma N, Dandamudi G, Sharma PS, et al. On-treatment comparison between corrective His bundle pacing and biventricular pacing for cardiac resynchronization: a secondary analysis of the His-SYNC Pilot Trial. Heart Rhythm. (2019) 16:1797–807. doi: 10.1016/j.hrthm.2019.05.009
44. Vinther M, Risum N, Svendsen JH, Møgelvang R, Philbert BTA. Randomized trial of his pacing versus biventricular pacing in symptomatic hf patients with left bundle branch block (his-alternative). JACC Clin Electrophysiol. (2021) 7:1422–32. doi: 10.1016/j.jacep.2021.04.003
45. Vijayaraman P, Herweg B, Ellenbogen KA, Gajek J. His-optimized cardiac resynchronization therapy to maximize electrical resynchronization: a feasibility study. Circ Arrhythm Electrophysiol. (2019) 12:e006934. doi: 10.1161/CIRCEP.118.006934
46. Deshmukh A, Sattur S, Bechtol T, Heckman LIB, Prinzen FW, Deshmukh P. Sequential his bundle and left ventricular pacing for cardiac resynchronization. J Cardiovasc Electrophysiol. (2020) 31:2448–54. doi: 10.1111/jce.14674
47. Padeletti L, Pieragnoli P, Ricciardi G, Innocenti L, Checchi L, Padeletti M, et al. Simultaneous his bundle and left ventricular pacing for optimal cardiac resynchronization therapy delivery: acute hemodynamic assessment by pressure-volume loops. Circ Arrhythm Electrophysiol. (2016) 9:5. doi: 10.1161/CIRCEP.115.003793
48. Senes J, Mascia G, Bottoni N, Oddone D, Donateo P, Grimaldi T, et al. Is his-optimized superior to conventional cardiac resynchronization therapy in improving heart failure? Results from a propensity-matched study. Pacing Clin Electrophysiol. (2021) 44:1532–9. doi: 10.1111/pace.14336
49. Padala SK, Cabrera JA, Ellenbogen KA. Anatomy of the cardiac conduction system. Pacing Clin Electrophysiol. (2021) 44:15–25. doi: 10.1111/pace.14107
50. Qi J, Jia X, Wang Z. His bundle pacing for cardiac resynchronization therapy: a systematic literature review and meta-analysis. J Interv Card Electrophysiol. (2020) 59:463–70. doi: 10.1007/s10840-020-00827-6
51. Upadhyay GA, Cherian T, Shatz DY, Beaser AD, Aziz Z, Ozcan C, et al. Intracardiac delineation of septal conduction in left bundle-branch block patterns. Circulation. (2019) 139:1876–88. doi: 10.1161/CIRCULATIONAHA.118.038648
52. Chen X, Wu S, Su L, Su Y, Huang W. The characteristics of the electrocardiogram and the intracardiac electrogram in left bundle branch pacing. J Cardiovasc Electrophysiol. (2019) 30:1096–101. doi: 10.1111/jce.13956
53. Wu S, Chen X, Wang S, Xu L, Xiao F, Huang Z, et al. Evaluation of the criteria to distinguish left bundle branch pacing from left ventricular septal pacing. JACC Clin Electrophysiol. (2021) 7:1166–77. doi: 10.1016/j.jacep.2021.02.018
54. Gao MY, Tian Y, Shi L, Wang YJ, Xie BQ, Qi J, et al. Electrocardiographic morphology during left bundle branch area pacing: characteristics, underlying mechanisms, and clinical implications. Pacing Clin Electrophysiol. (2020) 43:297–307. doi: 10.1111/pace.13884
55. Hou X, Qian Z, Wang Y, Qiu Y, Chen X, Jiang H, et al. Feasibility and cardiac synchrony of permanent left bundle branch pacing through the interventricular septum. Europace. (2019) 21:1694–702. doi: 10.1093/europace/euz188
56. Huang W, Wu S, Vijayaraman P, Su L, Chen X, Cai B, et al. Cardiac resynchronization therapy in patients with nonischemic cardiomyopathy using left bundle branch pacing. JACC Clin Electrophysiol. (2020) 6:849–58. doi: 10.1016/j.jacep.2020.04.011
57. Su L, Wang S, Wu S, Xu L, Huang Z, Chen X, et al. Long-term safety and feasibility of left bundle branch pacing in a large single-center study. Circ Arrhythm Electrophysiol. (2021) 14:e009261. doi: 10.1161/CIRCEP.120.009261
58. Zhong C, Xu W, Shi S, Zhou X, Zhu Z. Left bundle branch pacing for cardiac resynchronization therapy: a systematic literature review and meta-analysis. Pacing Clin Electrophysiol. (2021) 44:497–505. doi: 10.1111/pace.14174
59. Salden F, Luermans J, Westra SW, Weijs B, Engels EB, Heckman LIB, et al. Short-term hemodynamic and electrophysiological effects of cardiac resynchronization by left ventricular septal pacing. J Am Coll Cardiol. (2020) 75:347–59.
60. Wu S, Su L, Vijayaraman P, Zheng R, Cai M, Xu L, et al. Left bundle branch pacing for cardiac resynchronization therapy: nonrandomized on-treatment comparison with his bundle pacing and biventricular pacing. Can J Cardiol. (2021) 37:319–28.
61. Hua J, Chen Y, Yu J, Xiong Q, Xia Z, Xia Z, et al. Long-term outcomes of left bundle branch area pacing versus biventricular pacing in patients with heart failure and complete left bundle branch block. Heart Vessels. (2022) 37:1162–74. doi: 10.1007/s00380-021-02016-5
62. Liu J, Sun F, Wang Z, Sun J, Jiang X, Zhao W, et al. Left bundle branch area pacing vs. biventricular pacing for cardiac resynchronization therapy: a meta-analysis. Front Cardiovasc Med. (2021) 8:669301. doi: 10.3389/fcvm.2021.669301
63. Hua J, Wang C, Kong Q, Zhang Y, Wang Q, Xiong Z, et al. Comparative effects of left bundle branch area pacing, his bundle pacing, biventricular pacing in patients requiring cardiac resynchronization therapy: a network meta-analysis. Clin Cardiol. (2022) 45:214–23. doi: 10.1002/clc.23784
64. Jastrzêbski M, Moskal P, Huybrechts W, Curila K, Sreekumar P, Rademakers LM, et al. Left bundle branch-optimized cardiac resynchronization therapy (LOT-CRT): results from an international LBBAP collaborative study group. Heart Rhythm. (2022) 19:13–21. doi: 10.1016/j.hrthm.2021.07.057
65. Vijayaraman P, Herweg B, Verma A, Sharma PS, Batul SA, Ponnusamy SS, et al. Rescue left bundle branch area pacing in coronary venous lead failure or nonresponse to biventricular pacing: results from International LBBAP collaborative study group. Heart Rhythm. (2022) 19:1272–80. doi: 10.1016/j.hrthm.2022.04.024
66. De Pooter J, Calle S, Timmermans F, Van Heuverswyn F. Left bundle branch area pacing using stylet-driven pacing leads with a new delivery sheath: a comparison with lumen-less leads. J Cardiovasc Electrophysiol. (2021) 32:439–48. doi: 10.1111/jce.14851
67. Zanon F, Marcantoni L, Pastore G, Baracca E. Left bundle branch pacing by standard stylet-driven lead: preliminary experience of two case reports. HeartRhythm Case Rep. (2020) 6:614–7. doi: 10.1016/j.hrcr.2020.06.005
68. De Pooter J, Wauters A, Van Heuverswyn F, Le Polain de Waroux JB. A Guide to left bundle branch area pacing using stylet-driven pacing leads. Front Cardiovasc Med. (2022) 9:844152. doi: 10.3389/fcvm.2022.844152
69. Daniëls F, Adiyaman A, Aarnink KM, Oosterwerff FJ, Verbakel JRA, Ghani A, et al. The zwolle experience with left bundle branch area pacing using stylet-driven active fixation leads. Clin Res Cardiol. (2022) 2022:1–10. doi: 10.1007/s00392-022-02048-5
70. Anderson RH, Yanni J, Boyett MR, Chandler NJ, Dobrzynski H. The anatomy of the cardiac conduction system. Clin Anat. (2009) 22:99–113. doi: 10.1002/ca.20700
71. Li X, Li H, Ma W, Ning X, Liang E, Pang K, et al. Permanent left bundle branch area pacing for atrioventricular block: feasibility, safety, and acute effect. Heart Rhythm. (2019) 16:1766–73. doi: 10.1016/j.hrthm.2019.04.043
72. Chen X, Wei L, Bai J, Wang W, Qin S, Wang J, et al. Procedure-related complications of left bundle branch pacing: a single-center experience. Front Cardiovasc Med. (2021) 8:645947. doi: 10.3389/fcvm.2021.645947
73. Sieniewicz BJ, Gould JS, Rimington HM, Ioannou N, Rinaldi CA. transseptal delivery of a leadless left ventricular endocardial pacing electrode. JACC Clin Electrophysiol. (2017) 3:1333–5. doi: 10.1016/j.jacep.2017.04.020
74. Sieniewicz BJ, Betts TR, James S, Turley A, Butter C, Seifert M, et al. Real-world experience of leadless left ventricular endocardial cardiac resynchronization therapy: a multicenter international registry of the WiSE-CRT pacing system. Heart Rhythm. (2020) 17:1291–7. doi: 10.1016/j.hrthm.2020.03.002
75. Okabe T, Hummel JD, Bank AJ, Niazi IK, McGrew FA, Kindsvater S, et al. Leadless left ventricular stimulation with WiSE-CRT system - initial experience and results from phase I of SOLVE-CRT study (nonrandomized, roll-in phase). Heart Rhythm. (2022) 19:22–9. doi: 10.1016/j.hrthm.2021.06.1195
76. Montemerlo E, Pozzi M, De Ceglia S, Santini F, Piazzi E, Rovaris G. First-in-man fully leadless transvenous CRT-P with a transseptal implant of WISE-CRT(§) system and micra(§) PM. Pacing Clin Electrophysiol. (2019) 42:1489–92. doi: 10.1111/pace.13750
77. Funasako M, Neuzil P, Dujka L, Petru J, Sediva L, Simon J, et al. Successful implementation of a totally leadless biventricular pacing approach. HeartRhythm Case Rep. (2020) 6:153–7. doi: 10.1016/j.hrcr.2019.12.002
78. Galand V, Polin B, Martins RP, Leclercq C. An entirely leadless cardiac resynchronization therapy. Eur Heart J. (2019) 40:858–9. doi: 10.1093/eurheartj/ehy746
79. Carabelli A, Jabeur M, Jacon P, Rinaldi CA, Leclercq C, Rovaris G, et al. European experience with a first totally leadless cardiac resynchronization therapy pacemaker system. Europace. (2021) 23:740–7.
80. Wijesuriya N, Elliott MK, Mehta V, Sidhu BS, Behar JM, Niederer S, et al. Leadless left ventricular endocardial pacing for cardiac resynchronization therapy: a systematic review and meta-analysis. Heart Rhythm. (2022) 19:1176–83.
81. Sidhu BS, Lee AWC, Haberland U, Rajani R, Niederer S, Rinaldi CA. Combined computed tomographic perfusion and mechanics with predicted activation pattern can successfully guide implantation of a wireless endocardial pacing system. Europace. (2020) 22:298. doi: 10.1093/europace/euz227
Keywords: cardiac resynchronization therapy, biventricular pacing, His bundle pacing, left bundle branch area pacing, leadless LV pacing, review
Citation: Hua J, Kong Q and Chen Q (2022) Alternative pacing strategies for optimal cardiac resynchronization therapy. Front. Cardiovasc. Med. 9:923394. doi: 10.3389/fcvm.2022.923394
Received: 19 April 2022; Accepted: 06 September 2022;
Published: 27 September 2022.
Edited by:
Lina Marcantoni, Hospital Santa Maria della Misericordia of Rovigo, ItalyReviewed by:
Alwin Zweerink, Amsterdam UMC, NetherlandsYongquan Wu, Capital Medical University, China
Francesco Notaristefano, Hospital of Santa Maria della Misericordia in Perugia, Italy
Copyright © 2022 Hua, Kong and Chen. This is an open-access article distributed under the terms of the Creative Commons Attribution License (CC BY). The use, distribution or reproduction in other forums is permitted, provided the original author(s) and the copyright owner(s) are credited and that the original publication in this journal is cited, in accordance with accepted academic practice. No use, distribution or reproduction is permitted which does not comply with these terms.
*Correspondence: Qi Chen, efycq@189.cn