Mechanical circulatory support devices and treatment strategies for right heart failure
- 1Department of Biomedical Engineering, Lerner Research Institute, Cleveland Clinic, Cleveland, OH, United States
- 2Department of Biomedical Engineering, Cleveland Clinic Lerner College of Medicine of Case Western Reserve University, Cleveland, OH, United States
The importance of right heart failure (RHF) treatment is magnified over the years due to the increased risk of mortality. Additionally, the multifactorial origin and pathophysiological mechanisms of RHF render this clinical condition and the choices for appropriate therapeutic target strategies remain to be complex. The recent change in the United Network for Organ Sharing (UNOS) allocation criteria of heart transplant may have impacted for the number of left ventricular assist devices (LVADs), but LVADs still have been widely used to treat advanced heart failure, and 4.1 to 7.4% of LVAD patients require a right ventricular assist device (RVAD). In addition, patients admitted with primary left ventricular failure often need right ventricular support. Thus, there is unmet need for temporary or long-term support RVAD implantation exists. In RHF treatment with mechanical circulatory support (MCS) devices, the timing of the intervention and prediction of duration of the support play a major role in successful treatment and outcomes. In this review, we attempt to describe the prevalence and pathophysiological mechanisms of RHF origin, and provide an overview of existing treatment options, strategy and device choices for MCS treatment for RHF.
Introduction
The number of heart failure (HF) patients reached more than 6 million in the U.S. in 2018 and is expected to rise to 8 million by 2030 (1). The overall incidence is increasing in Europe, as well (2–4). The same trend is being observed worldwide (5). Among this population, right HF (RHF) is associated with increased mortality (6, 7). Furthermore, the pathogenesis of RHF varies, and right ventricular (RV) function has a close interactive relationship with left ventricular (LV) function. For example, LV contraction generates approximately 30% of RV contraction energy, since the ventricles share the interventricular septum and pericardium (7, 8); on the other hand, RV dilatation may decrease the LV preload and ventricular elastance by shifting the interventricular septum and distensions of the pericardium. Therefore, the RHF management has been rather cumbersome due to absence of a standardized strategies for RHF particularly with use of MCS devices. Additionally, the absence of the dedicate durable RVAD device, less advanced stage RHF at the time of LVAD, variable rates or RV deterioration post implantation, surgical adaptation of the LVAD for RVAD use, and early stage biventricular failure adds up when it comes to the decision making process for optimal management strategy.
Currently, the indication for mechanical circulatory support (MCS) treatment for RHF is for patients who are refractory to medical or surgical therapy (8), however, the timing and the strategies for MCS treatment differ by the pathogenesis of the RHF and the duration of the support. Additionally, it is important to select MCS device with a solid understanding of the underlying mechanisms of the disease and therefore to determine the most beneficial device performance to achieve optimal degree of ventricular unloading.
Right heart failure pathogenesis
Isolated right ventricle injury pathogenesis
The pathogenesis of RHF can be divided into three major categories: isolated RV injury, pulmonary etiology, and the one secondary to LV failure (6). Additionally, there is the “after cardiac transplantation” condition and congenital heart disease condition; however, due to the complexity of these conditions, we have not included these conditions in this review.
The isolated RV injury pathogenesis, mainly associated with the decreased RV contractility, is one of the ways RHF develops. Some cases of acute inferior myocardial infarction (MI) are representative of isolated RV injury. Acute inferior MI patients with high-grade proximal occlusion of the right coronary artery tend to show severe RV ischemic dysfunction, which may result in higher in-hospital mortality (9). However, many patients show clinical improvements within 3 to 10 days, global RV performance recovers to almost normal levels within 3 to 12 months, and pure secondary unilateral RHF is rare (10, 11). A prospective study (n = 69) documented the long-term mortality among patients with RV MI after the first year was at an additional 2/year to 3%/year through 10th year (12).
Pulmonary pathogenesis
Pulmonary pathogenesis, which caused by increased RV afterload inducing RV pressure overload and hypoxia, is a broad category. The representatives of this pathogenesis are pulmonary hypertension (PH) and acute pulmonary embolism (PE). Regarding PH, the world health organization (WHO) classification for PH and the European Society of Cardiology and European Respiratory Society guidelines for PH may help organize the topic (6, 13). In WHO classification, PH is classified as:
• Group 1: pulmonary arterial hypertension (PAH) (e.g., idiopathic, hereditary);
• Group 2: left heart disease (e.g., HF with preserved ejection fraction, HF with reduced ejection fraction);
• Group 3: lung disease (e.g., chronic obstructive pulmonary disease);
• Group 4: chronic thromboembolic disease (e.g., chronic thromboembolic PH);
• Group 5: miscellaneous (e.g., sarcoidosis, chronic hemolytic disorders).
It should be noted here that the most prevalent group is group 2 (68%), which is the group of PH due to left heart disease, such as systolic and diastolic dysfunction of LV (14). Therefore, strictly speaking, group 2 PH will be classified into left ventricular failure pathogenesis. The next prevalent group is group 5: miscellaneous (15%), Group 3: lung disease (9%), group 1: PAH (3%), and group 4: chronic thromboembolic disease (CTEPH) (2%) follows. A multi-center observational, prospective study (n = 2,635) showed a survival rate among PAH patients, which was 85 ± 1%, 68 ± 1%, 57 ± 1%, and 49 ± 1% at 1, 3, 5, and 7 years from diagnosis, respectively (15). The mortality among Group 2, 3, and 5 varies based on the disease and severity. Among CTEPH patients, a surgical treatment, pulmonary thromboendarterectomy, has shown to improve both short-term and long-term survival. In an international prospective study (n = 679) described that estimated survival at 1, 2, and 3 years was 93% (95% confidence interval [CI], 90–95), 91% (95% CI, 87–93), and 89% (95% CI, 86–92) in operated patients (n = 404), and only 88% (95% CI, 83–91), 79% (95% CI, 74–83), and 70% (95% CI, 64–76) in not-operated patients (n = 275).
Left ventricular failure pathogenesis
As noted earlier, the RV function has a close interactive relationship with the LV function. As a result, the cause of the RHF in this pathogenesis could be decreased RV contractility and/or RV pressure overload. Cases are equally distributed in a wide variety of causes, such as myocarditis, LVAD support, ischemic disease, and mitral/aortic valvular heart disease; however, as long as this pathogenesis is secondary, the MCS for RHF is considered after or while receiving the LV treatment. Also noted earlier, this pathogenesis will include the patients with group 2 PH. Particular attention should be paid to the HF with preserved ejection fraction (HFpEF). The initial step should be made to treat RHF due to HFpEF after or while receiving the LV treatment; however, the treatment for HFpEF is still limited. Several efforts are undergoing in both medical and device-based treatment (16–18). Regarding the survival for HF with reduced ejection fraction (HFrEF), despite of remarkable advance in management of HFrEF, the 5-year survival after hospitalization remains poor (24.7%), and it was similar to HFpEF (24.3%) (19).
Device descriptions
According to Kapur et al., the treatment mechanism of MCS devices for acute RHF can be divided into two categories; direct RV bypass and indirect RV bypass (20). The direct RV bypass devices, such as percutaneous temporal RV assist devices (RVADs), generally have the inflow in the inferior vena cava (IVC) or the right atrium (RA) and pump the blood to pulmonary artery (PA). On the other hand, the indirect RV bypass device, such as the veno-arterial extracorporeal membrane oxygenation (VA-ECMO), delivers blood from the systemic veins and provides oxygenated blood to systemic organs from the femoral artery access. Furthermore, we have extended Kapur's categories to include chronic RV support in which off-label use of the commercially implantable left ventricular assist device (LVAD) is used as RVAD. RVAD in this setup pumps blood through the graft anastomosed to PA. The difference from the previous two bypass groups is the implantability techniques and duration of support. Thus, it may be suitable for the long support duration in selected cases; however, surgical intervention will be needed. In this review, this option has been added as “chronic RV support” to explain the devices for both acute and chronic RHF (Figure 1). Additionally, for convenience of explanation, we included total artificial heart (TAH) in chronic RV support category.
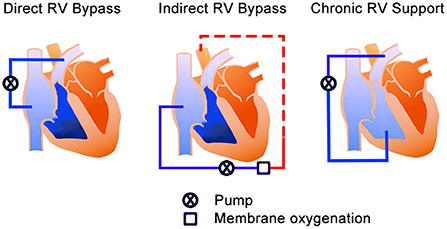
Figure 1. The treatment mechanisms of the MCS device for RHF. The blue color is un-oxygenated blood, and the red color is the oxygenated blood. Left—Direct RV bypass mechanism; takes blood from the systemic veins and pumps it to the pulmonary artery. Middle—Indirect RV bypass mechanism; takes the blood from systemic veins and provides systemic perfusion (red dash line) from the femoral artery. Right—RV support mechanism; takes blood from the RV and pumps it to the pulmonary artery. RV, right ventricle; MCS, mechanical circulatory support; RHF, right heart failure.
Direct RV bypass devices
Impella RP
The Impella RP® (Abiomed, Danvers, MA, USA) is a U.S. FDA-approved, 22 Fr micro axial pump mounted on an 11 Fr catheter (Figure 2A). The pump is designed to provide up to 4.0 L/min at 33,000 rpm and support up to 14 days. The system is delivered via percutaneous femoral vein access, and the optimal pump inflow is designed to be positioned in the inferior vena cava and the pump outflow in the distal main PA, below the bifurcation to the right PA, which falls into the category of a direct RV bypass device. The device may be used in the temporary support duration with isolated RV failure pathogenesis and also secondarily to LV failure pathogenesis as a part of biventricular support.
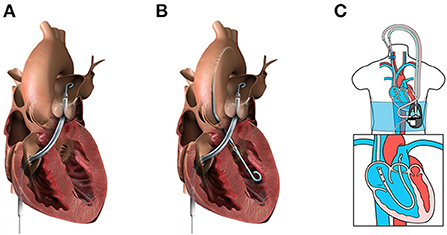
Figure 2. Percutaneous mechanical circulatory support devices for RHF. (A) Impella RP (image from Abiomed media kit, used with permission). (B) BiPella (image from Abiomed media kit, used with permission). (C) LifeSpark pump with ProtekDuo cannula (image from Livanova Investor Day 2021 presentation, used with permission).
The efficacy of the Impella RP was investigated in a prospective study, the RECOVER RIGHT study, in 2015 (21–25). The study consisted of two cohorts among 15 U.S. institutions: patients with RHF within 48 h post-LVAD implant (n = 31) and post-cardiotomy or post-MI patients with RHF (n = 29). The primary endpoint was survival at 30 days, hospital discharge post-device explant, or transition to the subsequent therapy, and was achieved in 73.3% of the study population. The total duration of device support in this study was ~ 3 days. The major adverse events at 30 days were major bleeding (60%) and hemolysis (13%). PA perforation occurred in one patient, which led to hemothorax, and was likely caused by the guidewire used during the device positioning. No PE was reported.
Recently, for severe biventricular support with suboptimal LV unloading using VA-ECMO, using two Impella pumps, known as BiPella (Figure 2B), can substitute V-A ECMO if oxygenation is not required. The BiPella therapy combines the LV Impella® systems (5.0, CP, and 2.0) and Impella RP (26). A retrospective study among five U.S. hospitals (n = 20) reported that in-hospital mortality was 50% (27). In addition, non-survivors had higher PA resistance than survivors, which suggests that non-survivors might not be a biventricular failure but PH following the LV failure. Therefore, there may still be room for improvement in outcomes with attention to the pulmonary vascular load.
LifeSPARC pump with protekduo cannula
LifeSPARC Pump® (LivaNova, Houston, TX, USA), formerly called TandemHeart, is an extracorporeal continuous-flow (CF) centrifugal flow pump with a magnetic pivot bearing (Figure 2C). The pump body priming volume is 16 mL and is designed to provide up to 4.5 L/min through the percutaneous catheter. With the development of the 29/31 Fr ProtekDuo dual-lumen cannula® (LivaNova, Houston, TX, USA), the LifeSPARC Pump is often used with the ProtekDuo cannula percutaneously via the right internal jugular vein (25, 28). The ProtekDuo cannula contains two lumens: one lumen works as inflow and the other as outflow. The delivery technique is similar to the Swan-Ganz catheter insertion. The optimal positioning is the inflow in RA and outflow in main PA. LifeSPARC Pump and ProtekDuo cannula gained approval for support of up to 30 days by the European Medicines Agency and up to 6 days by the U.S. FDA (25).
The outcome of this system is mixed. In a report of 17 patients (12 patients had a durable LVAD in place) who went through the implantation with this system, during the mean length of support of 10.5 ± 6.5 days, 23% of patients were successfully weaned, 35% required conversion to either a surgical temporary extracorporeal RVAD (sRVAD) or durable implantable RVAD, and 41% did not survive (29). Another study reported that 27 LVAD patients received the system implantation, and device weaning occurred in 86% of patients, with 15% in-hospital mortality (30). Other studies also report similar good outcomes (31, 32).
The insertion without touching the groin region will be one of the advantages of this system. Although the duration of the support might have an impact, infection does not appear to be an issue. In addition, the insertion location enables another advantage for rehabilitation. Furthermore, this system is able to add a membrane oxygenator to the circuit if needed (32, 33). Interestingly, the TandemLung® system (LivaNova, Houston, TX, USA) is developed to permit extracorporeal life support circuit to be wearable, and the system consists of LifeSPARC pump, ProtekDuo® cannula, VoyagerVest®, and TandemLung® (34).
Surgical extracorporeal RVAD
In LV failure pathogenesis, nearly 41% of RVAD implant occurred 0–2 days after LVAD implant, and 23.4% of RVAD implant occurred within 3–14 days (35). At the same time, successful RVAD weaning rates were reported as> 60%, with intermediate support duration of 13–17 days. Among those cases, sRVAD has been the standard procedure because the patients have fresh sternotomy incisions; therefore, access to the RA and PA may not be difficult. An outflow cannula of sRVAD is often surgically implanted directly via PA or anastomosed prosthetic vascular graft. The inflow cannula is implanted directly into the RA or via the femoral vein to the RA. The extracorporeal centrifugal pump, similar to the one used in ECMO, is the most commonly used in sRVAD, and the system is expected to provide the pump flow of approximately 4–5 L/min, working as a direct RV bypass system. This configuration is also used in post cardiotomy shock.
Indirect RV bypass device
Veno-arterial extracorporeal membrane oxygenation (VA-ECMO)
ECMO therapy has been increasingly used, and the term became popular in the non-medical population during the COVID-19 pandemic. VA-ECMO is one of the widely used configurations of ECMO, which works as an indirect RV bypass mechanism for RHF. The system is effective at any point of the pathogenesis, but especially in the pulmonary etiology to avoid pressurizing the pulmonary vasculature. However, the flow from the VA-ECMO may increase the afterload for the heart, which can lead to pulmonary edema secondary to LV failure; therefore, clinicians must balance the pump flow (36).
Commonly, the peripheral cannulation via the femoral artery and femoral vein will be performed and will be connected to the membrane oxygenator and extracorporeal centrifugal pumps, such as CentriMag® (Abbott, Abbott Park, IL, USA), Rotaflow II® (Getinge, Göteborg, Sweden), CAPIOX® (Terumo Cardiovascular, Ann Arbor, MI, USA), and MERA® Centrifugal Blood Pump (Senko Medical Instrument, Tokyo, Japan) (6). Due to the incomplete full unloading of the ventricles, LV venting options may be needed (37, 38). Anticoagulation is recommended with an activated clotting time of 180–220 sec or a partial thromboplastin time of 65–90 sec (24). The advantage of this device is ease of placement, which allows it to serve as a bridge to a decision in an emergent clinical scenario (36). The system can provide circulatory support for up to 30 days.
The clinical outcome data of using VA-ECMO to isolate RV failure pathogenesis is limited to small cases studies (39–41); however, RV function seems to recover during the intermediate support period. (The clinical data that support using VA-ECMO is discussed in the previous section). In cases that are secondary to LV failure, the combination therapy of VA-ECMO and LV Impella, known as ECPELLA, has been increasing. A meta-analysis reported that short-term mortality among the ECPELLA cohort was 56.1%, which was better than VA-ECMO-alone therapy (63.7%), and occurrence of the major bleeding did not show a significant difference; however, hemolysis and renal replacement therapy were observed with a higher incidence of 36.8 and 51.6% than VA-ECMO alone (42). The report also noted that the size choice of the Impella device might have an impact on the rate of major bleeding.
Chronic RV support devices
Dual HeartMate 3 device use
Due to the lack of long-term support for MCS, the durable implantable CF LVAD has become the off-label use for durable RVAD. In the U.S., Medtronic Inc. (Minneapolis, MN, USA) announced that it was withdrawing the HeartWare Ventricular Assist Device® (HVAD) from the global market in 2021; therefore, HeartMate 3® (HM3) (Abbott, Abbott Park, IL, USA) is the only U.S. Food and Drug Administration-(FDA) approved LVAD in 2022. HM3 is a CF centrifugal pump with full maglev bearing, displacing volume of 80 ml. For the anatomical limitation, the placement of the right pump may be a concern (43). In one report with 14 patients who received a dual HM3 implant as biventricular support configuration, the HM3 was implanted into the RA using felt spacers to decrease the intraluminal length of the inflow cannula (44). The pump pocket was made with polytetrafluoroethylene patches, as it protrudes into the right thoracic cavity. Moreover, due to the physiologic limitations, the RVAD must work in a below-designed afterload range; in other words, the pump must operate in a low pump speed range (43). As a result, pump thrombosis occurrence has been consistently reported at 36–37% (43, 45, 46). To avoid pump thrombosis, a modification to the outflow graft–making the graft diameter smaller–has been made to elevate the afterload of the RVAD (47).
In the same study of 14 patients who received dual HM3 implantation (44), five patients downsized the outflow graft, nine did not, and just one pump thrombosis was reported. The pump thrombosis occurred in the patient in whom both pumps were used as a TAH with excising both ventricles (unknown about graft downsizing).
In another study with dual HM3 implantation, 12 patients underwent surgery for the bridge to transplantation (BTT). The RVAD was implanted to the RA using the spacer and was wrapped with a Gore-Tex® (W.L. Gore, Flagstaff, AZ) Soft Tissue Patch and placed in the right pleural space. Graft downsizing was done in three cases, and pump thrombosis was observed in three patients (48). The relationship between pump thrombosis and graft downsizing was unknown; however, in two of the three cases, the pump thrombosis was likely dislodgement of an intracardiac thrombus and ingestion into the pump. Among these cases, the incidence of pump thrombosis seems to be lower than the previous reported series.
The authors also suggested that the extremely low thrombosis risk of the HM3 as an LVAD may explain this phenomenon (48). The major difference between the two pumps is their operation range, which is the result of pump-bearing design features. The investigation for the thrombogenicity among two pumps working at low range speed may provide the justification for their suggestion. The clinical outcome for dual HM3 varied among two studies; the survival at 18 months was 54.6–91.7%.
Dual Berlin Heart EXCOR
The Berlin Heart EXCOR® (Berlin Heart, GmbH, Berlin, Germany) is a paracorporeal pneumatic-drive pulsatile device with a lineup of different pump sizes that cover pediatric to adult (10, 15, 25, 30, 50, 60, and 80 ml) with different valves (tri-leaflet polyurethane or bileaflet carbon valves). For the variety of the pump sizes, dual Excor has been used as a BVAD, especially in the pediatric population (49–51). The cannula implantation for the right-side pump is made via the RA for the inflow and the PA for the outflow. The overall survival at 1 year was approximately 40–83% at 1 year and 75% at 5 years for the adult (49, 52). Mortality among the pediatric patients ranged from 6 to 39%, and the transplantation rate from 37 to 73% (53).
SynCardia total artificial heart
The SynCardia TAH® (SynCardia Systems, LLC, Tucson, Arizona, USA) is a pneumatically driven pulsatile TAH with independent ventricles that are capable of providing a flow of more than 9 L/min (54) (Figure 3A). In addition, the SynCardia is a U.S. FDA-approved TAH indicated for use as a BTT in biventricular HF patients. Both the 50 ml and 70 ml size pumps are approved as BTT in the U.S., Europe, and Canada. Furthermore, in 2022, a 70 ml pump is undergoing an FDA clinical trial for destination therapy approval.
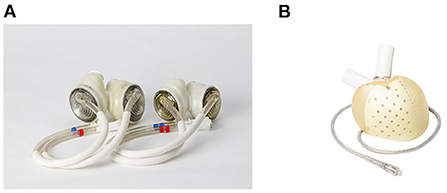
Figure 3. Current clinically available total artificial hearts. (A) SynCardia Total Artificial Heart 50 mL and 70 mL (55), used with permission). (B) Aeson Total Artificial Heart (used with permission by Carmat SA).
The clinical data supporting the SynCardia TAH, reported from an international registry, that the mortality on the transplantation waitlist was 7.4% for the 433 patients who underwent BTT therapy with TAH, and 87% of the patients reached heart transplant (HT) (56). Additionally, most of the patients were INTERMACS profile 1 (43%) or profile 2 (37%), and the risk factors for RHF were observed in 82% of patients (57).
Aeson total artificial heart
The Aeson® TAH (Carmat, Vélizy-Villacoublay, France) is a pulsatile TAH that is electro-hydraulically driven (Figure 3B). The pump consists mainly of four biological valves and two ventricle chambers with a membrane that separates the chambers for the blood and actuator fluid (58). For the clinical data, results from a pilot study are available. Between 2013 and 2015, four patients were implanted Aeson TAH (59). The support durations were 74, 270, 254, and 20 days, and the causes of death were: two device-related (details unknown), one respiratory failure, and one multi-organ failure. Two patients were able to discharge home. In 2021, a pivotal study was ongoing in Europe, and the pump was approved by the U.S. FDA for the conduction of an early feasibility study (60).
Right ventricle MCS device selection
Devices for isolated right ventricle injury pathogenesis
Table 1 summarizes the current strategy for each HF pathogenesis and RV support duration. The top row of the table consist of three pathogenesis explained in previous section and suitable treatment mechanism of MCS devices. For isolated RV injury pathogenesis, the support duration may be short-term as described in the previous section, therefore, temporary use devices may fit the strategy in selected cases. Either direct or indirect RV bypass of the treatment mechanism may be considered suitable option, unless the pulmonary resistance is within acceptable boundaries. The durable RV support mechanism should involve the use of MCS device capable to deliver full cardiac output and suitable for long term support. Thus, VA-ECMO, Impella RP, and LifeSPARC pump with ProtekDuo cannula system may be a choice.
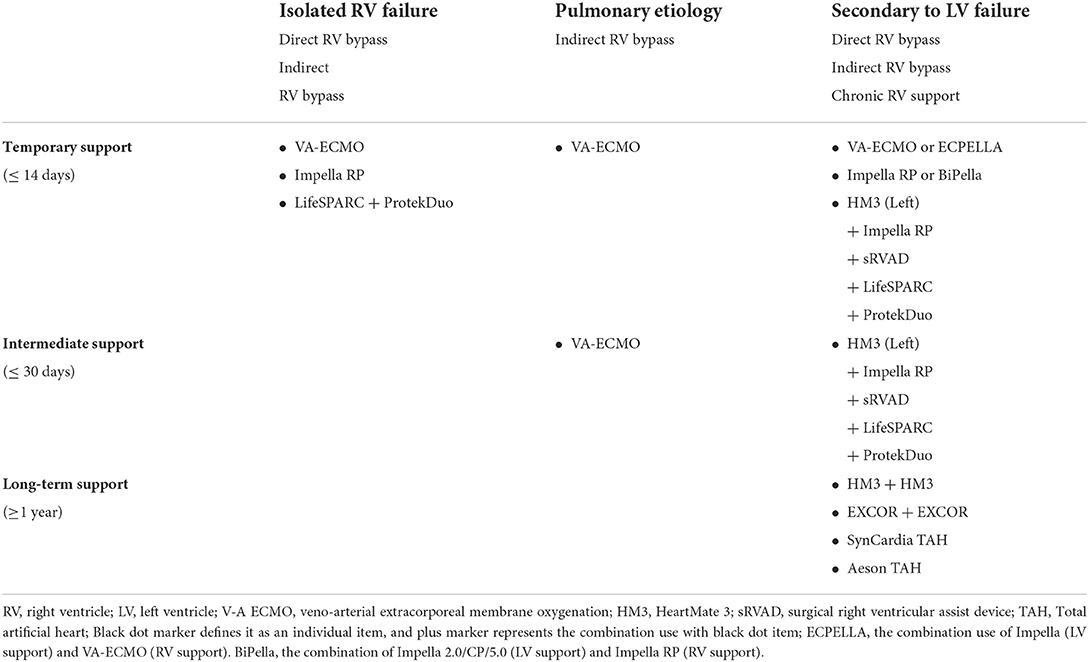
Table 1. Classification of the current mechanical circulatory support devices for RHF based on pathogenesis and the support duration.
In the rare case of isolated RV failure, such as the case of severe arrhythmogenic right ventricular cardiomyopathy, which requires RV long-term support, single report and study showed the feasibility of off-label use of HM3 and HVAD; however, the small number of patient remains to be insufficient to discuss the outcomes (61, 62).
Devices for pulmonary pathogenesis
For pulmonary pathogenesis, among group 1, 3, 4, and 5 PH patients, acute RHF cases can happen at the time of initial PH presentation or acute on chronic situation (63). The balloon atrial septostomy (BAS) is the most commonly performed as palliation for refractory PH and progression to RHF (64). A meta-analysis revealed that BAS showed relatively high postprocedural and short-term survival; however, the long-term survival was less impressive, suggesting the bridging role for BAS (65). There is another option to use MCS for this bridging situation. In the MCS option, the treatment may have to bypass the lung, therefore, a use of VA-ECMO may be considered. The concept of MCS therapy is bridge to recovery or bridge to transplantation. A retrospective (n = 6) study showed that three out of four PAH patients who underwent bridge to recovery therapy successfully survived to VA-ECMO decannulation (mean support duration, 12 ± 7 days) (66). In addition, several report demonstrated that awake VA-ECMO therapy for bridge to transplantation concept was feasible, even for several weeks support (67–69). Besides, a case report described that a RHF due to PAH patient (40 years of PAH history), who received staged direct RV bypass device (ProtekDuo cannula with CentriMag pump) and chronic RV support (HVAD on right atrium) therapies, showed no pulmonary hemorrhage, even patient's systolic PA pressure was around 100 to 120 mm Hg (70); however, successful long-term use of direct RV bypass devices for PAH has not been reported yet.
Acute PE and some of PH may need to be treated as acute RVF. For example, a post-operative period in CTEPH (group 4), and acute respiratory distress syndrome (ARDS) (group 3). The most suitable treatment option is indirect RV bypass with VA-ECMO. The 2019 European Society of Cardiology guidelines for the management of acute PE defined high-risk PE patients as having hemodynamic instability, PE severity index class III-V or simplified PE severity index ≥ 1, RV dysfunction on transthoracic echocardiogram or computed tomography pulmonary angiography, and elevated cardiac troponin levels. Furthermore, the guidelines suggest VA-ECMO therapy as class IIb, evidence level of C, in combination with surgical embolectomy or catheter-directed treatment and use of rapid short-term support (71, 72). One multicenter study in Europe found that overall 30-day mortality with ECMO-alone therapy was 77.7% and suggested using ECMO as a complement to surgical embolectomy (73).
Conversely, a group from China reported in its subgroup analysis that the earlier ECMO treatment was associated with lower in-hospital mortality and significant overall survival (74). In case cardiac function improves while severe respiratory failure remains, the reconfiguration to veno-arterio-venous ECMO is reported effective (75, 76). For chronic thromboembolic PH post-operative ECMO is recommended as the standard of care due to the reperfusion edema in the early post-operative period (77, 78).
The importance of RHF to ARDS has been magnified by the COVID-19 pandemic (79–82). A retrospective analysis (n = 39) reported that patients who received percutaneous RVAD with ECMO therapy had significantly lower in-hospital and 30-day mortality than patients treated with invasive mechanical ventilation only (83). Theoretically, a direct RV bypass device for pulmonary pathogenesis may over-pressurize the pulmonary vasculature and may cause pulmonary hemorrhage (84). In spite of this, pulmonary hemorrhage was reported in 12.5% of patients, and no statistic difference was achieved (83).
Devices for left ventricular failure pathogenesis
The treatment strategy for the LV failure pathogenesis should be made with a concern for each ventricle support duration. Also, the treatment mechanism can be chosen from either of direct RV bypass, indirect RV bypass, or chronic RV support, based on RV support duration. Regarding temporary to intermediate LV support, the representative cases may be cardiogenic shock, the post cardiotomy shock, and acute on chronic HF manifesting hemodynamic instability. Among those cases, the importance of trans-femoral or trans-axillary/trans-aortic percutaneous LVADs has been increasing (85, 86). If the symptom of RHF remains with enough LV unloading including LV Impella, the combination use of temporary MCS for RHF should be considered, because of its ease of implantation and explantation (20, 87). Therefore, Impella RP or LifeSPARC pump with ProtekDuo system may be an option for the right side. If patients manifest high pulmonary vascular resistance and a need for oxygenation, VA-ECMO device may be the choice. If only decreased RV contractility is observed, a direct RV bypass device may be suitable; however, a strategy in which direct RV bypass is connected to a membrane oxygenator may be suitable. Besides, the operators must concern which access (e.g., left or right, jugular or femoral) to cannulate the devices for building up the treatment strategy.
Regarding the long-term LV support for representative cases of durable LVAD implantation, RHF complicates 10% to 40% of LVAD implants (88, 89). LVADs will increase the incidence of RHF because they may shift the interventricular septum to the left and may decrease the septal contribution to RV contraction (90, 91). Furthermore, according to the 12th Interagency Registry for Mechanically Assisted Circulatory Support (INTERMACS) Report, 26,688 patients (96%) received isolated CF LVAD therapy, and 1,136 patients received a CF biventricular support device (BVAD) therapy, of whom 91.3% were supported with temporary CF RVADs (92).
In HM3 pivotal and post-pivotal trial study report, RHF was seen in 34.2–37.4% of patients, and 4.1–7.4% of patients required an RVAD implant (35). Notably, 40.6% of RVAD implants were performed within 2 days, and 23.4% of implants were performed between 3 and 14 days. Furthermore, the Mechanical Circulatory Support Academic Research Consortium divided the RHF after LVAD implantation into three groups:
• Early acute RHF, which is defined by the need for implantation of a temporary or durable RVAD before the patient leaves the operating room;
• Early post-implant RHF, which needs implantation of RVADs within 30 days following LVAD implantation or failure to wean from inotropic, vasopressor, or inhaled nitric oxide within 14 days following LVAD implantation or having to initiate these supports within 30 days;
• Lastly, late RHF, which need implantation of an RVAD more than 30 days after an LVAD or hospitalization with intravenous diuretics or inotropic support that occurs more than 30 days post-implant (93).
To summarize, approximately 40% of RHF after LVAD implantation patients may fall into early acute RHF, and 23% of RHF patients will present early post-implant RHF. However, the predictor for the RHF following LVAD implant still needs to be explored. In HM3 pivotal and post-pivotal studies, intra-aortic balloon pump use (odds ratio [OR]: 1.84), destination therapy (OR: 1.69), INTERMACS profiles 1–2 (OR: 1.60), and estimated glomerular filtration rate > 10 mL/min/1.73 m2 (OR: 0.9) were all reported as predictors for the RHF requiring RVAD (35).
The RV MCS device selection during long-term LV support may largely depend on the duration of the RV support. Unplanned temporary RVAD use was reported with successful RVAD weaning rates of over 60% with a median support duration of 13–17 days (25, 94–96). Therefore, short-duration RV MCS devices may be suitable for this strategy in selected cases. The treatment mechanism will be either direct or indirect RV bypass, which has more advantages with regard to conditions of pulmonary function and performance; however, there has been a single report of using a direct RV bypass device connected to a membrane oxygenator and aiming for early extubation; thus, pulmonary vascular resistance and removability may be the main concern (97).
As for long-term biventricular support, as discussed, the situation is rare, but it does exist. The options are limited due to the lack of durable MCS devices designed explicitly for RVAD. Currently, these options are: the off-label implantation of CF LVAD as an RVAD (Dual HM3); a paracorporeal pulsatile ventricular assist device as a biventricular support configuration (Dual Berlin Heart EXCOR); and TAH (SynCardia TAH or Aeson TAH). For the timing of RVAD intervention, severe late RHF, which is defined by the requirement for an RVAD at months 3–12, is very rare (98). However, recent data have been reported from Japan suggesting that late RHF is related to cardiac cachexia at HT, and increases the risk of infections within 6 months of HT (99). Thus, the timing of the intervention for late RVAD may change in the future with a prognosis of a specifically designed durable implantable RVAD.
As for the predictors for RHF, several research efforts are ongoing. The PA pulsatility index predicted the early RHF with a cutoff value of 2.0 (area under the curve, 0.77; sensitivity, 74%; specificity, 67%) (100). The RV global longitudinal strain predicted the early acute and post-implant RHF with a cutoff value of −9.7% (area under the curve, 0.86; sensitivity, 89%; specificity, 78%) (101).
Future perspectives
There seems to be major three work fields that should have explored. First, the development of specifically designed, durable RVAD may be required. The specifically designed ventricular assist device that is able to operate on both sides of the heart, such as the Cleveland Clinic Universal Ventricular Assist Device (UVAD, Figure 4), is seen as a promising, universal solution able to cover the hemodynamic needs of the broad HF patient population (102, 103). The UVAD is a hybrid of magnetically and hydrodynamically levitated centrifugal pumps, and is an innovative apparatus with a wide operating range, automatic regurgitant-flow shut-off, and pulse augmentation features (104). The unique design architecture of the UVAD permits accommodation of various hemodynamic profiles. The flexible operating range allows the pump to be used in a broad range of hemodynamic conditions, so it can be used on both right and left ventricular/atrium. Additionally, the automatic regurgitant-flow shut-off feature provides the feasibility for non-invasive pump-off tests, which plays an important role for RVAD weaning (105). The device is currently undergoing engineering optimization, and the developers are also focusing on addressing the need of dual-device operation using a single controller, for biventricular failure application as BVAD support.
Second, mobility of percutaneous RVAD must be improved. Currently, Impella RP is inserted from femoral vein because of the inlet of the cannula is designed to place in IVC and 11 Fr sheath (15 Fr outer diameter) stays at cannulation site, therefore, not suitable for rehabilitation. As for ProtekDuo cannula, 29 Fr cannula is inserted from right jugular vein; however, the large-bore cannula may still limit patients' outcome.
Third, the oxygenation support with RVAD may be interesting field to explore. RVAD circuit connecting to membrane oxygenator, known as Oxy-RVAD configuration, has been reported for use in the patient waiting for lung transplant (106). TandemLung® system is applicable for this category.
Discussion
With an increasing incidence of RHF in the last decade, the therapeutic and device options for this pathology remain unmet. While previously underestimated, the RV has triggered interest with the scope of better understanding of the underlying disease pathogenesis in order to provide potential therapeutic options and device solutions. With ongoing effort amongst multiple engineering and clinical groups, there has been a substantial spike in research interest and development of unique device-based target therapies.
The device selection for the shorter support duration seemed to have progressed to less invasive. To provide less invasive MCS therapy for RHF, the prediction for the support duration increases its importance. In the LV failure pathogenesis, more than 98% of patients received their LVAD therapy with centrifugal pumps in 2020, and more than 83% of patients were implanted HM3 (92). This trend is expected to accelerate in 2022 because the centrifugal pump with hybrid levitation is no longer available in the U.S. Therefore, most patients who present with RHF with LV failure pathogenesis will have HM3 implanted and will have a fresh sternotomy incision.
For this reason, sRVAD has been the gold standard for temporary- and intermediate-support for RHF. However, the effort to implant the HM3 with minimally invasive surgery has begun (107) and will have an impact on the current strategy because the ease of access to RA and PA will be unknown. Also, the less invasive method may be another key for the MCS therapy for RHF. If it is less invasive, the hurdle for making the decision to use MCS may lower, and intervention may begin earlier. The devices that will have the greatest advantage may be the percutaneous RVADs (pRVADs). Regarding the survival outcomes between the pRVAD and sRVAD groups, one study reported that there was no significant difference in 30-day mortality, 1-year survival, or 2-year survival, but the length of intensive care unit stay was significantly shorter in the pRVAD group (21 days vs. 34 days, p = 0.01) (108), however, data still remain scarce.
Regarding percutaneous MCS, the combination of Impella devices to support both ventricles has been increasing. The most typical examples are ECPELLA and BiPella. The difference between these treatment methods is whether to use Impella RP or VA-ECMO for the RV support. A meta-analysis showed that there was no significant difference between the ECPELLA cohort and BiPella cohort in mortality (p = 0.93) (109). Additionally, there was no significant difference in adverse events, major bleeding, hemolysis, and limb ischemia; however, the authors further prospective studies due to small sample sizes and the lack of hemodynamic data.
For long-term RVAD support, the currently available option is mainly limited to HM3 off-label use. The most concerning issue with this treatment is RVAD pump thrombosis. Regarding the necessity of outflow graft downsizing, one study operated the HM3 RVAD with a mean RVAD speed of 4,991 rpm and mean RVAD flow of 4.3 L/min (48). It was consistent with the pressure head curve of HM3 (110); if 4–5 L/min flow was demanded, 4,000–5,000 rpm pump speed would create a pump pressure rise of approximately 30–60 mm Hg, which is the pressure difference that may not need the graft downsizing. As a result, pump thrombosis was rarely reported. In addition, the HM3 has an artificial pulse mode feature, which improves the pump washout, and it will start of 4,000 rpm. Therefore, if the demanding flow is below 4 L/min, graft downsizing may be needed to operate the pump with increased speed.
Moreover, in this review, we discussed about one-to-one correspondence treatment strategy for each pathogenesis; however, in the real clinical world, the most of the patients are expected to have multiple pathogenesis. For example, the patient in acute exacerbation of American College of Cardiology/American Heart Association stage C heart failure with comorbidity of severe chronic obstructive pulmonary disease, may not be a rare case. Therefore, there may be a situation that LV is not bad as receiving LVAD therapy but RV requires MCS device, to overcome the acute decompensation. For these patients, applying the algorithm for RV MCS device us may be helpful (20).
Lastly, we did not include the RHF after HT because of the complexity of the mechanism and pathogenesis; however, the importance of MCS therapy for RHF is increasing. Moreover, since the first successful human HT was reported in 1967 in South Africa, more than 120,000 patients have received HT therapy (111), and the number who receive second and third HT transplants is growing (112). For those patients, each surgery becomes higher risk, and the risk of graft failure also increases. The need for MCS devices, including the TAH, may increase.
Author contributions
TK: manuscript preparation. CM: critical manuscript review. KF: critical revision of article. JK: critical revision of article, approval of article. All authors contributed to the article and approved the submitted version.
Funding
The Universal Ventricular Assist Device Program is supported by federal funding from the National Heart, Lung, and Blood Institute under grant 5R21HL133871 (to KF).
Conflict of interest
Author KF is a co-inventor of Universal Assist Device.
The remaining authors declare that the research was conducted in the absence of any commercial or financial relationships that could be construed as a potential conflict of interest.
The reviewer MS declared a past co-authorship with one of the authors CM, KF, and JK to the handling Editor.
Publisher's note
All claims expressed in this article are solely those of the authors and do not necessarily represent those of their affiliated organizations, or those of the publisher, the editors and the reviewers. Any product that may be evaluated in this article, or claim that may be made by its manufacturer, is not guaranteed or endorsed by the publisher.
References
1. Tsao CW, Aday AW, Almarzooq ZI, Alonso A, Beaton AZ, Bittencourt MS, et al. Heart disease and stroke statistics-2022 update: a report from the American Heart Association. Circulation. (2022) 145:e153–639. doi: 10.1161/CIR.0000000000001052
2. McDonagh TA, Metra M, Adamo M, Gardner RS, Baumbach A, Böhm M, et al. 2021 esc guidelines for the diagnosis and treatment of acute and chronic heart failure: Developed by the task force for the diagnosis and treatment of acute and chronic heart failure of the european society of cardiology (esc) with the special contribution of the heart failure association (hfa) of the esc. Eur Heart J. (2021) 42:3599–726. doi: 10.1093/eurheartj/ehab368
3. Conrad N, Judge A, Tran J, Mohseni H, Hedgecott D, Crespillo AP, et al. Temporal trends and patterns in heart failure incidence: a population-based study of 4 million individuals. Lancet. (2018) 391:572–80. doi: 10.1016/S0140-6736(17)32520-5
4. Savarese G, Lund LH. Global public health burden of heart failure. Card Fail Rev. (2017) 3:7–11. doi: 10.15420/cfr.2016:25:2
5. Metra M, Teerlink JR. Heart failure. Lancet. (2017) 390:1981–95. doi: 10.1016/S0140-6736(17)31071-1
6. Konstam MA, Kiernan MS, Bernstein D, Bozkurt B, Jacob M, Kapur NK, et al. Evaluation and management of right-sided heart failure: a scientific statement from the american heart association. Circulation. (2018) 137:e578–622. doi: 10.1161/CIR.0000000000000560
7. Iglesias-Garriz I, Olalla-Gómez C, Garrote C, López-Benito M, Martín J, Alonso D, et al. Contribution of right ventricular dysfunction to heart failure mortality: a meta-analysis. Rev Cardiovasc Med. (2012) 13:e62–9. doi: 10.3909/ricm0602
8. Skhiri M, Hunt SA, Denault AY, Haddad F. [Evidence-based management of right heart failure: a systematic review of an empiric field]. Rev Esp Cardiol. (2010) 63:451–71. doi: 10.1016/S0300-8932(10)70066-X
9. Goldstein JA. Pathophysiology and management of right heart ischemia. J Am Coll Cardiol. (2002) 40:841–53. doi: 10.1016/S0735-1097(02)02048-X
10. Italia LJ, Lembo NJ. Starling MR, Crawford MH, Simmons RS, Lasher JC, et al. Hemodynamically important right ventricular infarction: Follow-up evaluation of right ventricular systolic function at rest and during exercise with radionuclide ventriculography and respiratory gas exchange. Circulation. (1987) 75:996–1003. doi: 10.1161/01.CIR.75.5.996
11. Yasuda T, Okada RD, Leinbach RC, Gold HK, Phillips H, McKusick KA, et al. Serial evaluation of right ventricular dysfunction associated with acute inferior myocardial infarction. Am Heart J. (1990) 119:816–22. doi: 10.1016/S0002-8703(05)80317-5
12. Gumina RJ, Murphy JG, Rihal CS, Lennon RJ, Wright RS. Long-Term survival after right ventricular infarction. Am J Cardiol. (2006) 98:1571–3. doi: 10.1016/j.amjcard.2006.07.033
13. Galiè N, Humbert M, Vachiery JL, Gibbs S, Lang I, Torbicki A, et al. 2015 ESC/ERS guidelines for the diagnosis and treatment of pulmonary hypertension: the joint task force for the diagnosis and treatment of pulmonary hypertension of the European Society of Cardiology (ESC) and the European Respiratory Society (ERS): Endorsed by: Association for European Paediatric and Congenital Cardiology (AEPC), International Society For Heart And Lung Transplantation (ishlt). Eur Heart J. (2016) 37:67–119. doi: 10.1093/eurheartj/ehv317
14. Strange G, Playford D, Stewart S, Deague JA, Nelson H, Kent A, et al. Pulmonary hypertension: prevalence and mortality in the armadale echocardiography cohort. Heart. (2012) 98:1805–11. doi: 10.1136/heartjnl-2012-301992
15. Benza RL, Miller DP, Barst RJ, Badesch DB, Frost AE, McGoon MD. An evaluation of long-term survival from time of diagnosis in pulmonary arterial hypertension from the reveal registry. Chest. (2012) 142:448–56. doi: 10.1378/chest.11-1460
16. Miyagi C, Miyamoto T, Karimov JH, Starling RC, Fukamachi K. Device-based treatment options for heart failure with preserved ejection fraction. Heart Fail Rev. (2021) 26:749–62. doi: 10.1007/s10741-020-10067-5
17. Anker SD, Butler J, Filippatos G, Ferreira JP, Bocchi E, Böhm M, et al. Empagliflozin in heart failure with a preserved ejection fraction. N Engl J Med. (2021) 385:1451–61. doi: 10.1056/NEJMoa2107038
18. Solomon SD, McMurray JJV, Anand IS, Ge J, Lam CSP, Maggioni AP, et al. Angiotensin-neprilysin inhibition in heart failure with preserved ejection fraction. N Engl J Med. (2019) 381:1609–20. doi: 10.1056/NEJMoa1908655
19. Shah KS, Xu H, Matsouaka RA, Bhatt DL, Heidenreich PA, Hernandez AF, et al. Heart failure with preserved, borderline, and reduced ejection fraction: 5-year outcomes. J Am Coll Cardiol. (2017) 70:2476–86. doi: 10.1016/j.jacc.2017.08.074
20. Kapur NK, Esposito ML, Bader Y, Morine KJ, Kiernan MS, Pham DT, et al. Mechanical circulatory support devices for acute right ventricular failure. Circulation. (2017) 136:314–26. doi: 10.1161/CIRCULATIONAHA.116.025290
21. Anderson M, Morris DL, Tang D, Batsides G, Kirtane A, Hanson I, et al. Outcomes of patients with right ventricular failure requiring short-term hemodynamic support with the impella rp device. J Heart Lung Trans. (2018) 37:1448–58. doi: 10.1016/j.healun.2018.08.001
22. Anderson MB, Goldstein J, Milano C, Morris LD, Kormos RL, Bhama J, et al. Benefits of a novel percutaneous ventricular assist device for right heart failure: the prospective recover right study of the impella rp device. J Heart Lung Trans. (2015) 34:1549–60. doi: 10.1016/j.healun.2015.08.018
23. Montisci A, Sala S, Maj G, Cattaneo S, Pappalardo F. Comprehensive evaluation of impella rp(®) in right ventricular failure. Future Cardiol. (2022) 18:285–98. doi: 10.2217/fca-2021-0075
24. Bhatia M, Jia S, Smeltz A, Kumar PA. Right heart failure management: focus on mechanical support options. J Cardiothorac Vasc Anesth. (2022). doi: 10.1053/j.jvca.2022.02.022
25. Shimada S, Nawata K, Kinoshita O, Ono M. Mechanical circulatory support for the right ventricle in combination with a left ventricular assist device. Expert Rev Med Devices. (2019) 16:663–73. doi: 10.1080/17434440.2019.1635006
26. Tschöpe C, Van Linthout S, Klein O, Mairinger T, Krackhardt F, Potapov EV, et al. Mechanical unloading by fulminant myocarditis: Lv-impella, ecmella, bi-pella, and propella concepts. J Cardiovasc Transl Res. (2019) 12:116–23. doi: 10.1007/s12265-018-9820-2
27. Kuchibhotla S, Esposito ML, Breton C, Pedicini R, Mullin A, O'Kelly R, et al. Acute biventricular mechanical circulatory support for cardiogenic shock. J Am Heart Assoc. (2017) 6:6670. doi: 10.1161/JAHA.117.006670
28. Schmack B, Weymann A, Popov AF, Patil NP, Sabashnikov A, Kremer J, et al. Concurrent left ventricular assist device (lvad) implantation and percutaneous temporary rvad support via cardiacassist protek-duo tandemheart to preempt right heart failure. Med Sci Monit Basic Res. (2016) 22:53–7. doi: 10.12659/MSMBR.898897
29. Ravichandran AK, Baran DA, Stelling K, Cowger JA, Salerno CT. Outcomes with the tandem protek duo dual-lumen percutaneous right ventricular assist device. ASAIO J. (2018) 64:570–2. doi: 10.1097/MAT.0000000000000709
30. Salna M, Garan AR, Kirtane AJ, Karmpaliotis D, Green P, Takayama H, et al. Novel percutaneous dual-lumen cannula-based right ventricular assist device provides effective support for refractory right ventricular failure after left ventricular assist device implantation. Interact Cardiovasc Thorac Surg. (2020) 30:499–506. doi: 10.1093/icvts/ivz322
31. Oliveros E, Collado FM, Poulin MF, Seder CW, March R, Kavinsky CJ. Percutaneous right ventricular assist device using the tandemheart protekduo: Real-world experience. J Invasive Cardiol. (2021) 33:E407–E11.
32. Bermudez CA, Lagazzi L, Crespo MM. Prolonged support using a percutaneous oxyrvad in a patient with end-stage lung disease, pulmonary hypertension, and right cardiac failure. ASAIO J. (2016) 62:e37–40. doi: 10.1097/MAT.0000000000000343
33. Wang D, Zou X, Liu X, Lick SD, Zwischenberger JB. Oxyrvad for total right heart and respiratory support. Conf Proc IEEE Eng Med Biol Soc. (2006) 2006:5390–1. doi: 10.1109/IEMBS.2006.260849
34. Ramzy D, Chung J. The 19 description, tandem: implantation, life system device and clinical results. Mech Cir Sup: Principles Appl. (2021) 1:138. doi: 10.1093/med/9780190909291.003.0019
35. Mehra MR, Cleveland Jr JC, Uriel N, Cowger JA, Hall S, Horstmanshof D, et al. Primary results of long-term outcomes in the momentum 3 pivotal trial and continued access protocol study phase: A study of 2200 heartmate 3 left ventricular assist device implants. Eur J Heart Fail. (2021) 23:1392–400. doi: 10.1002/ejhf.2211
36. Grant C. Jr., Richards JB, Frakes M, Cohen J, Wilcox SR. Ecmo and right ventricular failure: review of the literature. J Intensive Care Med. (2021) 36:352–60. doi: 10.1177/0885066619900503
37. Desai SR, Hwang NC. Strategies for left ventricular decompression during venoarterial extracorporeal membrane oxygenation—a narrative review. J Cardiothorac Vasc Anesth. (2020) 34:208–18. doi: 10.1053/j.jvca.2019.08.024
38. Truby L, Mundy L, Kalesan B, Kirtane A, Colombo PC, Takeda K, et al. Contemporary outcomes of venoarterial extracorporeal membrane oxygenation for refractory cardiogenic shock at a large tertiary care center. ASAIO J. (2015) 61:403–9. doi: 10.1097/MAT.0000000000000225
39. Michels G, Krausgrill B, Rahmanian P, Wahlers T, Baldus S, Pfister R. [Extracorporeal membrane oxygenation as bridge to recovery in infarction-related refractory right heart failure]. Dtsch Med Wochenschr. (2013) 138:2246–9. doi: 10.1055/s-0033-1349539
40. Wang Y, Zhu Z, Xu R, Li D, Wang T, Liu K, et al. Complete occlusion of right coronary artery due to stanford type a aortic dissection - successful treatment with Extracorporeal Membrane Oxygenation (ECMO). Braz J Cardiovasc Surg. (2019) 34:491–4. doi: 10.21470/1678-9741-2018-0060
41. Suguta M, Hoshizaki H, Anno M, Naito S, Tada H, Nogami A, et al. Right ventricular infarction with cardiogenic shock treated with percutaneous cardiopulmonary support: a case report. Jpn Circ J. (1999) 63:813–5. doi: 10.1253/jcj.63.813
42. Fiorelli F, Panoulas V. Impella as unloading strategy during va-ecmo: systematic review and meta-analysis. Rev Cardiovasc Med. (2021) 22:1503–11. doi: 10.31083/j.rcm2204154
43. Karimov JH, Sunagawa G, Horvath D, Fukamachi K, Starling RC, Moazami N. Limitations to chronic right ventricular assist device support. Ann Thorac Surg. (2016) 102:651–8. doi: 10.1016/j.athoracsur.2016.02.006
44. Lavee J, Mulzer J, Krabatsch T, Marasco S, McGiffin D, Garbade J, et al. An international multicenter experience of biventricular support with heartmate 3 ventricular assist systems. J Heart Lung Transplant. (2018) 37:1399–402. doi: 10.1016/j.healun.2018.08.008
45. Shah P, Ha R, Singh R, Cotts W, Adler E, Kiernan M, et al. Multicenter experience with durable biventricular assist devices. J Heart Lung Transplant. (2018) 37:1093–101. doi: 10.1016/j.healun.2018.05.001
46. Tran HA, Pollema TL, Silva Enciso J, Greenberg BH, Barnard DD, Adler ED, et al. Durable biventricular support using right atrial placement of the heartware hvad. ASAIO J. (2018) 64:645 doi: 10.1097/MAT.0000000000000645
47. Hetzer R, Krabatsch T, Stepanenko A, Hennig E, Potapov EV. Long-term biventricular support with the heartware implantable continuous flow pump. J Heart Lung Transplant. (2010) 29:822–4. doi: 10.1016/j.healun.2010.02.012
48. McGiffin D, Kure C, McLean J, Marasco S, Bergin P, Hare JL, et al. The results of a single-center experience with heartmate 3 in a biventricular configuration. J Heart Lung Transplant. (2021) 40:193–200. doi: 10.1016/j.healun.2020.12.006
49. Michel S, Buchholz S, Buech J, Veit T, Fabry T, Abicht J, et al. Bridging patients in cardiogenic shock with a paracorporeal pulsatile biventricular assist device to heart transplantation-a single-centre experience. Eur J Cardiothorac Surg. (2022) 61:942–9. doi: 10.1093/ejcts/ezab547
50. Araki K, Ueno T, Taira M, Kanaya T, Watanabe T, Tominaga Y, et al. Pediatric patient with restrictive cardiomyopathy on staged biventricular assist device support with berlin heart excor® underwent heart transplantation successfully: the first case in japan. J Artif Organs. (2021) 24:269–72. doi: 10.1007/s10047-020-01208-6
51. Bhatia I, Ho KC, Rocha BA, Yam N, Lun KS, Yung TC, et al. Pediatric ventricular assist device therapy for advanced heart failure-hong kong experience. J Artif Organs. (2020) 23:133–9. doi: 10.1007/s10047-019-01140-4
52. Bartfay SE, Dellgren G, Hallhagen S, Wåhlander H, Dahlberg P, Redfors B, et al. Durable circulatory support with a paracorporeal device as an option for pediatric and adult heart failure patients. J Thorac Cardiovasc Surg. (2021) 161:1453–64.e4. doi: 10.1016/j.jtcvs.2020.04.163
53. Rohde S, Antonides CFJ, Dalinghaus M, Muslem R, Bogers A. Clinical outcomes of paediatric patients supported by the berlin heart excor: a systematic review. Eur J Cardiothorac Surg. (2019) 56:830–9. doi: 10.1093/ejcts/ezz092
54. Hulman M, Artemiou P, Hudec V, Olejarova I, Goncalvesova E. Syncardia, total artificial heart, as a bridge to transplant. Bratisl Lek Listy. (2019) 120:325–30. doi: 10.4149/BLL_2019_053
55. Villa CR, Morales DLS. The total artificial heart in end-stage congenital heart disease. Front Physiol. (2017) 8:131. doi: 10.3389/fphys.2017.00131
56. Coyan GN, Huckaby LV, Diaz-Castrillon CE, Miguelino AM, Kilic A. Trends and outcomes following total artificial heart as bridge to transplant from the unos database. J Card Surg. (2022) 37:1215–21. doi: 10.1111/jocs.16329
57. Arabía FA, Cantor RS, Koehl DA, Kasirajan V, Gregoric I, Moriguchi JD, et al. Interagency registry for mechanically assisted circulatory support report on the total artificial heart. J Heart Lung Transplant. (2018) 37:1304–12. doi: 10.1016/j.healun.2018.04.004
58. Carpentier A, Latrémouille C, Cholley B, Smadja DM, Roussel J-C, Boissier E, et al. First clinical use of a bioprosthetic total artificial heart: report of two cases. Lancet. (2015) 386:1556–63. doi: 10.1016/S0140-6736(15)60511-6
59. Latrémouille C, Carpentier A, Leprince P, Roussel J-C, Cholley B, Boissier E, et al. A bioprosthetic total artificial heart for end-stage heart failure: results from a pilot study. J Heart Lung Transplant. (2018) 37:33–7. doi: 10.1016/j.healun.2017.09.002
60. Han JJ. Aeson—the carmat total artificial heart is approved for enrollment in the united states. Artif Organs. (2021) 45:445–6. doi: 10.1111/aor.13959
61. Ricklefs M, Hanke JS, Dogan G, Chatterjee A, Feldmann C, Deniz E, et al. Successful heartmate 3 implantation in isolated right heart failure-first in man experience of right heart configuration. J Thorac Dis. (2018) 10:S1834–s7. doi: 10.21037/jtd.2018.05.35
62. Bernhardt AM, De By TMMH, Reichenspurner H, Deuse T. Isolated permanent right ventricular assist device implantation with the heartware continuous-flow ventricular assist device: first results from the european registry for patients with mechanical circulatory support. Eur J Cardio-Thoracic Surgery. (2014) 48:158–62. doi: 10.1093/ejcts/ezu406
63. Tsao AL, Opotowsky AR. Ask the expert: Is there a role for percutaneous or surgically implanted right ventricular assist devices in pulmonary arterial hypertension? Adv Pulm. Hypertension. (2020) 19:33–4.
64. Hyder SN, Chatterjee S, Aggarwal V. Percutaneous treatments for pulmonary hypertension: Reviewing the growing procedural role for interventional cardiology. Interv Cardiol Clin. (2022) 11:293–305.
65. Khan MS, Memon MM, Amin E, Yamani N, Khan SU, Figueredo VM, et al. Use of balloon atrial septostomy in patients with advanced pulmonary arterial hypertension: A systematic review and meta-analysis. Chest. (2019) 156:53–63.
66. Rosenzweig EB, Brodie D, Abrams DC, Agerstrand CL, Bacchetta M. Extracorporeal membrane oxygenation as a novel bridging strategy for acute right heart failure in group 1 pulmonary arterial hypertension. ASAIO J. (2014) 60:129–33. doi: 10.1097/MAT.0000000000000021
67. Fuehner T, Kuehn C, Hadem J, Wiesner O, Gottlieb J, Tudorache I, et al. Extracorporeal membrane oxygenation in awake patients as bridge to lung transplantation. Am J Respir Crit Care Med. (2012) 185:763–8. doi: 10.1164/rccm.201109-1599OC
68. Olsson KM, Simon A, Strueber M, Hadem J, Wiesner O, Gottlieb J, et al. Extracorporeal membrane oxygenation in nonintubated patients as bridge to lung transplantation. Am J Transplant. (2010) 10:2173–8. doi: 10.1111/j.1600-6143.2010.03192.x
69. Hoeper MM, Benza RL, Corris P, de Perrot M, Fadel E, Keogh AM, et al. Intensive care, right ventricular support and lung transplantation in patients with pulmonary hypertension. Eur Respir J. (2019) 53:2018. doi: 10.1183/13993003.01906-2018
70. Rosenzweig EB, Chicotka S, Bacchetta M. Right ventricular assist device use in ventricular failure due to pulmonary arterial hypertension: lessons learned. J Heart Lung Transplant. (2016) 35:1272–4. doi: 10.1016/j.healun.2016.07.010
71. Konstantinides SV, Meyer G, Becattini C, Bueno H, Geersing GJ, Harjola VP, et al. 2019 ESC guidelines for the diagnosis and management of acute pulmonary embolism developed in collaboration with the European Respiratory Society (ERS): The task force for the diagnosis and management of acute pulmonary embolism of the European Society of Cardiology (ESC). Eur Respir J. (2019) 54:2019. doi: 10.1183/13993003.01647-2019
72. Yusuff HO, Zochios V, Vuylsteke A. Extracorporeal membrane oxygenation in acute massive pulmonary embolism: a systematic review. Perfusion. (2015) 30:611–6. doi: 10.1177/0267659115583377
73. Meneveau N, Guillon B, Planquette B, Piton G, Kimmoun A, Gaide-Chevronnay L, et al. Outcomes after extracorporeal membrane oxygenation for the treatment of high-risk pulmonary embolism: a multicentre series of 52 cases. Eur Heart J. (2018) 39:4196–204. doi: 10.1093/eurheartj/ehy464
74. Tsai HY, Wang YT, Lee WC, Yen HT, Lo CM, Wu CC, et al. Efficacy and safety of veno-arterial extracorporeal membrane oxygenation in the treatment of high-risk pulmonary embolism: a retrospective cohort study. Front Cardiovasc Med. (2022) 9:799488. doi: 10.3389/fcvm.2022.799488
75. Matsuyoshi T, Shimizu K, Kaneko H, Kohsen D, Suzuki H. Reconfiguration from veno-arterial to veno-arterio-venous extracorporeal membrane oxygenation for massive pulmonary embolism. J Artif Organs. (2022). doi: 10.1007/s10047-022-01327-2
76. Blandino Ortiz A, Belliato M, Broman LM, Lheureux O, Malfertheiner MV, Xini A, et al. Early findings after implementation of veno-arteriovenous ecmo: a multicenter european experience. Membranes (Basel). (2021) 11:81. doi: 10.3390/membranes11020081
77. Jenkins D, Madani M, Fadel E, D'Armini AM, Mayer E. Pulmonary endarterectomy in the management of chronic thromboembolic pulmonary hypertension. Eur Respir Rev. (2017) 26:2016. doi: 10.1183/16000617.0111-2016
78. Abdelnour-Berchtold E, Donahoe L, McRae K, Asghar U, Thenganatt J, Moric J, et al. Central venoarterial extracorporeal membrane oxygenation as a bridge to recovery after pulmonary endarterectomy in patients with decompensated right heart failure. J Heart Lung Transplant. (2022). doi: 10.1016/j.healun.2022.02.022
79. Argulian E, Sud K, Vogel B, Bohra C, Garg Vaani P, Talebi S, et al. Right ventricular dilation in hospitalized patients with covid-19 infection. JACC. (2020) 13:2459–61. doi: 10.1016/j.jcmg.2020.05.010
80. Huang L, Zhao P, Tang D, Zhu T, Han R, Zhan C, et al. Cardiac involvement in patients recovered from covid-2019 identified using magnetic resonance imaging. JACC. (2020) 13:2330–9. doi: 10.1016/j.jcmg.2020.05.004
81. Corica B, Marra AM, Basili S, Cangemi R, Cittadini A, Proietti M, et al. Prevalence of right ventricular dysfunction and impact on all-cause death in hospitalized patients with covid-19: a systematic review and meta-analysis. Sci Rep. (2021) 11:17774. doi: 10.1038/s41598-021-96955-8
82. Patel SP, Solomon BJ, Pascotto RD, D'Orazio SE, Navas EV, Cubeddu RJ, et al. Right ventricular failure manifesting in corona virus disease 2019 acute respiratory distress syndrome: a call to transition from venovenous extracorporeal membranous oxygenation to right ventricular assist device extracorporeal membranous oxygenation. J Cardiothorac Vasc Anesth. (2022). doi: 10.1053/j.jvca.2022.02.026
83. Cain MT, Smith NJ, Barash M, Simpson P, Durham LA. 3rd, Makker H, et al. Extracorporeal membrane oxygenation with right ventricular assist device for COVID-19 ards. J Surg Res. (2021) 264:81–9. doi: 10.1016/j.jss.2021.03.017
84. Connolly MW, Lim KH, Rose DM, Tan IP, Grossi EA, Baumann GF, et al. Efficacy of right ventricular unloading during right coronary artery occlusion in an experimental model. Surgery. (1986) 100:143–9.
85. Attinger-Toller A, Bossard M, Cioffi GM, Tersalvi G, Madanchi M, Bloch A, et al. Ventricular unloading using the impella(tm) device in cardiogenic shock. Front Cardiovasc Med. (2022) 9:856870. doi: 10.3389/fcvm.2022.856870
86. Panuccio G, Neri G, Macrì LM, Salerno N, De Rosa S, Torella D. Use of impella device in cardiogenic shock and its clinical outcomes: a systematic review and meta-analysis. Int J Cardiol Heart Vasc. (2022) 40:101007. doi: 10.1016/j.ijcha.2022.101007
87. Chioncel O, Parissis J, Mebazaa A, Thiele H, Desch S, Bauersachs J, et al. Epidemiology, pathophysiology and contemporary management of cardiogenic shock – a position statement from the heart failure association of the european society of cardiology. Eur J Heart Fail. (2020) 22:1315–41. doi: 10.1002/ejhf.1922
88. Lampert BC, Teuteberg JJ. Right ventricular failure after left ventricular assist devices. J Heart Lung Transplant. (2015) 34:1123–30. doi: 10.1016/j.healun.2015.06.015
89. Dang NC, Topkara VK, Mercando M, Kay J, Kruger KH, Aboodi MS, et al. Right heart failure after left ventricular assist device implantation in patients with chronic congestive heart failure. J Heart Lung Transplant. (2006) 25:1–6. doi: 10.1016/j.healun.2005.07.008
90. Farrar DJ. Ventricular interactions during mechanical circulatory support. Semin Thorac Cardiovasc Surg. (1994) 6:163–8.
91. Moon MR, Bolger AF, Deanda A, Komeda M, Daughters Ii GT, Nikolic SD, et al. Septal function during left ventricular unloading. Circulation. (1997) 95:1320–7. doi: 10.1161/01.CIR.95.5.1320
92. Shah P, Yuzefpolskaya M, Hickey GW, Breathett K, Wever-Pinzon O, Khue-Ton V, et al. Twelfth interagency registry for mechanically assisted circulatory support report: Readmissions after left ventricular assist device. Ann Thorac Surg. (2022) 113:722–37. doi: 10.1016/j.athoracsur.2021.12.011
93. Kormos RL, Antonides CFJ, Goldstein DJ, Cowger JA, Starling RC, Kirklin JK, et al. Updated definitions of adverse events for trials and registries of mechanical circulatory support: a consensus statement of the mechanical circulatory support academic research consortium. J Heart Lung Transplant. (2020) 39:735–50. doi: 10.1016/j.healun.2020.03.010
94. Kormos RL, Teuteberg JJ, Pagani FD, Russell SD, John R, Miller LW, et al. Right ventricular failure in patients with the heartmate ii continuous-flow left ventricular assist device: Incidence, risk factors, and effect on outcomes. J Thorac Cardiovasc Surg. (2010) 139:1316–24. doi: 10.1016/j.jtcvs.2009.11.020
95. Yoshioka D, Takayama H, Garan RA, Topkara VK, Han J, Kurlansky P, et al. Contemporary outcome of unplanned right ventricular assist device for severe right heart failure after continuous-flow left ventricular assist device insertion. Interact Cardiovasc Thorac Surg. (2017) 24:828–34. doi: 10.1093/icvts/ivw409
96. Khorsandi M, Schroder J, Daneshmand M, Bishawi M, Bouamra O, Winterton P, et al. Outcomes after extracorporeal right ventricular assist device combined with durable left ventricular assist device support. Ann Thorac Surg. (2019) 107:1768–74. doi: 10.1016/j.athoracsur.2018.11.051
97. Deschka H, Holthaus AJ, Sindermann JR, Welp H, Schlarb D, Monsefi N, et al. Can perioperative right ventricular support prevent postoperative right heart failure in patients with biventricular dysfunction undergoing left ventricular assist device implantation? J Cardiothorac Vasc Anesth. (2016) 30:619–26. doi: 10.1053/j.jvca.2016.02.023
98. Rame JE, Pagani FD, Kiernan MS, Oliveira GH, Birati EY, Atluri P, et al. Evolution of late right heart failure with left ventricular assist devices and association with outcomes. J Am Coll Cardiol. (2021) 78:2294–308. doi: 10.1016/j.jacc.2021.09.1362
99. Tsuji M, Kakuda N, Bujo C, Ishida J, Amiya E, Hatano M, et al. Sarcopenia and risk of infection in adult heart transplant recipients in japan. ESC Heart Fail. (2022) 9:1413–23. doi: 10.1002/ehf2.13835
100. Kang G, Ha R, Banerjee D. Pulmonary artery pulsatility index predicts right ventricular failure after left ventricular assist device implantation. J Heart Lung Transplant. (2016) 35:67–73. doi: 10.1016/j.healun.2015.06.009
101. Liang LW, Jamil A, Mazurek JA, Urgo KA, Wald J, Birati EY, et al. Right ventricular global longitudinal strain as a predictor of acute and early right heart failure post left ventricular assist device implantation. ASAIO J. (2022) 68:333–9. doi: 10.1097/MAT.0000000000001467
102. Karimov JH, Horvath D, Horvath D, Kado Y, Miyamoto T, Kuban B, et al. Mechanical circulatory support for biventricular heart failure using advanced ventricular assist device. J Heart Lung Transplant. (2020) 39(Suppl. 4):S414. doi: 10.1016/j.healun.2020.01.180
103. Miyamoto T, Kado Y, Horvath DJ, Kuban BD, Sale S, Fukamachi K, et al. An advanced universal circulatory assist device for left and right ventricular support: First report of an acute in vivo implant. JTCVS Open. (2020). doi: 10.1016/j.xjon.2020.06.006
104. Fukamachi K, Horvath DJ, Massiello AL, Fumoto H, Horai T, Rao S, et al. An innovative, sensorless, pulsatile, continuous-flow total artificial heart: device design and initial in vitro study. J Heart Lung Transplant. (2010) 29:13–20. doi: 10.1016/j.healun.2009.05.034
105. Miyamoto T, Byram N, Karimov JH, Adams J, Dessoffy R, Kuban BD, et al. The design modification of advanced ventricular assist device to enhance pulse augmentation and regurgitant flow shut-off. Artif Organs. (2019) 43:961–5. doi: 10.1111/aor.13484
106. Joubert K, Harano T, Pilewski J, Sanchez PG. Oxy-rvad support for lung transplant in the absence of inferior vena cava. J Card Surg. (2020) 35:3603–5. doi: 10.1111/jocs.15040
107. Ahmad U, Khattab MA, Schaelte G, Goetzenich A, Foldenauer AC, Moza A, et al. Combining minimally invasive surgery with ultra-fast-track anesthesia in heartmate 3 patients: a pilot study. Circ Heart Fail. (2022) 15:e008358. doi: 10.1161/CIRCHEARTFAILURE.121.008358
108. Coromilas EJ, Takeda K, Ando M, Cevasco M, Green P, Karmpaliotis D, et al. Comparison of percutaneous and surgical right ventricular assist device support after durable left ventricular assist device insertion. J Card Fail. (2019) 25:105–13. doi: 10.1016/j.cardfail.2018.12.005
109. Amancherla K, Patel J, Holder T, O'Leary J. Abstract 14670: a meta-analysis of survival outcomes between two uncommon mechanical circulatory support device combinations. Circulation. (2020) 142(Suppl. 3):A14670-A. doi: 10.1161/circ.142.suppl_3.14670
110. Corporation, USCT,. Heartmate 3™ Left Ventricular Assist System Instructions for Use 2017 Available online at: https://www.accessdata.fda.gov/cdrh_docs/pdf16/P160054C.pdf (accessed June 9, 2022).
111. Quader M, Toldo S, Chen Q, Hundley G, Kasirajan V. Heart transplantation from donation after circulatory death donors: present and future. J Card Surg. (2020) 35:875–85. doi: 10.1111/jocs.14468
Keywords: biventricular heart failure, right ventricular assist device, heart transplantation, ECMO, left ventricular assist device, mechanical circulatory support device
Citation: Kuroda T, Miyagi C, Fukamachi K and Karimov JH (2022) Mechanical circulatory support devices and treatment strategies for right heart failure. Front. Cardiovasc. Med. 9:951234. doi: 10.3389/fcvm.2022.951234
Received: 23 May 2022; Accepted: 29 August 2022;
Published: 23 September 2022.
Edited by:
Guido Tavazzi, University of Pavia, ItalyReviewed by:
Mossab Y. Saeed, Boston Children's Hospital and Harvard Medical School, United StatesKuo Yang Wang, China Medical University Hospital, Taiwan
Copyright © 2022 Kuroda, Miyagi, Fukamachi and Karimov. This is an open-access article distributed under the terms of the Creative Commons Attribution License (CC BY). The use, distribution or reproduction in other forums is permitted, provided the original author(s) and the copyright owner(s) are credited and that the original publication in this journal is cited, in accordance with accepted academic practice. No use, distribution or reproduction is permitted which does not comply with these terms.
*Correspondence: Jamshid H. Karimov, Karimoj@ccf.org