New insights of platelet endocytosis and its implication for platelet function
- 1Department of Cardiology, Cardiovascular Center, Henan Key Laboratory of Hereditary Cardiovascular Diseases, The First Affiliated Hospital of Zhengzhou University, Zhengzhou, Henan, China
- 2National Clinical Research Centre for Cardiovascular Diseases, Department of Cardiology, Beijing Anzhen Hospital, Capital Medical University, Beijing, China
Endocytosis constitutes a cellular process in which cells selectively encapsulate surface substances into endocytic vesicles, also known as endosomes, thereby modulating their interaction with the environment. Platelets, as pivotal hematologic elements, play a crucial role not only in regulating coagulation and thrombus formation but also in facilitating tumor invasion and metastasis. Functioning as critical components in the circulatory system, platelets can internalize various endosomal compartments, such as surface receptors, extracellular proteins, small molecules, and pathogens, from the extracellular environment through diverse endocytic pathways, including pinocytosis, phagocytosis, and receptor-mediated endocytosis. We summarize recent advancements in platelet endocytosis, encompassing the catalog of cargoes, regulatory mechanisms, and internal trafficking routes. Furthermore, we describe the influence of endocytosis on platelet regulatory functions and related physiological and pathological processes, aiming to offer foundational insights for future research into platelet endocytosis.
1 Introduction
Platelets are small, anuclear blood cells that derived from bone marrow megakaryocytes (MKs) or distant organs such as lung or liver, and play an essential role in hemostasis and thrombogenesis (1–3). At sites of vascular injury, platelets are instantly captured from blood stream, adhering to the subendothelial matrix at exposed endothelial surfaces to form thrombi (4). In addition to hemostatic function, platelets are instrumental in diverse physiological and pathological processes, including inflammation and immune response, antibacterial host defense, liver regeneration, tumorigenesis and metastasis (5). It has been proved that platelet activation is accompanied by the occurrence of exocytosis, during which platelet granules release their procoagulant contents to extracellular milieu. Furthermore, research on platelets has highlighted the critical role of endocytosis in their biological functions. Platelet endocytosis conception was first proposed more than 30 years ago, while the underlying mechanism of platelets endocytic pathways are relatively limited in definition (6).
Endocytosis usually refers to as the uptake of extracellular cargoes and the transport of cell surface receptor through various membrane-binding chambers named endosomes (7). With the plasma membrane invagination, extracellular substances are wrapped into cells and delivered to early endosomes. After that, early endosomes transmit the cargoes to distinct intracellular locations, such as degrading in the late endosomes, recycling to the platelet membrane, or secretion (8).
The phenomenon of platelet endocytosis has been acknowledged since the 1980s when the existence of active internal systems in platelets was first identified (7, 9, 10). Technological advancements have enabled the observation of platelets internalizing small particles (such as fibrinogen, IgG, and albumin) into membranous structures through a phagocytosis-like mechanism, as revealed by transmission electron microscopy (TEM) (11–15). Further research has unequivocally established the substantial role of endocytosis in platelet physiology. Beyond influencing the transport of granules and cargoes, platelet endocytosis is implicated in hemostasis and various pathophysiological processes (16). In this review, we aim to provide a concise summary of platelet endocytosis, covering the transported contents, molecular mechanisms, transport pathways, and its impact on platelet-associated physiological and pathological phenomena. This overview is intended to offer a systematic introduction and to foster novel insights for subsequent investigations into platelet endocytosis.
2 Cargoes uptake by platelets endocytosis
2.1 Plasma contents ingested by platelet granules
Platelets release more than 300 bioactive substances including multiple proteins, peptides and small molecules (nucleotides, serotonin, Ca2+, etc.), which mediate the diverse functions of platelets (17, 18). The bioactive substances are mainly packed in three kinds of intracellular secretory granules in platelet: α-granules, dense granules and lysosomes (19–21).
Platelet α-granules contain more than 280 proteins including coagulation factors, which are of fundamental importance in hemostasis (22). Some of these proteins are platelet-specific, for example, β3-thromboglobulin. Some counterparts are from plasma, including albumin, fibrinogen, fibronectin, von Willebrand factor (vWF), etc. Originally, it was believed that proteins in granules originated exclusively from de novo synthesis during megakaryocyte (MK) development. However, subsequent studies have revealed that circulating plasma proteins can be internalized into MKs or platelet granules via endocytic pathways, and then returned to the plasma (14, 23, 24).
Factor I (fibrinogen) is the primary adhesion protein secreted by platelets, accounting for 10% of α-granule proteins (12). Harrison provide the first clear evidence for in vivo endocytic uptake of fibrinogen from plasma by human platelet α-granules (23). Fibrinogen in platelets is not synthesized by MKs (25), and can only be obtained from plasma by endocytosis (12, 23, 26). It has been shown that factor III (Tissue Factor, TF) can be internalized by platelets into the open canalicular system (OCS) channels, followed by its accumulation in the platelet cytoplasm and, occasionally, within α-granules (24, 27, 28). However, the storage of TF in α-granule of unstimulated platelets is a subject of ongoing debate, since there is evidence of TF-mRNA in platelets (29). Factor V, necessary for the optimal conversion of prothrombin to thrombin, is present in significant concentrations in blood platelets and localized within the α-granules of unstimulated platelets. Research has localized factor V within the α-granules of unstimulated platelets (30, 31). Different from fibrinogen, factor V was endocytosed by MKs, but not by platelets (32). In addition to these coagulation factors, vascular endothelial growth factor (VEGF) (33), intravenously injected albumin, immunoglobulin G (14), insulin-like growth factor (IGF) I and IGF-binding protein-3 are also known to be ingested by MKs and incorporated into platelet α-granules (34), highlighting the complex and selective nature of protein uptake by platelets.
Dense granules within platelets contain small molecular substances, categorically divided into four primary types: 1. Nucleotides, including Adenosine Diphosphate (ADP) and Adenosine Triphosphate (ATP); 2. Amines, exemplified by serotonin; 3. Cations, such as calcium ions; and 4. Phosphates, notably pyrophosphate and polyphosphates (35). 5-hydroxytryptamine (5-HT), also known as serotonin, is a neurotransmitter. Platelets do not synthesize 5-hydroxytryptamine (5-HT), commonly known as serotonin, but rather acquire it from the bloodstream via the serotonin transporter (SERT), subsequently storing it in their dense granules (36). Serving as the primary carrier of serotonin in the blood, platelets play a pivotal role. The release of serotonin from platelets is crucial in enhancing the wound healing process in various organs (37).
2.2 Endocytic recycling surface receptors in platelets
A variety of receptors are expressed on the surface of platelets, enabling the recognition of extracellular ligands, matrix components, and receptors on other cells. Upon platelet activation, extracellular ligands bind to these surface receptors, providing a physical anchor and triggering the intracellular signaling events (38). Moreover, stimulation by agonist such as thrombin and ADP causes migration of platelet receptors from the interior to the cell surface and re-distribution (39–41). The dynamic turnover of endocytic and exocytic receptor trafficking contributes to cell invasion, metastasis and cytokinesis (42).
2.2.1 Integrin αIIbβ3
Integrins are a family of adhesive receptors whose functional regulation depends not only on the conformational change but also via integrin trafficking (43). Recycling of integrins can rapidly deliver integrins back to the plasma membrane, thus providing the cell with a constant fresh reservoir for new adhesions (38).
Integrin αIIbβ3, uniquely expressed by platelets, serves as a key adhesion molecule pivotal in thrombosis control (44). Previous study has shown that there is a dynamic pool of αIIbβ3 in human platelets that is obviously internalized through the endocytic vesicles formation. Intriguingly, upon thrombin stimulation, these vesicles are capable of migrating to the cell surface (45). Consequently, the internalization process of integrin αIIbβ3 in platelets may constitute a regulatory mechanism for adhesion receptors (46). Huang et al. have elucidated the crucial role of αIIbβ3 endocytosis in platelets and proved that changes of integrin αIIbβ3 trafficking may influence platelet functions, particularly in terms of spreading and clot retraction (47).
2.2.2 P2Y1 and P2Y12 purinergic receptors
ADP is of great significance for platelets activation. Two types of G protein-coupled receptors (GPCRs) expressed on platelet surface, P2Y1 and P2Y12, are activated by ADP (48, 49). Upon stimulation, P2Y1 is coupled to Gq and PLCβ, while P2Y12 is negatively coupled to adenylyl cyclase by Gi) thus promoting complete platelet aggregation. ADP induced platelet activation is usually initiated by P2Y1 and amplified by P2Y12 receptor (50).
Mundell and colleagues have showed that both P2Y1 and P2Y12 could be internalized rapidly in human platelets by a radioligand binding approach (51). Their findings also revealed that inhibitors of protein kinase C (PKC) significantly diminish ADP-induced internalization of the P2Y1 receptor, while having no substantial effect on the P2Y12 receptor (51). With further research, they demonstrated that the endocytosis of P2Y1 and P2Y12 receptors relies on dynamin, which is indispensable for resensitization of responses (50). An intact putative postsynaptic density 95/disc large/zonula occludens-1 (PDZ)-binding motif is essential for proper internalization and subsequent recycling of the P2Y12 purinoceptor in human platelets (52). Moreover, The PDZ-binding protein Na+/H+ exchanger regulatory factor (NHERF) binds to the P2Y12 receptor to promote agonist-dependent internalization. In this process, arrestin can act as an adaptor to scaffold NHERF1 to these GPCRs thus motivating receptor internalization (53). Arf6 also participate in P2Y receptors internalization which will be discussed in the following text.
2.2.3 CLEC-2
The hemi-immunoreceptor tyrosine-based activation motif–containing C-type lectinlike receptor 2 (CLEC-2) is a transmembrane protein that highly expressed in platelets and plays crucial roles in platelet activation (54). Besides contribution to thrombosis, platelet CLEC-2 is also involved in the regulation of tumor metastasis, blood-lymphatic vascular development, and inflammatory bleeding (55). CLEC2 regulates platelet activation mainly by influencing a Src- and spleen tyrosine kinase (Syk)-dependent tyrosine phosphorylation cascades.
Lorenz et al. have showed that by injecting INU1 (a monoclonal anti-body) in vivo can specifically remove CLEC-2 from platelets and MKs. Nonetheless, this intervention was observed to cause a marked decrease in CLEC-2 levels, leading to thrombocytopenia, which poses a challenge for its therapeutic use (55). The research indicated that the reduction in CLEC-2 due to INU1 is primarily a consequence of receptor internalization within the platelets, governed by the activity of Src-family kinases. Intriguingly, while the Syk kinase is not crucial for the decrease in CLEC-2 triggered by INU1, it is vital for the onset of thrombocytopenia. Thus, selectively inhibiting platelet Syk can counteract the thrombocytopenia caused by INU1, without interfering with the process of CLEC-2 internalization (55).
2.2.4 GPIb
Platelet membrane glycoprotein (GP) Ib is a receptor for vWF. Binding of vWF to GPIb is important in the initiation of the process of platelet adhesion to damaged blood vessel walls (56). Previous studies have observed the presence of GPIb pools inside the platelets (57, 58). Redistribution of platelet surface and internal cisterna of GPIb was observed within platelets, during which endocytosis of GPIb receptor may occur (59). Jones et al. have established that platelet endothelial cell adhesion molecule-1 (PECAM-1) could regulate platelet GPIb internalization through dual AKT/protein kinase B/glycogen synthase kinase-3/dynamin-dependent and αIIbβ3-dependent pathways, so as to inhibit thrombin and vWF induced platelet activation (60).
2.2.5 Mpl/TPOR
Thrombopoietin (TPO), together with its receptor, Mpl, can facilitate megakaryocyte differentiation, promote survival and proliferation of hematopoietic stem cells and progenitor cells (61). Receptor-mediated internalization appears to be the primary regulating way of plasma TPO level. Mpl could be internalized in human platelets, without recycling to the cell surface (62). Recent research suggested that endocytosis of Mpl was restricted in Dynamin 2 (DNM2) knock out platelets, which also resulted in phosphorylation of the tyrosine kinase JAK2 and increased TPO levels (63). In conclusion, in MKs, DNM2-dependent endocytosis plays important roles during its development.
2.3 Virus and bacteria
In addition to the hemostatic function, platelets also act as innate immune cells involved in inflammation and immune response in vivo (64). Platelets express some pathogen recognition receptors, e.g., complement receptors, αIIbβ3, FcγRIIa, CLEC-2, GPIbα and dendritic cell-specific intercellular adhesion molecule-3-grabbing non-integrin (DC-SIGN), which enable platelets to detect and interact with bacterial and viral particles (65). Several studies have shown that platelets can bind and phagocytose infectious microorganisms (66–69). Platelets could promote the transport of infection in vivo by taking up viruses, and they also may help the host organism to resist infection. It is proposed that herpes virus with larger DNA, such as herpes simplex virus (HSV)-1, only bind to platelets without internalization (70–72). In contrast, some smaller RNA viruses, are endocytosed by platelets, as shown in Figure 1 and described below.
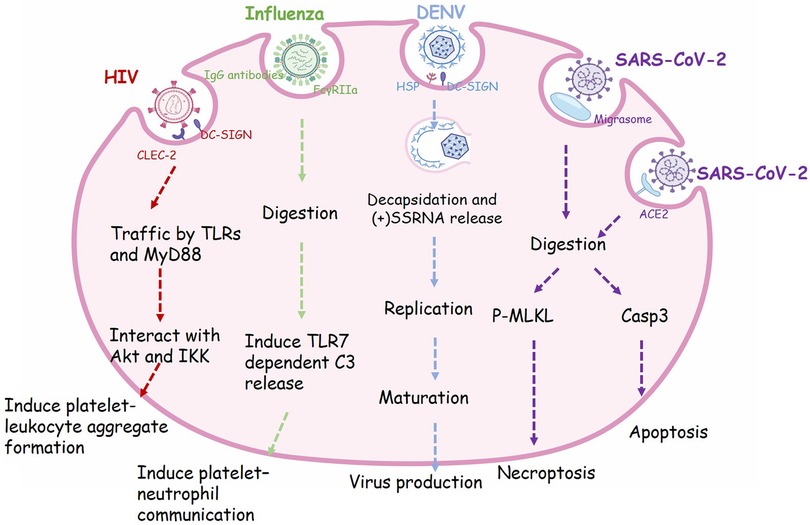
Figure 1. The phagocytic receptor, trafficking routes and regulatory functions of viral endocytosis in platelets. Many receptors expressed on the surface of platelets are involved in the process of virus phagocytosis. HIV enter platelets by binding to dendritic cell-specific ICAM-grabbing non-integrin (DC-SIGN) and C-type lectin-like receptor 2 (CLEC-2), then traffic via Toll like receptors (TLRs) and Myeloid Differentiation primary response protein 88 (MyD88) and interact with Akt and (IKK), finally induce granule release and platelet-leukocyte aggregate formation. Influenza viruses are recognized by IgG-specific antibodies and form an immune complex, which in turn is recognized by FcγRIIa, resulting in the internalization of this complex. Engulfment of influenza will activate platelet TLR7 and initiate granule release of complement C3 (C3), thus lead to NETosis. Dengue virus (DENV) binds to platelets via DC-SIGN and heparin sulfate proteoglycans (HSP). The viral particle go through decapsidation, ssRNA replication, maturation, and virus production. SARS-CoV-2 virions can be taken up into platelets through Angiotensin converting enzyme-2 (ACE2) or by attaching to microparticles. Phagocytosis of SARS-CoV-2 will induce apoptosis mediated by caspase 3 (Caps-3) and necroptosis mediated by phosphorylation of mixed lineage kinase domain-like pseudokinase (MLKL), finally lead to programmed cell death in platelets.
2.3.1 Human immunodeficiency virus type I (HIV1)
HIV1 is a single positive-stranded RNA virus belonging to the Retroviridae family, genus Lentivirus. In 1990, Zucker-Franklin D et al. first observed internalization of HIV1 and other retroviruses in MKs and platelets (73). In vitro experiments, Flaujac and colleagues incubated platelets with HIV viruses for 30 min. They found that HIV was completely internalized, maintaining the integrity of its ultrastructure. Characteristic endocytic vacuoles containing HIV particles were found near the plasma membrane (66). In addition, the endocytosis of HIV also occurred in vivo after test in platelets from clinical patients with AIDS (67). HIV-1 can be completely encased in platelets, transported through the body by circulating platelets and protected from attack by the host immune system (64). Platelets that internalize viruses undergo activation and exhibit P-selectin expression on their membrane. Subsequently, these P-selectin positive platelets are recognized by macrophages and efficiently cleared from the circulation (66). The thrombocytopenia complication after HIV infection may be partly caused by this defense mechanism (66).
2.3.2 Influenza virus
Influenza is a respiratory virus, and infection of it will lead to barrier damage of epithelial-endothelial, allowing the entrance of virus into blood (68). In 1959, the incorporating of influenza virus into platelets was first observed by electron microscopy (74). Koupenova et al. proposed that incubation of platelets with H1N1 strain (WSN/33) leads to internalization of the viral particles by platelets, which is morphologically similar to phagocytosis, and the internalized virus are rapidly digested (75). As a matter of fact, almost all influenza A strains (H1N1, H5N1, and H3N2, etc.) can bind to platelets, albeit with varying affinities (H5N1 > H1N1 > H3N2) (76). Further studies are required to confirm whether and how these viruses are endocytosed into platelets. Overall, platelets may be a line of intravascular defense against influenza, as well as other immunity cells.
2.3.3 Dengue virus (DENV)
Dengue fever is a mosquito-borne viral disease mainly found in tropical and subtropical regions. Infection of dengue virus in patients will cause capillary leakage, bleeding, and organ compromise, and in severe cases, it can lead to shock or even death (77). Platelets are crucial in the pathogenesis of DENV, as the infection is often accompanied by thrombocytopenia (78). Therefore absolute platelet count is used as lab indication to examine the disease progression of DENV (77). Researches have established that dengue induces platelet activation, mitochondrial dysfunction and apoptosis (79, 80). It is also suggest that DC-SIGN may participate as a key receptor in DENV mediated platelet activation (79). Ayo Y. Simon et al. found that DENV in blood can utilize DC-SIGN and heparan sulfate proteoglycan as primary receptors to bind to platelets directly. Platelets could endocytose this virus, replicate and produce infectious DENV. Usually platelets serve as an important line of defense against blood-borne viruses. However dengue appears to take advantage of this feature by getting inside the platelets and replicating further, increasing the survival chances of virus (78). The mechanism of DENV taking up by platelets remains unclear and requires further study, which can serve as new target for the DENV treatment.
2.3.4 Severe acute respiratory syndrome coronavirus 2 (SARS-CoV-2)
SARS-CoV-2 is a new type of positive-sense single-stranded RNA beta coronavirus which induced coronavirus disease 2019 (COVID-19) pandemic (81). Infection of SARS-CoV-2 not only causes acute respiratory distress syndrome (ARDS), but also evokes a series of thrombotic complications, and leads to organ failure and even mortality (81, 82). These clinical complications of cardiovascular disease successfully raise concern about relationship between platelet activation and SARS-CoV-2.
Platelets in patients with COVID-19 exhibit hyper-activity, evidenced by elevated integrin αIIbβ3 activation and P-selectin expression (69). Observation of platelets from patients with severe SARS-CoV-2 infection showed viruses could enter platelets surface and intracellular platelets, suggesting that SARS-CoV-2 might be ingested by platelets. The mRNA traces of SARS-CoV-2 were detected in the isolated platelets, and virions could be observed by electron microscopy within platelets sections (69, 83, 84).
Milka Koupenova et al. found that the internalization and digestion of the SARS-CoV-2, changes in platelet morphology, and release of extracellular vesicles can be observed after co-incubating platelets with purified virions (85). Angiotensin converting enzyme-2 (ACE2) is recognized as the primary receptor for entry of SARS-CoV-2 into host cells (69). Koupenova and colleagues propose that both ACE2 dependent and independent endocytosis of SARS-CoV-2 occur in platelets. They also observed a class of microparticle related to platelets which might be migrasome (85). During cell migration, migrasome functions as local chemo-attractants and mediating release of cytoplasmic contents (86). Platelets can take up SARS-CoV-2 virions attached to microparticles (85). Following the internalization of SARS-CoV-2 by platelets, researchers have observed a colocalization of the virus with phospho-MLKL (mixed lineage kinase domain-like pseudokinase) and caspase 3. This interaction leads to a rapid onset of platelet cell death, during which the virus seems to fragment, inhibiting its further replication and spread. This suggests that platelets may play a protective role in the immune response against the virus. However, with the death of platelets, their internal active substances are also released, resulting in pro-thrombotic complications in COVID-19 patients.
2.3.5 Bacteria
During bacterial infection and sepsis, direct and indirect interactions through a wide range of cellular mechanisms between platelets and bacteria may contribute to thrombocytopenia (87). In the microvasculature, bacterial cells could activate platelets, and cause thrombosis (88). Several studies have shown that Staphylococcus aureus (S. aureus) can induce aggregation and ATP release of platelets, and it is occasionally observed that S. aureus can be internalized by individual platelets (89–91). Moreover, platelet activation could increase the internalization of bacteria. During endocytosis, platelets appeared to elongate pseudopods that surrounded the S. aureus and completely enclosed the particles in a vacuole, in a manner similar to phagocytes engulfing bacteria. In the final stage of endocytosis, S. aureus seems to be closely contact with α-granule secretory fibrinogen within platelets (67).
In addition to S. aureus (a gram-positive bacterium), Porphyromonas gingivalis (P. gingivalis, a gram-negative bacterium) have also been reported to interact with platelets and cause aggregation of platelets (92). Xiangfeng Li et al. found that during bacterial induced platelet activation, P. gingivalis was engulfed in the OCS, and then internalized into the platelet cytoplasm. Streptococci and other bacteria have also been proved to interplay with platelets (88), however the detailed process of bacterial endocytosis into platelets has not been discussed and awaits further study.
2.4 Drugs endocytosis by platelet as smart drug delivery system
The controlled and efficient delivery system of drugs is essential for the clinical treatment of disease. An intelligent and controllable drug delivery system that effectively targets specific organs or tissues should maintain its bioavailability and minimize side effects (93). Platelets are endowed with the capacities of storage, transport, and release, making them potential carries for drug delivery systems (94). Moreover, when the drug is loaded in platelets, it could be protected from the clearance of immune system, and thus may allow a prolonged circulation time in blood.
Previous study has shown a strong link between platelets and cancer (95). Tumor cells can induce platelet adhesion, aggregation and release. During this process, the drugs loaded in platelets are released along with particulate components to the tumor site (96). The endocytosis properties of platelets can be used to load drugs, providing a promising way for guiding drugs to the blood circulatory system and complex tumor microenvironment.
2.4.1 Doxorubicin
Doxorubicin (DOX) is a chemo-therapeutic drug used in the treatment of lymphoma, but it has many side effects such as short biological life time and cardiotoxicity due to non-specific bio-distribution (97). The utilization of DOX-loaded platelets as a drug delivery system, achieved by incubating doxorubicin (DOX) with a platelet suspension at 37°C, has been investigated in multiple studies, particularly focusing on lymphoma treatment (93, 96). By this means, the researchers successfully prolonged DOX retention time compared to synthetic drug delivery systems (96, 98). Dox-loaded platelets facilitate the gathering of intracellular drug through platelet aggregation caused by tumor cells and release DOX in a pH-controlled manner. This method could reduce the side reaction of chemotherapy drugs to the patient and improve the therapeutic effect in clinical treatment of lymphoma (99). However, the precise mechanism underlying platelets' internalization of doxorubicin (DOX) remains unclear, as it is not explicitly detailed in these studies. The process of DOX encapsulation within platelets likely involves intricate biochemical pathways, possibly engaging the open canalicular system (OCS) of the platelets (96, 98). This area warrants further investigation to elucidate the exact mechanisms involved.
2.4.2 Monoclonal antibody bevacizumab
Platelets are the main source of VEGF in blood. Platelets activation leads to release of growth factors from α-granule, including VEGF, which promotes angiogenesis (100). Bevacizumab is a monoclonal antibody that blocks VEGF and has been used in the treatment of cancer disease. Previous study demonstrated that platelets can take up bevacizumab (101). Immunofluorescence microscopy displayed that bevacizumab phagocytosed in platelets colocalized with P-selectin. They found that platelets from patients receiving bevacizumab treatment can endocytose bevacizumab (101). Platelet endocytosis is still worthy of further study in the direction of drug delivery with platelets as carriers, which is of great significance for targeted treatment of tumors and cardiovascular diseases.
3 Regulators of platelet endocytic machinery
Platelet endocytosis has been long recognized, however, the molecular machinery, remain understudied. Up to now, several molecules have been proved to be involved in the regulation of platelet endocytic mechanism (Figure 2). According to existing studies, multiple pathways of endocytosis could be broadly classified as clathrin-dependent and clathrin-independent internalization (102).
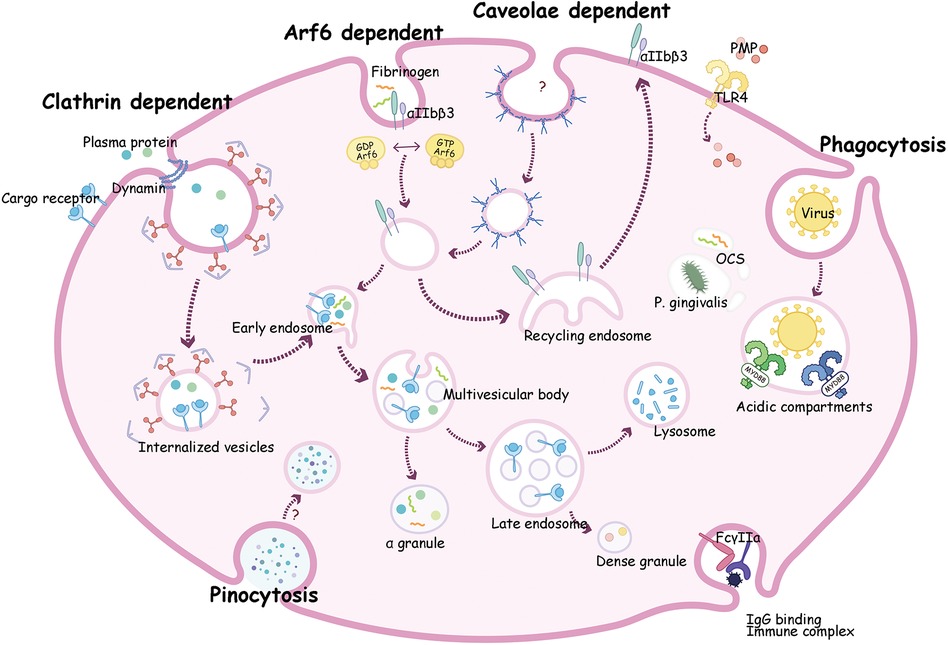
Figure 2. A composite description for endocytosis mechanism and transportation pathways in platelets. Clathrin dependent endocytosis can transport plasma proteins such as vWF and fibrinogen (Fg) with or without receptor into platelets. These proteins go through early endosome then be sorted to multivesicular bodies (MVB) and ultimately into α-granules for storage. Alternatively, cargoes can move into late endosomes then transit into dense granules or to lysosomes where it may be degraded or stored. Receptors such as P2Y12 and αIIbβ3 are taken up by Arf6, then circulate to recycling endosome, and finally return to the plasma membrane. Virions may enter platelets by phagocytosis, then bind to endosomal TLRs, and activate a myeloid differentiation primary response protein 88 (MyD88)- based signaling cascade, and finally been digested. Proteins like fibrinogen and bacteria such as P. gingivalis can be internalized into the open canalicular system (OCS) channels.
3.1 Clathrin dependent endocytosis
Clathrin is a protein which can form a polymer on the downside of the coated pit. Endocytosis mediated by clathrin usually depends on clathrin-coated vesicles (103). In platelets, clathrin-coated membranes were first noticed in the 1980s (9). The formation of clathrin encapsulated vesicles requires the following five steps: initial stage, selection of transport goods, coat assembly, scission and coating removal (103). In this process, extracellular molecules integrate with the ectodomain of receptors, then accumulate in coated pits on the cell membrane (16). The pits are further transformed into clathrin-coated vesicles with short-life (104). Subsequently, the coat of vesicles falls off, and the remaining portion fuses with endosomes and is transport along the endocytosis pathway (42, 105). By utilizing a novel inhibitor of clathrin named pitstop 2, Wen Gao et al. suggest that integrin αIIbβ3 trafficking mediated by clathrin controls spreading during platelet activation (42).
3.1.1 Dynamin
Dynamins (DNMs) are highly conserved mechanochemical GTPases regulate endocytosis and vesicle transport (63, 106). After the formation of clathrin-coated vesicles, DNM is recruited to promote the vesicle scission from the neck (42). There are three members of DNM family: DNM1, DNM2, and DNM3. Human platelets express all DNMs, whereas mouse platelets mainly express DNM2 (107, 108).
Platelets contain low levels DNM1, as it mainly expressed in brain (109). Previous studies have suggested DNM3 participate in MK maturation and platelet formation (110, 111). DNM2 mutations are associated with thrombocytopenia and hematopoietic diseases (112, 113). Bender et al. found that deletion of DNM2 in platelets significantly suppressed the endocytosis of the TPO receptor, Mpl. In conclusion, DNM2-dependent endocytosis is essential for megakaryopoiesis, thrombopoiesis, and bone marrow homeostasis (63).
3.1.2 Disabled-2 (Dab2)
Disabled-2 (Dab2) acts as an adaptor protein in clathrin-mediated endocytosis, and participates in transport of many receptors and intracellular signaling (114). Dab2 has two alternative splicing isoforms p82 and p59 (115). P82-Dab2 is mainly distributed in the cytosol and on α-granules of human platelets, which regulates fibrinogen binding and platelet aggregation (116). P59-Dab2 is abundant in mouse platelets, and it is essential for fibrinogen ingestion, RhoA-ROCK activation, ATP secretion, and integrin αIIbβ3-mediated signaling (117). Knock out of Dab2 specifically in mice platelets showed a bleeding tendency and impaired thrombosis. It has been proved that Dab2 shows strong interaction with Gα₁₂/₁₃-mediated thrombin signaling in hemostasis (118). Dab2 is a key player in the endocytosis of platelet Integrin-β3. Cheng-han Yu and colleagues have reported that the turnover of Integrin-β3 in platelets is modulated in a manner dependent on cell-matrix force interactions. Under the influence of traction forces, talin directly associates with Integrin-β3, leading to the formation and maturation of focal adhesions. Conversely, the absence of physical forces on RGD-glass triggers the recruitment of Dab2 and clathrin, culminating in the internalization of activated integrins (119).
3.1.3 IFN-induced transmembrane protein 3 (IFITM3)
IFITM3, an type I interferon (IFN)-responsive gene that plays critical roles in restriction of viral replication (120). During infection of dengue and SARS-CoV-2 virus, expression of IFITM3 significantly increased in MKs and platelets, thus limiting the entry and replication of virus in the cytoplasm of MKs (121). IFITM3 has been shown to interact with clathrin and αIIb and change their plasma membrane localization into lipid rafts, thereby mediating endocytic transport process in platelets (122). After administration of IFN in mice, endocytosis of fibrinogen and platelets reactivity significantly increased in an IFITM3-dependent manner. In nonviral sepsis, platelet IFITM3 expression elevated, leading to an increase fibrinogen content in platelets and thrombosis. These data support IFITM3 as a regulator of clathrin-dependent endocytosis in platelets, hyperreactivity, and thrombosis during inflammatory stress (122).
3.2 Clathrin independent endocytosis
The most common non clathrin-coated pits are known as caveolae (123). Caveolae-mediated endocytosis forms flask-shaped invaginations decorated with caveolin proteins on the surface of plasma membrane (124, 125). There are three types of caveolin proteins in mammals: caveolin1, caveolin 2, caveolin 3. Caveolin 3 is muscle specific, while the other two are widely expressed in non-muscle cells (123). Human platelets contain all three types of caveolin; however, whether and how these proteins coupled to endocytosis in platelets is still unknown (126).
RhoA and Cdc42 (small G proteins from the Rho family) have been widely involved in clathrin-independent endocytic regulation and the control of cytoskeletal recombination and intracellular signaling events (123). RhoA recruits the actin machinery to produce membrane invaginations in the process of endocytosis (16). Deficiency of RhoA not only significantly inhibits platelet aggregation, granule secretion, spreading on fibrinogen and other coagulation functions, but also induces macrothrombocytopenia (127). Meanwhile, Cdc42 plays crucial roles in platelet aggregation in response to collagen and integrin α2β1 activity (128). However, whether they showed priority for platelet endocytosis contents remains unclear and required further investigation.
3.2.1 The Arf GTPase family
The Ras-like, small guanosine triphosphate (GTP)–binding proteins named adenosine 5′-diphosphate–ribosylation factors (Arf's), play essential roles in intracellular trafficking (47). Based on sequence homology, they are separated into three types largely as Class I (Arfs 1–3), Class II (Arfs 4–5) and Class III (Arf6) (6). Among them, Arf6 has been most concerned in platelets. In rest platelets, Arf6 exists in an active, GTP-bound state. Upon stimulation by collagen and convulxin, the level of Arf6 in platelets rapidly decreased, by converting it to an inactive Arf6-GDP form (129). This process is regulated by the primary signaling activated by PAR receptor and GPVI, or contact-dependent signaling by integrin αIIbβ3 (130). When switch between active and inactive state, Arf6 facilitates internalization of ligand and recycling of receptors (131). In human platelets, Arf6 regulates the internalization and function of P2Y1 and P2Y12 purinoceptors. Moreover, Arf6 can regulate Nm23-H1 activation, a nucleoside diphosphate kinase, then facilitate fission of coated vesicles in a dynamin-dependent manner during endocytosis (132). Huang et al. generated mice with Arf6 deficiency in platelets, they found that hemostasis phenotypes (e.g., aggregation, ADP secretion, tail bleeding times, and occlusion time of arterial thrombosis) were normal, yet, Arf6 deficient platelets displayed enhanced spreading on fibrinogen and accelerated clot retraction. It is worth noting that fibrinogen uptake and storage were defective in Arf6−/− platelets (47). Thus, Arf6 may selectively regulate endocytic trafficking of platelet αIIbβ3 and the efficacy of platelet function. Arf1 and Arf3 are more abundant than Arf6 in platelets (6). In addition, several Arf regulators such as the GAPs, GEFs, ASAP1/2, ArfGAP1/2, and ARAP1 are present in platelets (107). Their regulatory roles in platelet endocytosis required further investigations.
3.2.2 Toll like receptor-4
Platelets can remove pathogens quickly through toll-like receptors (TLRs), and their endocytic and phagocytic abilities proved their association with the innate immune mechanisms (133). A variety of TLRs are expressed in platelets, some of which are expressed on platelet membranes and some of which are expressed inside platelets. Previous study revealed that platelets internalized circulating platelet microparticles (PMP) through TLR-4 and TLR-4 related components (133). It has been confirmed that PMP internalization in platelets could proceeds thrombus formation. Thus blockade of TLR-4 mediated endocytosis might be a potential target for antithrombotic therapies in pathological situations.
3.3 Phagocytosis and pinocytosis
In certain cells, extracellular substances waiting to be internalized are gradually surrounded by the invaginations forming on the plasma membrane, and then taken up into cells. This process is called phagocytosis (123). Phagocytosis usually refers to engulfment of large particles such as dead cells or invading microbes (16). Bacteria and viruses seem to be ingested by platelets in a process akin to true phagocytosis (67, 68, 134). Platelets express some pathogen recognition receptors, such as FcγRIIa, αIIbβ3, GPIbα, CLEC-2, and DC-SIGN, which can sense bacterial and viral particles and mediate phagocytosis of platelets (65). FcγRIIa is indispensable for IgG-containing complex clearance. Platelets can phagocytose immune complexes from circulation in a FcγRIIa-dependent manner (135, 136). It has been proved that during viral infection, influenza viruses are recognized by IgG-specific antibodies and form an immune complex, which in turn is recognized by FcγRIIa, resulting in the internalization of this complex, and activating platelets (68). Besides FcγRIIa, DC-SIGN, mainly expressed in macrophages and dendritic cells, also gets involved in in phagocytosis of platelets (66). As mentioned before, DENV directly bind to platelets utilizing DC-SIGN and heparan sulfate proteoglycan as primary receptors, and mediating entry of DENV into platelets (78, 137). Moreover, previous findings support that DC-SIGN can modulate HIV phagocytosis in platelets with CLEC-2 acting as its partner (138). In conclusion, these lectins are required for efficient binding of HIV and platelets, and might play important roles in phagocytosis of virus into platelets (Figure 1).
Different from phagocytosis, pinocytosis is another form of endocytosis that small particles are taken into the cell suspended within small vesicles. Pinocytosis usually participates in the absorption of extracellular fluids. It has been reported that fibrinogen might be taken up into platelets by pinocytosis, but its specific regulatory mechanisms and influencing factors need further research (31).
4 Endocytic trafficking routes in platelets
Platelets take up different cargoes, but what happens next? After particles enter platelets, they will go through different transport pathways, and exerting their respective regulatory functions.
Platelets contain distinct membrane-bound compartments (endosomes) that exercise the function of “transfer stations” for internalized cargo molecules (47). Platelets have a weak ability to synthesize proteins, so most cargo is taken from plasma. OCS is a complex a system of tunneling invaginations of the plasma membrane found in platelets that connects with membrane channels and cell surfaces to control the endocytosis and exocytosis (139, 140). The role of the OCS in the regulation of platelet functions can be outlined as follows: 1. The OCS is instrumental in the endocytosis of plasma proteins and other circulating molecules for example fibrinogen and TF (23, 24). This process allows platelets to acquire components that they do not synthesize themselves. 2. The OCS aids in the internalization of bacteria such as P. gingivalis and viral pathogens such as HIV (67, 92). This interaction is crucial for the immune functions of platelets, contributing to their role in inflammation and defense against infections (141). 3. The potential of the OCS in clinical treatments, particularly as a drug delivery system, is highlighted by its role in the encapsulation of drugs like DOX in platelets, as demonstrated in previous studies (96). This suggests that manipulating the OCS structure could be a novel approach in drug delivery applications. It has been observed that a distinct closed canalicular system of dispersed membrane-bound structures also exist in platelets (142). During early platelets activation, the closed canalicular system fuses with the plasma membrane and becomes open, thus these closed canalicular systems might represent endosomes in platelets (16, 142).
Platelets can sort endocytosed cargoes into different endosomal compartments (Figure 2). To analyze the sorting routes in platelets, researchers use anti-Rab4 (represent early endosomes), anti-Rab11 (recycling endosomes), and anti-Rab7 (late endosomes) antibodies as markers of different compartments. Both Rab4 and Rab11 compartments were present in platelets, but not overlapped, indicating that resting platelets contained both early and recycling endosomes (7, 8, 129). The endocytosis trafficking in platelet is time-dependent. As shown in Figure 2, internalized cargo transports through early endosomes, where it can be selected to recycling endosomes, then return back to the plasma membrane (e.g., αIIbβ3, P2Y12, TPOR), or to multivesicular bodies (MVB) and finally enter α-granules for storage (e.g., fibrinogen, vWF, thrombospondin-1). Either directly from early endosomes or through MVB, cargoes can also transit into late endosomes, and ultimately move into dense granules or lysosomes for storage or degradation (143, 144).
In the process of platelet endocytosis transport, a series of proteins are required to facilitate membrane fusion. Soluble N-ethylmaleimide-sensitive factor attachment protein receptor (SNARE) can mediate inter-compartmental transport in most cells, but its function in platelets is still under investigation. Cellubrevin/Vesicle-Associated Membrane Protein-3 (VAMP-3) is a v-SNARE protein localized to punctate structures within platelets. It has been reported that VAMP-3 not only regulate membrane, but also facilitate platelet aggregation and secretion (145, 146). Banerjee and colleagues have described the role of VAMP-3 in mediating endocytosis and endosomal trafficking in platelets, as well as controlling intracellular uptake/accumulation of fibrinogen and transferrin, platelet spreading, clot retraction, and proper regulation of TPOR signaling (7).
As mentioned before, TLR4 is involved in platelet endocytosis of extracellular pathogens. After the internalization of pathogens such as bacteria and viruses, platelets may also interact with other TLRs of endosomes. For example, TLR7 in the endosome recognizes single-stranded RNA and can be activated by retroviruses (147–149). Platelet TLR9 is detected both on the platelet membrane and in the endosome (150). HIV-1 pseudovirions incubation could induce endocytosis and trafficking of virions to an acidic, degradative compartment in platelets. Subsequently, platelets were activated with granule release, and platelet-leukocyte aggregate (PLA) formation through the pathway involved in activation of TLR7/TLR9 and myeloid differentiation primary response protein 88 (MyD88), as well as the participation of downstream interleukin 1 receptor associated kinase 4 (IRAK4), Akt, and IκB kinase (IKK) cascade signaling (65).
5 Perspectives and conclusion
In contrast with karyocytes, platelets are anucleated but relatively complex cells, with extensive endomembrane systems, and more hectic intracellular transport activities than ever thought. Endocytosis process has great impact on the up take, storage, and activity of proteins in platelet granules. This may have significant implications in clinical treatment of human diseases, as alterations in platelet granule proteins may contribute to thrombosis or bleeding. Endocytosis is a multi-step process that utilizes several routes of cargo entry/transit/exit (16), it also involved in various functions of platelets. Endocytosis might permit platelets to take up several prothrombotic and proinflammatory mediators (e.g., fibrinogen, vWF, IgG) from plasma; these messengers can then be stored into α-granules and later released during activation. Platelets endocytosis mediates surface receptors recycling: for example, integrin αIIbβ3 trafficking may affect platelet spreading and clot retraction, and internalization of both P2Y1 and P2Y12 receptors is required for resensitization of platelet responses (50). In addition, TPO receptor Mpl endocytosed by MK/platelets could regulate megakaryopoiesis, thrombopoiesis, and bone marrow homeostasis (63). Endocytosis may also enable platelets to function as immune cells since it can phagocyte bacteria and virus. Moreover, endocytic contents from the extracellular environment may provide platelets with the ability to differentially respond to corresponding physiological and pathological stimuli, thus modulating platelet interactions with other cells (16).
From the perspective of clinical disease treatment, platelet endocytosis may also play important roles. For example, in patients with nonviral sepsis, the expression of IFITM3 and contents of fibrinogen significantly increase in platelets, indicating that endocytosis mediated by IFITM3 may affect platelets reactivity and thrombosis during inflammatory stress (122). A recent report has suggested that endosomes play a crucial role in platelet activation, particularly in disease-specific contexts such as immune-mediated inflammatory diseases (151). Hydroxychloroquine (HCQ), the mainstay treatment for systemic lupus erythematosus, has been reported to function through limiting acidification of endosome in platelets (152). This underscores the considerable potential of platelet endosome research in revolutionizing diagnostics and therapeutic strategies across a spectrum of clinical disciplines. Additionally, a deeper understanding of platelet endocytosis and its integral role in platelet biogenesis is essential, not only for the validation of ex vivo platelet production for transfusion purposes but also for pioneering applications in the realms of inflammation, infection, and oncology. Circulating platelets, as temporally-regulated reservoirs, are replete with vital constituents sourced from the bone marrow and finely tuned by the circulatory environment. Looking ahead, we envision platelets, with their unique endocytic, transport, and release capabilities, as formidable candidates for the development of advanced, targeted drug delivery systems, especially in the context of clinical oncology.
In this review, we have encapsulated the latest research on platelet endocytosis, delving into the intricacies of endosome contents, the key regulatory elements, and the complex pathways of delivery. Research in the field of platelet endocytosis is still nascent, with numerous aspects of this process yet to be unraveled. As technological advancements continue to emerge, we anticipate a deeper and more comprehensive exploration of this domain. We hope that this review will provide researchers with a comprehensive insight into the intricacies of platelet endocytosis and transport, paving the way for further discoveries.
Author contributions
YZ: Formal Analysis, Funding acquisition, Project administration, Writing – original draft, Writing – review & editing. JD: Conceptualization, Writing – review & editing. MW: Investigation, Writing – review & editing. YL: Conceptualization, Formal Analysis, Writing – original draft.
Funding
The author(s) declare financial support was received for the research, authorship, and/or publication of this article.
This study was funded by the National Natural Science Foundation of China [82204385, 81903603], Henan Province Medical Science and Technology Key Project (Union construction) [LHGJ20220275].
Conflict of interest
The authors declare that the research was conducted in the absence of any commercial or financial relationships that could be construed as a potential conflict of interest.
Publisher's note
All claims expressed in this article are solely those of the authors and do not necessarily represent those of their affiliated organizations, or those of the publisher, the editors and the reviewers. Any product that may be evaluated in this article, or claim that may be made by its manufacturer, is not guaranteed or endorsed by the publisher.
References
1. Aggarwal A, Jennings CL, Manning E, Cameron SJ. Platelets at the vessel wall in non-thrombotic disease. Circ Res. (2023) 132(6):775–90. doi: 10.1161/CIRCRESAHA.122.321566
2. Lefrancais E, Ortiz-Munoz G, Caudrillier A, Mallavia B, Liu F, Sayah DM, et al. The lung is a site of platelet biogenesis and a reservoir for haematopoietic progenitors. Nature. (2017) 544(7648):105–9. doi: 10.1038/nature21706
3. Tilburg J, Becker IC, Italiano JE. Don't you forget about me(gakaryocytes). Blood. (2022) 139(22):3245–54. doi: 10.1182/blood.2020009302
4. Zhou Y, Hu M, Chen X, Wang S, Li J, Sa L, et al. Migfilin supports hemostasis and thrombosis through regulating platelet alphaIIbbeta3 outside-in signaling. Haematologica. (2020) 105(11):2608–18. doi: 10.3324/haematol.2019.232488
5. Jurk K, Kehrel BE. Platelets: physiology and biochemistry. Semin Thromb Hemost. (2005) 31(4):381–92. doi: 10.1055/s-2005-916671
6. Walsh TG, Li Y, Wersall A, Poole AW. Small GTPases in platelet membrane trafficking. Platelets. (2019) 30(1):31–40. doi: 10.1080/09537104.2018.1535703
7. Banerjee M, Joshi S, Zhang J, Moncman CL, Yadav S, Bouchard BA, et al. Cellubrevin/vesicle-associated membrane protein-3-mediated endocytosis and trafficking regulate platelet functions. Blood. (2017) 130(26):2872–83. doi: 10.1182/blood-2017-02-768176
8. Lowenstein CJ. VAMP-3 mediates platelet endocytosis. Blood. (2017) 130(26):2816–8. doi: 10.1182/blood-2017-10-808576
10. Klinger MH, Kluter H. Immunocytochemical colocalization of adhesive proteins with clathrin in human blood platelets: further evidence for coated vesicle-mediated transport of von Willebrand factor, fibrinogen and fibronectin. Cell Tissue Res. (1995) 279(3):453–7. doi: 10.1007/BF00318157
11. Zucker-Franklin D. Endocytosis by human platelets: metabolic and freeze-fracture studies. J Cell Biol. (1981) 91(3 Pt 1):706–15. doi: 10.1083/jcb.91.3.706
12. Handagama P, Scarborough RM, Shuman MA, Bainton DF. Endocytosis of fibrinogen into megakaryocyte and platelet alpha-granules is mediated by alpha IIb beta 3 (glycoprotein IIb-IIIa). Blood. (1993) 82(1):135–8. doi: 10.1182/blood.V82.1.135.bloodjournal821135
13. Handagama PJ, George JN, Shuman MA, McEver RP, Bainton DF. Incorporation of a circulating protein into megakaryocyte and platelet granules. Proc Natl Acad Sci U S A. (1987) 84(3):861–5. doi: 10.1073/pnas.84.3.861
14. Handagama PJ, Shuman MA, Bainton DF. Incorporation of intravenously injected albumin, immunoglobulin G, and fibrinogen in guinea pig megakaryocyte granules. J Clin Invest. (1989) 84(1):73–82. doi: 10.1172/JCI114173
15. Handagama P, Bainton DF, Jacques Y, Conn MT, Lazarus RA, Shuman MA. Kistrin, an integrin antagonist, blocks endocytosis of fibrinogen into guinea pig megakaryocyte and platelet alpha-granules. J Clin Invest. (1993) 91(1):193–200. doi: 10.1172/JCI116170
16. Banerjee M, Whiteheart SW. The ins and outs of endocytic trafficking in platelet functions. Curr Opin Hematol. (2017) 24(5):467–74. doi: 10.1097/MOH.0000000000000366
17. Golebiewska EM, Poole AW. Secrets of platelet exocytosis—what do we really know about platelet secretion mechanisms? Br J Haematol. (2013) 165(2):204–16. doi: 10.1111/bjh.12682
18. Golebiewska EM, Poole AW. Platelet secretion: from haemostasis to wound healing and beyond. Blood Rev. (2015) 29(3):153–62. doi: 10.1016/j.blre.2014.10.003
19. Rendu F, Brohard-Bohn B. The platelet release reaction: granules’ constituents, secretion and functions. Platelets. (2001) 12(5):261–73. doi: 10.1080/09537100120068170
20. Heijnen H, van der Sluijs P. Platelet secretory behaviour: as diverse as the granules…or not? J Thromb Haemost. (2015) 13(12):2141–51. doi: 10.1111/jth.13147
21. Blair P, Flaumenhaft R. Platelet alpha-granules: basic biology and clinical correlates. Blood Rev. (2009) 23(4):177–89. doi: 10.1016/j.blre.2009.04.001
22. Maynard DM, Heijnen HF, Horne MK, White JG, Gahl WA. Proteomic analysis of platelet alpha-granules using mass spectrometry. J Thromb Haemost. (2007) 5(9):1945–55. doi: 10.1111/j.1538-7836.2007.02690.x
23. Harrison P, Wilbourn B, Debili N, Vainchenker W, Breton-Gorius J, Lawrie AS, et al. Uptake of plasma fibrinogen into the alpha granules of human megakaryocytes and platelets. J Clin Invest. (1989) 84(4):1320–4. doi: 10.1172/JCI114300
24. Escolar G, Lopez-Vilchez I, Diaz-Ricart M, White JG, Galan AM. Internalization of tissue factor by platelets. Thromb Res. (2008) 122(Suppl 1):S37–41. doi: 10.1016/S0049-3848(08)70017-3
25. Handagama P, Rappolee DA, Werb Z, Levin J, Bainton DF. Platelet alpha-granule fibrinogen, albumin, and immunoglobulin G are not synthesized by rat and mouse megakaryocytes. J Clin Invest. (1990) 86(4):1364–8. doi: 10.1172/JCI114848
26. Handagama PJ, Shuman MA, Bainton DF. In vivo defibrination results in markedly decreased amounts of fibrinogen in rat megakaryocytes and platelets. Am J Pathol. (1990) 137(6):1393–9.2260627
27. Engelmann B, Luther T, Muller I. Intravascular tissue factor pathway–a model for rapid initiation of coagulation within the blood vessel. Thromb Haemost. (2003) 89(1):3–8. doi: 10.1055/s-0037-1613535
28. Muller I, Klocke A, Alex M, Kotzsch M, Luther T, Morgenstern E, et al. Intravascular tissue factor initiates coagulation via circulating microvesicles and platelets. FASEB J. (2003) 17(3):476–8. doi: 10.1096/fj.02-0574fje
29. Mezzano D, Matus V, Saez CG, Pereira J, Panes O. Tissue factor storage, synthesis and function in normal and activated human platelets. Thromb Res. (2008) 122(Suppl 1):S31–6. doi: 10.1016/S0049-3848(08)70016-1
30. Tracy PB, Mann KG. Abnormal formation of the prothrombinase complex: factor V deficiency and related disorders. Hum Pathol. (1987) 18(2):162–9. doi: 10.1016/s0046-8177(87)80334-9
31. Harrison P, Cramer EM. Platelet alpha-granules. Blood Rev. (1993) 7(1):52–62. doi: 10.1016/0268-960x(93)90024-x
32. Bouchard BA, Williams JL, Meisler NT, Long MW, Tracy PB. Endocytosis of plasma-derived factor V by megakaryocytes occurs via a clathrin-dependent, specific membrane binding event. J Thromb Haemost. (2005) 3(3):541–51. doi: 10.1111/j.1538-7836.2005.01190.x
33. Klement GL, Yip TT, Cassiola F, Kikuchi L, Cervi D, Podust V, et al. Platelets actively sequester angiogenesis regulators. Blood. (2009) 113(12):2835–42. doi: 10.1182/blood-2008-06-159541
34. Chan K, Spencer EM. Megakaryocytes endocytose insulin-like growth factor (IGF) I and IGF-binding protein-3: a novel mechanism directing them into alpha granules of platelets. Endocrinology. (1998) 139(2):559–65. doi: 10.1210/endo.139.2.5727
35. McNicol A, Israels SJ. Platelet dense granules: structure, function and implications for haemostasis. Thromb Res. (1999) 95(1):1–18. doi: 10.1016/s0049-3848(99)00015-8
36. Wolf K, Braun A, Haining EJ, Tseng YL, Kraft P, Schuhmann MK, et al. Partially defective store operated calcium entry and hem(ITAM) signaling in platelets of serotonin transporter deficient mice. PLoS One. (2016) 11(1):e0147664. doi: 10.1371/journal.pone.0147664
37. Mann DA, Oakley F. Serotonin paracrine signaling in tissue fibrosis. Biochim Biophys Acta. (2013) 1832(7):905–10. doi: 10.1016/j.bbadis.2012.09.009
38. De Franceschi N, Hamidi H, Alanko J, Sahgal P, Ivaska J. Integrin traffic—the update. J Cell Sci. (2015) 128(5):839–52. doi: 10.1242/jcs.161653
39. Michelson AD, Wencel-Drake JD, Kestin AS, Barnard MR. Platelet activation results in a redistribution of glycoprotein IV (CD36). Arterioscler Thromb. (1994) 14(7):1193–201. doi: 10.1161/01.atv.14.7.1193
40. Belitser N, Anischuk M, Veklich Y, Pozdnjakova T, Gorkun O. Fibrinogen internalization by ADP-stimulated blood platelets. Ultrastructural studies with fibrinogen-colloidal gold probes. Thromb Res. (1993) 69(5):413–24. doi: 10.1016/0049-3848(93)90230-l
41. Schober JM, Lam SC, Wencel-Drake JD. Effect of cellular and receptor activation on the extent of integrin alphaIIbbeta3 internalization. J Thromb Haemost. (2003) 1(11):2404–10. doi: 10.1046/j.1538-7836.2003.00417.x
42. Gao W, Shi P, Chen X, Zhang L, Liu J, Fan X, et al. Clathrin-mediated integrin alphaIIbbeta3 trafficking controls platelet spreading. Platelets. (2018) 29(6):610–21. doi: 10.1080/09537104.2017.1353682
43. Mana G, Valdembri D, Serini G. Conformationally active integrin endocytosis and traffic: why, where, when and how? Biochem Soc Trans. (2020) 48(1):83–93. doi: 10.1042/BST20190309
44. Shattil SJ, Newman PJ. Integrins: dynamic scaffolds for adhesion and signaling in platelets. Blood. (2004) 104(6):1606–15. doi: 10.1182/blood-2004-04-1257
45. Wencel-Drake JD. Plasma membrane GPIIb/IIIa. Evidence for a cycling receptor pool. Am J Pathol. (1990) 136(1):61–70.2404419
46. Wencel-Drake JD, Frelinger AL, Dieter MG, Lam SC. Arg-Gly-Asp-dependent occupancy of GPIIb/IIIa by applaggin: evidence for internalization and cycling of a platelet integrin. Blood. (1993) 81(1):62–9.8417803
47. Huang Y, Joshi S, Xiang B, Kanaho Y, Li Z, Bouchard BA, et al. Arf6 controls platelet spreading and clot retraction via integrin alphaIIbbeta3 trafficking. Blood. (2016) 127(11):1459–67. doi: 10.1182/blood-2015-05-648550
48. Kunapuli SP, Ding Z, Dorsam RT, Kim S, Murugappan S, Quinton TM. ADP receptors–targets for developing antithrombotic agents. Curr Pharm Des. (2003) 9(28):2303–16. doi: 10.2174/1381612033453947
49. Gachet C. Regulation of platelet functions by P2 receptors. Annu Rev Pharmacol Toxicol. (2006) 46:277–300. doi: 10.1146/annurev.pharmtox.46.120604.141207
50. Mundell SJ, Barton JF, Mayo-Martin MB, Hardy AR, Poole AW. Rapid resensitization of purinergic receptor function in human platelets. J Thromb Haemost. (2008) 6(8):1393–404. doi: 10.1111/j.1538-7836.2008.03039.x
51. Mundell SJ, Jones ML, Hardy AR, Barton JF, Beaucourt SM, Conley PB, et al. Distinct roles for protein kinase C isoforms in regulating platelet purinergic receptor function. Mol Pharmacol. (2006) 70(3):1132–42. doi: 10.1124/mol.106.023549
52. Nisar S, Daly ME, Federici AB, Artoni A, Mumford AD, Watson SP, et al. An intact PDZ motif is essential for correct P2Y12 purinoceptor traffic in human platelets. Blood. (2011) 118(20):5641–51. doi: 10.1182/blood-2011-02-336826
53. Nisar SP, Cunningham M, Saxena K, Pope RJ, Kelly E, Mundell SJ. Arrestin scaffolds NHERF1 to the P2Y12 receptor to regulate receptor internalization. J Biol Chem. (2012) 287(29):24505–15. doi: 10.1074/jbc.M112.347104
54. Suzuki-Inoue K, Fuller GL, Garcia A, Eble JA, Pohlmann S, Inoue O, et al. A novel syk-dependent mechanism of platelet activation by the C-type lectin receptor CLEC-2. Blood. (2006) 107(2):542–9. doi: 10.1182/blood-2005-05-1994
55. Lorenz V, Stegner D, Stritt S, Vogtle T, Kiefer F, Witke W, et al. Targeted downregulation of platelet CLEC-2 occurs through syk-independent internalization. Blood. (2015) 125(26):4069–77. doi: 10.1182/blood-2014-11-611905
56. Li Z, Delaney MK, O'Brien KA, Du X. Signaling during platelet adhesion and activation. Arterioscler Thromb Vasc Biol. (2010) 30(12):2341–9. doi: 10.1161/ATVBAHA.110.207522
57. Asch AS, Leung LL, Polley MJ, Nachman RL. Platelet membrane topography: colocalization of thrombospondin and fibrinogen with the glycoprotein IIb-IIIa complex. Blood. (1985) 66(4):926–34. doi: 10.1182/blood.V66.4.926.926
58. Wencel-Drake JD, Plow EF, Kunicki TJ, Woods VL, Keller DM, Ginsberg MH. Localization of internal pools of membrane glycoproteins involved in platelet adhesive responses. Am J Pathol. (1986) 124(2):324–34.2943164
59. Michelson AD, Adelman B, Barnard MR, Carroll E, Handin RI. Platelet storage results in a redistribution of glycoprotein Ib molecules. Evidence for a large intraplatelet pool of glycoprotein Ib. J Clin Invest. (1988) 81(6):1734–40. doi: 10.1172/JCI113513
60. Jones CI, Sage T, Moraes LA, Vaiyapuri S, Hussain U, Tucker KL, et al. Platelet endothelial cell adhesion molecule-1 inhibits platelet response to thrombin and von Willebrand factor by regulating the internalization of glycoprotein Ib via AKT/glycogen synthase kinase-3/dynamin and integrin alphaIIbbeta3. Arterioscler Thromb Vasc Biol. (2014) 34(9):1968–76. doi: 10.1161/ATVBAHA.114.304097
61. Hitchcock IS, Chen MM, King JR, Kaushansky K. YRRL motifs in the cytoplasmic domain of the thrombopoietin receptor regulate receptor internalization and degradation. Blood. (2008) 112(6):2222–31. doi: 10.1182/blood-2008-01-134049
62. Li J, Xia Y, Kuter DJ. Interaction of thrombopoietin with the platelet c-mpl receptor in plasma: binding, internalization, stability and pharmacokinetics. Br J Haematol. (1999) 106(2):345–56. doi: 10.1046/j.1365-2141.1999.01571.x
63. Bender M, Giannini S, Grozovsky R, Jonsson T, Christensen H, Pluthero FG, et al. Dynamin 2-dependent endocytosis is required for normal megakaryocyte development in mice. Blood. (2015) 125(6):1014–24. doi: 10.1182/blood-2014-07-587857
64. Chabert A, Hamzeh-Cognasse H, Pozzetto B, Cognasse F, Schattner M, Gomez RM, et al. Human platelets and their capacity of binding viruses: meaning and challenges? BMC Immunol. (2015) 16:26. doi: 10.1186/s12865-015-0092-1
65. Banerjee M, Huang Y, Joshi S, Popa GJ, Mendenhall MD, Wang QJ, et al. Platelets endocytose viral particles and are activated via TLR (toll-like receptor) signaling. Arterioscler Thromb Vasc Biol. (2020) 40(7):1635–50. doi: 10.1161/ATVBAHA.120.314180
66. Flaujac C, Boukour S, Cramer-Borde E. Platelets and viruses: an ambivalent relationship. Cell Mol Life Sci. (2010) 67(4):545–56. doi: 10.1007/s00018-009-0209-x
67. Youssefian T, Drouin A, Masse JM, Guichard J, Cramer EM. Host defense role of platelets: engulfment of HIV and Staphylococcus aureus occurs in a specific subcellular compartment and is enhanced by platelet activation. Blood. (2002) 99(11):4021–9. doi: 10.1182/blood-2001-12-0191
68. Bote J, Corkrey HA, Koupenova M. Human platelets and influenza virus: internalization and platelet activation. Platelets. (2022) 33(2):184–91. doi: 10.1080/09537104.2021.1961710
69. Zhang S, Liu Y, Wang X, Yang L, Li H, Wang Y, et al. SARS-CoV-2 binds platelet ACE2 to enhance thrombosis in COVID-19. J Hematol Oncol. (2020) 13(1):120. doi: 10.1186/s13045-020-00954-7
70. Bik T, Sarov I, Livne A. Interaction between vaccinia virus and human blood platelets. Blood. (1982) 59(3):482–7. doi: 10.1182/blood.V59.3.482.482
71. Forghani B, Schmidt NJ. Association of herpes simplex virus with platelets of experimentally infected mice. Arch Virol. (1983) 76(3):269–74. doi: 10.1007/BF01311111
72. Assinger A, Kral JB, Yaiw KC, Schrottmaier WC, Kurzejamska E, Wang Y, et al. Human cytomegalovirus-platelet interaction triggers toll-like receptor 2-dependent proinflammatory and proangiogenic responses. Arterioscler Thromb Vasc Biol. (2014) 34(4):801–9. doi: 10.1161/ATVBAHA.114.303287
73. Zucker-Franklin D, Seremetis S, Zheng ZY. Internalization of human immunodeficiency virus type I and other retroviruses by megakaryocytes and platelets. Blood. (1990) 75(10):1920–3. doi: 10.1182/blood.V75.10.1920.1920
74. Danon D, Jerushalmy Z, De Vries A. Incorporation of influenza virus in human blood platelets in vitro. Electron microscopical observation. Virology. (1959) 9:719–22. doi: 10.1016/0042-6822(59)90168-0
75. Koupenova M, Corkrey HA, Vitseva O, Manni G, Pang CJ, Clancy L, et al. The role of platelets in mediating a response to human influenza infection. Nat Commun. (2019) 10(1):1780. doi: 10.1038/s41467-019-09607-x
76. Jansen AJG, Spaan T, Low HZ, Di Iorio D, van den Brand J, Tieke M, et al. Influenza-induced thrombocytopenia is dependent on the subtype and sialoglycan receptor and increases with virus pathogenicity. Blood Adv. (2020) 4(13):2967–78. doi: 10.1182/bloodadvances.2020001640
77. Losada PX, DeLaura I, Narvaez CF. Dengue virus and platelets: from the biology to the clinic. Viral Immunol. (2022) 35(5):349–58. doi: 10.1089/vim.2021.0135
78. Rondina MT, Weyrich AS. Dengue virus pirates human platelets. Blood. (2015) 126(3):286–7. doi: 10.1182/blood-2015-05-647362
79. Hottz ED, Oliveira MF, Nunes PC, Nogueira RM, Valls-de-Souza R, Da Poian AT, et al. Dengue induces platelet activation, mitochondrial dysfunction and cell death through mechanisms that involve DC-SIGN and caspases. J Thromb Haemost. (2013) 11(5):951–62. doi: 10.1111/jth.12178
80. Hottz ED, Medeiros-de-Moraes IM, Vieira-de-Abreu A, de Assis EF, Vals-de-Souza R, Castro-Faria-Neto HC, et al. Platelet activation and apoptosis modulate monocyte inflammatory responses in dengue. J Immunol. (2014) 193(4):1864–72. doi: 10.4049/jimmunol.1400091
81. Manne BK, Denorme F, Middleton EA, Portier I, Rowley JW, Stubben C, et al. Platelet gene expression and function in patients with COVID-19. Blood. (2020) 136(11):1317–29. doi: 10.1182/blood.2020007214
82. Connors JM, Levy JH. COVID-19 and its implications for thrombosis and anticoagulation. Blood. (2020) 135(23):2033–40. doi: 10.1182/blood.2020006000
83. Zaid Y, Puhm F, Allaeys I, Naya A, Oudghiri M, Khalki L, et al. Platelets can associate with SARS-cov-2 RNA and are hyperactivated in COVID-19. Circ Res. (2020) 127(11):1404–18. doi: 10.1161/CIRCRESAHA.120.317703
84. Campbell RA, Boilard E, Rondina MT. Is there a role for the ACE2 receptor in SARS-CoV-2 interactions with platelets? J Thromb Haemost. (2021) 19(1):46–50. doi: 10.1111/jth.15156
85. Koupenova M, Corkrey HA, Vitseva O, Tanriverdi K, Somasundaran M, Liu P, et al. SARS-CoV-2 initiates programmed cell death in platelets. Circ Res. (2021) 129(6):631–46. doi: 10.1161/CIRCRESAHA.121.319117
86. Ma L, Li Y, Peng J, Wu D, Zhao X, Cui Y, et al. Discovery of the migrasome, an organelle mediating release of cytoplasmic contents during cell migration. Cell Res. (2015) 25(1):24–38. doi: 10.1038/cr.2014.135
87. Deppermann C, Kubes P. Platelets and infection. Semin Immunol. (2016) 28(6):536–45. doi: 10.1016/j.smim.2016.10.005
88. Yadav VK, Singh PK, Agarwal V, Singh SK. Crosstalk between platelet and bacteria: a therapeutic prospect. Curr Pharm Des. (2019) 25(38):4041–52. doi: 10.2174/1381612825666190925163347
89. Clawson CC. Platelet interaction with bacteria. 3. Ultrastructure. Am J Pathol. (1973) 70(3):449–71.4632008
90. Clawson CC, Rao GH, White JG. Platelet interaction with bacteria. IV. Stimulation of the release reaction. Am J Pathol. (1975) 81(2):411–20.811123
91. Clawson CC, White JG. Platelet interaction with bacteria. V. Ultrastructure of congenital afibrinogenemic platelets. Am J Pathol. (1980) 98(1):197–211.7350814
92. Li X, Iwai T, Nakamura H, Inoue Y, Chen Y, Umeda M, et al. An ultrastructural study of porphyromonas gingivalis-induced platelet aggregation. Thromb Res. (2008) 122(6):810–9. doi: 10.1016/j.thromres.2008.03.011
93. Sarkar S, Alam MA, Shaw J, Dasgupta AK. Drug delivery using platelet cancer cell interaction. Pharm Res. (2013) 30(11):2785–94. doi: 10.1007/s11095-013-1097-1
94. Shi Q, Montgomery RR. Platelets as delivery systems for disease treatments. Adv Drug Deliv Rev. (2010) 62(12):1196–203. doi: 10.1016/j.addr.2010.06.007
95. Contursi A, Grande R, Dovizio M, Bruno A, Fullone R, Patrignani P. Platelets in cancer development and diagnosis. Biochem Soc Trans. (2018) 46(6):1517–27. doi: 10.1042/BST20180159
96. Xu P, Zuo H, Chen B, Wang R, Ahmed A, Hu Y, et al. Doxorubicin-loaded platelets as a smart drug delivery system: an improved therapy for lymphoma. Sci Rep. (2017) 7:42632. doi: 10.1038/srep42632
97. Takemura G, Fujiwara H. Doxorubicin-induced cardiomyopathy from the cardiotoxic mechanisms to management. Prog Cardiovasc Dis. (2007) 49(5):330–52. doi: 10.1016/j.pcad.2006.10.002
98. White JG. Platelets are covercytes, not phagocytes: uptake of bacteria involves channels of the open canalicular system. Platelets. (2005) 16(2):121–31. doi: 10.1080/09537100400007390
99. Xiao G, Zhang Z, Chen Q, Wu T, Shi W, Gan L, et al. Platelets for cancer treatment and drug delivery. Clin Transl Oncol. (2022) 24(7):1231–7. doi: 10.1007/s12094-021-02771-x
100. Ferrara N, Kerbel RS. Angiogenesis as a therapeutic target. Nature. (2005) 438(7070):967–74. doi: 10.1038/nature04483
101. Verheul HM, Lolkema MP, Qian DZ, Hilkes YH, Liapi E, Akkerman JW, et al. Platelets take up the monoclonal antibody bevacizumab. Clin Cancer Res. (2007) 13(18 Pt 1):5341–7. doi: 10.1158/1078-0432.CCR-07-0847
102. Margadant C, Monsuur HN, Norman JC, Sonnenberg A. Mechanisms of integrin activation and trafficking. Curr Opin Cell Biol. (2011) 23(5):607–14. doi: 10.1016/j.ceb.2011.08.005
103. McMahon HT, Boucrot E. Molecular mechanism and physiological functions of clathrin-mediated endocytosis. Nat Rev Mol Cell Biol. (2011) 12(8):517–33. doi: 10.1038/nrm3151
104. Schmid SL. Clathrin-coated vesicle formation and protein sorting: an integrated process. Annu Rev Biochem. (1997) 66:511–48. doi: 10.1146/annurev.biochem.66.1.511
105. Irannejad R, Tsvetanova NG, Lobingier BT, von Zastrow M. Effects of endocytosis on receptor-mediated signaling. Curr Opin Cell Biol. (2015) 35:137–43. doi: 10.1016/j.ceb.2015.05.005
106. Ferguson SM, De Camilli P. Dynamin, a membrane-remodelling GTPase. Nat Rev Mol Cell Biol. (2012) 13(2):75–88. doi: 10.1038/nrm3266
107. Burkhart JM, Vaudel M, Gambaryan S, Radau S, Walter U, Martens L, et al. The first comprehensive and quantitative analysis of human platelet protein composition allows the comparative analysis of structural and functional pathways. Blood. (2012) 120(15):e73–82. doi: 10.1182/blood-2012-04-416594
108. Rowley JW, Oler AJ, Tolley ND, Hunter BN, Low EN, Nix DA, et al. Genome-wide RNA-seq analysis of human and mouse platelet transcriptomes. Blood. (2011) 118(14):e101–11. doi: 10.1182/blood-2011-03-339705
109. Ferguson SM, Brasnjo G, Hayashi M, Wolfel M, Collesi C, Giovedi S, et al. A selective activity-dependent requirement for dynamin 1 in synaptic vesicle endocytosis. Science. (2007) 316(5824):570–4. doi: 10.1126/science.1140621
110. Reems JA, Wang W, Tsubata K, Abdurrahman N, Sundell B, Tijssen MR, et al. Dynamin 3 participates in the growth and development of megakaryocytes. Exp Hematol. (2008) 36(12):1714–27. doi: 10.1016/j.exphem.2008.08.010
111. Wang W, Gilligan DM, Sun S, Wu X, Reems JA. Distinct functional effects for dynamin 3 during megakaryocytopoiesis. Stem Cells Dev. (2011) 20(12):2139–51. doi: 10.1089/scd.2011.0159
112. Zuchner S, Noureddine M, Kennerson M, Verhoeven K, Claeys K, De Jonghe P, et al. Mutations in the pleckstrin homology domain of dynamin 2 cause dominant intermediate Charcot-Marie-Tooth disease. Nat Genet. (2005) 37(3):289–94. doi: 10.1038/ng1514
113. Zhang J, Ding L, Holmfeldt L, Wu G, Heatley SL, Payne-Turner D, et al. The genetic basis of early T-cell precursor acute lymphoblastic leukaemia. Nature. (2012) 481(7380):157–63. doi: 10.1038/nature10725
114. Tao W, Moore R, Smith ER, Xu XX. Endocytosis and physiology: insights from disabled-2 deficient mice. Front Cell Dev Biol. (2016) 4:129. doi: 10.3389/fcell.2016.00129
115. Maurer ME, Cooper JA. Endocytosis of megalin by visceral endoderm cells requires the Dab2 adaptor protein. J Cell Sci. (2005) 118(Pt 22):5345–55. doi: 10.1242/jcs.02650
116. Huang CL, Cheng JC, Stern A, Hsieh JT, Liao CH, Tseng CP. Disabled-2 is a novel alphaIIb-integrin-binding protein that negatively regulates platelet-fibrinogen interactions and platelet aggregation. J Cell Sci. (2006) 119(Pt 21):4420–30. doi: 10.1242/jcs.03195
117. Tsai HJ, Tseng CP. The adaptor protein disabled-2: new insights into platelet biology and integrin signaling. Thromb J. (2016) 14(Suppl 1):28. doi: 10.1186/s12959-016-0101-5
118. Tsai HJ, Huang CL, Chang YW, Huang DY, Lin CC, Cooper JA, et al. Disabled-2 is required for efficient hemostasis and platelet activation by thrombin in mice. Arterioscler Thromb Vasc Biol. (2014) 34(11):2404–12. doi: 10.1161/ATVBAHA.114.302602
119. Yu C-h, Rafiq NBM, Cao F, Zhou Y, Krishnasamy A, Biswas KH, et al. Integrin-beta3 clusters recruit clathrin-mediated endocytic machinery in the absence of traction force. Nat Commun. (2015) 6(1). doi: 10.1038/ncomms9672
120. Diamond MS, Farzan M. The broad-spectrum antiviral functions of IFIT and IFITM proteins. Nat Rev Immunol. (2013) 13(1):46–57. doi: 10.1038/nri3344
121. Campbell RA, Schwertz H, Hottz ED, Rowley JW, Manne BK, Washington AV, et al. Human megakaryocytes possess intrinsic antiviral immunity through regulated induction of IFITM3. Blood. (2019) 133(19):2013–26. doi: 10.1182/blood-2018-09-873984
122. Campbell RA, Manne BK, Banerjee M, Middleton EA, Ajanel A, Schwertz H, et al. IFITM3 Regulates fibrinogen endocytosis and platelet reactivity in nonviral sepsis. J Clin Invest. (2022) 132(23). doi: 10.1172/JCI153014
123. Doherty GJ, McMahon HT. Mechanisms of endocytosis. Annu Rev Biochem. (2009) 78:857–902. doi: 10.1146/annurev.biochem.78.081307.110540
124. Parton RG, Simons K. The multiple faces of caveolae. Nat Rev Mol Cell Biol. (2007) 8(3):185–94. doi: 10.1038/nrm2122
125. Parton RG, Hanzal-Bayer M, Hancock JF. Biogenesis of caveolae: a structural model for caveolin-induced domain formation. J Cell Sci. (2006) 119(Pt 5):787–96. doi: 10.1242/jcs.02853
126. Jayachandran M, Miller VM. Human platelets contain estrogen receptor alpha, caveolin-1 and estrogen receptor associated proteins. Platelets. (2003) 14(2):75–81. doi: 10.1080/0953710031000080562
127. Pleines I, Hagedorn I, Gupta S, May F, Chakarova L, van Hengel J, et al. Megakaryocyte-specific RhoA deficiency causes macrothrombocytopenia and defective platelet activation in hemostasis and thrombosis. Blood. (2012) 119(4):1054–63. doi: 10.1182/blood-2011-08-372193
128. Pula G, Poole AW. Critical roles for the actin cytoskeleton and cdc42 in regulating platelet integrin alpha2beta1. Platelets. (2008) 19(3):199–210. doi: 10.1080/09537100701777303
129. Choi W, Karim ZA, Whiteheart SW. Arf6 plays an early role in platelet activation by collagen and convulxin. Blood. (2006) 107(8):3145–52. doi: 10.1182/blood-2005-09-3563
130. Karim ZA, Choi W, Whiteheart SW. Primary platelet signaling cascades and integrin-mediated signaling control ADP-ribosylation factor (Arf) 6-GTP levels during platelet activation and aggregation. J Biol Chem. (2008) 283(18):11995–2003. doi: 10.1074/jbc.M800146200
131. Rondina MT, Weyrich AS. Arf6 arbitrates fibrinogen endocytosis. Blood. (2016) 127(11):1383–4. doi: 10.1182/blood-2016-01-692145
132. Kanamarlapudi V, Owens SE, Saha K, Pope RJ, Mundell SJ. ARF6-dependent Regulation of P2Y receptor traffic and function in human platelets. PLoS One. (2012) 7(8):e43532. doi: 10.1371/journal.pone.0043532
133. Jerez-Dolz D, Torramade-Moix S, Palomo M, Moreno-Castano A, Lopez-Vilchez I, Hernandez R, et al. Internalization of microparticles by platelets is partially mediated by toll-like receptor 4 and enhances platelet thrombogenicity. Atherosclerosis. (2020) 294:17–24. doi: 10.1016/j.atherosclerosis.2019.12.017
134. Meseguer J, Esteban MA, Rodriguez A. Are thrombocytes and platelets true phagocytes? Microsc Res Tech. (2002) 57(6):491–7. doi: 10.1002/jemt.10102
135. Huang ZY, Chien P, Indik ZK, Schreiber AD. Human platelet FcgammaRIIA and phagocytes in immune-complex clearance. Mol Immunol. (2011) 48(4):691–6. doi: 10.1016/j.molimm.2010.11.017
136. Maugeri N, Rovere-Querini P, Evangelista V, Covino C, Capobianco A, Bertilaccio MT, et al. Neutrophils phagocytose activated platelets in vivo: a phosphatidylserine, P-selectin, and {beta}2 integrin-dependent cell clearance program. Blood. (2009) 113(21):5254–65. doi: 10.1182/blood-2008-09-180794
137. Simon AY, Sutherland MR, Pryzdial EL. Dengue virus binding and replication by platelets. Blood. (2015) 126(3):378–85. doi: 10.1182/blood-2014-09-598029
138. Chaipan C, Soilleux EJ, Simpson P, Hofmann H, Gramberg T, Marzi A, et al. DC-SIGN and CLEC-2 mediate human immunodeficiency virus type 1 capture by platelets. J Virol. (2006) 80(18):8951–60. doi: 10.1128/JVI.00136-06
139. White JG. Platelet membrane interactions. Platelets. (1999) 10(6):368–81. doi: 10.1080/09537109975843
140. Selvadurai MV, Hamilton JR. Structure and function of the open canalicular system—the platelet’s specialized internal membrane network. Platelets. (2018) 29(4):319–25. doi: 10.1080/09537104.2018.1431388
141. Seyoum M, Enawgaw B, Melku M. Human blood platelets and viruses: defense mechanism and role in the removal of viral pathogens. Thromb J. (2018) 16:16. doi: 10.1186/s12959-018-0170-8
142. Pokrovskaya ID, Aronova MA, Kamykowski JA, Prince AA, Hoyne JD, Calco GN, et al. STEM tomography reveals that the canalicular system and alpha-granules remain separate compartments during early secretion stages in blood platelets. J Thromb Haemost. (2016) 14(3):572–84. doi: 10.1111/jth.13225
143. Heijnen HF, Debili N, Vainchencker W, Breton-Gorius J, Geuze HJ, Sixma JJ. Multivesicular bodies are an intermediate stage in the formation of platelet alpha-granules. Blood. (1998) 91(7):2313–25. doi: 10.1182/blood.V91.7.2313
144. Grant BD, Donaldson JG. Pathways and mechanisms of endocytic recycling. Nat Rev Mol Cell Biol. (2009) 10(9):597–608. doi: 10.1038/nrm2755
145. Bernstein AM, Whiteheart SW. Identification of a cellubrevin/vesicle associated membrane protein 3 homologue in human platelets. Blood. (1999) 93(2):571–9. doi: 10.1182/blood.V93.2.571
146. Schraw TD, Rutledge TW, Crawford GL, Bernstein AM, Kalen AL, Pessin JE, et al. Granule stores from cellubrevin/VAMP-3 null mouse platelets exhibit normal stimulus-induced release. Blood. (2003) 102(5):1716–22. doi: 10.1182/blood-2003-01-0331
147. Barton GM. Viral recognition by toll-like receptors. Semin Immunol. (2007) 19(1):33–40. doi: 10.1016/j.smim.2007.01.003
148. Crozat K, Beutler B. TLR7: a new sensor of viral infection. Proc Natl Acad Sci U S A. (2004) 101(18):6835–6. doi: 10.1073/pnas.0401347101
149. Lund JM, Alexopoulou L, Sato A, Karow M, Adams NC, Gale NW, et al. Recognition of single-stranded RNA viruses by toll-like receptor 7. Proc Natl Acad Sci U S A. (2004) 101(15):5598–603. doi: 10.1073/pnas.0400937101
150. Thon JN, Peters CG, Machlus KR, Aslam R, Rowley J, Macleod H, et al. T granules in human platelets function in TLR9 organization and signaling. J Cell Biol. (2012) 198(4):561–74. doi: 10.1083/jcb.201111136
151. Scherlinger M, Richez C, Tsokos GC, Boilard E, Blanco P. The role of platelets in immune-mediated inflammatory diseases. Nat Rev Immunol. (2023) 23(8):495–510. doi: 10.1038/s41577-023-00834-4
Keywords: platelet, endocytosis, cargo contents, thrombosis, phagocytosis
Citation: Zhou Y, Dong J, Wang M and Liu Y (2024) New insights of platelet endocytosis and its implication for platelet function. Front. Cardiovasc. Med. 10:1308170. doi: 10.3389/fcvm.2023.1308170
Received: 6 October 2023; Accepted: 21 December 2023;
Published: 9 January 2024.
Edited by:
Cristina Tudoran, Victor Babes University of Medicine and Pharmacy, RomaniaReviewed by:
Larisa Anghel, Institute of Cardiovascular Diseases, RomaniaSilvio Antoniak, University of North Carolina at Chapel Hill, United States
© 2024 Zhou, Dong, Wang and Liu. This is an open-access article distributed under the terms of the Creative Commons Attribution License (CC BY). The use, distribution or reproduction in other forums is permitted, provided the original author(s) and the copyright owner(s) are credited and that the original publication in this journal is cited, in accordance with accepted academic practice. No use, distribution or reproduction is permitted which does not comply with these terms.
*Correspondence: Mengyu Wang fccwangmy@zzu.edu.cn Yangyang Liu fccliuyy8@zzu.edu.cn