Time course of cardiac rupture after acute myocardial infarction and comparison of clinical features of different rupture types
- 1Department of Cardiology, Shanxi Cardiovascular Hospital, Taiyuan, Shanxi, China
- 2Department of Medical Oncology, Beijing YouAn Hospital, Capital Medical University, Beijing, China
- 3Precision Laboratory of Vascular Medicine, Shanxi Cardiovascular Hospital, Taiyuan, Shanxi, China
Objective: This study aimed to investigate the time course of cardiac rupture (CR) after acute myocardial infarction (AMI) and the differences among different rupture types.
Method: We retrospectively analyzed 145 patients with CR after AMI at Shanxi Cardiovascular Hospital from June 2016 to September 2022. Firstly, according to the time from onset of chest pain to CR, the patients were divided into early CR (≤24 h) (n = 61 patients) and late CR (>24 h) (n = 75 patients) to explore the difference between early CR and late CR. Secondly, according to the type of CR, the patients were divided into free wall rupture (FWR) (n = 55) and ventricular septal rupture (VSR) (n = 90) to explore the difference between FWR and VSR.
Results: Multivariate logistic regression analysis showed that high white blood cell count (OR = 1.134, 95% CI: 1.019–1.260, P = 0.021), low creatinine (OR = 0.991, 95% CI: 0.982–0.999, P = 0.026) were independently associated with early CR. In addition, rapid heart rate (OR = 1.035, 95% CI: 1.009–1.061, P = 0.009), low systolic blood pressure (OR = 0.981, 95% CI: 0.962–1.000, P = 0.048), and anterior myocardial infarction (OR = 5.989, 95% CI: 1.978–18.136, P = 0.002) were independently associated with VSR.
Conclusion: In patients with CR, high white blood cell count and low creatinine were independently associated with early CR, rapid heart rate, low systolic blood pressure, and anterior myocardial infarction were independently associated with VSR.
1 Introduction
Cardiac rupture (CR) is a rare but severe complication of acute myocardial infarction (AMI), including free wall rupture (FWR), ventricular septal rupture (VSR), and papillary muscle rupture. With the advancement of reperfusion therapies (especially percutaneous coronary intervention), the mortality and complication rate of AMI have dropped remarkably. However, the mortality caused by CR remains high (1–3). As a rare complication, although most studies have investigated the risk factors of CR after AMI, but the time course of CR and the differences among different rupture types are still unknown. Therefore, we analyzed the data of CR patients from the Shanxi Cardiovascular Hospital to explore the time course of CR and the differences among different rupture types.
2 Materials and methods
We retrospectively analyzed 145 patients with CR after AMI from Shanxi Cardiovascular Hospital between June 2016 and September 2022, including 55 patients with FWR and 90 patients with VSR. The study was approved by the ethics committee of Shanxi Cardiovascular Hospital.
2.1 Definition
AMI was defined as the presence of clinical evidence of acute myocardial injury with acute myocardial ischaemia, with detectable increase and/or decrease in cardiac troponin, which at least one value above the 99th percentile of the upper reference limit, and at least one of the following: (1) Symptoms of acute myocardial ischemia; (2) New ischemic electrocardiographic changes; (3) Development of pathogenic Q waves; (4) Imaging evidence of new loss of viable myocardium or new regional wall motion abnormality; and (5) Coronary thrombus confirmed by coronary angiography or intracavitary imaging, or autopsy (4).
The diagnosis of FWR was based on sudden cardiogenic shock or hypotension and echocardiography indicating a large pericardial effusion. The diagnosis of VSR was based on an emerging systolic murmur, and echocardiography confirms a shunt at the ventricular level.
Early CR was defined as the time from onset of chest pain to CR ≤ 24 h. Late CR was defined as the time from onset of chest pain to CR > 24 h.
2.2 Data collection
We extracted the following data from the medical records: basic clinical characteristics of the patients, medical history, time from onset of chest pain to admission, vital signs at admission, haematological parameters, etc.
2.3 Statistical analysis
SPSS 19.0 software was used for statistical analysis. Normally distributed continuous variables were expressed using mean ± standard deviation and analyzed using a t-test. Non-normally distributed continuous variables were expressed using the median (interquartile range) and analyzed using the Mann–Whitney U-test. Dichotomous variables were expressed by percentage and analyzed using chi-square tests. Multivariate logistic regression models were constructed using the above variables with P < 0.1 to evaluate the differences between early CR and late CR, FWR and VSR. A two-sided P < 0.05 was considered statistically different.
3 Results
3.1 Time course of CR
Of the 145 patients with CR after AMI, 136 patients had detailed records of the time from onset of chest pain to CR. As shown in Figure 1A, CR occurred to 61 (44.9%) patients within 24 h of chest pain onset, 79 (58.1%) patients within the first 48 h, and 122 (89.7%) patients within the first week. As shown in Figure 1B, 6–12 h after chest pain onset is the peak time of CR occurrence.
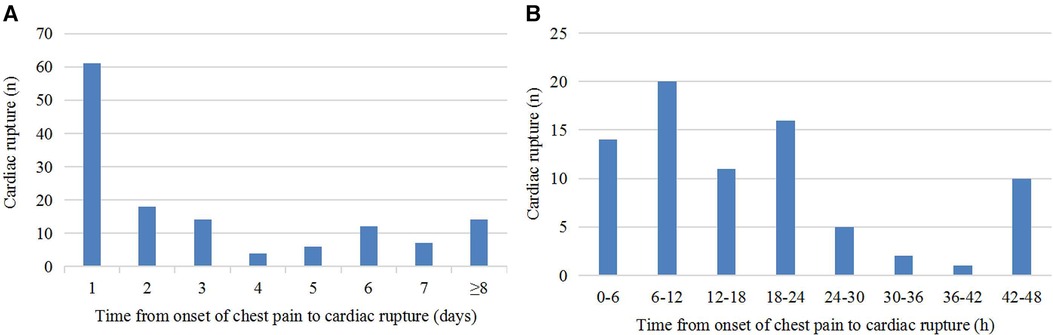
Figure 1. Time course of CR. (A) Time from onset of chest pain to CR. (B) Distribution of patients with CR within 48 h after onset of chest pain.
3.2 Comparison of early CR and late CR
Of the 145 patients with CR after AMI, 136 patients had detailed records of the time from onset of chest pain to CR, including 61 patients with early CR (≤24 h) and 75 patients with late CR (>24 h). The clinical characteristics of patients with early rupture and late rupture are shown in Table 1. Compared with the early CR group, the late CR group had a higher proportion of males and smokers. In terms of medications, P2Y12 receptor antagonists and statins were used more frequently in the late CR group. In addition, the incidence of abnormal Q waves was higher in the late CR group, while the white blood cell count in the late CR was significantly lower than in the early CR group. After adjusting for potential confounders, multivariate logistic regression analysis showed that high white blood cell count (OR = 1.134, 95% CI: 1.019–1.260, P = 0.021) and low creatinine (OR = 0.991, 95% CI: 0.982–0.999, P = 0.026) were independently associated with early CR (see Table 2).
3.3 Comparison of FWR and VSR
Of the 145 patients with CR after AMI, including 55 patients with FWR and 90 patients with VSR. The clinical characteristics of patients with FWR and VSR are shown in Table 3. Compared with the FWR group, the VSR group had faster heart rates, lower systolic blood pressure and higher urea nitrogen levels. In terms of medications, statins were used more frequently in the VSR group. In addition, anterior wall myocardial infarction was more frequent in the VSR patients, while the FWR patients presented more frequently with inferior wall myocardial infarction and posterior wall myocardial infarction. After adjusting for potential confounders, multivariate logistic regression analysis showed that rapid heart rate (OR = 1.035, 95% CI: 1.009–1.061, P = 0.009), low systolic blood pressure (OR = 0.981, 95% CI: 0.962–1.000, P = 0.048), anterior wall myocardial infarction (OR = 5.989, the 95% CI: 1.978–18.136, P = 0.002) were independently associated with VSR (see Table 4).
4 Discussion
CR is a rare but severe life-threatening complication of AMI. In the pre-reperfusion era, the incidence of CR after AMI was as high as 6.2%. With the development of reperfusion technology, especially the widespread use of percutaneous coronary intervention (PCI), the incidence of CR after AMI has been significantly reduced (5). Elbadawi et al. (2) enrolled 3,951,861 patients with ST-segment elevation myocardial infarction (STEMI) and 5,114,270 patients with non-ST-segment elevation myocardial infarction (NSTEMI) between 2003 and September 2015, and the incidence of CR was 0.27% and 0.06%, respectively. A multicentre study from Japan (6) found that as the proportion of PCI increased, the incidence of CR decreased from 3.7% between 1997 and 2004 to 1.9% between 2011 and 2014. Although the incidence of CR after AMI has declined, the death rate of CR can still be as high as 50% (1).
In this study, we selected patients with CR after AMI and attempted to compare the clinical characteristics of early CR and late CR, FWR and VSR. Multivariate logistic regression analysis showed that high white blood cell count (OR = 1.134, 95% CI: 1.019–1.260, P = 0.021), low creatinine (OR = 0.991, 95% CI: 0.982–0.999, P = 0.026) were independently associated with early CR, rapid heart rate (OR = 1.035, 95% CI: 1.009–1.061, P = 0.009), low systolic blood pressure (OR = 0.981, 95% CI: 0.962–1.000, P = 0.048), and anterior myocardial infarction (OR = 5.989, 95% CI: 1.978–18.136, P = 0.002) were independently associated with VSR.
FWR refers to the rupture of the left ventricular wall other than the interventricular septum, including the anterior, lateral, and posterior walls of the left ventricle. Rupture is common in the anterior and lateral walls of the anterior descending branch distribution, is more frequent in the apical region, and often occurs at the junction of normal and infarcted myocardium. VSR is caused by occlusion of the left anterior descending branch, the dominant right coronary artery, or the dominant left circumflex branch. VSR caused by anterior wall myocardial infarction is more often near the apex of the heart, and that caused by inferior wall myocardial infarction is at the base of the interventricular septum. As far as we know, cardiac rupture usually occurs within first week after AMI, especially within the first 24 h and 3–5 days (7), which means that the mechanism of early CR may be different from that of late CR. Becker and van Mantgem (8) categorized FWR into three types: Type I rupture is characterized by a sudden slit-like rupture that usually occurs in the acute phase of AMI (<24 h); Type II rupture is an erosive rupture, which usually occurs 24 h after AMI and is caused by erosion and chronic tearing of the infarct site; and type III rupture usually occurs in the late phase of AMI (which usually occurs more than one week later), and is a ventricular wall rupture due to overexpansion of the ventricular aneurysm. For early CR, AMI resulted in massive apoptosis of cardiomyocytes in the infarction area and decreased myocardial contractility and ventricular wall compliance. The infarct area showed increased brittleness compared to the non-infarct area. Therefore, when the myocardium contracts and diastoles, the non-infarcted area will form a shear effect with the infarct area, resulting in the occurrence of cardiac rupture (9). For advanced CR, a massive aggregate infiltration of inflammatory cells into the infarct zone begins 2–3 days after AMI with a peak around 1 week (10), among which white blood cells can release matrix metalloproteinases (MMPs). MMPs, a family of structurally similar zinc-dependent endopeptidases, can promote the degradation of extracellular matrix, activate cytokines and other MMP precursors, and play a key role in tissue remodeling (11). In the early stage of myocardial infarction, the massive infiltration of inflammatory cells will promote the activation of MMPs, leading to the massive degradation of the extracellular matrix such as myocardial collagen, fibronectin, and laminin, resulting in the loss of myocardial mesenchymal skeleton support and protection, and the disruption of the tissue structure, promoting disproportionate thinning and expansion of the infarcted areas and ultimately leading to CR (12, 13). In addition, animal studies have shown that myocardial tensile strength begins to decline within 24 h after the onset of AMI, and it can be reduced by 60% during the peak period (3–4 days) (14). Gong et al. (15) found that 6–24 h after STEMI was the peak period of FWR by analyzing 78 patients with FWR after STEMI. In addition, Shoji et al. (16) found that within 24 h and 6 days after AMI was the peak period of CR. This paper also confirms that 6–12 h after AMI is the peak time for the occurrence of CR.
Leukocytes play a critical role in the development of AMI. It has been shown that high leukocyte levels after AMI are closely associated with higher mortality, severe ventricular arrhythmias, cardiogenic shock, and acute heart failure (17). Dharma et al. (18) analyzed 585 patients with NSTEMI and found that high leukocyte levels (>11,000/μl) increased the incidence of major adverse cardiovascular events (MACE). In addition, lowering the white blood cell count significantly reduced the inflammatory response and improved cardiac function in rats with AMI (19). Qian et al. (20) found that AMI patients with elevated white blood cells were more likely to suffer CR. Our research showed that high white blood cell count was closely related to early CR (≤24 h), which may be related to the massive infiltration of white blood cells and the massive expression of MMP-9 in the early stage of myocardial infarction resulting in structural changes in the myocardial extracellular matrix. Renal dysfunction is considered a risk factor for cardiovascular disease and is strongly associated with poor prognosis of coronary heart disease (21). We found that patients with early CR (≤24 h) had lower creatinine levels. The effect of renal dysfunction on the occurrence time of CR after AMI still needs further study.
AMI is usually accompanied by sympathetic activation, which is mainly manifested by massive secretion of catecholamines, antidiuretic hormones, and increased activity of the renin-angiotensin-aldosterone system, resulting in increased cardiac afterload and rapid heart rate (22). The rapid heart rate will frequently pull the myocardium in the infarcted area and contribute to the occurrence of CR. It has been reported that for every 30 bpm increase in heart rate, there is a 1.32 times increase in the risk of CR (23). Early use of beta-blockers can reduce the risk of CR, and this beneficial effect is closely associated with the decrease in heart rate (24). A meta-analysis including four randomized controlled trials found that early use of beta-blockers reduced the incidence of CR after AMI by 32% (25). However, hypertension was recognized as a risk factor for CR in the past few decades, but due to recent changes in the definition of hypertension and the use of antihypertensive medications, hypertension is no longer considered to be significantly associated with CR (1). It is currently believed that good blood pressure reflects better ventricular function, and higher systolic blood pressure on admission is considered to be an important marker of good prognosis for CR (26). Anterior myocardial infarction is considered a risk factor for CR (1), and VSD is more common in anterior wall STEMI (2). The anterior upper 2/3 of the ventricular septum is supplied by the anterior descending branch, whereas the posterior lower 1/3 is supplied by the posterior descending branch. Acute lesions of the anterior descending branch can cause paradoxical movement of the infarcted ventricular septum during the systolic period, thus contributing to the development of VSR, which is more common in complete occlusion of the anterior descending branch (27). In this study, we found that rapid heart rate, low systolic blood pressure, and anterior myocardial infarction were strongly associated with VSR.
As we all know, primary PCI can reduce the risk of CR after AMI, whereas thrombolysis can increase this trend. Bueno et al. (28) analyzed 706 patients with AMI and found that the incidence of FWR was higher in patients treated with thrombolytic therapy (17.1%) than in patients who did not receive reperfusion therapy (7.9%) or who underwent PCI (4.9%) (P < 0.0001). However, it should be noted that the timing of thrombolytic therapy is closely related to the risk of CR. A meta-analysis (29) including 1,638 patients found that the risk of CR was 0.4 (95% CI: 0.17–0.93) for patients who received thrombolytic therapy at 7 h after AMI, 0.93 (95% CI: 0.53–1.6) at 11 h, and 3.21 (95% CI: 1.10–10.1) at 17 h. It indicates that thrombolytic therapy in the early stage of AMI can reduce the risk of CR, while late thrombolytic therapy can increase the risk of CR. In addition, different treatment strategies reveal different pathologic mechanisms of CR. Honda et al. (1) found a high incidence of myocardial hemorrhage in the infarcted area in 63 patients with CR who treated with PCI or thrombolysis. Becker type 1 and 2 ruptures were common in patients who received reperfusion therapy, whereas Becker type 3 rupture was common in patients who did not receive reperfusion therapy. However, because this study was retrospective and limited by clinical data, the number of thrombolysis and PCI patients were not counted. Further attention will be paid to this aspect in subsequent clinical studies.
There are some limitations in this study. Firstly, as a retrospective study, some information was unavailable or incomplete. In addition, due to the low incidence of CR, the sample size included in this study was small, and there were some errors and limitations in stating the conclusions. Therefore, multicenter studies are still needed for further evaluation.
5 Conclusion
In patients with CR, high white blood cell count and low creatinine were independently associated with early CR, rapid heart rate, low systolic blood pressure, and anterior myocardial infarction were independently associated with VSR.
Data availability statement
The raw data supporting the conclusions of this article will be made available by the authors, without undue reservation.
Ethics statement
The studies involving human participants were reviewed and approved by the Human Research Ethics Committee of the Shanxi Cardiovascular Hospital. Written informed consent for participation was not required for this study in accordance with the national legislation and the institutional requirements.
Author contributions
CL: Methodology, Writing – original draft. XW: Methodology, Writing – original draft. PY: Supervision, Writing – review & editing. RZ: Supervision, Writing – review & editing. LL: Investigation, Writing – review & editing. ZW: Investigation, Writing – review & editing. YG: Supervision, Writing – review & editing.
Funding
The author(s) declare that no financial support was received for the research, authorship, and/or publication of this article.
Conflict of interest
The authors declare that the research was conducted in the absence of any commercial or financial relationships that could be construed as a potential conflict of interest.
The reviewer [CY] declared a shared parent affiliation with the author [XW] to the handling editor at the time of review.
Publisher's note
All claims expressed in this article are solely those of the authors and do not necessarily represent those of their affiliated organizations, or those of the publisher, the editors and the reviewers. Any product that may be evaluated in this article, or claim that may be made by its manufacturer, is not guaranteed or endorsed by the publisher.
References
1. Honda S, Asaumi Y, Yamane T, Nagai T, Miyagi T, Noguchi T, et al. Trends in the clinical and pathological characteristics of cardiac rupture in patients with acute myocardial infarction over 35 years. J Am Heart Assoc. (2014) 3(5):e000984. doi: 10.1161/JAHA.114.000984
2. Elbadawi A, Elgendy IY, Mahmoud K, Barakat AF, Mentias A, Mohamed AH, et al. Temporal trends and outcomes of mechanical complications in patients with acute myocardial infarction. JACC Cardiovasc Interv. (2019) 12(18):1825–36. doi: 10.1016/j.jcin.2019.04.039
3. Puerto E, Viana-Tejedor A, Martínez-Sellés M, Domínguez-Pérez L, Moreno G, Martín-Asenjo R, et al. Temporal trends in mechanical complications of acute myocardial infarction in the elderly. J Am Coll Cardiol. (2018) 72(9):959–66. doi: 10.1016/j.jacc.2018.06.031
4. Thygesen K, Alpert JS, Jaffe AS, Chaitman BR, Bax JJ, Morrow DA, et al. Fourth universal definition of myocardial infarction (2018). J Am Coll Cardiol. (2018) 72(18):2231–64. doi: 10.1016/j.jacc.2018.08.1038
5. Figueras J, Alcalde O, Barrabés JA, Serra V, Alguersuari J, Cortadellas J, et al. Changes in hospital mortality rates in 425 patients with acute ST-elevation myocardial infarction and cardiac rupture over a 30-year period. Circulation. (2008) 118(25):2783–9. doi: 10.1161/CIRCULATIONAHA.108.776690
6. Koeda Y, Itoh T, Ishikawa Y, Morino Y, Mizutani T, Ako J, et al. A multicenter study on the clinical characteristics and risk factors of in-hospital mortality in patients with mechanical complications following acute myocardial infarction. Heart Vessels. (2020) 35(8):1060–9. doi: 10.1007/s00380-020-01586-0
7. Birnbaum Y, Fishbein MC, Blanche C, Siegel RJ. Ventricular septal rupture after acute myocardial infarction. N Engl J Med. (2002) 347(18):1426–32. doi: 10.1056/NEJMra020228
8. Becker AE, van Mantgem JP. Cardiac tamponade. A study of 50 hearts. Eur J Cardiol. (1975) 3(4):349–58.1193118
9. Matsusaka H, Ide T, Matsushima S, Ikeuchi M, Kubota T, Sunagawa K, et al. Targeted deletion of p53 prevents cardiac rupture after myocardial infarction in mice. Cardiovasc Res. (2006) 70(3):457–65. doi: 10.1016/j.cardiores.2006.02.001
10. Zidar N, Jeruc J, Balazic J, Stajer D. Neutrophils in human myocardial infarction with rupture of the free wall. Cardiovasc Pathol. (2005) 14(5):247–50. doi: 10.1016/j.carpath.2005.04.002
11. Bräuninger H, Krüger S, Bacmeister L, Nyström A, Eyerich K, Westermann D, et al. Matrix metalloproteinases in coronary artery disease and myocardial infarction. Basic Res Cardiol. (2023) 118(1):18. doi: 10.1007/s00395-023-00987-2
12. Becirovic-Agic M, Chalise U, Daseke MJ II, Konfrst S, Salomon JD, Mishra PK, et al. Infarct in the heart: what’s MMP-9 got to do with it? Biomolecules. (2021) 11(4):491. doi: 10.3390/biom11040491
13. Deleon-Pennell KY, Altara R, Yabluchanskiy A, Modesti A, Lindsey ML. The circular relationship between matrix metalloproteinase-9 and inflammation following myocardial infarction. IUBMB Life. (2015) 67(8):611–8. doi: 10.1002/iub.1408
14. Gao XM, Xu Q, Kiriazis H, Dart AM, Du XJ. Mouse model of post-infarct ventricular rupture: time course, strain- and gender-dependency, tensile strength, and histopathology. Cardiovasc Res. (2005) 65(2):469–77. doi: 10.1016/j.cardiores.2004.10.014
15. Gong W, Shi H, Yan M, Yan Y, Wang X, Li S, et al. Clinical manifestation, timing course, precipitating factors, and protective factors of ventricular free wall rupture following ST-segment elevation myocardial infarction. Int Heart J. (2020) 61(4):651–7. doi: 10.1536/ihj.19-541
16. Shoji K, Yanishi K, Kawamata H, Hori Y, Fujioka A, Kohno Y, et al. New risk factors for early- and late-onset cardiac rupture in ST-elevation myocardial infarction patients after primary percutaneous coronary intervention. J Cardiol. (2022) 79(3):400–7. doi: 10.1016/j.jjcc.2021.10.006
17. Ghaffari S, Nadiri M, Pourafkari L, Sepehrvand N, Movasagpoor A, Rahmatvand N, et al. The predictive value of total neutrophil count and neutrophil/lymphocyte ratio in predicting in-hospital mortality and complications after STEMI. J Cardiovasc Thorac Res. (2014) 6(1):35–41. doi: 10.5681/jcvtr.2014.007
18. Dharma S, Hapsari R, Siswanto BB, van der Laarse A, Jukema JW. Blood leukocyte count on admission predicts cardiovascular events in patients with acute non-ST elevation myocardial infarction. Int J Angiol. (2015) 24(2):127–32. doi: 10.1055/s-0035-1544178
19. Zhu G, Yao Y, Pan L, Zhu W, Yan S. Reduction of leukocyte counts by hydroxyurea improves cardiac function in rats with acute myocardial infarction. Med Sci Monit. (2015) 21:3941–7. doi: 10.12659/msm.893744
20. Qian G, Wu C, Chen YD, Tu CC, Wang JW, Qian YA. Predictive factors of cardiac rupture in patients with ST-elevation myocardial infarction. J Zhejiang Univ Sci B. (2014) 15(12):1048–54. doi: 10.1631/jzus.B1400095
21. Tsai IT, Wang CP, Lu YC, Hung WC, Wu CC, Lu LF, et al. The burden of major adverse cardiac events in patients with coronary artery disease. BMC Cardiovasc Disord. (2017) 17(1):1. doi: 10.1186/s12872-016-0436-7
22. McAlpine HM, Morton JJ, Leckie B, Rumley A, Gillen G, Dargie HJ. Neuroendocrine activation after acute myocardial infarction. Br Heart J. (1988) 60(2):117–24. doi: 10.1136/hrt.60.2.117
23. López-Sendón J, Gurfinkel EP, Lopez de Sa E, Agnelli G, Gore JM, Steg PG, et al. Factors related to heart rupture in acute coronary syndromes in the global registry of acute coronary events. Eur Heart J. (2010) 31(12):1449–56. doi: 10.1093/eurheartj/ehq061
24. Fox K, Borer JS, Camm AJ, Danchin N, Ferrari R, Lopez Sendon JL, et al. Resting heart rate in cardiovascular disease. J Am Coll Cardiol. (2007) 50(9):823–30. doi: 10.1016/j.jacc.2007.04.079
25. Gong W, Feng S, Wang X, Fan J, Li A, Nie SP. Beta-blockers reduced the risk of cardiac rupture in patients with acute myocardial infarction: a meta-analysis of randomized control trials. Int J Cardiol. (2017) 232:171–5. doi: 10.1016/j.ijcard.2017.01.035
26. Sulzgruber P, El-Hamid F, Koller L, Forster S, Goliasch G, Wojta J, et al. Long-term outcome and risk prediction in patients suffering acute myocardial infarction complicated by post-infarction cardiac rupture. Int J Cardiol. (2017) 227:399–403. doi: 10.1016/j.ijcard.2016.11.037
27. Yip HK, Fang CY, Tsai KT, Chang HW, Yeh KH, Fu M, et al. The potential impact of primary percutaneous coronary intervention on ventricular septal rupture complicating acute myocardial infarction. Chest. (2004) 125(5):1622–8. doi: 10.1378/chest.125.5.1622
28. Bueno H, Martínez-Sellés M, Pérez-David E, López-Palop R. Effect of thrombolytic therapy on the risk of cardiac rupture and mortality in older patients with first acute myocardial infarction. Eur Heart J. (2005) 26(17):1705–11. doi: 10.1093/eurheartj/ehi284
Keywords: cardiac rupture, acute myocardial infarction, CR, AMI, time course, clinical features
Citation: Liang C, Wang X, Yang P, Zhao R, Li L, Wang Z and Guo Y (2024) Time course of cardiac rupture after acute myocardial infarction and comparison of clinical features of different rupture types. Front. Cardiovasc. Med. 11:1365092. doi: 10.3389/fcvm.2024.1365092
Received: 3 January 2024; Accepted: 27 March 2024;
Published: 9 April 2024.
Edited by:
Hiroki Teragawa, JR Hiroshima Hospital, JapanReviewed by:
Chengzhi Yang, Department of Cardiology and Macrovascular Disease, Beijing Tiantan Hospital, Capital Medical University, ChinaManoj Kumar Mahata, Belle Vue Clinic, India
© 2024 Liang, Wang, Yang, Zhao, Li, Wang and Guo. This is an open-access article distributed under the terms of the Creative Commons Attribution License (CC BY). The use, distribution or reproduction in other forums is permitted, provided the original author(s) and the copyright owner(s) are credited and that the original publication in this journal is cited, in accordance with accepted academic practice. No use, distribution or reproduction is permitted which does not comply with these terms.
*Correspondence: Yanqing Guo gyqsxsxxgbyy@163.com
†These authors have contributed equally to this work and share first authorship