Low rates of myocardial fibrosis and ventricular arrhythmias in recreational athletes after SARS-CoV-2 infection
- 1Department of Cardiology, Antwerp University Hospital, Antwerp, Belgium
- 2Research Group Cardiovascular Diseases, Department GENCOR, University of Antwerp, Antwerp, Belgium
- 3Department of Internal Medicine, Ghent University, Ghent, Belgium
- 4Department of Cardiology, Jan Palfijn Hospital, Ghent, Belgium
- 5Exercise Physiology Research Group, Department of Movement Sciences, KU Leuven, Leuven, Belgium
- 6Department of Cardiology, Hartcentrum, Jessa Hospital, Hasselt, Belgium
- 7Faculty of Medicine and Life Sciences, Hasselt University, Hasselt, Belgium
- 8Artsenpraktijk Spikdorenveld, Westerlo, Belgium
- 9Department of Cardiology, Leuven University Hospitals, Leuven, Belgium
- 10Department of Cardiovascular Sciences, Faculty of Medicine, KU Leuven, Leuven, Belgium
- 11Department of Imaging and Pathology, KU Leuven, Leuven, Belgium
- 12Department of Radiology, University Hospitals Leuven, Leuven, Belgium
Introduction: High rates of cardiac involvement were reported in the beginning of the coronavirus disease 2019 (COVID-19) pandemic. This led to anxiety in the athletic population. The current study was set up to assess the prevalence of myocardial fibrosis and ventricular arrhythmias in recreational athletes with the recent severe acute respiratory syndrome coronavirus 2 (SARS-CoV-2) infection.
Methods: Consecutive adult recreational athletes (≥18 years old, ≥4 h of mixed type or endurance sports/week) underwent systematic cardiac evaluation after a prior confirmed COVID-19 infection. Evaluation included clinical history, electrocardiogram (ECG), 5-day Holter monitoring, and cardiac magnetic resonance (CMR) imaging with simultaneous measurement of high-sensitive cardiac Troponin I. Data from asymptomatic or mildly symptomatic athletes (Group 1) were compared with those with moderate to severe symptoms (Groups 2–3). Furthermore, a comparison with a historical control group of athletes without COVID-19 (Master@Heart) was made.
Results: In total, 35 athletes (18 Group 1, 10 female, 36.9 ± 2.2 years, mean 143 ± 20 days following diagnosis) were evaluated. The baseline characteristics for the Group 1 and Groups 2–3 athletes were similar. None of the athletes showed overt myocarditis on CMR based on the updated Lake Louise criteria for diagnosis of myocarditis. The prevalence of non-ischemic late gadolinium enhancement [1 (6%) Group 1 vs. 2 (12%) Groups 2–3; p = 0.603] or ventricular arrhythmias [1 Group 1 athlete showed non-sustained ventricular tachycardia (vs. 0 in Groups 2–3: p = 1.000)] were not statistically different between the groups. When the male athletes were compared with the Master@Heart athletes, again no differences regarding these criteria were found.
Conclusion: In our series of recreational athletes with prior confirmed COVID-19, we found no evidence of ongoing myocarditis, and no more detection of fibrosis or ventricular arrhythmias than in a comparable athletic pre-COVID cohort. This points to a much lower cardiac involvement of COVID-19 in athletes than originally suggested.
Introduction
In early 2020, immediately following the first reports of severe acute respiratory syndrome coronavirus-2 (SARS-CoV-2)-induced coronavirus disease (COVID)-19, signs of acute cardiac injury or myocarditis were reported to be seen in between 7.2% and 40.9% of (hospitalized) patients (1). These findings were confirmed in non-hospitalized patients, with cardiovascular magnetic resonance (CMR) studies showing cardiac involvement in up to (and even over) 50% of recovered patients, including a deterioration of ejection fraction (2, 3).
Subsequently, a small series of 26 athletes (15 male, mean age 19.5 years) who suffered from COVID-19 (12 of them symptomatic) was reported (4). Four athletes had CMR signs of myocarditis (15%), and eight additional athletes demonstrated late gadolinium enhancement (LGE) on CMR (31%). These observations led to a high level of anxiety in the athletic population, for both myocarditis and the presence of LGE after myocarditis have been associated with sudden cardiac death in athletes and non-athletes (5, 6). The frequent occurrence of palpitations after SARS-CoV-2 infection raised further concern, for the fear of ventricular arrhythmias (7).
This and other series were hindered by the non-uniform and often single modality (only CMR) evaluation of the study subjects, while consensus documents underscore the importance of multimodality evaluation of athletes with suspected pathology (8, 9). Therefore, we set up the current study to systematically assess the prevalence of myocardial inflammation, fibrosis, and arrhythmias in athletes after SARS-CoV-2 infection.
Methods
We used the “strengthening of the reporting of observational studies in the epidemiology cohort” (STROBE) checklist when writing this report (10). Approval of the local ethics committee was obtained. The trial was registered at www.clinicaltrials.gov (NCT04726150). All participants gave their written informed consent.
An “athlete” was defined as someone who performed endurance or mixed-type sports (11) for at least 4 h per week (12) and had done so for at least 5 consecutive years. For inclusion, SARS-CoV-2 infection had to be proven by polymerase chain reaction (PCR), serology, or chest computed tomography. The evaluation was performed at least 1 month after the onset of symptoms or the first positive polymerase chain reaction test.
Various sports confederations and their physicians were made aware of the project via email or word of mouth. Athletes who were willing to participate were directed toward a study website (www.covidex.be). This was set up in agreement with the most recent General Data Protection Regulation (GDPR) guidelines. Upon self-registration (through filling in name, date of birth, and email address) the secured online application on this website generated a unique code. This code could then be used by the athletes to gain access to a screening questionnaire via a separate link on the website. In this manner, the questionnaire data were coded and not directly linked to the personal data. The screening questionnaire was provided by REDCap, a web-based, electronic data capture tool for research studies (https://gbiomed.kuleuven.be/english/IT/it-solutions/Redcap) (13, 14). All research data were coded and stored in REDCap in accordance with GDPR.
Informed consent was obtained in two stages. The first stage consisted of a digital consent, stating that the athlete consented to be potentially contacted by the study office and with the use of his or her coded data for scientific purposes. Contact data for potential questions or withdrawal of consent was made available on the website. The second stage consisted of an in-person consent with the investigators. This stage applied only to those randomized to undergo further testing.
Exclusion criteria were known prior cardiac fibrosis, history of ventricular arrhythmias, known or newly diagnosed coronary artery disease, allergy or contraindications for gadolinium contrast, claustrophobia, and unwillingness or impossibility to give informed consent. Prior vaccination was not considered an exclusion criterion.
Three groups of patients were defined:
• Group 1: Asymptomatic or mildly symptomatic individuals. Mild symptoms included anosmia, ageusia, headache, mild fatigue, fever ≤3 days, myalgias ≤3 days, mild upper respiratory tract illness, and mild gastrointestinal illness.
• Group 2: Moderate symptoms and/or cardiac symptoms. Moderate symptoms included at least two of: persistent fever ≥4 days, chills ≥4 days, myalgias ≥4 days, lethargy impairing activities of daily living ≥4 days, dyspnea during activities of daily living ≥4 days, and chest tightness ≥4 days. Cardiac symptoms included dyspnea, exercise intolerance, chest tightness, dizziness, (pre)syncope, and (new onset) palpitations.
• Group 3: Any hospitalization for COVID-19 (whether or not on the intensive care unit).
In the first step, individuals not fulfilling the inclusion criteria and/or having exclusion criteria were excluded. Per protocol, all Group 3 subjects were included. Subsequently, self-registered athletes were randomly chosen for complete evaluation according to study protocol based on the number of available study slots in the central study site that needed to perform the CMR. Capacity was limited owing to pandemic-related restrictions and a high demand for CMR within a clinical context. The study evaluation comprised a thorough medical history, clinical examination, 12-lead electrocardiogram (ECG), laboratory testing for high-sensitive Troponin I (hsTnI; Atellica, Siemens®), a long-term (≥5 days) rhythm recorder (Rooti Rx, Healthstim), and a CMR.
Power calculations led us to aim for 73 inclusions, based on the 5% prevalence of non-ischemic fibrosis on CMR in our available athlete cohort (without COVID-19) (15) vs. a previously reported prevalence of at least 20% (as a conservative estimate) in COVID-19 athletes (3, 4). This sample size was calculated to achieve a power of 0.8 and a confidence level of 0.95.
The primary outcome measures were as follows:
• the proportion of athletes with positive updated Lake Louise (ULL) criteria (16) on CMR and
• the arrhythmic burden on Holter, defined as the average number of atrial and ventricular premature beats over 24 h.
Secondary endpoints consisted of persistent symptoms, ECG findings, and cardiac markers [high-sensitive cardiac Troponin I (hsTnI)].
As mentioned, findings of the present study could be compared with those of an available cohort of athletes included in the Master@Heart trial (17). That study included male endurance athletes between 45 and 70 years of age who performed either ≥6 h per week of running or ≥8 h per week of cycling with a control group of sedentary men (≤3 h of exercise per week). The subjects with cardiac risk factors were stringently excluded. The database consisted of 558 individuals at the time of analysis (18). From this database, athletes <50 years of age for whom CMR data were available were selected. Comparison was done with the male subjects of the current study.
Cardiac magnetic resonance
CMR scans were performed on a 1.5T scanner. The CMR protocol consisted of localizers, a balanced steady-state free precession cine short-axis stack, and three long-axis views (4-, 2-, and 3-chamber views), and native T1 mapping and T2 mapping of 5–7 short-axis slices covering the left ventricle. Approximately 10 min after intravenous injection of 0.2 mmol/kg gadolinium, LGE imaging was performed of the left ventricular short-axis stack and three apical long-axis views. All CMRs were analyzed using CVI42 software (Circle Cardiovascular Imaging). Ventricular volumes and function were derived from the short-axis cine stack. The ULL were evaluated as recommended (16). LGE was scored as present when seen in two orthogonal planes. LGE at the right ventricular insertion point was not regarded as significant myocardial fibrosis.
Statistical analysis
All analyses were performed using SPSS® 27.0.1.0. Continuous data were evaluated for normal distribution using the Shapiro–Wilk test. Normally distributed data were compared with an independent sample t-test, otherwise the Mann–Whitney U test was used. Frequencies were compared with the Chi-square test or Fisher exact test as appropriate. A two-tailed p < 0.05 was considered statistically significant. All data are reported as mean [standard error of mean (SEM)] unless stated otherwise.
Results
Between 9 March and 12 August 2021, a total of 137 individuals registered via the website. Registration occurred 124 ± 12 days (median 72, range 0–624 days) after SARS-CoV-2 infection, the mean age of the registrants was 36.7 ± 1.0 years, and 33 (24%) were women. After filtering for exclusion criteria (step 1, Figure 1) 112 patients were withheld for potential randomization. Of those, 39 were randomized following the protocol. One of those withdrew consent, hence 38 were scheduled for testing. Three of those eventually did not undergo CMR, resulting in inclusion of 35 patients in the final analysis. Of these, 18 athletes (4 female—22%) belonged to Group 1, 15 (6 female—40%) to Group 2, and 2 (both male) to Group 3.
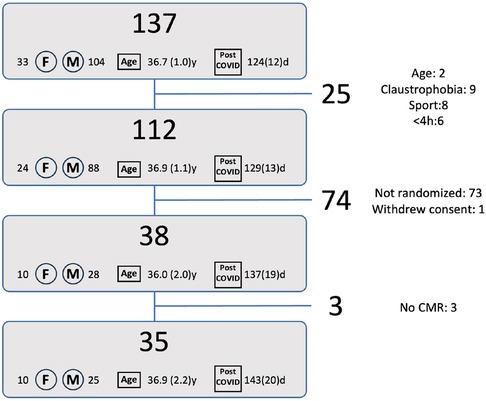
Figure 1. Flow diagram for patient inclusion. The numbers next to F and M represent the number of females/males, the number at “age” represent the average age [years(SE)], the numbers next to “post COVID” represent the time after initial diagnosis [days(SE)]. Reasons for exclusion are given in the right column: Step 1: age: 2 athletes were younger than 18 years, claustrophobia: 9 reported claustrophobia, sport: 8 did not perform an endurance or mixed type sport, <4 h: 6 athletes performed less than 4 h of sports per week. Step 2: Not randomized: 73 athletes were not randomized to undergo the study related tests. Withdrew consent: 1 athlete withdrew consent after randomization. Step 3: No CMR: 3 randomized athletes eventually did not undergo a CMR.
Table 1 summarizes the symptoms at the time of diagnosis. In Group 1, 4 athletes (22%) were asymptomatic, while 14 (78%) showed mild symptoms (8 fatigue, 7 anosmia/dysgeusia, 6 cough, 5 myalgia, 3 headache, 3 fever, and 3 chills). In Groups 2 and 3, none were asymptomatic (per protocol). In Group 2, seven (47%) had cardiac symptoms (five chest pain, four dyspnea, one presyncope, and two palpitations).
For further analysis Groups 2 and 3 were merged, combining moderate-to-severe symptomatic patients. Table 2 summarizes the baseline characteristics of these groups, demonstrating no statistically significant differences. Patients in Group 1 had a mean age of 35.2 ± 3.0 years, 14 (78%) were male and average body mass index was 22.5 ± 0.5 kg/m2. In Groups 2–3, the average age was 38.7 ± 3.2 years, 11 (65%) were men and the average body mass index was 22.6 ± 0.6 kg/m2 (all p = NS). Group 1 athletes performed an average of 9.6 ± 1.0 h of sports per week, while in Groups 2–3 this was 8.8 ± 1.1 h per week (p = 0.560). Group 1 contained a single former smoker (who quit smoking for more than 20 years), while in Groups 2–3, there were four former smokers (three had quit less than 1 year before inclusion, one more than 20 years). None of the athletes were current smokers. Overall, no differences in the presence of cardiac risk factors were found.
Primary outcome measures
Signs of myocarditis on CMR
In Group 1, CMR was performed 245 ± 27 days and in Groups 2–3, 209 ± 29 days after the diagnosis of SARS-CoV-2 infection (p = 0.384; overall range 35–499). The CMR-results are summarized in Table 3. The patients had generally mildly dilated left and right ventricles, as is commonly observed in athletes, without differences between Groups 1 and 2–3. None of the patients demonstrated signs of acute myocarditis based on the updated Lake Louise criteria. There were no patients with regional wall motion abnormalities of the left or right ventricle.
In Group 1, one male patient (6%) showed subtle midwall LGE of the basal inferolateral wall. In Groups 2–3, two male patients (12%) showed LGE: one patient had two small foci of midwall LGE in the basal inferoseptum and basal inferolateral wall (Figure 2), and one had subepicardial LGE of the basal inferolateral wall. There was no statistically significant difference in the presence of LGE between the two groups (p = 0.603). Inferior hinge point fibrosis was present in three athletes (8.5%): one athlete in Group 1 and two in Groups 2–3 (p = 0.603).
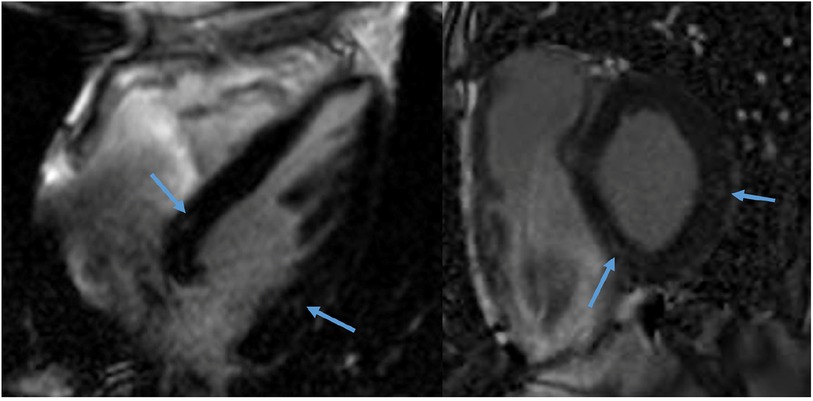
Figure 2. CMR of a 58-year-old male recreational athlete showing LGE. The late gadolinium enhancement (LGE) imaging showed two very small foci of midwall LGE (1.3% of total left ventricular mass) in the basal inferoseptum and basal (infero) lateral wall (arrows).
Arrhythmias
Both in Group 1 and Groups 2–3, 1 single athlete did not undergo Holter monitoring, resulting in 17 patients in Group 1 and 16 patients in Group 2–3 (Table 4). In Group 1, Holter monitoring was performed 280 ± 26 days after the diagnosis of SARS-CoV-2 infection, whereas the time interval was 214 ± 33 days in Groups 2–3 (p = 0.120). Group 1 patients had a median number [interquartile range (IQR)] of Ventricular ExtraSystoles (VES)/24 h of 1.6 (7). In Groups 2–3, this was 2.4 (15; p = 0.664). The range of VES/24 h was 0–492 in Group 1 and 0–513 in Groups 2–3. There was also no significant difference between the number of patients with more than 100 VES/24 h, more than 500 VES/24 h, patients with ventricular couplets, and patients with ventricular triplets. Only one Group 1 patient showed a non-sustained ventricular tachycardia (14 beats of monomorphic ventricular tachycardia during the night with a QRS duration of 120 ms and a very short RSnadir). This was a 41-year-old asymptomatic female cyclist with a total number of VES of 278 over 5 d and an entirely normal CMR. Similarly, no significant differences could be found in the occurrence of atrial extrasystoles between the two groups. In 1 Groups 2–3 patient, atrial fibrillation was detected. This patient had a history of atrial fibrillation already before the pandemic.
Secondary outcome measures
Electrocardiogram
All patients were in sinus rhythm at presentation. Two patients showed an incomplete right bundle branch block (one in each group). There were no patients with pathological ST-segment alterations. Three Groups 2–3 patients showed T-wave inversion (TWI) beyond V2. All three had normal CMRs (Table 5).
Cardiac troponin I levels
Mean hsTnI was 10.5 ± 2.0 ng/mL in 15 Group 1 patients and 8.7 ± 4.4 ng/mL in 9 Groups 2–3 patients (p = 0.540). None of the patients had an elevated hsTnI.
Comparison with healthy athletes
Of the 558 individuals in the Master@Heart database, CMR data had been analyzed for 214 subjects at the time of the present study. Of those, 34 were <50 years of age and were included before 1 January 2020 (i.e., before the start of the pandemic). Of those, 21 performed ≥4 h of endurance exercise per week. This group was compared with the male subjects (n = 25) from our study.
Baseline characteristics
Master@Heart subjects were on average 10 years older than the current study patients (47.9 ± 2.8 years vs. 37.1 ± 2.2 years; p < 0.001) and performed 3 h of sports per week more [median (IQR) 12.0 (4.0) vs. 9.0 (6.0); p = 0.019]. There were no other significant differences between the two groups (Table 6).
Signs of myocarditis on CMR
As in the current series, none of the Master@Heart athletes was positive for the ULL criteria. LGE was present in three patients in both groups: p = 1.000). In the Master@Heart population, one athlete had midwall LGE in the basal inferoseptum and inferior wall, one athlete had LGE in the midinferolateral and lateral wall, and one athlete had subepicardial LGE in the inferior wall (Table 7). Hinge point fibrosis was present in eight Master@Heart athletes (23.5%), as compared with the three in the current study (8.5%; p = 0.110).
In line with the higher number of hours of sports, the indexed end-diastolic ventricular volumes were higher (reaching statistical significance for the right ventricle) and left ventricular ejection fraction was significantly lower (51 ± 1% vs. 53 ± 1%) than in the Covidex group (p = 0.011).
Arrhythmias
In the Master@Heart athletes, average heart rate (HR) (63 ± 1 bpm vs. 69 ± 1 bpm; p = 0.003) and maximal HR (122 ± 5 as compared with 169 ± 4 bpm; p < 0.001) were significantly lower. There were no other significant differences between the cohorts (Table 8).
Discussion
In our series of recreational athletes with prior confirmed SARS-CoV-2 infection, we found no evidence of ongoing myocarditis, and no more detection of fibrosis or ventricular arrhythmias than in a comparable athletic pre-COVID-19 cohort, pointing to much lower cardiac involvement of COVID-19 in athletes than initially suggested.
Myocarditis
None of the athletes fulfilled the ULL criteria for myocarditis on average of 227 ± 20 days after SARS-CoV-2 infection. Other series with similar numbers of athletes also did not find any ULL positive individuals (19–21). Subsequent larger series equally demonstrated lower prevalences than reported in the initial reports (0.6%‒2.3%) (22–24). The timing of the CMR in our series was considerably later than in the other reports (which had mostly median intervals of 30 days) (25). Also, our subjects had a higher average age (36.9 ± 2.2 years as opposed to 20‒23 years in most other studies) and were recreational athletes (as opposed to professional or college athletes in the other series). These factors have previously been identified as potential sources of variance in the identification of myocarditis in different series (23, 24). However, despite the differences found in the various studies, the synthesis of these data does suggest a low actual prevalence of myocarditis post the SARS-CoV-2 infection, although the gold standard diagnostic (endomyocardial biopsy) is rarely reported or employed (26).
Late gadolinium enhancement
A non-ischemic pattern of LGE was present in 3/35 (8.5%) of athletes in our series. In the Master@Heart control group, 3/21 athletes (14%) showed such a pattern, which did not significantly differ from the Covidex athletes (p = 1.000). This is in line with previous findings: prior to the COVID-19 pandemic, a non-ischemic pattern of LGE was reported in 4.7%–6.8% of master athletes (15, 27). More recent data (post-COVID-19) on LGE mostly found a lower prevalence (0.3%–2.3%) (22, 23), although some reports found prevalences as high as 17% (21) or even 46% (4).
Data from previous work suggest that a higher athletic exposure might be an important factor explaining the differences found between the different series: in three studies either cumulative training years (28, 29) or the number of completed marathons were associated with a higher prevalence of LGE (29, 30). This was corroborated by a recent meta-analysis (31). Yet, the evidence regarding this matter is not unequivocal: other studies found a correlation with age (although age and training load per definition go hand in hand) (32) or no correlation at all (33, 34). Future studies are underway to shed more light on this matter, along with the clinical relevance of such LGE patterns (17).
Hinge point fibrosis was present in 23.5% of Master@Heart athletes, as compared with the 8.5% in the current study (p = 0.110). In previous series of mostly master athletes, such fibrosis has been identified in 20%–30% of athletes and is generally regarded as a physiological adaptation to exercise (35).
We found no differences between the mildly and the more severely symptomatic groups. This is in part corroborated by the findings of the Big Ten registry, where symptoms alone only identified 5/37 (13.5%) cases of myocarditis, suggesting that most instances of myocarditis were subclinical (i.e., without symptoms suggestive of myocarditis) (23). Interestingly, in the Outcomes Registry for Cardiac Conditions in Athletes (ORCCA) registry, cardiopulmonary symptoms during COVID-19 were linked to cardiac involvement with an odds ratio of 3.1 (24). Our series is too small to draw definitive conclusions.
Further comparison between the Covidex and Master@heart athletes revealed no additional relevant differences. Previous work already found that higher levels of endurance are associated with more ventricular dilation and lower ejection fractions at rest, findings that were confirmed in our analysis (36).
Arrhythmias
Overall, arrhythmias were rare. One female athlete showed a non-sustained ventricular tachycardia of 14 beats, another female athlete showed more than 500 VES/24 h, and one male athlete with a history of atrial fibrillation had arrhythmia recurrences during the monitoring period. When compared with the Master@Heart data, no significant differences were found, except for a lower resting and maximum heart rate in the latter group. This can be expected from the 10-year age difference and higher exercise load. We were not able to analyze whether palpitations were associated with specific arrhythmias, because only two athletes experienced palpitations. One of them reported palpitations at rest and had 5 isolated VES, 1 ventricular triplet, and 403 single atrial extrasystoles over 5 days. The other reported palpitations during exercise and had 11 isolated VES and 525 single atrial extrasystoles over 5 days.
Although early reports suggested very high incidences of arrhythmic events (in hospitalized COVID-19 patients) (37), later reports have put these data into perspective, suggesting that the COVID-19 associated critical illness, rather than the SARS-CoV-2 infection itself, was the more likely cause of the observed arrhythmias (38). A study in an ambulatory post-COVID-19 population (median 75 days) did not observe a higher-than-expected arrhythmic burden (39). Interestingly, most incidences of palpitations in that study (78% of patient-recorded events) were associated with sinus rhythm or sinus tachycardia. The authors stated in a different publication that “there is presently no definitive data to establish a causal, viral-specific association between COVID-19 and incident arrhythmia” (40). Our results certainly fit with this conclusion.
Secondary endpoints
Resting electrocardiograms were generally normal. TWI beyond V2 was found in three female athletes. Anterior TWI is more common in women and in athletes and does not necessarily hold prognostic significance (41). However, TWI beyond V3 is rare. All three athletes had normal CMRs and a negative family history.
No elevation in hsTnI was found in this population. This could be expected from the late postdiagnosis evaluation in our series.
Overall, we found no significant differences between Group 1 and Groups 2–3 athletes. This suggests that symptoms per se are not indicative of cardiac clinical consequences of an SARS-CoV-2 infection. This is in part corroborated by the findings of the Big Ten registry, where symptoms alone only identified 5/37 (13.5%) cases of myocarditis, suggesting that most instances of myocarditis were subclinical (i.e., without symptoms suggestive of myocarditis) (23).
Limitations
After publication of the ORCCA registry with confirmation of the lower than previously feared prevalence of myocarditis post-COVID-19 (24), we decided to stop testing more individuals, as the original power calculation was based on a 20% prevalence. This resulted in an underpowering of our study. Nevertheless, we found no presence of ULL criteria, thus confirming the more recent findings, and found it worthwhile to still report our findings to add to the literature.
The study was observational in nature, so no conclusions can be drawn about a causal effect of SARS-CoV-2 on our findings (of e.g., non-ischemic LGE). The similarities between the Covidex and Master@Heart (a non-SARS-CoV-2 exposed population), however, seem to indicate that SARS-CoV-2 by itself does not pose a particular threat for myocarditis in athletes.
We may have underdiagnosed SARS-CoV-2-related myocarditis in our series because of the later timing of CMR. This was a deliberate choice as we aimed to gain an insight into the potential late sequelae of COVID-19: fibrosis may predispose to arrhythmias, which are of particular concern in athletes. Fortunately, myocarditis is known to heal without sequelae in most patients. In the Big Ten registry, 40.7% of athletes with signs of COVID-19 myocarditis showed complete resolution of CMR abnormalities 8 (4–10) weeks after the first CMR, which had been taken within a month from the initial diagnosis. The remaining athletes showed resolution of the edema (T2 criteria) but not LGE after 12 (4–14) weeks (23). In the ORCCA registry, 70% showed complete resolution, 10% resolution of T2 abnormalities, and 20% showed persistent positive ULL criteria on repeat CMR (42).
Public health implications
Although COVID-19 can cause serious illness and carry major complications, in general the course of the disease is benign, with 0.05% adverse cardiac events in a recent series of young competitive athletes (43). This implies that general rules regarding return-to-play after viral disease can also be applied to COVID-19 (44): the infection must be cleared, without any remaining symptoms and the process should be gradual and closely monitored. Athletes need to be reminded that aerobic capacity may be decreased for prolonged periods of time after SARS-CoV-2 infection (45). Further work-up is specifically warranted when exercise-related cardiac symptoms, especially chest pain, persist beyond 3-week postinfection (7). Within these contours, an individualized approach may be adapted when reasons are clear, after informed decision-making, preferably with all parties involved reaching a consensus. Our data extend the evidence of a low cardiac involvement to recreational athletes that were older than those in the previous series. Thus, although ongoing vigilance is warranted, a similar return-to-play approach is warranted in this population.
Conclusion
In our series of recreational athletes, we found no evidence of ongoing myocarditis or increases in myocardial fibrosis on average 6 months after SARS-CoV-2 infection. Also, the arrhythmic burden was comparable with historical controls. The results from CMR and heart rhythm monitoring were similar between the asymptomatic or mildly symptomatic athletes and the moderately to severely symptomatic groups.
The results of our series add to other reports with reassuring findings in athletes post-COVID-19. Together, these observations can provide guidance in counseling athletes with an SARS-CoV-2 infection history.
Data availability statement
The raw data supporting the conclusions of this article will be made available by the authors, without undue reservation.
Ethics statement
The studies involving humans were approved by the Antwerp University Hospital Ethics Committee. The studies were conducted in accordance with the local legislation and institutional requirements. The participants provided their written informed consent to participate in this study.
Author contributions
HM: Conceptualization, Data curation, Formal Analysis, Investigation, Methodology, Supervision, Validation, Visualization, Writing – original draft, Writing – review & editing. KF: Data curation, Investigation, Project administration, Supervision, Writing – review & editing. CH: Data curation, Formal Analysis, Methodology, Visualization, Writing – review & editing. BC: Data curation, Formal Analysis, Methodology, Project administration, Writing – review & editing, Writing – original draft. CD: Conceptualization, Data curation, Investigation, Methodology, Project administration, Software, Writing – review & editing. LH: Data curation, Formal Analysis, Project administration, Writing – review & editing. TT: Investigation, Software, Writing – review & editing. YB: Data curation, Investigation, Writing – review & editing. ML: Investigation, Writing – review & editing. JB: Data curation, Writing – review & editing. HH: Conceptualization, Data curation, Investigation, Methodology, Resources, Supervision, Validation, Writing – review & editing. GC: Conceptualization, Data curation, Formal Analysis, Investigation, Methodology, Project administration, Resources, Supervision, Validation, Writing – review & editing.
Funding
The author(s) declare that no financial support was received for the research, authorship, and/or publication of this article.
Conflict of interest
HH received personal lecture and consultancy fees from Bayer, Biotronik, Bristol-Myers Squibb, Centrix Healthcare Ltd, Daiichi-Sankyo, Downtown Europe, Pfizer-BMS, European Society of Cardiology, Medscape, Springer Healthcare Ltd, and Viatris Pharmaceuticals Inc. He received unconditional research grants through the University of Antwerp and/or the University of Hasselt from Abbott, Bayer, Biosense-Webster, Boston-Scientific, Daicchi-Sankyo, Fibricheck/Qompium, Medtronic, and Pfizer-BMS, all outside the scope of this work. HM received personal lecture and consultance fees from Bristol-Myers Squibb, Pfizer and Abbott, all outside the scope of this work.
The remaining authors declare that the research was conducted in the absence of any commercial or financial relationships that could be construed as a potential conflict of interest.
Publisher's note
All claims expressed in this article are solely those of the authors and do not necessarily represent those of their affiliated organizations, or those of the publisher, the editors and the reviewers. Any product that may be evaluated in this article, or claim that may be made by its manufacturer, is not guaranteed or endorsed by the publisher.
References
1. Wei Z-Y, Geng Y-J, Huang J, Qian H-Y. Pathogenesis and management of myocardial injury in coronavirus disease 2019. Eur J Heart Fail. (2020) 22:1994–2006. doi: 10.1002/ejhf.1967
2. Huang L, Zhao P, Tang D, Zhu T, Han R, Zhan C, et al. Cardiac involvement in patients recovered from COVID-2019 identified using magnetic resonance imaging. JACC Cardiovasc Imaging. (2020) 13:2330–9. doi: 10.1016/j.jcmg.2020.05.004
3. Puntmann VO, Carerj ML, Wieters I, Fahim M, Arendt C, Hoffmann J, et al. Outcomes of cardiovascular magnetic resonance imaging in patients recently recovered from coronavirus disease 2019 (COVID-19). JAMA Cardiol. (2020) 5:1265–73. doi: 10.1001/jamacardio.2020.3557
4. Rajpal S, Tong MS, Borchers J, Zareba KM, Obarski TP, Simonetti OP, et al. Cardiovascular magnetic resonance findings in competitive athletes recovering from COVID-19 infection. JAMA Cardiol. (2021) 6:116–8. doi: 10.1001/jamacardio.2020.4916
5. Harmon KG, Asif IM, Maleszewski JJ, Owens DS, Prutkin JM, Salerno JC, et al. Incidence and etiology of sudden cardiac arrest and death in high school athletes in the United States. Mayo Clin Proc. (2016) 91:1493–502. doi: 10.1016/j.mayocp.2016.07.021
6. Grun S, Schumm J, Greulich S, Wagner A, Schneider S, Bruder O. Long-term follow-up of biopsy-proven viral myocarditis: predictors of mortality and incomplete recovery. J Am Coll Cardiol. (2012) 59:1604–15. doi: 10.1016/j.jacc.2012.01.007
7. Petek BJ, Moulson N, Baggish AL, Kliethermes SA, Patel MR, Churchill TW, et al. Prevalence and clinical implications of persistent or exertional cardiopulmonary symptoms following SARS-CoV-2 infection in 3597 collegiate athletes: a study from the Outcomes Registry for Cardiac Conditions in Athletes (ORCCA). Br J Sports Med. (2022) 56:913–8. doi: 10.1136/bjsports-2021-104644
8. Palermi S, Cavarretta E, D’Ascenzi F, Castelletti S, Ricci F, Vecchiato M, et al. Athlete’s heart: a cardiovascular step-by-step multimodality approach. Rev Cardiovasc Med. (2023) 24:151. doi: 10.31083/j.rcm2405151
9. La Gerche A, Baggish AL, Knuuti J, Prior DL, Sharma S, Heidbuchel H, et al. Cardiac imaging and stress testing asymptomatic athletes to identify those at risk of sudden cardiac death. JACC Cardiovasc Imaging. (2013) 6:993–1007. doi: 10.1016/j.jcmg.2013.06.003
10. Von Elm E, Altman DG, Egger M, Pocock SJ, Gøtzsche PC, Vandenbroucke JP, et al. The Strengthening the Reporting of Observational Studies in Epidemiology (STROBE) statement: guidelines for reporting observational studies. Lancet. (2007) 370:1453–7. doi: 10.1016/S0140-6736(07)61602-X
11. Pelliccia A, Caselli S, Sharma S, Basso C, Bax JJ, Corrado D, et al. European Association of Preventive Cardiology (EAPC) and European Association of Cardiovascular Imaging (EACVI) joint position statement: recommendations for the indication and interpretation of cardiovascular imaging in the evaluation of the athlete’s heart. Eur Heart J. (2018) 39:1949–69. doi: 10.1093/eurheartj/ehx532
12. McKinney J, Velghe J, Fee J, Isserow S, Drezner JA. Defining athletes and exercisers. Am J Cardiol. (2019) 123:532–5. doi: 10.1016/j.amjcard.2018.11.001
13. Harris PA, Taylor R, Minor BL, Elliott V, Fernandez M, O’Neal L, et al. The REDCap consortium: building an international community of software platform partners. J Biomed Inform. (2019) 95:103208. doi: 10.1016/j.jbi.2019.103208
14. Harris PA, Taylor R, Thielke R, Payne J, Gonzalez N, Conde JG. Research electronic data capture (REDCap)—a metadata-driven methodology and workflow process for providing translational research informatics support. J Biomed Inform. (2009) 42:377–81. doi: 10.1016/j.jbi.2008.08.010
15. Merghani A, Maestrini V, Rosmini S, Cox AT, Dhutia H, Bastiaenan R, et al. Prevalence of subclinical coronary artery disease in Masters endurance athletes with a low atherosclerotic risk profile. Circulation. (2017) 136:126–37. doi: 10.1161/CIRCULATIONAHA.116.026964
16. Ferreira VM, Schulz-Menger J, Holmvang G, Kramer CM, Carbone I, Sechtem U, et al. Cardiovascular magnetic resonance in nonischemic myocardial inflammation: expert recommendations. J Am Coll Cardiol. (2018) 72:3158–76. doi: 10.1016/j.jacc.2018.09.072
17. De Bosscher R, Dausin C, Claus P, Bogaert J, Dymarkowski S, Goetschalckx K, et al. Endurance exercise and the risk of cardiovascular pathology in men: a comparison between lifelong and late-onset endurance training and a non-athletic lifestyle—rationale and design of the Master@Heart study, a prospective cohort trial. BMJ Open Sport Exerc Med. (2021) 7:e001048. doi: 10.1136/bmjsem-2021-001048
18. De Bosscher R, Dausin C, Claus P, Bogaert J, Dymarkowski S, Goetschalckx K, et al. Lifelong endurance exercise and its relation with coronary atherosclerosis. Eur Heart J. (2023) 44:2388–99. doi: 10.1093/eurheartj/ehad152
19. Vago H, Szabo L, Dohy Z, Merkely B. Cardiac magnetic resonance findings in patients recovered from COVID-19. JACC Cardiovasc Imaging. (2021) 14:1279–81. doi: 10.1016/j.jcmg.2020.11.014
20. Małek ŁA, Marczak M, Miłosz-Wieczorek B, Konopka M, Braksator W, Drygas W, et al. Cardiac involvement in consecutive elite athletes recovered from COVID-19: a magnetic resonance study. J Magn Reson Imaging. (2021) 53:1723–9. doi: 10.1002/jmri.27513
21. Brito D, Meester S, Yanamala N, Patel HB, Balcik BJ, Casaclang-Verzosa G, et al. High prevalence of pericardial involvement in college student athletes recovering from COVID-19. JACC Cardiovasc Imaging. (2021) 14:541–55. doi: 10.1016/j.jcmg.2020.10.023
22. Martinez MW, Tucker AM, Bloom OJ, Green G, DiFiori JP, Solomon G, et al. Prevalence of inflammatory heart disease among professional athletes with prior COVID-19 infection who received systematic return-to-play cardiac screening. JAMA Cardiol. (2021) 6:745. doi: 10.1001/jamacardio.2021.0565
23. Daniels CJ, Rajpal S, Greenshields JT, Rosenthal GL, Chung EH, Terrin M, et al. Prevalence of clinical and subclinical myocarditis in competitive athletes with recent SARS-CoV-2 infection. JAMA Cardiol. (2021) 6:1078. doi: 10.1001/jamacardio.2021.2065
24. Moulson N, Petek BJ, Drezner JA, Harmon KG, Kliethermes SA, Patel MR, et al. SARS-CoV-2 cardiac involvement in young competitive athletes. Circulation. (2021) 144:256–66. doi: 10.1161/CIRCULATIONAHA.121.054824
25. Van Hattum JC, Spies JL, Verwijs SM, Verwoert GC, Planken RN, Boekholdt SM, et al. Cardiac abnormalities in athletes after SARS-CoV-2 infection: a systematic review. BMJ Open Sport Exerc Med. (2021) 7:e001164. doi: 10.1136/bmjsem-2021-001164
26. Caforio AL, Pankuweit S, Arbustini E, Basso C, Gimeno-Blanes J, Felix SB. Current state of knowledge on aetiology, diagnosis, management, and therapy of myocarditis: a position statement of the European Society of Cardiology Working Group on Myocardial and Pericardial Diseases. Eur Heart J. (2013) 34:2636–48. doi: 10.1093/eurheartj/eht210
27. Breuckmann F, Mohlenkamp S, Nassenstein K, Lehmann N, Ladd S, Schmermund A. Myocardial late gadolinium enhancement: prevalence, pattern, and prognostic relevance in marathon runners. Radiology. (2009) 251:50–7. doi: 10.1148/radiol.2511081118
28. La Gerche A, Burns AT, Mooney DJ, Inder WJ, Taylor AJ, Bogaert J. Exercise-induced right ventricular dysfunction and structural remodelling in endurance athletes. Eur Heart J. (2012) 33:998–1006. doi: 10.1093/eurheartj/ehr397
29. Wilson M, O’Hanlon R, Prasad S, Deighan A, Macmillan P, Oxborough D. Diverse patterns of myocardial fibrosis in lifelong, veteran endurance athletes. J Appl Physiol (1985). (2011) 110:1622–6. doi: 10.1152/japplphysiol.01280.2010
30. Mohlenkamp S, Lehmann N, Breuckmann F, Brocker-Preuss M, Nassenstein K, Halle M, et al. Running: the risk of coronary events : prevalence and prognostic relevance of coronary atherosclerosis in marathon runners. Eur Heart J. (2008) 29:1903–10. doi: 10.1093/eurheartj/ehn163
31. Zhang CD, Xu SL, Wang XY, Tao LY, Zhao W, Gao W. Prevalence of myocardial fibrosis in intensive endurance training athletes: a systematic review and meta-analysis. Front Cardiovasc Med. (2020) 7:585692. doi: 10.3389/fcvm.2020.585692
32. Androulakis E, Mouselimis D, Tsarouchas A, Antonopoulos A, Bakogiannis C, Papagkikas P, et al. The role of cardiovascular magnetic resonance imaging in the assessment of myocardial fibrosis in young and veteran athletes: insights from a meta-analysis. Front Cardiovasc Med. (2021) 8:784474. doi: 10.3389/fcvm.2021.784474
33. Abdullah SM, Barkley KW, Bhella PS, Hastings JL, Matulevicius S, Fujimoto N, et al. Lifelong physical activity regardless of dose is not associated with myocardial fibrosis. Circ Cardiovasc Imaging. (2016) 9:e005511. doi: 10.1161/CIRCIMAGING.116.005511
34. Missenard O, Gabaudan C, Astier H, Desmots F, Garnotel E, Massoure PL. Absence of cardiac damage induced by long-term intensive endurance exercise training: a cardiac magnetic resonance and exercise echocardiography analysis in masters athletes. Am J Prev Cardiol. (2021) 7:100196. doi: 10.1016/j.ajpc.2021.100196
35. Banks L, Altaha MA, Yan AT, Dorian P, Konieczny K, Deva DP, et al. Left ventricular fibrosis in middle-age athletes and physically active adults. Med Sci Sports Exerc. (2020) 52:2500–7. doi: 10.1249/MSS.0000000000002411
36. Brown B, Millar L, Somauroo J, George K, Sharma S, La Gerche A, et al. Left ventricular remodeling in elite and sub-elite road cyclists. Scand J Med Sci Sports. (2020) 30:1132–9. doi: 10.1111/sms.13656
37. Wang D, Hu B, Hu C, Zhu F, Liu X, Zhang J, et al. Clinical characteristics of 138 hospitalized patients with 2019 novel coronavirus-infected pneumonia in Wuhan, China. JAMA. (2020) 323:1061–9. doi: 10.1001/jama.2020.1585
38. Bhatla A, Mayer MM, Adusumalli S, Hyman MC, Oh E, Tierney A, et al. COVID-19 and cardiac arrhythmias. Heart Rhythm. (2020) 17:1439–44. doi: 10.1016/j.hrthm.2020.06.016
39. Dewland TA, Whitman IR, Win S, Sanchez JM, Olgin JE, Pletcher MJ, et al. Prospective arrhythmia surveillance after a COVID-19 diagnosis. Open Heart. (2022) 9:e001758. doi: 10.1136/openhrt-2021-001758
40. Dewland TA, Marcus GM. SARS-CoV-2 infection and cardiac arrhythmias. Nat Cardiovasc Res. (2022) 1:1109–10. doi: 10.1038/s44161-022-00166-x
41. Malhotra A, Dhutia H, Gati S, Yeo T-J, Dores H, Bastiaenen R, et al. Anterior T-wave inversion in young white athletes and nonathletes. J Am Coll Cardiol. (2017) 69:1–9. doi: 10.1016/j.jacc.2016.10.044
42. Petek BJ, Moulson N, Drezner JA, Harmon KG, Kliethermes SA, Churchill TW, et al. Cardiovascular outcomes in collegiate athletes after SARS-CoV-2 infection: 1-year follow-up from the outcomes registry for cardiac conditions in athletes. Circulation. (2022) 145:1690–2. doi: 10.1161/CIRCULATIONAHA.121.058272
43. Moulson N, Petek BJ, Baggish AL, Harmon KG, Kliethermes SA, Patel MR, et al. The cardiac effects of COVID-19 on young competitive athletes: results from the Outcomes Registry for Cardiac Conditions in Athletes (ORCCA). J Cardiovasc Dev Dis. (2023) 10:72. doi: 10.3390/jcdd10020072
44. Börjesson M, Arvidsson D, van Rensburg CJ, Schwellnus M. Return to play after infectious disease. In: Musahl V, Karlsson J, Krutsch W, Mandelbaum B, Espregueira-Mendes J, d'Hooghe P, editors. Return to Play in Football: An Evidence-Based Approach. Berlin: Springer (2018). p. 755–70. doi: 10.1007/978-3-662-55713-6_56
Keywords: COVID-19, athlete, myocarditis, arrhythmias, recreational
Citation: Miljoen H, Favere K, Van De Heyning C, Corteville B, Dausin C, Herbots L, Teulingkx T, Bekhuis Y, Lyssens M, Bogaert J, Heidbuchel H and Claessen G (2024) Low rates of myocardial fibrosis and ventricular arrhythmias in recreational athletes after SARS-CoV-2 infection. Front. Cardiovasc. Med. 11:1372028. doi: 10.3389/fcvm.2024.1372028
Received: 17 January 2024; Accepted: 26 February 2024;
Published: 2 April 2024.
Edited by:
Niek H. J. Prakken, University Medical Center Groningen, NetherlandsReviewed by:
Tatjana Miljkovic, University of Novi Sad, SerbiaStefano Palermi, University of Naples Federico II, Italy
© 2024 Miljoen, Favere, Van De Heyning, Corteville, Dausin, Herbots, Teulingkx, Bekhuis, Lyssens, Bogaert, Heidbuchel and Claessen. This is an open-access article distributed under the terms of the Creative Commons Attribution License (CC BY). The use, distribution or reproduction in other forums is permitted, provided the original author(s) and the copyright owner(s) are credited and that the original publication in this journal is cited, in accordance with accepted academic practice. No use, distribution or reproduction is permitted which does not comply with these terms.
*Correspondence: Hielko Miljoen hielko.miljoen@uza.be