Non-invasive assessment of proarrhythmic risks associated with isoprenaline and the dietary supplement ingredient synephrine using human induced pluripotent stem cell-derived cardiomyocytes
- School of Life Sciences, Beijing University of Chinese Medicine, Beijing, China
Background: There have been conflicting reports about the proarrhythmic risk of p-synephrine (SYN). To address this, human induced pluripotent stem cell-derived cardiomyocytes (hiPSC-CMs) combined with the microelectrode array (MEA) system have been utilized to assess arrhythmia risks, particularly in the context of adrenomimetic drugs.
Aim: This study aims to determine whether MEA recordings from hiPSC-CMs could predict the proarrhythmic risk of adrenomimetic drugs and to investigate the cardiovascular effects and mechanisms of SYN.
Materials and methods: We employed MEA recordings to assess the electrophysiological properties of hiPSC-CMs and conducted concentration-response analyses to evaluate the effects of SYN and Isoprenaline (ISO) on beating rate and contractility. A risk scoring system for proarrhythmic risks was established based on hiPSC-CMs in this study. ISO, a classic beta-adrenergic drug, was also evaluated. Furthermore, the study evaluated the risk of SYN and recorded the concentration-response of beating rate, contractility and the change in the presence or absence of selective β1, β2 and β3 adrenergic blockers.
Results: Our results suggested that ISO carries a high risk of inducing arrhythmias, aligning with existing literature. SYN caused a 30% prolongation of the field potential duration (FPD) at a concentration of 206.326 μM, a change significantly different from baseline measurements and control treatments. The half maximal effective concentration (EC50) of SYN (3.31 μM) to affect hiPSC-CM beating rate is much higher than that of ISO (18.00 nM). The effect of SYN at an EC50 of 3.31 μM is about ten times more potent in hiPSC-CMs compared to neonatal rat cardiomyocytes (34.12 μM). SYN increased the contractility of cardiomyocytes by 29.97 ± 11.65%, compared to ISO's increase of 50.56 ± 24.15%. β1 receptor blockers almost eliminated the beating rate increase induced by both ISO and SYN, while neither β2 nor β3 blockers had a complete inhibitory effect.
Conclusion: The MEA and hiPSC-CM system could effectively predict the risk of adrenomimetic drugs. The study concludes that the proarrhythmia risk of SYN at conventional doses is low. SYN is more sensitive in increasing beating rate and contractility in human cardiomyocytes compared to rats, primarily activating β1 receptor.
1 Introduction
p-synephrine (SYN), a natural alkaloid extracted from Citrus aurantium L. (commonly known as bitter orange or sour orange), is frequently used in dietary supplements for weight management and sports performance as an alternative to ephedra. SYN is also found in various citrus foods. However, its safety has been a subject of debate. The Food and Drug Administration (FDA) (1), Health Canada (2) and other institutions have reported numerous adverse events related to SYN, predominantly cardiovascular issues such as QT prolongation-induced exercise syncope, tachycardia, ventricular fibrillation, cardiac arrest, and sudden death (3). Several case reports and articles (4–10) suggest a link between SYN, bitter orange extract, and cardiovascular events, including an increased heart rate (11). This is further supported by animal studies (12, 13). In contrast, other investigations on SYN (14–17) report no observed cardiovascular effects. Moreover, while some studies (11, 18–20) indicate that SYN might accelerate heart rate, we hypothesize that this could be due to impurities (ephedrine/m-synephrine/N-methyltyramine) or its combination with caffeine. To determine the cardiovascular effects of SYN and its potential to cause arrhythmias, thorough safety assessments are essential.
Human induced pluripotent stem cell-derived cardiomyocytes (hiPSC-CMs) exhibit properties akin to adult cardiomyocytes (21, 22), such as the expression of crucial structural and functional genes, ultrastructural characteristics like Z disks and T-tubules, electrophysiological phenotypes, and ion channel functions (23), as well as mature excitation-contraction coupling (24, 25). Thus, hiPSC-CMs are increasingly used as renewable experimental materials and are anticipated to become a comprehensive tool for in vitro torsadogenic liability assessment (26). Despite the wide use of hiPSC-CMs in drug evaluation, a comprehensive methodology to accurately predict the arrhythmic risk posed by β-adrenergic agonists, including the widely used SYN, is notably lacking. This gap is critical, considering the widespread consumption of SYN-containing supplements and their potential cardiovascular risks. The multi-electrode array (MEA) system can record field potentials (FP) of spontaneously beating cardiomyocyte monolayers, analogous to clinical electrocardiogram (ECG). The field potential duration (FPD) is comparable to the QT interval, and can be used to analyze the repolarization times. Additionally, early afterdepolarization (EAD) and torsadogenic and arrest events are instrumental in assessing proarrhythmia risks (27–30). However, the methodology for predicting arrhythmia risks using hiPSC-CM and MEA is not yet fully established. While certain drugs like E4031 and Quinidine, that directly affect ion channels have been evaluated using this method, studies on β receptor agonist-related ligands are scarce. Unlike ion channel blockers that prolong the QT interval, isoprenaline (ISO) can shorten it. This shortening also poses arrhythmia risks. ISO, a full β-receptor agonist, has been shown in numerous studies to shorten the QT interval, thereby increasing arrhythmias risks. Despite its widespread use, the safety of SYN remains debated. Numerous studies, such as those by Stohs et al. (20) and Jordan et al. (2), have highlighted potential cardiovascular risks including increased heart rate and blood pressure, while others like Shara and Stohs (16) report no observed adverse cardiovascular effects under controlled conditions. Therefore, in this study, we assessed the arrhythmic risks of β receptor agonists.
In this study, we tested the classic beta-adrenergic receptor agonist ISO and the potential adrenomimetic drug SYN. To evaluate the arrhythmic risk of ISO and SYN, we measured FPD prolongation, EAD occurrence, and Torsades de pointes (TdP) incidences. We detected the concentration-response relationship of ISO and SYN in hiPSC-CMs and analyzed SYN's effects on two types of cells: hiPSC-CMs and neonatal rat cardiomyocyte (NRCM). We also assessed the inotropic effects of these drugs on hiPSC-CMs. To better understand the toxic mechanism of these drugs and explore their potential therapeutic value, we utilized inhibitors to identify their action targets. We hypothesize that through the application of MEA recordings and analysis of hiPSC-CMs, we can delineate the arrhythmic risk profile of SYN in a cell-based model, offering insights into its safety and mechanistic effects on the cardiovascular system. This study aims to bridge the existing knowledge gap by employing hiPSC-CMs to assess the proarrhythmic potential of SYN and compare its effects with ISO, thereby providing a clearer understanding of SYN's cardiovascular implications.
2 Method and materials
2.1 Experimental materials
HiPSC-CMs were obtained from HELP Therapeutics (Nanjing, China). DMEM/F-12 was obtained from Gibco (now part of Invitrogen, Carlsbad, CA). Fetal bovine serum (FBS) and horse serum (HS) were purchased from Corning-Cellgro (Manassas, Virginia, USA) and BIODEE (Beijing, China), respectively. SYN was purchased from MedChemExpress (New Jersey, USA). ISO and laminin were obtained from Sigma (Merck, USA). Collagenase Ⅱ and Penicillin-Streptomycin were sourced from Invitrogen (Carlsbad, CA). Trypsin was procured from Life Science (now part of Alphabet, USA). 5-bromo-2′-deoxyuridine (5-BrdU) was obtained from Solarbio (Beijing, China). To investigate the specific roles of β1, β2, and β3 adrenergic receptors in mediating the effects of ISO and SYN on beating rate and contractility, we employed selective blockers targeting each receptor subtype. The blockers were chosen based on their high selectivity for their respective target receptors, as demonstrated in previous studies (31, 32). By comparing the extent to which each selective blocker inhibits the changes in beating rate and contractility induced by ISO and SYN, we can infer the relative contributions of β1, β2, and β3 receptors to their mechanisms of action.
2.2 Cultivation and preparation of hiPSC-CMs
HiPSC-CMs were seeded on MEA chips and confocal dishes, which were pre-coated with 1% fibronectin. The cells were allowed to adhere for 48 h using recovery medium (Help Stem Cell Innovations). This was followed by replacing the medium with cardiac maintenance medium (Help Stem Cell Innovations). The medium was refreshed bi-daily. Approximately 7–10 days post-seeding, the spontaneous beating frequency of hiPSC-CMs stabilized at around 1 Hz. This maturation stage is critical as it closely resembles the functional characteristics of adult cardiomyocytes, making our model especially relevant for studying cardiac electrophysiology and drug responses.
2.3 Isolation and culture of NRCM
Neonatal Sprague Dawley rats, aged between 24 and 48 h, were euthanized rapidly by decapitation (33, 34). The thoracic cavity was opened under aseptic conditions to expose the heart, which was promptly removed and washed with pre-cooled D-Hanks solution. Subsequently, the hearts were separated from cardiopulmonary tissue and minced into approximately 1 mm3 pieces using scissors. These pieces were then transferred to a 50 ml centrifuge tube and digested with a 0.05% type II collagenase solution in a constant temperature water bath at 37°C with shaking at 220 rpm for 40 min. Post enzymatic digestion, the resultant single cell suspension was filtered through a cell strainer and centrifuged at 300 g for 5 min, followed by resuspension in DMEM/F12-based culture medium with 10% FBS, 5% HS, 1% penicillin/streptomycin, and 100 μM 5-BrdU. 5-BrdU was added to the culture medium as part of our protocol to inhibit fibroblast proliferation within the cardiomyocyte cultures. Differential centrifugation, based on the differing gravities of fibroblasts and myocytes (33), was used for cell separation. The isolated neonatal rat ventricular myocytes (NRVMs) were then cultured on 0.1% laminin-coated petri dishes. The experimental protocol received approval from the Ethics Committee of Beijing University of Chinese Medicine (BUCM).
2.4 Concentration-response design and EC50 calculation
The selection of concentration ranges for ISO and SYN was based on a comprehensive review of existing literature and preliminary dose-response studies. The chosen concentrations aimed to cover the therapeutically relevant range and beyond to assess both efficacy and safety. The process was as follows: Initially, the range of concentrations was broad and then progressively narrowed. In the first experiment, ISO was tested at concentrations of 0, 1 nM, 1 μM and 1 mM. It was observed to be effective within the range of 1 nM–1 μM, with stopping of beating at 1 μM. Consequently, for the second experiment, the concentrations were refined to 0, 0.001 nM, 0.01 nM, 0.1 nM, 1 nM, 10 nM, 100 nM and 1 μM based on the initial effective concentration range. Similarly, for SYN, the range was chosen to explore its potential arrhythmic effects at both low and high doses. The initial experiment tested concentrations of 0, 1 nM, 1 μM and 1 mM, finding effectiveness in the range of 1 μM–1 mM. The subsequent experiment, therefore, used a narrower concentration selection: 0, 0.3 μM, 1 μM, 3 μM, 27 μM, 81 μM, 243 μM and 729 μM. The half maximal effective concentrations (EC50) values were calculated using GraphPad Prism version 8.0.0 for Windows (GraphPad Software, San Diego, California USA).
2.5 Field potential (FP) recordings
The MEA system records the electrical activity of cardiomyocytes by measuring the field potentials across a grid of electrodes. We chose an MEA chip equipped with 120 electrodes because it provides extensive spatial resolution that allows for a detailed mapping of the electrophysiological properties across the cardiomyocyte monolayer. This choice was based on comparative analyses with other electrode configurations, which showed that 120 electrodes offer superior data fidelity essential for accurate measurement of subtle changes in cardiomyocyte activity. This technique mimics human cardiac electrophysiology by capturing the collective electrical signals from cardiomyocyte monolayers, thus providing insights into the arrhythmic potential of compounds tested. For this purpose, cardiomyocytes were cultured on a laminin-coated MEA chip for 7–10 days. The electrophysiological characteristics of the cardiomyocytes were recorded using the Multi-Channel Systems (MEA2100-120-System, Multi Channel Systems), under controlled conditions of 37°C and 5% CO2. The recorded signals were amplified and processed through a filter amplifier, then transferred to the Cardio2D+ software (Multi Channel Systems) for subsequent data acquisition and analysis.
2.6 FPD data analysis and estimation the risk of proarrhythmia
FPD is defined as the interval between the onset of the first sharp deflection and the second positive deflection peak in a waveform. For analysis, a stable recording signal is selected and observed for a duration of 30 s. Considering that β receptor activation can increase beating rate, Bazett's correction formula is employed to calculate the corrected field potential duration (FPDc). This correction involved adjusting FPD by the RR interval, using the formula: . The absolute values of FPDc at each concentration are expressed as a percentage change from baseline values. To establish the equations for calculating the concentration that causes a 10% (FPDc10) and a 30% (FPDc30) change in FPDc, linear regression analysis is performed on the change in FPDc with increasing administration concentration.
The Relative torsadogenic risk of each drug is estimated based on scoring criteria outlined in Table 1. This relative risk is determined using FPDcF10, FPDcF30, and EAD values. The possible TdP risk scores ranged from 0 to 3 (35). A score of 0 is assigned when there is no change in FPD; a score of 1 when the percentage change in FPDc is between 10% and 30%; a score of 2 when the change exceeds 30%; and a score of 3 is assigned in the event of EAD, TdP, or cardiac arrest occurrences.
2.7 Contractility measurement
Cardiac contractility measurement was conducted using the brightfield (BF) channels of an inverted confocal microscope (FV3000, Olympus). The cardiomyocyte was placed in a specialized chamber maintained at 37 °C and 5% CO2. Sequential images of the cardiomyocyte contractions were captured using Olympus CellSens software. The analysis of these contractions was performed using the MUSCLEMOTION plugin in Image J (36).
2.8 Statistical analysis
Quantitative data in this study are presented as the mean ± standard error (SE). Statistical analyses were performed using GraphPad Prism version 9.0.0 for Windows (GraphPad Software, San Diego, California USA). Depending on the data characteristics, either one-way repeated measures ANOVA, one-way ANOVA, or paired t-tests were utilized, as appropriate. Statistical significance was established as P < 0.01 or P < 0.001.
3 Results
3.1 Torsadogenic risk assessment
The results indicated that FPDc varied with increasing concentrations of ISO and SYN. Notably, ISO shortened the FPDc within a certain concentration range (Figure 1A). The dose-dependent effects of ISO and SYN on FPDc, highlight their differential modulation of cardiomyocyte electrophysiology. When these changes induced by ISO were analyzed with Cardio2D+ software, and the subsequent linear fitting was performed using GraphPad software (Figure 1B). The concentrations of ISO causing a 10% (FPDc10) and 30% (FPDc30) reduction in FPDc were calculated, with the findings presented in Table 2. Conversely, SYN treatment generally led to the elongation of FPDc, demonstrated in Figure 1C. The progressive increase in FPDc with the increasing concentrations of SYN is shown in Figure 1D. The calculated concentrations of SYN that induce a 10% and 30% elongation of FPDc were 206.326 μM and 681.48 μM, respectively, detailed in Table 2. However, at an accumulated ISO concentration of 1 μM, TdP-like changes in cardiomyocyte electrical signals were noted in Table 2 and Figure 2. The significant shortening of FPDc by ISO underscores its proarrhythmic potential, while the more modest changes induced by SYN suggest a lower risk at clinical doses.
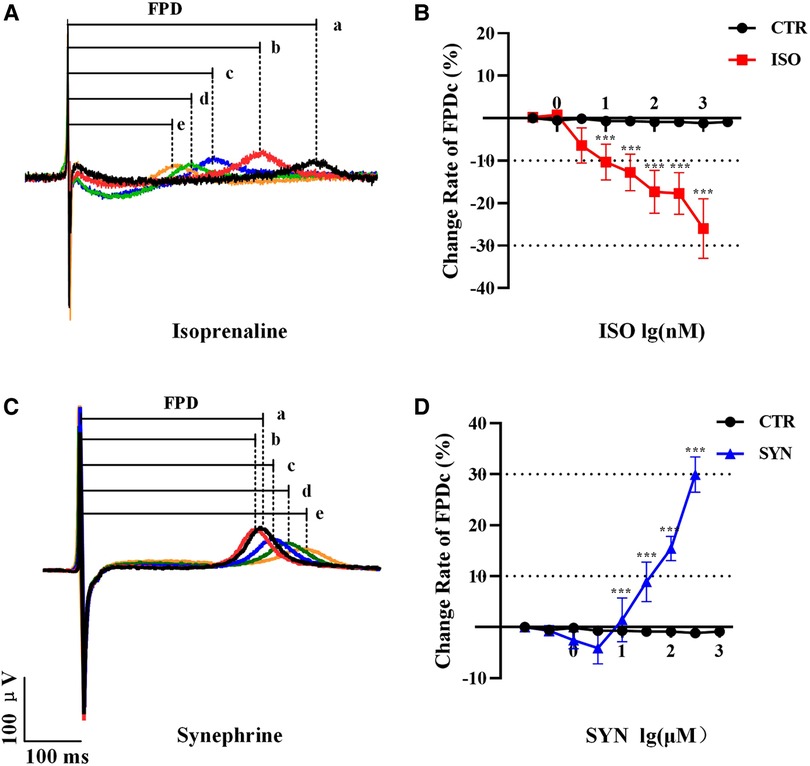
Figure 1. Representative MEA recordings, and the a, b, c, d, e represent increasing doses sequentially. The effect of ISO treatment on field potential duration (FPD) (A). FPDc change under ISO treatment (n = 10 electrode data per condition from four independent preparations, ***P < 0.001 vs control group) (B). The effect of synephrine treatment on FPD (C). Quantification the prolongation of FPDc with synephrine (n = 10 electrode data per condition from four independent preparations, ***P < 0.001 vs control group) (D).
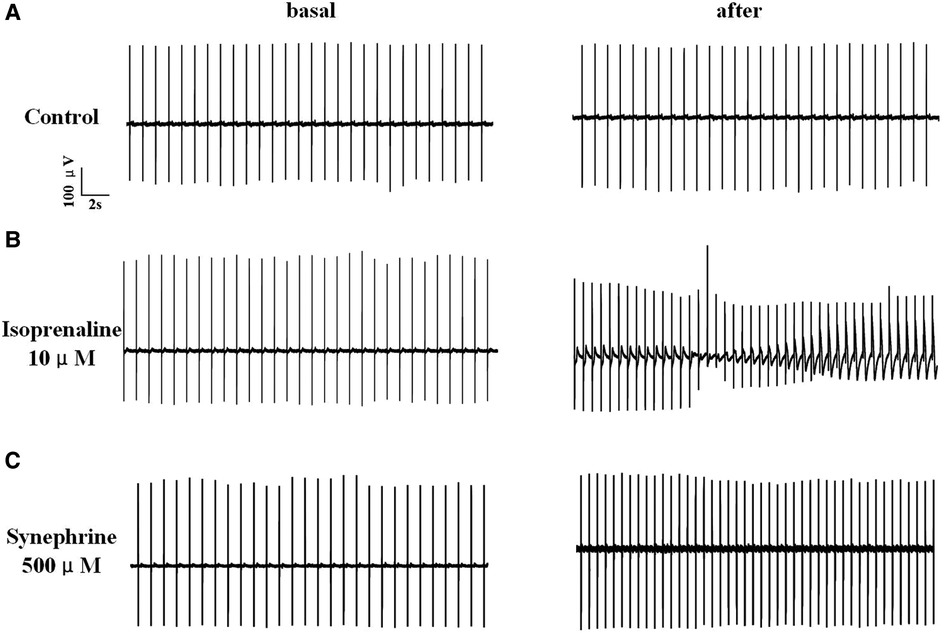
Figure 2. Electrical signals of hiPSC-CM recorded by MEA. The record of solvent control group (A). After treated with 10 μM isoprenaline, hiPSC-CM showed TdP-like arrhythmia (B). Under high concentration of 500 μM, synephrine increases heart rate without TdP-like curve (C).
3.2 Concentration-response of beating rate to ISO and SYN
Both ISO and SYN were administered cumulatively to hiPSC-CMs, resulting in an increase in the beating rate of cardiomyocytes. The concentration of ISO was varied in a ten-fold gradient ranging from 1 to 104 nM, whereas the SYN concentration was adjusted in three-fold increments from 1 to 81 μM. The increase in cardiomyocytes' beating rate was proportional to the cumulative concentration of ISO and SYN (Figure 3). The effect of ISO is characterized by an EC50 value calculated at 18.00 nM (95% CI: 8.825–40.30 nM) (Table 3).
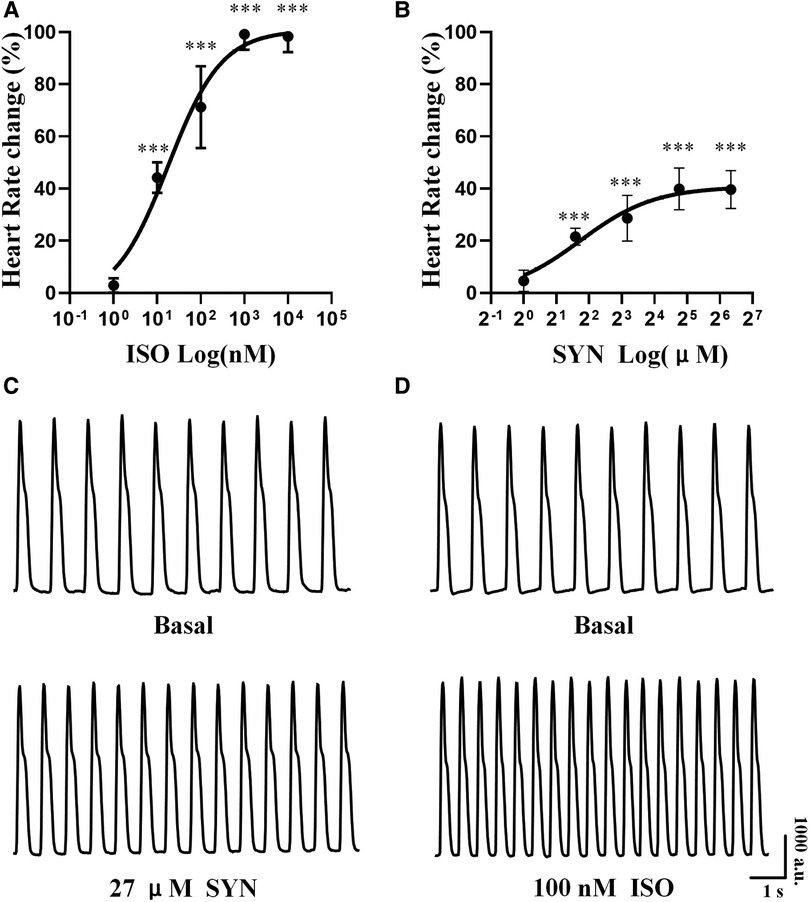
Figure 3. Cumulative concentration-response curve to isoprenaline (ISO) and synephrine (SYN) in hiPSC-CM. The percentage change in heart rate at each concentration (A, B). Representative curve of hiPSC-CM spontaneous contraction before and after exposure to 100 nM ISO and 27 μM SYN (C, D).
Conversely, the concentration-response curve for SYN on hiPSC-CMs yielded an EC50 value of 3.31 μM (95% CI: 1.751–9.806 μM). These effects are further compared to NRCMs in Table 4, where the EC50 value for NRCMs was found to be 34.12 μM (95% CI: 24.66–49.37 μM). The graphical representation of SYN's effects on cardiomyocytes is illustrated in Figure 3.
3.3 Positive inotropic response to ISO and SYN
The effect of ISO and SYN on cardiomyocyte contractility was shown in Figure 4A. To determine the effect of ISO and SYN on contraction functionality, four key parameters were analyzed: contraction amplitude, rise time (time from baseline to peak), decay time (time from peak to baseline), and full width at half maximum (FWHM). At a concentration of 100 nM, ISO significantly (P < 0.001) increased the contraction amplitude. SYN at 27 μM also resulted in an increase of contraction amplitude (Figure 4B). Both compounds reduced the rise time (Figure 4C). Conversely, both ISO and SYN significantly increased the decay time (Figure 4D). Furthermore, the FWHM for ISO and SYN also increased (Figure 4E).
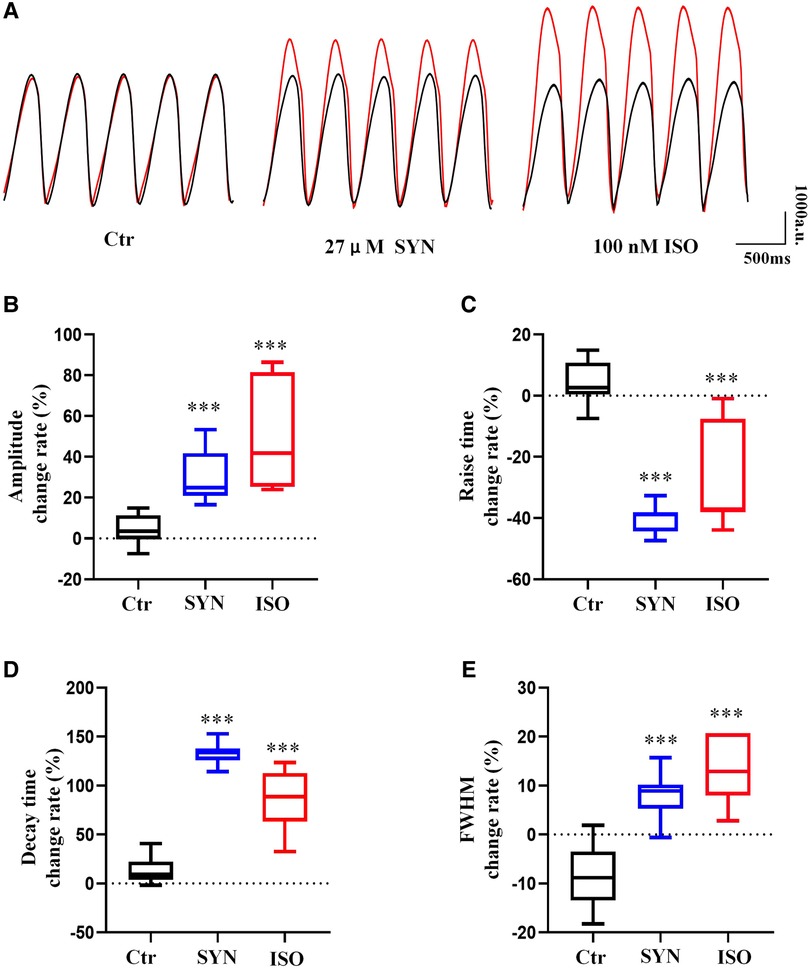
Figure 4. Cardiomyocytes recorded under 2 Hz field stimulation. The parameters of the beat are recorded by microscopic bright field mode and analyzed using MUSCLEMOTION software (A). The result of the presentation was the percentage change relative to the baseline. (B) Peak amplitude. The cell contraction is depicted by the displacement of the pixels, and the units are expressed in “a.u”. (C) Peak rise time. (D) Peak decay time. (E) Full width at half maximum. The symbol “***” represents a significant difference compared to control (P < 0.001).
3.4 Response dependence of the β-adrenoceptor subtype
Research into the specific roles of β1, β2, and β3 adrenergic receptors in modulating beating rate and contractility was performed through studies employing selective blockers. The results revealed that blockers targeting either β2 or β3 adrenergic receptors were unable to completely negate the increase in beating rate and contractility induced by ISO. In contrast, the application of β1 receptor blockers almost entirely abolished these effects, aligning with findings from previous reports. The effect of SYN mirrored some aspects of ISO's effects. When β1 receptor blockers were used, the influence of SYN on beating rate and contractility was almost entirely neutralized. This indicates that SYN likely to accelerate beating rate predominantly by stimulating β1 receptors (Figures 5). This observation implies that β2 and β3 receptors might play a secondary role in the modulation of SYN's effects.
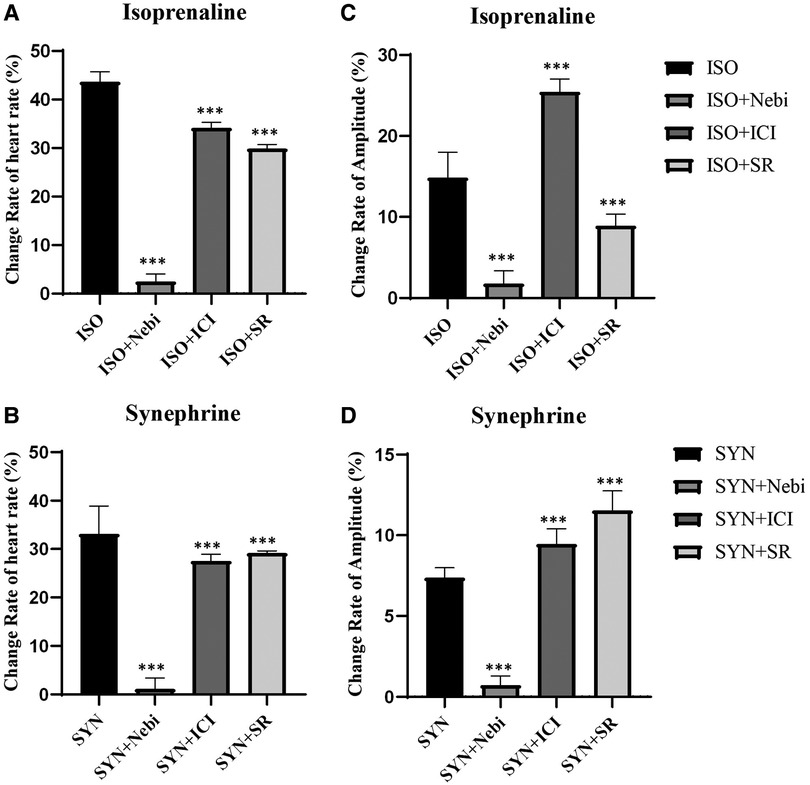
Figure 5. Changes in heart rate and contractility in the presence of the 10 nM β1 receptor selective blocker nebivolol, the 10 nM β2 selective receptor blocker ICI118551, and the 50 nM β3 selective receptor blocker SR59230. The Y axis is the rate of change compared to the basal heart rate (A, B) and contractility (C, D). SYN, synephrine; ISO, isoprenaline; Nebi, nebivolol; ICI, ICI118551; SR, SR59230.
4 Discussion
This study assesses the impact of ISO and SYN on arrhythmia risk and their underlying mechanisms, highlighting the potential of hiPSC-CM and MEA in predicting the risk of TdP induced by adrenomimetic drugs. SYN has a lower risk of arrhythmia at standard doses compared to ISO. Our findings also indicated that SYN's cardiovascular effects, including increased beating rate and myocardial contractility by directly stimulating β1, β2, and β3 receptors, are akin to those of ISO. The potential therapeutic mechanism of SYN on myocardial cells was presented in Figure 6. However, SYN's maximum influence on myocardial contractility and beating rate is less pronounced than ISO's. Notably, SYN demonstrates higher sensitivity in hiPSC-CMs compared to rat cardiomyocytes. The study suggests that ISO, a classic adrenomimetic drug, carries a higher risk of arrhythmia, whereas SYN has a lower risk of arrhythmia at clinical doses. Arrhythmia risk with SYN escalates at doses above 200 μM, significantly higher than the clinical dose. ISO has been clinically reported to have a higher risk of arrhythmia due to the shortening of the QT interval and the induction of TdP (37), which is consistent with our findings. Our study has investigated the SYN's arrhythmia risk in human cardiomyocytes, offering valuable insights for future research. In adrenomimetic drug studies, both shortening and prolonging of the FPD are noted (38), correlating with increased clinical arrhythmia risks. Thus, attention should be given not only to the prolongation of FPD, but also to arrhythmia risks from drug-induced FPD shortening. The International Council for Harmonisation (ICH) guidelines address arrhythmia risks associated with long QT intervals and drug-induced QT interval prolongation. The Comprehensive In vitro Proarrhythmia Assay (CIPA) project evaluates the risk of arrhythmia based on FPD prolongation, significantly benefiting preclinical cardiac safety assessment (39). However, current literature often overlooks the shortening of repolarization time by drugs, potentially leading to false-negative assessments of arrhythmia risks in drugs that shorten FPD. This study emphasizes the importance of monitoring FPD changes, and we have refined the comprehensive scoring system, contributing to the literature and reducing false-negative results to some extent. Nevertheless, further research with drugs known to have positive effects is necessary to improve the accuracy of the assessment.
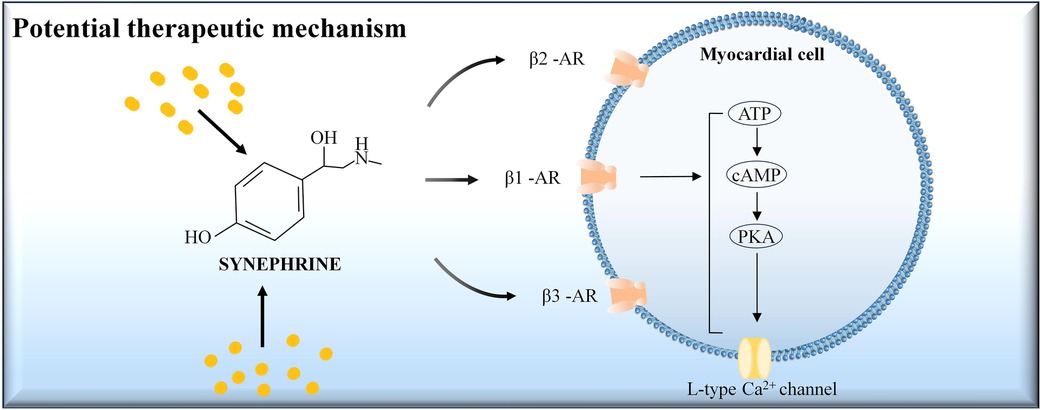
Figure 6. The potential therapeutic mechanism of synephrine on myocardial cells. Synephrine acts on beta-adrenergic receptors (β1-AR, β2-AR, and β3-AR) located on the myocardial cell membrane. Activation of these receptors stimulates the conversion of ATP to cAMP, which in turn activates protein kinase A (PKA). PKA phosphorylates the L-type Ca2+ channels, leading to increased calcium influx into the cell. This cascade enhances myocardial contractility and heart rate.
The study further indicates that SYN is more reactive to human-derived cardiomyocytes than to rat-derived cardiomyocytes. Remarkably, SYN's EC50 on human-derived cardiomyocytes is approximately tenfold different from that on neonatal rat cardiomyocytes. This finding contrasts with the previous report of phenylephrine's heightened sensitivity in rodents over humans (40). This discrepancy underscores that clinical reports may not always align with rodent study outcomes, especially regarding SYN's cardiovascular effects. Therefore, caution is advised when extrapolating rat study findings to human arrhythmia risk assessments. This underscores the value of hiPSC-CMs as preclinical experimental models. The observed changes in rise time and decay time induced by SYN and ISO may be attributed to their effects on calcium handling in cardiomyocytes. The reduced rise time suggests an increased rate of calcium release from the sarcoplasmic reticulum, leading to faster contraction. Conversely, the prolonged decay time indicates a slower rate of calcium reuptake into the sarcoplasmic reticulum, resulting in a prolonged relaxation phase. These effects are likely mediated through the activation of β-adrenergic receptors, which are known to modulate calcium cycling in cardiomyocytes via the cAMP/PKA signaling pathway (41). Further studies investigating the specific effects of SYN and ISO on calcium-handling proteins, such as ryanodine receptors and SERCA, could provide a more detailed understanding of the mechanisms underlying the observed changes in contractility parameters.
Interestingly, our findings contradict previous reports that claim SYN scarcely binds to β1 receptors and lacks cardiovascular effects (42). This discrepancy may be attributed to variations in research materials and dosages used. Our data reveal that SYN's EC50 is much higher than that of ISO. Therefore, we hypothesize that SYN may act as a partial agonist. This potential partial agonism could be influenced by SYN's chemical structure, notably the absence of alkyl substitution on the α carbon in its phenylethanolamine side chain and the presence of a single p-hydroxyl group in its benzene ring. These structural characteristics likely affect SYN's receptor binding and pharmacokinetics, resulting in its comparatively lower efficacy relative to ISO. Contrary to some reports suggesting SYN does not significantly activate β1 and β2 receptors or has low affinity for them (31, 43). Our study indicates SYN may stimulate cardiomyocyte beating rate and contractility by activating β1, β2, and β3 receptors. Previous research often utilized animal cells or organs, such as guinea pig atrium and trachea (44). SYN has been noted to activate β3 receptors, promoting fat breakdown. Given the increased expression of β3 receptors in heart failure cardiomyocytes (45), they could be a viable target for treatment. The differential responses elicited by ISO and SYN could be partly attributed to their distinct structural configurations and their consequent interactions with β-adrenergic receptors. ISO, with its classic phenethylamine structure, is known to have a high affinity for both β1 and β2 receptors, which can result in pronounced cardiostimulatory effects. On the other hand, SYN possesses a similar but not identical structure, with subtle differences that may influence receptor binding and efficacy. The partial agonism of SYN could explain the more tempered physiological response when compared to the full agonist activity of ISO. Further research into the binding kinetics and second messenger systems activated by these compounds could elucidate the nuances of their interactions with cardiac cells and the resulting electrophysiological effects. SYN's capability to increase heart rate suggests its potential in treating bradycardia. While ISO is known for its robust agonistic effects on β-adrenergic receptors, SYN, with its lower arrhythmogenic potential and moderate effects on heart rate and contractility, may offer therapeutic benefits for chronic bradycardia.
The distinct pharmacological profile of SYN could have implications for its use in clinical settings where moderate increases in contractility are desired, highlighting their ability to enhance contraction amplitude. Notably, SYN is proposed to have therapeutic potential in the treatment of chronic heart failure, especially in combination with slow arrhythmia. Generally, β-blockers are often recommended in the treatment guidelines for heart failure, as β-receptor activation accelerates heart rate, increasing myocardial oxygen consumption and potentially exacerbating heart failure. In advanced heart failure patients with bradycardia, SYN, as a partial agonist, could offer certain advantages. Partial agonists exhibit minimal physiological effects post-receptor binding. They also function antagonistically in the presence of full agonists or at high concentrations. SYN's treatment can accelerate heart rate and increase myocardial contractility without excessively increasing heart rate. Moreover, SYN's partial agonist nature may counteract physiological hormones like epinephrine or β-receptor agonists, avoiding excessive cardiac load for a more balanced therapeutic effect. Consequently, SYN shows promise for heart failure treatment, though in vivo studies are imperative for further validation.
It is worth noting that the concentrations of SYN and ISO used in our in vitro study are higher than their clinically relevant doses. The discrepancy between our experimental concentrations and clinical doses can be attributed to several factors. First, in vitro studies directly expose cardiomyocytes to the compounds, whereas in vivo administration requires consideration of pharmacokinetic processes such as absorption, distribution, metabolism, and excretion (46). Second, our study aimed to investigate the proarrhythmic potential of SYN and ISO, necessitating the use of a wide concentration range to fully assess their safety profiles. Clinical therapeutic doses are typically lower than those causing severe adverse effects. Third, hiPSC-CMs used in our study might have different drug sensitivities compared to human adult cardiomyocytes in vivo (47). Lastly, the primary goal of our study was to compare the relative potencies of SYN and ISO and elucidate their proarrhythmic mechanisms, rather than determining clinically equivalent doses. Further studies are needed to extrapolate our findings to clinical settings and establish safe and effective dosing regimens for SYN and ISO. The observed effects of SYN and ISO on hiPSC-CMs, particularly the changes in beating rate, contractility, and proarrhythmic potential, are likely to be clinically significant. These in vitro findings provide valuable insights into the potential cardiovascular risks associated with the use of these compounds, especially at high doses or in susceptible individuals.
While the use of hiPSC-CMs presents a robust in vitro model for studying cardiomyocyte behavior under drug exposure, the extrapolation of these findings to in vivo human heart physiology warrants a cautious approach. HiPSC-CMs, while closely resembling human cardiomyocytes, are not perfect replicas. Therefore, additional research is needed to understand the reasons behind the varied FPD responses to adrenomimetic drugs like SYN and ISO, the differential dose responses of SYN in humans and rats, and the unexplored in vivo cardiovascular effects of SYN, as well as its therapeutic potential for bradycardia and heart failure. Although hiPSC-CMs express vital cardiac markers and replicate key aspects of adult cardiomyocyte function, hiPSC-CMs may not entirely capture the complex interplay of cellular interactions, mechanical forces, and systemic factors present in the whole heart. The microenvironment in a living organism, including neurohormonal modulation, shear stress from blood flow, and the multicellular architecture of the heart, can significantly influence drug response. Consequently, while the proarrhythmic risks identified in this study provide valuable insights, they represent a facet of the overall cardiotoxicity profile that must be validated through comprehensive in vivo studies. Further investigations utilizing animal models and clinical trials are imperative to confirm the translatability of the proarrhythmic potential of SYN and ISO observed in hiPSC-CMs.
5 Conclusion
Our findings indicate that ISO poses a higher risk of arrhythmia, whereas SYN demonstrates a lower risk of arrhythmia at clinical doses. In addition, SYN is shown to increase beating rate and myocardial contractility by directly activating β1, β2 and β3 receptors. This suggests SYN may have significant clinical utility in the treatment of conditions like bradycardia and complex heart failure, highlighting the need for further research in these areas.
Data availability statement
The original contributions presented in the study are included in the article, further inquiries can be directed to the corresponding author.
Ethics statement
The animal study received approval from the Ethics Committee of Beijing University of Chinese Medicine (BUCM). The study was conducted in accordance with the local legislation and institutional requirements.
Author contributions
XY: Writing – original draft, Writing – review & editing, Conceptualization, Data curation, Formal Analysis, Investigation. TY: Conceptualization, Data curation, Formal Analysis, Investigation, Writing – review & editing. ZZ: Conceptualization, Data curation, Formal Analysis, Investigation, Writing – review & editing. SL: Writing – original draft, Writing – review & editing.
Funding
The author(s) declare financial support was received for the research, authorship, and/or publication of this article.
This study was supported by the National Natural Science Foundation of China (Grant No. 81973698 and 81703942), the Young Elite Scientists Sponsorship Program by CACM (Grant No. 2019-QNRC2-B08), the BUCM Precision Cultivation Program (Grant No. JZPY-202205), the Science Fund for Distinguished Young Scholars in BUCM (Grant No. BUCM-2019-JCRC004) and the BUCM Research Development Fund (Grant No. 2021-ZXFZJJ-052).
Conflict of interest
The authors declare that the research was conducted in the absence of any commercial or financial relationships that could be construed as a potential conflict of interest.
Publisher's note
All claims expressed in this article are solely those of the authors and do not necessarily represent those of their affiliated organizations, or those of the publisher, the editors and the reviewers. Any product that may be evaluated in this article, or claim that may be made by its manufacturer, is not guaranteed or endorsed by the publisher.
References
1. Stohs SJ. Assessment of the adverse event reports associated with Citrus aurantium (bitter orange) from April 2004 to October 2009. J Funct Foods. (2010) 2(4):235–8. doi: 10.1016/j.jff.2010.10.003
2. Jordan S, Murty M, Pilon K. Products containing bitter orange or synephrine: suspected cardiovascular adverse reactions. CMAJ. (2004) 171(8):993–4.15497209
3. Rossato LG, Costa VM, Limberger RP, Bastos Mde L, Remião F. Synephrine: from trace concentrations to massive consumption in weight-loss. Food Chem Toxicol. (2011) 49(1):8–16. doi: 10.1016/j.fct.2010.11.007
4. Gange CA, Madias C, Felix-Getzik EM, Weintraub AR, Estes NA 3rd. Variant angina associated with bitter orange in a dietary supplement. Mayo Clin Proc. (2006) 81(4):545–8. doi: 10.4065/81.4.545
5. Bouchard NC, Howland MA, Greller HA, Hoffman RS, Nelson LS. Ischemic stroke associated with use of an ephedra-free dietary supplement containing synephrine. Mayo Clin Proc. (2005) 80(4):541–5. doi: 10.4065/80.4.541
6. Stephensen TA, Sarlay R Jr. Ventricular fibrillation associated with use of synephrine containing dietary supplement. Mil Med. (2009) 174(12):1313–9. doi: 10.7205/MILMED-D-01-5009
7. Nasir JM, Durning SJ, Ferguson M, Barold HS, Haigney MC. Exercise-induced syncope associated with QT prolongation and ephedra-free xenadrine. Mayo Clin Proc. (2004) 79(8):1059–62. doi: 10.4065/79.8.1059
8. Penzak SR, Jann MW, Cold JA, Hon YY, Desai HD, Gurley BJ. Seville (sour) orange juice: synephrine content and cardiovascular effects in normotensive adults. J Clin Pharmacol. (2001) 41(10):1059–63. doi: 10.1177/00912700122012652
9. Hoffman JR, Kang J, Ratamess NA, Jennings PF, Mangine G, Faigenbaum AD. Thermogenic effect from nutritionally enriched coffee consumption. J Int Soc Sports Nutr. (2006) 3(1):35–41. doi: 10.1186/1550-2783-3-1-35
10. Hoffman JR, Kang J, Ratamess NA, Rashti SL, Tranchina CP, Faigenbaum AD. Thermogenic effect of an acute ingestion of a weight loss supplement. J Int Soc Sports Nutr. (2009) 6:1. doi: 10.1186/1550-2783-6-1
11. Bui LT, Nguyen DT, Ambrose PJ. Blood pressure and heart rate effects following a single dose of bitter orange. Ann Pharmacother. (2006) 40(1):53–7. doi: 10.1345/aph.1G488
12. Hansen DK, George NI, White GE, Pellicore LS, Abdel-Rahman A, Fabricant D. Physiological effects following administration of Citrus aurantium for 28 days in rats. Toxicol Appl Pharmacol. (2012) 261(3):236–47. doi: 10.1016/j.taap.2012.04.006
13. Calapai G, Firenzuoli F, Saitta A, Squadrito F, Arlotta MR, Costantino G, et al. Antiobesity and cardiovascular toxic effects of Citrus aurantium extracts in the rat: a preliminary report. Fitoterapia. (1999) 70(6):586–92. doi: 10.1016/S0367-326X(99)00093-3
14. Haller CA, Duan M, Jacob P 3rd, Benowitz N. Human pharmacology of a performance-enhancing dietary supplement under resting and exercise conditions. Br J Clin Pharmacol. (2008) 65(6):833–40. doi: 10.1111/j.1365-2125.2008.03144.x
15. Kaats GR, Miller H, Preuss HG, Stohs SJ. A 60day double-blind, placebo-controlled safety study involving Citrus aurantium (bitter orange) extract. Food Chem Toxicol. (2013) 55:358–62. doi: 10.1016/j.fct.2013.01.013
16. Shara M, Stohs SJ, Mukattash TL. Cardiovascular safety of oral p-synephrine (bitter orange) in healthy subjects: a randomized placebo-controlled cross-over clinical trial. Phytother Res. (2016) 30(5):842–7. doi: 10.1002/ptr.5590
17. Stohs SJ. Safety, efficacy, and mechanistic studies regarding Citrus aurantium (bitter orange) extract and p-synephrine. Phytother Res. (2017) 31(10):1463–74. doi: 10.1002/ptr.5879
18. Gutiérrez-Hellín J, Del Coso J. Effects of p-synephrine and caffeine ingestion on substrate oxidation during exercise. Med Sci Sports Exerc. (2018) 50(9):1899–906. doi: 10.1249/MSS.0000000000001653
19. Pellati F, Benvenuti S. Chromatographic and electrophoretic methods for the analysis of phenethylamine [corrected] alkaloids in Citrus aurantium. J Chromatogr A. (2007) 1161(1-2):71–88. doi: 10.1016/j.chroma.2007.05.097
20. Stohs SJ, Preuss HG, Shara M. The safety of Citrus aurantium (bitter orange) and its primary protoalkaloid p-synephrine. Phytother Res. (2011) 25(10):1421–8. doi: 10.1002/ptr.3490
21. Karakikes I, Ameen M, Termglinchan V, Wu JC. Human induced pluripotent stem cell-derived cardiomyocytes: insights into molecular, cellular, and functional phenotypes. Circ Res. (2015) 117(1):80–8. doi: 10.1161/CIRCRESAHA.117.305365
22. Blinova K, Stohlman J, Vicente J, Chan D, Johannesen L, Hortigon-Vinagre MP, et al. Comprehensive translational assessment of human-induced pluripotent stem cell derived cardiomyocytes for evaluating drug-induced arrhythmias. Toxicol Sci. (2017) 155(1):234–47. doi: 10.1093/toxsci/kfw200
23. Ma J, Guo L, Fiene SJ, Anson BD, Thomson JA, Kamp TJ, et al. High purity human-induced pluripotent stem cell-derived cardiomyocytes: electrophysiological properties of action potentials and ionic currents. Am J Physiol Heart Circ Physiol. (2011) 301(5):H2006–17. doi: 10.1152/ajpheart.00694.2011
24. Li S, Cheng H, Tomaselli GF, Li RA. Mechanistic basis of excitation-contraction coupling in human pluripotent stem cell-derived ventricular cardiomyocytes revealed by Ca2+ spark characteristics: direct evidence of functional Ca2+-induced Ca2+ release. Heart Rhythm. (2014) 11(1):133–40. doi: 10.1016/j.hrthm.2013.10.006
25. Li S, Chen G, Li RA. Calcium signalling of human pluripotent stem cell-derived cardiomyocytes. J Physiol. (2013) 591(21):5279–90. doi: 10.1113/jphysiol.2013.256495
26. McKeithan WL, Savchenko A, Yu MS, Cerignoli F, Bruyneel AAN, Price JH, et al. An automated platform for assessment of congenital and drug-induced arrhythmia with hiPSC-derived cardiomyocytes. Front Physiol. (2017) 8:766. doi: 10.3389/fphys.2017.00766
27. Braam SR, Tertoolen L, van de Stolpe A, Meyer T, Passier R, Mummery CL. Prediction of drug-induced cardiotoxicity using human embryonic stem cell-derived cardiomyocytes. Stem Cell Res. (2010) 4(2):107–16. doi: 10.1016/j.scr.2009.11.004
28. Clements M, Millar V, Williams AS, Kalinka S. Bridging functional and structural cardiotoxicity assays using human embryonic stem cell-derived cardiomyocytes for a more comprehensive risk assessment. Toxicol Sci. (2015) 148(1):241–60. doi: 10.1093/toxsci/kfv180
29. Harris K, Aylott M, Cui Y, Louttit JB, McMahon NC, Sridhar A. Comparison of electrophysiological data from human-induced pluripotent stem cell-derived cardiomyocytes to functional preclinical safety assays. Toxicol Sci. (2013) 134(2):412–26. doi: 10.1093/toxsci/kft113
30. Nakamura Y, Matsuo J, Miyamoto N, Ojima A, Ando K, Kanda Y, et al. Assessment of testing methods for drug-induced repolarization delay and arrhythmias in an iPS cell-derived cardiomyocyte sheet: multi-site validation study. J Pharmacol Sci. (2014) 124(4):494–501. doi: 10.1254/jphs.13248FP
31. Motiejunaite J, Amar L, Vidal-Petiot E. Adrenergic receptors and cardiovascular effects of catecholamines. Ann Endocrinol (Paris). (2021) 82(3-4):193–7. doi: 10.1016/j.ando.2020.03.012
32. Myagmar BE, Flynn JM, Cowley PM, Swigart PM, Montgomery MD, Thai K, et al. Adrenergic receptors in individual ventricular myocytes: the beta-1 and alpha-1B are in all cells, the alpha-1A Is in a subpopulation, and the beta-2 and beta-3 are mostly absent. Circ Res. (2017) 120(7):1103–15. doi: 10.1161/CIRCRESAHA.117.310520
33. Chlopcíková S, Psotová J, Miketová P. Neonatal rat cardiomyocytes–a model for the study of morphological, biochemical and electrophysiological characteristics of the heart. Biomed Pap Med Fac Univ Palacky Olomouc Czech Repub. (2001) 145(2):49–55. doi: 10.5507/bp.2001.011
34. Estevez MD, Wolf A, Schramm U. Effect of PSC 833, verapamil and amiodarone on Adriamycin toxicity in cultured rat cardiomyocytes. Toxicol in Vitro. (2000) 14(1):17–23. doi: 10.1016/S0887-2333(99)00087-9
35. Ando H, Yoshinaga T, Yamamoto W, Asakura K, Uda T, Taniguchi T, et al. A new paradigm for drug-induced torsadogenic risk assessment using human iPS cell-derived cardiomyocytes. J Pharmacol Toxicol Methods. (2017) 84:111–27. doi: 10.1016/j.vascn.2016.12.003
36. Sala L, van Meer BJ, Tertoolen LGJ, Bakkers J, Bellin M, Davis RP, et al. MUSCLEMOTION: a versatile open software tool to quantify cardiomyocyte and cardiac muscle contraction in vitro and in vivo. Circ Res. (2018) 122(3):e5–16. doi: 10.1161/CIRCRESAHA.117.312067
37. Roden DM. Drug-induced prolongation of the QT interval. N Engl J Med. (2004) 350(10):1013–22. doi: 10.1056/NEJMra032426
38. Ackerman MJ, Khositseth A, Tester DJ, Hejlik JB, Shen WK, Porter CB. Epinephrine-induced QT interval prolongation: a gene-specific paradoxical response in congenital long QT syndrome. Mayo Clin Proc. (2002) 77(5):413–21. doi: 10.1016/S0025-6196(11)62209-X
39. Wallis R, Benson C, Darpo B, Gintant G, Kanda Y, Prasad K, et al. CiPA challenges and opportunities from a non-clinical, clinical and regulatory perspectives. An overview of the safety pharmacology scientific discussion. J Pharmacol Toxicol Methods. (2018) 93:15–25. doi: 10.1016/j.vascn.2018.06.005
40. Blin N, Nahmias C, Drumare MF, Strosberg AD. Mediation of most atypical effects by species homologues of the beta 3-adrenoceptor. Br J Pharmacol. (1994) 112(3):911–9. doi: 10.1111/j.1476-5381.1994.tb13167.x
41. Papa A, Kushner J, Marx SO. Adrenergic regulation of calcium channels in the heart. Annu Rev Physiol. (2022) 84:285–306. doi: 10.1146/annurev-physiol-060121-041653
42. Stohs SJ, Preuss HG, Shara M. A review of the human clinical studies involving Citrus aurantium (bitter orange) extract and its primary protoalkaloid p-synephrine. Int J Med Sci. (2012) 9(7):527–38. doi: 10.7150/ijms.4446
43. Evans PD, Thonoor CM, Midgley JM. Activities of octopamine and synephrine stereoisomers on octopaminergic receptor subtypes in locust skeletal muscle. J Pharm Pharmacol. (1988) 40(12):855–61. doi: 10.1111/j.2042-7158.1988.tb06288.x
44. Jordan R, Midgley JM, Thonoor CM, Williams CM. Beta-adrenergic activities of octopamine and synephrine stereoisomers on guinea-pig atria and trachea. J Pharm Pharmacol. (1987) 39(9):752–4. doi: 10.1111/j.2042-7158.1987.tb06986.x
45. Arioglu-Inan E, Kayki-Mutlu G, Michel MC. Cardiac β(3) -adrenoceptors-A role in human pathophysiology? Br J Pharmacol. (2019) 176(14):2482–95. doi: 10.1111/bph.14635
46. Andrade EL, Bento AF, Cavalli J, Oliveira SK, Schwanke RC, Siqueira JM, et al. Non-clinical studies in the process of new drug development - Part II: good laboratory practice, metabolism, pharmacokinetics, safety and dose translation to clinical studies. Braz J Med Biol Res. (2016) 49(12):e5646. doi: 10.1590/1414-431X20165646
Keywords: p-synephrine (SYN), isoprenaline (ISO), adrenomimetic drugs, proarrhythmia, cardiovascular effect
Citation: Yuan X, Yu T, Zhang Z and Li S (2024) Non-invasive assessment of proarrhythmic risks associated with isoprenaline and the dietary supplement ingredient synephrine using human induced pluripotent stem cell-derived cardiomyocytes. Front. Cardiovasc. Med. 11:1407138. doi: 10.3389/fcvm.2024.1407138
Received: 26 March 2024; Accepted: 23 May 2024;
Published: 7 June 2024.
Edited by:
Yang Yang, First Affiliated Hospital of Zhengzhou University, ChinaReviewed by:
Xiangyang Guo, Emory University, United StatesJuan Qin, University of California, San Francisco, United States
Yi-Yue Zhang, Central South University, China
© 2024 Yuan, Yu, Zhang and Li. This is an open-access article distributed under the terms of the Creative Commons Attribution License (CC BY). The use, distribution or reproduction in other forums is permitted, provided the original author(s) and the copyright owner(s) are credited and that the original publication in this journal is cited, in accordance with accepted academic practice. No use, distribution or reproduction is permitted which does not comply with these terms.
*Correspondence: Sen Li, senli@bucm.edu.cn
†These authors have contributed equally to this work and share first authorship