Coumarin-1,2,3-triazole hybrids as leading-edge anticancer agents
- Laboratory of Chemistry, Department of Applied Sciences, Rajkiya Engineering College (Affiliated with Dr. A.P.J. Abdul Kalam Technical University Lucknow), Churk, India
Cancer is one of the most feared and dreaded diseases across the world. In clinical practice, a variety of anticancer agents of natural, semi-synthetic and synthetic origin exist, but they suffer from side effects and drug resistance, so they are insufficient to combat the disease. Coumarins are bicyclic benzene-pyrone-fused phytomolecules with a wide range of biological effects, including powerful anticancer activity on numerous cell lines. Additionally, they serve as an adaptable synthetic scaffold and research hub for medicinal chemists. On the other hand, triazoles are nitrogen-containing heterocycles having remarkable pharmacological effects including anticancer activities. Due to a better compatibility with the human metabolic system, the synthesis of nature inspired hybrid compounds as anticancer agents for a wide range of activity and fewer side effects is at the forefront of current research. In the last decade, huge research has been published on coumarin-1,2,3-triazole hybrids showing potent anticancer activities on various types of cancer. This review offers a recent, thorough literature compilation of contemporary research on the development of hybrid compounds based on coumarin-1,2,3-triazoles as potential anticancer leads throughout the previous 10 years.
Introduction
Cancer is the rapid creation of abnormal cells in the body, growing beyond their usual boundaries (Cooper and Hausman, 2007a; 2007b). The cancer cells can spread to other organs by invading the adjoining parts of the body, the process is called metastasis, which may be considered as the primary cause of death due to cancer (Cooper and Hausman, 2007b). Most of the cancer occurs in the form of malignant tumors with a few notable exceptions, such as leukemia (Cooper and Hausman, 2007a; 2007b). The common type of cancer, known as “sarcoma”, which affects the epithelial cells lining internal organs or the skin, includes lung cancer, skin cancer, pancreatic cancer, ovarian cancer, etc. The other forms of cancer are lymphoma, melanoma, myeloma and mixed type cancers (Cooper and Hausman, 2007a; New Global Cancer Data: GLOBOCAN, 2018). Across the world, the lung, prostate, colorectal, stomach and liver cancer are the most common types of cancer in men, whereas, breast, colorectal, lung, cervical and thyroid cancer are the most common among women (Bray et al., 2018; WHO, 2021). Out of total deaths across the globe in 2018, the one-sixth, accounting for an estimated 9.6 million were due to cancer (Bray et al., 2018). In United States, the projected new cancer cases and cancer deaths are 1,918,030 and 609,360 respectively, for the year 2022; specifying 350 deaths per day from lung cancer, the leading cause of cancer death (Siegel et al., 2022). In 2040, it is anticipated that there would be 29.5 million new instances of cancer per year and 16.4 million cancer-related deaths (National Cancer Institute, 2021). The common methods of cancer treatment are chemotherapy, radiation therapy or surgery with chemotherapy (Schirrmacher, 1985; Zugazagoitia et al., 2016). One or more ways of combinatorial treatment may be applied simultaneously based on the level of advancement in cancer. Some other therapies are hormone therapy, hyperthermia, immunotherapy, photodynamic therapy, stem cell transplant, surgery targeted therapy (Schirrmacher, 1985; Zugazagoitia et al., 2016). However, still the chemotherapy is the essential mode of cancer treatment. Although the burden of cancer is increasing globally, it is having a nearly unaffordable impact on the medical infrastructure in low- and middle-income nations, which is why the death rate from cancer is so high (Sung et al., 2021; Siegel et al., 2022).
Although there are many anticancer medications in use, the number of adverse effects and the emergence of resistance have rendered the current anticancer medications ineffective (New Global Cancer Data: GLOBOCAN, 2018). New chemotherapeutic drugs are always required for the treatment of diverse cancer kinds. The drugs based on natural products have always been a great choice for a medicinal chemist for the treatment of a variety of diseases and illness because of their syncing with human metabolic system (Dwivedi et al., 2014; Saxena et al., 2018; Upadhyay, 2019). More than half of the drugs approved by United States Food and Drug Administration (USFDA) are either natural product or their prototype in form of semi-synthetic or synthetic drug (Upadhyay et al., 2014, 2020; Newman and Cragg, 2020; Atanasov et al., 2021). Only 37% of the 974 small molecules that were developed as novel anticancer drugs between 1981 and 2006 were actually synthetic; the remainder were either naturally occurring, their derivatives, or modelled after natural products (Cragg and Newman, 2009). Of the 175 small molecules used as anticancer medications between the years of the 1940s and 2010, 131 (74.8%) are non-synthetic and 85 (48.6%) are truly either natural compounds or directly derived from them (Newman and Cragg, 2012). In this way, natural ingredients have served as medications for practically all prevalent illnesses and disabilities either directly or indirectly, and the process is still ongoing (Cragg and Newman, 2009; Newman and Cragg, 2012). With improvements in anticancer drug development and discovery over the past few decades, the FDA has approved more than 100 drugs for cancer treatments (Blagosklonny, 2004; Kinch, 2014). Currently available plant-based anticancer drugs fall into four categories: vinca alkaloids (vinblastine, vincristine, and vindesine), epipodophyllotoxins (etoposide and teniposide), taxanes (paclitaxel and docetaxel), and camptothecin derivatives (camptotecin and irinotecan) (Desai et al., 2008; Zaid et al., 2017; Yedjou et al., 2019). Based on their modes of action, these drugs can be broadly divided into two basic categories: cytotoxic and targeted agents (Masui et al., 2013; Winkler et al., 2014).
Both coumarin and 1,2,3-triazole moieties are reported to possess a diverse range of pharmacological activities including anticancer, anti-HIV, antimalarial, anti-tubercular, anti-microbial, anti-inflammatory, antioxidant, antiviral, and diabetic activities (Stefanachi et al., 2018; Bozorov et al., 2019). In the past 10 years, many biologically active pharmacophores with coumarin-1,2,3-triazole moiety have been created by synthetic chemists in an effort to obtain multi-targeted single molecules (Emami and Dadashpour, 2015; Upadhyay, 2021). The numerous advancements and uses of coumarin-1,2,3-triazole hybrid compounds as potential bioactive leads have already been outlined in a number of reviews (Emami and Dadashpour, 2015; Fan et al., 2018; Al-Warhi et al., 2020; Song et al., 2020; Alam, 2022). Significant work has been done in recent years on the design, synthesis, and assessment of numerous hybrid compounds based on coumarin-1,2,3-triazoles as anticancer agents, the compilation of which may generate important findings for the development of novel anticancer drugs. An effort has been made to outline recent advancements in the development of hybrid compounds based on coumarin-1,2,3-triazoles as anticancer agents in this stand-alone review. The original research articles were searched in the Pubmed and Google Scholar databases under the keywords “Coumarin-1,2,3-triazole hybrid molecules as anticancer agents”, “Coumarin-1,2,3-triazole + anticancer’, Coumarin-1,2,3-triazole + antiproliferative” etc. The considered range of publication year was January 2012-July 2022, under which more than twenty-five original research articles on various coumarin-1,2,3-triazole hybrids with anticancer activity on a total of thirty-two cancer cell lines were finally taken in consideration. The current review will enable researchers to develop novel anticancer coumarin-1,2,3-triazole hybrids that are far more precise and have greater activity.
Coumarin and 1,2,3-triazole: The scaffolds with broad range biological activities
Coumarin (2H-1-Benzopyran-2-one) are phenolic compounds which is association of β-pyrone rings with benzene, widely distributed in plants bacteria and fungi (Abyshev et al., 2006; Katsori and Hadjipavlou-Litina, 2014). Fundamentally, the benzopyrones have been split into two groups: benzo-α-pyrones, which are the primary class of coumarin, and benzo-γ-pyrones, which mostly contain flavonoids (Lacy and O’Kennedy, 2004; Lacy, 2005). In plants, most of the benzopyrones are reported to exist in the form of glycosides making them highly polar (Matos et al., 2015). Coumarins are a varied class of naturally occurring pharmacophores with a wide range of bioactivities viz. anti-inflammatory, antioxidant, antinociceptive, hepatoprotective, antithrombotic, antiviral, antimicrobial, antituberculosis, anticancer, antidepressant, antihyperlipidemic, anti-Alzheimer, anticholinesterase, and antiviral activities (Upadhyay et al., 2012; Katsori and Hadjipavlou-Litina, 2014; Kraljević et al., 2016; Singh et al., 2019; Wang et al., 2020). There are numerous clinically effective compounds, both natural and synthetic, containing coumarin scaffolds (Emami and Dadashpour, 2015; Upadhyay, 2019) (Figure 1). Coumarins are reported to possess anticancer activity with minimal side effects (Musa et al., 2008; Stefanachi et al., 2018; Rawat and Vijaya Bhaskar Reddy, 2022). They have the ability to regulate a wide variety of cellular pathways involved in cancer, including those involved in kinase inhibition, cell cycle arrest, angiogenesis, heat shock protein (HSP90) inhibition, telomerase inhibition, antimitotic activity, carbonic anhydrase inhibition, monocarboxylate transporters inhibition, aromatase inhibition, and sulfatase inhibition, each of which can be explored for specific anticancer activity (Thakur et al., 2015; Goud et al., 2020; Wu et al., 2020).
Nitrogen-based heterocyclic secondary metabolites are widely reported from plants, fungi, algae etc. Numerous heterocyclic compounds with nitrogen have been found to have a variety of pharmacological effects, including anti-cancer, anti-HIV, anti-malarial, anti-tubercular, anti-microbial, and antidiabetic properties (Joule, 2016; Shang et al., 2018; Gopalakrishnan et al., 2021). Because nitrogen can interact in a variety of ways with biological targets, both naturally occurring and synthesized nitrogen-based heterocyclic molecules have drawn the interest of medicinal chemists (Kerru et al., 2020; Upadhyay, 2021). The importance of nitrogen-based heterocycles can be judged not only in the form of approximately countless published research articles but also in the form of clinical molecules in the therapy of various diseases and debilities (Kerru et al., 2020; Gopalakrishnan et al., 2021). A lot of representative nitrogen heterocycles viz. Ciprofloxacin, Tazobactum, Cefatrizine (antibiotic); Oxiconazole, Clotrimazole (Antifungal); Celecoxib (Anti-inflammatory), Rimonabant (Anti-obesity), Difenamizole (Anti-analgesic), Fezolamine (Antidepressant), Bedaquiline (Anti-TB), Pitavastatin (Cholesterol-lowering agent), Gefitinib (Growth factor receptor (EGFR) tyrosine kinase inhibitor), Ezetimibe (Cholesterol absorption inhibitor), Clavulanic acid (β-Lactamase inhibitor) are in regular clinical practice (Kerru et al., 2020). Some of the noticeable nitrogen heterocycle-based molecules as anticancer drugs are Erlotinib, Lapatinib, Ibrutinib, Capecitabine, Folinic acid, Monastrol, Dacarbazine, and Carboxyamidotriazole (Perabo et al., 2004; Romero et al., 2004; Dorababu, 2020; Kerru et al., 2020; Upadhyay, 2021). Amongst all the nitrogen-based molecules, the 1,2,3-triazole is a privileged moiety in biologically active leads putting its importance in the field of medicinal chemistry (Upadhyay, 2021). Figure 2 shows a few examples of typical pharmaceuticals with a 1,2,3-triazole moiety. Due to the triazole unit’s strong dipole properties driven by the presence of three nitrogen atoms, a wide range of biological targets can be bound to it with a high affinity (Liang et al., 2021). The 1,2,3-triazoles have been claimed to have a variety of pharmacological effects as well as anticancer effects through a variety of mechanisms of action (Liang et al., 2021; Alam, 2022). Their anticancer property is due to inhibition of the enzymes that contribute to the development of this deadly disease, such as the carbonic anhydrases (CAs), thymidylate synthase (TS), aromatase, tryptophan-2,3-dioxygenase (TDO), vascular endothelial growth factor receptor (VEGFR), and epidermal growth factor receptor (EGFR) (Perabo et al., 2004; Liang et al., 2021).
Coumarin-1,2,3-triazole hybrid molecules as anticancer agents
Combining biologically relevant moieties with different modes of action to create hybrid therapeutic molecules is a brilliant strategy that may lead to the development of drugs with improved pharmacological properties for the treatment of complicated disorders, such as cancer (Shaveta et al., 2016; Mohamed and Abuo-Rahma, 2020). Hybrid drugs can affect several targets involved in the growth of cancer cells at once, and as a result, they have received a lot of attention in recent years (Xu et al., 2019a; Bozorov et al., 2019; Liang et al., 2021; Alam, 2022). Recently there is a burst in the synthesis and assessment of coumarin-1,2,3-triazole based anticancer hybrid molecules. The major anticancer hybrid molecules covered in this review are simple coumarin-1,2,3-triazoles, chalcone-coumarin-1,2,3-triazoles, 1,2,3-triazole tethered C5-curcuminoid coumarin hybrids, 1,2,3-triazole tethered isatin-coumarin hybrids, morpholines linked coumarin-1,2,3-triazole hybrids, tetraethylene glycol-tethered isatin–1,2,3-triazole–coumarin hybrids, coumarin-1,2,3-triazole-podophyllotoxin hybrids, α, β-unsaturated carbonyl linked coumarin-triazole hybrids, and 1,2,3-triazole-coumarin-glycosyl hybrids.
Duan et al. Synthesised a series of coumarin-1,2,3-triazole-dithiocarbamate hybrids, which displayed good anticancer activity against human gastric carcinoma (MGC-803) and human breast cancer (MCF-7) cell lines, but all the derivatives were inactive against esophageal cancer (EC-109) cell line (IC50 > 128 µM). The substitution of coumarin moiety with 1,2,3-triazole-dithiocarbamate residue at position 4 (derivative 1) led to potent anticancer activity against the MGC-803 (IC50 4.96 µM), MCF-7 (IC50 10.44 µM) and prostate cancer (PC-3) cell line (IC50 36.84 µM), while the substitution with same residue at position 7 led to molecules with decreased anticancer activity (derivatives 2a-b) in range of 67.05 µM–90.38 µM (Duan et al., 2013). A series of chalcone-coumarin derivatives linked by the 1,2,3-triazole ring (3–9) were prepared and assessed for cytotoxic activity against human liver bile duct (HuCCA-1), human hepatocellular (HepG2), adenocarcinomic human alveolar basal epithelial (A549) and human malignant T-lymphoblastic (MOLT-3) cancer cell lines by Pingaew et al. Most of the synthesized hybrids, except for hybrid 5, exhibited cytotoxicity against MOLT-3 cell line without affecting normal cells. SAR study systematically disclosed the effect of substitution pattern in both rings (A & B) of chalcone moiety. The hybrids with, 3/4 triazole at ring A and 2,3-di-OMe at ring B show potent HepG2 inhibitory effect as evident from IC50 values 15.70, 8.18 and 4.26 µM for compounds 4, 5 and 7 respectively. Meantime, hybrids with 3/4 triazole at ring A and tri-OMe substitution at ring B (8, 9) were shown to lose the cytotoxic effect against HuCCA-1, HepG2, A549 except for 8, which showed IC50 6.13 µM against HuCCA-1 (Pingaew et al., 2014). A series of 4-(1,2,3-triazol-1-yl)-coumarin derivatives were synthesized and evaluated for their anticancer activity against MCF-7, A549, and colorectal (SW480) human cancer cell lines. Most of the molecules exhibited significant antiproliferative activities. Compounds 10a-d exhibited IC50 values of 9.45, >50, 36.83 and 1.72 respectively against MCF-7 cell line, from which SAR may be established that–CH2O–bridge at C-4 position of 1,2,3-triazole nucleus on phenyl moiety is the best linker for cytotoxic activity (Zhang et al., 2014). Further, a close observation on 2/4 substituted phenoxy derivatives 11–23 (IC50 values 34.14, 28.91, 24.82, 12.67, 2.04, 1.92, 11.41, 7.00, 3.13, 5.84, 8.56, 4.62, and 5.89 μM respectively against MCF-7 cell line) reveals that the anticancer action is enhanced by the presence of a hydrogen bond acceptor, such as a fluorine atom at the C-4 position of the phenoxy moiety and a methoxy group at the C-7 position of coumarin (Zhang et al., 2014). Amongst all, the 4-(4-((4-fluorophenoxy) methyl)-1,2,3-triazol-1-yl)-7-methoxycoumarin (23) was found potentially active against all the tested cancer cell lines with IC50 values of 5.89, 1.99 and 0.52 μM against MCF-7, SW480 and A549 respectively. In further studies, the compound 23 was found to possess ability to arrest the cell-cycle at G2/M phase (Zhang et al., 2014). A series of 2-(4-R-triazolyl)-substituted 3-oxo-2,3-dihydrofurocoumarins was synthesized and evaluated against acute lymphoblastic leukaemia (CEM-13), membrane-type 4 (MT-4), myeloid leukaemia (U-937) human cancer cell lines using MTT assays. Most of the synthesized hybrids were active against the tested cell lines. It was observed that the selective cytotoxic activity to cancer cell lines interestingly increased in hybrid 24 bearing the 4-hydroxy-3-methoxybenzamidomethyl moiety on the triazole ring which showed 50% cytotoxic dose (CTD50) values 9.00, 10.00 and 8.00 µM respectively against CEM-13, MT-4, U-937 cancer cell lines. The hybrid 24 showed higher binding affinity (-9.2 kcal/mol) to active sites of the anticancer target PDE4B (Phosphodiesterase-4B), comparable to Rolipram (-8.3 kcal/mol), the standard drug (Lipeeva et al., 2015). The structure of coumarin-1,2,3-triazole hybrids 1–24 have been depicted in Figure 3.
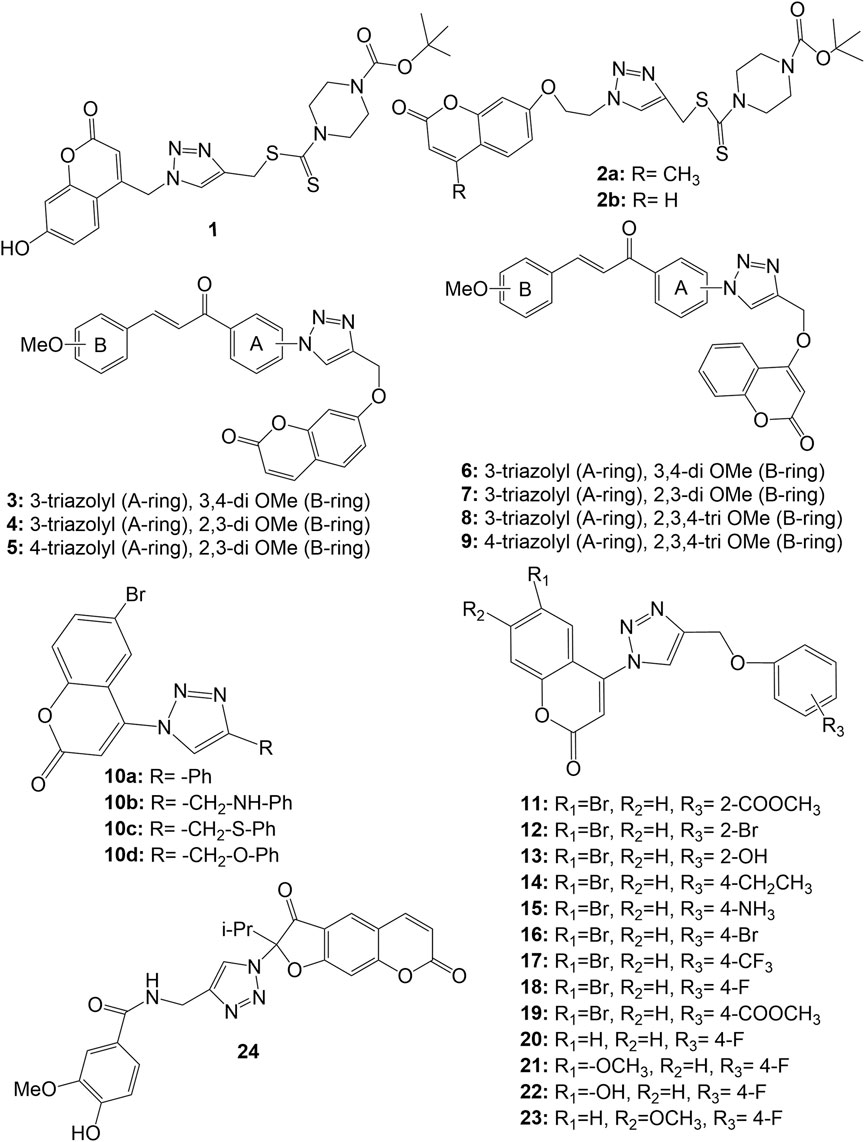
FIGURE 3. Structure of coumarin-1,2,3-triazole-dithiocarbamate hybrids (1,2), 1,2,3-triazole linked chalcone-coumarin hybrids (3–9), 4-(1,2,3-triazol-1-yl)-coumarin hybrids (10–23), and 2-(4-R-triazolyl)-substituted 3-oxo-2,3-dihydrofurocoumarin hybrid (24).
A series of 1,2,3-triazole tethered coumarin 4-substituted alkyl, phenyl, alkylphenyl, dithiocarbamate, benzenesulfonamide, and benzofused heterocycle subunit were designed and synthesized by Kraljevic et al. for the evaluation of in vitro antiproliferative activity against human cancer cell lines A549, HepG2, CFPAC-1 (ductal pancreatic), HeLa (cervical cancer) and SW620 (colorectal cancer). Most of the compounds showed inhibitory effects against A549 and HeLa cell lines (IC50 ≤ 30 μM). It was noticed that there is a strong correlation between lipophilicity and antiproliferative activities, suggesting that lipophilic 1,2,3-triazole-coumarin hybrids with the subunits phenylethyl (25), 3,5-difluorophenyl (26), 5-iodoindole (27) and benzimidazole (28 and 29) (Figure 4) may very well have cytostatic potential (Kraljević et al., 2016). The 7-methylcoumarin–1,2,3-triazole–2-methylbenzimidazole hybrid 28 showed maximum cytotoxicity against HepG2 cells with IC50 value of 0.9 μM with high selectivity (SI = 50). The strong antiproliferative activity of 28 may be related to its suppression of 5-lipoxygenase (5-LO) activity and disruption of sphingolipid signalling by interfering with intracellular acid ceramidase (ASAH) activity (Kraljević et al., 2016). Singh et al. synthesized 1,2,3-triazole tethered C5-curcuminoid coumarin bifunctional hybrids and evaluated them against THP-1 (acute monocytic leukemia), COLO-205 (colon), HCT-116 (colon) and PC-3 human cancer cell lines. The hybrid compounds 30a-b and 31 (Figure 4) exhibited cytotoxicity against THP-1, COLO-205, and HCT-116 with IC50 values ranging from 0.82 to 4.68, 2.34–6.78, and 4.48–9.95 μM respectively. The hybrid compounds, however, had no effect on the prostate cancer cell line (PC-3). It has been observed that the anticancer effect depends on the presence of a two-carbon spacer between the coumarin and triazole molecule (Singh et al., 2016). In another strategy to design novel anticancer hybrid molecules, the authors synthesized a series of 1,2,3-triazole tethered isatin-coumarin hybrids by applying click chemistry approach and evaluated their cytotoxic potential. The compounds 32–44 (Figure 4) displayed significant cytotoxicity against THP-1 (IC50 values 0.73, 1.99, 5.47, 6.43, 7.01, 8.57, 5.03, 5.76, 6.99, 7.14, 5.12, 7.10, 7.56 µM respectively), COLO-205 (IC50 values 3.45, 6.67, 8.87, 10.53, 12.01, 12.97, 5.71, 8.88, 10.66, 12.99, 7.67, 9.11, 13.14 respectively), and HCT-116 (IC50 values 3.04, 5.41, 5.77, 8.09, 8.99, 9.54, 5.18, 7.26, 9.66, 11.46, 8.05, 10.44, 12.76 respectively) human cancer cell lines. The most potent compound 32 displayed IC50 0.73, 3.45 and 3.04 µM against THP-1, COLO-205, and HCT-116 respectively, and also endowed with most prominent tubulin polymerization inhibition potential with an IC50 value of 1.06 μM. Compound 33 having fluoro substitution on isatin, exhibited IC50 1.99, 6.67, and 5.41 µM against THP-1, COLO-205, and HCT-116 respectively. SAR indicates that both increase in length of carbon-bridge connecting isatin moiety with triazole ring, and substitution on isatin retards the cytotoxic potential (Singh et al., 2017). Goud et. al. synthesized a series of morpholine linked coumarin-1,2,3-triazole hybrids and evaluated against five human cancer cell lines, namely, bone (MG-63), lung (A549), breast (MDA-MB-231), colon (HCT-15) and liver (HepG2), using MTT assay. Among the synthesized, compounds 45–53 (Figure 4) showed far better activity than the standard drug cisplatin against MG-63 and A549 cancer cell lines except 47 (IC50 19.47 µM) against MG-63. The compounds 46 and 50 showed better cytotoxic activity than cisplatin against MDA-MB-231, HCT-15, and HepG2 cancer cell lines than cisplatin. Among all, compound 50 was found most potent, showing IC50 values 0.80, 2.97, 4.05, 3.93, and 7.19 µM against MG-63, A549, HCT-15, MDA-MB-231, and HepG2 respectively. Meantime, cisplatin showed IC50 17.42, 24.15, 19.45, 16.97, and 25.73 µM against MG-63, A549, HCT-15, MDA-MB-231, and HepG2 respectively. It was concluded that dimethylmorpholine derivatives with an electron-withdrawing group viz. 50 and 51 are more cytotoxic than simple morpholine derivatives like 45, 46, and 48. The compound 50 induced sub-G1 phase arrest, increased apoptosis, and promoted the production of reactive oxygen species justifying the potential cytotoxic activity (Goud et al., 2019). In vitro anticancer activities of several isatin-1,2,3 triazole-tethered coumarin hybrids were investigated against HepG2, Hela, A549, DU145 (prostate cancer), SKOV3 (ovarian carcinoma), MCF-7, and drug-resistant MCF-7/DOX (doxorubicin-resistant MCF-7) human cancer cell lines. The hybrids 54–55 (Figure 4) showed weak to moderate (IC50 17.96 to >50 μM) in vitro anticancer activities. SAR indicated that hydrogen-bond donor–NOH group at C-3, and electron-donating methyl group at C-5 positions of isatin motif could significantly enhance the anticancer activity as evident in case of hybrid 55 (Diao et al., 2019).
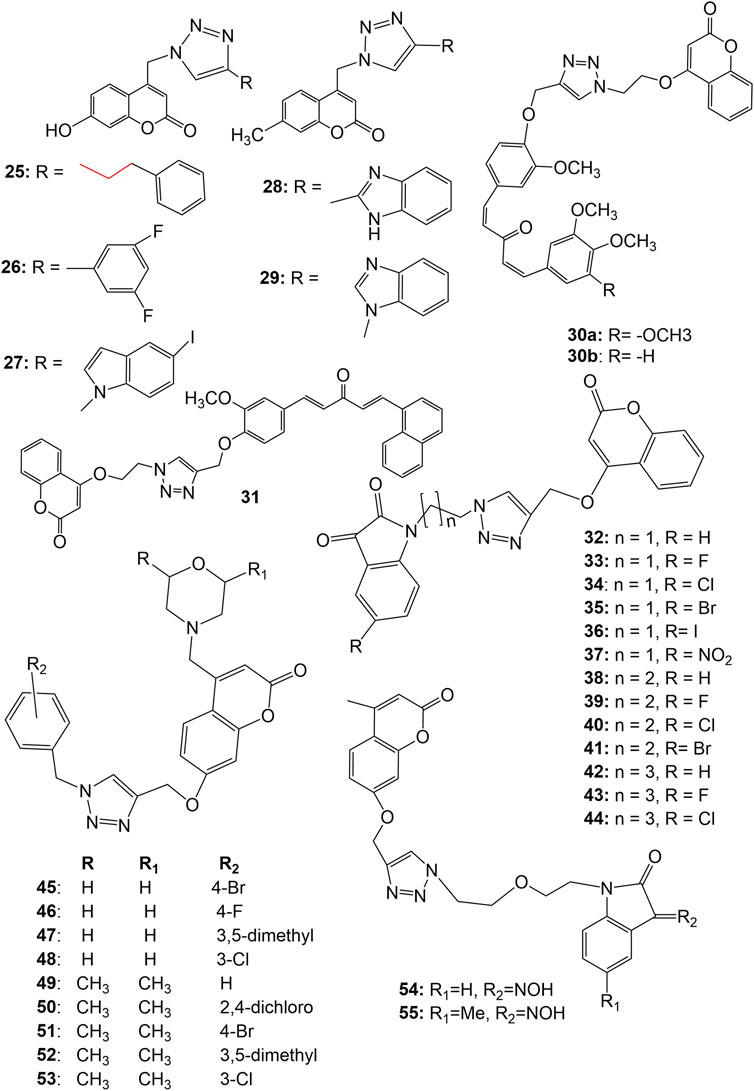
FIGURE 4. Structure of 1,2,3-triazole tethered coumarin 4-substituted hybrids (25–29), 1,2,3-triazole tethered C5-curcuminoid coumarin hybrids (30–31), isatin-1,2,3 triazole-4-coumarin hybrids (32–44), morpholines linked coumarin-1,2,3-triazole hybrids (45–53), isatin-1,2,3 triazole-7-coumarin hybrids (54–55).
Xu et al. synthesized a series of tetraethylene glycol-tethered isatin–1,2,3-triazole–coumarin hybrids and assessed for in vitro cytotoxic activities against seven human cancer cell lines, namely HepG2, Hela, A549, DU145 (prostate), SKOV3 (ovarian adenocarcinoma), MCF-7 and MCF-7/DOX. Weak to moderate anticancer activity were displayed by the hybrid compounds. The hybrids 56–58 (Figure 5) were found active against all the tested cell lines in a range of IC50 values 20.09–49.24 µM. The SAR indicated that the electron withdrawing group at C-5 position of isatin motif enhances the anticancer activity (Xu et al., 2019b). Hao et al. synthesized a series of coumarin-1,2,3-triazole-podophyllotoxin hybrid molecules using the click reaction, and evaluated for their cytotoxic potential against A549, HepG2, HeLa and LoVo (colorectal) cancer cell lines using the MTT assay. Most of the hybrids exhibited equivalent or slightly weaker cytotoxic activities in all the 4 cell lines compared with that of etoposide (VP-16), the standard drug. The hybrids 59 exhibited IC50 8.6, 9.3, 15.2, and 8.7; while 60 exhibited IC50 13.3, 13.6, 19.8, and 7.3 μM against A549, HepG2, HeLa, and LoVo cells, respectively. Among all, hybrid 61 exhibited the most potent cytotoxicity, with IC50 values of 17.5, 9.9, 9.7, and 4.9 μM against A549, HepG2, HeLa, and LoVo cells, respectively, which was far better than the standard VP-16 (IC50 25.6, 10.5, 12.2, and 14.9 μM against A549, HepG2, HeLa, and LoVo cells, respectively). Additional research showed that compound 61 entered into the DNA of LoVo cells, selectively inhibited Topo IIβ than Topo IIα, arrested the cell cycle in the G1 phase, and disrupted microtubule organisation. It was observed that 1,2,3-triazole-4-methylamine is a more suitable linker than 1,2,3-triazole-4-methyloxy in this series conjugates (Hao et al., 2019). Narsimha et al. synthesized various hybrid molecules using 3-fluoro-4-morpholinophenyl-1,2,3-triazolyl moiety. Among them the hybrid having 7-hydroxy-4-methylcoumarin at fourth position of 1,2,3-triazole (62), exhibited potent activity against both MCF-7 and HeLa cell lines with IC50 values 3.12 and 2.77 μM, respectively. The cytotoxic activity of 62 was comparable to the standard drug doxorubicin (IC50 values 2.63 μM in MCF-7 and 1.23 μM in HeLa cells). The cytotoxic activity of the hybrid having 4-hydroxycoumarin at fourth position of 1,2,3-triazole (63) also exhibited significant cytotoxicity against the two cancer cell lines MCF-7 and HeLa with IC50 values 5.19 and 12.42 μM, respectively. Both the compounds were almost inactive (IC50 values 58.11 and 66.38 μM respectively) against HEK-293 cancer cell lines (Narsimha et al., 2020). Coumarin-tagged β-lactam triazole hybrids (64a-b) showed moderate cytotoxic activity against MCF-7 cancer cell lines with IC50 values of 53.55 and 58.62 μM, respectively. Both the derivatives showed high binding affinity (binding energies −11.3 and −10.9 kcal/mol respectively) with the target estrogen receptor-α (ER-α) (Dhawan et al., 2020). A range of bifunctional molecular hybrids based on uracil and coumarin, roped with 1,2,3-triazole moiety were synthesized and assessed against six human cancer cell lines, namely Colo-205, MCF-7, A549, PA-1 (ovarian), PC-3 (prostate) and Hela cells by Sulforhodamine B assay. The synthesized molecules (65–68) were potentially active against MCF-7 cancer cell proliferation (Sanduja et al., 2020). The compounds 65a-f, 66a-e, 67a-c and 68a-b showed 50% cell growth inhibitory concentration (GI50) 1.55–6.88 µM, even better than the standard drug 5-Fluorouracil (GI50 5.28 µM). The compounds 67d, 67e, 68c, 68d, and 68e (Figure 5) also exhibited potential activity against MCF-7 cancer cell line with GI50 values 7.75, 9.77, 7.29, 9.33, and 12.24 µM respectively. Amongst all, 65a (with a fluorine atom as R with two carbon chain length between triazole and coumarin moieties) was found to be a most potent hybrid (GI50 = 1.55 µM). The hybrid 65a strongly inhibited the proliferation of MCF-7 cells by deferring the G2/M phase in cell cycle analysis studies. Further, in attempt to gain insight on interactions between 65a and the tubulin protein, docking studies were performed. The results indicated that 65a fit well at the interface of α2 and β1 subunits of tubulin (vinblastine binding site) and is stabilized by hydrogen bonding as well as van der Waal’s interactions suggesting it a potential lead for the discovery and development of novel treatments of breast cancer (Sanduja et al., 2020). Noticeable that, hybrids with substituted uracil (electron withdrawing or electron donating) were more active than the hybrids with unsubstituted uracil. With the length of the chain increasing, the hybrid molecules’ activity reduced. Fluorine-substituted hybrids had the strong activity, and halogen substitution at C5 of uracil was found necessary for the activity (Sanduja et al., 2020). A series of α, β-unsaturated carbonyl linked coumarin-1,2,3-triazole hybrids, 69–71 were synthesized by microwave irradiation conditions and were screened in vitro for their anticancer activity. The hybrid 70 was found most potent, exhibiting IC50 10.538 and 9.845 µM respectively against PC-3 and DU-145 cell lines. The hybrids 69 and 71 were equally effective against PC-3 cell lines (IC5016.254 and 16.652 µM respectively) (Vagish et al., 2021). Figure 5 shows the structure of the coumarin-1,2,3-triazole hybrids 56–71, and Supplementary Tables S1, S2 of the supplementary material contains in vitro cytotoxicity potential of these hybrids against various cancer cell lines.
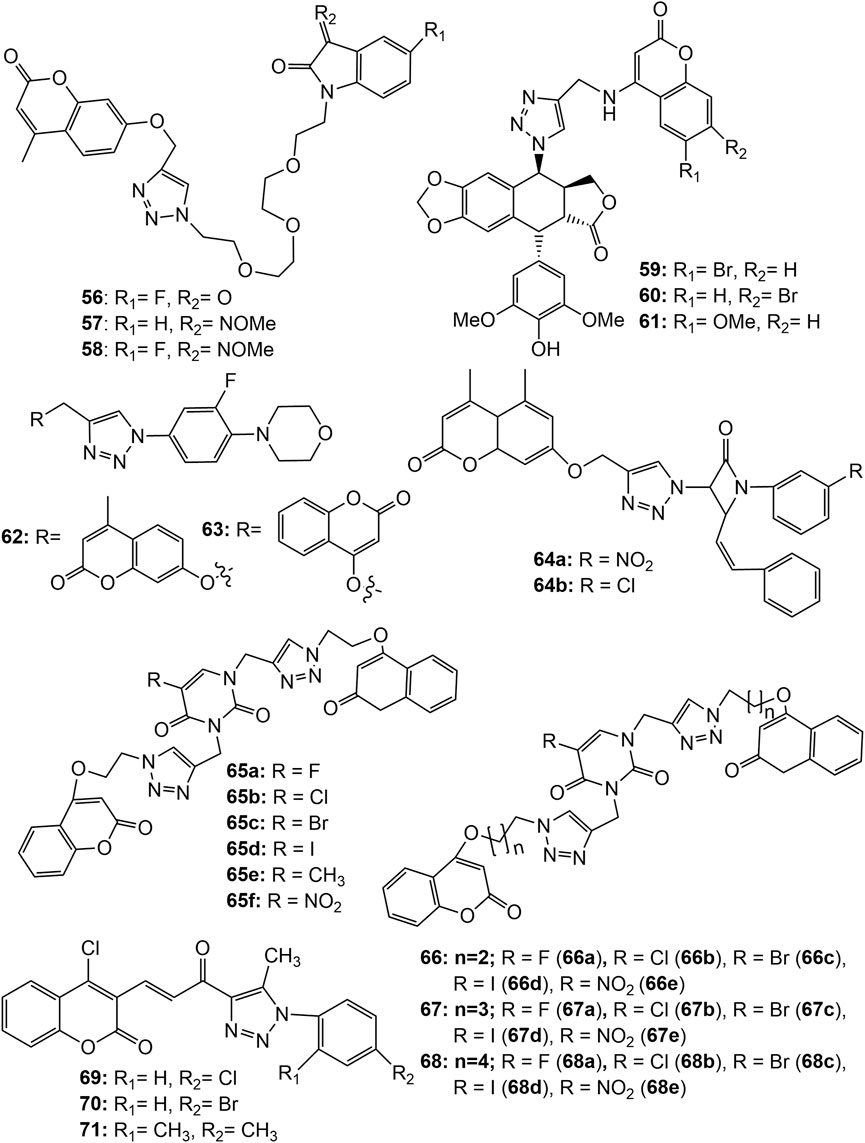
FIGURE 5. Structure of tetraethylene glycol-tethered isatin–1,2,3-triazole–coumarin hybrids (56–58), coumarin-1,2,3-triazole-podophyllotoxin hybrids (59–61), 3-fluoro-4-morpholinophenyl-1,2,3-triazolyl coumarin hybrids (62–63), Coumarin-tagged β-lactam triazole hybrid (64), 1,2,3-triazole roped uracil-coumarin bifunctional hybrids (65–68), α, β-unsaturated carbonyl linked coumarin-1,2,3-triazole hybrids (69–71).
The Zinc-binding enzymes called human carbonic anhydrases (hCAs) catalyse the reversible hydration of carbon dioxide to bicarbonate (Kumar et al., 2021). Various hCA isoforms have been isolated, and are reported to play crucial physiological roles. The over expressing of hCA isoforms, I, II, IX, and XII is found in maintaining neutral intracellular pH necessary for tumour cells to tolerate hypoxic conditions, hence, hCA inhibitors may be a good anticancer agent (Pastorek et al., 2008; Singh et al., 2018; Supuran, 2018; Al-Warhi et al., 2020). Many hCA IX/XII inhibitors belonging to the coumarin and sulfocoumarin classes completed a successful Phase I clinical trial for the treatment of advanced, metastatic solid tumors (Pastorek et al., 2008). In an attempt to search novel hCA inhibitors, a series of bis-coumarin derivatives linked with 1,2,3-triazole ring and alkyl chain (72a-d) (Figure 6) were synthesized. The derivatives showed selective inhibitory activity against hCA isoforms IX and XII. Among them 72c showed highest hCA IX inhibition (Ki = 144.6 nM) while 72b showed the highest hCA XII inhibition (Ki = 71.5 nM). The derivatives showed potent in vitro cytotoxicity (IC50 0.383–13.552 µM) against renal adenocarcinoma (769P), HepG2 and MDA-MB-231 cell lines. The derivative 72a showed the strongest cytotoxic effect against HepG2 with an IC50 value of 0.383 μM, which is almost 2-fold more than that of standard drug Doxorubicin (Kurt et al., 2019). The results were fully supported by in silico binding energies with hCA isoform in docking studies (Kurt et al., 2019). Another series of hybrids comprising coumarin-1,2,3-triazole-benzaldehyde and coumarin-1,2,3-triazole-sulphonamide were synthesized and evaluated for their hCA I, II, IX, and XII inhibition potential. All the coumarin-1,2,3-triazole-benzaldehyde hybrids (73–76) (Figure 6) were not inhibiting hCA I, II and XII (Ki > 10,000 nM) except 73 which showed strong inhibition to XII (Ki = 60.9 nM). The hybrid 73 was also a potential inhibitor of IX (Ki = 127.5 nM) and showed strong cytotoxic effect against HT-29 (human colorectal adenocarcinoma) cancer cell line exhibiting IC50 value of 18.89 µM. The hybrids 74, 75, and 76 showed IC50 values 13.3, 9.03, and 7.47 µM respectively against HT-29 cancer cell lines (Zengin Kurt et al., 2019). The coumarin-1,2,3-triazole-sulphonamide hybrids (77–80) (Figure 6) were found to be potential inhibitors of hCA II, IX, and XII at nanomolar levels of Ki. Further, hybrids, 77, 78, 79 and 80 showed IC50 values 14.29, 23.34, 17.7, and 17.01 µM respectively. Noticeable that except 80, rest of the derivatives were toxic to healthy HEK293T embryonic kidney cell lines, hence hybrid 80 may be a promising anticancer drug candidate (Zengin Kurt et al., 2019). Recently, a series of 6-coumarin-linked 4-anilinomethyl-1,2,3-triazole was synthesized and evaluated for their inhibitory potential against various hCA isoforms. Compounds 81a-e (Figure 6) exhibited the best inhibitory profiles (Ki < 100 nM) against both against CA IX, and XII (Thacker et al., 2021). New analogues of triazole-coumarin-glycosyl hybrids were synthesized and screened for their cytotoxic activities against Paca-2 (pancreatic), Mel-501 (female malignant), PC-3 (prostate) and A-375 (malignant human melanoma) cancer cell lines. The hybrids 82–84 (Figure 6) possessed potent cytotoxic activity showing 94.7–99.6% inhibition of cell growth. The hybrid molecules 82, 83, and 84 exhibited IC50 14.6, 33.4 and 16.9 µM respectively against Paca-2, and 16.7, 65.1 and 4.1 µM respectively against Mel-501 cancer cell lines. The in vitro cytotoxicity of 82 and 84 against Paca-2 were far better than the standard drug Doxorubicin (IC50 19.4 µM) (El-Sayed et al., 2022). However, the results were much more promising for coumarin-tetrazole derivative synthesized by the authors which displayed potent cytotoxic activity, broad superior inhibitory activity and high in silico binding affinity against anticancer enzymes EGFR (epidermal growth factor receptor), VEGFR-2 (Vascular endothelial growth factor receptor 2) and Cyclin dependent kinase 2 (CDK-2)/cyclin A2 Kinases (El-Sayed et al., 2022).
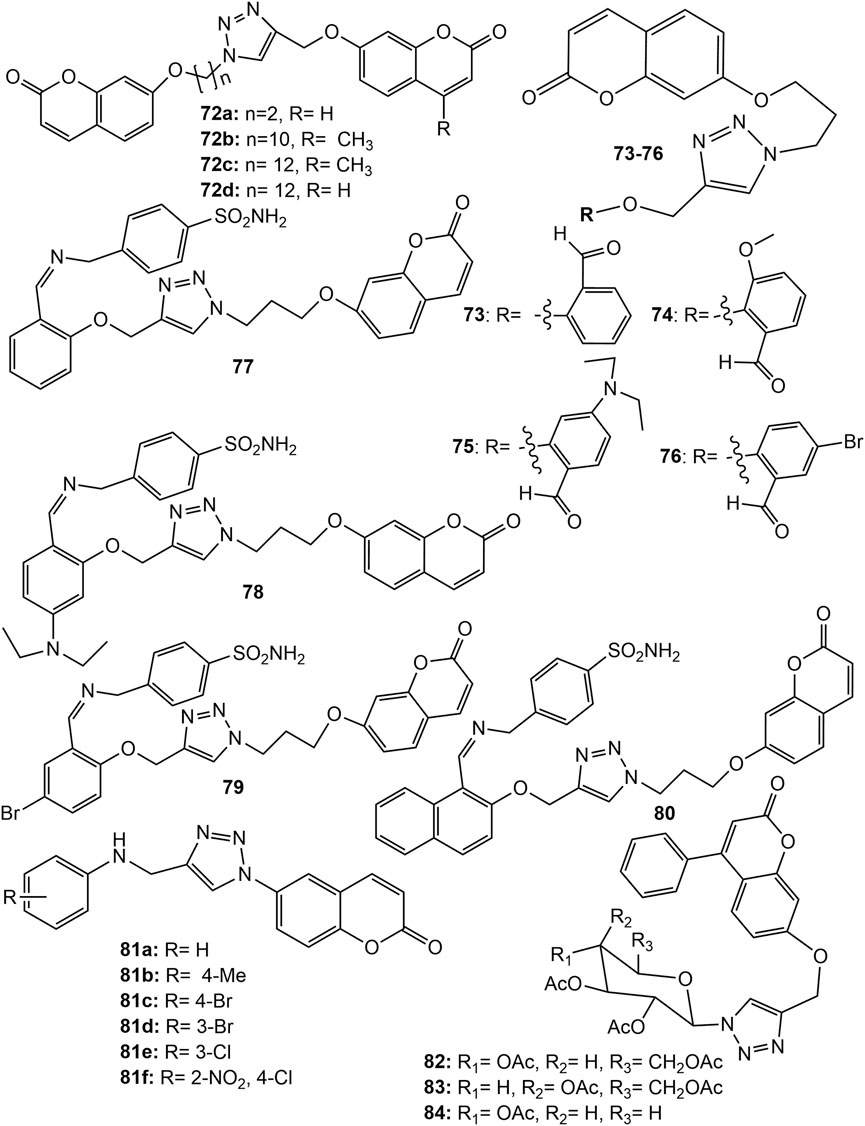
FIGURE 6. Structure of 1,2,3-triazole linked bis-coumarin hybrid (72), coumarin-1,2,3-triazole-benzaldehyde hybrids (73–76), coumarin-1,2,3-triazole-sulphonamide hybrids (77–80), 6-coumarin-linked 4-anilinomethyl-1,2,3-triazole hybrid (81), 1,2,3-triazole-coumarin-glycosyl hybrids (82–84).
Overall SAR could be established for most potent coumarin-1,2,3-triazole anticancer hybrids. Numerous attempts have been made for modifying different positions of coumarin nucleus resulting in a large number of compounds having a diverse mechanism of actions. Most of the efforts have been made onF modifications at positions 4 and 7 of coumarin moiety. Attachment of 1,2,3-triazole moiety at positions 4 and 7 of coumarin seems crucial for anticancer activity. A further substitution of triazole moiety by aromatic/heteroaromatic benzo-fused rings with methylene linker enhances the anticancer activity. The SAR for some of the pharmacophores with potential cytotoxic activity have been depicted in Figure 7.
Conclusion
Millions of individuals worldwide are impacted by cancer each year, making it one of the major causes of mortality. Although both coumarin and 1,2,3-triazole moieties have proven anticancer properties, the single target drug therapies are insufficient for the treatment of cancer. The design of hybrid anticancer drugs by combining coumarin and 1,2,3-triazole moieties may produce a drug with a broader range of action acting simultaneously on multiple targets. Various coumarin-1,2,3-triazole hybrids discussed in this review have shown potential anticancer activities in terms of IC50 comparable to standard clinical drugs against various cancer cell lines. The anticancer activity of potentially active hybrids has been further reported to be justified by selective inhibition of different enzymes such as EGFR, VEGFR-2 and CDK-2/cyclin A2 Kinases, Topo IIβ, and Topo IIα, involved in the progression of cancer, arrest of the cell cycle in G1 phase, and disturbing microtubule organization. Due to much better anticancer activity than clinically used reference drugs and interesting preliminary reports on mode of action, great opportunity can be seen for some of the hybrids viz. 46, 50, 61, 65–68 reported in this review, hence further studies are recommended to explore them as a potential multi-targeted anticancer drug for clinical use. The present review represents an avenue for medicinal chemists to design and develop novel coumarin-1,2,3-triazole anticancer hybrids with better efficacy and fewer side effects.
Author contributions
KM and HU conceptualized, collected the information, designed and wrote the manuscript.
Acknowledgments
The authors thank Prof. Geetam Singh Tomar, Director, Rajkiya Engineering College Sonbhadra for the facilities and constructive support.
Conflict of interest
The authors declare that the research was conducted in the absence of any commercial or financial relationships that could be construed as a potential conflict of interest.
Publisher’s note
All claims expressed in this article are solely those of the authors and do not necessarily represent those of their affiliated organizations, or those of the publisher, the editors and the reviewers. Any product that may be evaluated in this article, or claim that may be made by its manufacturer, is not guaranteed or endorsed by the publisher.
Supplementary material
The Supplementary Material for this article can be found online at: https://www.frontiersin.org/articles/10.3389/fddsv.2022.1072448/full#supplementary-material
References
Abyshev, A. Z., Gindin, V. A., Semenov, E. v., Agaev, E. M., Abdulla-Zade, A. A., and Guseinov, A. B. (2006). Structure and biological properties of 2H-1-benzopyran-2-one (coumarin) derivatives. Pharm. Chem. J. 40, 607–610. doi:10.1007/s11094-006-0203-7
Al-Warhi, T., Sabt, A., Elkaeed, E. B., and Eldehna, W. M. (2020). Recent advancements of coumarin-based anticancer agents: An up-to-date review. Bioorg. Chem. 103, 104163. doi:10.1016/j.bioorg.2020.104163
Alam, M. M. (2022). 1, 2, 3-triazole hybrids as anticancer agents: A review. Arch. Pharm. 355, e2100158. doi:10.1002/ardp.202100158
Atanasov, A. G., Zotchev, S. B., Dirsch, V. M., Orhan, I. E., Banach, M., Rollinger, J. M., et al. (2021). Natural products in drug discovery: Advances and opportunities. Nat. Rev. Drug Discov. 20, 200–216. doi:10.1038/s41573-020-00114-z
Blagosklonny, M. v. (2004). Analysis of FDA approved anticancer drugs reveals the future of cancer therapy. Cell Cycle 3, 1033–1040. doi:10.4161/cc.3.8.1023
Bozorov, K., Zhao, J., and Aisa, H. A. (2019). 1, 2, 3-Triazole-containing hybrids as leads in medicinal chemistry: A recent overview. Bioorg. Med. Chem. 27, 3511–3531. doi:10.1016/j.bmc.2019.07.005
Bray, F., Ferlay, J., Soerjomataram, I., Siegel, R. L., Torre, L. A., and Jemal, A. (2018). Global cancer statistics 2018: GLOBOCAN estimates of incidence and mortality worldwide for 36 cancers in 185 countries. Ca. Cancer J. Clin. 68, 394–424. doi:10.3322/caac.21492
Cooper, G. M., and Hausman, R. E. (2007b). The development and causes of cancer. The cell: A molecular approach.
Cragg, G. M., and Newman, D. J. (2009). Nature: A vital source of leads for anticancer drug development. Phytochem. Rev. 8, 313–331. doi:10.1007/s11101-009-9123-y
Desai, A., Qazi, G., Ganju, R., El-Tamer, M., Singh, J., Saxena, A., et al. (2008). Medicinal plants and cancer chemoprevention. Curr. Drug Metab. 9, 581–591. doi:10.2174/138920008785821657
Dhawan, S., Awolade, P., Kisten, P., Cele, N., Pillay, A. S., Saha, S. T., et al. (2020). Synthesis, cytotoxicity and antimicrobial evaluation of new coumarin-tagged β-lactam triazole hybrid. Chem. Biodivers. 17, e1900462. doi:10.1002/cbdv.201900462
Diao, Q. P., Guo, H., and Wang, G. Q. (2019). Design, synthesis, and in vitro anticancer activities of diethylene glycol tethered isatin-1, 2, 3-triazole-coumarin hybrids. J. Heterocycl. Chem. 56, 1667–1671. doi:10.1002/jhet.3538
Dorababu, A. (2020). Report on recently (2017–20) designed quinoline-based human cancer cell growth inhibitors. ChemistrySelect 5, 13902–13915. doi:10.1002/slct.202003888
Duan, Y. C., Ma, Y. C., Zhang, E., Shi, X. J., Wang, M. M., Ye, X. W., et al. (2013). Design and synthesis of novel 1, 2, 3-triazole-dithiocarbamate hybrids as potential anticancer agents. Eur. J. Med. Chem. 62, 11–19. doi:10.1016/j.ejmech.2012.12.046
Dwivedi, G. R., Upadhyay, H. C., Yadav, D. K., Singh, V., Srivastava, S. K., Khan, F., et al. (2014). 4-Hydroxy-α-tetralone and its derivative as drug resistance reversal agents in multi drug resistant Escherichia coli. Chem. Biol. Drug Des. 83, 482–492. doi:10.1111/cbdd.12263
El-Sayed, W. A., Alminderej, F. M., Mounier, M. M., Nossier, E. S., Saleh, S. M., and Kassem, A. F. (2022). Novel 1, 2, 3-triazole-coumarin hybrid glycosides and their tetrazolyl analogues: Design, anticancer evaluation and molecular docking targeting EGFR, VEGFR-2 and CDK-2. Molecules 27, 2047. doi:10.3390/molecules27072047
Emami, S., and Dadashpour, S. (2015). Current developments of coumarin-based anti-cancer agents in medicinal chemistry. Eur. J. Med. Chem. 102, 611–630. doi:10.1016/j.ejmech.2015.08.033
Fan, Y. L., Ke, X., and Liu, M. (2018). Coumarin–triazole hybrids and their biological activities. J. Heterocycl. Chem. 55, 791–802. doi:10.1002/jhet.3112
Gopalakrishnan, A. K., Angamaly, S. A., and Velayudhan, M. P. (2021). An insight into the biological properties of imidazole-based schiff bases: A review. ChemistrySelect 6, 10918–10947. doi:10.1002/slct.202102619
Goud, N. S., Kumar, P., and Bharath, R. D. (2020). Recent developments of target based coumarin derivatives as potential anticancer agents. Mini Rev. Med. Chem. 20, 1754–1766. doi:10.2174/1389557520666200510000718
Goud, N. S., Pooladanda, V., Mahammad, G. S., Jakkula, P., Gatreddi, S., Qureshi, I. A., et al. (2019). Synthesis and biological evaluation of morpholines linked coumarin–triazole hybrids as anticancer agents. Chem. Biol. Drug Des. 94, 1919–1929. doi:10.1111/cbdd.13578
Hao, S. Y., Feng, S. L., Wang, X. R., Wang, Z., Chen, S. W., and Hui, L. (2019). Novel conjugates of podophyllotoxin and coumarin: Synthesis, cytotoxicities, cell cycle arrest, binding CT DNA and inhibition of Topo IIβ. Bioorg. Med. Chem. Lett. 29, 2129–2135. doi:10.1016/j.bmcl.2019.06.063
Joule, J. A. (2016). Natural products containing nitrogen heterocycles—some highlights 1990–2015. Adv. Heterocycl. Chem. 119, 81–106. doi:10.1016/BS.AIHCH.2015.10.005
Katsori, A. M., and Hadjipavlou-Litina, D. (2014). Coumarin derivatives: An updated patent review (2012-2014). Expert Opin. Ther. Pat. 24, 1323–1347. doi:10.1517/13543776.2014.972368
Kerru, N., Gummidi, L., Maddila, S., Gangu, K. K., and Jonnalagadda, S. B. (2020). A review on recent advances in nitrogen-containing molecules and their biological applications. Molecules 25, E1909. doi:10.3390/molecules25081909
Kinch, M. S., Patridge, E., Plummer, M., and Hoyer, D. (2014). An analysis of FDA-approved drugs for infectious disease: Antibacterial agents. Drug Discov. Today 19, 1283–1287. doi:10.1016/j.drudis.2014.07.005
Kraljević, T. G., Harej, A., Sedić, M., Pavelić, S. K., Stepanić, V., Drenjančević, D., et al. (2016). Synthesis, in vitro anticancer and antibacterial activities and in silico studies of new 4-substituted 1, 2, 3-triazole–coumarin hybrids. Eur. J. Med. Chem. 124, 794–808. doi:10.1016/j.ejmech.2016.08.062
Kumar, S., Rulhania, S., Jaswal, S., and Monga, V. (2021). Recent advances in the medicinal chemistry of carbonic anhydrase inhibitors. Eur. J. Med. Chem. 209, 112923. doi:10.1016/j.ejmech.2020.112923
Kurt, B. Z., Dag, A., Doğan, B., Durdagi, S., Angeli, A., Nocentini, A., et al. (2019). Synthesis, biological activity and multiscale molecular modeling studies of bis-coumarins as selective carbonic anhydrase IX and XII inhibitors with effective cytotoxicity against hepatocellular carcinoma. Bioorg. Chem. 87, 838–850. doi:10.1016/j.bioorg.2019.03.003
Lacy, A., and O'Kennedy, R. (2005). Studies on coumarins and coumarin-related compounds to determine their therapeutic role in the treatment of cancer. Curr. Pharm. Des. 10, 3797–3811. doi:10.2174/1381612043382693
Lacy, A., and O’Kennedy, R. (2004). Studies on coumarins and coumarin-related compounds to determine their therapeutic role in the treatment of cancer. Curr. Pharm. Des. 10, 3797–3811. doi:10.2174/1381612043382693
Liang, T., Sun, X., Li, W., Hou, G., and Gao, F. (2021). 1, 2, 3-triazole-containing compounds as anti–lung cancer agents: Current developments, mechanisms of action, and structure–activity relationship. Front. Pharmacol. 12, 661173. doi:10.3389/fphar.2021.661173
Lipeeva, A. v., Pokrovsky, M. A., Baev, D. S., Shakirov, M. M., Bagryanskaya, I. Y., Tolstikova, T. G., et al. (2015). Synthesis of 1 H -1, 2, 3-triazole linked aryl(arylamidomethyl) - dihydrofurocoumarin hybrids and analysis of their cytotoxicity. Eur. J. Med. Chem. 100, 119–128. doi:10.1016/j.ejmech.2015.05.016
Masui, K., Gini, B., Wykosky, J., Zanca, C., Mischel, P. S., Furnari, F. B., et al. (2013). A tale of two approaches: Complementary mechanisms of cytotoxic and targeted therapy resistance may inform next-generation cancer treatments. Carcinogenesis 34, 725–738. doi:10.1093/carcin/bgt086
Matos, M. J., Santana, L., Uriarte, E., Abreu, O. A., Molina, E., and Yordi, E. G. (2015). “Coumarins — an important class of phytochemicals,” in Phytochemicals - isolation, characterisation and role in human health. doi:10.5772/59982
Mohamed, M. F. A., and Abuo-Rahma, G. E. D. A. (2020). Molecular targets and anticancer activity of quinoline-chalcone hybrids: Literature review. RSC Adv. 10, 31139–31155. doi:10.1039/d0ra05594h
Musa, M., Cooperwood, J., and Khan, M. O. (2008). A review of coumarin derivatives in pharmacotherapy of breast cancer. Curr. Med. Chem. 15, 2664–2679. doi:10.2174/092986708786242877
Narsimha, S., Nukala, S. K., Savitha Jyostna, T., Ravinder, M., Srinivasa Rao, M., and Vasudeva Reddy, N. (2020). One-pot synthesis and biological evaluation of novel 4-[3-fluoro-4-(morpholin-4-yl)]phenyl-1H-1, 2, 3-triazole derivatives as potent antibacterial and anticancer agents. J. Heterocycl. Chem. 57, 1655–1665. doi:10.1002/jhet.3890
National Cancer Institute (2021). Cancer statistics - national cancer Institute. Availableat: https://www.cancer.gov/about-cancer/understanding/statistics. October 3.
New Global Cancer Data: GLOBOCAN (2018). New global cancer Data: GLOBOCAN 2018. The global cancer observatory.
Newman, D. J., and Cragg, G. M. (2012). Natural products as sources of new drugs over the 30 years from 1981 to 2010. J. Nat. Prod. 75, 311–335. doi:10.1021/np200906s
Newman, D. J., and Cragg, G. M. (2020). Natural products as sources of new drugs over the nearly four decades from 01/1981 to 09/2019. J. Nat. Prod. 83, 770–803. doi:10.1021/acs.jnatprod.9b01285
Pastorek, J., Pastorekova, S., and Zatovicova, M. (2008). Cancer-associated carbonic anhydrases and their inhibition. Curr. Pharm. Des. 14, 685–698. doi:10.2174/138161208783877893
Perabo, F. G. E., Wirger, A., Kamp, S., Lindner, H., Schmidt, D. H., Müller, S. C., et al. (2004). Carboxyamido-triazole (CAI), a signal transduction inhibitor induces growth inhibition and apoptosis in bladder cancer cells by modulation of Bcl-2. Anticancer Res. 24, 2869–2877.
Pingaew, R., Saekee, A., Mandi, P., Nantasenamat, C., Prachayasittikul, S., Ruchirawat, S., et al. (2014). Synthesis, biological evaluation and molecular docking of novel chalcone-coumarin hybrids as anticancer and antimalarial agents. Eur. J. Med. Chem. 85, 65–76. doi:10.1016/j.ejmech.2014.07.087
Rawat, A., and Vijaya Bhaskar Reddy, A. (2022). Recent advances on anticancer activity of coumarin derivatives. Eur. J. Med. Chem. Rep. 5, 100038. doi:10.1016/j.ejmcr.2022.100038
Romero, D. H., Heredia, V. E. T., García-Barradas, O., López, M. E. M., Pavón, E. S., Narasimhan, B., et al. (2004). Recent advancement in imidazole as anti cancer agents : A review. Phosphorus Sulfur Silicon Relat. Elem. doi:10.1016/j.tet.2004.03.016
Sanduja, M., Gupta, J., Singh, H., Pagare, P. P., and Rana, A. (2020). Uracil-coumarin based hybrid molecules as potent anti-cancer and anti-bacterial agents. J. Saudi Chem. Soc. 24, 251–266. doi:10.1016/j.jscs.2019.12.001
Saxena, A., Upadhyay, H. C., Cheema, H. S., Srivastava, S. K., Darokar, M. P., and Bawankule, D. U. (2018). Antimalarial activity of phytol derivatives: In vitro and in vivo study. Med. Chem. Res. 27, 1345–1354. doi:10.1007/s00044-017-2132-2
Schirrmacher, V. (1985). Cancer metastasis: Experimental approaches, theoretical concepts, and impacts for treatment strategies. Adv. Cancer Res. 43, 1–73. doi:10.1016/S0065-230X(08)60942-2
Shang, X. F., Morris-Natschke, S. L., Yang, G. Z., Liu, Y. Q., Guo, X., Xu, X. S., et al. (2018). Biologically active quinoline and quinazoline alkaloids part II. Med. Res. Rev. 38, 1614–1660. doi:10.1002/med.21492
Shaveta, , Mishra, S., and Singh, P. (2016). Hybrid molecules: The privileged scaffolds for various pharmaceuticals. Eur. J. Med. Chem. 124, 500–536. doi:10.1016/j.ejmech.2016.08.039
Siegel, R. L., Miller, K. D., Fuchs, H. E., and Jemal, A. (2022). Cancer statistics, 2022. Ca. Cancer J. Clin. 72, 7–33. doi:10.3322/caac.21708
Singh, H., Kumar, M., Nepali, K., Gupta, M. K., Saxena, A. K., Sharma, S., et al. (2016). Triazole tethered C5-curcuminoid-coumarin based molecular hybrids as novel antitubulin agents: Design, synthesis, biological investigation and docking studies. Eur. J. Med. Chem. 116, 102–115. doi:10.1016/j.ejmech.2016.03.050
Singh, H., Singh, J. V., Bhagat, K., Gulati, H. K., Sanduja, M., Kumar, N., et al. (2019). Rational approaches, design strategies, structure activity relationship and mechanistic insights for therapeutic coumarin hybrids. Bioorg. Med. Chem. 27, 3477–3510. doi:10.1016/j.bmc.2019.06.033
Singh, H., Singh, J. v., Gupta, M. K., Saxena, A. K., Sharma, S., Nepali, K., et al. (2017). Triazole tethered isatin-coumarin based molecular hybrids as novel antitubulin agents: Design, synthesis, biological investigation and docking studies. Bioorg. Med. Chem. Lett. 27, 3974–3979. doi:10.1016/j.bmcl.2017.07.069
Singh, S., Lomelino, C. L., Mboge, M. Y., Frost, S. C., and McKenna, R. (2018). Cancer drug development of carbonic anhydrase inhibitors beyond the active site. Molecules 23, E1045. doi:10.3390/molecules23051045
Song, X. F., Fan, J., Liu, L., Liu, X. F., and Gao, F. (2020). Coumarin derivatives with anticancer activities: An update. Arch. Pharm. 353, e2000025. doi:10.1002/ardp.202000025
Stefanachi, A., Leonetti, F., Pisani, L., Catto, M., and Carotti, A. (2018). Coumarin: A natural, privileged and versatile scaffold for bioactive compounds. Molecules 23, E250. doi:10.3390/molecules23020250
Sung, H., Ferlay, J., Siegel, R. L., Laversanne, M., Soerjomataram, I., Jemal, A., et al. (2021). Global cancer statistics 2020: GLOBOCAN estimates of incidence and mortality worldwide for 36 cancers in 185 countries. Ca. Cancer J. Clin. 71, 209–249. doi:10.3322/caac.21660
Supuran, C. T. (2018). Carbonic anhydrase inhibitors as emerging agents for the treatment and imaging of hypoxic tumors. Expert Opin. Investig. Drugs 27, 963–970. doi:10.1080/13543784.2018.1548608
Thacker, P. S., Tiwari, P. L., Angeli, A., Srikanth, D., Swain, B., Arifuddin, M., et al. (2021). Synthesis and biological evaluation of coumarin-linked 4-anilinomethyl-1, 2, 3-triazoles as potent inhibitors of carbonic anhydrases ix and xiii involved in tumorigenesis. Metabolites 11, 225. doi:10.3390/metabo11040225
Thakur, A., Singla, R., and Jaitak, V. (2015). Coumarins as anticancer agents: A review on synthetic strategies, mechanism of action and SAR studies. Eur. J. Med. Chem. 101, 476–495. doi:10.1016/j.ejmech.2015.07.010
Upadhyay, H. C. (2021). Coumarin-1, 2, 3-triazole hybrid molecules: An emerging scaffold for combating drug resistance. Curr. Top. Med. Chem. 21, 737–752. doi:10.2174/1568026621666210303145759
Upadhyay, H. C., Dwivedi, G. R., Roy, S., Sharma, A., Darokar, M. P., and Srivastava, S. K. (2014). Phytol derivatives as drug resistance reversal agents. ChemMedChem 9, 1860–1868. doi:10.1002/cmdc.201402027
Upadhyay, H. C., Jaiswal, N., Tamrakar, A. K., Srivastava, A. K., Gupta, N., and Srivastava, S. K. (2012). Antihyperglycemic agents from Ammannia multiflora. Nat. Product. Commun. 7, 1934578X1200700–900. doi:10.1177/1934578x1200700724
Upadhyay, H. C. (2019). Medicinal chemistry of alternative therapeutics: Novelty and hopes with genus ammannia. Curr. Top. Med. Chem. 19, 784–794. doi:10.2174/1568026619666190412101047
Upadhyay, H. C., Singh, M., Prakash, O., Khan, F., Srivastava, S. K., and Bawankule, D. U. (2020). QSAR, ADME and docking guided semi-synthesis and in vitro evaluation of 4-hydroxy-α-tetralone analogs for anti-inflammatory activity. SN Appl. Sci. 2, 2069. doi:10.1007/s42452-020-03798-5
Vagish, C. B., Kumara, K., Vivek, H. K., Bharath, S., Lokanath, N. K., and Ajay Kumar, K. (2021). Coumarin-triazole hybrids: Design, microwave-assisted synthesis, crystal and molecular structure, theoretical and computational studies and screening for their anticancer potentials against PC-3 and DU-145. J. Mol. Struct. 1230, 129899. doi:10.1016/j.molstruc.2021.129899
Wang, G., Sun, S., Wu, B., and Liu, J. (2020). Coumarins as potential anti-drug resistant cancer agents: A mini review. Curr. Top. Med. Chem. 21, 1725–1736. doi:10.2174/1568026620999201113110041
Winkler, G. C., Barle, E. L., Galati, G., and Kluwe, W. M. (2014). Functional differentiation of cytotoxic cancer drugs and targeted cancer therapeutics. Regul. Toxicol. Pharmacol. 70, 46–53. doi:10.1016/j.yrtph.2014.06.012
Wu, Y., Xu, J., Liu, Y., Zeng, Y., and Wu, G. (2020). A review on anti-tumor mechanisms of coumarins. Front. Oncol. 10, 592853. doi:10.3389/fonc.2020.592853
Xu, Z., Zhao, S. J., and Liu, Y. (2019a). 1, 2, 3-Triazole-containing hybrids as potential anticancer agents: Current developments, action mechanisms and structure-activity relationships. Eur. J. Med. Chem. 183, 111700. doi:10.1016/j.ejmech.2019.111700
Xu, Z., Zhao, S. J., Lv, Z. S., Gao, F., Wang, Y. L., Zhang, F., et al. (2019b). Design, synthesis, and evaluation of tetraethylene glycol-tethered isatin–1, 2, 3-triazole–coumarin hybrids as novel anticancer agents. J. Heterocycl. Chem. 56, 1127–1132. doi:10.1002/jhet.3475
Yedjou, C. G., Mbemi, A. T., Noubissi, F., Tchounwou, S. S., Tsabang, N., Payton, M., et al. (2019). Prostate cancer disparity, chemoprevention, and treatment by specific medicinal plants. Nutrients 11, E336. doi:10.3390/nu11020336
Zaid, H., Silbermann, M., Amash, A., Gincel, D., Abdel-Sattar, E., and Sarikahya, N. B. (2017). Medicinal plants and natural active compounds for cancer chemoprevention/chemotherapy. Evid. Based. Complement. Altern. Med. 2017, 7952417. doi:10.1155/2017/7952417
Zengin Kurt, B., Sonmez, F., Ozturk, D., Akdemir, A., Angeli, A., and Supuran, C. T. (2019). Synthesis of coumarin-sulfonamide derivatives and determination of their cytotoxicity, carbonic anhydrase inhibitory and molecular docking studies. Eur. J. Med. Chem. 183, 111702. doi:10.1016/j.ejmech.2019.111702
Zhang, W., Li, Z., Zhou, M., Wu, F., Hou, X., Luo, H., et al. (2014). Synthesis and biological evaluation of 4-(1, 2, 3-triazol-1-yl)coumarin derivatives as potential antitumor agents. Bioorg. Med. Chem. Lett. 24, 799–807. doi:10.1016/j.bmcl.2013.12.095
Keywords: chemotherapy, drug resistance, hybrid molecules, coumarin, triazole, anticancer, anticytotoxic, apoptosis
Citation: Mishra KN and Upadhyay HC (2022) Coumarin-1,2,3-triazole hybrids as leading-edge anticancer agents. Front. Drug. Discov. 2:1072448. doi: 10.3389/fddsv.2022.1072448
Received: 17 October 2022; Accepted: 22 November 2022;
Published: 06 December 2022.
Edited by:
Cristina Maccallini, University of Studies G. d'Annunzio Chieti and Pescara, ItalyReviewed by:
Marwa Balaha, Kafrelsheikh University, EgyptAfzal Basha Shaik, Jawaharlal Nehru Technological University, India
Copyright © 2022 Mishra and Upadhyay. This is an open-access article distributed under the terms of the Creative Commons Attribution License (CC BY). The use, distribution or reproduction in other forums is permitted, provided the original author(s) and the copyright owner(s) are credited and that the original publication in this journal is cited, in accordance with accepted academic practice. No use, distribution or reproduction is permitted which does not comply with these terms.
*Correspondence: Harish C. Upadhyay, harishcu@gmail.com