Periodontal Ligament Cells Are Involved in the Formation of Intracanal Cementum-Like Tissue After Regenerative Endodontic Procedures: A Mouse in situ Model
- 1Department of Operative Dentistry, Cariology and Pulp Biology, Tokyo Dental College, Tokyo, Japan
- 2Tokyo Dental College, Tokyo, Japan
- 3Division in Anatomy and Developmental Biology, Department of Oral Biology, Oral Science Research Center, BK21 FOUR Project, Yonsei University College of Dentistry, Seoul, South Korea
Regenerative endodontic cell-homing procedures are frequently performed on injured immature teeth diagnosed with pulp necrosis and/or apical periodontitis. The representative histological finding after those procedures is cementum-like tissues filling in the root canal but details of the healing process remain unknown. We investigated that healing process histologically using a mouse in situ model. Regenerative endodontic procedures were experimentally performed on noninfected maxillary first molars of 6-week-old male C57BL/6 mice, after which the healing process was investigated using histology and immunohistochemistry. Immediately after the regenerative endodontic cell-homing procedures, blood clots were seen in the root canals that disappeared over time. On day 7, the blot clot in the root canal was replaced by granulation tissue. From day 14 onward, cementum-like tissues were filled in the root canals, while the amount of fibrous tissue was reduced. Immunohistochemically, positive reactions for periostin were seen in the fibrous tissue in the root canal, the apex, and periodontal ligament cells. On the other hand, positive reactions for nestin were not detected in the root canal. CD31-positive cells with a luminal structure were also observed in the fibrous tissue around the apex and around the newly formed cementum-like tissues in the root canal. Thus, in this study, we have established an in situ mouse model of regenerative endodontic procedures. The results of this study suggest that periodontal ligament cells and vascular endothelial cells grow into the root canals from the apex, replace the blood clots, and participate in the formation of cementum-like tissues with angiogenesis during the healing process of regenerative endodontic procedures.
Introduction
Endodontic treatment of immature permanent teeth due to dental caries or trauma is frequently encountered. Conventionally, apexification has been performed as a therapy for immature permanent teeth that has resulted in pulp necrosis or apical periodontitis (1, 2). That therapy promotes hard tissue formation at the dilated apical region of the root and closes the region (3), but root development with the thickness of the root canal wall and apical formation does not occur. As a result, teeth show a poor prognosis such as root fracture (4–7) and new therapies that overcome that disadvantage were needed.
In recent years, regenerative endodontics (pulp revascularization) has been in the limelight as a new regenerative endodontic therapy that overcomes that shortcoming. Since the first description by Iwaya et al. (8), many successful cases of pulp revascularization for immature permanent teeth with infected root canals have been reported (8–13). Regenerative endodontics has been defined as “biologically-based procedures designed to physiologically replace damaged tooth structures, including dentin and root structures, as well as cells of the pulp-dentin complex” (14, 15). The American Association of Endodontists has advocated the clinical use of this therapy, stating “After cleaning and disinfecting the root canal, the apical tissue is intentionally damaged mechanically and bleeding causes a blood clot in the root canal.” As a result, the thickness of the root canal wall and narrowing of the root canal space, and continuous root elongation and apical closure are observed radiographically (16, 17). Previous case reports showed histological findings of human immature permanent teeth after regenerative endodontics (18–21). The representative findings were fibrous connective tissue with bone-like or cementum-like tissue within the root canal, and no pulp-like tissue including odontoblasts or dentin-like hard tissue was observed. To investigate the wound healing process histologically after regenerative endodontics, animal models have been used. Earlier experimental models using large animals (22–28) are clinically similar to humans and can provide a large number of teeth for the experiment; however, small animals are easy to handle and more economical. However, one problem with experimental models using large animals is that it is difficult to perform detailed histological investigations during the healing process over time. Furthermore, the cells involved in the healing process, such as mesenchymal stem cells, vascular cells, and periodontal ligament (PDL) cells, were not well characterized. Moreover, the process of cell migration into the root canal and new hard tissue formation that was added to the root canal wall and the apical region has not been fully elucidated.
To overcome these problems, establishing a small animal model that can easily elucidate histological changes over time is necessary, and we, therefore, developed a mouse experimental model of regenerative endodontics. The purpose of this study was to elucidate the involvement of migrating cells into the root canal immunohistochemically and the formation of hard tissue added to the root canal wall and the apical region.
Materials and Methods
Animals
All animal experiments were performed according to the Guidelines for the Treatment of Animals at the Tokyo Dental College (Approval No. 212301). Eighty-three 6-week-old C57BL/6 male mice, which still had thin root dentin with wide root apex, were used in these experiments. Seven mice were used at each time point for histological and immunohistochemical analyses. In total, thirty-four mice that had their temporary seals removed during the postoperative course and had root canal infection or apical lesions were excluded.
The experimental group was a group in which blood clots were formed on the mesial root of the right maxillary first molar (M1) and then covered and filled. The untreated left maxillary first molars on the opposite side were used as a control.
Experimental Procedure of Regenerative Endodontics
The procedure was performed according to the method of Xu et al. (29). General anesthesia was administered intraperitoneally using a mixed anesthetic of medetomidine hydrochloride (0.3 mg/kg, Nippon Zenyaku Kogyo, Koriyama, Japan), midazolam (4 mg/kg, Astellas Pharma, Tokyo, Japan), and butorphanol tartrate (5 mg/kg, Meiji Seika Pharma, Tokyo, Japan). After the operative field was disinfected with ethanol, endodontic access opening into the pulp chamber was performed on the mesial occlusal surface of M1 using a round-type carbide bur (Zipperler; VDW GmbH, München, Germany) and the coronal pulp was removed. The dental pulp of the mesial root canal was mechanically removed with a #6 K-file (MANI, Utsunomiya, Japan) under a surgical microscope (Stemi 508; Zeiss, Oberkochen, Germany), and was chemically cleaned with 10% sodium hypochlorite solution (Neo Cleaner; Neo Dental Chemical Products Co., Tokyo, Japan) and 3% ethylenediaminetetraacetic acid (EDTA) solution (Smear Clean; Nishika, Shimonoseki, Japan). The #6 K-file was then approached via the mesial root canal and bleeding from the outside of the apical foramen was initiated. Hemostasis was done using a sterile article point to form a blood clot. The upper part of the blood clot (just below the root canal orifice) was covered with mineral trioxide aggregate (MTA) cement (Bio MTA cement, Morita, Tokyo, Japan), and the cavity was sealed with adhesive resin cement (Super-Bond, Sun Medical, Moriyama, Japan).
Tissue Preparation and Histological Observation
Mice were sacrificed at 1 h, 1 day, 3 days, 7 days, 14 days, 21 days, and 28 days after the operation. At each time point, perfusion fixation with 4% paraformaldehyde in 0.1 M phosphate buffer (PFA; pH 7.4) was performed transcardially, and the dissected upper jaws were immersed for 24 h in the same fixative. After decalcification with 10% EDTA for 1 week at 4°C, the tissues were trimmed at the midline of the palate with a razor and embedded into the paraffin with the midline facing the bottom. The paraffin block was cut from the palatal side and the 4-μm-thick serial sagittal sections were prepared. The sections were stained with Hematoxylin and eosin (H&E) and were observed and photographed using a universal photographic microscope (UPM Axiophoto2, Zeiss).
Immunohistochemistry (IHC)
After deparaffinization, antigen activation treatment was performed using the microwave method. Briefly, the slides were microwaved at 70°C continuously for 20 min using a microwave processor (Microwave Processor MI-77, Azumaya, Tokyo, Japan) and then were reacted at room temperature for 30 min. For the anti-nestin antibody, a 10 mM sodium citrate buffer (pH 6.0) was used, and for the anti-periostin and anti-CD31 antibodies, a Tris-EDTA buffer (pH 9.0) was used. The sections were immersed in 3% hydrogen peroxide in methanol for 30 min to inactivate endogenous peroxidase. After blocking with 3% bovine serum albumin (BSA; Sigma-Aldrich, Darmstadt, Germany) at room temperature for 1 h, they were incubated with an anti-nestin antibody (dilution 1:300; anti-nestin monoclonal antibody, clone rat-401, Merck Millipore, Darmstadt, Germany), an anti-periostin antibody (dilution 1:500; anti-periostin antibody, Abcam, Cambridge, UK), and an anti-CD31 antibody (dilution 1:500; anti-CD31 antibody, Abcam) at 4°C overnight. After washing in phosphate buffered saline (PBS) three times, the sections were incubated with horseradish peroxidase (HRP) conjugated secondary antibody (EnVision TM+ Dual Link System-HRP, Agilent) at room temperature for 1 h. After washing three times in PBS, a 3,3′-diaminobenzidine-tetrahydrochloride (DAB) solution (ImmPACT® DAB, Vector Laboratories, Burlingame, CA, USA) was used to visualize reactivity. The sections were counterstained with Mayer's hematoxylin solution for 60 s and then were observed and photographed using a photographic microscope (UPM Axiophoto2, Zeiss). As a negative control, the sections were incubated with 3% BSA instead of the primary antibodies.
Root Length and Width Measurements
We measured the root length and width of the mesial roots of the maxillary M1 in each group postoperatively. The root length was set as the distance from the mesial cement-enamel junction to the apical foramen and was measured at the longest distance. Measurement of root width was carried out according to the method of Lee et al. (30). The root width included the root dentin and cementum in the mesial and distal walls was measured at the widest site, and the average width was calculated. If the apex foramen or root canal was obliterated, the full width of the root from the mesial wall to the distal wall was measured, then half of the value was designated as the root width. As a control, the length and the width of untreated roots were measured. The time course for measuring those parameters was selected from 7 to 28 days postoperatively.
Statistical Analysis
All quantitative data in the experimental group and the untreated control group are expressed as the mean and SD of each group. Analysis software (IBM SPSS 27.0 J for Windows; SPSS Japan, Tokyo, Japan) was used for statistical processing and a p < 0.05 is considered significant. Statistical analysis of each measurement item was performed as follows: At each postoperative time, 2 groups were compared between the experimental group with the control group. After confirming the normality of the data and the uniformity of the homogeneity of variance, Student's t-test was performed on the comparison between the two homoscedastic groups.
Results
Histological Observations of the Healing Process After Regenerative Endodontics
In H&E-stained sections of the experimental group 1 h and 1 day after the operation, blood clots containing blood components such as erythrocytes and fibrin were observed in the mesial root canal (Figures 1A–D). At 3 days, a decrease of blood clots in the root canal and an increase of spindle-shaped fibroblast-like cells at the apical portion of the root canal were observed (Figures 1E,F). The tissues were continuous to the apical PDL. No blood clots were observed in the root canals at 7 days (Figures 1G,H) and the root canals were filled with fibrous connective tissue containing numerous spindle-shaped fibroblasts and dilated capillaries. At 14 days, newly formed mineralized tissue was seen in the root canal that was adjacent to the existing wall at the apical portion (Figures 1I,J). The mineralized tissue contained cells in the matrix that resembled cementocytes or osteocytes. The newly formed mineralized tissue also appeared immediately beneath the MTA that had been placed at the top of the blood clot. The area of fibrous connective tissue became narrow and decreased in the root canal. On the 21st and 28th days, bone-like or cementum-like mineralized tissues were increased in the root canal and the cells were embedded into the matrix (Figures 1K–N). The root canal wall and the apical foramen became thicker over time. The mineralized tissue obliterated the root canal and apical closure was seen. We confirmed that the root canal wall and the mineralized tissue were continuous and filled with the mineralized tissue rather than the existing dentin wall. However, no pulp- or dentin-like structures characterized by the presence of polarized odontoblast-like cells adjacent to the dentin-like tissue that had dentinal tubes were seen.
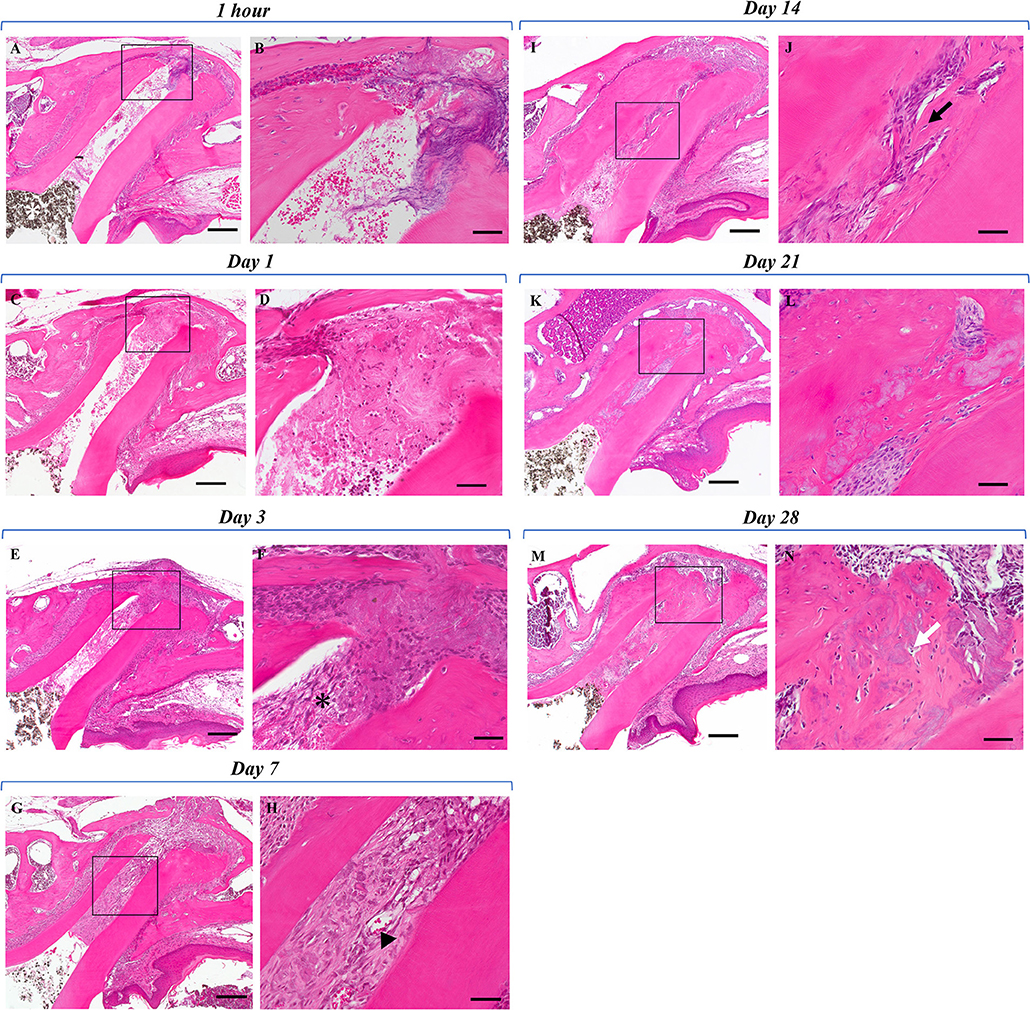
Figure 1. Histological evaluation of the postoperative healing process. (A) One hour post the regenerative endodontics procedure. The pulp tissue of the mesial root was removed and no odontoblasts were found. The root canal was filled with blood clot-like structures containing erythrocyte components. Periodontal ligament tissue around the apex showed debris of torn tissue and partial hemorrhage. Dark brown granules are MTA cement (white asterisk). (B) Higher magnification of the black box area in (A). (C) One day post the regenerative endodontics procedure. Compared with 1 h after the procedure, a decrease of blood clot-like structures was observed in the mesial root canal and around the apex. Although a decrease in erythrocytes was found around the apex, reticulated fibrin was partially observed. (D) Higher magnification of the black box area in (C). Ingrowth of fibrous tissue from the apex is seen. (E) Three days after the regenerative endodontics procedure. Further reduction of blood clot-like structures was seen in the root canal and around the apex, and the root canal was filled with loose fibrous tissues. (F) Higher magnification of the black box area in (E). Spindle-shaped fibroblast-like cells (black asterisk) were observed from the apex instead of blot clots. (G) Seven days post the regenerative endodontics procedure. No blood clot-like structures were observed in the root canal, and dense fibrous tissues with vessels were seen in the root canal. (H) Higher magnification of the black box area in (G). Dilated vessels were observed in the root canal (arrowhead). (I) Fourteen days post the regenerative endodontics procedure. Newly formed mineralized tissue was observed in the root canal. Mineralized tissue was added adjacent to the apex and the root canal wall, and was also found directly beneath the MTA cement. The area of fibrous connective tissue in the root canal was narrowing. (J) Higher magnification of the black box area in (I). The newly formed mineralized tissue in the root canal contained cellular components (black arrow). (K) Twenty-one days post the regenerative endodontics procedure. Compared with 14 days after the procedure, the mineralized tissue added to the root canal wall and the root width around the apex increased over time. (L) Higher magnification of the black box area in (K). Extensive mineralized tissue was seen in the root canal and cellular components were present in the matrix. (M) Twenty-eight days post the regenerative endodontics procedure. Bone-like or cementum-like mineralized tissue was widespread in the root canal wall and apex over time. (N) Higher magnification of the black box area in (N). The apical foramen was closed by the addition of mineralized tissue. Cells were embedded in the matrix of the mineralized tissue (white arrow) (Scale bars = 200 μm in A, C, E, G, I, K, M; scale bars = 50 μm in B, D, F, H, J, L, N).
Immunohistochemical Observations
To analyze the healing process after regenerative endodontics, specimens at 3, 7, and 14 days were immunohistochemically investigated. Immunoreactivity for nestin, an odontoblast marker, was not observed (Figures 2A,B), whereas nestin-positive cells were clearly observed along with the odontoblast layer of the pulp tissue in the control group (Supplementary Figure 1). At 3 days, marked periostin-positive cells were observed in the PDL region around the M1 root canal (Figures 2C,D). The cells of the fibrous connective tissues at the apical portion of the root canal were immunoreactive for periostin. Some spindle-shaped cells were positive for CD31, a marker of endothelial cells, at the apical portion (Figures 2E,F). At 7 and 14 days, periostin expression was strongly observed in the narrow fibrous tissues in the root canal (Figures 3C,D, 4C,D), whereas nestin-immunoreaction was not seen in the root canal (Figures 3A,B, 4A,B). Periostin expression was also seen at the edge of the newly formed mineralized tissue, and cells embedded in the cementum-like mineralized tissue. CD31 expression was observed at the center area of the root canal (Figures 3E,F, 4E,F).
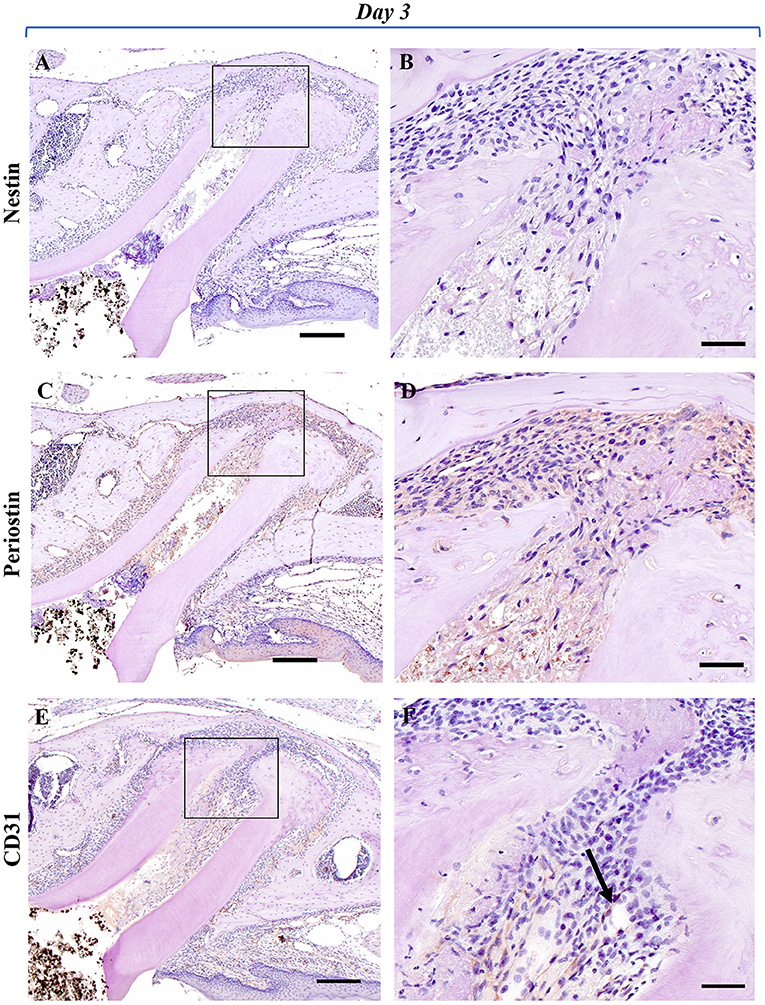
Figure 2. Representative IHC-stained images of the experimental group at 3 days. Nestin: (A,B); Periostin: (C,D); CD31: (E,F). (A) No nestin-positive cells were observed in the mesial root canal or around the apex. (B) Higher magnification of the black box area in (A). No cells showing a nestin-positive reaction were found around the fibroblast-like cells growing from the apex. (C) Localization of periostin-positive cells was markedly observed in the mesial root canal and the PDL area around the apex. (D) Higher magnification of the black box area in (C). Periostin-positive cells were observed around the spindle-shaped fibroblast-like cells growing in the root canal from the periapical tissue. (E) Positive cells showing immunoreactivity to CD31 were markedly observed in the PDL region around the mesial root, and directly under the gingival epithelium. (F) Higher magnification of the black box area in (E). Spindle-shaped vascular endothelial cells showing immunoreactivity to CD31 (black arrow) were observed near the apex of the root canal (Scale bars = 200 μm in A, C, E; scale bars = 50 μm in B, D, F).
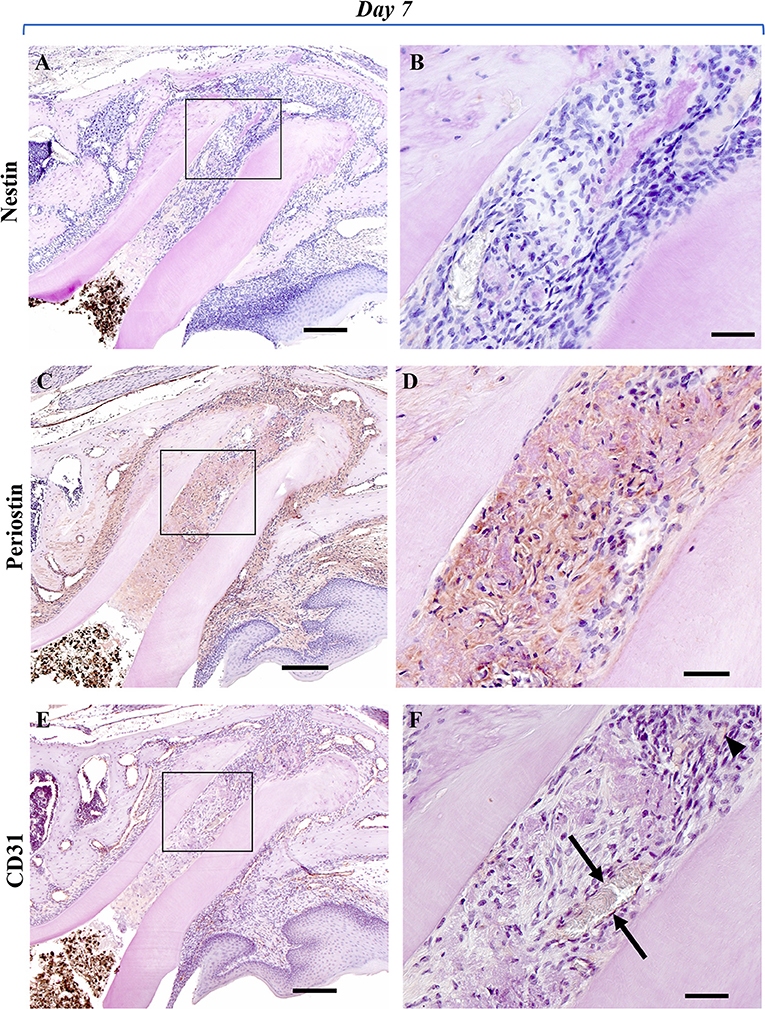
Figure 3. Representative IHC-stained images of the experimental group at 7 days. Nestin: (A,B); Periostin: (C,D); CD31: (E,F). (A) No nestin-positive cells were observed in the mesial root canal or around the apex. (B) Higher magnification of the black box area in (A). No cells showing a nestin-positive reaction were observed in the immature granulation tissue that filled the root canal. (C) Periostin-positive cells were markedly observed in the mesial root canal and in the surrounding PDL region. (D) Higher magnification of the black box area in (C). A marked periostin-positive reaction was observed in the periapical fibrotic connective tissue and in the immature granulation tissue that filled the root canal. (E) CD31-positive reaction was observed in the immature granulation tissue of the mesial root canal. (F) Higher magnification of the black box area in (E). In the center area of the root canal, spindle-shaped vascular endothelial cells (arrowhead) and new blood vessels with a luminal structure (black arrow) were observed that were positive for CD31 (Scale bars = 200 μm in A, C, E; scale bars = 50 μm in B, D, F).
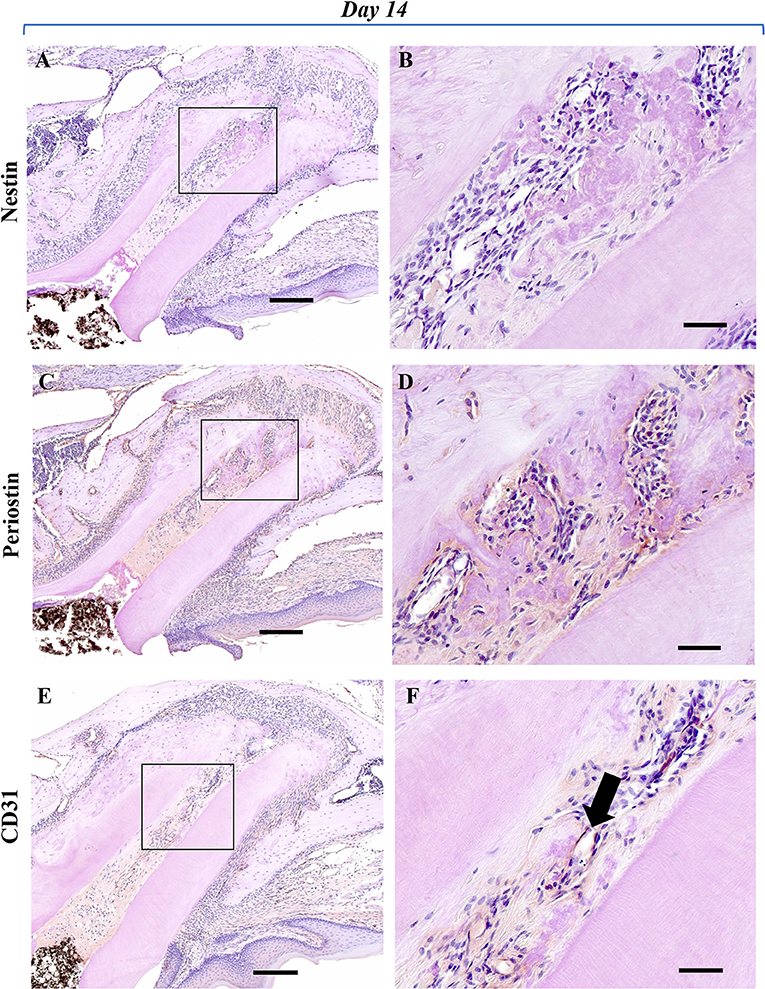
Figure 4. Representative IHC-stained images of the experimental group at 14 days. Nestin: (A,B); Periostin: (C,D); CD31: (E,F). (A) No cells showing a nestin-positive reaction were observed in the root canal and periapical tissues. (B) Higher magnification of the black box area in (A). No cells showing nestin-positive reaction were observed around the newly formed mineralized tissue in the root canal. (C) A marked periostin-positive reaction was observed in the PDL region around the mesial root and in the newly formed calcified tissue in the root canal. (D) Higher magnification of the black box area in (C). In the root canal, a marked periostin-positive reaction was observed around the newly formed mineralized tissue and in cells embedded in the matrix of the cementum-like hard tissue. (E) CD31-positive reaction was observed in the center area of the root canal. (F) Higher magnification of the black box area in (E). The presence of a new blood vessel with a lumen-like structure showing a CD31-positive reaction (black arrow) was observed at the center area of the root canal (Scale bars = 200 μm in A, C, and E; scale bars = 50 μm in B, D, and F).
Root Length
In both the control and the experimental groups, gradual root development was observed over time (Figure 5). The root length of the untreated control group became elongated from 890 to 950 μm over time. On the other hand, the root length of the experimental group became elongated from 860 to 940 μm over time. The root length in the control group was significantly higher than in the experimental group at 7 and 14 days but there was no significant difference between the two groups at 21 and 28 days.
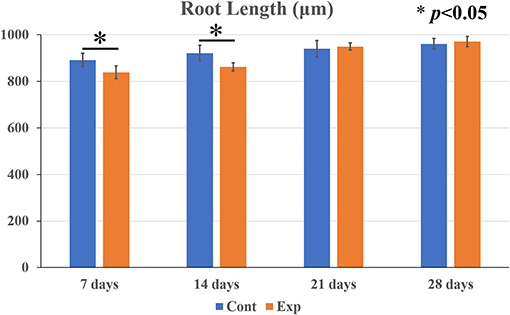
Figure 5. Comparison of postoperative root elongation between the experimental and the untreated control groups at 7, 14, 21, and 28 days. Data are expressed as means ± SD. In both the untreated control and the experimental groups with blood clots, gradual root elongation was observed over time. The root length of the control group became elongated from 890 to 950 μm over time. On the other hand, the root length of the experimental group became elongated from 860 to 940 μm over time. The root length in the control group was significantly higher than in the experimental group at 7 and 14 days. However, there was no statistically significant difference between the two groups at 21 and 28 days (n = 7 per group). *p < 0.05.
Root Width
In both the control and the experimental groups, a gradual increase in root width was observed over time (Figure 6). The root width in the untreated control group increased from 380 to 450 μm, and the root width in the experimental group increased from 410 to 530 μm. There was not a statistically significant difference in the root width between the control group and the experimental group at 7 days but the root width in the experimental group was significantly thicker than in the control group at 14, 21, and 28 days.
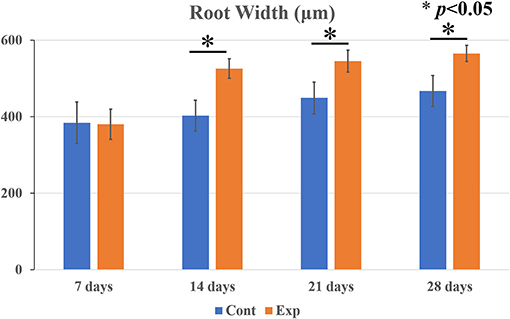
Figure 6. Comparison of postoperative root width between the experimental and the control groups at 7, 14, 21, and 28 days. Data are expressed as means ± SD. In both the untreated control and the experimental groups with blood clots, gradually thickened roots were observed over time. The root width in the control group increased from 380 to 450 μm, and that in the experimental group increased from 410 to 530 μm. There was not a statistically significant difference in the root width between the two groups at 7 days. However, the root width in the experimental group was significantly thicker than in the control group at 14, 21, and 28 days (n = 7 per group). *p < 0.05.
Discussion
Experimental Model of Regenerative Endodontics Using Mice
In this study, we established a new experimental animal model of regenerative endodontics using mice. Earlier reports described experimental models using larger animals such as dogs (22–26) and ferrets (27, 28) because there are technical difficulties involved in performing regenerative endodontics with smaller animals. However, it has been difficult to investigate the histologically detailed analysis of the healing process over time using large animal models. Furthermore, immunohistochemical analysis has been challenging, because antibodies specific to targets in larger animals are limited. Recently, a rat experimental model was reported, and histological analysis was performed, but only one time point was shown (31). Experimental models using mice have advantages to overcome the difficulties described aforementioned, and therefore, we decided to employ mice in this study. Our results showed detailed histological findings such as blood clot formation, fibrous tissue, and bone-like or cementum-like mineralized tissues over time. These histological findings are similar to those of earlier studies using larger animals such as dog models (22–26) and in human cases (18, 20, 32). This is the first report detailing histological findings during the healing process of regenerative endodontics over time as far as we know. These results should allow the identification of the origin of cells associated with the healing process using gene-altered mice in the future.
Origin of the Newly Mineralized Tissue
In this study, we observed newly mineralized tissue formation in the root canal. Earlier studies demonstrated various types of newly mineralized tissues such as bone-like or cementum-like mineralized tissue with various combinations (18, 20, 32). On the contrary, one case showed dentin-like tissue formation after regenerative endodontics (33, 34). One recent report demonstrated that the type of hard tissue formation depends on the remnant pulp (31). Our results suggest that we definitely removed the dental pulp tissue in the root canal during the procedure. Our immunohistochemical analysis also showed that no nestin-positive cells were detected in the root canal and around the mineralized tissue but the localization of periostin-positive cells was observed. These findings suggest that the newly formed mineralized tissue is not derived from odontoblasts but rather from PDLs. However, it remains unclear whether the newly formed mineralized tissue is bone-like or cementum-like hard tissue because the periostin is thought to be a marker of PDLs that can differentiate into both cementoblasts and osteoblasts (35). In our histological findings, the mineralized tissue contains cells in the matrix and would be osteoid but the tissue is continuous with the cellular cementum at the apex along the root canal wall. Furthermore, cementum does not contain vessels in the matrix, and our immunohistochemical finding also shows the newly formed mineralized tissue does not contain CD31 positive blood vessels in the matrix. Therefore, our results suggest that the mineralized tissue is cementum-like hard tissue.
Vascular Growth and Angiogenesis
Angiogenesis is necessary for tissue regeneration or repair to supply oxygen and eliminate waste products. Although regenerative endodontics has been called pulp revascularization, angiogenesis during the procedure has not well been investigated histologically. We assessed the localization of CD31-positive cells to detect endothelial cells and confirmed that CD31-positive vascular cells had grown in from the apex. Furthermore, CD31-positive cells with a vascular luminal structure were observed in the root canal and around the mineralized tissue over time. The histological replacement of blot clots with fibrous tissues with vessels is well described in the earlier reports but was not characterized. The results of this study show histological evidence of that replacement process during the wound healing after the procedure.
Blood clot formation in the root canal plays an important role because blood clots serve as a scaffold and contain various growth factors such as vascular endothelial growth factor (VEGF), platelet-derived growth factor (PDGF), and fibroblast growth factor (FGF), which signal endothelial precursor cells and perivascular progenitor cells (36) and promote angiogenesis. Furthermore, mesenchymal stem cells reside in the microenvironment (niche) that is located at perivascular sites, and the maintenance and regulation of stem cell populations are controlled at the sites of regeneration or repair of injured tissues (37–39). This study identified new vessels that had grown in from the apical tissue and were observed adjacent to newly mineralized tissue. These findings imply that the ingrowth of vessels contributes not only to angiogenesis but also to the formation of newly mineralized tissue during the wound healing process. Further study is needed to further clarify the mechanism involved.
Root Development
Apexification, which has conventionally been performed on immature teeth diagnosed with pulp necrosis and/or apical periodontitis, has various disadvantages such as a short root length and a thin root canal wall. A short root length makes a higher crown root ratio, and a thin root canal wall becomes fragile and prone to root fracture (4, 40). Regenerative endodontic procedures (REPs) are performed to overcome those two problems. An earlier in vivo study that used beagle dog showed that fracture resistance was significantly more than two times as high in the REP group compared to the control group (41). However, few studies have been reported that investigated root length and root width after the procedures (41, 42). Our results show that the root length in the control group was significantly higher than in the experimental group at 7 and 14 days after the procedure, but there was not a significant difference between those two groups at 21 or 28 days. Cementum-like mineralized tissue formation around the apex was histologically seen at the same time points. Regarding the root width, there was no significant difference between the experimental group and the control group 7 days after the procedure, whereas the root width of the experimental group was significantly higher than that of the control group since the 14th day. Narrowing or obliteration due to cementum-like mineralized tissue formation in the root canal was histologically observed at the same time points. These results suggest that the root length is complemented by the addition of newly mineralized tissue around the root apex and that the increase in root width is attributed to mineralized tissue formation in the root canal, resembling similar findings in human cases (18, 20, 32) and larger animal experiments (22–26). Increased root width ensures that the root is held firmly by the PDL and alveolar fossa. Therefore, we think that the new structure with an increase in root width results in resistance to external stress, which leads to the prevention of root fracture. However, we employed mice in this study, and the tooth size, shape, and occlusal force of the mice were different from those of humans and dogs. The point is a limitation of the fracture resistance.
Conclusion
In conclusion, we have established a new animal model of regenerative endodontics using mice. We demonstrated histological and immunohistochemical findings after the procedure in detail. The results of this study suggest that blood clots are replaced by PDL cells (periostin-positive cells) and that vessels around the apex have grown into the root canal. Furthermore, the fibrous tissue derived from PDL cells formed newly cementum-like mineralized tissue in the root canal and the apex over time. These results serve as a basis for understanding the healing process after the regenerative endodontics procedure in detail and should contribute to further investigations of the procedure in the future.
Data Availability Statement
The raw data supporting the conclusions of this article will be made available by the authors, without undue reservation.
Ethics Statement
The animal study was reviewed and approved by Animal Experiment Committee at Tokyo Dental College (approval number: 302101).
Author Contributions
TK, KM, MS, and TM: conceptualization. TK, TI, KM, H-SJ, MS, and TM: methodology. TK, TI, KM, and TM: investigation. TK, KM, H-SJ, and TM: data analysis. TK and KM: drafting original manuscript. TM: editing manuscript. All authors have read and agreed with submitting the article.
Funding
This research was supported, in part, by a Private University Research Branding Project from MEXT of Japan (Multidisciplinary Research Center for Jaw Disease (MRCJD); Archiving Longevity and Sustainability by Comprehensive Reconstruction of Oral and Maxillofacial Functions).
Conflict of Interest
The authors declare that the research was conducted in the absence of any commercial or financial relationships that could be construed as a potential conflict of interest.
Publisher's Note
All claims expressed in this article are solely those of the authors and do not necessarily represent those of their affiliated organizations, or those of the publisher, the editors and the reviewers. Any product that may be evaluated in this article, or claim that may be made by its manufacturer, is not guaranteed or endorsed by the publisher.
Acknowledgments
We thank Dr. Akira Yamaguchi (Visiting Professor, Tokyo Dental College), Dr. Toshihide Mizoguchi (Associate Professor, Tokyo Dental College), and Dr. Shin-ichi Iwaya (Iwaya Dental Clinic) for their helpful discussion.
Supplementary Material
The Supplementary Material for this article can be found online at: https://www.frontiersin.org/articles/10.3389/fdmed.2022.864406/full#supplementary-material
References
1. Trope M. Treatment of the immature tooth with a non-vital pulp and apical periodontitis. Dent Clin North Am. (2010) 54:313–24. doi: 10.1016/j.cden.2009.12.006
2. Rafter M. Apexification: a review. Dent Traumatol. (2005) 21:1–8. doi: 10.1111/j.1600-9657.2004.00284.x
3. Frank AL. Therapy for the divergent pulpless tooth by continued apical formation. J Am Dent Assoc. (1966) 72:87–93. doi: 10.14219/jada.archive.1966.0017
4. Andreasen JO, Farik B, Munksgaard EC. Long-term calcium hydroxide as a root canal dressing may increase risk of root fracture. Dent Traumatol. (2002) 18:134–7. doi: 10.1034/j.1600-9657.2002.00097.x
5. Tsilingaridis G, Malmgren B, Andreasen JO, Malmgren O. Intrusive luxation of 60 permanent incisors: a retrospective study of treatment and outcome. Dent Traumatol. (2012) 28:416–22. doi: 10.1111/j.1600-9657.2011.01088.x
6. Cvek M. Prognosis of luxated non-vital maxillary incisors treated with calcium hydroxide and filled with gutta-percha. A retrospective clinical study. Endod Dent Traumatol. (1992) 8:45–55. doi: 10.1111/j.1600-9657.1992.tb00228.x
7. Jeeruphan T, Jantarat J, Yanpiset K, Suwannapan L, Khewsawai P, Hargreaves KM. Mahidol study 1: comparison of radiographic and survival outcomes of immature teeth treated with either regenerative endodontic or apexification methods: a retrospective study. J Endod. (2012) 38:1330–6. doi: 10.1016/j.joen.2012.06.028
8. Iwaya S, Ikawa M, Kubota M. Revascularization of an immature permanent tooth with apical periodontitis and sinus tract. Dent Traumatol. (2001) 17:185–7. doi: 10.1034/j.1600-9657.2001.017004185.x
9. Banchs F, Trope M. Revascularization of immature permanent teeth with apical periodontitis: new treatment protocol? J Endod. (2004) 30:196–200. doi: 10.1097/00004770-200404000-00003
10. Iwaya S, Ikawa M, Kubota M. Revascularization of an immature permanent tooth with periradicular abscess after luxation. Dent Traumatol. (2011) 27:55–8. doi: 10.1111/j.1600-9657.2010.00963.x
11. Shah N, Logani A, Bhaskar U, Aggarwal V. Efficacy of revascularization to induce apexification/apexogensis in infected, nonvital, immature teeth: a pilot clinical study. J Endod. (2008) 34:919–25. doi: 10.1016/j.joen.2008.05.001
12. Nosrat A, Seifi A, Asgary S. Regenerative endodontic treatment (revascularization) for necrotic immature permanent molars: a review and report of two cases with a new biomaterial. J Endod. (2011) 37:562–7. doi: 10.1016/j.joen.2011.01.011
13. Ding RY, Cheung GS, Chen J, Yin XZ, Wang QQ, Zhang CF. Pulp revascularization of immature teeth with apical periodontitis: a clinical study. J Endod. (2009) 35:745–9. doi: 10.1016/j.joen.2009.02.009
14. Xie Z, Shen Z, Zhan P, Yang J, Huang O, Huang S, et al. Functional dental pulp regeneration: Basic research and clinical translation. Int J Mol Sci. (2021) 22:8991. doi: 10.3390/ijms22168991
15. Murray PE, Garcia-Godoy F, Hargreaves KM. Regenerative endodontics: a review of current status and a call for action. J Endod. (2007) 33:377–90. doi: 10.1016/j.joen.2006.09.013
16. Diogenes A, Ruparel NB. Regenerative endodontic procedures: Clinical outcomes. Dent Clin North Am. (2017) 61:111–25. doi: 10.1016/j.cden.2016.08.004
17. ŽiŽka R, Belák Š, Šedý J, Fačevicová K, Voborná I, Marinčák D. Clinical and radiographic outcomes of immature teeth treated with different treatment protocols of regenerative endodontic procedures: a retrospective cohort study. J Clin Med. (2021) 10:1600. doi: 10.3390/jcm10081600
18. Martin G, Ricucci D, Gibbs JL, Lin LM. Histological findings of revascularized/revitalized immature permanent molar with apical periodontitis using platelet-rich plasma. J Endod. (2013) 39:138–44. doi: 10.1016/j.joen.2012.09.015
19. Shimizu E, Ricucci D, Albert J, Alobaid AS, Gibbs JL, Huang GTJ, et al. Clinical, radiographic, and histological observation of a human immature permanent tooth with chronic apical abscess after revitalization treatment. J Endod. (2013) 39:1078–83. doi: 10.1016/j.joen.2013.04.032
20. Lei L, Chen Y, Zhou R, Huang X, Cai Z. Histologic and immunohistochemical findings of a human immature permanent tooth with apical periodontitis after regenerative endodontic treatment. J Endod. (2015) 41:1172–9. doi: 10.1016/j.joen.2015.03.012
21. Nosrat A, Kolahdouzan A, Hosseini F, Mehrizi EA, Verma P, Torabinejad M. Histologic outcomes of uninfected human immature teeth treated with regenerative endodontics: 2 case reports. J Endod. (2015) 41:1725–9. doi: 10.1016/j.joen.2015.05.004
22. Thibodeau B, Teixeira F, Yamauchi M, Caplan DJ, Trope M. Pulp revascularization of immature dog teeth with apical periodontitis. J Endod. (2007) 33:680–9. doi: 10.1016/j.joen.2007.03.001
23. Wang X, Thibodeau B, Trope M, Lin LM, Huang GT. Histologic characterization of regenerated tissues in canal space after the revitalization/revascularization procedure of immature dog teeth with apical periodontitis. J Endod. (2010) 36:56–63. doi: 10.1016/j.joen.2009.09.039
24. Yamauchi N, Yamauchi S, Nagaoka H, Duggan D, Zhong S, Lee SM, et al. Tissue engineering strategies for immature teeth with apical periodontitis. J Endod. (2011) 37:390–7. doi: 10.1016/j.joen.2010.11.010
25. Gomes-Filho JE, Duarte PCT, Ervolino E, Bomfim SRM, Abimussi CJX, Santos LM, et al. Histologic characterization of engineered tissues in the canal space of closed-apex teeth with apical periodontitis. J Endod. (2013) 39:1549–56. doi: 10.1016/j.joen.2013.08.023
26. Londero CDLDC, Pagliarin CML, Felippe MCS, Felippe WT, Danesi CC, Barletta FB. Histologic analysis of the influence of a gelatin-based scaffold in the repair of immature dog teeth subjected to regenerative endodontic treatment. J Endod. (2015) 41:1619–25. doi: 10.1016/j.joen.2015.01.033
27. Torabinejad M, Milan M, Shabahang S, Wright KR, Faras H. Histologic examination of teeth with necrotic pulps and periapical lesions treated with 2 scaffolds: an animal investigation. J Endod. (2015) 41:846–52. doi: 10.1016/j.joen.2015.01.026
28. Verma P, Norsrat A, Kim JR, Price JB, Wang P, Bair E, et al. Effect of residual bacteria on the outcome of pulp regeneration in vivo. J Dent Res. (2017) 96:100–6. doi: 10.1177/0022034516671499
29. Xu W, Jiang S, Chen Q, Yanyan Y, Chen J, Heng BC, et al. Systemically transplanted bone marrow-derived cells contribute to dental pulp regeneration in a chimeric mouse model. J Endod. (2016) 42:263–8. doi: 10.1016/j.joen.2015.10.007
30. Lee DJ, Lee SJ, Lee MJ, Kim EJ, Ohshima H, Jung HS. The role of angiogenesis and pulpal healing in tooth replantation and allograft transplantation. Biochem Biophys Rep. (2021) 25:100945. doi: 10.1016/j.bbrep.2021.100945
31. Edanami N, Yoshiba K, Shirakashi M, Belal RSI, Yoshiba N, Ohkura N, et al. Impact of remnant healthy pulp and apical tissue on outcomes after simulated regenerative endodontic procedure in rat molars. Sci Rep. (2020) 10:20967. doi: 10.1038/s41598-020-78022-w
32. Becerra P, Ricucci D, Loghin S, Gibbs JL, Lin LM. Histologic study of a human immature permanent premolar with chronic apical abscess after revascularization/revitalization. J Endod. (2014) 40:133–9. doi: 10.1016/j.joen.2013.07.017
33. Peng C, Zhao Y, Wang W, Yang Y, Qin M, Ge L. Histologic findings of a human immature revascularized/regenerated tooth with symptomatic irreversible pulpitis. J Endod. (2017) 43:905–9. doi: 10.1016/j.joen.2017.01.031
34. Austah O, Joon R, Fath WM, Chrepa V, Diogenes A, Ezeldeen M, et al. Comprehensive characterization of 2 immature teeth treated with regenerative endodontic procedures. J Endod. (2018) 44:1802–11. doi: 10.1016/j.joen.2018.09.007
35. Romanos GE, Asnani KP, Hingorani D, Deshmukh VL. PERIOSTIN: role in formation and maintenance of dental tissues. J Cell Physiol. (2014) 229:1–5. doi: 10.1002/jcp.24407
36. Gunal E, Bezgin T, Ocak M, Bilecenoglu B. Effects of various thicknesses and levels of mineral trioxide aggregate coronal plugs on nanoleakage and fracture resistance in revascularization: an in vitro study. Aust Endod J. (2021) 47:608–15. doi: 10.1111/aej.12531
37. Lovelace TW, Henry MA, Hargreaves KM, Diogenes A. Evaluation of the delivery of mesenchymal stem cells into the root canal space of necrotic immature teeth after clinical regenerative endodontic procedure. J Endod. (2011) 37:133–8. doi: 10.1016/j.joen.2010.10.009
38. Shi S, Gronthos S. Perivascular niche of postnatal mesenchymal stem cells in human bone marrow and dental pulp. J Bone Miner Res. (2003) 18:696–704. doi: 10.1359/jbmr.2003.18.4.696
39. Mitsiadis TA, Feki A, Papaccio G, Catón J. Dental pulp stem cells, niches, and notch signaling in tooth injury. Adv Dent Res. (2011) 23:275–9. doi: 10.1177/0022034511405386
40. Zacher A, Marretta SM. Decision-making and management of immature permanent teeth with crown fractures in small animals- a review. J Vet Dent. (2021) 38:81–92. doi: 10.1177/08987564211046325
41. Zhou R, Wang Y, Chen Y, Chen S, Lyu H, Cai Z. Radiographic, Histologic, and Biomechanical Evaluation of Combined Application of Platelet-rich Fibrin with Blood Clot in Regenerative Endodontics. J Endod. (2017) 43:2034–40. doi: 10.1016/j.joen.2017.07.021
Keywords: regenerative endodontic procedures, pulp revascularization, cementum, cell-homing, mouse in situ model, periodontal ligament, periostin, angiogenesis
Citation: Komada T, Mitomo K, Ikarashi T, Shimono M, Jung H-S and Muramatsu T (2022) Periodontal Ligament Cells Are Involved in the Formation of Intracanal Cementum-Like Tissue After Regenerative Endodontic Procedures: A Mouse in situ Model. Front. Dent. Med. 3:864406. doi: 10.3389/fdmed.2022.864406
Received: 28 January 2022; Accepted: 06 April 2022;
Published: 11 May 2022.
Edited by:
Akira Nifuji, Tsurumi Univesity, JapanReviewed by:
Shahrokh Shabahang, Aditx Therapeutics, Inc., United StatesMilad Altaii, University of Adelaide, Australia
Copyright © 2022 Komada, Mitomo, Ikarashi, Shimono, Jung and Muramatsu. This is an open-access article distributed under the terms of the Creative Commons Attribution License (CC BY). The use, distribution or reproduction in other forums is permitted, provided the original author(s) and the copyright owner(s) are credited and that the original publication in this journal is cited, in accordance with accepted academic practice. No use, distribution or reproduction is permitted which does not comply with these terms.
*Correspondence: Takashi Muramatsu, tmuramat@tdc.ac.jp