Performance of Seedlings of a Shade-Tolerant Tropical Tree Species after Moderate Addition of N and P
- Plant Ecology and Ecosystems Research, University of Göttingen, Göttingen, Germany
Nitrogen deposition to tropical forests is predicted to increase in future in many regions due to agricultural intensification. We conducted a seedling transplantation experiment in a tropical premontane forest in Ecuador with a locally abundant late-successional tree species (Pouteria torta, Sapotaceae) aimed at detecting species-specific responses to moderate N and P addition and to understand how increasing nutrient availability will affect regeneration. From locally collected seeds, 320 seedlings were produced and transplanted to the plots of the Ecuadorian Nutrient Manipulation Experiment (NUMEX) with three treatments (moderate N addition: 50 kg N ha−1 year−1, moderate P addition: 10 kg P ha−1 year−1 and combined N and P addition) and a control (80 plants per treatment). After 12 months, mortality, relative growth rate, leaf nutrient content and leaf herbivory rate were measured. N and NP addition significantly increased the mortality rate (70 vs. 54% in the control). However, N and P addition also increased the diameter growth rate of the surviving seedlings. N and P addition did not alter foliar nutrient concentrations and leaf N:P ratio, but N addition decreased the leaf C:N ratio and increased SLA. P addition (but not N addition) resulted in higher leaf area loss to herbivore consumption and also shifted carbon allocation to root growth. This fertilization experiment with a common rainforest tree species conducted in old-growth forest shows that already moderate doses of added N and P are affecting seedling performance which most likely will have consequences for the competitive strength in the understory and the recruitment success of P. torta. Simultaneous increases in growth, herbivory and mortality rates make it difficult to assess the species' overall performance and predict how a future increase in nutrient deposition will alter the abundance of this species in the Andean tropical montane forests.
Introduction
Phosphorus (P) and nitrogen (N) are supposed to limit stand productivity in the majority of terrestrial ecosystems as well as in tropical forests (Tanner et al., 1998; Elser et al., 2007; Vitousek et al., 2010; Harpole et al., 2011; Homeier et al., 2012). Anthropogenic influences have modified natural nutrient availability worldwide by pollutants released to the atmosphere and the resulting increased deposition to soils. It is expected that increasing nutrient deposition has the potential to alter the dynamics of tropical forest ecosystems causing structural and compositional changes of the plant communities in response to modified availability of limiting elements (e.g., Lewis and Tanner, 2000; Homeier et al., 2012).
Nutrient effects on tropical forests largely depend on the local conditions; factors such as temperature, precipitation, soil type and pedogenesis, and local biota determine overall nutrient availability at a specific site and if an element is limiting plant growth. Thus, for example tropical lowland rainforests growing on old, highly weathered soils under humid conditions at high temperatures are most likely to be P limited, whereas tropical montane forests that are typically exposed to a cooler and wetter climate most often grow on younger or rejuvenated soils where N is likely to limit plant growth (Tanner et al., 1998; Unger et al., 2010; Vitousek et al., 2010; Wolf et al., 2011; Fisher et al., 2013). In general, terrestrial ecosystems in many cases seem to be limited by both N and P. Hence, addition of either N or P or of both elements is stimulating plant growth (Elser et al., 2007; Harpole et al., 2011).
Since compositional shifts in communities of long-lived organisms like trees need decades to become visible, studies on mature trees will rarely provide information about future changes in forest dynamics caused by increased nutrient availability. In fact, seedlings of tropical rainforest trees have been found to respond more sensitively to changes in nutrient availability (e.g., Lawrence, 2003; Yavitt and Wright, 2008; Dent and Burslem, 2009; Andersen et al., 2010; Lu et al., 2010; Holste et al., 2011) due to the strong physiological dependence of this stage on resource availability in a highly competitive environment (Kitajima, 1996).
It is known that processes affecting the seedling stage act as a strong selective filter controlling patterns of recruitment and are thereby influencing the future species composition of the forest (Swaine, 1996; Whitmore, 1996; Metz et al., 2008). Therefore, it is crucial to understand the impact of changed nutrient availability at this stage of the life cycle. Several studies have used seedlings of locally abundant tropical tree species to study if these species are able to optimize their resource use after nutrient addition (e.g., Lawrence, 2001; Yavitt and Wright, 2008; Andersen et al., 2010; Pasquini and Santiago, 2012; Alvarez-Clare et al., 2013) and concluded that changes in establishment and growth rates might be considered as a key driver of structural changes of tree communities to altered nutrient availability.
Responses to changed nutrient availability likely will depend on the species' life strategy. Some species are expected to rapidly invest extra nutrients to increase growth especially under favorable light conditions, whereas others rather may store nutrients in their tissues (e.g., Huante et al., 1995b; Raaimakers and Lambers, 1996; Lawrence, 2003). Biomass allocation varies, depending on how seedlings accumulate carbohydrates to aboveground tissues vs. root surface (Burslem et al., 1995; Wan Juliana et al., 2009). Carbon gain and plant growth are highly dependent on leaf traits, in particular photosynthetic capacity and leaf area, which both tend to increase with nutrient addition (Burslem et al., 1995; Wan Juliana et al., 2009; Pasquini and Santiago, 2012).
Large increases in nutrient availability, however, may also lead to a decrease in density and diversity of the seedling community by altering survival and establishment rates of a share of species because mortality could be altered by increased herbivory, e.g., as a consequence of N or P accumulation in leaf tissues (Andersen et al., 2010; Santiago et al., 2012). In addition, seedling establishment might be impeded due to soil acidification (Lu et al., 2010) or due to modified competition with other species (Lawrence, 2001).
To study the effects of increased nutrient availability on a locally abundant climax species, we took advantage of the ongoing nutrient manipulation experiment NUMEX in Ecuador. NUMEX was started in 2008 to study if and to what extent N and P limitation are controlling tropical montane forest functioning at three elevation levels (1000, 2000, and 3000 m) and to simulate the effects of future increasing nutrient availability from atmospheric deposition on different ecosystem processes. In southern Ecuador, nutrient deposition has been suggested to largely origin from extensive biomass burning occurring in the Amazon basin leading to increasing depositions of >10 kg N ha−1 in the last decade (Fabian et al., 2005; Wilcke et al., 2013). The continued nutrient addition in this experiment had already shown effects on various belowground to aboveground compartments in the experiment's first years: Homeier et al. (2012) found in the common tree species a positive growth response after N (two out of four species), P (two out of four species) and N+P addition (three out of four species) and an increase of leaf litter production after N and NP addition suggesting that at 2000 m. a.s.l. N and P might be co-limiting. At the same site, Camenzind et al. (2014) detected changes in the abundance and species richness of arbuscular mycorrhizal fungi (AMF) after N and P addition. At 1000 m. a.s.l., four years of N and N+P addition (but not P addition alone) resulted in increased mineral N production and decreased microbial N retention and microbial biomass C and C:N ratio (Baldos et al., 2015). N2O emissions were only increased during the first two years of N and NP addition (but not P addition alone), and P addition tended to reduce soil N cycling and to decrease N2O fluxes (Martinson et al., 2013; Müller et al., 2015). Morevover, P (but not N) additions increased the amount of water stable soil macroaggregates, which play an important role for soil stability and nutrient cycling (Camenzind et al., submitted). Krashevska et al. (2014) found that the decomposer system (microorganisms and saprophytic fungi) of the studied NUMEX plots responded to the changes in nutrient inputs concluding that at all elevations microorganisms were generally limited by N and saprophytic fungi also by P. In addition, P and N+P treatments increased the bioavailable inorganic P concentrations and decreased phosphatase activity (Dietrich et al., submitted).
At the premontane study site at 1000 m. a.s.l. we found an average foliar N:P ratio of 28.0 in 80 unfertilized trees from 25 different plant families sampled at mid slope position (Homeier et al., unpublished data). We assume that P is the prevailing limiting nutrient at this site, since according to Townsend et al. (2007) N:P ratios >16 suggest P-limitation. The two other NUMEX sites show also average foliar N:P ratios >16 (2000 m. a.s.l.: N:P ratio = 22.1, 78 trees from 31 plant families; 3000 m. a.s.l.: 26.0, 65, 16; Homeier et al., unpublished data). Thus, the foliar N:P ratio does not indicate a shift to N limitation toward higher elevations.
To test the response of seedlings of the locally most abundant climax tree species Pouteria torta to continued nutrient addition, we transplanted 9-month-old seedlings to the NUMEX experimental plots at 1000 m. a.s.l. in 2011. Our investigation aimed to test how continued nutrient addition (a) will alter mortality and growth rates in seedlings, (b) whether leaf morphology, folivory, and foliar nutrient concentrations will indicate changed nutrient use (e.g., higher area loss and high foliar N concentration after N addition) and (c) how biomass partitioning to above and belowground organs will change as a response to extra nutrient availability.
We aimed to understand how N and P limitation are influencing seedling growth and vitality in this abundant species and how its dominant role in the forest might change under increasing nutrient deposition. Based on earlier findings on the nutrient response of shade-tolerant tropical tree seedlings, we hypothesized that Pouteria torta seedlings after being released from potential nutrient limitation would respond primarily by accumulating N and P in the biomass rather than by increasing its growth rate.
Material and Methods
Study Site
The study was conducted in Podocarpus National Park, southern Ecuador (province Zamora-Chinchipe), close to the Bombuscaro entrance (S 4° 7′, W 78° 58′) in the transition zone between tropical lowlands and lower montane forests. Vegetation has been described as evergreen premontane forest with tree heights up to 40 m (Homeier et al., 2008), but average stand height is between 20 and 25 m. Most abundant tree families are Fabaceae, Melastomataceae, Moraceae, Myristicaceae, Rubiaceae, and Sapotaceae; common tree species are Pouteria torta (Mart.) Radlk. (Sapotaceae) and Clarisia racemosa Ruiz & Pav. (Moraceae; Homeier et al., 2008, 2013).
Mean annual temperature at the study site is around 20°C and annual mean precipitation ~2200 mm, without a clear seasonal pattern (Emck, 2007; Peters, unpublished data). Soils are Dystric Cambisols developed from deeply-weathered granodioritic rock of the Jurassic Zamora granitoide formation (Wolf et al., 2011). The mineral soil is covered by a thin layer of decomposing leaves (Ol layer) (Homeier et al., 2013). Soil characteristics (means ± SE) of the mineral topsoil (0–5 cm) after 4 years of nutrient addition (April 2012) are summarized in Supplementary Table 1.
Study Species
The locally most abundant tree species of the old-growth forests at Bombuscaro, Pouteria torta subsp. glabra T. P. Penn., is a late successional shade-tolerant tree species (Wittich et al., 2015; Homeier, own observations), accounting for 9.5% of the stems ≥ 10 cm dbh or about 69 stems ha−1 in the NUMEX plots. It reaches heights up to 30 m and about 50 cm stem diameter and its wood specific gravity is high with 0.79–0.87 g cm−3 (Homeier, unpublished data). The species is widely distributed in the Neotropics from Colombia to the Guianas and in western South America to Bolivia. It is known to occur from the lowlands up to 1500 m. a.s.l. (Pennington, 2007).
Experimental Design
The full-factorial Ecuadorian nutrient manipulation experiment (NUMEX) was set up at 990–1100 m.a.s.l. in a stratified random design which consists of four blocks with four treatments (control, N, P, and N+P addition) assigned to every block (16 experimental plots). Experimental plots (20 × 20 m) were installed in old-growth forest with closed canopy at mid slope position; the plots are separated from each other by at least 10 m-wide strips of untreated forest (Supplementary Figure 1).
Fertilizations started in February 2008. Fertilizers are applied by hand to the experimental plots at rates of 50 kg N ha−1 (as urea) in the N treatment plots, or 10 kg P ha−1 (NaH2PO4) in the P treatment plots, or 50 kg N and 10 kg P (NP treatment plots) applied in two portions per year (Homeier et al., 2013). For the seedling experiment, four new subplots of 1 m2 were randomly placed into each NUMEX experimental plot (64 subplots in total). Irradiance at the forest floor was quantified in every subplot with hemispherical photos (Nikon D5000 camera with 8 mm-fish eye lens) taken at the midpoint 1 m above ground under uniform sky conditions in 2012. Images were analyzed using Gap Light Analyzer 2.0 software (Frazer et al., 1999). Light availability in the subplots indicated a mean canopy openness of 8.85 ± 4.1(SD) % with a range of 1.25–19.48%.
Seedlings of Pouteria torta were raised after a massive fruiting event that occurred in 2010. More than 400 seeds from about 15 trees were collected in September 2010 at the study site and sown in a greenhouse supplied by a local farmer. In June 2011, 320 seedlings were selected and transported to the study site. All seedlings were measured for total height and labeled before planting; mean initial height was 17.4 ± 4.9 (SE) cm (Table 1). At that time, there were no cotyledons left on the plants and seedlings had completely consumed the reserves stored in the seeds.
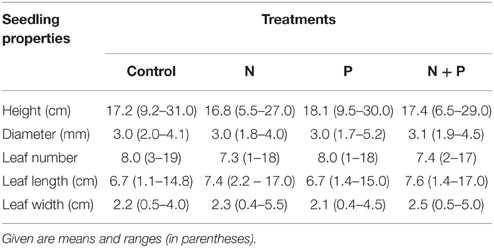
Table 1. Properties of the initial seedling cohort before transplantation to the NUMEX plots in June 2011.
Five seedlings were assigned to each experimental subplot. Because of small differences in initial height, we classified seedlings into height categories before planting and then equally distributed them to the 64 subplots to ensure a similar seedling height distribution in each plot. Subplots were also cleaned from other tree seedlings and ground herbs to avoid root competition. Once seedlings were planted, initial size characteristics were measured (stem height and basal diameter, leaf number, length, and width of the largest leaf).
After 1 year, in May 2012, all surviving seedlings were carefully harvested after a final assessment of basal diameter and leaf area loss. We excavated all seedlings, removed all soil from the roots, and then separated the plants into root, stem and leaf fractions. Fresh leaves were scanned immediately after harvesting seedlings (color, 150 dpi) with a Cannon LIDE 1000 flatbed scanner. All seedling fractions were dried for 72 h at 60°C and then transferred to Germany.
Leaf Analyses
The scanned images of the Pouteria leaves were analyzed using Win Folia 2005b software (Régent Intruments Inc., Quebec City, QC, Canada) to calculate total leaf area including damaged areas (hole area). Subsequently, images were manually adjusted by filling in missing leaf sections, to estimate the original leaf area prior to damage. Specific leaf area (SLA, cm2 g−1) was calculated for each seedling by dividing the entire remaining leaf area of all leaves by its mass. Proportional leaf area loss was determined as an estimate of herbivory rate, the damage inflicted over the life span of the leaves with the following formula:
Leaf length ratio and leaf width ratio were calculated by dividing the respective dimension of the biggest leaf at harvest and before planting to estimate changes in leaf dimensions during our experiment:
The leaf area ratio (LAR) was calculated as the amount of leaf area per unit of total plant mass.
Nutrient Analyses
We determined total N and C concentrations of the leaf mass with a C/N elemental analyzer (Vario EL III, Elementa, Hanau, Germany). Total P concentrations were analyzed using an Inductively Coupled Plasma Analyzer (Optima 5300DV ICP-OES, Perkin Elmer, Waltham, Massachusetts, USA) after digesting the leaf samples with concentrated HNO3. Nutrient analyses were conducted at the laboratory of the Department of Ecology and Ecosystems Research, University of Göttingen, Germany for all individual seedlings harvested at the end of the experiment.
Data Treatment and Statistical Analyses
Mortality rates were calculated from the proportion of dead individuals in the subplots (i.e., number of dead seedlings divided by seedlings planted). Effects of nutrients on mortality were tested with a generalized linear mixed model (GLMM) for a binomial distribution using counts of the dead individuals in a full factorial approach using the function “glmer” in the lme4 package (Bates et al., 2014) with N and P as fixed effects and “block” as a random factor. Significance values were obtained after testing the model with the “cftest” function from the multcomp package (Hothorn et al., 2008). This function calculates, in a univariate testing way, the p-values from estimated model coefficients obtained from a z-test. The relative stem diameter growth rate (RGRD) was calculated from diameter measurements for the study interval as:
where d2 represents the final diameter and d1 the initial diameter, and t1-t0 represents the time span between planting and harvesting dates.
The mass fractions for leaves (LMF), stems (SMF) and roots (RMF) were calculated by relating organ mass to total plant mass for each seedling following Poorter et al. (2012).
Data were transformed to reduce heteroscedasticity when required (log transformations for biomass ratios, leaf area and leaf nutrient contents and square root transformations for biomass, leaf fractions, and leaf area loss).
To test specifically how nutrients are influencing seedling performance, we used a full factorial linear mixed model (Zuur et al., 2009) with N and P as fixed effects using the “lme” function of the nlme package (Pinheiro et al., 2014) for all seedlings measured and harvested in 2012 (n = 124). For all parameters assessed (RGRD, SLA, leaf area loss, foliar N/P, and C/N ratio, root/shoot and root/leaf ratios, LAR, biomass fractions, foliar nutrient contents), canopy openness was evaluated first in order to check for interaction with fixed effects (treatment). Since it had no influence on the responses to nutrient addition, canopy openness was not included in the final models. In all models, we included “block” as a random factor.
All comparisons contrasted differences between treatments (N, P or interaction of N and P) with control. All analyses and figures were done with R 3.1.1 software (R Development Core Team, 2014). Specific information about the structure of the fitted models all parameters analyzed is summarized in Supplementary Table 2.
Results
Seedling Mortality and Growth
The seedlings suffered high mortality (Figure 1); only 124 (con = 37, N = 24, P = 39, NP = 24) of the initially planted 320 Pouteria torta seedlings survived the first year. On average, 54% (n = 43) of all seedlings in the control and 51% (n = 41) of those in the P treatment died. N fertilization increased seedling mortality significantly (p = 0.016) to 70% (n = 56) in both N treatments compared with the control (Table 2).
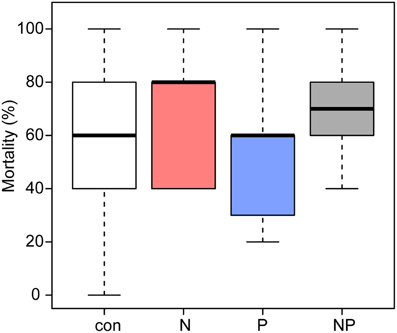
Figure 1. Mean percentages of mortality in Pouteria torta seedlings observed during 1 year of exposure to moderate nutrient additions (2011–2012). Addition of N and of N + P resulted in a significantly higher mortality of the seedlings (p = 0.016).
The relative diameter growth rate (RGRD) of the surviving seedlings was 0.23 year−1 in the control subplots (Figure 2). N fertilization (p = 0.006) and P fertilization (p = 0.021) significantly increased diameter growth by 30–50% compared to the control.
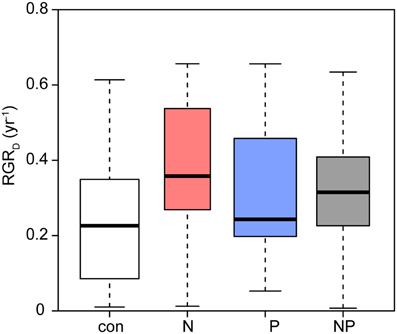
Figure 2. Relative diameter growth rates of Pouteria torta seedlings as a response to moderate nutrient addition. Single addition of N or P caused increased growth rates (N: p = 0.006; P: p = 0.021), interaction of both nutrients resulted in a smaller effect than when the elements were added alone (N × P: p = 0.017).
Leaf Properties and Herbivory
Leaf area showed no significant difference between treatments although largest leaves were found in the N and NP treatments (Table 2). Compared to the control, SLA showed a significant increase of more than 40% after N addition (Figure 3, Table 2). In addition, we observed higher leaf length and leaf width ratios after N addition, whereas leaves in the control and in the P addition plots did not increase their dimensions.
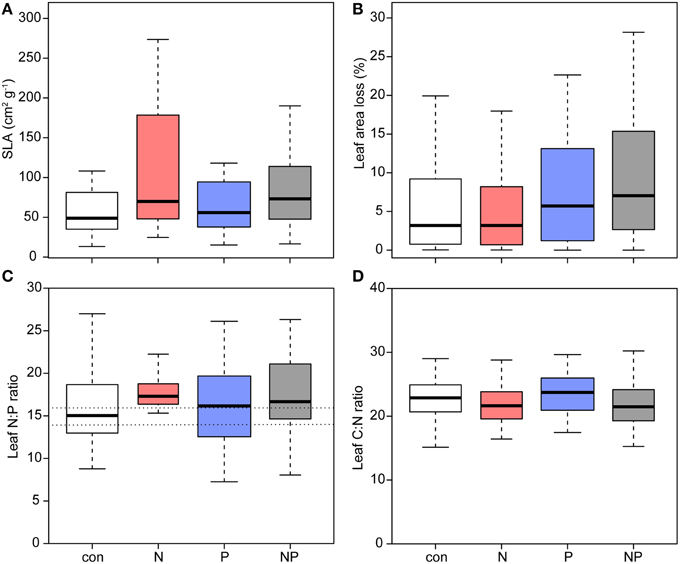
Figure 3. Leaf morphology, leaf area loss and nutrient ratios of Pouteria torta seedlings after 1 year of experimental exposure to nutrient addition. (A) Specific leaf area (SLA) increased after N addition (p = 0.009), (B) the proportion of lost leaf area showed a significant increment after P addition (p = 0.020), (C) foliar N:P ratio was not affected in the treatments (dotted lines mark the range of N:P ratios indicating a balanced supply of both nutrients after Townsend et al., 2007), and (D) leaf C:N ratio was decreased in the two N treatments (p = 0.024).
Seedlings grown in the P and NP treatments had highest leaf area losses compared with seedling in control plots indicating a stimulation of herbivory by P fertilization (Figure 3, Table 2).
Mass based foliar N and P concentrations were only weakly affected by the fertilization since we found only marginally higher foliar N after N addition, but the foliar C/N ratio was lowered (Figure 3). Area-based foliar N and P concentrations were lower in the N treatment.
N and P foliar contents on a mass basis (i.e., total foliar nutrient content per plant) showed no differences between treatments. The mean values of foliar N/P ratios varied between the treatments from 15.9 in the control to 17.9 in the NP treatment.
Biomass Allocation
Across all treatments, Pouteria seedlings allocated most of their biomass to the roots (Table 2, Figure 4). P addition shifted allocation even more to roots as expressed by an increasing root mass fraction (P: 55%, NP: 56% vs. control: 51%) and a higher root/shoot ratio (P: 1.87, NP: 2.07 vs. control: 1.69).
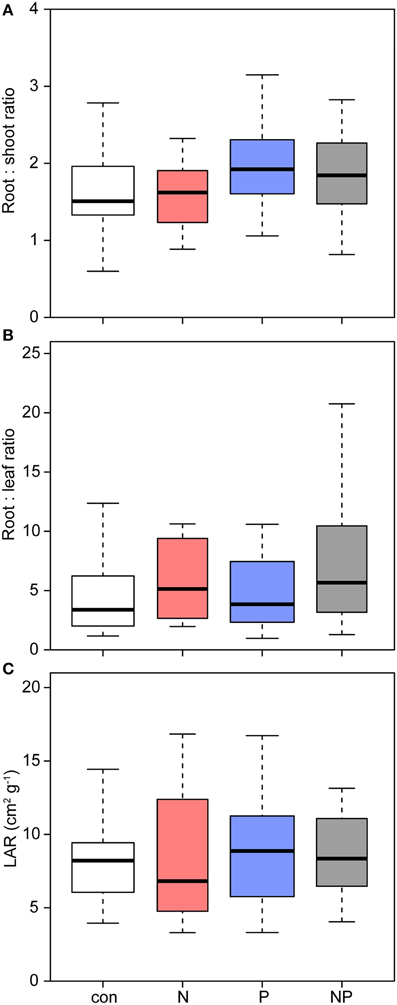
Figure 4. Effects of nutrient addition on the biomass allocation of Pouteria torta seedlings. (A) Changes in the root:shoot ratio indicate a shift in allocation toward the roots after P addition (p = 0.003), (B) N addition increased the root:leaf ratio marginally (p = 0.061), (C) but the leaf area ratio (LAR) was not affected.
The leaf mass fraction of the seedlings in the N treatments was significantly reduced (N: 12%, NP: 13% vs. control: 16%) and the stem mass fraction was higher after N addition (N: 0.35 vs. control: 0.32). Mean LAR was slightly but not significantly higher in the fertilized plots compared to the control.
Discussion
Seedling Mortality and Growth
During the life cycle of a tree, the seedling stage is the most vulnerable phase with susceptibility to physical harm (e.g., litterfall, trampling, herbivory, or pathogen attack) and carbohydrate shortage (Alvarez-Clare and Kitajima, 2007, 2009). Seedling mortality is highly related to the plants' physical and physiological robustness to withstand adverse conditions in order to establish successfully (Kitajima and Fenner, 2000; Alvarez-Clare and Kitajima, 2007).
Under natural forest conditions, seedling transplantation experiments in tropical forests commonly experience high mortality during the first 2 months after seedling transplantation (Eichhorn et al., 2006; Alvarez-Clare and Kitajima, 2009; Brenes-Arguedas et al., 2011), which is mainly attributed to damage by herbivores and defoliation (Paine et al., 2012). In our study, Pouteria torta experienced a high mortality of >50% in the first year after planting, although transplantation occurred 6 months after germination when we assumed that the seedlings already had reached a “robust” stage.
The increase in mortality in the two N treatments is contrary to results of Vincent and Tanner (2013) who reported no reduction of survival rate in transplanted seedlings of two late-successional tree species after NPK addition in a Panamanian lowland forest. Since there was no significant change in soil pH in our plots after 4 years of nutrient addition (Supplementary Table 1; Baldos et al., 2015), we cannot argue with impeded seedling establishment by soil acidification as reported by Lu et al. (2010).
In spite of the likely complex interaction among drivers of seedling growth under natural forest conditions, we found positive effects of either N or P addition on diameter growth of the surviving Pouteria seedlings. Both elements N and P are essential for photosynthesis, since ribulose 1-5-bisphosphate oxygenase/carboxylase (RuBisCO) protein accounts for ~30% of leaf N, and P plays an important role in membrane solubility, ATP, and NADPH production (Marschner, 1995; Walker et al., 2014). Since foliar N and P did not increase while SLA increased, an increase in photosynthetic rate cannot be the cause of higher productivity; rather, the (non-significant) increase in plant leaf area must have led to the higher productivity in the fertilized plants. Several other pot experiments with tropical tree seedling showed positive effects of N and/or P addition on growth as well but other studies reported no effects (see compilation in Lawrence, 2003). In general, tree growth limitation by N and P strongly depends on specific site conditions and on co-limitation by other nutrients. Santiago et al. (2012) showed a 24% increase in relative height growth in naturally established tropical tree seedlings as a response to K addition and a smaller but also positive effect of combined long-term addition of N and P. Increased growth rates are probably the result of enhanced photosynthetic productivity as reported by Pasquini and Santiago (2012) for Alseis blackiana seedlings from the same tropical lowland forest in Panama.
Leaf Properties and Herbivory
Leaf properties are recognized as key traits in determining establishment and survival success of tree seedling under varying site conditions and they are usually a good indicator of potential growth rates. There is a strong trade-off between high leaf lifespan, high leaf toughness and low herbivory and low mortality on the one hand, and high foliar nutrient concentrations and fast growth rates on the other hand (e.g., Poorter and Bongers, 2006; Wright et al., 2010; Kitajima et al., 2013; Philipson et al., 2014).
We observed a strong positive effect of N addition on SLA. Other studies reported a higher SLA in tropical tree seedlings after P addition (e.g., Burslem et al., 1995: Antidesma cuspidatum in Singapore, Wan Juliana et al., 2009: Lagerstroemia floribunda in Malaysia) or found no effect of fertilization on SLA (Santiago et al., 2012: five shade-tolerant tree species). SLA is known to be a good predictor of growth rates in seedlings (Wright and Westoby, 1999, 2000), so the increased growth rates in the N treatments are not surprising. Strong effects of herbivory in the P treatments were probably masking an even larger productivity stimulation by the nutrient addition (especially in the NP treatment, where herbivory and also SLA were significantly increased compared to the control); a similar masking of growth increase by herbivory was shown by Andersen et al. (2010) for different palm species after N addition. Increased leaf damage through herbivory after P addition was also reported by Santiago et al. (2012) in Panama where the proportion of herbivory in seedlings was higher after P or K addition. Werner and Homeier (2015) found a positive correlation of eaten leaf area with both foliar N and P concentrations in a montane forest close to our study site. All these studies show that herbivore choice is strongly driven by resource quality (i.e., nutrient content and palatability of leaves). Hence, herbivory can play a fundamental role in structuring seedling communities by directly affecting the survival of plants or mediating responses to soil nutrient availability (e.g., Andersen et al., 2010; Eichhorn et al., 2010; Barton and Hanley, 2013).
Leaf tissue N:P ratios of 15.9 in the control plots point at a balanced supply of both nutrients (Townsend et al., 2007) and after addition of N or P, Pouteria seedlings did not accumulate the respective nutrient in their leaves. This is contrary to the results of several studies that found an increase of foliar nutrient concentrations in shade-tolerant tree seedlings after fertilization (Burslem et al., 1995; Lawrence, 2003; Andersen et al., 2010; Santiago et al., 2012). It appears that improved nutrient availability induced higher nutrient uptake only at a rate proportional to the increase in growth rate, resulting in a dilution of the added nutrients by carbon and fairly stable foliar nutrient concentrations. Furthermore, Pouteria seedlings may store the added nutrients in stems or roots as investment in long-term survival. Such a strategy of late-successional species was reported by Raaimakers and Lambers (1996) for Lecythis corrugata seedlings in Guyana.
Biomass Allocation
The high root mass fraction of Pouteria seedlings compared to other shade-tolerant tropical tree seedlings (Burslem et al., 1995; Huante et al., 1995a; Wan Juliana et al., 2009) could be explained as an adaptation to generally low nutrient availability at the study site, as it was proven for our study site by the stimulation of tree root growth upon N and P (and also K) addition in the ingrowth core experiment of Graefe et al. (2010). These results indicate that trees put high priority on the maximization of belowground resource acquisition at this site as also reported by Paz (2003) and Poorter et al. (2012) for other tropical forest.
Upon nutrient addition we expected a compensatory change in carbon allocation to aboveground tissues as a result of increased soil nutrient availability (e.g., Marschner, 1995; Poorter et al., 2012), but surprisingly SMF was reduced and root:shoot ratio increased after P addition, and the root:leaf ratio was higher after N addition. In addition, LAR was not affected by nutrient addition. Enhanced C allocation to roots in a shade-tolerant species suggests that carbon and other elements are mainly stored in belowground organs to improve seedling survival under the very low light intensity at the forest floor of this stand (Raaimakers and Lambers, 1996).
Conclusions
Foliar nutrient concentrations are apparently not a good indicator of nutrient limitation in the rainforest tree Pouteria torta, since nutrient addition did change neither N or P concentration nor the ratio of both nutrients. The species' high abundance in the forests is another indication that it is well-adapted to the current growing conditions at this site. Nevertheless, the shade-tolerant species was capable of responding to improved nutrient supply with higher seedling growth rates, if the plants were able to survive in the relatively dark and nutrient-poor environment. On the population level, however, it is difficult to make predictions on how a future increase in nutrient deposition will change the recruitment success of this species, because individual growth increases are associated by increased herbivory and higher mortality. In any case, the moderate addition of 50 kg N ha−1 and 10 kg P ha−1 has provoked a number of morphological and physiological responses that likely will alter the performance of this common tree species and will have consequences for the competition with other tree species. In further studies it would be interesting to investigate if the co-occurring tree species respond similarly to N and P addition or if currently less abundant species gain competitive strength thus indicating potential changes in the composition of future forests under increased nutrient deposition.
Conflict of Interest Statement
The authors declare that the research was conducted in the absence of any commercial or financial relationships that could be construed as a potential conflict of interest.
Acknowledgments
We gratefully acknowledge Jaime Peña, Nelson Jaramillo, Marlene Kroner, María López, and Adrian Thiele for their assistance in the field. We also thank Marion Schmelz for help with the leaf analysis and the Ministerio de Ambiente del Ecuador for granting the research permit. This research was supported by the German Research Foundation (DFG) as part of Project A1 (DFG HO 3296/2 and HO 3296/4) of the DFG research unit 816. Daisy Cárate Tandalla received a scholarship of the German Academic Exchange Service (DAAD). We acknowledge support by the German Research Foundation and the Open Access Publication Funds of the Göttingen University.
Supplementary Material
The Supplementary Material for this article can be found online at: https://www.frontiersin.org/article/10.3389/feart.2015.00075
References
Alvarez-Clare, S., and Kitajima, K. (2009). Susceptibility of tree seedlings to biotic and abiotic hazards in the understory of a moist tropical forest in Panama. Biotropica 41, 47–56. doi: 10.1111/j.1744-7429.2008.00442.x
Alvarez-Clare, S., and Kitajima, K. (2007). Physical defence traits enhance seedling survival of neotropical tree species. Funct. Ecol. 21, 1044–1054. doi: 10.1111/j.1365-2435.2007.01320.x
Alvarez-Clare, S., Mack, M. C., and Brooks, M. (2013). A direct test of nitrogen and phosphorus limitation to net primary productivity in a lowland tropical wet forest. Ecology 94, 1540–1551. doi: 10.1890/12-2128.1
Andersen, K. M., Corre, M. D., Turner, B. L., and Dalling, J. W. (2010). Plant-soil associations in a lower tropical forest: physiological acclimation and herbivore-mediated responses to nitrogen addition. Funct. Ecol. 24, 1171–1180. doi: 10.1111/j.1365-2435.2010.01731.x
Baldos, A., Corre, M. D., and Veldkamp, E. (2015). Responses of N cycling to nutrient inputs in forest soils across a 1000-3000 m elevation gradient in the Ecuadorian Andes. Ecology 96, 749–761. doi: 10.1890/14-0295.1
Barton, K. E., and Hanley, M. E. (2013). Seedling–herbivore interactions: insights into plant defence and regeneration patterns. Ann. Bot. 112, 643–650. doi: 10.1093/aob/mct139
Bates, D., Maechler, M., Bolker, B., and Walker, S. (2014). _lme4: Linear Mixed-effects Models Using Eigen and S4_. R package version 1.1-7, Available online at: http://CRAN.R-project.org/package=lme4
Brenes-Arguedas, T., Roddy, A. B., Coley, P. D., and Kursar, T. A. (2011). Do differences in understory light contribute to species distributions along a tropical rainfall gradient? Oecologia 166, 443–456. doi: 10.1007/s00442-010-1832-9
Burslem, D. F. R. P., Grubb, P. J., and Turner, I. M. (1995). Responses to nutrient addition among shade-tolerant tree seedlings of lowland tropical rain forest in Singapore. J. Ecol. 83, 113–122. doi: 10.2307/2261155
Camenzind, T., Hempel, S., Homeier, J., Horn, S., Velescu, A., Wilcke, W., et al. (2014). Nitrogen and phosphorus additions impact arbuscular mycorrhizal abundance and molecular diversity in a tropical montane forest. Global Change Biol. 20, 3646–3659. doi: 10.1111/gcb.12618
Dent, D. H., and Burslem, D. F. (2009). Performance trade-offs driven by morphological plasticity contribute to habitat specialization of Bornean tree species. Biotropica 41, 424–434. doi: 10.1111/j.1744-7429.2009.00505.x
Eichhorn, M. P., Compton, S. G., and Hartley, S. E. (2006). Seedling species determines rates of leaf herbivory in a Malaysian rain forest. J. Tropical Ecol. 22, 513–519. doi: 10.1017/S026646740600335X
Eichhorn, M. P., Nilus, R., Compton, S. G., Hartley, S. E., and Burslem, D. F. (2010). Herbivory of tropical rain forest tree seedlings correlates with future mortality. Ecology 91, 1092–1101. doi: 10.1890/09-0300.1
Elser, J. J., Bracken, M. E., Cleland, E. E., Gruner, D. S., Harpole, W. S., Hillebrand, H., et al. (2007). Global analysis of nitrogen and phosphorus limitation of primary producers in freshwater, marine and terrestrial ecosystems. Ecol. Lett. 10, 1135–1142. doi: 10.1111/j.1461-0248.2007.01113.x
Emck, P. (2007). A Climatology of South Ecuador with Special Focus on the Major Andean Ridge as Atlantic-Pacific Climate Divide. Ph.D. thesis, University of Erlangen, Erlangen.
Fabian, P., Kohlpaintner, M., and Rollenbeck, R. (2005). Biomass burning in the Amazon-fertilizer for the mountaineous rain forest in Ecuador. Environ. Sci. Pollut. Res. 12, 290–296. doi: 10.1065/espr2005.07.272
Fisher, J. B., Malhi, Y., Cuba Torres, I., Metcalfe, D. B., van de Weg, M. J., Meir, P., et al. (2013). Nutrient limitation in rainforests and cloud forests along a 3000m elevation gradient in the Peruvian Andes. Oecologia 172, 889–902. doi: 10.1007/s00442-012-2522-6
Frazer, G. W., Canham, C. D., and Lertzman, K. P. (1999). Gap light analyzer (GLA), Version 2.0: Imaging Software to Extract Canopy Structure and Gap Light Transmission Indices Fron True-colour Fisheye Photographs, User's Manual and Program Documentation. Copyright 1999. Burnaby, BC; Millbrook, NY: Simon Fraser University; The Institute of Ecosystem Studies.
Graefe, S., Hertel, D., and Leuschner, C. (2010). N, P and K limitation of fine root growth along an elevation transect in tropical mountain forests. Acta Oecologica 36, 537–542. doi: 10.1016/j.actao.2010.07.007
Harpole, W. S., Ngai, J. T., Cleland, E. E., Seabloom, E. W., Borer, E. T., Bracken, M. E. S., et al. (2011). Nutrient co-limitation of primary producer communities. Ecol. Lett. 14, 852–862. doi: 10.1111/j.1461-0248.2011.01651.x
Holste, E. K., Kobe, R. K., and Vriesendorp, C. F. (2011). Seedling growth responses to soil resources in the understory of a wet tropical forest. Ecology 92, 1828–1838. doi: 10.1890/10-1697.1
Homeier, J., Hertel, D., Camezind, T., Cumbicus, N. L., Maraun, M., Martinson, G. O., et al. (2012). Tropical Andean Forests are highly susceptible to nutrient inputs- Rapid effects of experimental N and P addition to an Ecuadorian montane forest. PLoS ONE 7:e47128. doi: 10.1371/journal.pone.0047128
Homeier, J., Leuschner, C., Bräuning, A., Cumbicus, N. L., Hertel, D., Martinson, G. O., et al. (2013). “Effects of nutrient addition on the productivity of montane forests and implications for the carbon cycles,” in Ecosystem Services, Biodiversity and Environmental Change in a Tropical Mountain Ecosystem of South Ecuador. Ecological Studies Vol. 221, eds J. Bendix, E. Beck, F. M. Bräuning, R. Mosandl, and S. Scheu (Heidelberg: Springer), 315–329. doi: 10.1007/978-3-642-38137-9_23
Homeier, J., Werner, F. A., Gradstein, S. R., Breckle, S.–W., and Richter, M. (2008). “Potential vegetation and floristic composition of Andean forests in South Ecuador, with a focus on the RBSF,” in Gradients in a Tropical Mountain Ecosystem of Ecuador. Ecological Studies, Vol. 198, eds E. Beck, J. Bendix, I. Kottke, F. Makeschin, and R. Mosandl (Berlin; Heidelberg; Springer), 87–100.
Hothorn, T., Bretz, F., and Westfall, P. (2008). Simultaneous inference in general parametric models. Biom. J. 50, 346–363. doi: 10.1002/bimj.200810425
Huante, P., Rincón, E., and Acosta, I. (1995a). Nutrient availability and growth rate of 34 woody species from a tropical deciduous forest in Mexico. Funct. Ecol. 9, 849–858. doi: 10.2307/2389982
Huante, P., Rincón, E., and Chapin, F. S. III. (1995b). Responses to phosphorus of contrasting successional tree-seedling species from the tropical deciduous forest of Mexico. Funct. Ecol. 9, 760–766. doi: 10.2307/2390249
Kitajima, K. (1996). “Ecophysiology of tropical tree seedlings,” in Tropical Forest Plant Ecophysiology, eds S. Mulkey, R. Chazdon, and A. Smith (New York NY: Chapman and Hall), 559–596.
Kitajima, K., Cordero, R. A., and Wright, S. J. (2013). Leaf life span spectrum of tropical woody seedlings: effects of light and ontogeny and consequences for survival. Ann. Bot. 112, 685–699. doi: 10.1093/aob/mct036
Kitajima, K., and Fenner, M. (2000). “Ecology of seedlings regeneration,” in Seeds: The Ecology Regeneration in Plant Communities, 2nd Edn., ed M. Fenner (London: CABI), 331–359.
Krashevska, V., Sandmann, D., Maraun, M., and Scheu, S. (2014). Moderate changes in nutrient input alter tropical microbial and protist communities and belowground linkages. ISME J. 8, 1126–1134. doi: 10.1038/ismej.2013.209
Lawrence, D. (2001). Nitrogen and phosphorus enhance growth and luxury consumption of four secondary forest tree species in Borneo. J. Trop. Ecol. 17, 859–869. doi: 10.1017/S0266467401001638
Lawrence, D. (2003). The response of tropical tree seedlings to nutrient supply: meta-analysis for understanding a changing tropical landscape. J. Trop. Ecol. 19, 239–250. doi: 10.1017/S0266467403003274
Lewis, S. L., and Tanner, E. V. (2000). Effects of above-and belowground competition on growth and survival of rain forest tree seedlings. Ecology 81, 2525–2538. doi: 10.1890/0012-9658(2000)081[2525:EOAABC]2.0.CO;2
Lu, X., Mo, J., Gilliam, F. S., Zhou, G., and Fang, Y. (2010). Effects of experimental nitrogen additions on plant diversity in an old-growth tropical forest. Global Change Biol. 16, 2688–2700. doi: 10.1111/j.1365-2486.2010.02174.x
Martinson, G. O., Corre, M. D., and Veldkamp, E. (2013). Responses of nitrous oxide fluxes and soil nitrogen cycling to nutrient additions in montane forests along an elevation gradient in southern Ecuador. Biogeochemistry 112, 625–636. doi: 10.1007/s10533-012-9753-9
Metz, M. R., Comita, L. S., Chen, Y. Y., Norden, N., Condit, R., Hubbell, S. P., et al. (2008). Temporal and spatial variability in seedling dynamics: a cross-site comparison in four lowland tropical forests. J. Tropical Ecol. 24, 9–18. doi: 10.1017/S0266467407004695
Müller, A. K., Matson, A. L., Corre, M. D., and Veldkamp, E. (2015). Soil N2O fluxes along an elevation gradient of tropical montane forests during five years of chronic nutrient inputs. Front. Earth Sci. 3:66. doi: 10.3389/feart.2015.00066
Paine, C. E., Stenflo, M., Philipson, C. D., Saner, P., Bagchi, R., Ong, R. C., et al. (2012). Differential growth responses in seedlings of ten species of Dipterocarpaceae to experimental shading and defoliation. J. Trop. Ecol. 28, 377–384. doi: 10.1017/S0266467412000326
Pasquini, S. C., and Santiago, L. S. (2012). Nutrients limit photosynthesis in seedlings of a lowland tropical forest tree species. Oecologia 168, 311–319. doi: 10.1007/s00442-011-2099-5
Paz, H. (2003). Root/Shoot allocation and root architecture in seedlings: variation among Forest Sites, Microhabitats, and Ecological Groups1. Biotropica 35, 318–332. doi: 10.1111/j.1744-7429.2003.tb00586.x
Pennington, T. D. (2007). Sapotaceae. Flora of Ecuador, Vol. 80, Department of Plant and Environmental Sciences, Göteborg: Göteborg University.
Philipson, C. D., Dent, D. H., O'Brien, M. J., Chamagne, J., Dzulkifli, D., Nilus, R., et al. (2014). A trait-based trade-off between growth and mortality: evidence from 15 tropical tree species using size-specific relative growth rates. Ecol. Evol. 4, 3675–3688. doi: 10.1002/ece3.1186
Pinheiro, J., Bates, D., DebRoy, S., Sarkar, D., and R Core Team (2014). _nlme: Linear and Nonlinear Mixed Effects Models_. R package version 3.1-117, Available online at: http://CRAN.R-project.org/package=nlme
Poorter, H., Niklas, K. J., Reich, P. B., Oleksyn, J., Poot, P., and Mommer, L. (2012). Biomass allocation to leaves, stems and roots: meta-analyses of interspecific variation and environmental control. New Phytol. 193, 30–50. doi: 10.1111/j.1469-8137.2011.03952.x
Poorter, L., and Bongers, F. (2006). Leaf traits are good predictors of plant performance across 53 rain forest species. Ecology 87, 1733–1743. doi: 10.1890/0012-9658(2006)87[1733:LTAGPO]2.0.CO;2
Raaimakers, D., and Lambers, H. (1996). Response to phosphorus supply of tropical tree seedlings: a comparison between a pioneer species Tapirira obtusa and a climax species Lecythis corrugata. New Phytol. 132, 97–102. doi: 10.1111/j.1469-8137.1996.tb04513.x
R Development Core Team (2014). R: A Language and Environment for Statistical Computing. Vienna: R Foundation for Statistical Computing.
Santiago, L. S., Wright, S. J., Harms, K. E., Yavitt, J. B., Korine, C., Garcia, M. N., et al. (2012). Tropical tree seedling growth responses to nitrogen, phosphorus and potassium addition. J. Ecol. 100, 309–316. doi: 10.1111/j.1365-2745.2011.01904.x
Swaine, M. D. (1996). Rainfall and soil fertility as factors limiting forest species distributions in Ghana. J. Ecol. 419–428. doi: 10.2307/2261203
Tanner, E. V. J., Vitousek, P. M., and Cuevas, E. (1998). Experimental investigation of nutrient limitation of forest growth on wet tropical mountains. Ecology 79, 10–22. doi: 10.1890/0012-9658(1998)079[0010:EIONLO]2.0.CO;2
Townsend, A. R., Cleveland, C. C., Asner, G. P., and Bustamante, M. M. (2007). Controls over foliar N:P ratios in tropical rain forests. Ecology 88, 107–118. doi: 10.1890/0012-9658(2007)88[107:COFNRI]2.0.CO;2
Unger, M., Leuschner, C., and Homeier, J. (2010). Variability of indices of macronutrient availability in soils at different spatial scales along an elevation transect in tropical moist forests (NE Ecuador). Plant Soil 336, 443–458. doi: 10.1007/s11104-010-0494-z
Vincent, A. G., and Tanner, E. V. (2013). Major litterfall manipulation affects seedling growth and nutrient status in one of two species in a lowland forest in Panama. J. Trop. Ecol. 29, 449–454. doi: 10.1017/S0266467413000424
Vitousek, P. M., Porder, S., Houlton, B. Z., and Chadwick, O. A. (2010). Terrestrial phosphorus limitation: mechanisms, implications, and nitrogen-phosphorus interactions. Ecol. Appl. 20, 5–15. doi: 10.1890/08-0127.1
Walker, A. P., Beckerman, A. P., Gu, L., Kattge, J., Cernusak, L. A., Domingues, T. F., et al. (2014). The relationship of leaf photosynthetic traits–Vcmax and Jmax–to leaf nitrogen, leaf phosphorus, and specific leaf area: a meta-analysis and modeling study. Ecol. Evol. 4, 3218–3235. doi: 10.1002/ece3.1173
Wan Juliana, W. A., Burslem, D. F. R. P., and Swaine, M. D. (2009). Nutrient limitation of seedling growth on contrasting soils from Pasoh Forest Reserve, Peninsular Malaysia. J. Tropical Forest Sci. 21, 316–327.
Werner, F. A., and Homeier, J. (2015). Is tropical montane forest heterogeneity promoted by a resource-driven feedback cycle? Evidence from nutrient relations, herbivory and litter decomposition along a topographical gradient. Funct. Ecol. 29, 430–440. doi: 10.1111/1365-2435.12351
Whitmore, T. C. (1996). “A review of some aspects of tropical rain forest seedling ecology with suggestions for the further enquiry,” in The Ecology of Tropical Forest Tree Seedling. Man and the Biosphere Series, Vol. 17, ed M. D. Swaine (Paris: UNESCO), 3–39.
Wilcke, W., Leimer, S., Peters, T., Emck, P., Rollenbeck, R., Trachte, K., et al. (2013). The nitrogen cycle of tropical montane forest in Ecuador turns inorganic under environmental change. Global Biogeochem. Cycles 27, 1194–1204. doi: 10.1002/2012GB004471
Wittich, B., Homeier, J., and Leuschner, C. (2015). Ammonium, nitrate and glycine uptake of six Ecuadorian tropical montane forest tree species: an in situ pot experiment with saplings. J. Trop. Ecol. 31, 139–152. doi: 10.1017/s0266467414000650
Wolf, K., Veldkamp, E., Homeier, J., and Martinson, G. O. (2011). Nitrogen availability links forest productivity, soil nitrous oxide and nitric oxide fluxes of a tropical montane forest in southern Ecuador. Global Biogeochem. Cycles 25:GB4009. doi: 10.1029/2010gb003876
Wright, I. J., and Westoby, M. (1999). Differences in seedling growth behaviour among species: trait correlations across species, and trait shifts along nutrient compared to rainfall gradients. J. Ecol. 87, 85–97. doi: 10.1046/j.1365-2745.1999.00330.x
Wright, I. J., and Westoby, M. (2000). Cross-species relationships between seedling relative growth rate, nitrogen productivity and root vs leaf function in 28 Australian woody species. Funct. Ecol. 14, 97–107. doi: 10.1046/j.1365-2435.2000.00393.x
Wright, S. J., Kitajima, K., Kraft, N. J., Reich, P. B., Wright, I. J., Bunker, D. E., et al. (2010). Functional traits and the growth-mortality trade-off in tropical trees. Ecology 91, 3664–3674. doi: 10.1890/09-2335.1
Yavitt, J. B., and Wright, S. J. (2008). Seedling growth responses to water and nutrient augmentation in the understorey of a lowland moist forest, Panama. J. Trop. Ecol. 24, 19–26. doi: 10.1017/S0266467407004713
Keywords: Ecuador, tree seedlings, diameter growth, herbivory, foliar nutrients, nutrient manipulation experiment, Pouteria torta, seedling transplantation experiment
Citation: Cárate-Tandalla D, Leuschner C and Homeier J (2015) Performance of Seedlings of a Shade-Tolerant Tropical Tree Species after Moderate Addition of N and P. Front. Earth Sci. 3:75. doi: 10.3389/feart.2015.00075
Received: 22 May 2015; Accepted: 13 November 2015;
Published: 02 December 2015.
Edited by:
Samuel Abiven, University of Zurich, SwitzerlandReviewed by:
Margaret Metz, Lewis & Clark College, USALindsay Banin, Centre for Ecology and Hydrology, UK
Copyright © 2015 Cárate-Tandalla, Leuschner and Homeier. This is an open-access article distributed under the terms of the Creative Commons Attribution License (CC BY). The use, distribution or reproduction in other forums is permitted, provided the original author(s) or licensor are credited and that the original publication in this journal is cited, in accordance with accepted academic practice. No use, distribution or reproduction is permitted which does not comply with these terms.
*Correspondence: Jürgen Homeier, jhomeie@gwdg.de