Variable Late Holocene 14C Reservoir Ages in Lake Bosten, Northwestern China
- 1State Key Laboratory of Loess and Quaternary Geology, Institute of Earth Environment, Chinese Academy of Sciences, Xi’an, China
- 2University of Chinese Academy of Sciences, Beijing, China
- 3Institute of Surface-Earth System Science, Tianjin University, Tianjin, China
- 4College of Resources and Environment, Zunyi Normal University, Zunyi, China
- 5Key Laboratory of Disaster Monitoring and Mechanism Simulating of Shaanxi Province, Baoji University of Arts and Sciences, Baoji, China
Radiocarbon (14C) dating has been widely used in paleoclimate reconstruction. However, the reliability of the 14C age in lake sediments is sensitive to the 14C reservoir effect, especially for a lake in arid regions. In this study, we evaluated the 14C reservoir ages under different hydroclimatic conditions over the past ∼2300 years in Lake Bosten, northwestern China, by comparing with different dating results and with multi-proxy indices of a vertical-down sediment core collected in this lake. The 14C reservoir ages during ∼1800 to 650 BP (a dry interval) are estimated to be approximately 1170 years older than those during ∼650–100 BP and those during 2200–1800 BP (wet intervals). We proposed that variation in 14C exchange rate between the dissolved CO2 in lake water and CO2 in the atmosphere, as well as the changing proportion of organic matter in the lake and the catchment, could have contributed to the variable 14C reservoir ages in Lake Bosten. The result of this study suggests that the 14C reservoir effect may be larger in dry periods than in wet periods in the arid/semi-arid area, which should be considered when establishing age models of lake sediment cores from these regions.
Introduction
Radiocarbon (14C) dating has been widely used to establish geochronology less than ∼50,000 years (Hughen et al., 2004; Reimer et al., 2009), using a variety of dating materials, including fossils of terrestrial and aquatic plants, bulk organic matter, etc. (Snyder et al., 1994; Bennike, 2000). The basis of 14C dating is that the 14C concentration of the living dating material, which exchanges 14C with atmosphere CO2 directly, is equal to the contemporaneous atmospheric 14C concentration (e.g., Deevey et al., 1954). For example, a terrestrial plant absorbs atmospheric CO2 during photosynthesis, and its 14C concentration is generally regarded to be identical to the atmospheric 14C concentration. Therefore, land plant debris has been widely used as an ideal 14C dating material in paleolimnology.
However, in many cases, due to the paucity of sufficient terrestrial plant debris in lake sediments, bulk organic matter and aquatic shells are frequently used in radiocarbon dating, and the 14C ages of these materials are generally more or less contaminated by the 14C reservoir effect. This is due to the disequilibrium of 14C concentration between aquatic CO2 and atmospheric CO2, and due to the partial utilization of the dissolved inorganic carbon (DIC) in lake waters to synthesize both organic and inorganic materials. In addition, the input of “old” materials from the catchment to the lake will also lead to “older” 14C ages of bulk organic matter than their real ages.
Previous studies have reported markedly different 14C reservoir ages among different lakes. For example, the 14C reservoir ages in Lake Wulungu (Liu et al., 2008a), Lake Qinghai (Shen et al., 2005), Chaka Salt Lake (Liu et al., 2008b), Lake Zigê Tangco (Herzschuh et al., 2006), Lake Kusai (Liu et al., 2009), and Lake Harnur (Lan et al., 2018) in northwestern China are estimated to be 760, 1073, 1700, 2010, 3400, and 3560 years, respectively, suggesting that the 14C reservoir effects in different lakes can vary from hundreds to thousands of years (Table 1). The reservoir ages in the same lake estimated by different studies are also sometimes different. For example, Chen et al. (2006) and Huang et al. (2009) estimated a 1140-year 14C reservoir age in Lake Bosten. Zhang et al. (2004) proposed an old carbon age of 650 years in the same lake, whereas Wünnemann et al. (2006) contended that the reservoir effect in Lake Bosten is minor and neglectable.
Mischke et al. (2013) showed that the 14C reservoir ages change spatially even in different sites of a same lake on the Tibetan Plateau. These spatially variable reservoir ages may be related to different degrees of 14C exchange between atmospheric CO2 and aquatic CO2, and may be partly attributed to the different “old” carbon released from erosion of carbon-contained bedrocks. ChongYi et al. (2018) also found that the 14C ages of total organic carbon in surface sediments in Lake Qinghai vary spatially and attributed these ages to different degrees of 14C exchange between aquatic and atmospheric CO2. Hou et al. (2012) studied the 14C reservoir effect of different lakes in Tibetan Plateau, and they suggested that the 14C reservoir ages are related to geological settings of the catchment, residence time of lake water, peat/wetland development within the lake catchment, etc.
In addition, 14C reservoir ages of different periods in a same lake may be variable as well. For example, Zhou et al. (2014) got different 14C reservoir ages (135, 1143, and 2523 years, respectively) at different depths of a sediment core in Lake Qinghai. The old carbon ages in Lake Xingyun were estimated to vary between 960 and 2200 years over the past 8000 years (Zhou et al., 2015), and these variable old carbon reservoir ages were likely related to the variable input of “old materials” from the catchment under different hydrological conditions. Since changes of 14C reservoir ages in the same lake may largely affect the accuracy of the 14C age model of vertical-down sediment cores, it is necessary to assess the 14C reservoir ages in the same lake in different periods.
Lakes in the arid/semi-arid regions of China, most of which are closed and/or semi-closed lakes, are often high in salinity and alkalinity, and the 14C reservoir ages of these lakes are variable. Lake Bosten is a widely noted site to study paleoclimatic changes, in Xinjiang, northwestern China, arid central Asia (Mischke and Wünnemann, 2006; Wünnemann et al., 2006). However, previous studies have not fully considered the possibly variable 14C reservoir ages, and therefore parts of the previously published age models may need to be improved/corrected. In this study, we assessed the old carbon effects by comparison between the 14C ages of bulk organic matter and the 137Cs and 210Pb ages of sediment in Lake Bosten and by comparison between multi-proxy indices extracted from this lake and those from other works.
Background and Methods
Lake Bosten is a fault lake located at the southern foot of central Tianshan Mountains (41°56′–42°14′N, 86°40′–87°26′E; modern lake level: 1048 m asl). The catchment area is ∼55,600 km2, and the modern lake area is ∼1000 km2, with a maximum water depth of ∼17 m (Xiao et al., 2010). Lake Bosten is supplied by 13 rivers, among which the Kaidu River is the largest one. River water of the Kaidu River mainly comes from alpine glacier and permanent snowmelt water, as well as seasonal snowmelt water and summer precipitation (Wünnemann et al., 2006).
Annual mean temperature around Lake Bosten is ∼8.3°C, and the average temperature in January and July are −9.2°C and 23.6°C, respectively (Huang et al., 2009). Annual precipitation is ∼70 mm, mostly falling in the warm season (Figure 1). Annual evaporation is ∼2000 mm (Huang et al., 2009). Precipitation in the lower reaches of the lake basin is relatively high; for example, it reaches ∼400 mm/a in the eastern part of Tianshan Mountain (Hu, 2004). Water vapor in the study area comes from the Atlantic, Mediterranean, and Caspian Sea water supply (Aizen et al., 2006; Chen et al., 2008; Xu et al., 2019b; Yan et al., 2019). Salinity of modern lake water is unevenly distributed, with a spatially increasing trend from west to east (Jiu et al., 1990). The lowest salinity of the lake is in the Kaidu River estuary (0.20–0.28%; Mischke and Wünnemann, 2006), and the maximum value is in the eastern part of the lake (>2.5%; Jiu et al., 1990). The pH value of lake water is 8.3–9.0 (Mischke and Wünnemann, 2006).
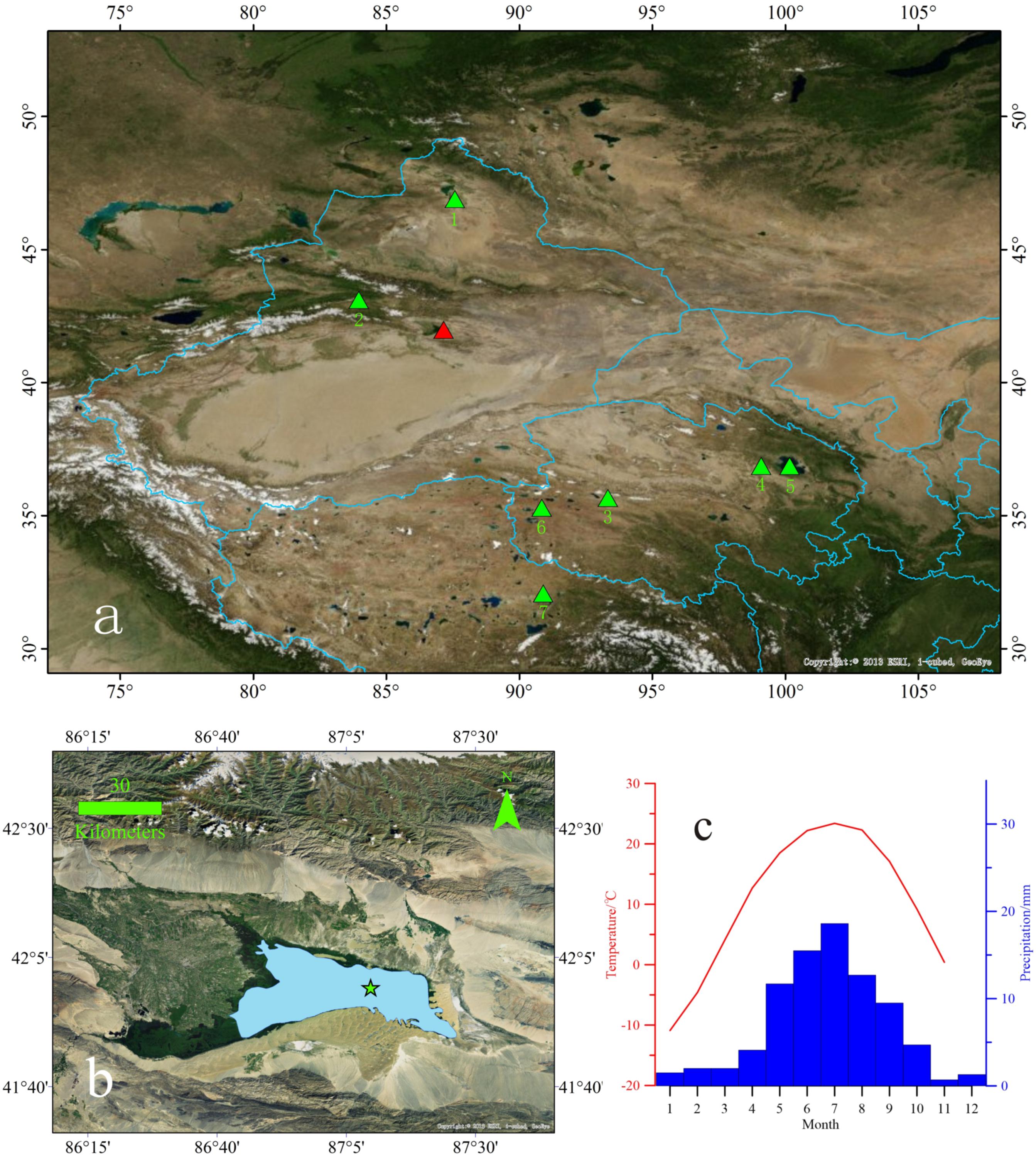
Figure 1. Locations of Lake Bosten (red triangle in panel a) and some lakes mentioned in the text (green triangles). 1, Lake Wulungu (Liu et al., 2008a); 2, Lake Harnur (Lan et al., 2018); 3, Lake Kusai (Liu et al., 2009); 4, Lake Chaka (Liu et al., 2008b); 5, Lake Qinghai (Shen et al., 2005); 6, Lake Donggi Cona (Mischke et al., 2013); 7, Lake Zigê Tangco (Herzschuh et al., 2006). (b) Shows the modern lake and the sampling site of this study (green star). (c) Shows the seasonal temperature and precipitation at Yanqi County (∼80 km to Lake Bosten; data from http://data.cma.cn/data/weatherBk.html; 1981–2010).
A 153-cm-long sediment core (BL13-1-4; 41°59′10.29″N, 87°09′43.70″E; water depth: 12 m) was collected from the central lake (Figure 1), in August 2013, using a gravity corer (UWITEC). The uppermost 24 cm is typical gray lacustrine sediment; 25 –40 cm is gray sandy sediment; 41–137 cm is gray lacustrine sediment; 138–144 cm is sandy gray-white sediment; and 145–153 cm is gray lacustrine sediment. The core was sectioned at every 1-cm interval in situ and was stored under low temperature conditions (−20°C) until freeze-dried.
Radioactivities of 210Pb and 137Cs were determined using a gamma detector [Ortec Germanium (HPGe) well detector; GWL-15-250; Figure 2]. No macroscopic plant debris for radiocarbon dating was observed in the core, and 12 samples were dated using bulk organic carbon at Institute of Surface-Earth System Science (ISESS), Tianjin University, and Beta Analytic (Figure 3 and Table 2). Sedimentary carbonate contents (carb%) were determined by titration with HClO4 (0.1 mol/L) and NaOH (0.1 mol/L), with uncertainty less than 0.5%. Sedimentary grain size was determined on a Malvern Mastersizer 2000 laser grain-size analyzer, with uncertainty less than 3%. Total organic carbon content (TOC), total nitrogen content (TN), and the organic carbon and nitrogen isotopes were determined (δ13C and δ15N) on an elementary analyzer–mass spectrometer (Flash2000-MAT253), with uncertainty less than 0.1% (Figure 4).
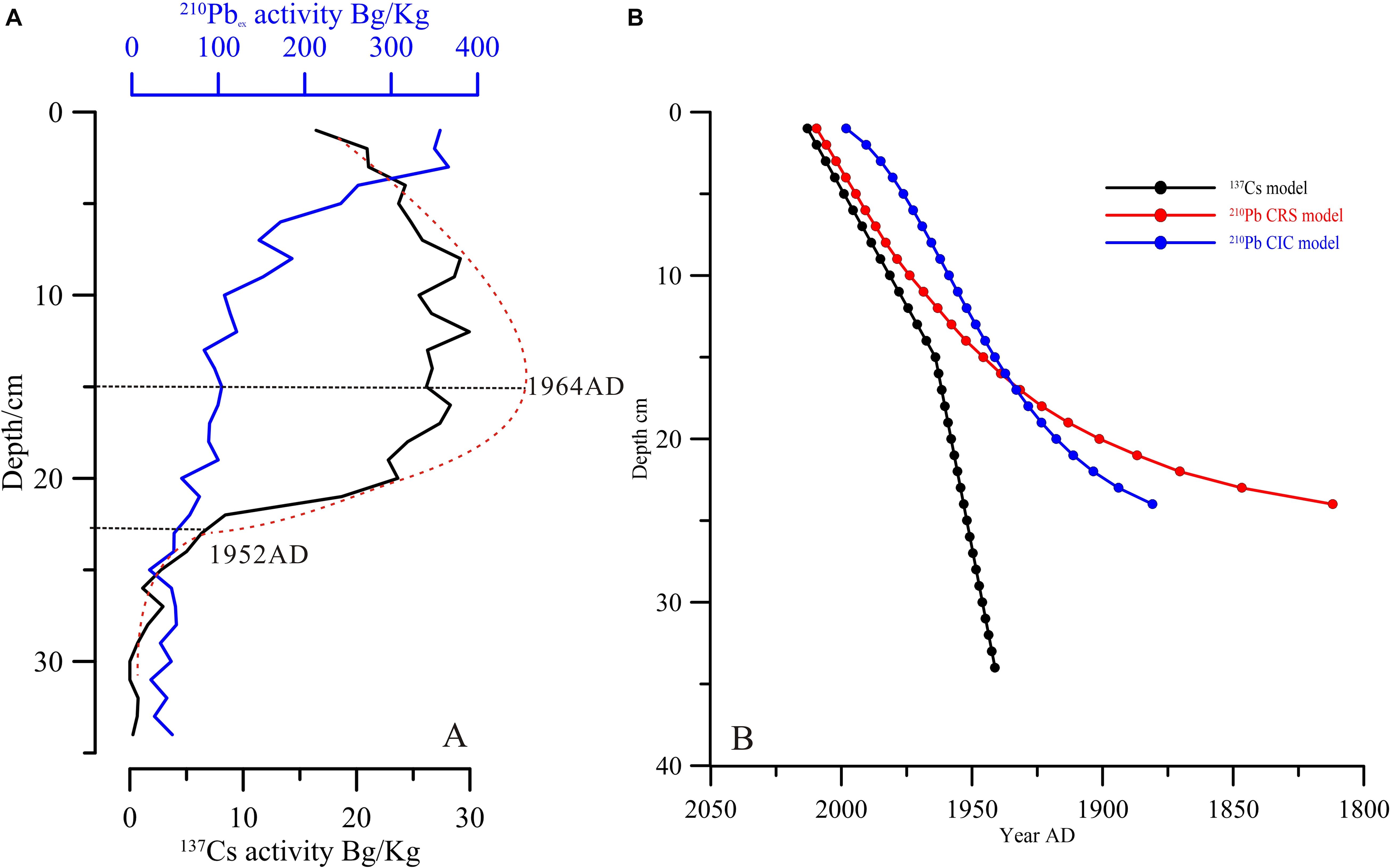
Figure 2. (A) 137Cs and 210Pb activity of the surface sediments in core BL13-1-4. (B) 137Cs and 210Pb age models (see text for details).
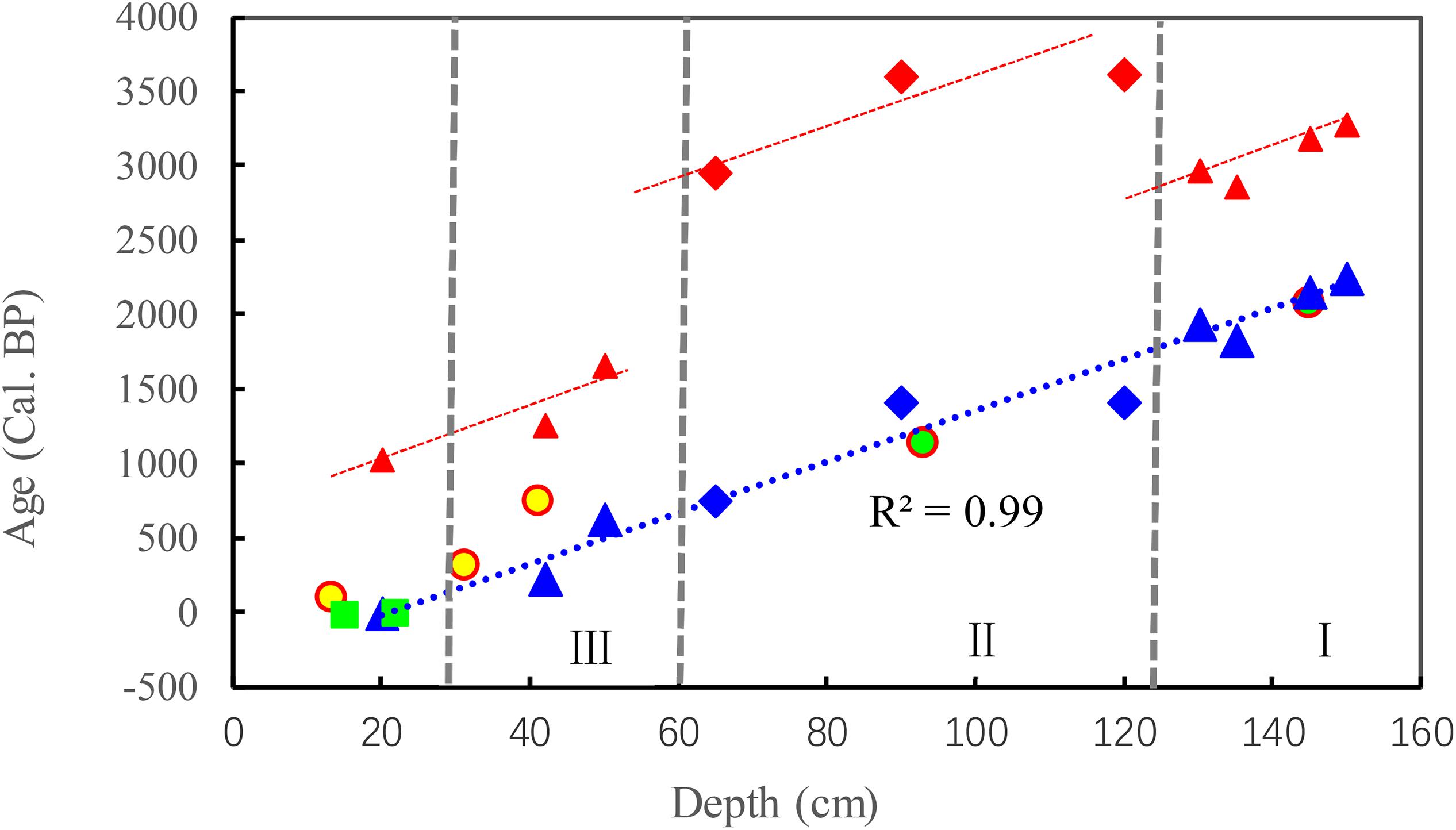
Figure 3. Radiocarbon ages of sediment samples in core BL13-1-4 from Lake Bosten (the red points denote the original 14C ages, blue points denote the 14C ages after old carbon correction). Blue triangles denote 14C ages of wet intervals (interval I and III; see text for details) after a correction of 1033 years. Blue diamonds denote 14C ages of dry interval (interval II) after a correction of 2200 years. The green squares denote the 137Cs time markers (1964-year and 1952-year). Green and yellow filled circles denote 14C ages of plant debris and those of bulk organic matter and humic materials, respectively (data from others’ previous work; Table 3).
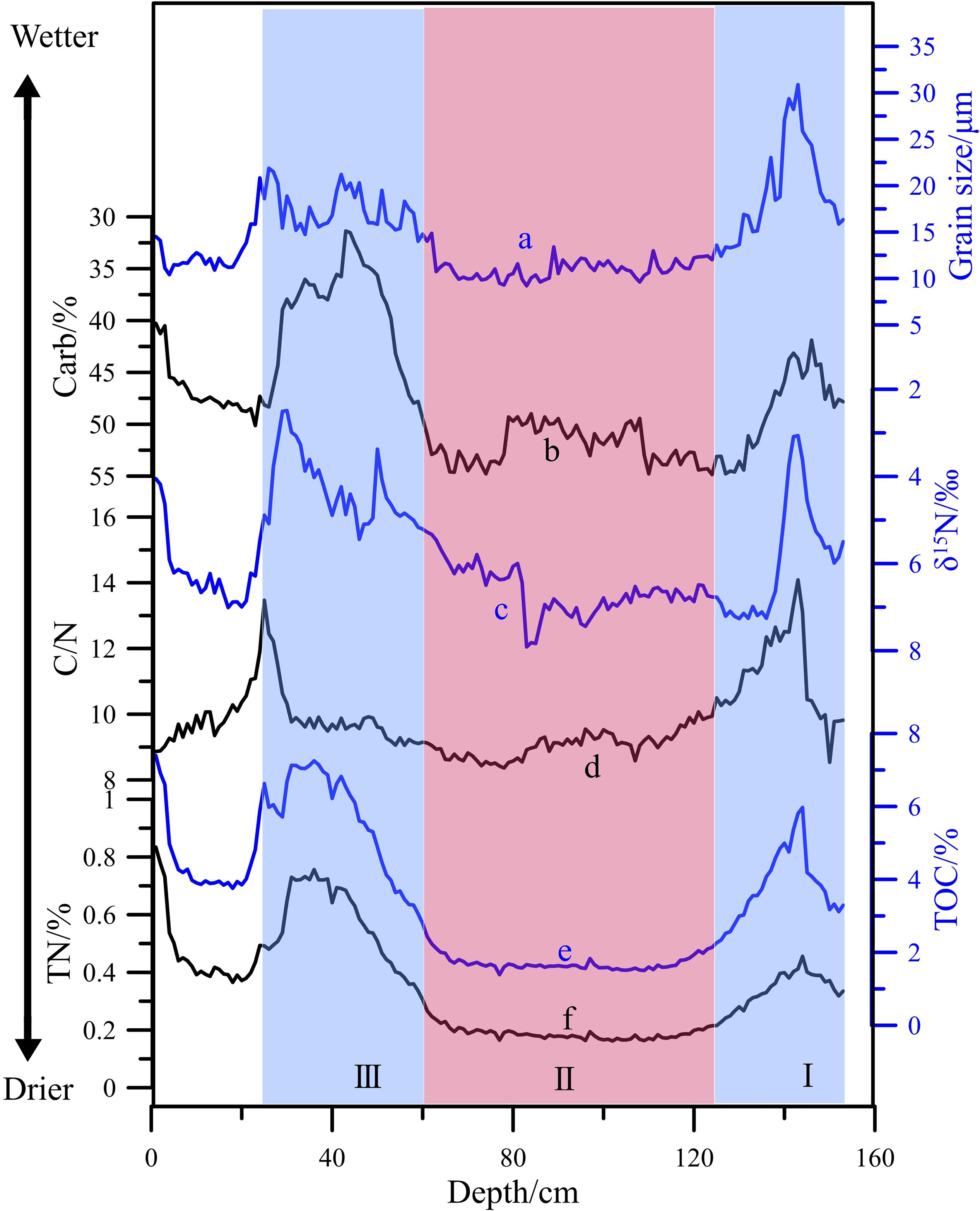
Figure 4. Proxy indices in core BL13-1-4 in Lake Bosten. (a) Grain size. (b) Carbonate content (Carb%). (c) Organic matter δ15N. (d) organic matter C/N ratio. (e,f) Total organic carbon content and nitrogen content.
Results
137Cs and 210Pb Dating
137Cs is an artificial nuclide with a half-life of 30.17 years. After 1945, especially in the 1950s, nuclear tests were widely carried out in the world, which led to the rapid increase of atmospheric 137Cs concentration. A rapid increase in 137Cs activity from natural background (zero) was detected in a large number of undisturbed or weakly disturbed lake sediment cores, and this point was assigned as a time marker of 1952 AD, which is particularly evident in lakes in northwest China (e.g., Yu et al., 2017; Lan et al., 2018; Yan et al., 2019). The atmospheric 137Cs fallout peak in the northern hemisphere occurred at 1963 AD (Robbins and Edgington, 1975), and this peak is widely used as a time marker of 1964 AD in lake sediments. Although the 1986 Chernobyl nuclear leak out may generate a 137Cs peak in lake sediment, this peak can be hardly detected in northwestern China (Xu et al., 2010; Yu et al., 2017; Yan et al., 2019).
The 137Cs curve of the core BL13-1-4 shows no clear peak, suggesting considerable disturbance of the sediments in modern times. Although this disturbance may lead to broadening of the peak, it does not affect the central position of the peak (Xu et al., 2010). The central position of the 137Cs peak of core BL13-1-4 occurred at ∼15 cm, and it can be recognized as the 1964 AD time marker. Sedimentation rate based on this 1964-year time marker is ∼0.29 cm/year. 137Cs activity increases rapidly from zero (the background) at 22 cm, indicating the beginning of atmospheric 137Cs fallout, corresponding to the 1952 AD time marker (Yu et al., 2017; Yan et al., 2019). Sedimentation rate based on this time marker (1952-year) is ∼0.83 cm/year. Such big difference in modern sedimentation rates suggests strong anthropogenic impacts during the recent/modern epoch, such as changes in land use in the catchment and/or roads/factories construction around the lake.
210Pb is a daughter of uranium series, with a half-life of 22.23 years. It has been widely used to determine the age of lake sediments of the past 150 years (e.g., Robbins and Edgington, 1975). The commonly used 210Pb dating methods include constant initial concentration mode (CIC) and constant rate of supply mode (CRS; Robbins and Edgington, 1975). The 210Pb ages of core BL13-1-4 derived from CRS model are similar to the 137Cs ages for the uppermost 10 cm, but deviate more and more as depth increases (Figure 2). The 210Pb ages derived from the CIC model are quite different with those derived from CRS model and those derived from the 137Cs time marker (Figure 2), and such big differences are most likely due to instable sedimentation rates. Because of such variable sedimentation rates, the 210Pb dating method can hardly generate reliable ages for the upper section of core BL13-1-4. Therefore, we do not use the 210Pb ages, but only use the 137Cs ages to assess the old carbon reservoir effect in this study (see below).
14C Dating
The 14C ages show linear trends in three intervals (20–50 cm, 60–120 cm, 120–153 cm; Figure 3). 137Cs age at 20 cm is −8 a BP, while the corresponding 14C age is 1025 a BP. We therefore got an old carbon reservoir age of 1033 years for the upper 20–50 cm. Since the 14C ages of 120–153 cm are linearly correlated to those of 20–50 cm (r2 = 0.99; Figure 3), we assume similar old carbon reservoir ages for both intervals. The 14C ages of 60–120 cm are bigger than those of the upper and lower intervals. After a correction of 2200 years, the corrected 14C ages of 60–120 cm fall on the regression line of 14C ages of the upper and bottom sections (Figure 3). The final age model of core BL13-1-4 is a combination of the 137Cs ages of the upper section (0–22 cm) and the corrected 14C ages of the lower section (23–153 cm). We acknowledge that the chronology of core BL13-1-4 may not be accurate enough regarding high-resolution records; however, it does not influence the topic involved in this study (see below).
Proxy Indices and the Climatic Significance
The carbonate content of core BL13-1-4 varies between 31.36% and 54.85%, with an average of 47.61% (Figure 4). Autogenic carbonate is generally precipitated faster and more in waters with higher ion concentration, and thus its content in lake sediment can be used to reflect the budget of water input and evaporation of a lake (Chen et al., 2006). In general, lower carb% in lake sediment reflects wetter hydroclimatic condition, while higher carb% reflects dryer condition (Chen et al., 2006).
The grain size of core BL13-1-4 varies between 9.21 and 30.87 μm, with an average of 14.38 μm. Sedimentary grain size has different climatic significance under different conditions and on different time scales. On long-term time scales, the grain size can reflect lake level variations (Liu et al., 2008a), while on short time scales, sediment grain size can record changes in surface runoff intensity, and then changes in precipitation (Xu et al., 2015). As Lake Bosten is an open lake, the decrease in sedimentary grain size in the late Holocene most likely indicates decrease in surface runoff intensity and precipitation; on the contrary, the increase in grain size indicates increase in regional runoff intensity and precipitation.
The δ15N of core BL13-1-4 ranges from 2.49% to 7.91%, with an average of 5.88%. δ15N of organic matter in lake sediments is closely related to primary productivity in lakes (Talbot and Johannessen, 1992; Xu et al., 2014, 2016b). During photosynthesis, the fractionation of nitrogen isotope is mainly controlled by nitrogen isotope value of dissolved inorganic nitrogen (DIN) in lake water. Phytoplankton in water tends to absorb 14N in photosynthesis, which leads to gradual enrichment of δ15N in lake water DIN pool (Talbot and Johannessen, 1992; Xu et al., 2014, 2016b). Increasing primary productivity in lake would therefore result in higher δ15N values in organic matter, and vice versa.
Total organic carbon content of core BL13-1-4 varies between 1.39 and 7.40%, with an average of 3.46. Sedimentary TOC is a sum of endogenous and exogenous organic carbon, and can reflect the biomass both in lake and in catchment (Meyers, 2003). The primary productivity in the lake is closely related to temperature variations and nutrients supply, while the amount of organic matter transported from the catchment to the lake is also closely related to the biomass and surface runoff intensity in the catchment (Cohen, 2003).
The atomic C/N ratio in core BL13-1-4 varies between 8.36 and 13.47, with an average value of 9.79. Because aquatic phytoplankton and algae contain higher protein content, their C/N ratio is relatively low and generally falls within the range of 4–10. In contrast, terrestrial plants contain more lignin and cellulose, so their C/N values are higher (generally higher than 20). As a result, C/N ratio can reflect the relative contribution of different sources of organic matter in lake sediments; decrease in sedimentary C/N ratio suggests high fraction of organic matter produced in lake, and vice versa (Meyers, 2003).
Late Holocene Climatic Changes at Lake Bosten
The sedimentary proxy indices in core BL13-1-4 in Lake Bosten indicate significant climate change during the late Holocene, and it can be broadly divided into four intervals:
Interval I (126–153 cm), corresponding to 1800–2200 BP: the average sedimentary grain size was relatively big, indicating strong runoff intensities; the carb% was relatively low, both of which suggest higher precipitation during this period. C/N ratio increases, indicating increase in terrestrial organic matter input, which also indicates enhanced runoff transport in the catchment. The coarse sand during 138–144 cm (about 2100–2000 BP) indicates that precipitation may be higher during this period. The sediment δ15N is relatively low, indicating relatively weak lake productivity during this period.
Interval II (60–125 cm), the carb% was at a higher level in the corresponding period of 650–1800 BP, indicating that the lake water salinity was higher and chemical deposition rate was faster, suggesting that the water influx to the lake was less than the evaporation. The average sedimentary grain size decreased obviously compared with that of the previous period, indicating that the runoff intensity was weakened and the surface runoff transport capacity was decreased, suggesting that the precipitation may decrease in that period. The C/N ratio was relatively small, suggesting relatively low contribution of terrestrial organic matter in this period. The high δ15N value in this period indicates that the relative contribution of lake productivity is increasing. All these suggest that the biomass in the catchment was relatively low during this period due to less precipitation.
Interval III (28–59 cm), corresponding to 650–100 BP: the carb% firstly decreased and then increased, and the averaged value was relatively low. Sedimentary grain size shows a first increasing and then slightly decreasing trend, and the averaged grain size of this section was coarser than that of the previous section (60–125 cm; corresponding to 650–1800 BP). A sandy layer (25–40 cm) was found in this section. The TOC, TN, and the C/N ratios are higher, suggesting higher biomass in the catchment and larger contribution of terrestrial organic matter to the bulk sedimentary organic matter. The averaged δ15N is lower, suggesting low primary productivity of the lake. All these suggest that the catchment was wetter in general, but experienced obvious dry and wet transitions during 650–100 BP.
The surface (1–27 cm) sedimentation rates are strongly influenced by human activities, which can be seen from two aspects. (1) From the 210Pb-137Cs chronology of surface sediments, it can be seen that the deposition rate has changed dramatically in the modern times as mentioned above. (2) The correlation between different proxy indices in this interval is weak, implying strong human activities during the modern epoch (strong human activity disturbs the relationship between proxy indices and climatic changes under natural background).
Discussion
Old Carbon Reservoir
The basis of the 14C dating is that the initial 14C concentration of the living sample is equal to the atmospheric 14C concentration of the same period (Deevey et al., 1954). However, factors influencing the old carbon reservoir ages are diverse, including (1) recharge of surface/underground water that contains “old carbon” (Deevey et al., 1954; Hendy and Hall, 2006). When the surface/underground water flows through the limestone area, it erodes the limestone or soils in the catchment, and brings “old carbon” into the lake (Olsson, 2009). (2) The 14C exchange between lake water and atmospheric CO2. Due to the slow convection rate of some lakes and the lake water stratification, the 14C concentration of lake water is lower than that of atmospheric CO2 of the same period, and the calculated 14C ages of materials produced in lake are older than the corresponding real ages. For example, some aquatic plants in the lake use CO2 in atmosphere and DIC in water for photosynthesis, which will result in parts of the “old carbon” used in photosynthesis (Olsson, 2009). (3) the pH value, salinity, and nutrient concentration of lake water may affect the primary productivity of lake, the 14C exchange rate (between aquatic and atmospheric CO2), and the old carbon reservoir age (Fontes et al., 1996; Shen et al., 2005). (4) The organic matter in lake sediments may be a mixture of terrestrial plants and aquatic plants (phytoplankton/algae), and the relative fraction of these two sources will change with the lake levels and primary productivity over time, which may also affect the age of old carbon reservoir.
Old Carbon Correction
Methods used to correct old carbon reservoir ages generally include three categories: linear regression, stratigraphic correlation, and geochemical modeling.
Linear Regression
Linear regression method has been widely used to estimate the old carbon reservoir age (e.g., Fontes et al., 1996; Sheng et al., 2015; Zhang et al., 2016). Ages of the surface sediments are generally considered to be the time when they were sampled, then the intercept between the linear regression line and age coordinate (at the water-sediment interface) is regarded as the carbon reservoir age. For example, Shen et al. (2005) used linear regression method to estimate the carbon reservoir age in Lake Qinghai, and Fontes et al. (1996) and Hou et al. (2017) used linear regression method to estimate the carbon reservoir ages in Lake Bangongco. By comparing the 14C ages of the bulk organic matter with those of plant residue and inorganic carbonates in sediment core of Lake Zigê Tangco, and by linear regression, Wu et al. (2010) got the carbon reservoir age of 2010 years for this lake. However, the climate, hydrology, and deposition rate should be relatively stable when using linear regression method to evaluate the old carbon effect. If the environment changes largely in different periods, it is better to use piecewise linear regression. For example, by separating linear regression of different layers, Zhou et al. (2014) got old carbon reservoir ages of 135 years, 1143 years, and 2523 years for different sections of a core collected in Lake Qinghai.
Stratigraphic Correlation
Lake sediments of the last hundred years can be determined by 210Pb and 137Cs dating methods; by comparison with the 14C ages of the surface sediment and 210Pb and 137Cs ages of the same depth, the old carbon reservoir ages can be estimated. By comparison between the 14C ages of terrestrial plant residues (no old carbon effect), those of the bulk sedimentary organic matter, aquatic plants, and snail shells in the lake sediments of the same layers, the 14C reservoir effect can also be estimated. The old carbon reservoir ages can also be evaluated by comparing the 14C ages of the same layer with the luminescent ages and varve-counting ages (e.g., Hall and Henderson, 2001; Long et al., 2011). For example, by comparing 14C ages of different samples collected from the same layers in sediment core in Xingyun Lake, Zhou et al. (2015) estimated that the carbon reservoir ages of this lake changed from 1150 to 2200 years in the past 8500 years. By comparing the 14C age of organic matter and inorganic carbonate in the surface layer, and 14C age of dissolved organic matter in lake water in Lake Qinghai, Henderson et al. (2010) got an old carbon reservoir age of 658 years.
Modeling of the Old Carbon Reservoir Age
If different 14C sources of a lake can be identified and quantified, then old carbon reservoir age can be estimated by geochemical modeling. For example, Yu et al. (2007) used geochemical modeling to study the old carbon reservoir ages in Lake Qinghai, and they got an old carbon reservoir age of ∼1500 years in this lake. Watanabe et al. (2013) calculated the relative contribution of organic matter produced in lake and those in lakeshore wetland at Lake Pumoyum Co, and estimated the old carbon reservoir age. However, as lakes may have experienced considerable environmental changes in the past, the uncertainty of the parameters limits the application of modeling to evaluate the carbon reservoir ages of the past times. It is therefore generally limited to determine the old carbon effect of modern lakes.
Changing Old Carbon Reservoir Effect in Lake Bosten
The different linear correlations of the 14C ages (as shown in Figure 3) and the variation patterns of the proxy indices (Figure 4) imply that the carbon reservoir effect of Lake Bosten was relatively smaller in the humid interval I (59–28 cm, 650–100 BP) and interval III (153–126 cm, 2200–1800 BP), but was significantly bigger (about 1170 years) during the dry interval II (125–60 cm, 1800–650 BP). To verify the changing carbon reservoir effect and the reliability of the age model for core BL13-1-4 in Lake Bosten, we compared the age model of this study with those published by some previous work. The results show that parts of previous 14C ages (e.g., Zhang et al., 2004; Wünnemann et al., 2006) have a good correlation with the age model obtained in this study (Figure 3). In particular, the 14C ages based on plant residues of some previous work fall on the regression line of this paper, suggesting that our age model (based on separated correction of different old carbon ages in different periods) is rational.
Comparison of Proxy Indices
To further verify the age model in this paper, we compared the proxy indices developed in this study with those of some previous work. As shown in Figure 5, the time series of proxy index based on the abovementioned age model in this study show good synchroneity to those of some other previous work. For example, the grain size, δ15N, TOC, and carb% of the core BL13-1-4 show that there is a transition from wet to dry in Lake Bosten area around 2100 BP, while some other records, like the grain size and C/N ratio of Lake Sayram (Lan et al., 2019), North Atlantic NAO index (Olsen et al., 2012), etc., show similar transitions from wet to dry contemporaneously (Figure 5). Another example is that during the period of about 650–500 BP, the grain size, δ15N, TOC, and carb% of Lake Bosten recorded a transition from dry to wet, which is similar to the climatic transitions inferred from grain size and C/N ratio in Lake Sayram (Lan et al., 2019), the NAO index (Olsen et al., 2012), and the Alpine flood records (Wirth et al., 2013). It is interesting to note that such an obvious hydroclimatic transition from dry to wet seems to have an even wider extension, e.g., in the extended Indian summer monsoon areas (Xu et al., 2016a, 2019a). However, the nature of this hydroclimatic transition needs further study and is outside the scope of this work.
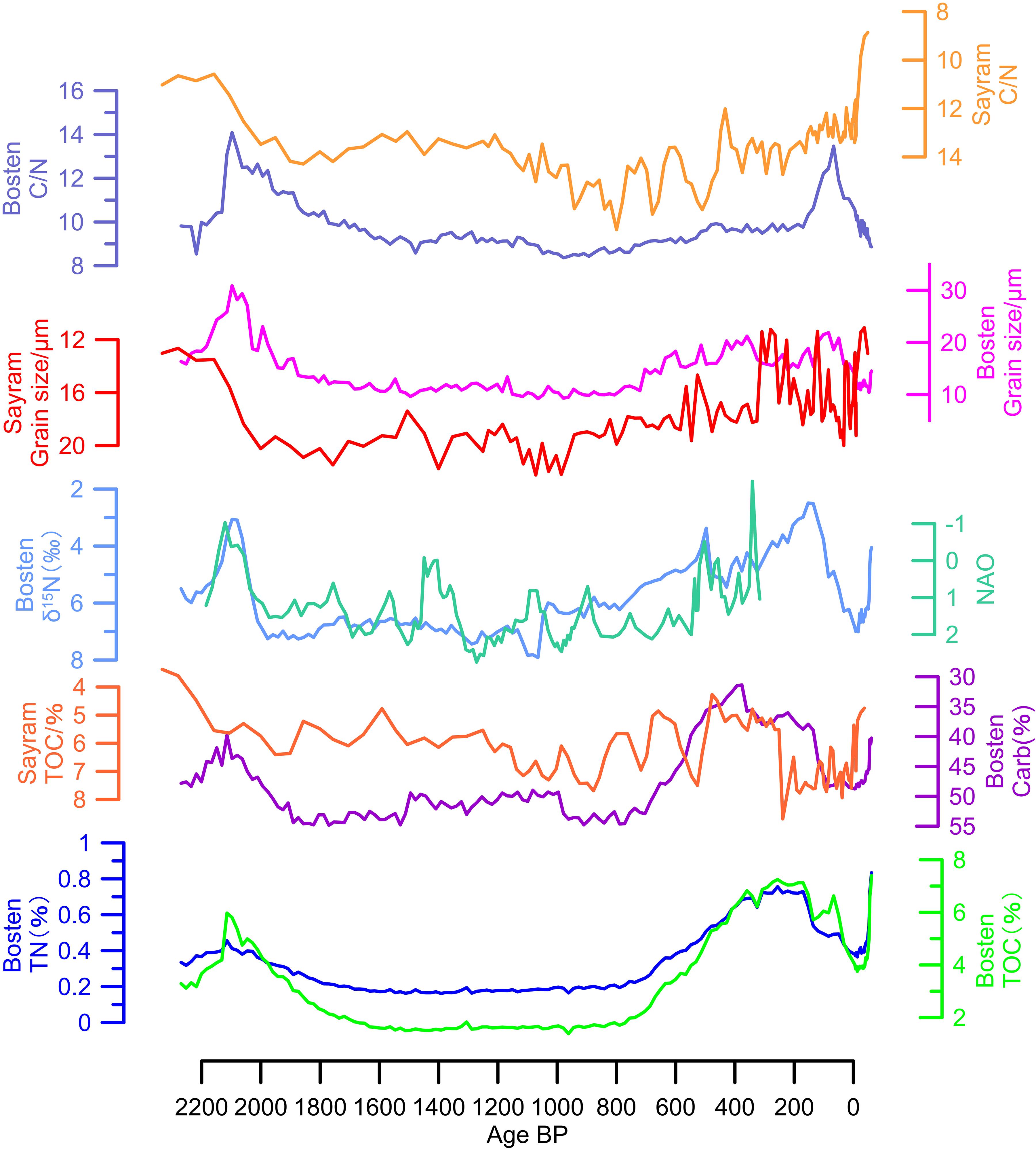
Figure 5. Comparison between proxy indices in core BL13-1-4 in Lake Bosten (this study) and those from some other work. The grain size, C/N, and carbonate content were from Lan et al. (2019) and NAO index is from Olsen et al. (2012).
However, some differences do exist between the climate changes reconstructed by different studies, and these may be due to (1) certain errors or methodological differences in different chronologies, (2) differences in the resolution of different indicators, and (3) different responses of proxy indices to climate events or atmospheric circulation in different regions. Neglecting these differences, the results of this study are in good agreement with those obtained by previous studies. Therefore, it is rational to evaluate and correct the different old carbon effects in different periods of Lake Bosten.
Possible Causes of the Changing Old Carbon Reservoir Ages in Lake Bosten
The old carbon effect in core BL13-1-4 of Lake Bosten is significantly higher in interval II than those in other intervals, which may be related to the variable proportion of organic matter produced in lake compared to those of the bulk sediment (due to the different climatic conditions). TOC values are relatively low during 1800–650 BP (60–125 cm), suggesting less organic matter transported from the catchment to the lake during this period. The higher lake water salinity would lead to faster chemical deposition rate, and the high sedimentary carb% (as high as 55%) strongly supports the above inference.
The dry hydroclimatic condition during this interval may lead to relatively low lake levels, consistent with the switch from lacustrine sediment to peaty mud during the interval of ∼1000–500 BP in a core collected near the western shoreline in this lake (Mischke and Wünnemann, 2006). The decrease of lake water level and increase of water salinity may have slowed down the 14C exchange between dissolved CO2 and atmospheric CO2, resulting in increase of old carbon age of the DIC pool. At the same time, the decrease of lake level during this period favored nutrient transport to the sampling site, resulting in increasing primary productivity surround the sampling site. The higher δ15N values during this period also suggest that the primary productivity around the sampling site was strong. Due to the decrease of organic matter input from the catchment to lake, the relative contribution of organic matter produced in lakes should increase during this period, which can be strongly supported by the significant decreases in sedimentary C/N ratios during this period.
According to the above analysis, the contribution of endogenous organic matter increased during the relatively dry period at Lake Bosten. Due to partial utilization of DIC to synthesize organic matter, and due to the larger reservoir ages in dry period, the bulk organic matter 14C age of lake sediments is older. In contrast, the relative lower contribution of endogenous organic matter in wet periods (100–650 BP and 1800–2200 BP) resulted in a smaller old carbon effect in lake sediment.
Conclusion
We focused on influence of different hydroclimatic conditions of the old carbon reservoir effect in Lake Bosten. By comparison between 137Cs/210Pb dating results and radiocarbon ages of the surface sediments and by comparison between multi-proxy indices developed in this study and proxy indices from some previous work, we found that the old carbon effect existed and varied over the past ∼2300 years at Lake Bosten. The old carbon reservoir ages of dry periods are likely to be bigger than those of wet periods. We contend that the changing old carbon reservoir ages could be ascribed to different degrees of 14C exchange between lake water CO2 and atmospheric CO2 under different hydroclimatic conditions and to the variable proportion between organic matter produced in the lake and those in the catchment.
As the old carbon effect varies on different temporal and spatial scales for some specific lakes, it would lead to large uncertainty by simply correcting the old carbon effect using one unique old carbon reservoir age. It is necessary to correct parts of the previous 14C ages (especially those over the arid zone) by a proper method. The method of this study, i.e., by comparing the 14C ages with other robust dating results and by comprehensive comparison of multi-proxy indices, may serve as a reference method to get reliable age models in paleolimnology.
Data Availability Statement
All datasets generated for this study are included in the article/supplementary material.
Author Contributions
HX designed the research. HX, KZ, JL, DY, ES, KY, JZ, and YS performed the research. KZ, HX, JL, PF, and SX analyzed the data. KZ and HX wrote the manuscript.
Funding
This study was supported by the National Natural Science Foundation of China (Nos. 41672169 and 41761144070).
Conflict of Interest
The authors declare that the research was conducted in the absence of any commercial or financial relationships that could be construed as a potential conflict of interest.
Acknowledgments
We thank the reviewers for their valuable comments and suggestions.
References
Aizen, V. B., Aizen, E. M., Joswiak, D. R., Fujita, K., Takeuchi, N., and Nikitin, S. A. (2006). Climatic and atmospheric circulation pattern variability from ice-core isotope/geochemistry records (Altai, Tien Shan and Tibet). Ann. Glaciol. 43, 43–49.
Bennike, O. (2000). Palaeoecological studies of Holocene lake sediments from west Greenland. Palaeogeogr. Palaeoclimatol. Palaeoecol. 155, 285–304. doi: 10.1016/s0031-0182(99)00121-2
Chen, F., Huang, X., Zhang, J., Holmes, J. A., and Chen, J. (2006). Humid Little Ice Age in arid central Asia documented by Bosten Lake, Xinjiang, China. Sci. China Ser. D Earth Sci. 49, 1280–1290. doi: 10.1007/s11430-006-2027-4
Chen, F. H., Yu, Z. C., Yang, M. L., Ito, E., Wang, S. M., Madsen, D. B., et al. (2008). Holocene moisture evolution in arid central Asia and its out-of-phase relationship with Asian monsoon history. Quat. Sci. Rev. 27, 351–364. doi: 10.1016/j.quascirev.2007.10.017
ChongYi, E., Sun, Y. J., Liu, X. J., Hou, G. L., Lv, S. C., Yuan, J., et al. (2018). A comparative study of radiocarbon dating on terrestrial organisms and fish from Qinghai Lake in the northeastern Tibetan Plateau, China. Holocene 28, 1712–1719. doi: 10.1177/0959683618788671
Cohen, A. S. (2003). Paleolimnology: The History and Evolution of Lake Systems. Oxford: Oxford University Press.
Deevey, E. S., Gross, M. S., Hutchinson, G. E., and Kraybill, H. L. (1954). The Natural C Contents of Materials from Hard-Water Lakes. Proc. Natl. Acad. Sci. U.S.A. 40, 285–288. doi: 10.1073/pnas.40.5.285
Fontes, J. C., Gasse, F., and Gibert, E. (1996). Holocene environmental changes in Lake Bangong basin (Western Tibet). Part 1: Chronology and stable isotopes of carbonates of a Holocene lacustrine core. Palaeogeogr. Palaeoclimatol. Palaeoecol. 120, 25–47. doi: 10.1016/0031-0182(95)00032-1
Hall, B. L., and Henderson, G. M. (2001). Use of uranium–thorium dating to determine past 14C reservoir effects in lakes: examples from Antarctica. Earth Planet. Sci. Lett. 193, 565–577. doi: 10.1016/s0012-821x(01)00524-6
Henderson, A. C. G., Holmes, J. A., and Leng, M. J. (2010). Late Holocene isotope hydrology of Lake Qinghai, NE Tibetan Plateau: effective moisture variability and atmospheric circulation changes. Quat. Sci. Rev. 29, 2215–2223. doi: 10.1016/j.quascirev.2010.05.019
Hendy, C. H., and Hall, B. L. (2006). The radiocarbon reservoir effect in proglacial lakes: Examples from Antarctica. Earth Planet. Sci. Lett. 241, 413–421. doi: 10.1016/j.epsl.2005.11.045
Herzschuh, U., Winter, K., Wünnemann, B., and Li, S. J. (2006). A general cooling trend on the central Tibetan Plateau throughout the Holocene recorded by the Lake Zigetang pollen spectra. Quat. Int. 154, 113–121. doi: 10.1016/j.quaint.2006.02.005
Hou, J., D’andrea, W. J., and Liu, Z. (2012). The influence of 14C reservoir age on interpretation of paleolimnological records from the Tibetan Plateau. Quat. Sci. Rev. 48, 67–79. doi: 10.1016/j.quascirev.2012.06.008
Hou, J. Z., D’andrea, W. J., Wang, M. D., He, Y., and Liang, J. (2017). Influence of the Indian monsoon and the subtropical jet on climate change on the Tibetan Plateau since the late Pleistocene. Quat. Sci. Rev. 163, 84–94. doi: 10.1016/j.quascirev.2017.03.013
Hu, R. J. (2004). Physical Geography of the Tianshan Mountains in China. Beijing: China Environmental Science Press.
Huang, X. Z., Chen, F. H., Fan, Y. X., and Yang, M. L. (2009). Dry late-glacial and early Holocene climate in arid central Asia indicated by lithological and palynological evidence from Bosten Lake. China. Quat. Int. 194, 19–27. doi: 10.1016/j.quaint.2007.10.002
Hughen, K., Lehman, S., Southon, J., Overpeck, J., Marchal, O., Herring, C., et al. (2004). 14C activity and global carbon cycle changes over the past 50,000 years. Science 303, 202–207. doi: 10.1126/science.1090300
Jiu, X., Liu, H., Tu, Q., Zhang, Z., and Zhu, H. (eds) (1990). “Eutrophication of lakes in China” in Proceeding of the 4th International Conference on the Conservation and Management of lakes, Hangzhou’90, Hangzhou.
Lan, J. H., Xu, H., Sheng, E. G., Yu, K. K., Wu, H. X., Zhou, K. G., et al. (2018). Climate changes reconstructed from a glacial lake in High Central Asia over the past two millennia. Quat. Int. 487, 43–53. doi: 10.1016/j.quaint.2017.10.035
Lan, J. H., Xu, H., Yu, K. K., Sheng, E. G., Zhou, K. E., Wang, T. L., et al. (2019). Late Holocene hydroclimatic variations and possible forcing mechanisms over the eastern Central Asia. Sci. China Earth Sci. 62, 1288–1301. doi: 10.1007/s11430-018-9240-x
Liu, X., Dong, H., Yang, X., Herzschuh, U., Zhang, E., Stuut, J. B. W., et al. (2009). Late Holocene forcing of the Asian winter and summer monsoon as evidenced by proxy records from the northern Qinghai–Tibetan Plateau. Earth Planet. Sci. Lett. 280, 276–284. doi: 10.1016/j.epsl.2009.01.041
Liu, X., Herzschuh, U., Shen, J., Jiang, Q. F., and Xiao, X. Y. (2008a). Holocene environmental and climatic changes inferred from Wulungu Lake in northern Xinjiang. China. Quat. Res. 70, 412–425. doi: 10.1016/j.yqres.2008.06.005
Liu, X., Dong, H., Rech, J. A., Matsumoto, R., Bo, Y., and Wang, Y. (2008b). Evolution of Chaka Salt Lake in NW China in response to climatic change during the Latest Pleistocene–Holocene. Quat. Sci. Rev. 27, 867–879. doi: 10.1016/j.quascirev.2007.12.006
Long, H., Lai, Z. P., Wang, N. A., and Zhang, J. R. (2011). A combined luminescence and radiocarbon dating study of Holocene lacustrine sediments from arid northern China. Quat. Geochronol. 6, 1–9. doi: 10.1016/j.quageo.2010.06.001
Meyers, P. A. (2003). Applications of organic geochemistry to paleolimnological reconstructions: a summary of examples from the Laurentian Great Lakes. Org. Geochem. 34, 261–289. doi: 10.1016/s0146-6380(02)00168-7
Mischke, S., Weynell, M., Zhang, C., and Wiechert, U. (2013). Spatial variability of 14C reservoir effects in Tibetan Plateau lakes. Quat. Int. 31, 147–155. doi: 10.1016/j.quaint.2013.01.030
Mischke, S., and Wünnemann, B. (2006). The Holocene salinity history of Bosten Lake (Xinjiang, China) inferred from ostracod species assemblages and shell chemistry: Possible palaeoclimatic implications. Quat. Int. 154, 100–112. doi: 10.1016/j.quaint.2006.02.014
Olsen, J., Anderson, N. J., and Knudsen, M. F. (2012). Variability of the North Atlantic Oscillation over the past 5,200 years. Nat. Geosci. 5, 808–812. doi: 10.1038/ngeo1589
Olsson, I. U. (2009). Radiocarbon Dating History: Early Days, Questions, and Problems Met. Radiocarbon 51, 1–43. doi: 10.1017/s0033822200033695
Reimer, P. J., Bard, E., Bayliss, A., Beck, J. W., Blackwell, P. G., Ramsey, C. B., et al. (2009). IntCal13 and Marine13 Radiocarbon Age Calibration Curves 0–50,000 Years cal BP. Radiocarbon 55, 1869–1887. doi: 10.1016/j.dib.2018.10.040
Robbins, J. A., and Edgington, D. N. (1975). Determination of recent sedimentation rates in Lake Michigan using Pb-210 and Cs-137. Geochim. Cosmochim. Acta 39, 285–304. doi: 10.1016/0016-7037(75)90198-2
Shen, J., Liu, X. Q., Wang, S. M., and Matsumoto, R. (2005). Palaeoclimatic changes in the Qinghai Lake area during the last 18,000 years. Quat. Int. 136, 131–140. doi: 10.1016/j.quaint.2004.11.014
Sheng, E. G., Yu, K. K., Xu, H., Lan, J. H., Liu, B., and Che, S. (2015). Late Holocene Indian summer monsoon precipitation history at Lake Lugu, northwestern Yunnan Province, southwestern China. Palaeogeogr. Palaeoclimatol. Palaeoecol. 438, 24–33. doi: 10.1016/j.palaeo.2015.07.026
Snyder, J. A., Miller, G. H., Werner, A., Jull, A. J. T., and Stafford, T. W. (1994). AMS-radiocarbon dating of organic-poor lake sediment, an example from Linnévatnet, Spitsbergen, Svalbard. Holocene 4, 413–421. doi: 10.1177/095968369400400409
Stuiver, M., Reimer, P. J., Bard, E., Beck, J. W., Burr, G. S., Hughen, K. A., et al. (1998). INTCAL98 radiocarbon age calibration, 24,000-0 cal BP. Radiocarbon 40, 1041–1083. doi: 10.1017/s0033822200019123
Talbot, M. R., and Johannessen, T. (1992). A high resolution palaeoclimatic record for the last 27,500 years in tropical West Africa from the carbon and nitrogen isotopic composition of lacustrine organic matter. Earth Planet. Sci. Lett. 110, 23–37. doi: 10.1016/0012-821x(92)90036-u
Watanabe, T., Nakamura, T., Matsunaka, T., Nara, F. W., Zhu, L., Wang, J., et al. (2013). A chronology of the PY608E–PC sediment core (Lake Pumoyum Co, southern Tibetan Plateau) based on radiocarbon dating of total organic carbon. Nucl. Instrum. Meth. 294, 520–523. doi: 10.1016/j.nimb.2012.07.015
Wirth, S. B., Glur, L., Gilli, A., and Anselmetti, F. S. (2013). Holocene flood frequency across the Central Alps - solar forcing and evidence for variations in North Atlantic atmospheric circulation. Quat. Sci. Rev. 80, 112–128. doi: 10.1016/j.quascirev.2013.09.002
Wu, Y., Li, S., Lücke, A., Wünnemann, B., Zhou, L., Reimer, P., et al. (2010). Lacustrine radiocarbon reservoir ages in Co Ngoin and Zigê Tangco, central Tibetan Plateau. Quat. Int. 212, 21–25. doi: 10.1016/j.quaint.2008.12.009
Wünnemann, B., Mischke, S., and Chen, F. H. (2006). A Holocene sedimentary record from Bosten Lake, China. Palaeogeogr. Palaeoclimatol. Palaeoecol. 234, 223–238. doi: 10.1016/j.palaeo.2005.10.016
Xiao, M., Wu, F., Liao, H., Li, W., Lee, X., and Huang, R. (2010). Characteristics and distribution of low molecular weight organic acids in the sediment porewaters in Bosten Lake, China. J. Environ. Sci. 22, 328–337. doi: 10.1016/s1001-0742(09)60112-1
Xu, H., Lan, J. H., Sheng, E. G., Liu, B., Yu, K. K., Ye, Y. D., et al. (2016a). Hydroclimatic contrasts over Asian monsoon areas and linkages to tropical Pacific SSTs. Sci. Rep. 6:33177. doi: 10.1038/srep33177
Xu, H., Yu, K., Lan, J., Sheng, E., Liu, B., Ye, Y., et al. (2016b). Different responses of sedimentary δ15N to climatic changes and anthropogenic impacts in lakes across the Eastern margin of the Tibetan Plateau. J. Asian Earth Sci. 123, 111–118. doi: 10.1016/j.jseaes.2016.03.024
Xu, H., Liu, X. Y., An, Z. S., Hou, Z. H., Dong, J. B., and Liu, B. (2010). Spatial pattern of modern sedimentation rate of Qinghai Lake and a preliminary estimate of the sediment flux. Chin. Sci. Bull. 55, 621–627. doi: 10.1007/s11434-009-0580-x
Xu, H., Sheng, E. G., Lan, J. H., Liu, B., Yu, K. K., and Che, S. (2014). Decadal/multi-decadal temperature discrepancies along the eastern margin of the Tibetan Plateau. Quat. Sci. Rev. 89, 85–93. doi: 10.1016/j.quascirev.2014.02.011
Xu, H., Song, Y., Goldsmith, Y., and Lang, Y. (2019a). Meridional ITCZ shifts modulate tropical/subtropical Asian monsoon rainfall. Sci. Bull. 64, 1737–1739. doi: 10.1016/j.scib.2019.09.025
Xu, H., Zhou, K., Lan, J., Zhou, X., and Zhang, G. (2019b). Arid central Asia saw mid-Holocene drought. Geology 47, 255–258. doi: 10.1130/g45686.1
Xu, H., Zhou, X. Y., Lan, J. H., Liu, B., Sheng, E. G., Yu, K. K., et al. (2015). Late Holocene Indian summer monsoon variations recorded at Lake Erhai, Southwestern China. Quat. Res. 83, 307–314. doi: 10.1016/j.yqres.2014.12.004
Yan, D. N., Xu, H., Lan, J. H., Zhou, K. G., Ye, Y. D., Zhang, J. X., et al. (2019). Solar activity and the westerlies dominate decadal hydroclimatic changes over arid Central Asia. Glob. Planet. Change 173, 53–60. doi: 10.1016/j.gloplacha.2018.12.006
Yu, K. K., Xu, H., Lan, J. H., Sheng, E. G., Liu, B., Wu, H. X., et al. (2017). Climate change and soil erosion in a small alpine lake basin on the Loess Plateau, China. Earth Surf. Proc. Land. 42, 1238–1247. doi: 10.1002/esp.4071
Yu, S. Y., Shen, J., and Colman, S. M. (2007). Modeling the radiocarbon reservoir effect in lacustrine systems. Radiocarbon 49, 1241–1254. doi: 10.1017/s0033822200043150
Zhang, C., Cao, J., Lei, Y., and Shang, H. (2004). The Chronological Characteristics of Bosten Lake Holocene Sediment Environment in Xinjiang, China. Acta Sediment. Sin. 22, 494–499.
Zhang, J. W., Ma, X. Y., Qiang, M. R., Huang, X. Z., Li, S., Guo, X. Y., et al. (2016). Developing inorganic carbon-based radiocarbon chronologies for Holocene lake sediments in arid NW China. Quat. Sci. Rev. 144, 66–82. doi: 10.1016/j.quascirev.2016.05.034
Zhou, A., He, Y., Wu, D., Zhang, X., Zhang, C., Liu, Z., et al. (2015). Changes in the radiocarbon reservoir age in Lake Xingyun, Southwestern China during the Holocene. PLoS One 10:e0121532. doi: 10.1371/journal.pone.0121532
Keywords: 14C reservoir age, hydroclimate change, late Holocene, Lake Bosten, arid central Asia
Citation: Zhou K, Xu H, Lan J, Yan D, Sheng E, Yu K, Song Y, Zhang J, Fu P and Xu S (2020) Variable Late Holocene 14C Reservoir Ages in Lake Bosten, Northwestern China. Front. Earth Sci. 7:328. doi: 10.3389/feart.2019.00328
Received: 24 October 2019; Accepted: 27 November 2019;
Published: 22 January 2020.
Edited by:
Michaël Hermoso, Université du Littoral Côte d’Opale, FranceReviewed by:
Philip Alan Meyers, University of Michigan, United StatesLi Wu, Anhui Normal University, China
Copyright © 2020 Zhou, Xu, Lan, Yan, Sheng, Yu, Song, Zhang, Fu and Xu. This is an open-access article distributed under the terms of the Creative Commons Attribution License (CC BY). The use, distribution or reproduction in other forums is permitted, provided the original author(s) and the copyright owner(s) are credited and that the original publication in this journal is cited, in accordance with accepted academic practice. No use, distribution or reproduction is permitted which does not comply with these terms.
*Correspondence: Hai Xu, xuhai@tju.edu.cn