Novel Bathymetry of Lake Afdera Reveals Fault Structures and Volcano-Tectonic Features of an Incipient Transform Zone (Afar, Ethiopia)
- 1Department of Geosciences, University of Fribourg, Fribourg, Switzerland
- 2School of Agricultural, Earth and Environmental Sciences, University of Kwazulu-Natal, Westville Campus, Durban, South Africa
- 3School of Earth Sciences, Addis Ababa University, Addis Ababa, Ethiopia
Lake Afdera is a hypersaline endorheic lake situated at 112 m below sea-level in the Danakil Depression. The Danakil Depression is located in the northern part of the Ethiopian Afar and features an advanced stage of continental rifting. The remoteness and inhospitable environment explain the limited scientific research and knowledge about this lake. Bathymetric data were acquired during 2 weeks expeditions in January/February 2016 and 2017 using an easily deployable echosounder system mounted on an inflatable motorized boat. This study presents the first complete bathymetric map of the lake Afdera. Bathymetric results show that the lake has an average depth of 20.9 m and a total volume of 2.4 km3. The maximum measured depth is 80 m, making Lake Afdera the deepest known lake in Afar and the lowest elevation of the Danakil Depression. Comparison with historical reports shows that the lake level did not fluctuate significantly during the last 50 years. Two distinct tectonic basins to the north and the south are recognized. Faults of different orientations control the morphology of the northern basin. In contrast, the southern basin is affected by volcano-tectonic processes, unveiling a large submerged caldera. Comparison between the orientation of faults throughout the lake with the regional fault pattern indicates that the lake is part of two transfer zones: the major Alayta–Afdera Transfer Zone and the smaller Erta Ale–Tat’Ali Transfer Zone. The interaction between these Transfer Zones and the rift axis forms the equivalent of a developing nodal basin which explains the lake’s position as the deepest point of the depression. This study provides evidence for the development of an incipient transform fault on the floor of the Afar depression.
Introduction
Lake Afdera is a hypersaline endorheic lake (Martini, 1969) located in the southern part of the Danakil Depression, in the Afar region, in northern Ethiopia (Figure 1). Afdera is a name initially given by Afari people to the largest nearby active volcano (“Afe” meaning “mouth” and “Deda” standing for “large,” or the large mouth of the volcano”). The lake is also sometimes called “Lake Afrera,” “Lake Giulietti” or “Egogi Bad.” Sedimentological (Gasse, 1974; Gasse and Street, 1978) and ecological data (Chiozzi et al., 2018) confirm that the lake has been persistent for at least the last 10 ka, although the lake level has been up to 50 m higher than today during the Mid-Holocene (Gasse, 1974; Schaegis et al., 2017).
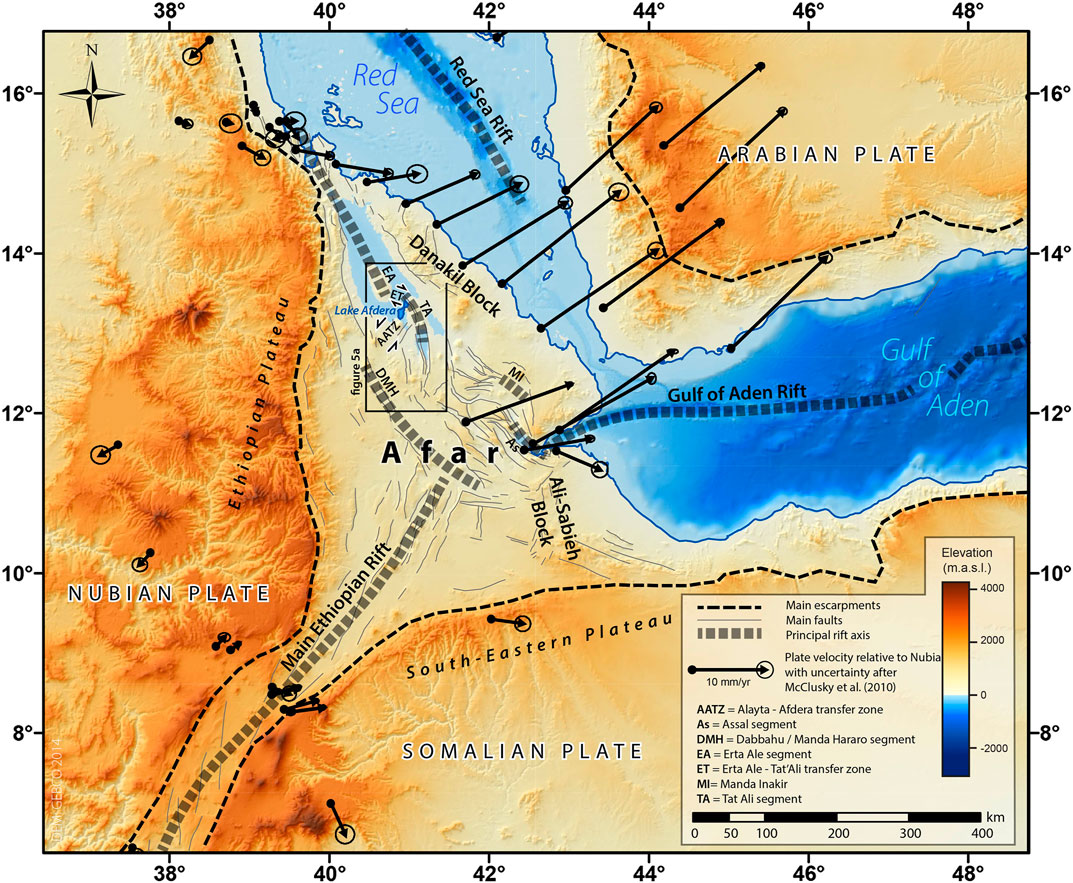
FIGURE 1. Elevation map of the Afar triple junction (East Africa), defined by the divergence of the Arabian, Nubian and Somalian plates as well as the propagation of the Rea Sea, Gulf of Aden and Main Ethiopian Rifts. Black arrows show direction and velocity of the different plates (McClusky et al., 2010), relative to the Nubian plate. The black rectangle represents Figure 5A.
First Explorers
Western exploration of the “Danakil Country” and the Afdera region was initially launched in 1867 by Munzinger, the first explorer to describe the depression from a geomorphological perspective (Munzinger, 1869). The expeditions of 1875, 1881 and 1884 led by Munzinger, Giulietti and Bianchi were unsuccessful as none of the explorers survived (Nesbitt, 1935). The objective was to cross the Danakil desert from the Ethiopian plateau—“Abyssinia”—to the Red Sea. The next generation of explorers was more successful. Thus, Vinassa De Regny in 1919, Nesbitt in 1928 and Franchetti in 1929 all managed to cross the Danakil desert and collect geographical and geological data (Lupi, 2009). On January 26th, 1920, Vinassa de Regny was the first explorer to see the lake, which he named “Afrera”—most likely a misspelling from the volcano Afdera in its surroundings (Vinassa de Regny, 1931). Despite having visited the region a decade later, Nesbitt and Franchetti were the first to publish their results on the Danakil Depression in 1929 and 1930, respectively. Nesbitt called the lake “Egogi Bad,” a name given by his local guide. Franchetti named it lake Giulietti, as a tribute to Giuseppe-Maria Giulietti after discovering the remains of the explorer and his team near Beylul (Nesbitt, 1935; Taillibert, 1996). Franchetti also called the island in the middle of the lake Afdera–the lowest proven island in the world–after himself for being the first known person setting foot on it. Franchetti extensively explored the lake, and his name was assigned to one of the two endemic fishes of the lake Afdera: Danakilia franchettii (Vinciguerra, 1931; Getahun, 2001).
Geological Setting
The Afdera lake is situated in a tectonically and volcanically active rift valley—the Danakil Depression—positioned between the Ethiopian plateau to the west and the Danakil block to the east (Figure 1). This region is currently experiencing crustal stretching in an extensional environment representing the transition between continental rifting and seafloor spreading (Bastow and Keir, 2011; Keir et al., 2013; Daniels et al., 2014; Bastow et al., 2018). The Afar depression opened up due to the separation of the Arabian plate from the Nubian plate following the Oligocene eruption of trap basalts related to the Afar plume (Bosworth et al., 2005). The eruption of the Ethio-Yemen traps 30 Ma ago was followed by the breakup of the Afro-Arabian plate and the formation of incipient proto–East African and Red Sea rift fractures (Baker et al., 1972; Hofmann et al., 1997; George et al., 1998). Simultaneously, the Gulf of Aden ridge propagated in west-southwest direction toward the present-day Afar region as stop-and-go propagation series (Manighetti et al., 1997; Audin et al., 2004). This kinematic process eventually isolated two rigid tectonic blocks known as the Danakil and Ali-Sabieh blocks which rotated in opposite direction (counterclockwise and clockwise respectively), opening the Afar Depression by a “saloon-door” mechanism (Manighetti et al., 2001; Kidane, 2015). This anticlockwise rotation of the Danakil block formed the Danakil Depression. The individual rift segments are well developed in the Afar depression, consistent with the tectonic features observed in Mid-Oceanic ridge settings (Bridges et al., 2012; Dumont et al., 2018). However, the evidence for a mature and well-individualized transform fault in the Afar depression has not yet been documented. The Makarrasou Transfer Zone between the Assal and Manda Inakir rift segments; the Gamarri-Allol Transfer Zone between two aforementioned segments and the Manda Hararo rift segment in central Afar; the Alayta-Afdera Transfer Zone and the Erta Ale-Tat’Ali Transfer Zone (Figure 1) are all characterized by normal faults with orientations oblique to the rift axis, without forming a well-developed and defined transform fault zone.
Lake Afdera is situated in an area influenced by two transfer zones linking different rift segments. The Alayta–Afdera Transfer Zone links Erta Ale and Tat’Ali rift segments in the NE and the Dabbahu Manda–Hararo in the SW (Tazieff et al., 1972; Barberi et al., 1974; Barberi and Varet, 1977; Stab et al., 2016; Gardo and Varet, 2018; Le Gall et al., 2018; Varet, 2018; Ayalew et al., 2019; Zwaan et al., 2020; see also discussion in Structural Lineaments) and the Erta Ale–Tat’Ali Transfer Zone links Erta Ale and the Tat’Ali rift segments (Illsley-Kemp et al., 2018; La Rosa et al., 2019; 2021). The lake is bounded by two volcanic ranges, the Erta Ale range in the NW and the Tat’Ali range in the E, of which the Borwali volcano directly overlooks the lake. Both volcanic ranges are considered as recent (<0.6 Ma; Barberi et al., 1972; Kidane et al., 2003; Lahitte et al., 2003; Stab et al., 2016). In the S, the lake is dominated by the Afdera volcano. Toward the W and the N, the lake opens up to relatively flat lava plains covered by a thin layer of Holocene to Recent lacustrine (Gasse, 1974) or aeolian sediments punctuated by hyaloclastite rings and volcanic cones (Tazieff et al., 1969). The lake features a small island (Franchetti Island or Āfrerā Desēt) in its southern basin. It is formed by volcanic rocks covered by a thin veneer of lake sediments and unique gypsum microbialites (Foubert et al., 2019).
Because of its isolation in the Danakil Depression and difficulties to access the lake, only a few studies have been carried out (Martini, 1969; Gonfiantini et al., 1973; Bonatti et al., 2017). Bathymetric soundings have been attempted in the 1970s (Bonatti et al., 2017), but the precise lake floor mapping has never been performed, despite geological interest for this region. The bathymetry of lake Afdera and its comparison with outcropping structures is essential for the understanding of the regional structural architecture. Moreover, mapping Lake Afdera is valuable for economic and social reasons. The local population in the village of Afdera and its surroundings are economically dependent on the lake’s salt production (Locatelli-Rossi, 1998; Bekele, 2016). The salt is extracted since the late 1950s by pumping salty lake water into salt evaporation ponds (Locatelli-Rossi, 1998, Supplementary Figure S1). It is not clear whether hydrothermal springs considered as inflow compensate for the natural and artificial evaporation of the lake, but quantifying the lake volume will help to evaluate the stability of the lake Afdera and its fragile ecosystem, as well as the local salt exploitation. This study aims to estimate the lake volume and architecture by bathymetric mapping using an echosounder system mounted on a Rigid Inflatable Boat (RIB). Additionally, this study explores the feasibility of developing portable and easily deployable solutions for bathymetric mapping in challenging environments.
Materials and Methods
The setup of an echosounder system on a mobile RIB has been essential to the success of the mapping survey (Figure 2) and can be applied to the mapping of other lake systems or water bodies with limited access.
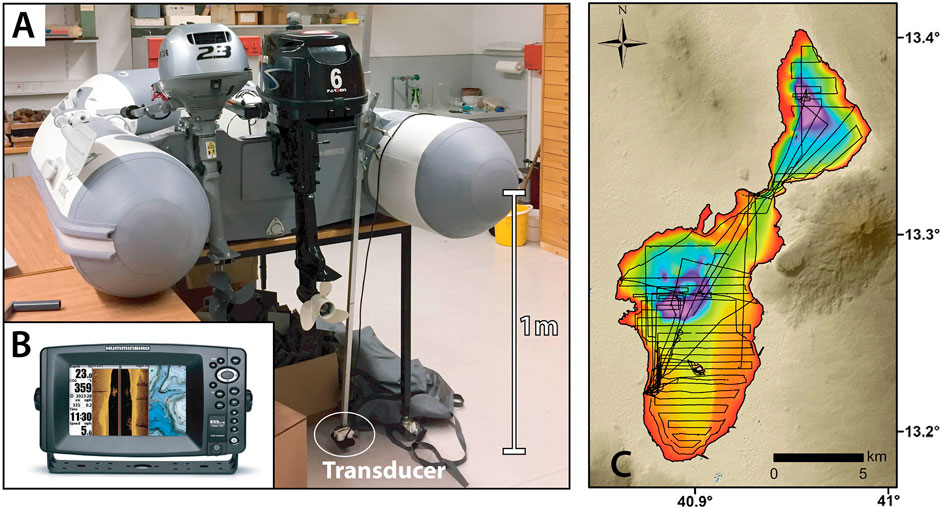
FIGURE 2. Material used during the bathymetric mapping. (A). Rigid Inflatable Boat (Zodiac Cadet 270 Aero) with two engines (Parson 6HP water-cooled and Honda 2.3 HP air-cooled engines). The sonar transducer is attached to a perch plunged at 1 m below lake level. (B). Echosounder Humminbird 899ci hd si. (C). Map of the multibeam survey tracklines acquired during the two surveys in January 2016 and January 2017.
Bathymetric Survey
Two-weeks expeditions were performed in January 2016 and 2017 to map the entire lake Afdera. About 500 km of multibeam survey tracklines (Figure 2C) composed of 242'000 data points spaced on average by 2.1 m, with each point being measured every second at an average speed of 8 km/h. The first mission in 2016 lasted 6 days and allowed to examine the feasibility of the project and improve technical difficulties. During the second mission in 2017, seven full days were dedicated to complete the bathymetric mapping of Lake Afdera. Weather and logistical issues reduced the operational time to five full working days. Along the lakeshore, only a few locations rendered the deployment of the RIB system feasible with the most accessible location being Aligenda hot spring at the southwestern shoreline of the lake, close to the Afdera village. Therefore, all the survey tracks started at that point. The survey tracks were planned with 400 m distance between each track to obtain the most optimal spatial resolution taking into account the limited operational time.
The sonar system has been mounted on a small RIB system, resp. a Zodiac Cadet 270 Aero (Figure 2A). The Zodiac Cadet is 2.7 m in length, has an internal surface of about 1 m2, and can carry up to three passengers for a maximum load of 420 kg. For safety reasons, the boat was powered with two thermic off-board engines, resp. a Parsun F6ABMS 6 horsepower (water cooled) as main engine and a Honda 2.3 horsepower air-cooled engine as backup.
The echosounder used was the mobile depth/fishfinder 899ci hd si system from Humminbird (Figure 2B). This echo sonar uses a dual-beam transducer type (XHS-9-HDSI-180-T) capable of giving instant temperature and side imaging, having a maximum working depth of 500 m and target separation of 63.5 mm. The echosounder operated at 83 kHz with a swath width of 60°. During acquisition, the echosounder has been set to “marine mode” with a fixed conductivity setting of 55 mS/cm. The system integrates a 10 Hz internal GPS receiver built into the head unit with live chart plotting capabilities. To power the system, two packs of 12 V and 13.5 Ah Li-ion batteries—two H2B182-B from HY-LINE plugged in series—were used. Data were temporally stored on a regular SDHC memory card. In 2016, only the air-cooled engine was used, and the echosounder transponder was attached on the right tail of the rugged boat. Consequently, the sonar signal was severely affected by the engine vibrations and swash. Moreover, heavy weather conditions resulted in depth anomalies removed during processing. In 2017, the transponder was attached to an adjustable perch plunged at 1 m below lake level and a water-cooled engine used to reduce vibrations.
Data Processing
Sonar data were processed using ArcGIS software. Artefacts, depth aberrations and faulty readings (less than 1% of the total signal) were manually extracted and removed. Depths have been homogenized between the two missions where the probe was installed at different depths. Sonar data were depth-corrected through the calculated in-situ sound velocity of water samples collected at three different water depths and measured temperature and conductivity values (Table 1). The correction factor of 1.0721 was determined to correct the sonar data to the lake Afdera high conductivity/salinity setting, with an accuracy of ±0.35%. The map was constructed using the “topo to raster” algorithm and the ANUDEM elevation interpolation procedure (Hutchinson et al., 2011).
Fault Analysis
Onshore faults have been mapped using satellite imagery (ESRI) and DEM (SRTM, 1 Arc-Second Global Elevation data) in a zone extending from Erta Ale in the north to Manda Harraro in the south. These faults are basically the same as already identified by Varet et al. (1975), Illsley-Kemp et al. (2018) and La Rosa et al. (2019, 2021). The lineaments of an area of 60 × 60 km around the lake were analyzed and data plotted in a rose diagram. The orientation of faults larger than 500 m (97% of the set) were split into straight 500 m segments to obtain a fault-length weighted result. Smaller faults and/or segments were considered as single segments.
Results
The main results of the bathymetry are shown in Figure 3. All data (raw bathymetry datapoints, interpreted bathymetry raster, interpreted immerged and outcropping faults) are available in a data repository (Schaegis et al., submitted). The lake covers an area of 117 km2 with an average depth of 20.9 m. The total volume represents 2.4 km3 of water. The lake is divided into two distinct parts: the southern basin, being the largest with 87 km2 surface coverage and containing 1.5 km3 of water, and the northern basin covering 30 km2 with a volume of 0.9 km3. The southern part of the basin in the South is mostly flat and shallow with a depth ranging from 5 to 15 m. The Franchetti Island represents a 300 m diameter emerged land surface with abrupt topography. Further north, a depression (from 50 to 76 m) is located within a 5 km diameter circular structure with a noticeable elevation (40 m) in its central part. The northern basin of the lake displays sharp bathymetric changes and a triangular shape with the edges oriented N-S; NNW-SSE; SW-NE. The western edge (N-S axis) is the steepest. The sinuosity of this escarpment is an artefact related to the interpolation algorithm applied between the multibeam survey tracks. Despite being disconnected, both basins exhibit maximum depths of 76 and 80 m below lake level, or −188 m asl. and −192 m asl. for the southern and northern basin, respectively.
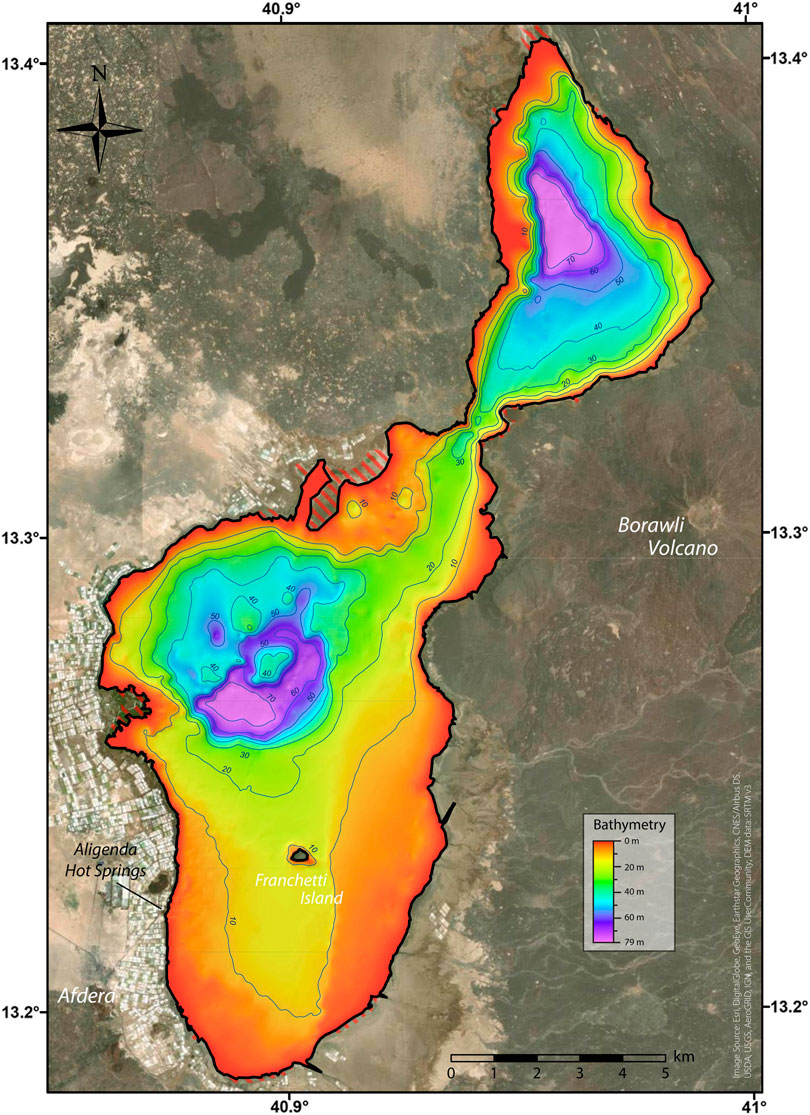
FIGURE 3. Bathymetric map of Lake Afdera with contour lines indicating depth in meters below lake level. The deepest point of the northern basin is 80 m below the lake level surface and the deepest point of the southern basin is 76 m below lake level surface. The lake surface is at 112 m below sea level. The red dashed surfaces indicate area periodically flooded by a few tens of cm of water.
Discussion
Elevation and Dimensions of the Lake
The elevation of the lake Afdera water level is today at −112 m asl covering a total surface of 117 km2 (SRTM, 1 arc-second DEM). With a minimum elevation of −92 m asl., Lake Afdera is the deepest lake and one of the lowest topographies in the Afar, after Lake Assal in Djibouti, at ca. −195 m asl. (Langguth and Pouchan, 1975). It is also the deepest known point of the Danakil Depression as the depths of lakes Bakili and Karum remain unknown.
The fluctuation in dimensions and elevation of the lake since the 1960s expeditions remain unclear, as data are incoherent between the reporting authors. The surface of the lake was estimated by Martini (1969) at 70 km2 and as such cited by other authors (Gonfiantini et al., 1973; Bonatti et al., 2017). The first estimation by Martini (1969) was probably only referring to the southern basin, the only part he mapped and studied, and was mistakenly cited as the total lake surface by the other authors.
The elevation of the lake level has been estimated differently by several authors. Martini (1969) only mentioned that it is situated below sea level. Gonfiantini et al. (1973) estimated the altitude at −70 m asl. Gasse (1974), Gasse et al. (1974) and Gasse and Street (1978) estimated the lake level at −80, −82 and −80 m asl., respectively. Finally, Bonatti et al. (2017) estimated the elevation of the lake in 1969 between −90 and −100 m asl. Based on these estimations and the present day measured elevation of −112 m asl., Bonatti et al. (2017) conclude that “lake’s level appears to have dropped by over 10 m during the last ∼50 years.” Due to the flat topography, a higher lake level, even only at −100 m asl., would cover an area twice as big as today’s and would completely change its geometry (Figure 4). The shoreline drawn by Martini (1969, Supplementary Figure S2) and other geological map data (Brinckmann and Kürsten, 1970; Varet et al., 1975) fit exactly the current shape of the lake. Furthermore, local inhabitants never noticed any lake level variation (pers. comm. with local chairman, 2020). This fact is corroborated by the small variations, but the overall constant lake extent observed by remote sensing data collected between 1998 and 2016 (max 6.2% expansion and 4.8% shrinkage relative to the average, Nanis and Aly, 2020). To quantify these minor changes, images of the shore of a period of relatively high water (January 2016) were compared with other images of a period of relatively low water (January 2019, Supplementary Figure S3). It shows a lake-level change of 25 ± 10 cm. Analysis of Landsat eight images show that at the same time, the lake surface increased by 4.1 km2, i.e., 3.5%. Assuming a linear correlation between lake surface and lake level change, a maximum variation of 11% reported by Nanis and Aly (2020) would correspond to a lake-level change of 80 ± 30 cm. This corresponds to a total lake volume change of 4 ± 2%, which is surprisingly small. We therefore conclude that the lake level and water volume remained relatively stable for at least the last 50 years, despite evaporation in this arid environment and intensive artificial pumping. The mistakes in the estimation of lake elevation during the previous century are probably caused by imprecize maps or high uncertainties related to the barometric measurement of elevation. Thus, we consider the conclusion of Bonatti et al. (2017) about lake level drop as incorrect.
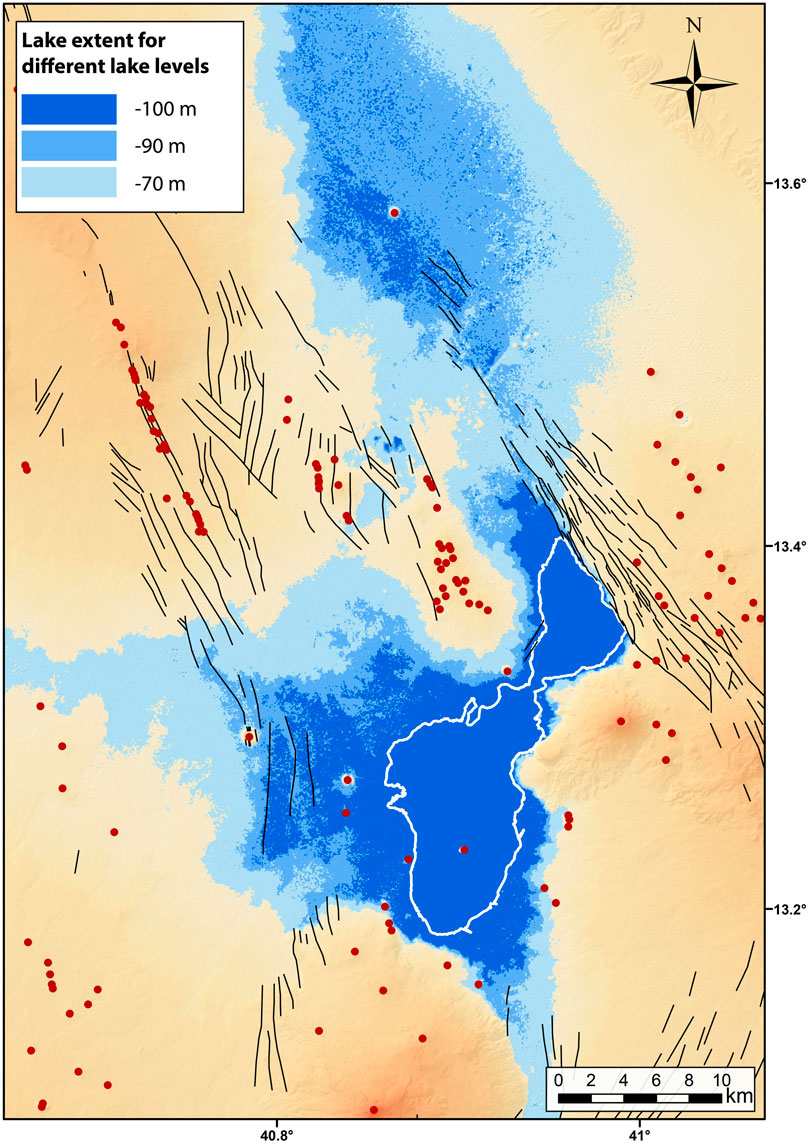
FIGURE 4. Map showing the potential extent of Lake Afdera assuming lake level elevation of −100, −90 and −70 m asl.
Lake Afdera is the second largest lake in the Afar after lake Abbé in Djibouti. Lake Abbé covers an area of 320 km2, but its volume is not well documented. According to Karimi et al. (2015), lake Abbé has a maximum recorded depth of 36 m, but its water level varies greatly depending on the influx through the largest river in the Afar: the Awash River. Indeed, during dry periods, the lake Abbé level can decrease by 5 m (Karimi et al., 2015). On the contrary, no major rivers feed Lake Afdera. The only identified water sources are hot springs (Martini, 1969; Bonatti et al., 2017; Foubert et al., 2019). Lake Afdera is probably the largest stable lake in Afar. Its stability could potentially be explained by largely hidden water reservoirs in the surrounding faulted subsurface (Gasse et al., 1974).
Structural Lineaments
Fault mapping around Lake Afdera shows four main structural orientations (Figures 5A,C) under the same regional NE-SW stress field, which are interpreted to be either part of the main NNW orientation of the Red Sea rift axis of the Danakil Depression or part of the two active transfer zones in the area (Figure 5A).
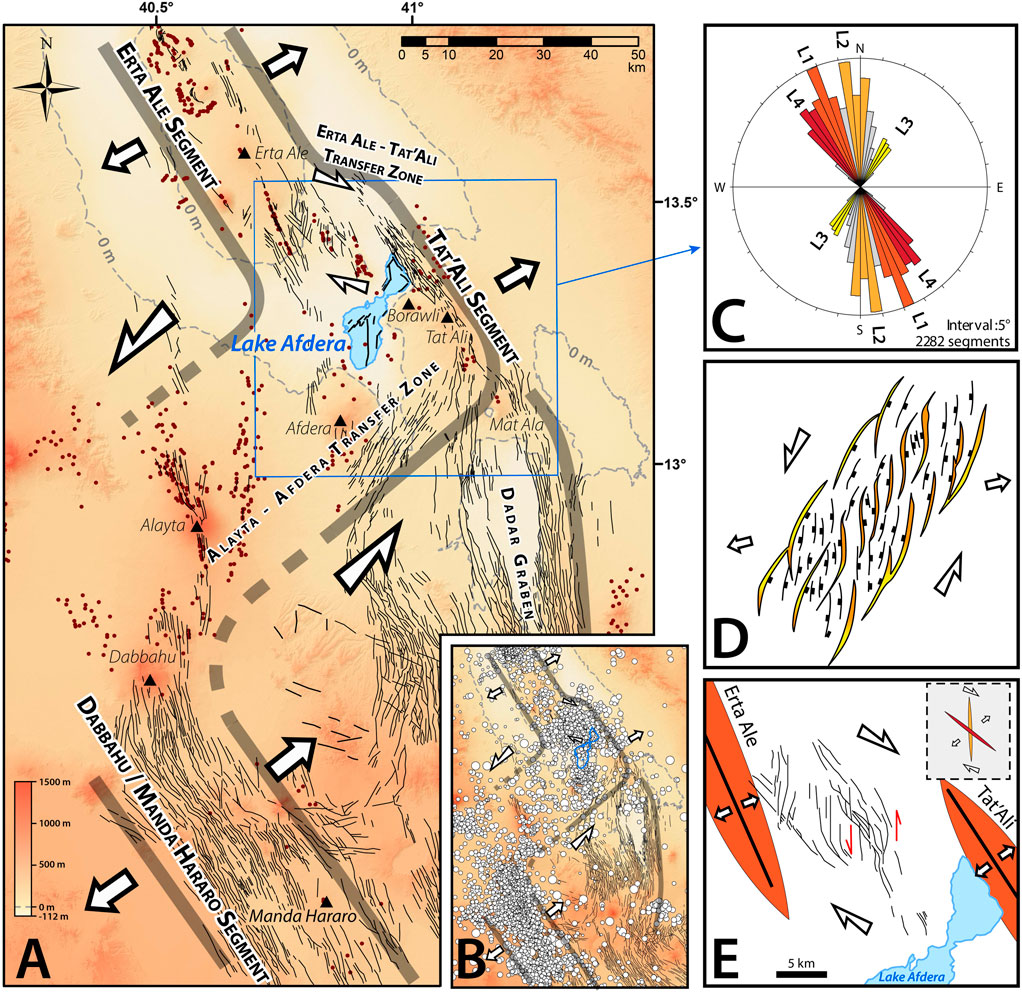
FIGURE 5. Structural analysis of the larger Afdera region. (A): Mapping of tectonic lineaments (in black) and of volcanic cones or vents (in brown). (B): Earthquake compilation in the study area by Zwaan et al. (2020) including data from Keir et al. (2006), Belachew et al. (2011) and Illsely-Kemp et al. (2018). (C): Length-weighted rose diagram showing the orientation of the tectonic lineaments around lake Afdera in the area represented by the blue square on (A). (D): Results of analogue modeling of oblique rifting by McClay et al. (2002). The colors represent the orientations shown on panel (B). Comparison between the Alayta–Afdera Transfer Zone and the model of McClay et al. (2002) shows striking similarities in fault orientation. This transtentional system is hence interpreted to have formed the L2 and L3 fault orientations. (E): Structural interpretation of the Erta Ale–Tat’Ali Transfer Zone redrawn after La Rosa et al. (2019, 2021). It shows that this transfer zone formed L2 and L4 orientations.
The first one is the sinistral Alayta–Afdera Transfer Zone which links the main Dabbahu/Manda Hararo rift segment to the Erta Ale and the Tat’Ali rift segments. Although this transfer zone has been mentioned by several authors, no detailed description of its nature and fault orientation has been made. This first recognition of the en échelon structure of the Alayta and Tat’Ali range was mentioned by Tazieff et al. (1972). The transversal nature of the faults south of Afdera is discussed by Barberi et al. (1974) who also suggest that Lake Afdera might be part of the same transverse structure. Barberi and Varet (1977) then proposed that these faults represented a sinistral transform boundary. This concept of transfer zone between Dabbahu/Manda Hararo rift segment and the Erta Ale/Tat’Ali rift segment has also been assumed in several recent publications (Stab et al., 2016; Gardo and Varet, 2018; Le Gall et al., 2018; Varet, 2018; Ayalew et al., 2019; Zwaan et al., 2020). It is also supported by earthquake data and seismic moment release (Zwaan et al., 2020, Figure 5B). A comparison between the fault pattern of the Alayta-Afdera Transfer Zone and analogue modeling of oblique rifting (McClay et al., 2002, Figure 5D) shows striking similarities, thus providing a conceptual model for the fracture pattern development. The Alayta-Afdera Transfer Zone would thus constitute a broad area of diffuse deformation and important magmatism forming a sinistral linkage zone between the Dabbahu/Manda Hararo rift segment and the Erta Ale/Tat’Ali rift segments.
The second transfer zone is the smaller dextral Erta Ale–Tat’Ali Transfer Zone, which links the Erta Ale and Tat’Ali rift segments and that has been investigated based on seismicity, InSAR, structural geology and described in great details by Illsley-Kemp et al. (2018) and La Rosa et al. (2019, 2021) see Figure 5E.
The first structural group mapped around the lake (L1, Figure 5C) is oriented NNW-SSE and corresponds to the Red Sea rift orientation. It constitutes the main rift-axis orientation and is identical to the directions observed on the Erta’Ale range, the Tat’Ali and between Dabbahu and Manda Hararo. The Alayta–Afdera Transfer Zone is interpreted to have generated the L2 (N-S) and L3 (NE-SW) structural trends. The L2 (N-S) orientation is present west of Lake Afdera, on the Afdera volcano and around the Alayta, both as faults and volcano alignments. L3 (NE-SW) is mainly found south of Tat’Ali and Afdera volcano.
As demonstrated by Illsley-Kemp et al. (2018) and La Rosa et al. (2019, 2021), the Erta Ale–Tat’Ali Transfer Zone formed the L4 (NW-SE) orientation but also the L2 (N-S), which is shared with the Alayta–Afdera transfer system. The L2 orientation is hence common for the two transfer systems. The four identified main structural orientations are consistent with the overall NE-SW opening kinematics of the Danakil depression. Lake Afdera is therefore situated in a unique position at the intersection of two transfer zones and the rift axis, possibly marking the position of an incipient transform fault (Illsley-Kemp et al., 2018).
Underwater Topography
The bathymetry of the lake gives insights into the lake floor geomorphology. The slope map (Figure 6A) indicates steep elongated features in the northern basin that are interpreted as faults. Their orientation coincides with the structural trends identified around the lake. The L4, L2 and L3 structural trends are all expressed in this northern basin. Hence, this basin is interpreted as a triangular tectonic graben caused by the intersection of three different fault orientations (Figures 6B,C). The southern basin is marked by a clear L2 fault lined up along its length axis. The 5 km-wide circular feature representing the deepest part of this basin is interpreted as a submarine caldera. This caldera is structured by L3 and L2 faults. The elevation in the central part of the caldera is interpreted as a resurgent scoria cone (Figures 6B,D). Its shape and size mirror the volcanic cone forming the Franchetti Island. This morphology is similar to the nearby Tat’Ali volcano which shows a 3 km wide caldera structured by L1 to L2 faults and similarly featuring a nested volcanic cone. Many other volcanoes in the Afar depression or the Main Ethiopian Rift feature comparable tectono-volcanic morphologies (caldera structured by faults and with nested volcanic cones, e.g., Hunt et al., 2019). The arcuate features at the foot of the Erta Ale range, the Borawli and Afdera volcanos are interpreted to be lava flows. They are situated just offshore of lava flows either observed in the field or visible on satellite images.
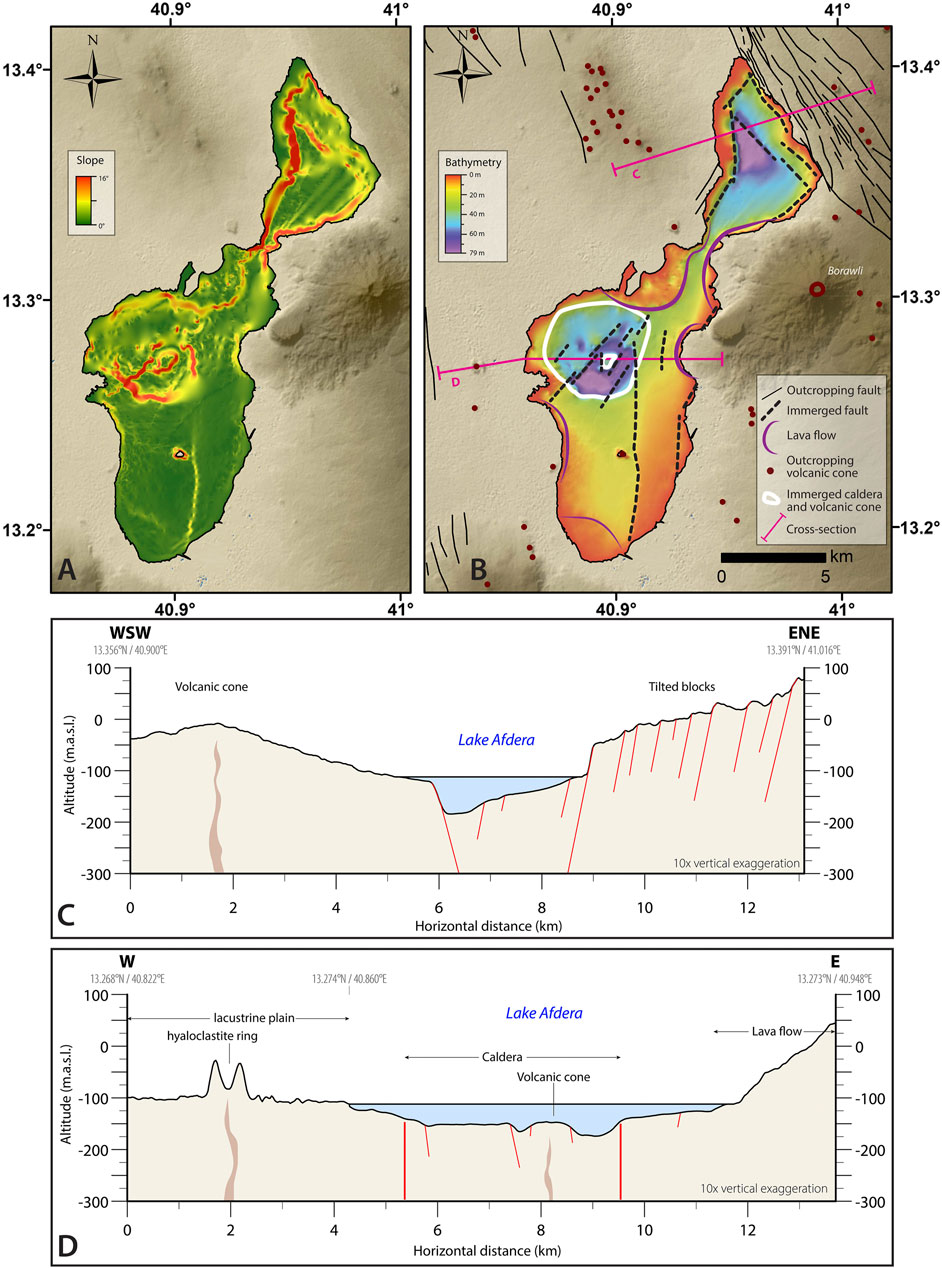
FIGURE 6. Interpretation of the lake morphology. Slope map (A) allows to identify several faults as well as a volcanic edifice in the lake (B). (C,D) show cross-sections of the northern and southern basin. The northern basin shows a graben morphology while the southern basin indicates a faulted caldera with a nested volcanic cone.
The presence of a caldera in a depression is less common. However, several calderas in the area are found on nearly flat shield volcanoes (e.g., Erta Ale, Mat Ala, unnamed volcanoes at 12.09N, 40.89E; 12.165N, 40.927E) and several volcanoes are situated at very low elevations [e.g., Catherine volcano (13.912N, 40.449E, −123 m), Dallol volcano (14.240N, 40.299E, −92 m, no lava outcropping)]. The combination of both is thus not surprising. We therefore interpret that this caldera is now situated at lower elevation because of the lower magma production compared to the neighboring volcanic ranges (Erta Ale Range, Tat’Ali Range and Afdera Volcano).
Overall, these interpretations imply that both basins are genetically different. The northern basin is a tectonic influenced basin, while the southern one is controlled by volcano-tectonic morphologies. However, both form in response to the same regional stress field and at the intersection of fault sets of different orientations forming small-scale pull-apart basins.
Lake Afdera in the Danakil Rift System
The geomorphological structures interpreted in Lake Afdera can be directly related to other rift structures in the Afar Rift System. The large caldera inferred in the southern basin is situated on the axis of the Erta-Ale rift segment, featuring numerous recent volcanoes. The fault pattern with respect to the regional stress field indicates a strong influence of transverse structures in the local tectonics. Two transfer zones are linking the en echelon arranged rift axes segments. Lake Afdera is mainly structured by the major Alayta–Afdera Transfer Zone as shown by the predominance of L2 and L3 orientations (see Figure 5A). The smaller Erta Ale–Tat’Ali Transfer Zone probably only influences the northern basin with L2 and L4 orientations. Regionally, the transverse nature of the zone is also expressed by petrological characteristics typical of a transverse structure (Barberi et al., 1974).
As discussed above, both basins formed mainly due to the interaction between the rift axis and the Alayta–Afdera Transfer Zone. Like Bonatti et al. (2017), we consider this lake and chiefly its northern basin to be the equivalent of the oceanic “nodal basins,” deep basins forming at slow-spreading ridge-transform intersections (RTI) (Sleep and Biehler, 1970; Fox and Gallo, 1984; Karson et al., 1984; Goud and Karson, 1985; Pockalny et al., 1988; Thiede et al., 1990). The triangular shape of the northern basin is typical for this structure. It can hence be considered as an incipient transform zone (see also Illsley-Kemp et al., 2018). The position of the lake Afdera as the deepest point of the Danakil Depression is therefore not fortuity but the result of the interaction between the rift axis and transverse structures. In contrast to their oceanic counterparts, faults of the Alayta–Afdera Transfer Zone are not perpendicular but oblique to the rift axis and, as such, the dominant kinematics probably involve oblique movement along the exposed faults. The zone acts as a transtensional relay expressed in continental crust, with a broader area of strain partitioning than known from pure, more narrow oceanic transform faults affecting thinner oceanic lithosphere.
Conclusion
This study unveils the first complete bathymetric map of the lake Afdera with an area of 117 km2, a volume of 2.4 km3, an average depth of 20.9 m and a maximal measured depth of 80 m below lake level. The lake Afdera is therefore the largest and deepest endorheic stable lake in Afar, hosting the lowest measured topography in the Danakil Depression. This study provides a present state of knowledge of the lake Afdera from its discovery in 1920 to the different attempts to estimate its depth. We evidence that the lake water volume did not fluctuate by more than a few percent during the last 50 years, despite artificial pumping and climatic evaporation. This could potentially be explained by large hidden water reservoirs in the surrounding faulted subsurface.
The bathymetric data indicate that the morphology of the northern basin is controlled by tectonics with faults oriented in three different directions, forming a triangular graben structure. The southern basin is interpreted to be influenced by volcano-tectonic processes with a large submerged caldera structured by faults and featuring a major caldera and associated resurgent volcanic cone. The fault orientations throughout the lake indicate that the lake is influenced by two transfer zones, the Alayta–Afdera Transfer Zone and the Erta Ale–Tat’Ali Transfer Zone. The former probably having a stronger influence on the lake structure than the latter. The interaction between the Alayta–Afdera Transfer Zone and the rift axis in the northern part of the lake Afdera forms the equivalent of an oceanic nodal basins and explains the very low elevations encountered. Lake Afdera represents as such an incipient transform fault zone on the floor of the Afar depression.
Finally, this study attests that deploying portable and easy bathymetric mapping systems in remote areas result in the acquisition of valuable datasets in challenging environments.
Data Availability Statement
All raw data have been submitted to a data repository (pangaea.de). They are also available through direct contact with the authors.
Author Contributions
J-CS: Concept/Design/Material/Data Collection (bathymetric survey)/Analysis and interpretation/Literature research/Writing. VR: Data Collection (lineament mapping)/Data Processing (bathymetric survey)/Analysis and interpretation/Figures/Literature research/Writing. TK: Supervision/Critical review/Interpretation. JM: Critical review/Interpretation. EG: Data Collection (bathymetric survey)/Critical review. BA: Critical review. AF: Supervision/Concept/Design/Critical review/Interpretation.
Funding
This study was supported by the Swiss National Science Foundation (grant number 200021_163114; SERENA (SEdimentary REcord of the Northern Afar). Start-up and seed funding have been provided by the project TOTAL-COCARDE (FR00005695).
Conflict of Interest
The authors declare that the research was conducted in the absence of any commercial or financial relationships that could be construed as a potential conflict of interest.
Acknowledgments
We thank Andres Rüggeberg for its precious help with the depth conversion. Special thanks to Léa Perrochet, Patrizia Wyler and Haileyesus Negga for their help during the mapping. We also would like to thank Christoph Neururer for helping to set up the equipment and finding a power solution for the bathymetric surveys. Thank also the local Afdera community, the people from the camp site, Ethioder (cfr. accommodation and logistics on-site), Ministry of Mines for granting access for sample export, local and regional administration offices for granting research permission and support in the field.
Supplementary Material
The Supplementary Material for this article can be found online at: https://www.frontiersin.org/articles/10.3389/feart.2021.706643/full#supplementary-material
References
Audin, L., Quidelleur, X., Coulié, E., Courtillot, V., Gilder, S., Manighetti, I., et al. (2004). Palaeomagnetism and K-Ar and40Ar/39Ar Ages in the Ali Sabieh Area (Republic of Djibouti and Ethiopia): Constraints on the Mechanism of Aden ridge Propagation into southeastern Afar during the Last 10 Myr. Geophys. J. Int. 158, 327–345. doi:10.1111/j.1365-246X.2004.02286.x
Ayalew, D., Pik, R., Bellahsen, N., France, L., and Yirgu, G. (2019). Differential Fractionation of Rhyolites during the Course of Crustal Extension, Western Afar (Ethiopian Rift). Geochem. Geophys. Geosyst. 20, 571–593. doi:10.1029/2018GC007446
Baker, B. H., Mohr, P. A., and Williams, L. A. J. (1972). Geology of the Eastern Rift System of Africa. Geological Society of America. doi:10.1130/SPE136
Barberi, F., and Varet, J. (1977). Volcanism of Afar: Small-Scale Plate Tectonics Implications. Geol. Soc. America Bull. 88, 1251. doi:10.1130/0016-7606(1977)88<1251:voaspt>2.0.co;2
Barberi, F., Borsi, S., Ferrara, G., Marinelli, G., Santacroce, R., Tazieff, H., et al. (1972). Evolution of the Danakil Depression (Afar, Ethiopia) in Light of Radiometric Age Determinations. J. Geology. 80, 720–729. doi:10.1086/627797
Barberi, F., Bonatti, E., Marinelli, G., and Varet, J. (1974). Transverse Tectonics during the Split of a Continent: Data from the Afar Rift. Tectonophysics 23, 17–29. doi:10.1016/0040-1951(74)90108-5
Bastow, I. D., and Keir, D. (2011). The Protracted Development of the Continent-Ocean Transition in Afar. Nat. Geosci 4, 248–250. doi:10.1038/ngeo1095
Bastow, I. D., Booth, A. D., Corti, G., Keir, D., Magee, C., Jackson, C. A. L., et al. (2018). The Development of Late‐Stage Continental Breakup: Seismic Reflection and Borehole Evidence from the Danakil Depression, Ethiopia. Tectonics 37, 2848–2862. doi:10.1029/2017TC004798
Bekele, K. (2016). Inferior Quality Salt Floods Local Market. Report. Ethiop./ English. Available at: https://www.thereporterethiopia.com/content/inferior-quality-salt-floods-local-market (Accessed November 14, 2018).
Belachew, M., Ebinger, C., Coté, D., Keir, D., Rowland, J. V., Hammond, J. O. S., et al. (2011). Comparison of dike Intrusions in an Incipient Seafloor-Spreading Segment in Afar, Ethiopia: Seismicity Perspectives. J. Geophys. Res. 116, 6405. doi:10.1029/2010JB007908
Bonatti, E., Gasperini, E., Vigliotti, L., Lupi, L., Vaselli, O., Polonia, A., et al. (2017). Lake Afrera, a Structural Depression in the Northern Afar Rift (Red Sea). Heliyon 3, e00301. doi:10.1016/j.heliyon.2017.e00301
Bosworth, W., Huchon, P., and McClay, K. (2005). The Red Sea and Gulf of Aden Basins. J. Afr. Earth Sci. 43, 334–378. doi:10.1016/j.jafrearsci.2005.07.020
Bridges, D. L., Mickus, K., Gao, S. S., Abdelsalam, M. G., and Alemu, A. (2012). Magnetic Stripes of a Transitional continental Rift in Afar. Geology 40, 203–206. doi:10.1130/G32697.1
Brinckmann, J., and Kürsten, M. (1970). Geological Sketchmap of the Danakil Depression (1:250’000). Hann. Bundesanstalt für Bodenforschung.. doi:10.3790/978-3-428-41818-3
Chiozzi, G., Stiassny, M. L. J., De Marchi, G., Lamboj, A., Fasola, M., and Fruciano, C. (2018). A Diversified Kettle of Fish: Phenotypic Variation in the Endemic Cichlid Genus Danakilia of the Danakil Depression of Northeastern Africa. Biol. J. Linn. Soc. 124, 690–705. doi:10.1093/biolinnean/bly085
Daniels, K. A., Bastow, I. D., Keir, D., Sparks, R. S. J., and Menand, T. (2014). Thermal Models of Dyke Intrusion during Development of Continent-Ocean Transition. Earth Planet. Sci. Lett. 385, 145–153. doi:10.1016/j.epsl.2013.09.018
Dumont, S., Klinger, Y., Socquet, A., Escartín, J., Grandin, R., Jacques, E., et al. (2019). Rifting Processes at a Continent‐Ocean Transition Rift Revealed by Fault Analysis: Example of Dabbahu‐Manda‐Hararo Rift (Ethiopia). Tectonics 38, 190–214. doi:10.1029/2018TC005141
Foubert, A., De Boever, E., Schaegis, J.-C., Rime, V., Hailu, A., Negga, H., et al. (2019). The Interaction between Hot spring Carbonates and gypsum Deposits in a Hypersaline Rift lake (Lake Afdera, Afar, Ethiopia).
Fox, P. J., and Gallo, D. G. (1984). A Tectonic Model for ridge-transform-ridge Plate Boundaries: Implications for the Structure of Oceanic Lithosphere. Tectonophysics 104, 205–242. doi:10.1016/0040-1951(84)90124-0
Gardo, I. A., and Varet, J. (2018). “Ta’Ali Geothermal Site, Afdera Woreda, Northern Afar, Ethiopia,” in Proceedings, 7th African Rift Geothermal Conference Kigali (Rwanda).
Gasse, E., and Street, F. A. (1978). Late Quaternary lake-level Fluctuations and Environments of the Northern Rift Valley and Afar Region (Ethiopia and Djibouti). Palaeogeogr. Palaeoclimatol. Palaeoecol. 24, 279–325. doi:10.1016/0031-0182(78)90011-1
Gasse, F., Fontes, J. C., and Rognon, P. (1974). Variations hydrologiques et extension des lacs holocenes du Desert Danakil. Palaeogeogr. Palaeoclimatol. Palaeoecol. 15, 109–148. doi:10.1016/0031-0182(74)90028-5
Gasse, F. (1974). Les Diatomées des Sédiments Holocènes du Bassin du Lac Afrera (Giulietti) (Afar Septentrional, Ethiopie). Essai de Reconstitution de l'Évolution du Milieu. Int. Revue Ges. Hydrobiol. Hydrogr. 59, 95–122. doi:10.1002/iroh.19740590112
George, R., Rogers, N., and Kelley, S. (1998). Earliest Magmatism in Ethiopia: Evidence for Two Mantle Plumes in One Flood basalt Province. Geol 26, 923. doi:10.1130/0091-7613(1998)026<0923:emieef>2.3.co;2
Getahun, A. (2001). Lake Afdera: a Threatened saline lake in Ethiopia. Sejs 24, 127–131. doi:10.4314/sinet.v24i1.18180
Gonfiantini, R., Borsi, S., Ferrara, G., and Panichi, C. (1973). Isotopic Composition of Waters from the Danakil Depression (Ethiopia). Earth Planet. Sci. Lett. 18, 13–21. doi:10.1016/0012-821X(73)90028-9
Goud, M. R., and Karson, J. A. (1985). Tectonics of Short-Offset, Slow-Slipping Transform Zones in the FAMOUS Area, Mid-Atlantic Ridge. Mar. Geophys. Researches 7, 489–514. doi:10.1007/BF00368952
Hofmann, C., Courtillot, V., Féraud, G., Rochette, P., Yirgu, G., Ketefo, E., et al. (1997). Timing of the Ethiopian Flood basalt Event and Implications for Plume Birth and Global Change. Nature 389, 838–841. doi:10.1038/39853
Hunt, J. A., Pyle, D. M., and Mather, T. A. (2019). The Geomorphology, Structure, and Lava Flow Dynamics of Peralkaline Rift Volcanoes from High‐Resolution Digital Elevation Models. Geochem. Geophys. Geosyst. 20, 1508–1538. doi:10.1029/2018GC008085
Hutchinson, M. F., Xu, T., and Stein, J. A. (2011). Recent Progress in the ANUDEM Elevation Gridding Procedure. Geomorphometry, 2011 19–22.
Illsley‐Kemp, F., Bull, J. M., Keir, D., Gerya, T. V., Pagli, C., Gernon, T., et al. (2018). Initiation of a Proto‐Transform Fault Prior to Seafloor Spreading. Geochem. Geophys. Geosyst 19. 2018GC007947. doi:10.1029/2018GC007947
Karimi, P., Bastiaanssen, W. G. M., Sood, A., Hoogeveen, J., Peiser, L., Bastidas-Obando, E., et al. (2015). Spatial Evapotranspiration, Rainfall and Land Use Data in Water Accounting - Part 2: Reliability of Water Accounting Results for Policy Decisions in the Awash basin. Hydrol. Earth Syst. Sci. Discuss. 11, 1125–1167. doi:10.5194/hessd-11-1125-2014
Karson, J. A., Fox, P. J., Sloan, H., Crane, K. T., Kidd, W. S. F., Bonatti, E., et al. (1984). The Geology of the Oceanographer Transform: The ridge-transform Intersection. Mar. Geophys. Res. 6, 109–141. doi:10.1007/BF00285956
Keir, D., Ebinger, C. J., Stuart, G. W., Daly, E., and Ayele, A. (2006). Strain Accommodation by Magmatism and Faulting as Rifting Proceeds to Breakup: Seismicity of the Northern Ethiopian Rift. J. Geophys. Res. 111, B05314. doi:10.1029/2005JB003748
Keir, D., Bastow, I. D., Pagli, C., and Chambers, E. L. (2013). The Development of Extension and Magmatism in the Red Sea Rift of Afar. Tectonophysics 607, 98–114. doi:10.1016/j.tecto.2012.10.015
Kidane, T., Courtillot, V., Manighetti, I., Audin, L., Lahitte, P., Quidelleur, X., et al. (2003). New Paleomagnetic and Geochronologic Results from Ethiopian Afar: Block Rotations Linked to Rift Overlap and Propagation and Determination of a ∼2 Ma Reference Pole for Stable Africa. J. Geophys. Res. 108. doi:10.1029/2001jb000645
Kidane, T. (2015). Strong Clockwise Block Rotation of the Ali-Sabieh/Aïsha Block: Evidence for Opening of the Afar Depression by a 'saloon-Door' Mechanism. Geol. Soc. Lond. Spec. Publications 420, 209–219. doi:10.1144/SP420.10
La Rosa, A., Pagli, C., Keir, D., Sani, F., Corti, G., Wang, H., et al. (2019). Observing Oblique Slip during Rift Linkage in Northern Afar. Geophys. Res. Lett. 46, 10782–10790. doi:10.1029/2019GL084801
La Rosa, A., Keir, D., Doubre, C., Sani, F., Corti, G., Leroy, S., et al. (2021). Lower Crustal Earthquakes in the March 2018 Sequence along the Western Margin of Afar. Geochem. Geophys. Geosyst 22, 1–15. doi:10.1029/2020gc009614
Lahitte, P., Gillot, P.-Y., and Courtillot, V. (2003). Silicic central Volcanoes as Precursors to Rift Propagation: The Afar Case. Earth Planet. Sci. Lett. 207, 103–116. doi:10.1016/S0012-821X(02)01130-5
Langguth, H. R., and Pouchan, P. (1975). Caractères physiques et conditions de stabilité du Lac Assal (TFAI). Afar Depression of Ethiopia: Proceedings of an International Symposium on the Afar Region and Related Rift Problems, 250–258.
Le Gall, B., Leleu, S., Pik, R., Jourdan, F., Chazot, G., Ayalew, D., et al. (2018). The Red Beds Series in the Erta Ale Segment, North Afar. Evidence for a 6 Ma-Old post-rift basin Prior to continental Rupturing. Tectonophysics 747-748, 373–389. doi:10.1016/j.tecto.2018.10.002
Locatelli-Rossi, L. (1998). The Gift of Salt : A Personal story from Lake Afdera. 1998–1999. Available at: https://www.ign.org/newsletter/idd_feb17_ethiopia.pdf (Accessed February 2017).
Lupi, L. (2009). L’Esplorazione Della Dancalia. La Contesa Per Il Primato. Boll. Della Soc. Geogr. Ital. 2, 827–875.
Manighetti, I., Tapponnier, P., Courtillot, V., Gruszow, S., and Gillot, P.-Y. (1997). Propagation of Rifting along the Arabia-Somalia Plate Boundary: The Gulfs of Aden and Tadjoura. J. Geophys. Res. 102, 2681–2710. doi:10.1029/96JB01185
Manighetti, I., Tapponnier, P., Courtillot, V., Gallet, Y., Jacques, E., and Gillot, P.-Y. (2001). Strain Transfer between Disconnected, Propagating Rifts in Afar. J. Geophys. Res. 106, 13613–13665. doi:10.1029/2000jb900454
McClay, K. R., Dooley, T., Whitehouse, P., and Mills, M. (2002). Physical Models. Am. Assoc. Pet. Geol. Bull. 86, 935–959. doi:10.1007/978-3-7091-0560-3_3
McClusky, S., Reilinger, R., Ogubazghi, G., Amleson, A., Healeb, B., Vernant, P., et al. (2010). Kinematics of the southern Red Sea-Afar Triple Junction and implications for plate dynamics. Geophys. Res. Lett. 37, 1–5. doi:10.1029/2009GL041127
Munzinger, W. (1869). Narrative of a Journey through the Afar Country. J. R. Geogr. Soc. Lond. 39, 188–232. doi:10.2307/1798551
Nanis, H., and Aly, M. H. (2020). Desegregation of Remote Sensing and GIS to Characterize Fluctuations in the Surface Water Area of Afar Lakes, Ethiopia. Geocarto Int. 35, 976–990. doi:10.1080/10106049.2018.1559884
Nesbitt, L. M. (1935). Hell-Hole of Creation: The Exploration of Abyssinian Danakil. New York -Alfred - A - Knopf.
Pockalny, R. A., Detrick, R. S., and Fox, P. J. (1988). Morphology and Tectonics of the Kane Transform from Sea Beam Bathymetry Data. J. Geophys. Res. 93, 3179–3193. doi:10.1029/JB093iB04p03179
Schaegis, J.-C., Jaramillo-Vogel, D., Wyler, P., Perrochet, L., Filfilu, E., Atnafu, B., et al. (2017). “Hot spring Carbonates Associated to a Hypersaline Lacustrine System in an Active Rift Setting (Lake Afdera, Danakil Depression, Ethiopia),” in International Meeting of Sedimentology (Toulouse, France).
Sleep, N. H., and Biehler, S. (1970). Topography and Tectonics at the Intersections of Fracture Zones with central Rifts. J. Geophys. Res. 75, 2748–2752. doi:10.1029/jb075i014p02748
Stab, M., Bellahsen, N., Pik, R., Quidelleur, X., Ayalew, D., and Leroy, S. (2016). Modes of Rifting in Magma-Rich Settings: Tectono-Magmatic Evolution of Central Afar. Tectonics 35, 2–38. doi:10.1002/2015TC003893
Taillibert, C. (1996). L’Expédition Franchetti en Dankalie (Italie, production Luce, 1929). 1895 Mille huit cent quatre-vingt-quinze 1, 151–162. doi:10.3406/1895.1996.1164
Tazieff, H., Marinelli, G., Barberi, F., and Varet, J. (1969). Géologie de l'Afar Septentrional. Bull. Volcanol. 33, 1039–1072. doi:10.1007/BF02597707
Tazieff, H., Varet, J., Barberi, F., and Giglia, G. (1972). Tectonic Significance of the Afar (Or Danakil) Depression. Nature 235, 144–147. doi:10.1038/235144a0
Thiede, J., Pfirman, S., Schenke, H.-W., and Reil, W. (1990). Bathymetry of Molloy Deep: Fram Strait between Svalbard and Greenland. Mar. Geophys. Res. 12, 197–214. doi:10.1007/BF02266713
Varet, J., Barberi, F., Borsi, S., Cheminée, J. L., Giglia, G., Marinelli, G., et al. (1975). Geological Map of central and Southern Afar (1:500’000). CNRS/CNR..
Vinciguerra, D. (1931). Spedizione del Barone Raimondo Franchetti in Dancalia. Rettili, Batraci e Pesci. Ann. del Mus. Civ. di Stor. .. Nat. “Giacomo Doria” 55, 96–108.
Keywords: lake Afdera, Danakil Depression, Afar, bathymetry, rift, transfer zone, nodal basin, volcano-tectonic processes
Citation: Schaegis J-C, Rime V, Kidane T, Mosar J, Gebru EF, Atnafu B and Foubert A (2021) Novel Bathymetry of Lake Afdera Reveals Fault Structures and Volcano-Tectonic Features of an Incipient Transform Zone (Afar, Ethiopia). Front. Earth Sci. 9:706643. doi: 10.3389/feart.2021.706643
Received: 07 May 2021; Accepted: 17 June 2021;
Published: 01 July 2021.
Edited by:
Nico Augustin, GEOMAR Helmholtz Center for Ocean Research Kiel, GermanyReviewed by:
Finnigan Illsley-Kemp, Victoria University of Wellington, New ZealandCarolina Pagli, University of Pisa, Italy
Copyright © 2021 Schaegis, Rime, Kidane, Mosar, Gebru, Atnafu and Foubert. This is an open-access article distributed under the terms of the Creative Commons Attribution License (CC BY). The use, distribution or reproduction in other forums is permitted, provided the original author(s) and the copyright owner(s) are credited and that the original publication in this journal is cited, in accordance with accepted academic practice. No use, distribution or reproduction is permitted which does not comply with these terms.
*Correspondence: Valentin Rime, valentin.rime@unifr.ch
†These authors contributed equally to this work and share first authorship