Controlling Factors Analyses of Sediment Dispersal From Source-to-Sink Systems of Lacustrine Rift Basin: A Case Study From Paleogene Shahejie-Dongying Formations of Nanpu Sag, Eastern China
- 1College of Earth Science and Engineering, Shandong University of Science and Technology, Qingdao, China
- 2Shandong Key Laboratory of Sedimentary Mineralization and Sedimentary Minerals, Qingdao, China
Tectonic activity not only shapes the basic stratigraphic framework of rift basins, but also profoundly affects the sediment dispersal in rift basins. In this study, analyses of heavy mineral assemblages in different periods demonstrate that there are three obvious tectono-sedimentary evolutionary stages (Es3–Es2, Es1–Ed2, and Ed1, respectively) in the Paleogene provenance area of Nanpu Sag, and the volume of sand bodies increases from the bottom of the Paleogene Shahejie (Es) Formation to the top of the Dongying (Ed) Formation in Nanpu Sag. Besides, this study comprehensively utilize the analyses of seismic interpretation, palynology, heavy mineral, and borehole core samples to investigate the controlling factors of sediment dispersal in the rift basin. The assemblages of heavy minerals in different periods reflect the rock composition and catchment area of different provenance areas, and their vertical differences reflect the evolution process of the provenance area and reflect the uplift-denudation process of the provenance area. The results reveal that the synergy of the evolution of tectonic activity and the adjustment of topographical evolution are the main controlling factors of sediment dispersal in Nanpu Sag, while climate change is not the main controlling factor. We conclude that an increased sediment supply rate in the long term reflects the control of tectonic activity on basin topography, rather than climate fluctuations. The differences in morphological modification result in differential sediment dispersal, which is principally related to the differential extrusion of the fault system. The catchment area and provenance distance adjustment is evidenced by the vertical changes of heavy mineral characteristics of single-well and interaction and linkage of boundary faults, and the adjustment of topography evolution. A consideration is that the interaction and linkage of boundary faults and complex subsidence history are multi-directional, and differential evolution of provenance area is universal in lacustrine rift basins, all of this highlights the adjustment of sediment pathways generated by this characteristic of rift basins and emphasizes the importance of controlling factors analyses in understanding differential sediment dispersal that presents in the rift basins. Besides, four sets of sediment dispersal patterns were delineated based on different developmental regions in the rift basin, which are fault segmental point and multi-stage fault terrace, single-stage fault terrace and axial fault valley, axial fault terrace, and paleo-terrace and axial fault valley, respectively. This study has a certain guiding significance for the prediction of the spatial distribution of sand bodies in the rift basin and the exploration of potential oil and gas targets in the rift basin.
Introduction
The source-to-sink (S2S) system has attracted wide attention, and has currently become an important issue covered in the Earth science field. As an important component of S2S, the continental rift basin, unlike the marine rift basin, consists of multiple units or elements, a complex sediment process, various sedimentary systems, and unpredictable coupling models (Anderson et al., 2016; Bhattacharya et al., 2016; Zhu et al., 2017). Sediment dispersion characteristics and their controlling factors have always been a critical issue in geological theory and oil and gas exploration (Liang et al., 2006; He et al., 2008). For the marine rift basin, the geometry of drainage outlets can provide important constraints on source-area dimensions in deep-time source-to-sink studies, positive scaling relationships exist between drainage outlet spacing and drainage basin length, and drainage outlet cross-sectional area and drainage basin area (Chen et al., 2020). However, at present, such domestic concerns of S2S in the continental rift basin is still at an early stage. The structural characteristics of the superimposed basin are developed by episodic tectonic movement, resulting in multiple provenances, complex sedimentary facies changes, and various controlling factors for the development of sand bodies, which greatly increase the difficulty of favorable sand body prediction. Many previous studies have proposed that a series of internal and external factors control the process of sediment generation, transport, and deposition, and it is essential to distinguish the influence of these factors, i.e., tectonic activity rate, base-level cycles, and climate change, etc. (Normark, 1970; Nardin et al., 1979; Xu, 2003; Deng et al., 2004; Ren et al., 2004; Lv et al., 2019; Shi et al., 2019; Lv et al., 2020; Li et al., 2021; Liu et al., 2021). Although the tectono-sedimentary system of the rift basin has received some attention, little work has targeted provenance (Xu et al., 2009; Zhang et al., 2019; Ewa et al., 2020; Shi et al., 2021), and even fewer have focused on the relationship between sediment dispersal, climate change, tectonic activity, and topography evolution of the from source-to-sink system in the rift basin.
At present, the development of sediment dispersal patterns in the continental lacustrine rift basin mainly includes the dispersal pattern of the river valley, broken-slope, and stratigraphic sequence, respectively (Normark, 1970; Nardin et al., 1979; Xu, 2003; Deng et al., 2004; Ren et al., 2004; Liu et al., 2009). However, in the study of the dispersion of favorable sand bodies in the Paleogene strata of Nanpu Sag, it is inferred that neither the dispersal pattern of river valley, broken-slope, nor the dispersion pattern of stratigraphic sequence can properly explain the dispersion pattern of favorable sand bodies in the lacustrine rift basin. So, what factors control the dispersion pattern of favorable sand bodies in Nanpu Sag? In this study, taking the Nanpu Sag as an example, we comprehensively utilize the analyses of seismic interpretation, palynology, wireline well logging, heavy mineral, and core samples to investigate the controlling factors of sediment dispersal in the rift basin.
The results imply that the geomorphological evolution of source-to-sink in a sediment dispersion system determine the areal of the catchment area, the spacing of catchment outlets, and the type of sediments delivered from the proximal to distal part of rivers (Talling and Talling, 1997; Syvitski and Saito, 2007; Nyberg et al., 2021). The variation of source-to-sink topography in the sediment dispersal system controls the catchment area, sediment pathway, and sediment types at different regions in the basin. Previous studies on sequence stratigraphy in continental lacustrine rift basins reveal that tectonic activities are the main driving force for sequence development and evolution. By controlling sediment supply and accommodating space, tectonic activities affect the balance between the two and thus affect the sediment filling process of the rift basin (Wu, 1996; Lin et al., 2000; Lin et al., 2005; Deng et al., 2008; Sømme et al., 2009; Pechlivanidou et al., 2017). Fault activity is one of the most influential forms of tectonic activity in the rift basins. Previous studies on the control effect of fault activity on sediment dispersion have achieved fruitful results (Wang et al., 2002; Ren et al., 2004; Lin et al., 2007; Feng and Xu, 2006; Liu et al., 2019; Sangster et al., 2019; Chen et al., 2020). However, there is still no accepted pattern that can perfectly explain the controlling factors of sediment dispersion in all basins, which requires us to select typical cases for further study. In this study, the typical continental lacustrine rift basin of Nanpu Sag in the Bohai Bay Basin is selected as the research object. Through the analyses of sequence stratigraphic dispersion, development and evolution of the sedimentary system and tectonic activity in Nanpu Sag, and combined with previous studies on provenance, paleo-climate, and paleo-lacustrine characteristics in the study area, the controlling factors of differential dispersion of sediments in Nanpu Sag are clarified, to provide support for increasing oil and gas storage and production in old petroliferous areas of Eastern China, and further enrich the sedimentary geological theory of the continental lacustrine rift basin.
Geological Setting
Tectonics
The Bohai Bay Basin is one of the most eminent petroliferous basins of Eastern China (Figure 1A), which is a secondary tectonic unit in the Huanghua Depression, Northwestern Bohai Bay Basin, covering an area of 2,462 km2 (Figure 1B). It !is a Mesozoic and Cenozoic rift basin developed on the basement of the Proterozoic metamorphic rock series under the North China Platform. The northern part of the basin is bounded by the Xinanzhuang Fault and the Baigezhuang Fault, and from west to east is adjacent to the Laowangzhuang Uplift, the Xinanzhuang Uplift, the Baigezhuang Uplift, and the Matouying Uplift, respectively. The southern part is adjacent to the Shabei Fault and the Shaleitian Uplift, and the southwestern part is connected with the Qikou Sag, and the southeastern part is connected with the Bozhong Sag. It can be subdivided into Beibao, Laoyemiao, Gaoshangbao, Liuzan, Nanpu No. 1, No. 2, No. 3, and No. 4 tectonic belts (Figure 1C). The Laowangzhuang Uplift, Xinanzhuang Uplift, the Baigezhuang Uplift, Matouying Uplift, and the Shalietian Uplift located in the northern Yanshan Orogenic Belt provide sufficient provenance for the sediment filling of Nanpu Sag (Xu et al., 2006; Zhang et al., 2012; Dong et al., 2008; Cong and Zhou, 1998).
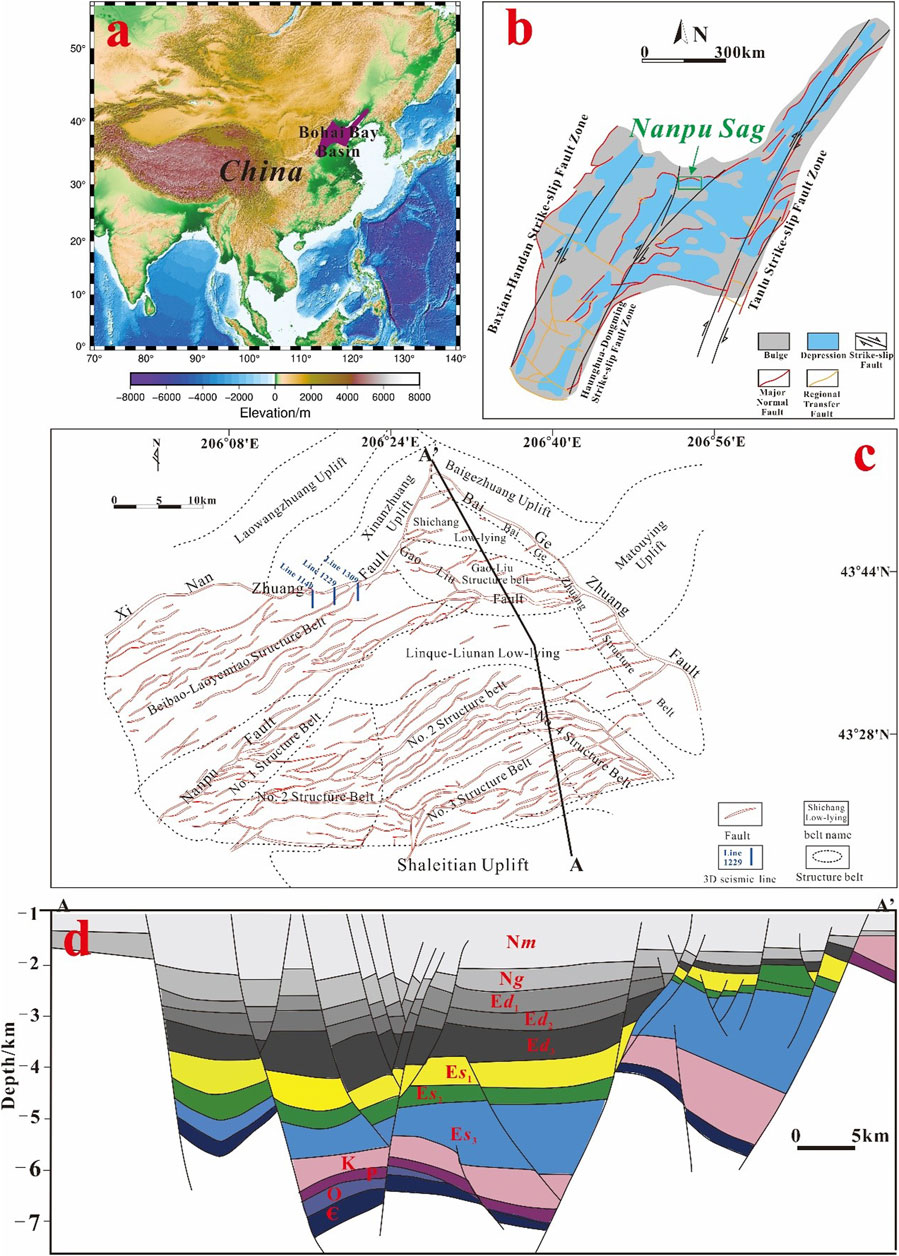
FIGURE 1. Map showing (A) the location of Bohai Bay Basin, (B) the location of Nanpu Sag, (C) the tectonic units of the study area, and (D) the SE–NW trending geological section of Nanpu Sag (Ng: Neogene Guantao Formation; Nm: Neogene Minghuazhen Formation; Ed: Paleogene Dongying Formation; Es: Paleogene Shahejie Formation; K: Cretaceous; P: Permian; O: Ordovician; €: Cambrian).
Nanpu Sag has gone through a half-graben extension fault depression period of the Es3–Es2 sedimentary period and strike-slip-extension period of the first Member of the Es Formation and Dongying (Ed) Formation (Cong and Zhou, 1998; Liu et al., 2006; Na et al., 2005). Previous studies have shown that the boundary faults in the northern part of Nanpu Sag exhibit the characteristics of early segmentation and late linkage, and the vertical fault drop in different periods also shows great differences. The temporal and spatial differences of boundary fault activity characteristics control the basin evolution and filling (Jiang et al., 2010; Shi et al., 2011; Zhang and Liu, 2012).
The gross tectono-stratigraphic architecture (Figure 1) of Nanpu Sag was described by Na et al. (2006) and Wang et al. (2012), and its age was described by Wang et al. (2012). These regional studies provide a tectono-sedimentary framework for the detailed sedimentological and provenance study presented here.
Stratigraphy
According to the analyses of drilling and seismic data, combined with the tectonic evolution background, Nanpu Sag can be divided from bottom to top into the Es1 to Es3 Member, Ed1–Ed3 Member, Guantao Formation, and Minghuazhen Formation (Figure 2) (Wang et al., 2011). Herein, the Es3 Member is a set of shore-shallow lacustrine-deep lacustrine, fan delta, and alluvial fan sedimentary strata in the early Paleogene rift. The total thickness of the Es3 Member is approximately 600–2000 m, and the overall thickness is thinning upward. The Es2 Member developed a set of sandy conglomerates with red mudstone strata, which is mainly deposited in a fan-delta system. The Es1 Member developed a set of interbedded gray-white, light gray gravel sandstone and gray, gray-green, biological limestone of fan delta-shallow lacustrine facies, which can be divided into upper and lower sections. The upper section of the Es1 Member is mainly gray-white, light gray glutenite, fine sandstone, siltstone, and light gray-dark gray mudstone, and there is a certain degree of biological limestone in the Gaoshangpu area. The lower section of the Es1 Member is a thin interbed of light gray fine sandstone, siltstone, and light gray mudstone. The Ed Formation as a whole, for a large third-order sequence cycle, is mainly distributed in the footwall of the Gaoliu Fault, according to the lithologic characteristics, and can be subdivided into Ed3, Ed2, and Ed1 Members. The Ed3 Member is at the bottom of the sequence as a system of coarse clastic alluvial fan and fan deltas. The Ed2 Member is a set of shore-shallow lacustrine-deep lacustrine-fan delta systems during lacustrine transgression. The Ed1 Member is an alluvial fan, fan delta system in the period of lacustrine basin atrophy, forming coarse-, fine-, to coarse-complete cycles (Gaun and Zhu, 2008; Zhou et al., 2009).
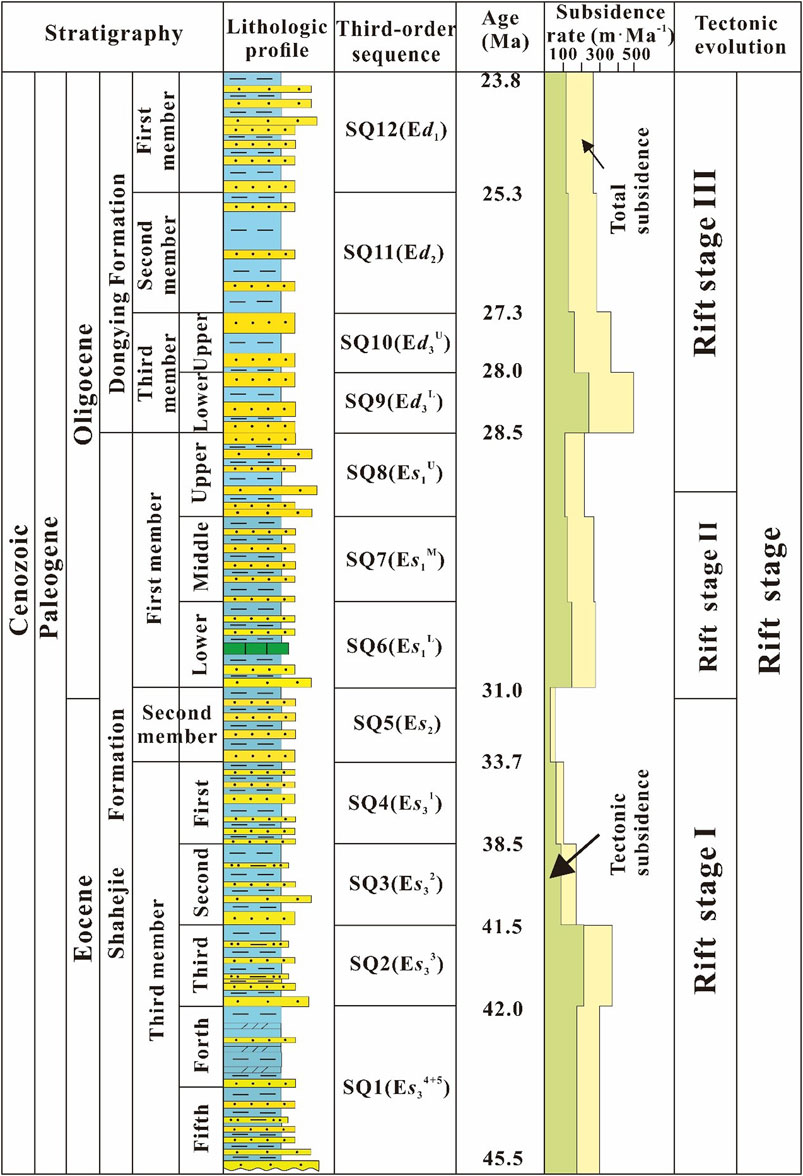
FIGURE 2. Stratigraphic nomenclature for the Paleogene of Nanpu Sag (Modified from Wang et al., 2011).
The syn-rift fill of Nanpu Sag is subdivided into three main lithostratigraphic units (Figure 2): 1) a lower group (Es3–Es2) consisting of fluvio-lacustrine deposits, 2) a middle group (Es1) consisting of fan delta-lacustrine deposits, and 3) an upper group (Ed) including fan deltas, turbidites, braided fan-deltas, and lacustrine deposits.
The age model used in this study is built based on previous work (Jiang et al., 2010; Wang et al., 2012). Accordingly, the lower group is Eocene (45.5–31.0 Ma), the middle group is early Oligocene (31.0–28.5 Ma), and the upper group is later Oligocene (28.5–23.8 Ma) (Figure 2).
Database and Methodology
Datasets
The current study is based on borehole data and relatively high-quality 3D seismic data provided by Jidong Oilfield of China National Petroleum Corporation (CNPC) in Nanpu Sag (Figure 1).
The borehole data include core data, thin sections, and heavy mineral data taken from 240 wells, as well as wireline well logging data including Gamma Ray (GR), True Formation Resistivity (RT), Density (DEN), and Compensated neutron logging (CNL). Both the core data and wireline well logging data were calibrated.
Seismic data utilized in this study covered a total area of 2,630 km2, which has been processed to zero phase, and were displayed in reversed polarity (SEG standard). The dominant frequency of the 3D seismic data is approximately 35 Hz in the interval of interest, yielding a vertical resolution of ∼15–25 m.
The sample set for the Nanpu Sag provenance study consists of subsurface core data samples collected from 25 wells, which cover every period from Es3 to Ed1, and cover the main tectonic belts including Beipu, Laoyemiao, Gaoshangpu, Liuzan, and Nanpu No. 1, No. 2, No. 3, No. 4.
Methods
Wireline log data from standard wells were used to provide age constraints on the seismic reflections and packages, to constrain the sedimentary facies and lithologies, and for creating synthetic seismograms used for the seismic-well tie. The wells also provided a quality control on thickness changes identified in the seismic data. Synthetic seismograms indicate that sandstone-dominated intervals are represented by packages of high-amplitude reflections. Fault activity was calculated based on seismic interpretation.
The sediment samples were cleaned of organic material using hydrogen peroxide, washed with deionized water, and dried. From the 0.063–2.00 mm sand fractions, as much as 50 g of each of two size fractions (0.063–0.25 mm and 0.25–0.5 mm) were weighed out for heavy mineral analyses. The samples were separated in tetrabromoethane and diluted to a specific gravity of 2.90. The heavy mineral separates were micro-split to provide about 1,000 grains. Magnetic grains were removed before the sample was mounted in epoxy cement (refractive inde×1.56) on a standard 47 × 26-mm glass slide with a coverslip. The method for examining multi-mineral populations (Garzanti and Andò, 2007) still relies on microscopic point-counting as described here. Mineral grains were identified based on optical properties with transmitted light on a petrographic microscope and occasionally supplemented by oblique illumination to view grain surface properties. Each sample slide was placed in a fixed orientation on a calibrated mechanical stage (label end in the notch).
The rock composition and catchment area of the provenance region were analyzed by vertical comparison of heavy mineral types.
Depositional system planar maps are utilized for the depositional system type and area analyses.
Results
Heavy Mineral Characteristics
The heavy mineral assemblages in different periods reflect the rock composition and basin range of the provenance area. The ZTR index of heavy mineral association refers to the sum of the content of rutile, zircon, and tourmaline in heavy minerals. The distribution of the ZTR index is of great significance to the provenance direction, abrasion degree, and sediment transport path. Besides, from the Es3 to Ed1 Member, there exists an obvious vertical difference between different intervals as follows:
1) The Es3–Es2 period, the heavy mineral assemblage of 18 samples of this period shows a dominant zircon (ranging from 16.99 to 80.35%, average on 40.96%), which is strongly consistent with the heavy mineral assemblage from the basement Archean granitic gneiss from Well Gao 25 (Figures 3, 4A, Table 1). It indicates that the provenance of this period is mainly Archean granitic gneiss coherent with the basement of the basin and Paleozoic-Mesozoic sedimentary rocks. The ZTR index exhibits a higher value with a range from 0.23 to 0.86 (average on 0.471).
2) The Es1–Ed2 period, the heavy minerals in this period are stabilized in a new assemblage, showing that it is dominated by garnet. The content of garnet of the 13 test wells varies from 23.57 to 68.59% (average: 41.63%). Compared with Es3–Es2, some new minerals, such as sphene and olandite, have emerged (Figures 3, 4B). The dispersion pattern of the abundant aluminum mineral of garnet, implies that the deeper and higher metamorphic geological bodies are the new provenance area of Nanpu Sag at this period. The emergence of new minerals such as sphene and epidote indicates the addition of new provenances, and the catchment area of the provenance is larger than that of the previous period. The ZTR index also exhibits a relatively lower value with a range from 0.17 to 0.48 (average on 0.283).
3) The Ed1 period, the heavy mineral assemblage shows the diversification of mineral types, presenting the following characteristics: 1) there are more types of heavy minerals, which are mainly garnet, and followed by zircon. Many new stable and unstable heavy minerals have appeared, and other minerals are metal oxides and altered epidote minerals (Figures 3, 4C); 2) showing obvious partitioning characteristics. The Liuzan, Gaoshangbao, Laoyemiao and Beipu tectonic belts in the north are typical orogenic provenance areas, while the Nanpu No. 1, No. 2, and No. 3 tectonic belts in the south are typical inland cratonic provenance areas. The complex and diverse types of heavy minerals indicate the diversity of provenance in this period, and the appearance of metal oxides or altered epidote minerals indicates the aging of the parent rocks in the provenance area. The ZTR index also exhibits the lowest value with a range from 0.20 to 0.30 (average on 0.254).
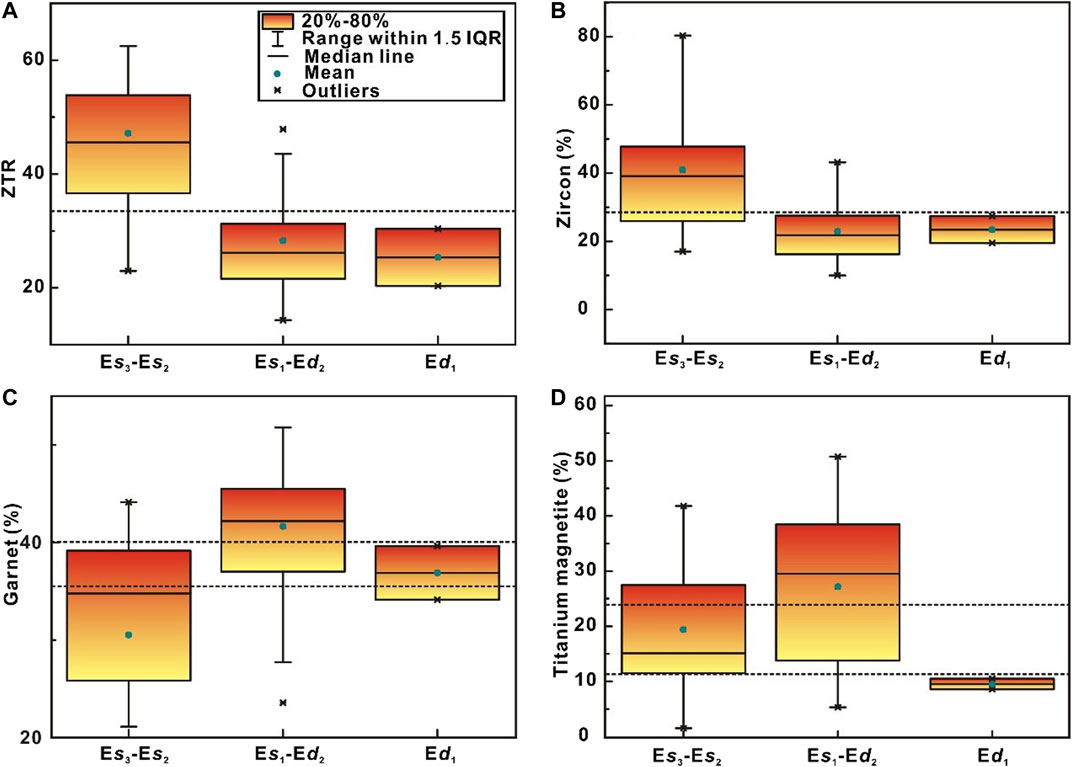
FIGURE 3. The box-plots of (A) ZTR index, the contents of (B) zircon, (C) garnet, and (D) titanium magnetite of different intervals of the Paleogene in Nanpu Sag.
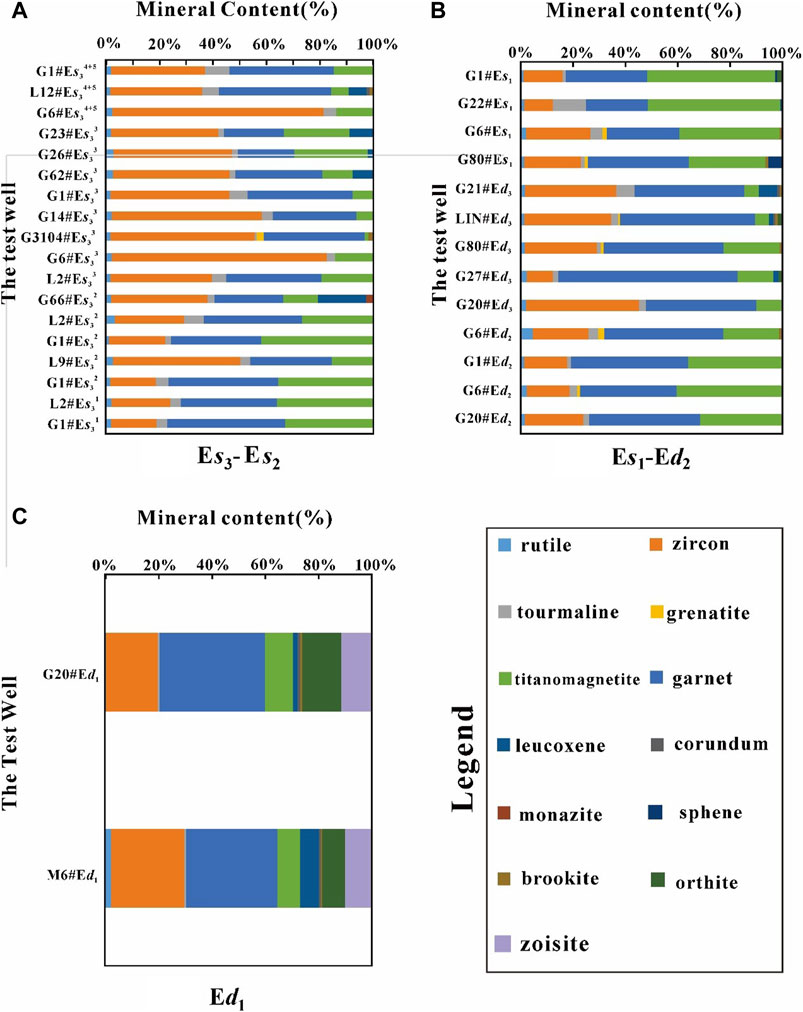
FIGURE 4. Histogram of the percentage of heavy minerals in (A) the Es3-Es2 period, (B) Es1-Ed2 period, and (C) Ed1 period.
Variations on Sediment Dispersal in Nanpu Sag
Sand body differential dispersion is a widespread phenomenon in the sediment-filling process of rift basins. Sand body differential dispersion under different geological background conditions shows different characteristics. The particularity of the sedimentary background in Nanpu Sag has such a differential dispersion of Paleogene sand bodies that emphasizes its own unique characteristics. Based on the drilling data, the characteristics of sand body distribution in the study area are defined: the development location and type of vertical fan body are relatively stable, and the size of the fan body increases continuously from the bottom of the Es Formation to the top of the Ed Formation (Figures 5–7).
The comparative analyses of sedimentary system development characteristics in different periods show that, on the one hand, the sand body types increase from the bottom of the Es Formation to the top of the Ed Formation. Sand body types are mainly fan delta and braided river delta sand bodies during the sedimentary period of the Es Formation, while the sand body types of the Ed Formation are significantly increased, and many sub-lacustrine fans and slump turbidites are developed. On the other hand, the volume of the sand body also shows an obvious trend of stable increase, which is not only manifested as a significant increase in the amplitude, but the thickness of the sand body is also increasing, especially in the sedimentary period of Ed1, showing a situation of whole basin sand (Figures 5–7).
The comparison of sand body development characteristics between different tectonic belts shows that the sand body range of the steep slope belt and gentle slope belt both are increasing from bottom to top, the sand body range of the gentle slope belt is more obvious, and finally, achieves a similar result as that in the northern steep slope belt. There are also differences in the same tectonic belt. In the northern steep slope belt, due to the steep slope and close to the provenance area, there are many coarse clastic particles, and the sediments are rapidly deposited, forming a fan delta. The fan delta sand body in the foot-wall of the Xinanzhuang Fault is characterized by strong lateral segmentation and extends far along the fault tendency direction, while the fan delta sand body in the foot-wall of the Baigezhuang Fault shows strong transverse continuity. In the southern gentle slope belt, due to the gentle slope and being farther from the provenance area, there are many fine-grained clastic particles, and sediments accumulate slowly, forming a braided river channel. Braided river delta sand bodies were relatively continuous in the early stage, had dispersion in the middle stage, and lateral connectivity in the sedimentary period of Ed1.
The Evolution of the Fault System and Reconstruction of the Catchment Area Control the Sediment Pathways and Occurrence Location of the Sand Body
From source to sink, terrigenous clastic sediments go through a variety of sediment pathways to the lacustrine rift basin. These different sediment pathways are formed due to the size of catchment area differences that control the differential dispersion of sediments. In the study area, complex geological background determines the diversification of sediment pathways and forms a variety of sand body dispersion models as follows:
1) Fault segmental point and multi-stage fault terrace
The fault segmental point refers to the segmental activity points of the boundary faults, while the multi-stage fault terrace refers to the secondary faults with parallel boundary fault strikes (Cullen et al., 2019; Tillmans et al., 2021). This kind of sediment dispersion pattern mainly develops in Laoyemiao and Gaoliu regions. The main feature is that the segmentation points formed by the segmentation activities of boundary faults serve as a drainage outlet and a provenance entrance. After the sediment enters the basin, under the control of the fault terrace formed by the secondary faults parallel to the boundary faults, the sand body is further distributed into the depocenters of the basin. Therefore, the fan extends farther along the strike of boundary faults, but has strong segmentation along the strike of those secondary faults.
As seen in Figure 8A, in the Laoyemiao area, under the control of segment activities of the Xinanzhuang Fault, a continuously developed lateral anticline was formed in the Laoyemiao area, which became the drainage outlet and provenance entrance of the continuous segment point of the Xinanzhuang Fault. Controlled by the continuous activity of the Xinanzhuang Fault, a series of secondary faults cutting lateral anticlines parallel to its strike were formed in the descending plate, and the fault anticlines perpendicular to the strike of the Xinanzhuang Fault were formed, which further pushed the sediment along the strike of the Xinanzhuang Fault to the depocenters of the basin. Due to the continuous development of segmental points and the multi-stage fault terrace in the Laoyemiao area, the inherited fan delta-slump turbidite-deep lacustrine system was developed (Figure 8A).
2) Single-stage fault terrace and axial fault valley
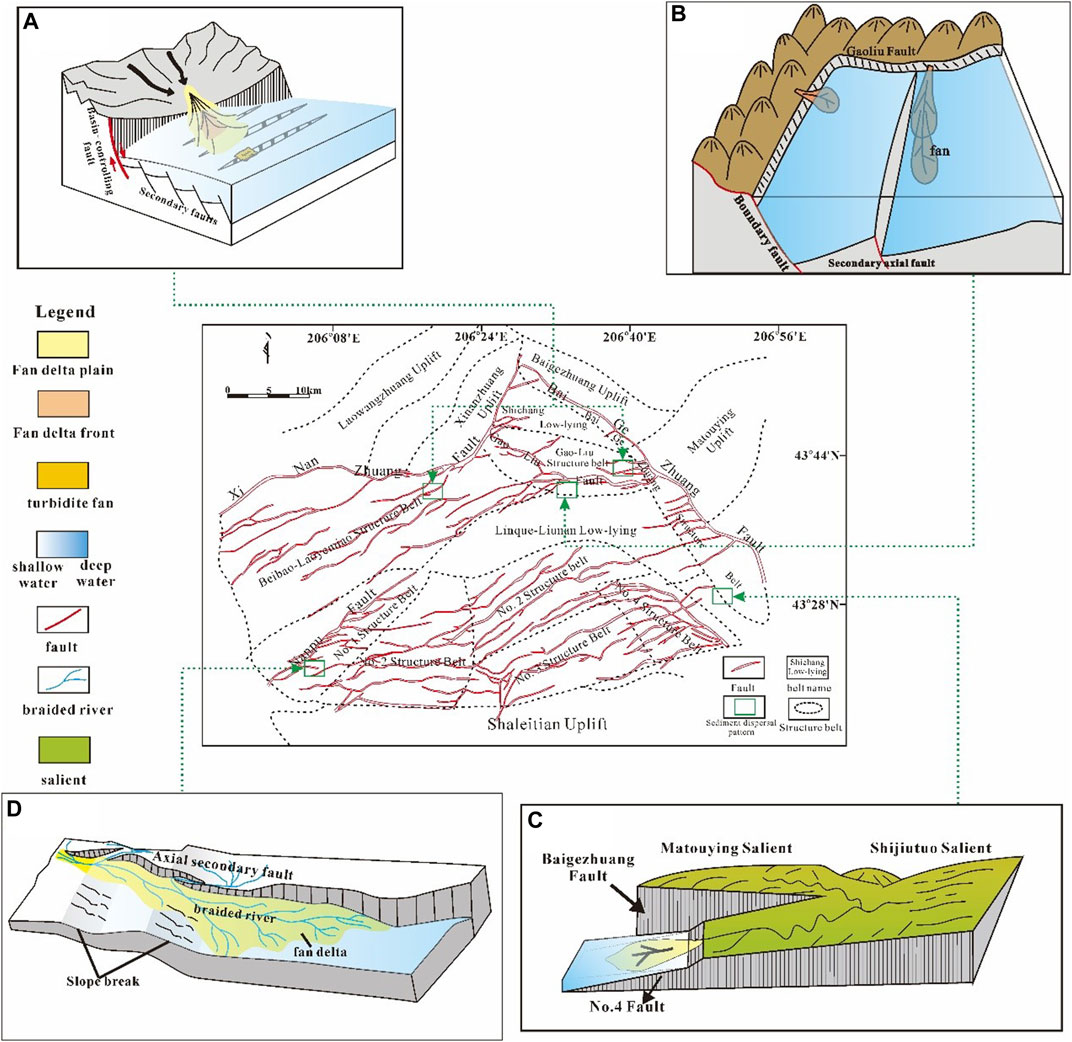
FIGURE 8. Schematic diagram of the different sediments dispersal pattern of (A) segmental point + multi-stage broken slope, (B) single-stage broken slope + axial rift valley, (C) axial broken slope, (D) paleo-slope + axial rift valley in Nanpu Sag.
An axial fault valley refers to secondary axial fractures in the depocenters of the basin (Deschamps et al., 2005; Asada et al., 2007). This sediments dispersion pattern is mainly developed on the foot-wall of the Gaoliu Fault. The dominant sand body transport channel is controlled by the combination of the active boundary faults and the secondary axial faults inside the basin. This pattern forms the synergy of a single-stage fault terrace + axial fault valley, and is mainly developed in steep terrain and deep water on the geomorphology. The severely active boundary fault served as the provenance entrance. And then the sediment was controlled by the axial fault valley after entering the basin, which mainly extended to the depocenters of the basin along the axial valley. The formed fan usually extended far, but the transverse width was limited.
In the Gaonan area, which mainly developed a replenishment channel-type gravity fan (Figure 8B), the Gaoliu Fault and the secondary axial faults within the basin control the dominant sand transport channel. Due to the violent activity of the Gaoliu Fault, the gap between the hanging wall and foot-wall of the Gaoliu Fault is large. The sediment enters the lacustrine along the Gaoliu Fault, and is further transported to the depocenters of the basin along the axial trench formed by the axial fault, thus forming the replenishment channel-type gravity fan-deep lacustrine (Figure 8B).
3) Axial fault terrace
An axial fault terrace means that the slope is formed between two faults with the same inclinations but with staggered plane positions. This sediment dispersion pattern mainly developed in the stacked part of the eastern Baigezhuang Fault and the Nanpu No. 4 Fault, and the vertical dispersion is relatively limited, mainly distributed in the sedimentary period of Ed1. The main feature is that the strike slope formed by the co-directional overlaying fault is complicated by the secondary fault to form the landform of an axial fault terrace. And is mainly developed in gentle terrain with a small topographic drop on the geomorphology. The sediment in this dispersion pattern can be transported over a long distance to form a fan body with a long extension distance.
As shown in Figure 8C, in the Nanpu No. 4 tectonic belt, the Baigezhuang Fault and Nanpu No. 4 Fault are parallel in the strike and have the same tendency, and there are stacked parts on the plane, forming a strike slope and secondary faults that obliquely cross the strike slope and complicate the strike slope, and finally develop into a broken axial slope. Sediment from the eastern Shijiutuo Uplift and western Matouying Uplift entered the lacustrine basin along the broken axial slope, and extended to the depocenters of the lacustrine basin, forming a large-scale fan delta fan body (Figure 8C).
4) Paleo-terrace and axial fault valley
This sediment dispersion pattern is mainly developed in the southern gentle slope belt, and mainly distributes in the Ed Formation vertically. Axial secondary faults in the gentle slope zone cut the paleo-terrace to form the dominant sediment transport channel. Owing to the existence of axial secondary faults, rift valleys were formed along the axial direction of the basin on the paleo-slope background of the gentle slope belt. Sediment first entered the basin where rift valleys existed, and then extended along the axial fault strike.
In the Nanpu No. 1 tectonic belt, which mainly developed a braided river delta system (Figure 8D), a series of secondary axial faults were developed in the Ed Formation under the background of paleo-terrace in the southern gentle slope belt. These faults have a drop difference in the axial direction, forming a fault terrace along the axial direction of the basin. The existence of axial faults forms low-lying areas and becomes the dominant sediment transport channel. The sand bodies extend a long distance to the depocenters of the basin along the axial fault valleys (Figure 8D).
Discussion
Controlling Factors of Sediment Differential Dispersion in Nanpu Sag
In recent years, the most prominent development of sedimentary geology in theoretical methods is the idea of “source-to-sink” (Wu et al., 2011), which believes that to truly understand the controlling factors of the differential dispersion of sediments, we must base investigations on the overall analyses of the system of “source-to-sink” to understand the fundamental factors that control sedimentation. Based on the comprehensive analyses of provenance characteristics, sedimentary characteristics, paleo-climate, and tectonic characteristics of Nanpu Sag, this study believes that the differential dispersion of sediments is the response to the increase of the catchment area in the provenance area and the temporal and spatial evolution of the tectonic activity.
The Influence of Fault Evolution on the Process of Sediment Differential Dispersal
1) Boundary fault control on geomorphological evolution of the provenance area
For closed inland lacustrine rift basins, surface runoff is the main factor controlling sediment supply, and runoff is controlled by erosion rate, catchment area, and precipitation in the provenance area. The erosion rate is controlled by tectonic activities and climatic conditions. Strong tectonic activities have caused the stratum to be uplifted and eroded, and the humid climate has more rainfall and severe erosion of surface runoff, all of those generally correspond to a higher erosion rate. Precipitation is controlled by climate. The increase in sand body volume indicates an increase in sediment supply, and sediment supply depends on the sediment rate and catchment area, while the sediment supply rate is controlled by the climate, provenance topography, and lithology (Leeder et al., 2010).
The profile analyses of the tomographic slice through the Xinanzhuang Fault shows that there are differences in fault activity rate and fault drops along the strike of the Xinanzhuang Fault (Figure 9). The activity difference of the Xinanzhuang Fault is obviously divided into four sections, which are the Nanpu No. 5 tectonic belt, Beipu Anticline, Laoyemiao Anticline, and Shichang Sub-sag, respectively. From the bottom of Es to the top of the Ed Formation, the difference of fault activity of different sections became larger and larger. By the sedimentary period of Ed1, the difference of fault activity was more obvious. The whole Xinanzhuang Fault presents a typical complete large fault with strong activity and a large drop in the central and eastern sections, and weak activity and a small drop in the Laoyemiao Anticline and Shichang Sub-sag of the eastern section (Figure 9).
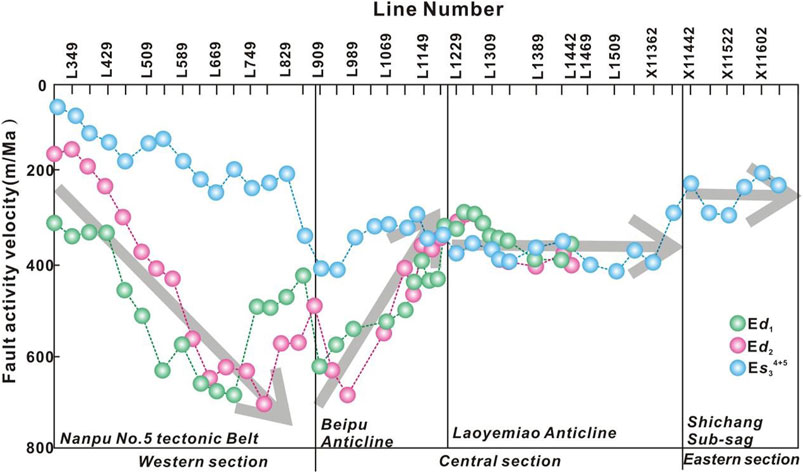
FIGURE 9. The line charts of (A) ZTR index, (B) zircon, (C) tourmaline showing the prevenance distance changes by heavy minerals analyses of single-well.
According to the above analyses (Figures 5–7), from bottom to top, there are more sand body types and the distribution amplitude increases, and the catchment area increases. Therefore, the correlation between the fault activity characteristics of the Xinanzhuang Fault and the evolution of the provenance area indicates that fault activity is an important controlling factor for the expansion of the provenance area.
2) The control of fault evolution on provenance direction, sediment transport channel, and fan type
The segmentation of the Xinanzhuang Fault and the difference in the drop along the strike control the formation and evolution of drainage outlets and provenance entrance of the Laoyemiao Anticline. The Xinanzhuang Fault has a relatively small gap on the Laoyamiao Anticline, forming a relatively low landform on the outside of the basin, which becomes a catchment area. However, due to the development of lateral folds in the Laoyamiao Anticline, the low landform in the inner basin of the Laoyamiao Anticline was formed, which became the main channel for the continuous distribution of sediment after entering the lake basin.
The extremity of the Xinanzhuang Fault controlled the development of the provenance entrance at the Beipu Anticline. The western section of the Xinanzhuang Fault is relatively gentle, shovel-shaped, and extends longer, forming a rolling anticline on the foot-wall of Xinanzhuang Fault. The extremity of the fault has a small drop, forming a catchment area. The rolling anticline inside the basin becomes a relatively high landform, which forms the main provenance entrance of the extremity of the fault in the Beipu Anticline. Different tectonic activities in different tectonic parts lead to the evolution of the terrain, thus affecting the transformation of the sediment transport channel, and fan type.
3) The influence of boundary fault evolution on the scale of sediment supply and distribution pattern of accommodating space
The evolution process of faults has an important influence on the subsidence pattern of tectonic active basins. The distribution characteristics of sedimentary-subsidence centers are largely dependent on the laws of fault activity. In the early period of the Ed Formation, the Xinanzhuang Fault had obvious segmental activity characteristics, and the sedimentary-subsidence centers were scattered, mainly distributed near the boundary faults. While in the late period of Ed Formation, as the segmental activity of the Xinanzhuang Fault gradually weakened and transformed into a unified large-scale fault, the sedimentary-subsidence center tended to be unified and migrated to the depocenters of the basin.
Climate Change is Not the Main Factor Controlling the Increase of Sediment Supply
Sporopollens are the germ cells of plants. Different plants produce different types of sporopollen assemblages, which can be used to restore ancient vegetation and paleo-climate (Zhang, 2010).
From the Es3 Member to the Ed1 Member of Nanpu Sag, the predominant sporopollen assemblages exhibit similar characteristics, with the domination of gymnosperm and angiosperm, and a small content of fern spores. The sporopollen of gymnosperms of Ed1-3 Members is dominated by Pinaceae, with the higher percentage of single- and double-beam pine pollen, which is a humid-loving wide-temperature plant and a coniferous tree, indicating the sub-high mountains environment in the northern temperate zone and cool climate conditions (Zhang, 2010; Xia et al., 2015).
The distribution of Hemlock and Cedar pollen is relatively stable among Ed1 to Ed3 intervals (ranging from 3.8 to 6.3%, and from 1.4 to 4.1%, respectively), and the relative content is low (Tables 2, 3), which both are heat-loving evergreen trees, representing a tropical-subtropical humid environment.
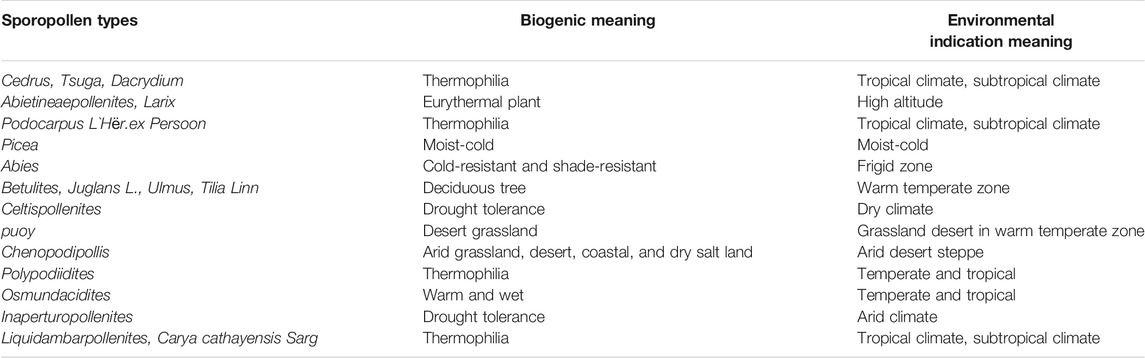
TABLE 2. Climatic and topographic characteristics reflected by pollen species in the Paleogene of Nanpu Sag.
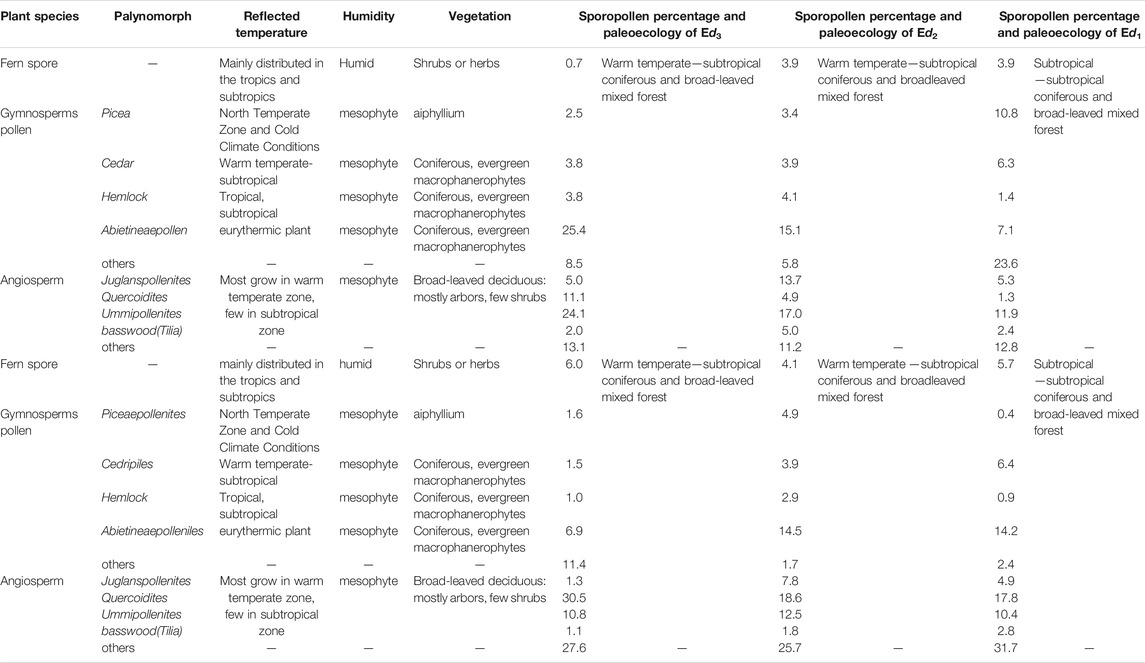
TABLE 3. Paleoclimate and paleo-vegetation reflected by the pollen assemblage of the Dongying Formation in Nanpu Sag (Some of the data were cited from Zhang, 2010).
Picea pollen was found in whole intervals of the Ed Formation (ranging from 2.5 to 10.8%), and especially in the Ed1 Member (10.8%). The Picea is an evergreen tree that prefers a cold and wet environment and is mostly distributed in areas with higher altitudes (Zhang, 2010).
Angiosperm pollen appears more in Juglanspollenites, Quercoidites, Ulmipollenites, and basswood (Tilia), with a relatively higher range of 20.9–42.2%, which indicates a humid or sub-humid climate of the warm temperate zone (Zhang, 2010).
In addition, there is a small content of sporopollen of tropical and subtropical plants, i.e., Hydraceae, Magnoliaceae, Sapindaceae, and Myrtaceae. These plants currently do not grow in North China, but mostly grow in areas south of the Yangtze River. Fern spores are rare in the Ed and Es Formations.
Compared with Ed1–3 Members, the Es1–3 Members are dominated by angiosperm pollen (66.4–71.3%), followed by gymnosperm pollen (22.4–27.9%), and the content of fern spore of Es1–3 Members also is very low (4.1–6.0%). The sporopollen of gymnosperms of the Es Formation is dominated by Pinaceae, with a higher percentage of single- and double-beam pine pollen. Pollen of angiosperms is mainly Juglanspollenites, Quercoidites, and Ulmipollenites, with a relatively higher range of 33.1–42.6%, which indicates a humid to sub-humid climate of the warm temperate zone.
From the Es3 to Ed1 Member of Nanpu Sag, the main types of sporopollen assemblages are similar. The sporopollens assemblages of the Ed1 Formation are dominated by Juglandaceae-Celtispollenites, the sporopollens assemblages of the Ed2-3 Formation are dominated by Ulmipollenites undulosus-Quercoidites-Piceaepollenites-Tsugaepollenites, the sporopollens assemblages of the Es1 Formation are dominated by Quercoidtes-Meliaceoidites, the sporopollens assemblages of the Es2 Formation are dominated by Ephedripites-Rutaceoipollis-Meliaceoidites, the sporopollens assemblages of the Es31–3 sub-member are dominated by Ulmipollenites minor-Quercoidites microhenrici. The comprehensive analyses reveal that the sporopollen of gymnosperms in the Ed and Es Formations in the Nanpu Sag reflects a warm and humid climate, which may be evergreen coniferous forest vegetation in the high mountains. Climate change has no obvious trend, which is inconsistent with the change of sand body volume (Tables 2, 3), and climate and vegetation were not the main factors controlling the increase of sediment supply.
The Influence of the Geomorphological Evolution of the Provenance Area on the Differential Dispersal of Sediments
In this study, analyses of stratigraphic heavy mineral assemblages in different periods of single-well demonstrates that there is an obvious vertical decreasing tendency in content of heavy minerals from Es34+5 to Ed1 Members, which reflects the evolution process of provenance and the uplift-erosion process (Table 1; Figure 10). As seen in Figure 10, the ZTR index, the content of zircon, and tourmaline of single-well gradually decreased from Es34+5 to Ed1 Members, indicating that the provenance distance is getting closer and the catchment area is getting smaller and smaller.
Dong et al. (2008) studied the provenance of Nanpu Sag through detrital heavy minerals. The results show that the Paleogene terrigenous clastic heavy mineral assemblage in Nanpu Sag can be divided into Es3–Es2, Es1–Ed2, and Ed1. There are not only differences in heavy mineral types, but also heavy minerals representing deeper provenance appear continuously from the bottom of Ed to the top of the Es Formation. It shows that due to continuous uplifting and erosion, the vertical deeper strata are exposed as provenance, and there are also new provenance areas in the plane, which means the increase of the provenance catchment area. There is a strong consistency between the change of catchment area and the change of sand body volume. Therefore, the increase of the catchment area in the provenance area is the main reason for the increase of the sand body range.
The analyses of the tectonic characteristics of Nanpu Sag show that the Paleogene Xinanzhuang and Baigezhuang Faults display an activity characteristic from segment to linkage. The subsidence history implies that the difference between fault blocks rises and falls to regional thermal subsidence and uplift. The unique tectonic evolution background controls the catchment area of the provenance area (Jiang et al., 2009; Wang et al., 2012). In the stage of fault segmentation, the fault scale is small, and the area of the hanging wall that can be controlled is small. In addition, in the early stage, the main local fault block rose and fell differentially, so the catchment area in the provenance area is small, and the sediment supply is small. In the interlocking period of faults, large-scale faults developed, Nanpu Sag entered the stage of regional thermal subsidence and uplift during the late Dongying Formation period, which makes the exposure and erosion of large-scale provenance possible. The catchment area increases significantly, and the sediment supply increases.
Conclusions
1) The vertical differences of heavy minerals reflect the evolution process of the provenance area and reflect the uplift-denudation process of the provenance area. Heavy minerals assemblages of different intervals of Paleogene of single-well reveal that the provenance distance is getting closer and the catchment area is getting smaller and smaller.
2) The Paleogene sediments of Nanpu Sag show obvious differential dispersion characteristics. From the bottom of the Es3 Member to the top of the Ed1 Member, the types of sand bodies increase and the range of dispersion increases.
3) Four sets of sediments dispersal patterns were proposed based on different developmental regions in the rift basin, which are fault segmental point and multi-stage fault terrace, single-stage fault terrace and axial fault valley, axial fault terrace, and paleo-terrace and axial fault valley, respectively.
4) The synergy of the evolution of tectonic activity and the adjustment of topographical evolution are the main controlling factors of sediment dispersal in Nanpu Sag, while the climate change is not the main controlling factor.
Data Availability Statement
The datasets presented in this study can be found in online repositories. The names of the repository/repositories and accession number(s) can be found in the article/Supplementary Material.
Author Contributions
All the authors have actively participated in the preparation of this manuscript. JY and HJ designed the research. These two researchers also reviewed and proofread of the manuscript. TL wrote the manuscript. ZJ collates and processes data. SW and SM drew the drawings.
Funding
This study received the support of sub-project of the National Major Oil and Gas Project “Study on Oil and Gas Enrichment Law and Forecast of Favorent Reservoirs in Nanpu Sag” (Grant Number: 2011ZX05006-006-02-002).
Conflict of Interest
The authors declare that the research was conducted in the absence of any commercial or financial relationships that could be construed as a potential conflict of interest.
Publisher’s Note
All claims expressed in this article are solely those of the authors and do not necessarily represent those of their affiliated organizations, or those of the publisher, the editors and the reviewers. Any product that may be evaluated in this article, or claim that may be made by its manufacturer, is not guaranteed or endorsed by the publisher.
Acknowledgments
We thank Jidong Oilfield of China National Petroleum Corporation (CNPC) for providing borehole data and high-quality 3D seismic data. Special thanks to Dr. Bingbing Shi at the College of Earth and Engineering, Shandong University of Science and Technology for advice on heavy mineral analyses.
Abbreviations
CNL: Compensated neutron logging; DEN: Density; GR: Gamma Ray; RT: True Formation Resistivity; ZTR: The sum of the percentage of rutile, zircon, and tourmaline in heavy minerals.
References
Anderson, J. B., Wallace, D. J., Simms, A. R., Rodriguez, A. B., and Taha, Z. P. (2016). Recycling Sediments between Source and Sink during a Eustatic Cycle: Systems of the Late Quaternary Northwestern Gulf of Mexico Basin. Earth-Science Rev. 153, 111–138. doi:10.1016/j.earscirev.2015.10.014
Asada, M., Deschamps, A., Fujiwara, T., and Nakamura, Y. (2007). Submarine Lava Flow Emplacement and Faulting in the Axial valley of Two Morphologically Distinct Spreading Segments of the Mariana Back-Arc basin from Wadatsumi Side-Scan Sonar Images. Geochem. Geophys. Geosyst. 8, a–n. doi:10.1029/2006GC001418
Bhattacharya, J. P., Copeland, P., Lawton, T. F., and Holbrook, J. (2016). Estimation of Source Area, River Paleo-Discharge, Paleoslope, and Sediment Budgets of Linked Deep-Time Depositional Systems and Implications for Hydrocarbon Potential. Earth-Science Rev. 153, 77–110. doi:10.1016/j.earscirev.2015.10.013
Chen, H., Wood, L. J., and Gawthorpe, R. L. (2020). Sediment Dispersal and Redistributive Processes in Axial and Transverse Deep‐time Source‐to‐sink Systems of marine Rift Basins: Dampier Sub‐basin, Northwest Shelf, Australia. Basin Res. 33, 227–249. doi:10.1111/bre.12462
Cong, L. Z., and Zhou, H. M. (1998). Polyphase Pulls and Aparts of Active Rift in Nanpu Depression and Their Relationship with Oil and Gas. J. Oil &Gas Geology. 19 (4), 296–301. doi:10.11743/ogg19980406
Cullen, T. M., Collier, R. E. L., Gawthorpe, R. L., Hodgson, D. M., and Barrett, B. J. (2019). Axial and Transverse Deep‐water Sediment Supply to Syn‐rift Fault Terraces: Insights from the West Xylokastro Fault Block, Gulf of Corinth, Greece. Basin Res. 32, 1105–1139. doi:10.1111/bre.12416
Deng, H., Wang, H., Wang, J., Xie, X., Su, Z., and Shang, E. (2004). Self-similarity of Constitution of Sequence Stratigraphy and Distribution of Sandbodies and Lithologic Reservoirs:taking delta-turbidite Fan System as an Example. J. Oil Gas Geology. 25 (5), 491–495. doi:10.11743/ogg20040503
Deng, H., Wang, R., Xiao, Y., Guo, J., and Xie, X. (2008). Tectono-Sequence Stratigraphic Analysis in Continental Faulted Basins. Earth Sci. Front. 15 (2), 1–7. doi:10.1016/S1872-5791(08)60024-X
Deschamps, A., Fujiwara, T., Asada, M., Montési, L., and Gente, P. (2005). Faulting and Volcanism in the Axial valley of the Slow-Spreading center of the Mariana Back Arc basin from Wadatsumi Side-Scan Sonar Images. Geochem. Geophys. Geosyst. 6, a–n. doi:10.1029/2004GC000881
Dong, Y.-x., Wang, Z.-c., Zheng, H.-j., and Xu, A.-n. (2008). Control of Strike-Slip Faulting on Reservoir Formation of Oil and Gas in Nanpu Sag. Pet. Exploration Dev. 35 (4), 424–430. doi:10.1016/s1876-3804(08)60090-7
Feng, Y. L., and Xu, X. S. (2006). Syndepositional Structural Slope-Break Zone Controls on Lithologic Reservoirs - A Case from Paleogene Bohai Bay Basin. J. Pet. Exploration Dev. 33 (01), 2–0. http://www.cpedm.com/CN/.
Garzanti, E., and Andò, S. (2007). Chapter 29 Plate Tectonics and Heavy Mineral Suites of Modern Sands. J. Dev. Sedimentology. 58, 741–763. doi:10.1016/S0070-4571(07)58029-5
Gaun, H., and Zhu, X. M. (2008). Sequence Framework and Sedimentary Facies of Ed Formation in Paleogene, Nanpu Sag, Bohai Bay Basin. J. Acta Sedimentologica Sinica. 26 (5), 730–736. doi:10.14027/j.cnki.cjxb.2008.05.012
He, J. X., Cheng, S. H., Yao, Y. J., Liu, H. L., and Wan, Z. F. (2008). Main Genetic Types of Oil & Gas and Characteristics of Their Accumulation and Distribution in North Marginal Basins,South China Sea. J. Nat. Gas Geosci. 19 (01), 34–40.
Jiang, H., Wang, H., Lin, Z. L., Fang, X. X., Zhao, S. E., and Ren, G. Y. (2009). Periodic Rifting Activity and its Controlling on Sedimentary Filling of Paleogene Period in Nanpu Sag. J. Acta Sedimentologica Sinica 27 (5), 976–982. 1000-0550(2009)05-0976-07.
Jiang, H., Wang, J. B., Zhang, L., Fang, X. X., and Yuan, S. Q. (2010). Segment Activity of Xi'nanzhuang Fault in Nanpu Sag and its Controlling on Sedimentary Process. J. Acta Sedimentologica Sinica. 28 (6), 1047–1053. doi:10.14027/j.cnki.cjxb.2010.06.004
Leeder, M. R., Harris, T., and Kirkby, M. J. (1998). Sediment Supply and Climate Change: Implications for basin Stratigraphy. Basin Res. 10 (1), 7–18. doi:10.1046/j.1365-2117.1998.00054.x
Li, J., Liu, Z., Liu, J., Chen, L., Liu, H., Huang, L., et al. (2021). Transformation of Sediment Delivery and Dispersal Patterns Controlled by Relay-Ramp Evolution along the Boundary Fault of a Lacustrine Rift: The Eocene Shahejie Formation, Dongying Sag, Bohai Bay Basin, NE China. Mar. Pet. Geology. 128, 105044. doi:10.1016/j.marpetgeo.2021.105044
Liang, X., Ye, Z., Wu, G., Zheng, H., and Liu, J. (2006). Sedimento-tectonic Features and Geological Evolution of the Poyang Basin. J. Scientia Geologica Sinica. 41 (3), 404–429. doi:10.1111/j.1745-4557.2006.00081.x
Lin, C. S., Liu, J. Y., and Zhang, Y. Z. (2005). Sequence Stratigraphy and Tectono-Stratigraphic Analysis of Tectonically Active Basins:A Case Study on the Cenozoic-Mesozoic Lacustrine Basins in China. J. Earth Sci. Front. 12 (4), 365–374. doi:10.3321/j.issn:1005-2321.2005.04.005
Lin, C. S., Pan, Y. L., Xiao, J. X., Kong, F. X., Liu, J. Y., and Zheng, H. R. (2000). Structural Slope-Break zone:Key Concept for Stratigraphic Sequence Analysis and Petroleum Forecasting in Fault Subsidence Basins. J. Earth Science-journal China Univ. Geosciences 9 (3), 260–266.
Liu, H., Loon, A. J., Xu, J., Tian, L., Du, X., Zhang, X., et al. (2019). Relationships between Tectonic Activity and Sedimentary Source‐to‐sink System Parameters in a Lacustrine Rift basin: A Quantitative Case Study of the Huanghekou Depression (Bohai Bay Basin, E China). Basin Res. 32 (4), 587–612. doi:10.1111/bre.12374
Liu, J., Tian, J. C., Zhang, X., and Wang, W. Z. (2009). Sedimentary Facies of Upper Triassic Xujiahe Formation in Maliuchang-Datachang Area, Southern Sichuan Basin. J. Lithologic Reservoirs 21 (3), 70–75.
Liu, L., Wen, H., Chen, H., Wang, Z., and Xu, W. (2021). Depositional Architectures and Evolutional Processes of Channel Systems in Lacustrine Rift Basins: The Eocene Shahejie Formation, Zhanhua Depression, Bohai Bay Basin. Mar. Pet. Geology. 131, 105155. doi:10.1016/j.marpetgeo.2021.105155
Liu, S. L., Lin, G., Zhou, Y., Gong, F. X., and Zhang, D. S. (2006). Distribution Characteristics of Elements in Sedimentary Rocks of Nanpu Sag and Their Multiple Statistics Analysis. J. Geology. Resour. 15 (3), 212–217. doi:10.13686/j.cnki.dzyzy.2006.03.009
Lv, D., Li, Z., Wang, D., Li, Y., Liu, H., Liu, Y., et al. (2019). Sedimentary Model of Coal and Shale in the Paleogene Lijiaya Formation of the Huangxian Basin: Insight from Petrological and Geochemical Characteristics of Coal and Shale. Energy Fuels, energy & fuels 33 (11), 10442–10456. doi:10.1016/j.marpetgeo.2019.10404110.1021/acs.energyfuels.9b01299
Lv, D. W., Song, Y., Shi, L. Q., Wang, Z, L., Cong, P. Z., and Van Loon, A. J. (2020). The Complex Transgression and Regression History of the Northern Margin of the Palaeogene Tarim Sea (NW China), and Implications for Potential Hydrocarbon Occurrences. Mar. Pet. Geology. 112 (104041), 1–17. doi:10.1016/j.marpetgeo.2019.104041Mueller
Nardin, T. R., Hein, F. J., Gorsline, D. S., and Edwards, B. D. (1979). A Review of Mass Movement Processes, Sediment and Acoustic Characteristics, and Contrasts in Slope and Base-Of-Slope Systems versus Canyon-fan-basin Floor Systems. Geology. Continental Slopes 27, 61–73. doi:10.2110/pec.79.27.0061
Nyberg, B., Helland‐Hansen, W., Gawthorpe, R., Tillmans, F., and Sandbakken, P. (2021). Assessing First‐order BQART Estimates for Ancient Source‐to‐sink Mass Budget Calculations. Basin Res. 33, 2435–2452. doi:10.1111/bre.12563
Pechlivanidou, S., Cowie, P. A., Hannisdal, B., Whittaker, A. C., Gawthorpe, R. L., Pennos, C., et al. (2017). Source-to-sink Analysis in an Active Extensional Setting: Holocene Erosion and Deposition in the Sperchios Rift, central Greece. Basin Res. 30 (3), 522–543. doi:10.1111/bre.12263
Ren, J. Y., Lu, Y. C., and Zhang, Q. L. (2004). Forming Mechanism of Structural Slope-Break and its Control on Sequence Style in Faulted basin. J. Earth Sci. 29 (5), 596–602. doi:10.1016/S0960-0779(03)00420-X
Sangster, C., Piper, D. J. W., Hawie, N., Pe‐Piper, G., and Saint‐Ange, F. (2019). Forward Stratigraphic Modelling of Sediment Pathways and Depocentres in Salt‐influenced Passive‐margin Basins: Lower Cretaceous, central Scotian Basin. Basin Res. 31 (4), 728–753. doi:10.1111/bre.12342
Shi, B., Chang, X., Liu, Z., Pang, Y., Xu, Y., Mao, L., et al. (2021). Intelligent Identification of Sequence Stratigraphy Constrained by Multipopulation Genetic Algorithm and Dynamic Time Warping Technique: A Case Study of Lower Cretaceous Qingshuihe Formation in Hinterland of Junggar Basin (NW China). Basin Res. 33, 2517–2544. doi:10.1111/bre.12567
Shi, B., Chang, X., Yin, W., Li, Y., and Mao, L. (2019). Quantitative Evaluation Model for Tight sandstone Reservoirs Based on Statistical Methods - A Case Study of the Triassic Chang 8 Tight Sandstones, Zhenjing Area, Ordos Basin, China. J. Pet. Sci. Eng. 173, 601–616. doi:10.1016/j.petrol.2018.10.035
Shi, G. Z., Wang, H., Xu, B., and Jiang, H. (2011). Activity of Baigezhuang Fault of Nanpu Depression and its Controlling on Sedimentation. J. Acta Scientiarum Naturalium Universitatis Pekinensis. 47 (1), 85–90. doi:10.13209/j.0479-8023.2011.013
Sømme, T. O., Helland-Hansen, W., Martinsen, O. J., and Thurmond, J. B. (2009). Relationships between Morphological and Sedimentological Parameters in Source-To-Sink Systems: a Basis for Predicting Semi-quantitative Characteristics in Subsurface Systems. Basin Res. 21, 361–387. doi:10.1111/j.1365-2117.2009.00397.x
Syvitski, J. P. M., and Saito, Y. (2007). Morphodynamics of Deltas under the Influence of Humans. Glob. Planet. Change 57 (3-4), 261–282. doi:10.1016/j.gloplacha.2006.12.001
Talling, K., and Talling, P. (1997). Geomorphic Evidence for Tear Faults Accommodating Lateral Propagation of an Active Fault-bend Fold, Wheeler Ridge, California. J. Struct. Geol. 19 (3), 397–411. doi:10.1016/S0191-8141(96)00089-2
Tillmans, F., Gawthorpe, R. L., Jackson, C. A. L., and Rotevatn, A. (2021). Syn‐rift Sediment Gravity Flow Deposition on a Late Jurassic Fault‐terraced Slope, Northern North Sea. Basin Res. 33, 1844–1879. doi:10.1111/bre.12538
Wang, E., Liu, G., Pang, X., Wu, Z., Li, C., Bai, H., et al. (2020). Sedimentology, Diagenetic Evolution, and Sweet Spot Prediction of Tight sandstone Reservoirs: A Case Study of the Third Member of the Upper Paleogene Shahejie Formation, Nanpu Sag, Bohai Bay Basin, China. J. Pet. Sci. Eng. 186, 106718. doi:10.1016/j.petrol.2019.106718
Wang, H., Jiang, S., Huang, C., Jiang, H., and Gan, H. (2011). Differences in Sedimentary Filling and its Controlling Factors in Rift Lacustrine Basins, East China: A Case Study from Qikou and Nanpu Sags. Front. Earth Sci. 5 (1), 82–96. doi:10.1007/s11707-011-0159-0
Wang, H., Zhao, S. E., Lin, Z. L., Jiang, H., Huang, C. Y., Liao, Y. T., et al. (2012). The Key Control Factors and its Petroleum and Geological Significance of Extra-thick Deposition in Dongying Formation, Nanpu Sag. J. Earth Sci. Front. 19 (1), 108–120. 1005-2321(2012)01-0108-13.
Wang, Y. M., Liu, H., Li, L. C., Qi, X. F., and Wang, Y. (2002). Types and Distribution Characteristics of Slope Breaks of Large-type Down-Warped Lake Basins. J. Earth Science-journal China Univ. Geosciences 27 (6), 683–688. doi:10.1007/s11769-002-0045-5
William R. Normark, W. R. (1970). Growth Patterns of Deep-Sea Fans. Bulletin 54 (11), 2170–2195. doi:10.1306/5D25CC79-16C1-11D7-8645000102C1865D
Wu, G. Y. (1996). Tectonic Sequence Stratigraphy. J. Adv. Earth Sci. 11 (3), 310–313. doi:10.11867/j.issn.1001-8166.1996.03.0310
Wu, Y. Y., Zou, C. N., Hu, S. Y., Li, Q., Zhang, T. S., and Guo, B. C. (2011). New Advances in Sedimentology and Sequence Stratigraphy of Foreland basin. J. Oil Gas Geology. 32 (4), 606–614. doi:10.11743/ogg20110415
Xia, X. F., Zhang, N., Yu, J. X., and Yi, Ceng. (2015). Eocene-oligocene Palynology and Biostratigraphic Correlation in the Nanpu Sag, Bohai bay basin, N. China. J. Acta Micropalaeontologica Sinica. 32 (3), 269–284. CNKI:SUN:WSGT.0.2015-03-005.
Xu, A. N., Dong, Y. X., Han, D. K., Y, F. C., Huo, C. L., Wang, Z. C., et al. (2009). Integrated Description and Evaluation of Reservoirs Based on Seismic, Logging, and Geological data:Taking Dongying Formation Member 1 Oil Reservoir of No. 1 Structure, Nanpu Sag as an Example. J. Pet. Exploration Dev. 36 (5), 541–551. doi:10.1016/S1876-3804(09)60146-4
Xu, A. N., Zheng, H. J., Dong, Y. X., Wang, Z. C., Yin, J. F., and Yan, W. P. (2006). Sequence Stratigraphic Framework and Sedimentary Facies Prediction in Dongying Formation of Nanpu Sag. J. Pet. Exploration Dev. 33 (4), 437–443. 1000-0747(2006)04-0437-07.
Xu, C. G. (2003). Controlling Sand Principle of Source to Sink Coupling in Time and Space in continental Rift basins:Basic Idea, Conceptual Systems and Controlling Sand Models. J. China Offshore Oil Gas 025 (004), 1–11. CNKI:SUN:ZHSD. 0.2013-04-002.
Zhang, C. M., and Liu, X. F. (2012). The Boundary Faults and basin-formation Mechanism of Nanpu Sag. J. Acta Petrol. Sinica 33 (4), 581–587. doi:10.7623/syxb201204006
Zhang, J., Gao, J., Wu, J., Lyu, Q., and Fang, D. (2019). Sedimentary Characteristics and Seismic Geomorphology of the Upper Third Member of Eocene Dongying Formation in Double Slope Systems of Laoyemiao Transverse Anticline, Nanpu Sag, Bohai Bay Basin, China. Mar. Pet. Geology. 109, 36–55. doi:10.1016/j.marpetgeo.2019.06.005
Zhang, Y. F. (2010). Research on the Palynoflora from the Paleogene Dongying Formation in Nanpu Sag, Hebei Province. DO thesis. Beijing): China University of Geosciences.
Zhou, T. W., Zhou, J. X., Dong, Y. X., Wang, X. D., and Chang, H. W. (2009). Formation Mechanism of Cenozoic Fault System of Nanpu Sag in Bohai Bay Basin. J. J. China Univ. Petroleum(Edition Nat. Science) 33 (1), 12–17. doi:10.1016/S1874-8651(10)60080-4
Keywords: sediment dispersal, catchment area, sediments pathways, fault activity, source to sink system, nanpu sag
Citation: Yu J, Liu T, Jia H, Jiang Z, Wei S, Li Y and Ma S (2021) Controlling Factors Analyses of Sediment Dispersal From Source-to-Sink Systems of Lacustrine Rift Basin: A Case Study From Paleogene Shahejie-Dongying Formations of Nanpu Sag, Eastern China. Front. Earth Sci. 9:716176. doi: 10.3389/feart.2021.716176
Received: 28 May 2021; Accepted: 31 August 2021;
Published: 20 September 2021.
Edited by:
Luca Mao, University of Lincoln, United KingdomReviewed by:
Hancheng Ji, China University of Petroleum, ChinaPiotr Gębica, University of Rzeszow, Poland
Copyright © 2021 Yu, Liu, Jia, Jiang, Wei, Li and Ma. This is an open-access article distributed under the terms of the Creative Commons Attribution License (CC BY). The use, distribution or reproduction in other forums is permitted, provided the original author(s) and the copyright owner(s) are credited and that the original publication in this journal is cited, in accordance with accepted academic practice. No use, distribution or reproduction is permitted which does not comply with these terms.
*Correspondence: Haibo Jia, jiahaibohb@163.com