Tidal Modulation of Hydrothermal Tremor: Examples From Ischia and Campi Flegrei Volcanoes, Italy
- 1Istituto Nazionale di Geofisica e Vulcanologia, Sezione di Napoli—Osservatorio Vesuviano, Naples, Italy
- 2Instituto Dom Luiz (IDL), University of Beira Interior, Covilhã, Portugal
Volcano dynamics results from an interplay between internal and external processes spanning different time scales. Unravelling how such processes interact may provide key insights into the mechanisms that may lead to the destabilization of the volcanoes and eruption, a critical information to forecast hazards. Studies dealing with tidal influence on volcanoes fall within this context, yet the cause-effect relationship between tides and eruptions is still poorly understood. In the present study, we investigate the tidal influence on two nearby volcanoes, Ischia and Campi Flegrei (Italy), characterized by intense hydrothermal activity. We analyze the seismic tremor of hydrothermal origin recorded by four seismic stations between January and June 2020 by using Singular Spectrum Analysis. We detect up to five long term tidal periodicities ranging from ∼5 to ∼29 days. The results indicate that the seismic tremor is modulated by Earth tides at both volcanoes. In addition, differences in phase and amplitude modulation between the response of both hydrothermal systems to tidal forcing reveal specific features related to the tremor source and to properties of the surrounding medium. These phenomena indicate an interplay between solid Earth and the dynamics of these two volcanoes. Similar approaches on hydrothermal systems at volcanoes would contribute to better characterize the hydrothermal circulation and their evolving conditions that may represent a precursor of a new phase of activity.
Introduction
Tidal influence has been evidenced at many volcanoes through the analysis of geological, geophysical, and geochemical time-series acquired during unrest and eruptive phases such as earthquake rates, frequency of explosive and/or eruptive events, volcanic tremor, gas, and lava fluxes (Dzurisin, 1980; McNutt and Beavan, 1987; Sottili et al., 2007; Sottili and Palladino, 2012; Girona et al., 2018; Dumont et al., 2020, 2021; Sahoo et al., 2021; Sottili et al., 2021). Some quiescently active volcanoes like Wakamiko Caldera (Japan) and Santorini (Greece), where activity can be reduced to degassing and/or seismicity, seem also to be sensitive to Earth tides (Yakiwara et al., 2013; Contadakis et al., 2017). At open volcanic systems for instance, gas emissions are mainly evacuated through the open conduit. Such passive gas emissions as at Llaima, Villarrica (Chile), and Cotopaxi (Ecuador) volcanoes have shown an oscillating pattern including tidal periodicities (Bredemeyer and Hansteen, 2014; Dinger et al., 2018). More generally, circulation of hydrothermal fluids affects wide areas, taking diverse forms such as fumaroles, diffuse degassing, and inducing rock alteration. This circulation can be tracked by gas measurements but also by seismic tremor. Monitoring hydrothermal activity at quiescently active volcanoes can provide insights on the dynamics of the underlying magmatic plumbing system (Battaglia et al., 2006; Gottsmann et al., 2007; Miller et al., 2017; Magee et al., 2018; Eichelberger et al., 2020). By studying the interplay between the hydrothermal system and external forcing such as Earth tides, we tackle processes that may lead to the destabilization of the volcanic system and eruption triggering. Actually, by inducing a global mass reorganization on Earth on different time and space scales for both solid and fluid envelopes (Nakiboglu and Lambeck, 1980), solid Earth and ocean tides participate in changing the stress, strain, or pore-fluid pressure conditions that may be critical in dynamical systems such as volcanoes and faults (Jupp et al., 2004; Scholz et al., 2019; Dumont et al., 2022). This is illustrated by studies that have shown a correlation between the onset of volcanic eruptions and the lunar cycle for instance, e.g., Mauk and Johnston (1973); Dzurisin, (1980), or a sensitivity to Earth tides that appears with migration of magma towards the surface or when the systems get closer to a critical state (Berrino and Corrado, 1991; De Lauro et al., 2013; Girona et al., 2018; Miguelsanz et al., 2021). However, such a response of volcanoes to tidal forcing has not been systematically reported, leaving many questions unsolved.
Here, we consider two volcanoes, Ischia island and Campi Flegrei caldera (Italy), characterized by different forms of hydrothermal activity. By analyzing and comparing the hydrothermally generated seismic tremor for two sites located in each volcano, we aim at gaining new insights on the conditions leading volcanic and hydrothermal systems to respond to tidal forces and therefore on those prone to destabilize them. We used time-series of the seismic tremor recorded between January and June 2020. For each site, we calculated the Root Mean Square (RMS) of the signal in the frequency bands recognized as of hydrothermal origin (De Lauro et al., 2013; Chiodini et al., 2017; Falanga et al., 2021). We applied Singular Spectrum Analysis (SSA) to detect any periodicities present in the time-series. We compared them to those extracted in the length-of-day (l.o.d.) to confirm their tidal origin, as l.o.d. represents a measure of the tidal action on the Earth’s rotation velocity (Lambeck 2005; Le Mouël et al., 2019). The results indicate that the seismic tremor at both volcanoes is partly modulated by Earth tides; this modulation in phase and amplitude seems to vary with the nature of hydrothermal activity and underlying medium.
Geological Setting
We investigate the tidal influence on two quiescently active coastal volcanoes characterized by intense hydrothermal activity, Ischia island and Campi Flegrei. Both volcanoes belong to the Phlegraean Volcanic District, Southern Italy. The island of Ischia is located in the westernmost part of the Phlegraean Volcanic District representing the subaerial portion of a larger volcanic system. Its activity started around 150 kyr, including eight eruptions over the last 2 kyr and is characterized by both effusive and explosive behaviors (de Vita et al., 2010). The most impacting eruption at Ischia was that of the Green Tuff of Mt. Epomeo, which occurred 55 kyr ago. This eruption caused the collapse of the central sector of the island and was followed by the resurgence of a polygonal block on the northern sector that represents the main source of seismicity on the island. In the last few years, seismicity has been generally low, except for a Mw 3.9 earthquake that occurred on August 21, 2017 along the border of Mt. Epomeo (Trasatti et al., 2019; Cusano et al., 2020a, 2020b). Ischia is affected by significant ground deformation. Presently, a slow subsidence, reflecting a gradual depressurization of the magmatic/hydrothermal system beneath Mt. Epomeo is observed (Ricco et al., 2019). The interactions between volcano-tectonic dynamics, ground deformation, and under-ground fluid circulation have been recognized as resulting from a complex geothermal system. Deep hot fluids rise from distinct superimposed hydrothermal reservoirs, in the northern and southern part of the island (Di Napoli et al., 2009), with temperatures from 150°C to 270°C and depths ranging from 150 to 1000 m b.s.l. In the shallow aquifer, they mix with meteoric and marine waters after which they rise to the surface along high-permeability pathways, e.g., fractures and faults, inducing surface manifestations such as fumarolic activity and thermal springs. This persistent hydrothermal activity leaves its fingerprints in the background seismic signal (Cusano et al., 2020b; Falanga et al., 2021). Recent studies have shown that the interaction of the circulating fluids within the rock matrix generates self-sustained oscillations observed as seismic tremor in the 1–2 Hz frequency band (Cusano et al., 2020b; Falanga et al., 2021).
The Campi Flegrei caldera, located to the west of the town of Naples, was generated by two major collapses, the Campanian Ignimbrite (∼39 kyr), and the Neapolitan Yellow Tuff (∼15 kyr). Campi Flegrei is subjected to ground deformation consisting of alternating phases of uplift and subsidence (Isaia et al., 2009; Del Gaudio et al., 2010). This typical activity is known as bradyseism. The deformation is centered in the town of Pozzuoli and gradually decreases towards the caldera margins. The most relevant bradyseismic crises occurred in 1969–1972 and 1982–1984, with a maximum vertical deformation of about 1.8 m. Uplifts are related to both magmatic and hydrothermal sources due to either the pressurization of a magmatic gas reservoir at 3–4 km depth, or caused by repeated CO2-rich fluid injections from the deeper reservoir into the shallow (∼2 km depth) hydrothermal system (Amoruso et al., 2014; Chiodini et al., 2017; Gresse et al., 2017; Petrosino and De Siena, 2021). The hot fluids (mainly H2O and CO2) exsolve from a deep magmatic body and then mix with meteoric components in the hydrothermal reservoir (Gresse et al., 2017). A large amount of hot hydrothermal fluids are released at the surface through diffuse degassing and fumaroles as well as boiling mud pools located at the Solfatara crater and Pisciarelli fumarolic field, which are the most thermally active areas of the caldera (Chiodini et al., 2017; Gresse et al., 2017; Young et al., 2020). This hydrothermal activity within Campi Flegrei is concentrated along fault zones, where highly fractured rocks facilitate fluid escape.
Uplift episodes are accompanied by seismic swarms of volcano-tectonic (VT) seismicity (Bellucci Sessa et al., 2021) and, less frequently, by long-period (LP) events (Saccorotti et al., 2007; Cusano et al., 2008). The majority of the earthquakes are located at shallow depths (up to 4 km b.s.l) beneath the Solfatara-Pozzuoli area. VTs are generated by brittle shear failure mechanisms induced by the pressurization of the hydrothermal system and fluid flow towards the surface (Saccorotti et al., 2007; De Lauro et al., 2016). LPs are caused by the acoustic resonance of cracks filled by a water-gas mixture of hydrothermal origin (Cusano et al., 2008; De Lauro et al., 2012; Falanga and Petrosino, 2012). Geothermal activity is the source of hydrothermal seismic tremor which shares the same frequency content (0.4–1 Hz) as LP events (De Lauro et al., 2013). In addition, at the Pisciarelli fumarolic field, shallow surface degassing and a boiling mud pool generate high frequency (5–15 Hz) tremor (Chiodini et al., 2017), which is only recorded at nearby stations.
The influence of Earth tides at the Campi Flegrei volcanic system has been evidenced on different time scales. Periodicities matching short (semidiurnal, diurnal) to long (fortnightly, monthly) term tidal constituents have been detected in several geophysical data: gravity changes (Berrino and Corrado, 1991), ground deformation (De Lauro et al., 2018; Ricco et al., 2019; Petrosino et al., 2020), ground temperature (Caputo et al., 2020; Cusano et al., 2021), LP and VT earthquake rates and energy (De Lauro et al., 2012; Petrosino et al., 2018), and hydrothermal tremor (De Lauro et al., 2013).
Data and Methods
Seismic data (Supplementary Figures S1, S2) were collected at four three-component seismic stations, two (CPIS and ASBG) installed at Campi Flegrei, and two (IOCA and T1367) at Ischia island (Figure 1). CPIS, ASBG, and IOCA are equipped with Guralp CMG 40T (60s) broadband seismometers; T1367 with a Lennartz LE-3Dlite (1 Hz) velocimeter. The CPIS station is located at the Pisciarelli site, near a boiling mud pool which is the shallow source of a high-frequency (5–15 Hz) continuous fumarolic tremor (Chiodini et al., 2017). The ASBG station is located inside the natural oasis of the Astroni tuff cone; in this site the instrument records low-frequency (0.4–1 Hz) tremor sourced from the hydrothermal activity in the nearby Solfatara crater (De Lauro et al., 2013). The two seismic stations deployed at Ischia are installed in the northern part, in the Casamicciola town area that was mostly struck by the earthquake of August 21, 2017. Hydrothermal activity at Ischia consists of shallow fluid circulation that generates a seismic tremor whose fundamental mode is centered on the 1–2 Hz band (Cusano et al., 2020a, 2020b; Falanga et al., 2021).
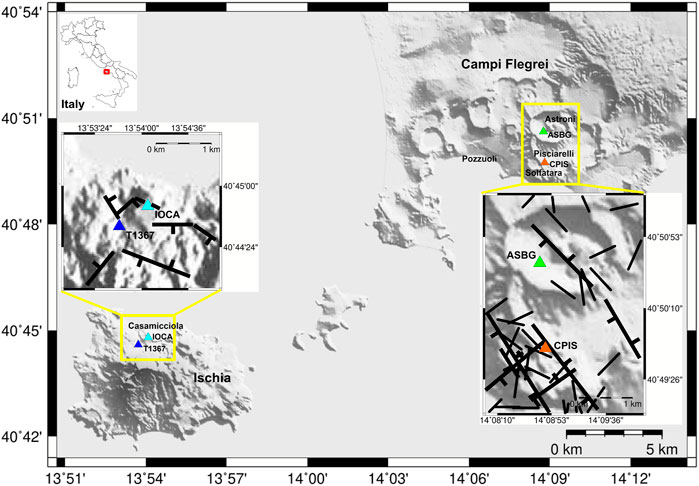
FIGURE 1. The studied areas (indicated by the red box on the map in the upper left corner) with the location of the seismic stations (triangles). The two insets corresponding to yellow boxes show the fault (black half-tick lines) and fracture distributions (black lines) at Campi Flegrei (Vitale and Isaia, 2014) and Ischia island (Di Giuseppe et al., 2017).
Data were recorded during the first 6 months of 2020 which was a particularly calm period. In fact, on 8 March, Italy started the lockdown period due to the COVID-19 pandemic (Falanga et al., 2021), so the anthropogenic seismic noise was strongly reduced as a consequence of the restrictions imposed to the human activities for containing the pandemic. A preliminary check of the signal gaps due to possible sensor breakdowns was performed; to reduce such gaps, the following intervals were considered for each station:
- CPIS: 1 Jan to 19 Apr (109 days)
- ASBG: 15 Feb to Jun 30, 2020 (136 days)
- IOCA: 1 Jan to Jun 29, 2020 (180 days)
- T1367: 24 Mar to Jun 8, 2020 (75 days)
The seismic tremor of hydrothermal origin was extracted from the raw data by filtering the waveforms in different frequency bands, depending on the stations under consideration: 1–2 Hz band for both Ischia stations, 5–15 Hz for CPIS, and 0.4–1 Hz for ASBG. The frequency bands were chosen on the basis of past studies and correspond to the frequency range in which hydrothermal tremor has been observed (De Lauro et al., 2013; Chiodini et al., 2017; Falanga et al., 2021). Then, we calculated the hourly RMS of the amplitude of the filtered signal hereinafter simply referred to as seismic tremor (De Lauro et al., 2013; Cusano et al., 2020b). As the squared amplitude of a seismic signal is proportional to its energy (Lay and Wallace, 1995), the RMS gives a measure of the energy release. For each station, RMS values were averaged over the three directions of motion.
We also considered the time-series of the length-of-day (l.o.d), a global parameter measured on Earth, that records the Earth’s rotation velocity whose short-term variations express the tidal action on Earth (Lambeck, 2005; Le Mouël et al., 2019). We used the daily measurements of l.o.d. from the EOP14C04 dataset covering the 1 January to June 30, 2020 interval and provided by the International Earth Rotation Service (IERS, Paris, France, (https://www.iers.org /IERS /EN /DataProducts /EarthOrientationData /eop.html).
Using a similar approach as Dumont et al. (2020), we applied Singular Spectrum Analysis (SSA) to the seismic tremor and l.o.d time-series. SSA is a non-parametric time-series analysis technique that has been used for a large variety of geophysical datasets (e.g., Bozzo et al., 2010; Lopes et al., 2017; Le Mouël et al., 2019). It allows the decomposition of time-series into a sum of small numbers of components such as slowly varying trend, (pseudo) oscillations, and noise. The algorithm is based on a two-step approach: first, the building of the trajectory matrix based on lagged copies of the original time-series; second, the decomposition using the Singular Value Decomposition (SVD) algorithm. Families of eigenvectors and eigenvalues are then identified through their behavior (pseudo/non-oscillatory) and periods obtained from the Fourier transform, allowing to determine the different components present in the signal. Eigenvalues are ranked in the decreasing order of amplitude (Supplementary Figure S3), allowing us to focus on the components contributing the most to the original time-series. These components extracted in the seismic tremor were then compared to those extracted in the l.o.d and the tidal periodicities listed in Ray and Erofeeva (2014), to identify them as tidal constituents. More on SSA method and applications can be found in Golyandina and Zhigljavsky (2013), Bozzo et al. (2010), Lopes et al. (2017), Le Mouël et al. (2019), and Dumont et al. (2020, 2021).
Results
At Campi Flegrei, the seismic tremor was simultaneously recorded at ASBG and CPIS stations over ∼2 months. Their variations do not exceed 5 μm/s (Figure 2A). Besides, the temporal variations of the seismic tremor amplitude at both stations are relatively different, except late February-early March 2020 when the amplitude of the seismic tremor shows relative higher and sustained values at both sites (Figure 2A). These differences are also expressed by their spectral content (Figure 2D) that also reflects the different frequency bands used to filter them (Figure 2D). On the contrary, at Ischia island (where simultaneous recordings are available over slightly more than 2 months), the seismic tremor variations at IOCA and T1367 sites are much more similar and also smaller, e.g., about half an order than those recorded at Campi Flegrei (Figures 2B,E). The seismic tremor measured at T1367 shows lower amplitude variations, that are about half of that at the IOCA station. Over this first half of 2020, the l.o.d variations do not exceed 2 ms, showing a declining trend from late April 2020 (Figure 2C and Supplementary Figure S4). A first-order comparison of the spectral content of these different time-series suggests that the seismic tremor of IOCA shares more common features with that of the l.o.d. (Figure 2E).
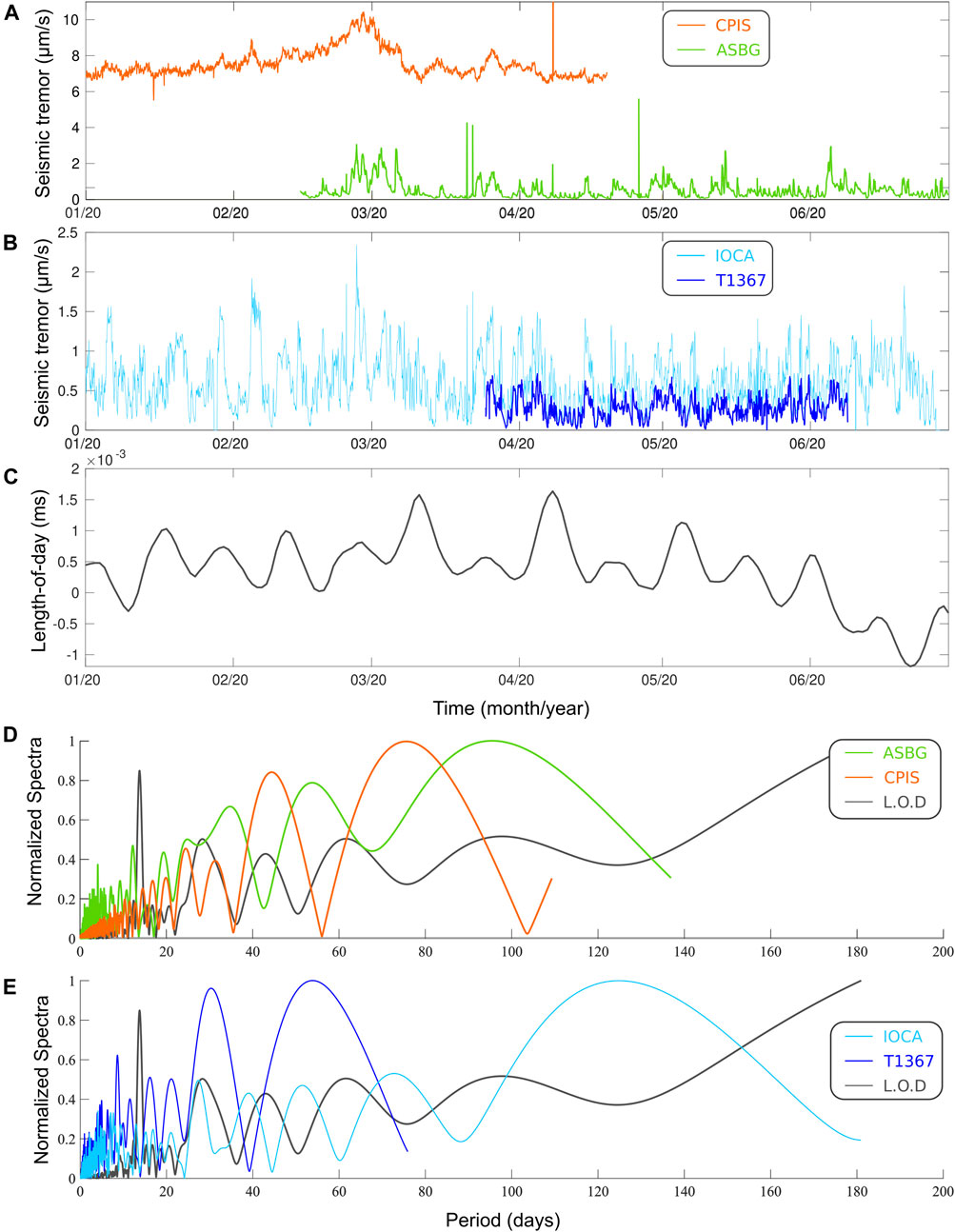
FIGURE 2. RMS amplitude of the seismic tremor at Campi Flegrei (A) and Ischia island (B). Length-of-day (l.o.d) (C) and their normalized spectra in the two lower panels (D–E).
We identified trends (Supplementary Figure S4) and six to seven oscillating components in each of the seismic tremor and l.o.d. time-series using the main first 15 eigenvalues (Supplementary Figure S3). All four seismic tremor time-series are mainly composed by periods of a few days to a few months among which we identified periodicities corresponding to long-term tidal constituents which we later refer to using their Darwin’s symbol (Table 1 and Supplementary Figure S3). Thus, we recognized three and five tidal periodicities at the T1367 and IOCA stations located on Ischia island, and four and five for the ASBG and CPIS stations in Campi Flegrei (Table 1).
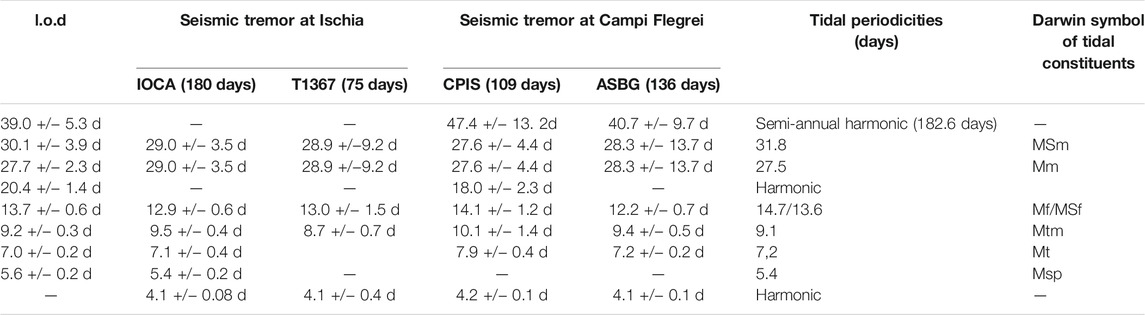
TABLE 1. Tidal constituents extracted in the l.o.d. and the seismic tremor for four seismic stations: IOCA and T1367 at Ischia island, CPIS and ASBG at Campi Flegrei, and identified using Ray and Erofeeva (2014).
The monthly and fortnightly constituents are common to all sites, although their uncertainties calculated from the half-width of their peaks at half-height do not allow us to discriminate between their lunisolar and lunar origin (MSm/Mm, MSf/Mf). The monthly tidal constituent represents the strongest component after the trend for ASBG and IOCA stations that are the longest time-series with 136 and 180 days, respectively (Supplementary Figure S3). This component is better differentiated from the other constituents for ASBG, whereas at IOCA, the contribution of Mm/MSm is of the same order as Mt (Supplementary Figure S3). The waveforms of the seismic tremor associated with the Mm/MSm period are locally in phase, i.e., on one hand for IOCA and T1367 stations and on the other hand for CPIS and ASBG (Figures 3A,B). For Campi Flegrei, the monthly tidal component clearly appears in quadrature with those extracted in the l.o.d. (Figures 3A,B). The waveform corresponding to Mm/MSm for the ASBG station shows a strong attenuation that is not observed at CPIS, the modulation in amplitude of this latter station being closer to those of the l.o.d. Some attenuation seems to also take place at Ischia, with the Mm/MSm component for the T1367 station when compared to that of IOCA.
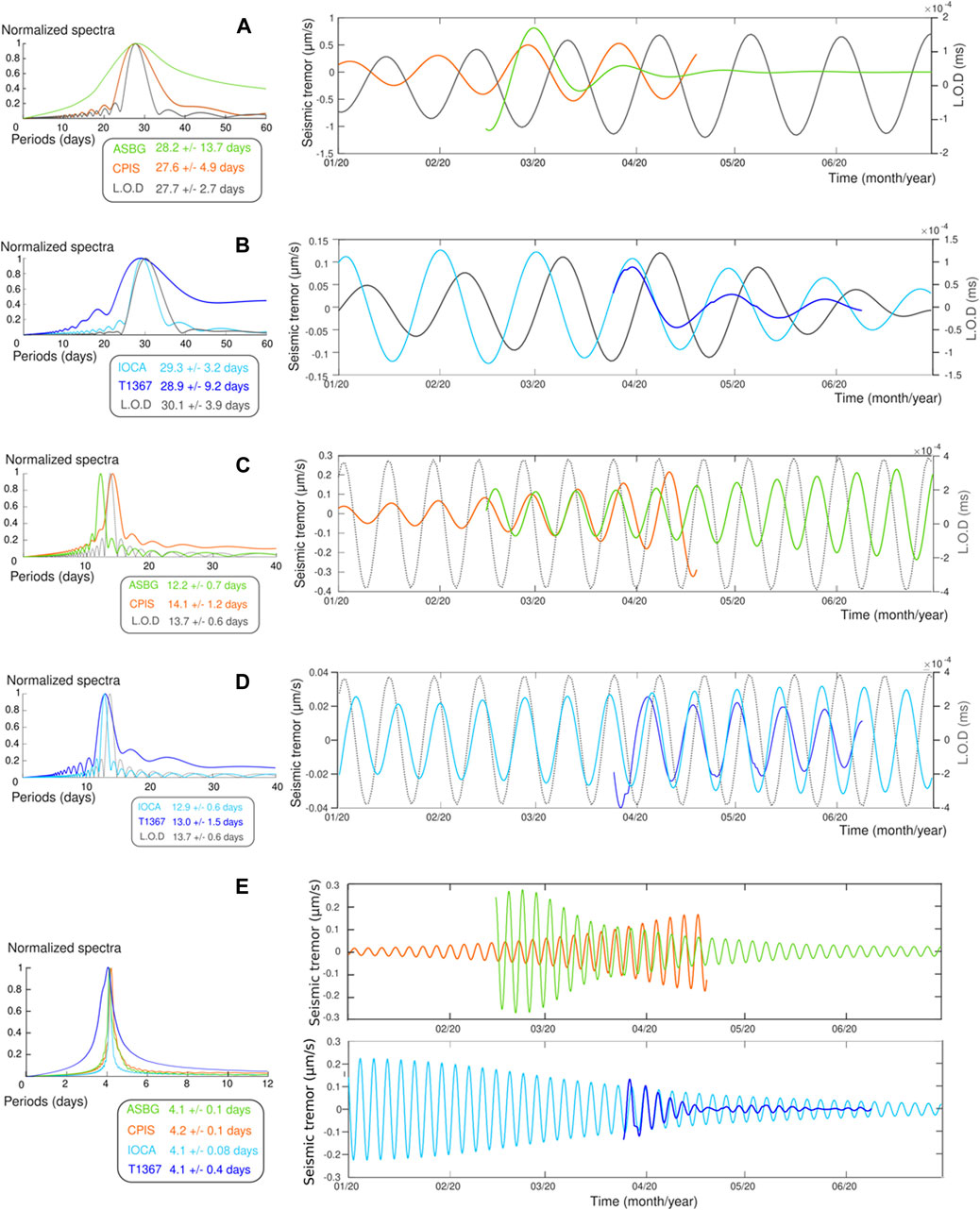
FIGURE 3. Tidal components extracted in the seismic tremor and l.o.d. time-series for (A–B) ∼27–31 (Mm/MSm) and (C–D) ∼13 days (Mf/MSf). Panel (E) represents the ∼4-day period extracted in all four seismic tremor time-series which likely corresponds to the harmonic of longer-period tides. The normalized spectra are shown on the left side and the corresponding waveforms on the right.
The fortnightly constituents appear in the first three components for the ASBG and T1367 stations (Supplementary Figure S3), as the fifth component for the CPIS station, and in a much lower rank for IOCA, identified as 21st and 22nd eigenvalues. For Campi Flegrei, the fortnightly components extracted in the seismic tremor at CPIS and ASBG both show an amplitude increase, while that of the l.o.d. is rather constant (Figure 3C). For Ischia, the Mf/MSf components are in phase for the seismic tremor of T1367 and IOCA, showing slight amplitude variations (Figure 3D).
In the four time-series of seismic tremor, we also extracted the ∼9-day tidal periodicity, known by the Darwin’s symbol Mtm, at both volcanoes (Table 1). The ∼7-day tidal periodicity or Mt was detected at all sites except at the T1367 station in Ischia, and that of ∼5 days corresponding to Msp tide was only observed at IOCA and in the l.o.d. (Table 1). In this table, we also listed a ∼4-day period that was extracted in all four seismic tremor time-series (Figure 3E). Such periodicity was also detected in co-eruptive geophysical time-series acquired in Cape Verde (Dumont et al., 2021) and might correspond to a harmonic of longer tidal period. An interesting observation of this periodicity resides in the modulation of its amplitude. At Ischia, this ∼4-day period shows a decreasing amplitude over the 6 months of observation at the IOCA station. At Campi Flegrei, a similar modulation in amplitude of the seismic tremor is observed but only at ASBG, even if the decrease is much more pronounced. This modulation appears to be opposite to that observed at CPIS, where the amplitude is regularly increasing from January to April, 2020 (Figure 3E).
Finally, one can note two additional long periods (∼40 and ∼18 days) in the seismic tremor recorded at Campi Flegrei, also detected in the l.o.d. Both may represent harmonics of longer tidal periods and in particular those of the solar annual tide.
Discussion
Campi Flegrei
The analysis of the periodicities in the seismic tremor recorded at Campi Flegrei highlights the presence of long-term tidal constituents ranging from ∼7 to ∼28 days. Our results, together with those from De Lauro et al. (2013), who evidenced semi-diurnal and diurnal components, indicate a broad tidal spectrum of the hydrothermal tremor. Some of the long-term tidal periodicities have also been detected in other geophysical observables, e.g., ground deformation (De Lauro et al., 2018; Ricco et al., 2019; Petrosino et al., 2020) and temperature time-series (Caputo et al., 2020; Cusano et al., 2021) as well as in rates of VT earthquakes (Petrosino et al., 2018), suggesting that these different tides and, in particular, the monthly and fortnightly constituents influence several manifestations of the caldera activity.
The waveforms associated with tidal constituents extracted in the seismic tremor by SSA provide another kind of information. In the examples presented in Figure 3, the modulation in amplitude of similar tidal components extracted in ASBG and CPIS time-series varies significantly from site to site, except maybe for the fortnightly constituents (Figure 3C). The amplitude at ASBG and CPIS evolves in an opposite manner, accompanied by an attenuation at ASBG which is not observed at CPIS. Such different behavior of the waveforms extracted in the hydrothermal tremor recorded at two nearby stations (<2 km) points towards a local effect. Two factors, the source of the seismic tremor and the site effect, could cause such variations. On one hand, CPIS is located close to the mud pool of the Pisciarelli fumarolic field. It records a tremor whose wavefield is dominated by high frequencies (5–15 Hz) due to the boiling and degassing phenomena at the surface (Chiodini et al., 2017; Gresse et al., 2017). In other words, we are dealing with a high frequency and very localized source of tremor for the CPIS station. On the other hand, the ASBG station is located at a distance of ∼1.6 km from the Pisciarelli site and records most exclusively the seismic tremor in a lower frequency band, e.g., 0.4–1 Hz as shown in its Power Spectral Density (Supplementary Figure S3; De Lauro et al., 2013; Capuano et al., 2017). The source of this tremor relates to a more complex origin likely due to hydrothermal fluid flow and circulation in the sub-superficial (∼2 km of depth) mixing zone of Solfatara (De Lauro et al., 2013; Gresse et al., 2017). Moreover, the results of a recent high-precision gravity survey indicate the presence of a low density body beneath the Astroni volcano where ASBG is located and which was interpreted as a sealed fluid reservoir. This reservoir, which produces no geothermal manifestation at the surface, is likely fed by the Solfatara-Pisciarelli system through a network of faults (Young et al., 2020).
The different responses at CPIS and ASBG can also be due to site effects. There are many geological and geophysical evidences of the heterogeneity of the Campi Flegrei shallow crustal structure. Indeed, differences in seismic velocity and attenuation as well as fracture density (Figure 1) characterize the two sites (De Siena et al., 2010, 2018; Vitale and Isaia, 2014). It is likely that soil and rocks beneath the two stations amplify or act as a damper according to their specific properties, e.g., rheology, porosity, permeability, fracture density. The water-saturation of the host rocks and rock properties vary with tides (Takano et al., 2014; Malagnini et al., 2019) and the seasonal hydrological cycle including rainfall (De Lauro et al., 2018; Ricco et al., 2019; Petrosino et al., 2020, Petrosino et al., 2021). These inherent properties are also highly modified by the hydrothermal activity that induces an alteration of the surrounding layers. Thus, the variations of rock properties of the first kilometers of the crust at ASBG and CPIS sites may significantly impact the wave propagation including that of tidal origin.
Such influence of the site effect on the tidal response of geophysical measurements is in agreement with observations made from the analysis of ground tilt and temperatures time-series (De Lauro et al., 2018; Caputo et al., 2020; Petrosino et al., 2020).
The seismic tremor recorded at Campi Flegrei also shows two additional periodicities (∼40 and ∼18 days). Interestingly, an ∼18-day periodicity was detected in ground tilt and VT occurrence and it was ascribed to the effect of the atmospheric pressure (De Lauro et al., 2018; Petrosino et al., 2018). Furthermore, the availability of long time-series (5 years) of ground temperature recorded at the Pisciarelli site allowed for a finer spectral analysis and revealed spectral components in the range 18.1–19.6 days. Most of these components are related to atmospheric factors, whereas only one was attributed to endogenous processes (Caputo et al., 2020). A similar analysis also evidenced the concomitance of external and internal forcing in modulating the ground temperature time-series in the band 30–50 days (Cusano et al., 2021).
Ischia
Similarly as we obtained for Campi Flegrei, our analysis of the seismic tremor measured at IOCA and T1367 stations reveals tidal components ranging from ∼5 to ∼29 days (Table 1), the higher number of tidal periodicities detected at IOCA being likely due to its longer time record compared with that of T1367. Contrary to Campi Flegrei, the waveforms associated with the tidal periodicities extracted at IOCA and T1367 are in phase and show very similar modulations of their amplitude (Figure 3), the amplitude of waveforms extracted for the seismic tremor of the T1367 station shows a light attenuation. Such similar behavior of the two sites suggests that these signals of seismic tremor are similarly first-order modulated by Earth tides, independently of the site of data acquisition. Indeed, the two stations IOCA and T1367 are very close (at a distance of less than 700 m): the seismic and magnetotelluric imaging indicate similar structures beneath them (Capuano et al., 2015; Di Giuseppe et al., 2017) as well as fault density (Figure 1, Di Giuseppe et al., 2017; Cusano et al., 2020b). A local amplification effect was indeed detected in the seismic tremor recorded at IOCA in the 1–2 Hz frequency band (Cusano et al., 2020b) and might explain the light difference in the modulation observed in the tidal constituents of IOCA and T1367 (Figure 3).
In addition, the seismic signals recorded at both sites are ascribable to the same tremor source related to the fluid flow within the hydrothermal system (Cusano et al., 2020b; Falanga et al., 2021). Past geophysical and geochemical studies have inferred the existence of a deep (>900 m) hydrothermal reservoir feeding a diffuse shallow aquifer (0–200 m of depth) located between the northern coast and the inner part of the island (Di Napoli et al., 2009; Di Giuseppe et al., 2017). Here, meteoric and sea water mix with the ascending deep fluids, causing in turn a pressure build-up in the shallow pipe-like conduits, which originates the hydrothermal seismic tremor. The similar behavior of the waveforms associated with tidal periodicities at IOCA and T1367 is thus consistent with the existence of this common source of tremor.
Conclusion
Here we explore the tidal influence on hydrothermal activity at Ischia and Campi Flegrei using seismic tremor data and the SSA technique. By analyzing the seismic tremor at each volcano, we characterize the tidal action on the fluid circulation through the identification of tidal periodicities. We also provide first insights on the response of hydrothermal systems to this external forcing by analyzing the waveforms of tidal constituents extracted by SSA.
At Campi Flegrei, past studies (De Lauro et al., 2013) of tidal modulation of low-frequency hydrothermal tremor were based on simple spectral analysis and focused on semi-diurnal and diurnal tidal constituents. Here, we provide complementary results which contribute in defining the long-period components of the tidal spectrum of both low-frequency and high-frequency tremor. In addition, this study presents the first evidence of tidal modulation of the hydrothermal tremor at Ischia too.
Our results evidence differences between the response of the two hydrothermal systems to tidal forcing. The two sites on Ischia seem to respond more easily and in a more similar manner to solid Earth tides when compared to those in Campi Flegrei. On the opposite side, in the Campi Flegrei caldera, the waveforms associated with tidal periodicities of the hydrothermal tremor show clear site-dependent attenuation likely related to more heterogeneity in the underlying layers.
Evidence of tidal forcing on hydrothermally generated seismic tremor has also been documented in other volcanic environments but mainly submarine ones (Tolstoy et al., 2002; Yakiwara et al., 2013; Meier and Schlindwein, 2018). Interestingly, the modulations of the seismic tremor have been recognized not only during eruptive phases but also in quiescent or unrest volcanoes. Focusing on the tidal influence on hydrothermal circulation of subaerial volcanoes and in particular tracking its temporal variations would provide a new way to monitor the state of the tidal stresses in the volcanic system.
Similar analyses performed on subaerial hydrothermal systems would allow to better explore the causes of the spatial and temporal variations of their response to Earth tides and how sensitive and prompt they are to respond to small environmental changes.
Data Availability Statement
The RMS dataset used for this study can be found in the Zenodo repository https://doi.org/10.5281/zenodo.5502069.
Author Contributions
SP conceived the research study and performed the RMS estimate of the seismic tremor time-series. SD performed the SSA analysis. SP and SD contributed to the interpretation of the results and the writing of the manuscript.
Funding
Details of all funding sources should be provided, including grant numbers if applicable. Please ensure to add all necessary funding information, as after publication this is no longer possible. SD acknowledges the Fundação para a Ciência e Tecnologia (FCT) and the European Union (UE) for their financial support through the postdoctoral fellowship SFRH/BPD/117714/2016, co-financed by the Ministério da Ciência, Tecnologia e Ensino Superior (MCTES), Fundo Social Europeu (FSE), and Programa Operacional Regional Centro (Centro 2020). This work is part of the projects PTDC/CTA-GEF/6674/2020—RESTLESS and UIDB/50019/2020, Instituto Dom Luiz (IDL) funded by FCT.
Conflict of Interest
The authors declare that the research was conducted in the absence of any commercial or financial relationships that could be construed as a potential conflict of interest.
Publisher’s Note
All claims expressed in this article are solely those of the authors and do not necessarily represent those of their affiliated organizations, or those of the publisher, the editors and the reviewers. Any product that may be evaluated in this article, or claim that may be made by its manufacturer, is not guaranteed or endorsed by the publisher.
Acknowledgments
The seismic data recorded by the permanent and mobile seismic networks of Ischia and Campi Flegrei were provided by the Istituto Nazionale di Geofisica e Vulcanologia, sezione di Napoli—Osservatorio Vesuviano. Figure 1 has been drawn by using the software Generic Mapping Tools (Wessel et al., 2013).
Supplementary Material
The Supplementary Material for this article can be found online at: https://www.frontiersin.org/articles/10.3389/feart.2021.775269/full#supplementary-material
References
Amoruso, A., Crescentini, L., and Sabbetta, I. (2014). Paired Deformation Sources of the Campi Flegrei Caldera (Italy) Required by Recent (1980-2010) Deformation History. J. Geophys. Res. Solid Earth 119 (2), 858–879. doi:10.1002/2013jb010392
Battaglia, M., Troise, C., Obrizzo, F., Pingue, F., and De Natale, G. (2006). Evidence for Fluid Migration as the Source of Deformation at Campi Flegrei Caldera (Italy). Geophys. Res. Lett. 33 (1). doi:10.1029/2005gl024904
Bellucci Sessa, E., Castellano, M., and Ricciolino, P. (2021). GIS Applications in Volcano Monitoring: the Study of Seismic Swarms at the Campi Flegrei Volcanic Complex, Italy. Adv. Geosci. 52, 131–144. doi:10.5194/adgeo-52-131-2021
Berrino, G., and Corrado, G. (1991). Tidal Signal in the Recent Dynamics of Campi Flegrei Caldera (Italy). J. Volcanology Geothermal Res. 48 (1-2), 93–101. doi:10.1016/0377-0273(91)90035-x
Bozzo, E., Carniel, R., and Fasino, D. (2010). Relationship between Singular Spectrum Analysis and Fourier Analysis: Theory and Application to the Monitoring of Volcanic Activity. Comput. Math. Appl. 60 (3), 812–820. doi:10.1016/j.camwa.2010.05.028
Bredemeyer, S., and Hansteen, T. H. (2014). Synchronous Degassing Patterns of the Neighbouring Volcanoes Llaima and Villarrica in South-central Chile: the Influence of Tidal Forces. Int. J. Earth Sci. (Geol Rundsch) 103 (7), 1999–2012. doi:10.1007/s00531-014-1029-2
Capuano, P., De Lauro, E., De Martino, S., Falanga, M., and Petrosino, S. (2017). Convolutive Independent Component Analysis for Processing Massive Datasets: a Case Study at Campi Flegrei (Italy). Nat. Hazards 86 (2), 417–429. doi:10.1007/s11069-016-2545-0
Capuano, P., De Matteis, R., and Russo, G. (2015). The Structural Setting of the Ischia Island Caldera (Italy): First Evidence from Seismic and Gravity Data. Bull. Volcanology 77 (9), 1–10. doi:10.1007/s00445-015-0965-4
Caputo, T., Cusano, P., Petrosino, S., Sansivero, F., and Vilardo, G. (2020). Spectral Analysis of Ground thermal Image Temperatures: what We Are Learning at Solfatara Volcano (Italy). Adv. Geosci. 52, 55–65. doi:10.5194/adgeo-52-55-2020
Chiodini, G., Giudicepietro, F., Vandemeulebrouck, J., Aiuppa, A., Caliro, S., De Cesare, W., et al. (2017). Fumarolic Tremor and Geochemical Signals during a Volcanic Unrest. Geology 45 (12), 1131–1134. doi:10.1130/g39447.1
Contadakis, M. E., Arabelos, D. N., and Vergos, G. (2017). Testing the Recent Santorini Seismic Activity for Possible Tidal Triggering Effect. geosociety 51, 1–17. doi:10.12681/bgsg.11653
Cusano, P., Caputo, T., De Lauro, E., Falanga, M., Petrosino, S., Sansivero, F., et al. (2021). Tracking the Endogenous Dynamics of the Solfatara Volcano (Campi Flegrei, Italy) through the Analysis of Ground Thermal Image Temperatures. Atmosphere 12 (8), 940. doi:10.3390/atmos12080940
Cusano, P., Petrosino, S., De Lauro, E., De Martino, S., and Falanga, M. (2020a). Characterization of the Seismic Dynamical State through Joint Analysis of Earthquakes and Seismic Noise: The Example of Ischia Volcanic Island (Italy). Adv. Geosci. 52, 19–28. doi:10.5194/adgeo-52-19-2020
Cusano, P., Petrosino, S., De Lauro, E., and Falanga, M. (2020b). The Whisper of the Hydrothermal Seismic Noise at Ischia Island. J. Volcanology Geothermal Res. 389, 106693. doi:10.1016/j.jvolgeores.2019.106693
Cusano, P., Petrosino, S., and Saccorotti, G. (2008). Hydrothermal Origin for Sustained Long-Period (LP) Activity at Campi Flegrei Volcanic Complex, Italy. J. Volcanology Geothermal Res. 177 (4), 1035–1044. doi:10.1016/j.jvolgeores.2008.07.019
De Lauro, E., De Martino, S., Falanga, M., and Petrosino, S. (2016). Fast Wavefield Decomposition of Volcano-Tectonic Earthquakes into Polarized P and S Waves by Independent Component Analysis. Tectonophysics 690, 355–361. doi:10.1016/j.tecto.2016.10.005
De Lauro, E., De Martino, S., Falanga, M., and Petrosino, S. (2013). Synchronization between Tides and Sustained Oscillations of the Hydrothermal System of Campi Flegrei (Italy). Geochem. Geophys. Geosyst. 14 (8), 2628–2637. doi:10.1002/ggge.20149
De Lauro, E., Falanga, M., and Petrosino, S. (2012). Study on the Long-Period Source Mechanism at Campi Flegrei (Italy) by a Multi-Parametric Analysis. Phys. Earth Planet. Interiors 206-207, 16–30. doi:10.1016/j.pepi.2012.06.006
De Lauro, E., Petrosino, S., Ricco, C., Aquino, I., and Falanga, M. (2018). Medium and Long Period Ground Oscillatory Pattern Inferred by Borehole Tiltmetric Data: New Perspectives for the Campi Flegrei Caldera Crustal Dynamics. Earth Planet. Sci. Lett. 504, 21–29. doi:10.1016/j.epsl.2018.09.039
De Siena, L., Del Pezzo, E., and Bianco, F. (2010). Seismic Attenuation Imaging of Campi Flegrei: Evidence of Gas Reservoirs, Hydrothermal Basins, and Feeding Systems. J. Geophys. Res. Solid Earth 115 (B9). doi:10.1029/2009jb006938
De Siena, L., Sammarco, C., Cornwell, D. G., La Rocca, M., Bianco, F., Zaccarelli, L., et al. (2018). Ambient Seismic Noise Image of the Structurally Controlled Heat and Fluid Feeder Pathway at Campi Flegrei Caldera. Geophys. Res. Lett. 45 (13), 6428–6436. doi:10.1029/2018gl078817
de Vita, S., Sansivero, F., Orsi, G., Marotta, E., and Piochi, M. (2010). Volcanological and Structural Evolution of the Ischia Resurgent Caldera (Italy) over the Past 10 Ky. Geol. Soc. Am. Spec. Pap. 464, 193–239. doi:10.1130/2010.2464(10)
Del Gaudio, C., Aquino, I., Ricciardi, G. P., Ricco, C., and Scandone, R. (2010). Unrest Episodes at Campi Flegrei: A Reconstruction of Vertical Ground Movements during 1905-2009. J. Volcanology Geothermal Res. 195 (1), 48–56. doi:10.1016/j.jvolgeores.2010.05.014
Di Giuseppe, M. G., Troiano, A., and Carlino, S. (2017). Magnetotelluric Imaging of the Resurgent Caldera on the Island of Ischia (Southern Italy): Inferences for its Structure and Activity. Bull. Volcanol 79 (12), 85. doi:10.1007/s00445-017-1170-4
Di Napoli, R., Aiuppa, A., Bellomo, S., Brusca, L., D'Alessandro, W., Candela, E. G., and Valenza, M. (2009). A Model for Ischia Hydrothermal System: Evidences from the Chemistry of thermal Groundwaters. J. Volcanology Geothermal Res. 186 (3-4), 133–159. doi:10.1016/j.jvolgeores.2009.06.005
Dinger, F., Bobrowski, N., Warnach, S., Bredemeyer, S., Hidalgo, S., Arellano, S., et al. (2018). Periodicity in the BrO∕SO2 Molar Ratios in the Volcanic Gas Plume of Cotopaxi and its Correlation with the Earth Tides during the Eruption in 2015. Solid Earth 9 (2), 247–266. doi:10.5194/se-9-247-2018
Dumont, S., Custódio, S., Petrosino, S., Thomas, A., and Sottili, G. (2022). “Tides, Earthquakes and Volcanic Eruptions,” in A Journey through Tides. Editors M. Green, and J. C. Duarte (Elsevier). in revision.
Dumont, S., Le Mouël, J.-L., Courtillot, V., Lopes, F., Sigmundsson, F., Coppola, D., et al. (2020). The Dynamics of a Long-Lasting Effusive Eruption Modulated by Earth Tides. Earth Planet. Sci. Lett. 536, 116145. doi:10.1016/j.epsl.2020.116145
Dumont, S., Silveira, G., Custódio, S., Lopes, F., Le Mouël, J.-L., Gouhier, M., et al. (2021). Response of Fogo Volcano (Cape Verde) to Lunisolar Gravitational Forces during the 2014-2015 Eruption. Phys. Earth Planet. Interiors 312, 106659. doi:10.1016/j.pepi.2021.106659
Dzurisin, D. (1980). Influence of Fortnightly Earth Tides at Kilauea Volcano, Hawaii. Geophys. Res. Lett. 7 (11), 925–928. doi:10.1029/gl007i011p00925
Eichelberger, J., Kiryukhin, A., Mollo, S., Tsuchiya, N., and Villeneuve, M. (2020). Exploring and Modeling the Magma-Hydrothermal Regime. Geosciences 10, 234. doi:10.3390/geosciences10060234
Falanga, M., Cusano, P., De Lauro, E., and Petrosino, S. (2021). Picking up the Hydrothermal Whisper at Ischia Island in the Covid-19 Lockdown Quiet. Sci. Rep. 11 (1), 1–11. doi:10.1038/s41598-021-88266-9
Falanga, M., and Petrosino, S. (2012). Inferences on the Source of Long-Period Seismicity at Campi Flegrei from Polarization Analysis and Reconstruction of the Asymptotic Dynamics. Bull. Volcanol 74 (6), 1537–1551. doi:10.1007/s00445-012-0612-2
Girona, T., Huber, C., and Caudron, C. (2018). Sensitivity to Lunar Cycles Prior to the 2007 Eruption of Ruapehu Volcano. Sci. Rep. 8 (1), 1476–1479. doi:10.1038/s41598-018-19307-z
Golyandina, N., and Zhigljavsky, A. (20132013). Singular Spectrum Analysis for Time Series. Berlin, Heidelberg: Springer.
Gottsmann, J., Carniel, R., Coppo, N., Wooller, L., Hautmann, S., and Rymer, H. (2007). Oscillations in Hydrothermal Systems as a Source of Periodic Unrest at Caldera Volcanoes: Multiparameter Insights from Nisyros, Greece. Geophys. Res. Lett. 34 (7). doi:10.1029/2007gl029594
Gresse, M., Vandemeulebrouck, J., Byrdina, S., Chiodini, G., Revil, A., Johnson, T. C., et al. (2017). Three-Dimensional Electrical Resistivity Tomography of the Solfatara Crater (Italy): Implication for the Multiphase Flow Structure of the Shallow Hydrothermal System. J. Geophys. Res. Solid Earth 122 (11), 8749–8768. doi:10.1002/2017jb014389
Isaia, R., Marianelli, P., and Sbrana, A. (2009). Caldera Unrest Prior to Intense Volcanism in Campi Flegrei (Italy) at 4.0 Ka BP: Implications for Caldera Dynamics and Future Eruptive Scenarios. Geophys. Res. Lett. 36 (21). doi:10.1029/2009gl040513
Jupp, T. E., Pyle, D. M., Mason, B. G., and Dade, W. B. (2004). A Statistical Model for the Timing of Earthquakes and Volcanic Eruptions Influenced by Periodic Processes. J. Geophys. Res. Solid Earth 109 (B2). doi:10.1029/2003jb002584
Lambeck, K. (2005). The Earth's Variable Rotation: Geophysical Causes and Consequences. Cambridge University Press.
Le Mouël, J. L., Lopes, F., Courtillot, V., and Gibert, D. (2019). On Forcings of Length of Day Changes: From 9-day to 18.6-year Oscillations. Phys. Earth Planet. Interiors 292, 1–11. doi:10.1016/j.pepi.2019.04.006
Lopes, F., Le Mouël, J.-L., and Gibert, D. (2017). The Mantle Rotation Pole Position. A Solar Component. Comptes Rendus Geosci. 349 (4), 159–164. doi:10.1016/j.crte.2017.06.001
Magee, C., Stevenson, C. T. E., Ebmeier, S. K., Keir, D., Hammond, J. O. S., Gottsmann, J. H., et al. (2018). Magma Plumbing Systems: a Geophysical Perspective. J. Pet. 59 (6), 1217–1251. doi:10.1093/petrology/egy064
Malagnini, L., Dreger, D. S., Bürgmann, R., Munafò, I., and Sebastiani, G. (2019). Modulation of Seismic Attenuation at Parkfield, before and after the 2004 M 6 Earthquake. J. Geophys. Res. Solid Earth 124 (6), 5836–5853. doi:10.1029/2019jb017372
Mauk, F. J., and Johnston, M. J. S. (1973). On the Triggering of Volcanic Eruptions by Earth Tides. J. Geophys. Res 78 (17), 3356–3362.
McNutt, S. R., and Beavan, R. J. (1987). Eruptions of Pavlof Volcano and Their Possible Modulation by Ocean Load and Tectonic Stresses. J. Geophys. Res. 92 (B11), 11509–11523. doi:10.1029/jb092ib11p11509
Meier, M., and Schlindwein, V. (2018). First In Situ Seismic Record of Spreading Events at the Ultraslow Spreading Southwest Indian Ridge. Geophys. Res. Lett. 45 (19), 10–360. doi:10.1029/2018gl079928
Miguelsanz, L., González, P. J., Tiampo, K. F., and Fernández, J. (2021). Tidal Influence on Seismic Activity during the 2011–2013 El Hierro Volcanic Unrest. Tectonics 40 (2), e2020TC006201. doi:10.1029/2020tc006201
Miller, C. A., Le Mével, H., Currenti, G., Williams-Jones, G., and Tikoff, B. (2017). Microgravity changes at the Laguna del Maule volcanic field: Magma-induced stress changes facilitate mass addition. J. Geophys. Res. Solid Earth 122 (4), 3179–3196. doi:10.1002/2017jb014048
Nakiboglu, S. M., and Lambeck, K. (1980). Deglaciation Effects on the Rotation of the Earth. Geophys. J. Int. 62 (1), 49–58. doi:10.1111/j.1365-246x.1980.tb04843.x
Petrosino, S., Cusano, P., and Madonia, P. (2018). Tidal and Hydrological Periodicities of Seismicity Reveal New Risk Scenarios at Campi Flegrei Caldera. Sci. Rep. 8 (1), 13808–13812. doi:10.1038/s41598-018-31760-4
Petrosino, S., and De Siena, L. (2021). Fluid Migrations and Volcanic Earthquakes from Depolarized Ambient Noise. Nat. Commun. 12, 6656. doi:10.1038/s41467-021-26954-w
Petrosino, S., Ricco, C., and Aquino, I. (2021). Modulation of Ground Deformation and Earthquakes by Rainfall at Vesuvius and Campi Flegrei (Italy). Front. Earth Sci. 9. doi:10.3389/feart.2021.758602
Petrosino, S., Ricco, C., De Lauro, E., Aquino, I., and Falanga, M. (2020). Time Evolution of Medium and Long-Period Ground Tilting at Campi Flegrei Caldera. Adv. Geosci. 52, 9–17. doi:10.5194/adgeo-52-9-2020
Ray, R. D., and Erofeeva, S. Y. (2014). Long-period Tidal Variations in the Length of Day. J. Geophys. Res. Solid Earth 119 (2), 1498–1509. doi:10.1002/2013jb010830
Ricco, C., Petrosino, S., Aquino, I., Del Gaudio, C., and Falanga, M. (2019). Some Investigations on a Possible Relationship between Ground Deformation and Seismic Activity at Campi Flegrei and Ischia Volcanic Areas (Southern Italy). Geosciences 9 (5), 222. doi:10.3390/geosciences905022210.3390/geosciences9050222
Saccorotti, G., Petrosino, S., Bianco, F., Castellano, M., Galluzzo, D., La Rocca, M., et al. (2007). Seismicity Associated with the 2004-2006 Renewed Ground Uplift at Campi Flegrei Caldera, Italy. Phys. Earth Planet. Interiors 165 (1-2), 14–24. doi:10.1016/J.PEPI.2007.07.006
Sahoo, S., Senapati, B., Panda, D., Tiwari, D. K., Santosh, M., and Kundu, B. (2021). Tidal triggering of micro-seismicity associated with caldera dynamics in the Juan de Fuca ridge. J. Volcanology Geothermal Res. 417, 107319. doi:10.1016/j.jvolgeores.2021.107319
Scholz, C. H., Tan, Y. J., and Albino, F. (2019). The Mechanism of Tidal Triggering of Earthquakes at Mid-ocean Ridges. Nat. Commun. 10 (1), 2526–2527. doi:10.1038/s41467-019-10605-2
Sottili, G., Lambert, S., and Palladino, D. M. (2021). Tides and Volcanoes: a Historical Perspective. Front. Earth Sci. 9. doi:10.3389/feart.2021.777548
Sottili, G., Martino, S., Palladino, D. M., Paciello, A., and Bozzano, F. (2007). Effects of Tidal Stresses on Volcanic Activity at Mount Etna, Italy. Geophys. Res. Lett. 34 (1). doi:10.1029/2006gl028190
Sottili, G., and Palladino, D. M. (2012). Tidal Modulation of Eruptive Activity at Open-Vent Volcanoes: Evidence from Stromboli, Italy. Terra Nova 24 (3), 233–237. doi:10.1111/j.1365-3121.2012.01059.x
Takano, T., Nishimura, T., Nakahara, H., Ohta, Y., and Tanaka, S. (2014). Seismic Velocity Changes Caused by the Earth Tide: Ambient Noise Correlation Analyses of Small-Array Data. Geophys. Res. Lett. 41 (17), 6131–6136. doi:10.1002/2014gl060690
Tolstoy, M., Vernon, F. L., Orcutt, J. A., and Wyatt, F. K. (2002). Breathing of the Seafloor: Tidal Correlations of Seismicity at Axial Volcano. Geol 30 (6), 503–506. doi:10.1130/0091-7613(2002)030<0503:botstc>2.0.co;2
Trasatti, E., Acocella, V., Di Vito, M. A., Del Gaudio, C., Weber, G., Aquino, I., et al. (2019). Magma Degassing as a Source of Long‐Term Seismicity at Volcanoes: The Ischia Island (Italy) Case. Geophys. Res. Lett. 46 (24), 14421–14429. doi:10.1029/2019gl085371
Vitale, S., and Isaia, R. (2014). Fractures and Faults in Volcanic Rocks (Campi Flegrei, Southern Italy): Insight into Volcano-Tectonic Processes. Int. J. Earth Sci. (Geol Rundsch) 103 (3), 801–819. doi:10.1007/s00531-013-0979-0
Wessel, P., Smith, W. H. F., Scharroo, R., Luis, J., and Wobbe, F. (2013). Generic Mapping Tools: Improved Version Released. EOS Trans. AGU 94 (45), 409–410. doi:10.1002/2013EO450001
Yakiwara, H., Hirano, S., Miyamachi, H., Takayama, T., Yamazaki, T., Tameguri, T., et al. (2013). Semi-Diurnal Tidal Periodicity Observed by an Ocean Bottom Seismometer Deployed at a Location Very Close to Seafloor Fumaroles in Wakamiko Caldera, Northeast of Sakurajima Volcano (Special Section Sakurajima Special Issue). Bull. Volcanological Soc. Jpn. 58 (1), 269–279.
Keywords: Campi Flegrei, Ischia, seismic tremor, hydrothermal activity, tidal modulation, SSA
Citation: Petrosino S and Dumont S (2022) Tidal Modulation of Hydrothermal Tremor: Examples From Ischia and Campi Flegrei Volcanoes, Italy. Front. Earth Sci. 9:775269. doi: 10.3389/feart.2021.775269
Received: 13 September 2021; Accepted: 06 December 2021;
Published: 01 February 2022.
Edited by:
Nico Fournier, GNS Science, New ZealandReviewed by:
Yosuke Aoki, The University of Tokyo, JapanCorentin Caudron, Université libre de Bruxelles, Belgium
Copyright © 2022 Petrosino and Dumont. This is an open-access article distributed under the terms of the Creative Commons Attribution License (CC BY). The use, distribution or reproduction in other forums is permitted, provided the original author(s) and the copyright owner(s) are credited and that the original publication in this journal is cited, in accordance with accepted academic practice. No use, distribution or reproduction is permitted which does not comply with these terms.
*Correspondence: Simona Petrosino, simona.petrosino@ingv.it
†These authors have contributed equally to this work and share first authorship