Simulation of exchange routes on the Qinghai-Tibetan Plateau shows succession from the neolithic to the bronze age and strong control of the physical environment and production mode
- 1School of Finance and Economics, Qinghai University, Xining, China
- 2Key Laboratory of Tibetan Plateau Land Surface Processes and Ecological Conservation (Ministry of Education), College of Geographical Science, Qinghai Normal University, Xining, China
- 3Qinghai Province Key Laboratory of Physical Geography and Environmental Process, College of Geographical Science, Qinghai Normal University, Xining, China
- 4Academy of Plateau Science and Sustainability, People’s Government of Qinghai Province and Beijing Normal University, Xining, China
- 5Key Laboratory of Geomatics Technology and Application of Qinghai Province, Provincial Geomatics Center of Qinghai, Xining, Qinghai, China
- 6State Key Laboratories of Plateau Ecology and Agriculture, Qinghai University, Xining, Qinghai, China
The Qinghai-Tibetan Plateau (QTP) is essential for converging eastern, western, and northern prehistoric cultural spheres of Asia and Europe and for human adaptation to extreme environments. Reconstruction of the location and development of prehistoric exchange routes on the Qinghai-Tibetan Plateau underpins understanding human response to harsh environments and interaction and exchange between the three cultural spheres. This study simulates exchange routes for the Neolithic and Bronze Ages, using elevation, slope, vegetation, and rivers as cost data and site points as node data. A weighted network consisting of nodes and lines is constructed within the Qinghai-Tibetan Plateau using a weighted cumulative cyclic connectivity model among nodes-the simulation abstracts exchange routes as a path search problem on this weighted network. The final simulated route is the road with the lowest incremental cost. The results give a total length of Neolithic routes of about 16,900 km, with 15 main roads, and a total length of Bronze Age routes of approximately 16,300 km, with 18 main roads. Pathway development from the Neolithic to the Bronze Age shows an apparent successional relationship, with a spatial evolution from the marginal corridor to the marginal hinterland. The simulated routes overlap highly with archaeological evidence for transmission routes of corn and millet agriculture and wheat agriculture-domesticated animals-bronze metallurgy technology, indicating the reliability of the simulation results. Further analysis showed that the unique physical geography of the QTP constrained the formation and evolution of routes. River valleys were commonly chosen as routes to acclimatize people to the high, cold, and low oxygen levels of the Qinghai-Tibetan Plateau. Scattered small agricultural bases, established in areas of the QTP suitable for agricultural planting, are the basis for intersecting exchange routes. Road formation also reflects the clear differentiation in the agro-pastoral industry between high and low altitudes related to climate, ecological environment, and elevation. Interaction between agricultural and nomadic populations is the crucial motivation for forming and developing the exchange routes.
1 Introduction
Long-distance migration laid the basic pattern for the distribution of modern humans and their cultures (Árnason, 2017) and was accompanied by cultural and technological exchanges that promoted the development of human civilization (Zeder, 2008; Anthony, 2010). The Qinghai-Tibetan Plateau (QTP) is an important area where the three major cultural spheres of the East, West, and North met in the early period of Asia and Europe (Han, 2021) and played an essential role in the intersection of Asian and European civilizations (Huo, 2017). The extreme and harsh natural environment of the QTP has a significant impact on human social organization (Chen et al., 2022), and mechanisms of human adaptation to harsh environments, especially plateau hypoxia, are currently a focus for academic research (Huerta-Sánchez et al., 2014). A complete picture of human occupation of the QTP should include the processes of adaptation by human physiology, production technology, habitat patterns, social organization, and cultural communication ability (Chen et al., 2022), which are critical to a more profound understanding of human adaptation mechanisms (Ding et al., 2020).
The first human presence on the QTP has been documented using archaeological materials, with hominin handprints and footprints found at Qusang, on the high plateau, dating to around 200ka.BP (Zhang D. et al., 2021), and Denisovan human bones at Xiahe, Gansu (160ka.BP) (Zhang et al., 2020). In the Late Pleistocene (40–30ka.BP), humans were present in the hinterland of the QTP, where the natural environment was very harsh (Zhang et al., 2018), and on the edge of the Qaidam Basin (Liu, 1995). Human expansion from the edge to the main body of the plateau is presumed to have occurred in stages from the Late Paleolithic to the Mesolithic (20–6ka.BP) (Hou et al., 2019). There is also evidence of exchange between populations from the hinterland of the QTP and those from the Qinghai Lake Basin during this period (Zhang et al., 2016) and of technological influences of “trans-Himalayan” dispersal in the southwestern QTP (Lv, 2014). In the Neolithic period, there was cultural expansion and penetration from east to west, represented by the Majiayao culture type and termed the “path of painted pottery” (Han, 2013). Wheat crops and bronze metallurgy were introduced in the Bronze Age through the Eurasian steppe–Xinjiang–Hexi Corridor route (Dong et al., 2022a). In the historical period, there is documentary evidence for migration and exchange routes on and around the QTP, including the Qiang people, ancient roads of the Tang Dynasty to the Tubo Dynasty, and trade routes of tea and horses. Isotope evidence has been used to trace ancient human migration activities at the Heishui ancient city site in Gansu Province and the Lajia site in Qinghai Province (Zhao et al., 2016). DNA analysis has provided insights into the timing of Sino-Tibetan divergence (Qin et al., 2010) and the origin of ethnic groups (Kang et al., 2010), from which routes of human migration can be estimated (Zhang et al., 2019). Overall, the current understanding of exchange routes on the QTP is mainly approximated from archaeological information, historical documents, genetics, and isotopes.
An alternative approach uses GIS combined with modelling to simulate and concretize communication routes. Applications have included the reconstruction of trade exchange routes for prehistoric and historical periods, for example, the simulation of Silk Roads in the Asian mountains (Frachetti et al., 2017) and the reconstruction of the overland Silk Road and the Central Asia-Xinjiang “Silk Road” (Ma et al., 2017). On the QTP, recent studies include GIS-based simulation of prehistoric exchange routes (Zhu et al., 2018), simulation of the “Tangfan Ancient Road” connection to the interior (LanCuo et al., 2019), and reconstruction of exchange routes within and between prehistoric cultural areas (Hou et al., 2021).
Archaeological and modelling studies suggest a diverse network of migration and exchange routes existed on the QTP over different periods and populations. However, quantitative and systematic analysis of exchange routes for the crucial Neolithic to the Bronze Age period is still lacking, which limits understanding of the evolution of early human interaction and exchange on the QTP. This study addresses this gap by simulating exchange routes on the QTP from the Neolithic to the Bronze period and analyzes factors controlling their formation to reveal the inner dynamics of their development. The results allow systematic and quantitative analysis of exchange route locations over time that provides insight into the unique cultural landscape of the QTP, mechanisms of human activities and environmental adaptation, and cultural exchange from a longer time scale.
2 Study area
2.1 Natural environment
The QTP has an average altitude of 4,400 m (above sea level), and it covers an estimated area of 2,580.9×103 km2 (area within China) (Figure 1). Topographically, the QTP is bordered to the north by the Altun Mountains and Qilian Mountains extending in a west-east direction; to the south by the Himalayan Mountains extending from northwest to southeast; to the west by the Karakoram Mountains and the Kunlun Mountains extending in an east-west direction; and in the southeast are the stands the north-south trending Hengduan Mountains. Geographically, the QTP is in southwest China and bounded by India, Nepal, and Bhutan to the south, Kashmir, Pakistan, and Tajikistan to the west. In the north, the plateau connects with the Tarim Basin and the Hexi Corridor; to the southeast, it connects with Yunnan-Guizhou and Sichuan; to the northeast, it is bounded by the Loess Plateau (Zhang Y.L et al., 2021).
The QTP is the source region of several major rivers, including the Yangtze River, Yellow River, and Lantsang. Outflowing river systems are mainly concentrated in the east and south of Tibet, with inland river systems in the northwest and the Qiangtang Plateau in the hinterland of the QTP (Zheng and Zhao, 2017). Lakes cover a large area of the QTP, with the Great Lakes of northern Tibet accounting for 48% of the total (Ma et al., 2011). The vegetation of the QTP generally shows a zonal turnover of montane forest–alpine meadow–mountain/alpine grassland–mountain/alpine desert, with apparent horizontal zone differentiation (Zheng et al., 1979). The altitude of the plateau decreases from west to east (Sun, 1996); the high-altitude results in relatively low temperatures and extreme coldness (Zheng and Zhao, 2017). Since the QTP is located at a low latitude with thin air, solar radiation is extreme (Wu and Ma, 2010). In summary, the most significant characteristic of the QTP study area is the exceptional and harsh environment for human survival.
2.2 Stage classification
The early cultures on the QTP mainly cover the Neolithic period (NP) and the Bronze -Age (BA). The Layihai site (6.745 ± 85ka.BP), in the upper reaches of the Yellow River on the QTP, is transitional from Late Paleolithic to the Neolithic (Gai and Wang, 1983). The Qijia culture (4–3.6ka.BP) represents the transition from the Neolithic to Bronze Age. Hence, this study takes the NP as 6–4ka.BP. The start of the BA coincides with the Qijia culture (4–3.6ka.BP) (Zhang and Dong, 2017), and it endured until the establishment of the Qin Dynasty, the first centralized state in the historical period. Therefore, the BA is defined in the study as 4–2ka.BP.
3 Materials and methods
3.1 Calculation procedures
Human movement and nodes are the essential elements that make up an exchange road network. Moreover, in practice, the cost of walking needs to be considered. This study assumes communication routes form a network structure composed of people accumulating along multiple nodes. The flow of people on the route chooses the relatively low-cost path between two points, so the network must be weighted. Human flow and nodes constitute the communication network elements, and edge lines connecting the nodes are the communication routes. Eq. 1 is used to build a weighted exchange network
Where, V is the node set, E is the network connection, and W is the cost.
Archaeological site points are used as the node set (V). The cost (W) is used to calculate the cost distance, an edge line (E) connects nodes based on cost distance, and a weighted network (G) is constructed based on node-edge lines. Finally, the optimal simulated route (S) is determined based on the route with the lowest cumulative cost in the weighted network.
The complete computational process incorporates (Step 1) Collection of archaeological site locations (Step 2) Cost calculation using geographic data (Step 3) Calculation of linkage routes between nodes based on costs; and (Step 4) Calculation of the minimum cost route in the network. The algorithm flow diagram is shown in (Figure 2), and full calculation instructions are in (Supplementary Material S3).
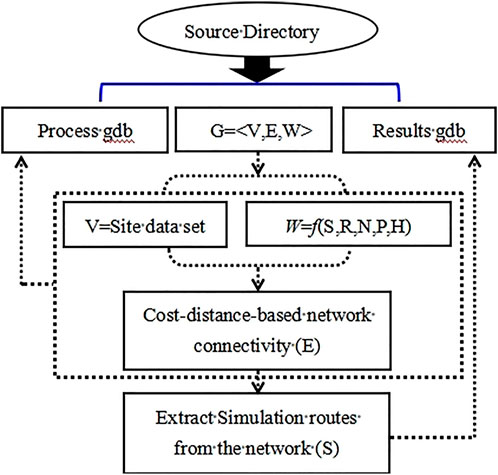
FIGURE 2. Flowchart showing workflow for calculation of elements in the exchange route simulation. G is a weighted network, E is a network connection, W is the cost, S is the optimal path between nodes V. S is the slope, R is the river, N is NDVI, P is NPP, and H is altitude. The calculation was designed using the Geographic Information Systems software ArcGIS (ESRI Corporation, Red lands, CA, United States) and executed in Python using the native Python geoprocessing tools in ArcGIS.
3.2 Data and materials
3.2.1 Archaeological site data set
Archaeological data comprised 4,431 sites, including 1,040 Neolithic period and 3,391 Bronze Age sites. Survey data were obtained from the State Administration of Cultural Heritage’s Atlas of Chinese Cultural Relics (State Administration of Cultural Heritage, 1996; 2001; 2009; 2010; 2011). The point coordinate information from the literature was collated and vectorized.
3.2.2 Geographic data set
1) QTP range and boundary (Zhang et al., 2021). 2) DEM with a resolution of 90 m (Chinese Academy of Sciences, 2000). 3) China 1:250,000 River Classification dataset (Chinese Academy of Sciences, 2001). 4) China 1:1,000,000 spatial distribution of vegetation types (Chinese Academy of Sciences, 2001). 5) Data sets of accumulated temperature ≥0°C and ≥10°C (Chinese Academy of Agriculture Sciences, 1995). 6) Annual mean precipitation in China on a 1-km grid (1971–2000) (Chinese Academy of Sciences, 1971–2000). 7) Monthly synthetic product data of 500 M NDVI for the Chinese region. Coordinate system: EPSG:4326 (WGS84). Calculated by MODND1D, NDVI is derived by taking the maximum daily value within a month; to eliminate the influence of the growing and withering seasons on overall values, the multi-year average for 2010–2016 was used as the NDVI data set in this study (Chinese Academy of Sciences, 2010–2016). 8) 1 km surface temperature data. Coordinate system: EPSG:4326 (WGS84). Temperature is obtained by synthetic calculation using MYDLT1D product, taking monthly averages with a temporal resolution of 1 day. This study used multi-year averages from 2010–2016 and monthly averages for the whole year (2010–2016) as the surface temperature data set (Chinese Academy of Sciences, 2010–2016).
4 Results
4.1 Neolithic period route simulation
The total length of simulated routes for the NP is 16,933 km, divided into four areas, northeast, east, southeast, and southwest, with 15 main routes (Figure 3A). The northeast route area has a total length of 1,438 km (Figure 3B), with four route segments, NP-MPX, NP-MHL, NP-HBJ, and NP-LGZ, forming a comparatively dense network. Route NP-MPX follows the Yellow River from the northeast of the QTP into the Xining Basin. Route segments NP-MXH and NP-GXG connect the northeastern margin of the QTP to the Gonghe Basin. The eastern route area has a total length of 1,880 km (Figure 3C), comprising three main routes that run along the eastern edge of the QTP connecting the present-day provinces of eastern Qinghai province, southern Gansu province, and western Sichuan Province. Route NP-TLD connects the Yangtze River’s main tributary and the Yellow River Tributary from north to south, while routes NP-MXD and NP-XDK follow the tributaries of the Yangtze River from east to west. The total length of the southeastern route area is 1,032 km (Figure 3D), comprising two single branches. Route NP-LMD follows a north-south direction along the deep mountain valley of the Lancang River basin, and route NP-KDD runs east-west across the Hengduan Mountains. Finally, the southwest route area has 12,584 km (Figure 3E), comprising four main routes. Route NP-SSA is located in the hinterland of northern Tibet, route NP-LRR connects the Lhasa Valley and western Tibet along the Yarlung Zangbo River basin, route NP-CMD is positioned in the Lhasa Valley, and route NP-DAQ connects the Lhasa Valley with northern Tibet province.
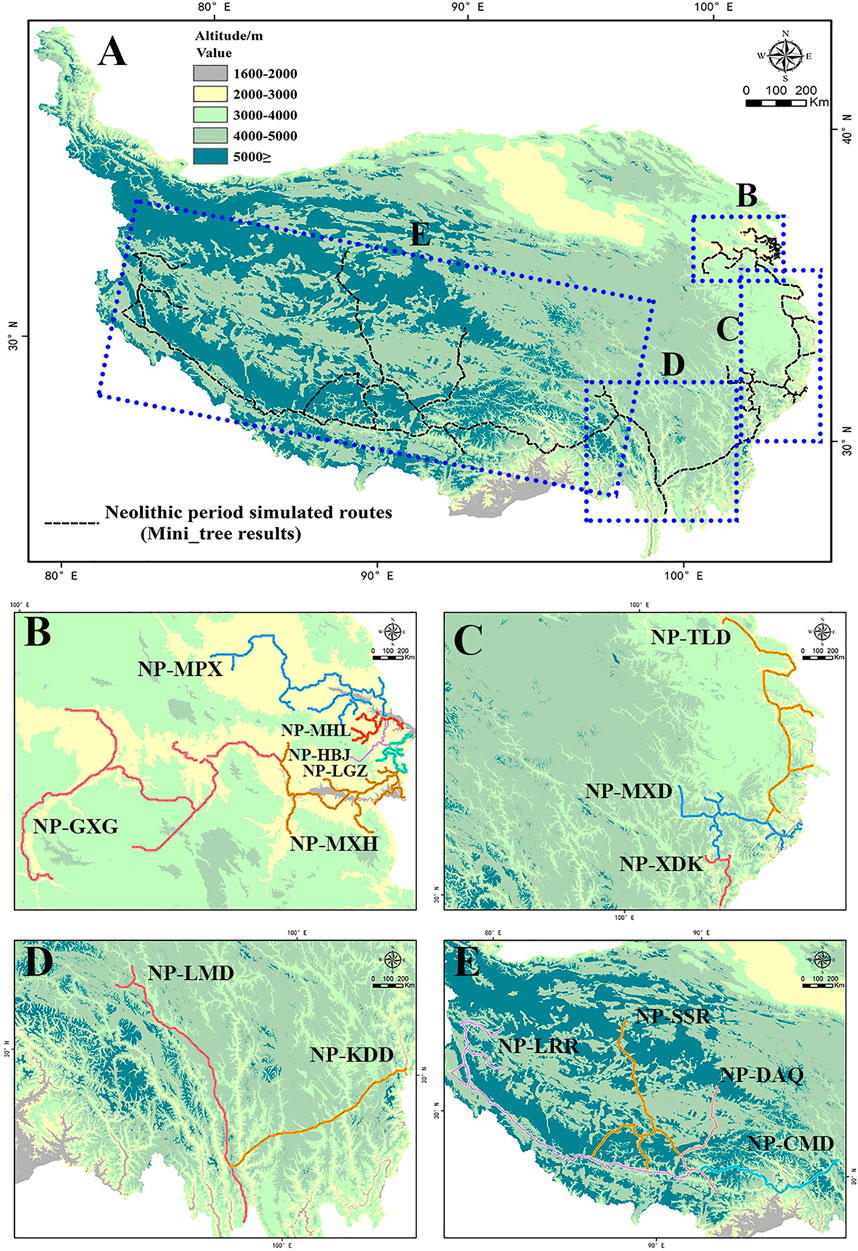
FIGURE 3. Simulated exchange routes for the neolithic period (NP). (A) overview of simulated routes and location of sub-areas; (B) northeast route area; (C) east route area; (D) southeast route area; (E) southwest route area. Individual route segments are labelled NP-XXX, with XXX referring to location codes.
4.2 Bronze age route simulation
The total length of simulated routes for the BA is 16,933 km, comprising 15 main routes across four areas: northeast, east, southeast, and southwest (Figure 4A). The northeast route area covers 5,371 km and is divided into six segments (Figure 4B). Segment BA-MHH runs east-west from the northeastern QTP to the vicinity of Gonghe Basin. Segment BA-HMG forms a dense network in northern Qinghai province. A branch of the BA-XGG route extends southward as far as the southern part of Qinghai province, while the BA-GGQ trunk route follows the northern edge of the Qinghai Lake Basin as far as the southern foothills of the Qilian Mountains. The main branch of segment BA-GDG extends westward to the north and south edges of the Qaidam Basin, connecting the Qinghai Lake Basin to the Qaidam Basin.
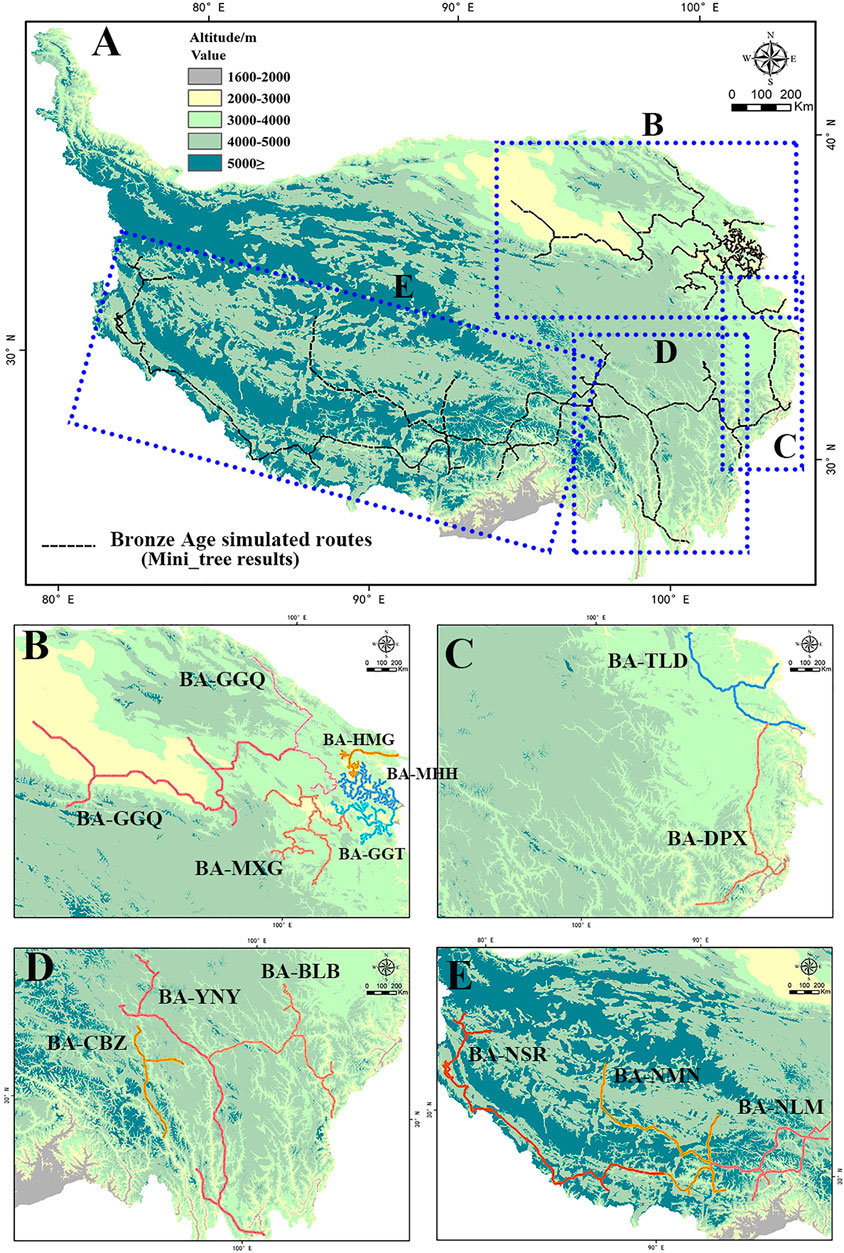
FIGURE 4. Simulated exchange routes for the Bronze age (BA). (A) overview of simulated routes and location of sub-areas; (B) northeast route area; (C) east route area; (D) southeast route area; (E) southwest route area. Individual route segments are labelled BA-XXX, with XXX referring to location codes.
The eastern route area has a total length of 1,047 km and comprises two north-south oriented main routes (Figure 4C), BA-TLD and BA-DPX. The trunk routes run from the present-day southeastern Qinghai province, through southern Gansu province, to the western Sichuan Province. The southeast route area has a total length of 2,570 km, comprising three main north-south segments (Figure 4D). Route BA-BLB travels along the deep mountain valleys of the Dadu and Minjiang River basins from the southern Qinghai province to the western Sichuan Province. Route BA-YNY extends along the source of the Yangtze River to the Upper Yangtze River, from southern Qinghai province to northwestern Yunnan Province. Route BA-CBZ route runs through the Changdu region of Tibet province. The southwest route area has a total length of 7,397 km, comprising three main routes (Figure 4E). Trunk line BA-NLM enters the Lhasa River Valley and South Tibet Valley from Qinghai province in an east-west direction. Trunk line BA-NMN forms a complex north-south to the east-west road network around the Lhasa River Valley and connects it to northern Tibet. Trunk line BA-NSR forms a direct route to western Tibet along the north and south banks of the Yarlung Zangbo River, connecting the Lhasa Valley, South Tibet Valley, West Tibet, and southwest Tibet. In the southwest route area, route BA-NSR emerges through the Pulan and Jilong basins as a connector to the southern Himalayan gorges.
4.3 Evolutionary process
The simulation results for the NP show that the routes in the northeast area follow the important tributaries of the upper reaches of the Yellow River. The route enters the QTP from the northeast, goes into the Xining Basin along the Yellow River, and extends from the Xining Basin to the Gonghe Basin. The eastern route area is a hub that connects the northeast and southeast route areas; it cuts across current administrative boundaries, connecting northeastern Qinghai Province, southern Gansu Province, and western Sichuan Province. Although there are only two routes in the southeast area, they make it possible for people to cross the Hengduan Mountains to connect with the southwest QTP. Southwest area routes follow the Yarlung Zangbo River, with the main trunk line extending to the hinterland of the QTP in the north and northeast of Tibet province.
For the BA, the simulation results indicate a core complex route network in the northeast comprising multiple trunks and branch lines, with extensions from the core area to the southern foothills of the Qilian Mountains and the Qaidam Basin. The eastern area remains a hub connecting northeastern and southeastern routes. In the southeast area, the route through the Hengduan Mountains allows unimpeded traffic with the southwest route area. It connects through Pulan Basin and Jilong Basin to the southern gorge of the Himalayas by a new route that was not present in the NP.
The northeast area shows the greatest change from NP to BA, with an increase in the total route length from 1,438 to 5,371 km. Extension of routes BA-GGQ and BA-GDG to the southern foothills of the Qilian Mountains and the Qaidam Basin accounts for most of the increase (adding about 3,900 km). Total route distance in the southeast area increased from 1,032 km in the NP to 2,570 km in the BA, with an increase from two to three main routes, including route BA-BLB, which was directly derived from NP-KDD. The total route length in the eastern area decreased from 1,880 to 1,047 km, with routes NP-MXD and NP-XDK merging and simplifying to form route BA-DPX. The total route distance in the southwest area decreased from 12,584 to 7,397 km due to routes extending less far into the northern Tibetan Plateau.
In summary, route development on the QTP from the NP to the BA shows characteristics of inheritance and evolution. Specifically, the BA route in the northeast area appears to have directly evolved and extended from the NP route, while routes in the eastern area shifted westwards from the NP to BA, and the southeast areas shifted from south to north. In the southwest area, the single route of the NP extended and increased in complexity to form a dense network between the Lhasa Valley and the Southern Tibetan Valley in the BA. Overall, the simulation results show the spatial evolution of routes from the NP to BA from the edge corridor to the hinterland.
4.4 Validation against archaeological evidence
4.4.1 Neolithic archaeological evidence
The Majiayao culture developed in the middle and late Holocene in the upper reaches of the Yellow River, in the present-day Gansu and Qinghai provinces, under the influence of the Yangshao culture (7–6ka.BP) that was present in the middle reaches of the Yellow River, between the present day Gansu and Henan provinces (Figure 5A). The distinctive features of the Majiayao culture are the cultivation of millet and the production of painted pottery. Based on the distribution of excavation sites with evidence for millet crop, it has been shown that millet agriculture spread to the Yellow River valley around 5.2ka.BP and continued to spread westward to the Hexi Corridor between 5–4.5ka.BP (Dong et al., 2017). Based on the spread of millet agriculture, several transmission routes of Majiayao culture on the QTP have been speculated. One potential route is through Minhe County–Xining–Huzhu County–Datong County to the Hexi Corridor in the northeastern QTP; another route is through northern Sichuan and Yunnan provinces along the eastern edge of the QTP (Hong et al., 2011).
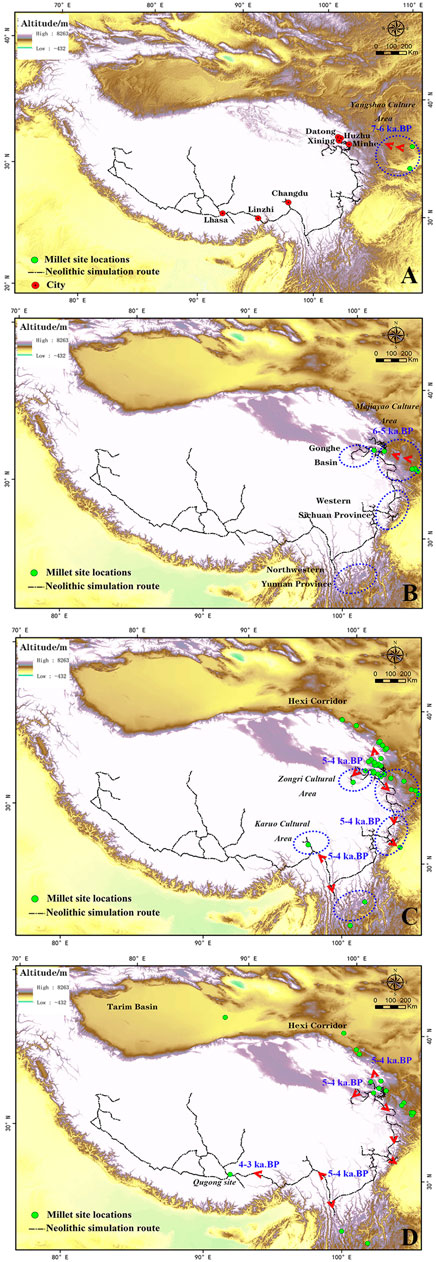
FIGURE 5. Simulated exchange routes compared with archaeological evidence for the spread of millet agriculture in the Neolithic period. (A) 7–6ka.BP; (B) 6–5ka.BP; (C) 5–4ka.BP; (D) 4–3ka.BP. Point data of site locations are from Dong et al. (2022a), Dong et al. (2022b). Blue dotted ovals mark the extent of cultural areas. Red arrows mark the assumed direction of movement.
To verify our simulated NP routes, we extracted and mapped archaeological sites on the QTP with millet agricultural elements for the period 6–2.5ka.BP and compared routes with site locations (Figure 5). The results show that in the period 6–5ka.BP, the millet-dominated Majiayao culture had already spread through the NP-MPX route in northeastern QTP (Figure 5B). Between 5–3.5ka.BP, millet agriculture spread westward in northeastern QTP through the NP-MPX route to Minhe County–Huzhu County–Datong County. However, it was not until the BA that millet sites spread to the Hexi Corridor, along routes BA-HMG and BA-GGQ. During this period, millet crops spread westward, with the NP-TLD route linking with NP-MXD and NP-XDK to reach western Sichuan Province (Figure 5C and Figure 5D). Dissemination to northwestern Yunnan Province was made possible by route NP-KDD, which is consistent with the Majiayao cultural dissemination route proposed by archaeologists in the region (Han, 2013; Xiang, 2018). The simulation results show that a cultural exchange between the Zongri and Majiayao cultures was realized through the NP-MXH connection to NP-GXG. The Zongri culture of the Gonghe Basin (5.2–4.1ka.BP) is a Neolithic culture that developed locally on the QTP but was strongly influenced by the Majiayao culture (Chen et al., 1998). Human bone isotope evidence also supports the Majiayao culture’s influence on the Zongri (Cui et al., 2006).
The earliest site with evidence of sarcophagus burial culture is Zongri, which appears to have spread to influence the western part of Sichuan Province and the northern part of Yunnan Province, as well as the Changdu and Linzhi regions of Tibet province (Li, 2011). In the western region of Sichuan Province, the Karuo culture is thought to have been influenced by both the Zongri and Majiayao cultures (Han, 2013). Moreover, Karuo culture or similar sites are also present in the southern Tibetan valley of Tibet province (Huo, 2013; Lv, 2014). The route simulation results show that an exchange between the Zongri and Karuo cultures would have been along NP-GXG and NP-MXH. The connection between the Majiayao and Karuo cultures in western Sichuan Province is provided by NP-TLD and NP-MXD, connecting to NP-KDD and NP-LMD. The spread of Karuo culture in the southern Tibetan valley and of the sarcophagus burial culture to sites such as Changdu and Linzhi in Tibet was realized along routes NP-CMD and NP-LMD.
Similarities between the Burzahom culture of Kashmir and the Karuo culture demonstrate connections between QTP cultures and those of Central Asia (Gao et al., 2021), and potential exchange routes have been speculated. The millet and barley dating at the Qugong Karuo culture site in Lhasa, which dated 3.4ka.BP, supports cross-cultural exchange between the Karuo culture and Middle Asia. Some suggested that the Karuo culture expanded westward along the Yarlung Tsangpo River, crossed the Himalayan Pass to reach the southern edge of the range, then expanded westward along the foothills of Kashmir (Han, 2013; Lv, 2014). Our simulation results confirm a bidirectional propagation route through NP-CMD and NP-LRR along the Yarlung Tsangpo River.
4.4.2 Bronze age archaeological evidence
Archaeological evidence from the BA suggests that barley and wheat from Central Asia were introduced to eastern Qinghai via the gateway of the Oasis Road in the Hexi Corridor on the northeastern QTP (Jia, 2012; Dong et al., 2022a). This is supported by isotope evidence from human bones that demonstrates a food source dominated by C3 crops (mainly barley) and studies that show wheat agriculture as the chosen survival strategy of people that settled on the northeastern QTP; these strategies contributed to the permanent settlement of the people on the high altitudes areas (Ma, 2013; Chen et al., 2015).
There is ample evidence for wheat and barley agriculture at archaeological sites on the high-altitude plateau in Tibet that was introduced along pathways from northeast India (Stevens et al., 2016). Plant remains of wheat crops are found at the Changguogou site in Tibet (Fu, 2001) and are believed to be associated with the spread of barley crops from Kashmir (Han, 2013). Stone grinding wheels and stone grinding sticks used to process grains at the Qugong site in Tibet indicate that agricultural production was an indispensable economic production category for the inhabitants (Tang, 2014). House remains at the Bangga, and Xiaoenda sites in Tibet indicate settlements formed by sedentary agriculture on a large scale (Huo, 2013). Genomic data show barley was introduced to southern Tibet around 4.5–3.5ka.BP through North Pakistan, India, and Nepal (Zeng et al., 2018), and carbonized plant remains identified as barley found at the Dingdong site, Tibet, dated to 2.4–1.9ka.BP (Lv, 2007).
To verify our simulated BA routes, we extracted and mapped archaeological sites on the QTP with barley agricultural elements for the period 4.5–2ka.BP and compared routes with site locations (Figure 6). Simulated routes BA-GGQ and BA-HMG border the Hexi Corridor and fit with archaeological evidence for the introduction of barley crops into the northeastern QTP, with route BA-GDG allowing northward expansion to the edge of the Qaidam Basin and route BA-MXG northwestward to the Gonghe Basin. The simulated route BA-NSR is in a suitable location to facilitate the spread of barley crops from South Asia to the southern part of the plateau. There may also be a branch route to spread into southern Tibet through the Jilong and Pulan valleys.
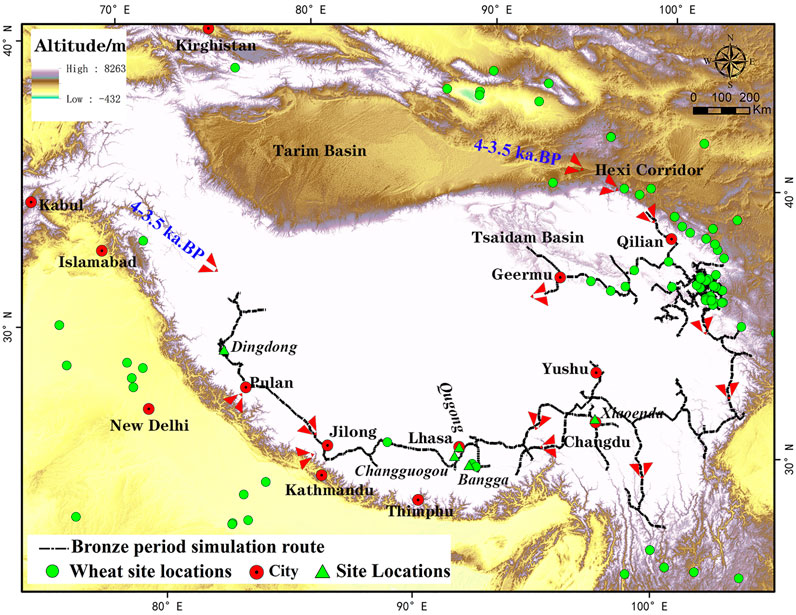
FIGURE 6. Simulated exchange routes compared with archaeological evidence for the spread of wheat agriculture in the Bronze Age, 4–3.5ka.BP. Red arrows mark the direction of movement. Point data of site locations are from Dong et al. (2022a), Dong et al. (2022b).
Archaeological findings suggest that the Bronze culture (arsenic copper) of the Qijia culture in the Gansu and Qinghai regions influenced the Siba culture, which then spread to Xinjiang (Zhang, 2016). Aside from barley/wheat agriculture, the influence of BA cultures can also be documented through the spread of Bronze metallurgy. The transmission route would likely be through the Yellow River and Qilian Mountains into the Hexi Corridor and up to the Xinjiang region (Cui, 2015).
Based on typological similarities of iron-handled bronze mirrors found at the Qugong site, Tibet (Tang, 2014), there are two possible routeways for their spread. One suggestion is that iron-handled bronze mirrors spread from Central Asia to Xinjiang province to Tibet province and continued eastward into the Hengduan Mountains region (Huo, 2000). Others argue that bronze mirrors from the Qugong site are more similar to those from northern South Asia (Zhao, 1994), with technique influence such as mounting style and the decorations at the back of the mirror from southern Central Asia or northern India (Lv, 2009). Some also argue that the bronze decoration style is influenced by bronzes technique from sites in Yunnan Province (Tong, 2010). Comparing the archaeological evidence with the simulated routes shows that Bronze culture transmission routes in the northeastern Tibetan Plateau are consistent with routes BA-GGQ and BA-HMG. Bronze metallurgy in Tibet may have been transmitted in both directions by connecting routes BA-BLB and BA-YNY through BA-NMN and BA-NLM.
5 Discussion
5.1 Relationship between route formation and cost
Table 1 compares the NP and BA route distance and cost properties (Table 1). Both the northeast and southeast route areas show increases in the total distance of the routes and total cost from the Neolithic to the Bronze Age, but the cost/km did not change in the former and increased in the latter. The eastern and southwestern route areas saw a decrease in the total distance, but the total cost and cost/km decreased in the former and increased in the latter.
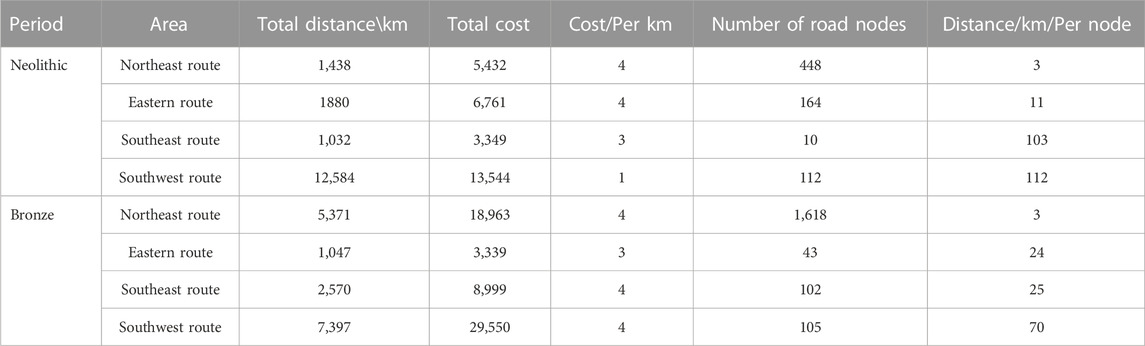
TABLE 1. Comparison of route distance and cost in the Neolithic to Bronze Age, from our exchange route simulation.
The average distance between nodes was significantly higher in the NP than the BA, largely due to a smaller number of nodes, with 743 on Neolithic routes compared with 1,863 on Bronze age routes. Specifically, route NP-KDD in the southeastern area utilized a smaller number of nodes in the NP, which ensures lower costs for long-distance communication. In contrast, increased nodes and shorter distance between nodes on the BA route BA-BLB achieves cost control. In the southwest route area, the distance between nodes of route NP-LRR is 232 km, and the cost per km is less than 1 (Supplementary Figure S1). By the BA, the average distance between nodes in the southwest route area is below 100 km for all routes, which was achieved by reducing the distance between nodes and thus balancing the relationship between distance and cost. In summary, a relatively low-cost route is created by increasing or decreasing the number of nodes and reducing the average distance between nodes.
We used the unordered multiple choice model estimate method to determine if elevation, slope, surface temperature, and vegetation influenced route selection. The results show that elevation, slope, surface temperature, and vegetation of the simulated routes are all essential factors influencing route selection (Supplementary Tabel S2). The cold temperatures of the QTP are a hazard to human health. Studies show that constant low temperatures can cause damage to body functions (Sun et al., 1998). Currently, the incidence of cardiovascular and cerebrovascular diseases in China is higher in the colder autumn and winter seasons (Yang and Ye, 2003). Therefore, the mortality rate of cardiovascular and cerebrovascular diseases is closely related to climate change (Tan and Qu, 2003). The effect of cold air on patient lethality is even more prominent (Wang et al., 2002). It has been shown that the relative oxygen concentration (ROC) in near-earth air in terms of atmospheric pressure and oxygen partial pressure decreases significantly with increasing altitude (Zha et al., 2016). Further studies have found that surface vegetation cover and temperature may also affect ROC; with constant altitude, ROC changes with vegetation cover and temperature (Shi et al., 2019).
The above analysis shows that although high mountain ranges block the QTP, the broad intermountain valleys and river canyons between high mountain ranges are natural routeways for ancient human passage. River valley passage was chosen because of the relatively low elevation, high surface temperature, and good vegetation cover (Figure 7). The most important vegetation types on the simulated route are concentrated in the relatively low-elevation river valleys of the QTP (Figure 8). This combination of factors leads to the relatively high oxygen content in the valleys. Ancient humans adapted their behaviour to deal with the low temperature, lack of oxygen, and gradually increasing plateau altitude.
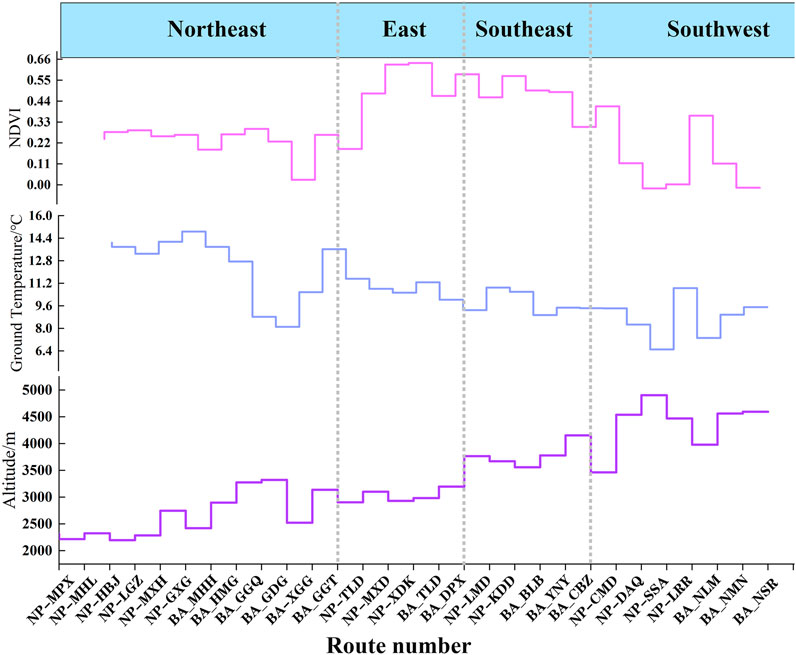
FIGURE 7. Altitude, ground temperature and NDVI with simulated Neolithic–Bronze Age exchange routes. See Figures 3, 4 for route locations.
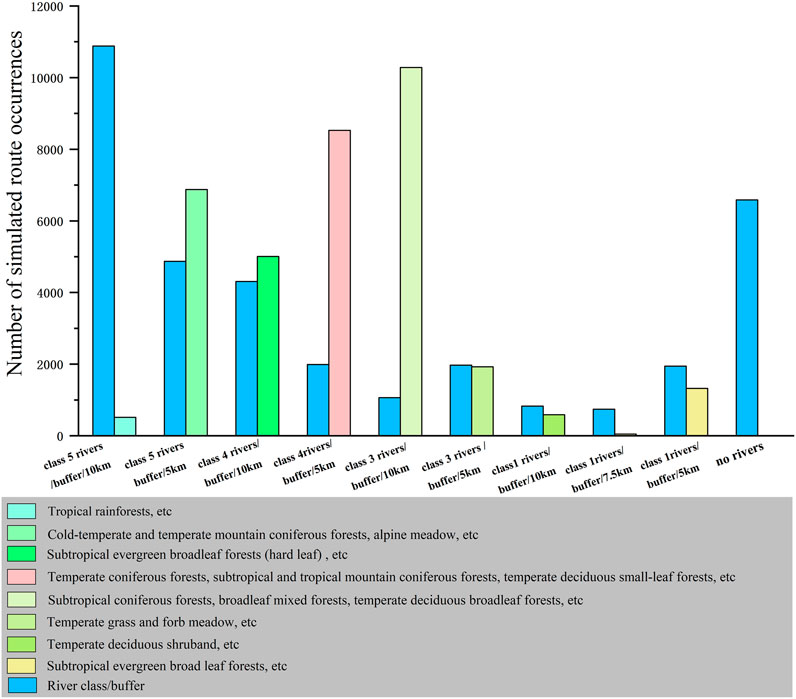
FIGURE 8. River and vegetation types associated with simulated Neolithic–Bronze Age exchange routes.
5.2 Formation of routes about agricultural cultivation
Millet is a typical temperature-loving and drought-tolerant crop, with a growth period requiring a cumulative temperature ≥10°C of 1,600–3,000°C. The most suitable temperature for the growing environment is 15°C–25°C, and the average annual temperature needs to reach 5°C–10°C. Millet has a higher yield and is suitable for environments with 450–550 mm of precipitation; it is more drought tolerant and can survive in environments with 350–450 mm of precipitation, so it has a broader distribution in the highlands (Li, 1987). The sensitive response of millet agriculture to water, heat, and frost prevents it from surviving in areas above 2,500 m in altitude (Chai, 1999). Wheat agriculture requires a temperature in the range of 10°C–15°C. Barley is a cool-loving crop and requires an effective cumulative temperature ≥0°C of 1,200°C–1,500°C (Chen, 2013), so it is the most important grain crop in the QTP. The climate of the QTP has significant altitudinal differentiation, with the warmest monthly mean temperature in river valleys at 2,500–4,000 m above sea level (12°C–18°C), while the plateau surface and high mountains at 4,000–4,500 m elevation have lower temperatures (6°C–10°C) (Zheng et al., 1979). In summary, the typical agroecology of the plateau is valley agriculture.
The Neolithic millet-cultivating agricultural population occupied the low-altitude valleys of the plateau, influenced by water, heat, and frost conditions. Archaeological sites associated with millet agriculture are concentrated at altitudes below 2,600 m (Figure 9J). Wheat crops replaced millet during the Bronze Age as the most critical crop resource utilized by prehistoric humans on the QTP (Chen et al., 2015). The cultivation of wheat crops expanded the space for agricultural production, raising the elevation of cultivation in plateau valleys upward by at least 1,000 m, as evidenced by the altitudinal distribution of wheat crop archaeological sites (Figure 9J).
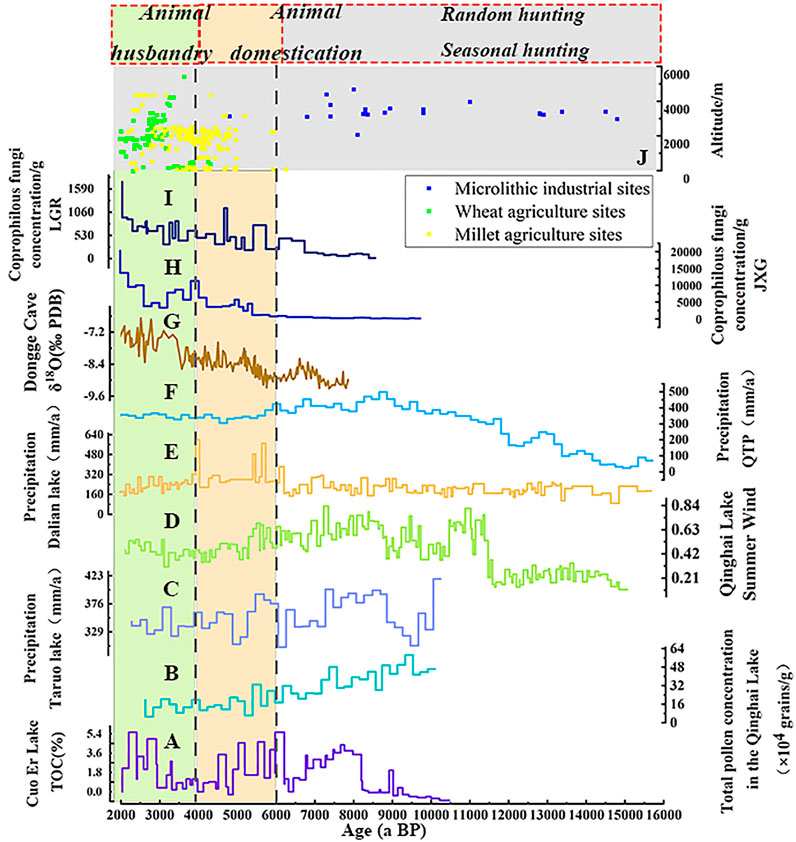
FIGURE 9. Comparison of Paleolithic–Bronze Age activities and various indicators of environmental change on the Qinghai-Tibetan Plateau (QTP) (A) Total organic carbon (TOC) (%) in the sedimentary record at Cuo Er Lake, central QTP (Wu et al., 2006); (B) Total pollen concentration in Qinghai Lake, northeastern QTP (×104 grains/g) (Shen et al., 2005); (C) Integrated precipitation curve in Taruo lake, southwest QTP (Ma et al., 2014); (D) Qinghai Lake summer monsoon index, northeastern QTP (An et al., 2012); (E) Quantified reconstruction of rainfall in Dalian Lake (Cheng et al., 2010); (F) Synthetic reconstruction of rainfall trend for the QTP (Hou et al., 2012); (G) Record of monsoon intensity from Dongge cave (Dykoski et al., 2005); (H) Total coprophilous fungi concentration curve at Jiangxi Gou 2 (JXG) site, Qinghai Lake Basin, northeastern QTP (Wei et al., 2020a); (I) Total coprophilous fungi concentration curve at Langgeri (LGR), Qinghai Lake Basin, northeastern QTP (Wei et al., 2020b). (J) Site altitude: corn site (yellow squares), wheat site (green squares), and hunter-gatherer site (blue squares).
The simulated Neolithic routes correspond to an area suitable for agricultural corn production, with ≥10°C accumulation temperatures of 1,600–3,000°C and within the 400–600 mm isopleth (Figure 10E). The simulated Bronze Age routes are located in an area with ≥0°C accumulation temperature of 1,000–1,500°C and 300–400 mm isopleth (Figure 10E), which is suitable for wheat crop cultivation. These dispersed small-scale agricultural locations are the basis for the tandem simulation routes.
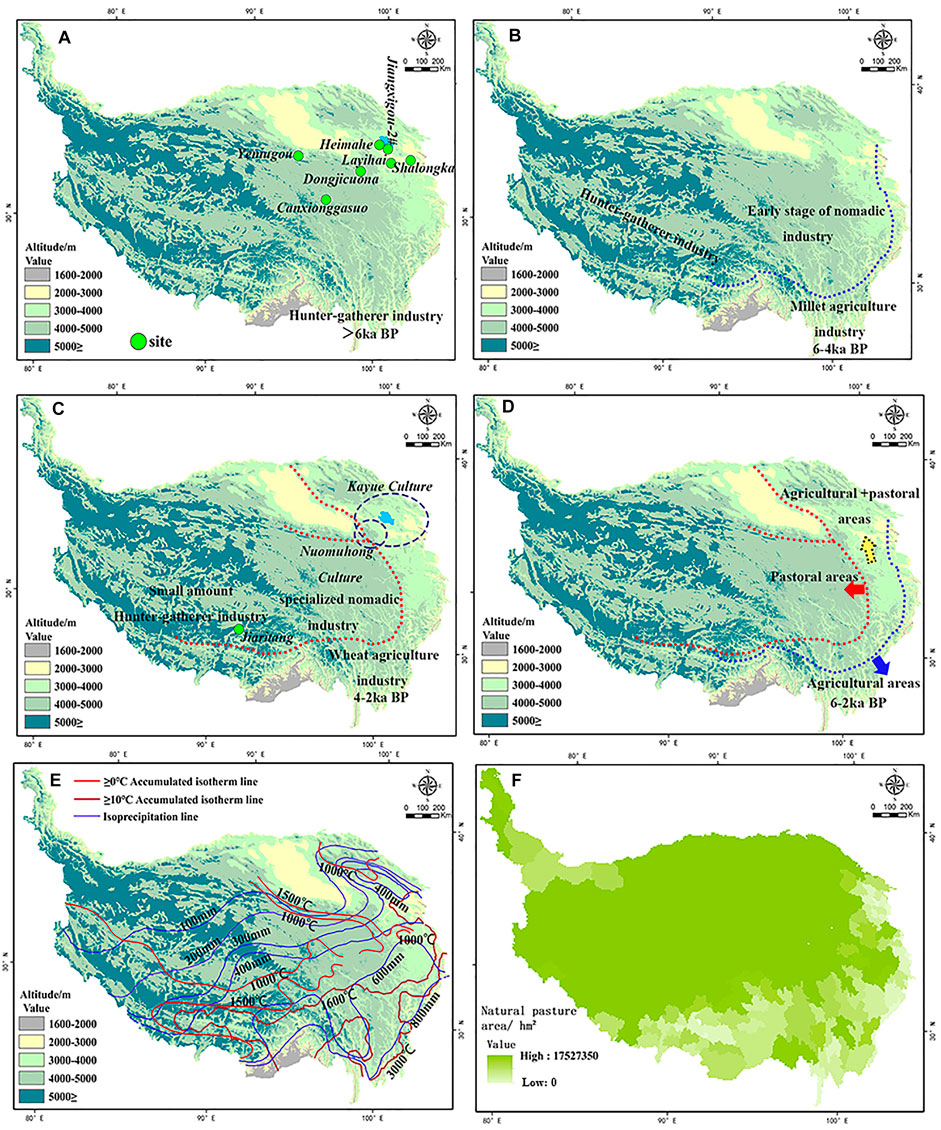
FIGURE 10. Evolution of agricultural and pastoral activity on the Qinghai-Tibetan Plateau 6–2ka.BP. (A) Hunter-gatherer industry, >6ka.BP; (B) Early stage of nomadic industry and emergence of millet agriculture, 6–4ka.BP; (C) Emergence of the wheat agriculture industry, 4–2ka.BP; (D) Trends in the location of agriculture and nomadic herding areas, 6–2ka.BP; (E) Temperature and precipitation trends; (F) Natural pasture area.
5.3 Relationship between route formation and regional segmentation of the production model
Climatic fluctuations from the Last Ice Age to the early Holocene drove hunter-gatherer populations to migrate frequently between high and low elevations (Zhang et al., 2016). The hunter-gatherer population exhibited a combination of random and seasonal fixed travel (Madsen et al., 2006). Faunal remains within Qinghai Lake Basin suggest that hunter-gatherer populations hunted randomly for high-return ungulates (Brantingham et al., 2013) to meet the needs of small-scale populations for short-term subsistence (Wang et al., 2020). The Jiangxigou and Layihai sites in the lake basin are central camps that were used repeatedly (Yi et al., 2011). In addition, travellers’ use of fire pits has also been recorded in the Kunlun Mountains region at Yeniugou (Tang et al., 2013) and Dongjicuona sites (Gao et al., 2020). Specific case studies show that early Holocene hunter-gatherers already developed a hunting pattern incorporating travelling between high and low altitudes in different seasons. In this model, the low-elevation Shalongka site (8.4–6.2ka.BP) is representative of a winter camp, and the high-elevation Canxionggasu site (8.3–7.5ka.BP) is representative of a summer camp (Hou et al., 2020) (Figure 10A).
Frequent wandering migration and short-term, small-scale survival strategies in different seasons result from adaptation to the plateau environment, with its uneven seasonal distribution of resources and significant diurnal temperature range. Seasonal resources on the QTP are easy to predict and imply a high success rate for hunters (Wang et al., 2020). Plants begin to grow in April-May, reach maturity in July-September, enter their terminal phase in mid-late September, the decline in mid-late October and enter dormancy from mid-November to April (Kong et al., 2017).
Vegetation reconstructions show that the QTP was dominated by desert vegetation during the LGM and evolved to meadow and grassland after 14ka.BP (Hou et al., 2017). An increase in temperature and precipitation after the LGM in the Qinghai Lake Basin led to a transition in vegetation type from desert grassland to alpine meadow/subalpine scrub (Shen et al., 2005). Stalagmite oxygen isotope records from Dongge cave show that the Asian monsoon began to weaken after 6ka.BP (Dykoski et al., 2005) (Figure 9G), resulting in a relatively dry cold environment and degraded vegetation. The summer winds index in the Qinghai Lake Basin also gradually weakened at this time, and regional precipitation decreased, resulting in a decrease in forest area and an increase in grassland area (An et al., 2012) (Figure 9D). After 4ka.BP, the spore pollen record of Qinghai Lake indicates a dry and cold climate, with forests in the area evolving to forested grasslands and dry grasslands (Shen et al., 2005) (Figure 9B). Various other paleoenvironmental indicators support the shift to a dry, cold climate and reduction in forest and the development of grassland vegetation at this time. These include a reduction in mean annual precipitation at Dalian Lake in the Gonghe Basin (Cheng et al., 2010) (Figure 9E), a decrease in total organic content at Cuo Er lake on the central plateau (Wu et al., 2006) (Figure 9A), decrease in precipitation at Taruo Lake on the southwest plateau (Ma et al., 2014) (Figure 9C), and decrease in average annual precipitation of the QTP (Hou et al., 2012) (Figure 9F). The harsh natural environment after 4ka.BP significantly reduced the potential for agricultural cultivation (Zhang and Dong, 2017).
At the present-day, alpine grassland and alpine meadow-based grassland ecosystems account for over 80% of the total area of the QTP, with only 10% of available land suitable for arable farming (Department of Animal Husbandry and Veterinary Medicine, Ministry of Agriculture of the People’s Republic of China, 1994). Alpine grassland dominated by Stipapupurea and alpine meadows dominated by Stipacapillacea provides suitable grazing for yak and sheep (Zheng and Zhao, 2017). The largest areas of pasture on the QTP are concentrated at altitudes of >3,000 m (Figure 10F) (Department of Animal Husbandry and Veterinary Medicine, 1994, Ministry of Agriculture of the People’s Republic of China, 1994). Whole genome sequencing shows that the domestication of yaks on the QTP occurred around 7.3ka.BP (Qiu et al., 2015). Yak domestication probably resulted from humans following the migratory route of wild yaks onto the northern QTP during the Paleolithic; humans migrated to the QTP alpine grassland region for grazing in the early to middle Holocene (Rhode et al., 2007). Early grazing in the Qinghai Lake Basin occurred between 6–5.5ka.BP, intensifying from 5.5–4.2ka.BP (Wei et al., 2020a; Wei et al., 2020b) (Figures 9H, I). Domesticated cattle, goats, and sheep spread from west Asia to the northeastern edge of the QTP through the Hexi Corridor around 4.5–4ka.BP; horses were domesticated around 4–3.6ka.BP and spread into the Gansu-Qinghai region via the Hexi Corridor (Ren and Dong, 2016). Specialized nomadic economies emerged in the Kayue (3.6–2.6ka.BP) and Nuomuhong (3.4–2.7ka.BP) cultures (Zhang and Dong, 2017). The large number of fine stone tools excavated from the Jiaritang site (3ka.BP–circa 7th century AD) suggests that the nomadic livelihoods continued on the QTP until the Late Bronze Age (Tang, 2012; Huo, 2013) (Figure 10C).
The above evidence highlights the long history of the hunter-gatherer industry, since before 6ka.BP, on the QTP due to the unique climate and ecosystem (Figure 10A). After the emergence of millet agriculture, the earliest industrial divide in the agricultural and nomadic economy of the plateau developed (Figure 10B). The beginnings of a scattered nomadic herding economy from 6–4ka.BP likely developed from the conversion of hunter-gatherer populations directly. Two factors facilitate the conversion: 1) the abundant pasture resource provides the basis for the domestication of animals by the highland hunting population; 2) the survival strategy of frequent moving between high and low altitudes adopted by hunting people has similarities in the typical nomadic practice of winter and summer pasture transitions at different altitudes. A specialised nomadic pastoral economy emerged with the spreading of domesticated animals from the Central Asia-Hexi Corridor around 4–2ka.BP. Wheat-based agriculture developed a new industrial division on the eastern plateau (Figure 10C).
A range of evidence indicates the existence of trade and exchange between hunter-gatherers and agricultural cultivators on the QTP around 5ka.BP (Hou et al., 2016). Studies of nomadic societies in Central Asia have found that in addition to economic products such as butter, cheese, yoghurt, and meat, nomadic populations exchanged grains from farmers from which they obtained their caloric intake (Frachetti, 2009). In addition to meat, yaks also provide secondary products, such as fuel (dung), milk, cheese, and hides, that may be traded. Seashells excavated from Majiayao and Zongri culture sites were probably circulated as currency (Chen et al., 1998). The complementary nature of the agricultural and nomadic economies led to forming of a mixed agro-pastoralist zone with solid altitudinal differentiation of activity. Agricultural cultivation is mainly in valley areas at 2,000–3,000 m elevation, with mixed agriculture and nomadic activity between 3,000–4,000 m, with hunter-gatherer and nomadic economies dominating areas >3,000 m. Thus, in addition to climatic and environmental factors, altitude is an important controlling factor in the location of agriculture and animal husbandry on the QTP (Figure 10D).
Analysis of the simulated routes in this study shows that agricultural cultivation areas and semi-agricultural zones are also essential requirements for forming stable routes; we argue that cultural and economic exchanges of agricultural and nomadic populations were essential in forming stable routes. The exchange between agricultural and nomadic populations began in the NP and intensified in the Bronze Age with the enhanced cross-continental exchange.
6 Conclusion
The unique physical geography has constrained the formation, development, and evolution of routes on the QTP. Exchange routes on the QTP show inheritance and development relationships from the Neolithic to the Bronze Age. Spatially, there is an evolution from marginal corridors to marginal hinterlands. The river valleys of the plateau were commonly chosen as route ways to allow people to adapt to the cold, lack of oxygen, and gradually increasing altitude of the QTP. Scattered small agricultural bases that developed in areas of the QTP suitable for agricultural planting were essential bases for string exchange routes. Route formation is also associated with the apparent differentiation of the agro-pastoral industry between high and low elevations. Climate, ecological environment, and altitude are critical factors leading to differentiation. Interaction between agricultural and nomadic populations is the key motivation for forming and developing exchange routes. The simulated routes present an integrated picture of the complex human interaction and exchange process in the Neolithic to Bronze Age on the QTP. Early plateau routes reflect a gradual process of adaptation in production mode by people to the QTP. Our analysis also shows that the QTP was not a barrier to transcontinental cultural exchange between Asia and Europe but played an excellent intermediary role, which is crucial to the formation of the unique human landscape of the QTP.
Data availability statement
The datasets presented in this study can be found in online repositories. The names of the repository/repositories and accession number(s) can be found in the article/Supplementary Material.
Author contributions
ZL and GH conceived and designed the study. ZL, GH, CX, YJ, WW, JG, and ZW conducted fieldwork. ZL, GH, and CX analyzed data. ZL wrote the first draft of the manuscript. ZL, GH, CX, and ZW revised the manuscript.
Funding
This study was supported by the Natural Science Foundation of China (Grant Nos 42261030 and 42171165), The Qinghai Provincial Project for Thousand Top Innovative Talents (Grant No. 2019006), and the Qinghai province “Kunlun Talent - High-end Innovation and Entrepreneurship Talent” Program.
Acknowledgments
We are grateful for the manuscript reviews by the Editor and two reviewers. We thank Xiao Han Su, Xin Yao Tang, Xin Yue Feng, and Xin Long from Qinghai University for their help with collating data. We also thank Dr Pei Yang for the helpful discussion.
Conflict of interest
The authors declare that the research was conducted in the absence of any commercial or financial relationships that could be construed as a potential conflict of interest.
Publisher’s note
All claims expressed in this article are solely those of the authors and do not necessarily represent those of their affiliated organizations, or those of the publisher, the editors and the reviewers. Any product that may be evaluated in this article, or claim that may be made by its manufacturer, is not guaranteed or endorsed by the publisher.
Supplementary material
The Supplementary Material for this article can be found online at: https://www.frontiersin.org/articles/10.3389/feart.2022.1079055/full#supplementary-material
References
An, Z. S., Colman, S. M., Zhou, W. J., Li, X. Q., Brown, E. T., Jull, A. J., et al. (2012). Interplay between the westerlies and asian monsoon recorded in lake Qinghai sediments since 32 ka. Sci. Rep. 2 (1), 619–627. doi:10.1038/srep00619
Anthony, D. W. (2010). The horse, the wheel, and language: How bronze Age riders from the eurasian steppes shaped the modern world. Princeton: Princeton University Press.
Árnason, Ú. (2017). A phylogenetic view of the out of Asia/Eurasia and out of Africa hypotheses in the light of recent molecular and palaeontological finds. Gene 627, 473–476. doi:10.1016/j.gene.2017.07.006
Brantingham, P. J., Gao, X., Madsen, D. B., Rhode, D., Perreault, C., Woerd, J., et al. (2013). Late occupation of the high-elevation northern Tibetan Plateau based on cosmogenic, luminescence, and radiocarbon ages. Geoarchaeology 28 (5), 413–431. doi:10.1002/gea.21448
Chen, F. H., Dong, G. H., Zhang, D. J., Liu, X. Y., Jia, X., An, C. B., et al. (2015). Agriculture facilitated permanent human occupation of the Tibetan Plateau after 3600 B.P. Science 347 (6219), 248–250. doi:10.1126/science.1259172
Chen, F. H., Xia, H., Gao, Y., Zhang, D. J., Yang, X. Y., and Dong, G. H. (2022). The processes of prehistoric human activities in the Tibetan plateau: Occupation, adaptation and permanent settlement. Geogr. Sci. 42 (01), 1–14. [In Chinese]. doi:10.13249/j.cnki.sgs.2022.01.001
Chen, H. H., Wang, G. S., Mei, D. Z., and Suo, N. (1998). Excavation on Zongri site, tongde, Qinghai. Archaeology. 000 (005), 1-14, 35+97–101. CNKI:SUN:KAGU.0.1998-05-000[In Chinese].
Chen, Y. H. (2013). Technical application and measures of barley production in Gannan Prefecture. Gansu Agric. 000 (007), 6–11. [In Chinese]. doi:10.15979/j.cnki.cn62-1104/f.2013.07.029
Cheng, B., Chen, F. H., and Zhang, J. W. (2010). Palaeovegetational and palaeoenvironmental changes in Gonghe Basin since last deglaciation. Acta Geogr. Sin. 65 (11), 1336–1344. doi:10.11821/xb201011003
Chinese Academy of Agriculture Sciences (1995). Data from: Institute of Agricultural Natural Resources and Regional Planning, Chinese Academy of Agricultural Sciences. Establishment of National basic resources and dynamic information service system of environment remote sensing(96-B02-01): Construction data at the temperature and humidity data level at the background level of the ecological environment(96-B02-01-02). Available at: http://www.caas.cn/.
Chinese Academy of Sciences (2010–2016). Data from: Geospatial data Cloud Platform, computer network information center, Chinese Academy of Sciences. China 1km Surface Temperature Monthly Synthesis Product. Available at: http://www.gscloud.cn.
Chinese Academy of Sciences (2000). Data from: International scientific &technical data mirror site. China: Computer Network Information Center, Chinese Academy of Sciences. China 90mDEM data.
Chinese Academy of Sciences (2010–2016). Data from: National earth systems science data center, Chinese Academy of Sciences. 500m NDVI monthly synthetic product data in China's land area. Available at: http://www.gscloud.cn.
Chinese Academy of Sciences (1971–2000). Data from: Resource discipline innovation Platform. Average annual precipitation data of a 1km raster in China. Available at: http://www.data.ac.cn.
Chinese Academy of Sciences (2001). Data from: Resources and environment science and data center, Chinese Academy of Sciences. Spatial distribution data of vegetation types in China 1:1,000,000. Available at: http://www.resdc.cn.
Chinese Academy of Sciences (2001). Data from: Resources and environment science and data center, Chinese Academy of Sciences. China 1:250,000 river grading data. Available at: http://www.resdc.cn.
Cui, Y. H. (2015). Research on the Silk road in Qinghai: Jade road Qiang tathagate. J. Qinghai Natl. Inst. Soc. Sci. 41 (03), 38–42. doi:10.3969/j.issn.1674-9227.2015.03.006
Cui, Y. P., Hu, Y. W., Chen, H. H., Dong, Y., Guan, L., Weng, G. Y., et al. (2006). Stable isotopic analysis on human bones form ZongRi site. Quat. Sci. 26 (4), 604–611. doi:10.3321/j.issn:1001-7410.2006.04.016
Department of Animal Husbandry and Veterinary Medicine (1994), Ministry of agriculture of the People's republic of China. Data form: China agricultural science and technology press. Grassland Resource Data in China. Available at: http://www.castp.cn/.
Ding, M., Wang, T., Ko, A. M. S., Chen, H., Wang, H., Dong, G., et al. (2020). Ancient mitogenomes show plateau populations from last 5200 years partially contributed to present-day Tibetans. Proc. R. Soc. B 287, 20192968. doi:10.1098/rspb.2019.2968
Dong, G. H., Du, L. Y., Yang, L., Lu, M. X., Qiu, M. H., Li, H. M., et al. (2022a). Dispersal of crop-livestock and geographical-temporal variation of subsistence along the Steppe and Silk Roads across Eurasia in prehistory. Sci. China Earth Sci. 65 (7), 1187–1210. doi:10.1007/s11430-021-9929-x
Dong, G. H., Lu, Y. X., Liu, P. L., and Li, G. (2022b). Spatio-temporal patterns of human activities and their influencing factors along the ancient Silk road in northwest China from 6000 a B.P. To 2000 a B.P. Quat. Sci. 42 (01), 1–16+332. doi:10.11928/j.issn.1001-7410.2022.01.01
Dong, G. H., Yang, Y. S., Han, J. Y., Wang, H., and Chen, F. H. (2017). Exploring the history of cultural exchange in prehistoric Eurasia from the perspectives of crop diffusion and consumption. Sci. China Earth Sci. 047 (005), 1110–1123. doi:10.1007/s11430-016-9037-x
Dykoski, C. A., Edwards, R. L., Cheng, H., Yuan, D. X., Cai, Y. J., Zhang, M. L., et al. (2005). A high-resolution, absolute-dated Holocene and deglacial Asian monsoon record from Dongge Cave, China. Earth Planet. Sci. Lett. 233 (1-2), 71–86. doi:10.1016/j.epsl.2005.01.036
Frachetti, M. D. (2009). Pastoralist landscapes and social interaction in bronze Age eurasia. California: University of California Press.
Frachetti, M. D., Smith, C. E., Traub, C. M., and Williams, T. (2017). Nomadic ecology shaped the highland geography of Asia’s Silk Roads. Nature 543 (7644), 193–198. doi:10.1038/nature21696
Fu, D. X. (2001). Discovery, identification and study of neolithic crop remains in Changguogou site, xizang province. Archaeology 000 (003), 66–74. CNKI:SUN:KAGU.0.2001-03-007 [In Chinese].
Gai, P., and Wang, G. D. (1983). Excavation report on a mesolithic site at Layihai, upper yellow river. Acta Anthropol. Sin. 2 (01), 49–116. CNKI:SUN:RLXB.0.1983-01-005.
Gao, J. Y., Hou, G. L., Wei, H. C., Chen, Y. C., E, C. Y., Chen, X. L., et al. (2020). Prehistoric human activity and its environmental background in Lake Donggi Cona basin, northeastern Tibetan Plateau. Holocene 30 (5), 657–671. doi:10.1177/0959683619895583
Gao, Y., Yang, J., Ma, Z., Tong, Y., and Yang, X. (2021). New evidence from the Qugong site in the central Tibetan plateau for the prehistoric highland Silk road. Holocene 31 (2), 230–239. doi:10.1177/0959683620941144
Han, J. Y. (2013). The polychrome ceramic roads and early sino-western cultural communications. Archaeol. Cult. Relics 000 (001), 28–37. doi:10.3969/j.issn.1000-7830.2013.01.003
Han, J. Y. (2021). Three grand cultural spheres on the Holocene eurasian continent. Archaeology 000 (011), 64–75+2. CNKI:SUN:KAGU.0.2021-11-006[In Chinese].
Hong, L. Y., Cui, J. F., Wang, H., and Chen, J. (2011). Analysis and discussion on the source of faience pottery of the Majiayao type in Western Sichuan. South Ethnology Archaeol. 7 (00), 1–58. CNKI:SUN:NFMZ.0.2011-00-003[In Chinese].
Hou, G. L., E, C. Y., and Xiao, J. Y. (2012). Synthetical reconstruction of the precipitation series of the Qinghai-Tibet Plateau during the Holocene. Prog. Geogr. 31 (09), 1117–1123. doi:10.11820/dlkxjz.2012.09.001
Hou, G. L., E, C. Y., Yang, Y., and Wang, Q. B. (2016). Codependent and exchange: The source analysis of prehistoric pottery in the northeast Tibetan plateau. J. Earth Environ. 7 (06), 556–569. doi:10.7515/JEE201606003
Hou, G. L., Gao, J. Y., Chen, Y. C., Xu, C. J., Lancuo, Z. M., Xiao, Y. M., et al. (2020). Winter-to-summer seasonal migration of microlithic human activities on the Qinghai-Tibet Plateau. Sci. Rep. 10 (1), 1–13. doi:10.1038/s41598-020-68518-w
Hou, G. L., Lancuo, Z. M., Zhu, Y., and Pang, L. H. (2021). Communication route and its evolution on the Qinghai-Tibet Plateau during prehistoric time. J. Geogr. 76 (05), 1294–1313. doi:10.11821/dlxb202105018
Hou, G. L., Xu, C. J., Lv, C. Q., Chen, Q., and Lancuo, Z. M. (2019). The environmental background of Yangshao culture expansion in the mid-holocene. Geogr. Res. 38 (02), 437–444. doi:10.11821/dlyj020171214
Hou, G. L., Yang, P., Cao, G. C., Chong, Y. E., and Wang, Q. B. (2017). Vegetation evolution and human expansion on the Qinghai-Tibet Plateau since the last deglaciation. Quatern. Int. 430, 82–93. doi:10.1016/j.quaint.2015.03.035
Huerta-Sánchez, E., Jin, X., Bianba, Z., Peter, B. M., Vinckenbosch, N., Liang, Y., et al. (2014). Altitude adaptation in Tibetans caused by introgression of Denisovan-like DNA. Nature 512 (7513), 194–197. doi:10.1038/nature13408
Huo, W. (2000). From the new archaeological materials on the problem of copper mirror with handle in southwest China. Sichuan Cult. relics 000 (002), 3–8. CNKI:SUN:SCWW.0.2000-02-000[In Chinese].
Huo, W. (2013). Prehistoric transportation and trade in Tibet from archaeological findings. China Tibetol. 000 (002), 5–24. CNKI:SUN:CTRC.0.2013-02-003[In Chinese].
Huo, W. (2017). The formation, development and historical significance of the "Plateau Silk Road. Soc. Sci. 000 (011), 19–24. doi:10.3969/j.issn.1002-3240.2017.11.004
Jia, X. (2012). “Study on the cultural evolution and plant remains of Neolithic-Bronze Age in the northeast region of Qinghai Province,”PhD Thesis (Lanzhou: Lanzhou University).
Kang, L., Li, S., Gupta, S., Zhang, Y., Liu, K., Zhao, J., et al. (2010). Genetic structures of the Tibetans and the Deng people in the Himalayas viewed from autosomal STRs. J. Hum. Genet. 55 (5), 270–277. doi:10.1038/jhg.2010.21
Kong, D. D., Zhang, Q., Huang, W. L., and Gu, X. H. (2017). Vegetation phenology change in Tibetan Plateau from 1982 to 2013 and its related meteorological factors. Acta Geogr. Sin. 72 (1), 39–52. doi:10.11821/dlxb201701004
Lancuo, Z. M., Hou, G. L., Xu, C. J., Liu, Y. Y., Zhu, Y., Wang, W., et al. (2019). Simulating the route of the tang-tibet ancient road for one branch of the Silk road across the Qinghai-Tibet Plateau. PLoS One 14 (12), e0226970. doi:10.1371/journal.pone.0226970
Li, S. C. (2011). The origin and proliferation of sarcophagus burial -- the case of China. Sichuan Cult. Relics 000 (006), 64–69. CNKI:SUN:SCWW.0.2011-06-007[In Chinese].
Li, S. K. (1987). Agroclimatic regionalization of China. J. Nat. Resour. 000 (001), 71–83. doi:10.11849/zrzyxb.1987.01.008
Liu, J. Z. (1995). Tibetan plateau small Qaidam lake and the observation of each hear stone products. World Antiq. 000 (003), 6–20. CNKI:SUN:WWJK.0.1995-03-001[In Chinese].
Lv, H. L. (2009). Addition discussion of bronze handle-mirror of Tibet. J. Tibetol. 00 (00), 33–45+291. CNKI:SUN:ZAXK.0.2009-00-004[In Chinese].
Lv, H. L. (2007). Excavation on the dwelling site at Dingdong in ari prefecture, Tibet. Archaeology 000 (011), 36–46+102. CNKI:SUN:KAGU.0.2007-11-004[In Chinese].
Lv, H. L. (2014). Neolithic age of Western Tibet: A trans-himalayan perspective. Archaeology 000 (012), 77–89. CNKI:SUN:KAGU.0.2014-12-007[In Chinese].
Ma, J., Li, F. Y., Pang, G. W., Li, C. R., and Liu, W. (2017). The restoration of the ancient Silk road on land and analysis of geographical features along the route. Geogr. Geo-Information Sci. 33 (4), 123–128. doi:10.3969/j.issn.1672-0504.2017.04.021
Ma, M. M. (2013). “Dietary changes and agricultural development in Hehuang and its adjacent areas during the 2000 BC -- stable isotope evidence,” [PhD Thesis]. (Lanzhou: Lanzhou University).
Ma, Q. F., Zhu, L. P., Lv, X. M., Guo, Y., Ju, J. T., Wang, J. P., et al. (2014). Pollen-inferred Holocene vegetation and climate histories in Taro Co, southwestern Tibetan Plateau. Chin. Sci. Bull. 59 (26), 2630–2642. doi:10.1360/csb2014-59-26-2630
Ma, R. H., Yang, G. S., Duan, H. T., Jiang, J. H., Wang, S. M., Feng, X. Z., et al. (2011). China’s lakes at present: Number, area and spatial distribution. Sci. China Earth Sci. 41 (3), 283–289. doi:10.1007/s11430-010-4052-6
Madsen, D. B., Ma, H. Z., Brantingham, P. J., Gao, X., Rhode, D., Zhang, H. Y., et al. (2006). The late upper paleolithic occupation of the northern Tibetan plateau margin. J. Archaeol. Sci. 33 (10), 1433–1444. doi:10.1016/j.jas.2006.01.017
Qin, Z. D., Yang, Y. J., Kang, L. L., Yan, S., Cho, K., Cai, X. Y., et al. (2010). A mitochondrial revelation of early human migrations to the Tibetan Plateau before and after the last glacial maximum. Am. J. Phys. Anthropol. 143 (4), 555–569. doi:10.1002/ajpa.21350
Qiu, Q., Wang, L. Z., Wang, K., Yang, Y. Z., Ma, T., Wang, Z. F., et al. (2015). Yak whole-genome resequencing reveals domestication signatures and prehistoric population expansions. Nat. Commun. 6 (1), 10283–10287. doi:10.1038/ncomms10283
Ren, L. L., and Dong, G. H. (2016). The history for origin and diffusion of “Six livestock”. Chin. J. Nat. 38 (04), 257–262. doi:10.3969/j.issn.0253-9608.2016.04.005
Rhode, D., Madsen, D. B., Brantingham, P. J., and Dargye, T. (2007). Yaks, yak dung, and prehistoric human habitation of the Tibetan Plateau. Dev. Quat. Sci. 9, 205–224. doi:10.1016/S1571-0866(07)09013-6
Shen, J. B., Liu, X. Q., Wang, S. M., and Matsumoto, R. (2005). Palaeoclimatic changes in the Qinghai Lake area during the last 18, 000 years. Quatern. Int. 136 (1), 131–140. doi:10.1016/j.quaint.2004.11.014
Shi, P. J., Chen, Y. Q., Zhang, A. Y., He, Y., Gao, M. N., Yang, J., et al. (2019). Factors contribution to oxygen concentration in Qinghai-Tibetan Plateau. Chin. Sci. Bull. 64 (07), 715–724. doi:10.1360/N972018-00655
State Administration of Cultural Heritage (2011). Atlas of Chinese cultural Relics: Gansu fascicule. Beijing: Surveying and Mapping Publishing House.
State Administration of Cultural Heritage (1996). Atlas of Chinese cultural Relics: Qinghai fascicule. Beijing: China Map Press.
State Administration of Cultural Heritage (2009). Atlas of Chinese cultural Relics: Sichuan fascicule. Beijing: Cultural Relics Publishing House.
State Administration of Cultural Heritage (2010). Atlas of Chinese cultural Relics: Tibet autonomous region fascicule. Beijing: Cultural Relics Publishing House.
State Administration of Cultural Heritage (2001). Atlas of Chinese cultural Relics: Yunnan fascicule. Kunming: Yunnan Science and Technology Press.
Stevens, C. J., Murphy, C., Roberts, R., Lucas, L., Silva, F., and Fuller, D. Q. (2016). Between China and South Asia: A middle asian corridor of crop dispersal and agricultural innovation in the bronze age. Holocene 26 (10), 1541–1555. doi:10.1177/0959683616650268
Sun, H. L. (1996). New advances in the study of the Qinghai Tibetan plateau. Adv. Earth Sci. 11 (06), 18–24. doi:10.11867/j.issn.1001-8166.1996.06.0536
Sun, Y. D., Pei, X. H., and Lin, Z. (1998). Effects of low temperatures on human health. J. Med. Res. 27 (8), 26–28. CNKI:SUN:YXYZ.0.1998-08-014[In Chinese].
Tan, J. G., and Qu, H. C. (2003). The relationship between prehospital sudden death and meteorological variation. Meteorological Sci. Technol. 31 (01), 58–61. doi:10.3969/j.issn.1009-5918.2001.03.003
Tang, H. S. (2014). Revisiting issues related to prehistoric sites in Tibet including Karo and Qugong sites. Tibetan Acad. J. 000 (001), 11–31+236. CNKI:SUN:ZAXK.0.2014-01-002[In Chinese].
Tang, H. S. (2012). The economic types and related issues of the bronze age in Tibetan plateau. J. Tsinghua Univ. Philosophy Soc. Sci. Ed. 27 (01), 148–158+161. doi:10.13613/j.cnki.qhdz.002052
Tang, H. S., Zhou, C. L., Li, Y. Q., and Liang, Z. (2013). A new discovery of microlithic information at the entrance to the northern qingzang plateau of the Kunlun mountains of Qinghai. Chin. Sci. Bull. 58 (03), 247–253. doi:10.1360/972012-550
Tong, T. (2010). The source of the decorative style of the three Tibetan bronze mirrors with handles. J. Tibetan Stud. 00 (01), 137–148+294. CNKI:SUN:ZAXK.0.2010-00-009[In Chinese].
Wang, J., Xia, H., Yao, J. T., Sheng, X. K., Cheng, T., Wang, Q. Q., et al. (2020). Subsistence strategies of prehistoric hunter-gatherers on the Tibetan plateau during the last deglaciation. Sci. China Earth Sci. 50 (03), 395–404. doi:10.1007/s11430-019-9519-8
Wang, X. M., Xie, J. F., Wang, B. S., and Qin, Y. M. (2002). Analysis of the relationship between Brain-Heart Vascular Syndrome and meteorological elements and medical meteorological grade prediction. Jilin Meteorol. 000 (003), 32–35. CNKI:SUN:JLQX.0.2002-03-010[In Chinese].
Wei, H. C., Chongyi, E., Zhang, J., Sun, Y. J., Li, Q. K., Hou, G. L., et al. (2020a). Climate change and anthropogenic activities in Qinghai Lake basin over the last 8500 years derived from pollen and charcoal records in an aeolian section. Catena 193, 104616. doi:10.1016/j.catena.2020.104616
Wei, H. C., Hou, G. L., Fan, Q. S., Madsen, D. B., Qin, Z. J., Du, Y. S., et al. (2020b). Using coprophilous fungi to reconstruct the history of pastoralism in the Qinghai Lake Basin, northeastern Qinghai-Tibetan plateau. Prog. Phys. Geogr. Earth Environ. 44 (1), 70–93. doi:10.1177/0309133319869596
Wu, R. S., and Ma, Y. M. (2010). Comparative analysis of the radiation characteristics of the Qinghai-Tibet Plateau different from that of the Earth and trees. Plateau meteorol. 29 (02), 251–259. Available at: http://iritpcas.ac.cn:8080/handle/131C11/1546.
Wu, Y. H., Lücke, A., Jin, Z. D., Wang, S. M., Schleser, G. H., Battarbee, R. W., et al. (2006). Holocene climate development on the central Tibetan plateau: A sedimentary record from cuoe lake. Palaeogeogr. Palaeoclimatol. Palaeoecol. 234 (2-4), 328–340. doi:10.1016/j.palaeo.2005.09.017
Xiang, J. H. (2018). Re-examination of the sources of faience pottery in Majiayao culture in Western Sichuan Province, focusing on the analysis of the chemical composition of pottery. Sichuan Cult. Relics 000 (004), 81–90. CNKI:SUN:SCWW.0.2018-04-010[In Chinese].
Yang, X. W., and Ye, D. X. (2003). Medical meteorological research on brain-heart vascular syndrome in China. Meteorological Sci. Technol. 31 (6), 376–380. doi:10.3969/j.issn.1671-6345.2003.06.012
Yi, M. J., Gao, X., Zhang, X. L., Sun, Y. J., Brantingham, P. J., Madsen, D. B., et al. (2011). A preliminary report on investigations in 2009 of some prehistoric sites in the Tibetan plateau marginal region. Acta Anthropol. Sin. 30 (002), 124–136. doi:10.16359/j.cnki.cn11-1963/q.2011.02.002
Zeder, M. A. (2008). Domestication and early agriculture in the Mediterranean Basin: Origins, diffusion, and impact. Proc. Natl. Acad. Sci. 105 (33), 11597–11604. doi:10.1073/pnas.0801317105
Zeng, X. Q., Guo, Y., Xu, Q. J., Mascher, M., Guo, G. G., Li, S. C., et al. (2018). Origin and evolution of qingke barley in Tibet. Nat. Commun. 9 (1), 5433–5511. doi:10.1038/s41467-018-07920-5
Zha, R. B., Sun, G. N., Dong, Z. B., and Yu, Z. K. (2016). Assessment of atmospheric oxygen practical pressure and plateau reaction of tourists in the Qinghai-Tibet Plateau. Ecol. Environ. Sci. 25 (01), 92–98. doi:10.16258/j.cnki.1674-5906.2016.01.013
Zhang, D. J., Dong, G. H., Wang, H., Ha, B. B., and Qiang, M. R. (2016). Historical processes and possible driving mechanisms of prehistoric human dispersal to the Qinghai-Tibetan Plateau. Sci. China Earth Sci. 46 (08), 1007–1023. doi:10.1007/s11430-015-5482-x
Zhang, D. J., Xia, H., Cheng, T., and Chen, F. H. (2020). New portraits of the denisovans. Sci. Bull. 65 (01), 1–3. doi:10.1016/j.scib.2019.10.013
Zhang, D., Matthew, R. B., Chen, H., Wang, L. B., Zhang, H. W., Sally, C. R., et al. (2021). Earliest parietal art: Hominin hand and foot traces from the middle Pleistocene of Tibet. Sci. Bull. 66 (24), 2506–2515. doi:10.1016/j.scib.2021.09.001
Zhang, M. H., Yan, S., Pan, W. Y., and Jin, L. (2019). Phylogenetic evidence for Sino-Tibetan origin in northern China in the late neolithic. Nature 569 (7754), 112–115. doi:10.1038/s41586-019-1153-z
Zhang, S. J., and Dong, G. H. (2017). Human adaptation strategies to different altitude environment during mid-late bronze age in northeast Tibetan plateau. Quat. Sci. 37 (4), 696–708. doi:10.11928/j.issn.1001-7410.2017.04.03
Zhang, X. L., Ha, B. B., Wang, S. J., Chen, Z. J., Ge, J. Y., Long, H., et al. (2018). The earliest human occupation of the high-altitude Tibetan Plateau 40 thousand to 30 thousand years ago. Science 362 (6418), 1049–1051. doi:10.1126/science.aat8824
Zhang, Y. L., Li, B. Y., Liu, L. S., and Zheng, D. (2021). Redetermine the region and boundaries of the Qinghai Tibetan plateau. Geogr. Res. 40 (6), 1543–1553. doi:10.11821/dlyj020210138
Zhang, Y. (2016). The discovery and dissemination of arsenic and copper in ancient China. World Antiq. 00 (02), 20–23. doi:10.3969/j.issn.1009-1092.2016.02.007
Zhao, C. Y., Wang, M. H., and Ye, M. L. (2016). Strontium isotope analysis of human teeth and bones from the Lajia site in Qinghai province. Acta Anthropol. Sin. 35 (02), 212–222. doi:10.16359/j.cnki.cn11-1963/q.2016.0019
Zhao, H. M. (1994). Problems related to the iron handle bronze mirror unearthed in Qugong, Tibet. Archaeology 000 (007), 642–649. CNKI:SUN:KAGU.0.1994-07-008[In Chinese].
Zheng, D., Zhang, R. Z., and Yang, Q. Y. (1979). Discussion on the natural zone of the Qinghai-Tibet Plateau. Acta Geogr. Sin. 34 (1), 1–11. CNKI:SUN:DLXB.0.1979-01-000[In Chinese].
Zheng, D., and Zhao, D. S. (2017). Characteristics of the natural environment of the Tibetan Plateau. Sci. Technol. Rev. 35 (06), 13–22. doi:10.3981/j.issn.1000-7857.2017.06.001
Keywords: Qinghai-Tibet Plateau, route simulation, development and evolution, Neolithic, bronze
Citation: Lancuo Z, Hou G, Xu C, Jiang Y, Wang W, Gao J and Wende Z (2023) Simulation of exchange routes on the Qinghai-Tibetan Plateau shows succession from the neolithic to the bronze age and strong control of the physical environment and production mode. Front. Earth Sci. 10:1079055. doi: 10.3389/feart.2022.1079055
Received: 25 October 2022; Accepted: 21 December 2022;
Published: 10 January 2023.
Edited by:
Ren Lele, Lanzhou University, ChinaReviewed by:
Xiaolin Ren, Institute for the History of Natural Sciences (CAS), ChinaLi Wu, Anhui Normal University, China
Copyright © 2023 Lancuo, Hou, Xu, Jiang, Wang, Gao and Wende. This is an open-access article distributed under the terms of the Creative Commons Attribution License (CC BY). The use, distribution or reproduction in other forums is permitted, provided the original author(s) and the copyright owner(s) are credited and that the original publication in this journal is cited, in accordance with accepted academic practice. No use, distribution or reproduction is permitted which does not comply with these terms.
*Correspondence: Zhuoma Lancuo, lczm1980@163.com; Guangliang Hou, hgl20@163.com