Soils and terrestrial sediments on the seafloor: Refining archaeological paleoshoreline estimates and paleoenvironmental reconstruction off the California coast
- 1Department of Anthropology, Natural History Museum Los Angeles County, Los Angeles, CA, United States
- 2Department of Geological Sciences, San Diego State University, San Diego, CA, United States
- 3Department of Anthropology, San Diego State University, San Diego, CA, United States
- 4Department of Earth Sciences, University of Oregon, Eugene, OR, United States
- 5Museum of Natural and Cultural History, University of Oregon, Eugene, OR, United States
- 6Department of Earth and Ocean Sciences, University of North Carolina Wilmington, Wilmington, NC, United States
- 7The Center for Marine Science, University of North Carolina Wilmington, Wilmington, NC, United States
- 8Department of Anthropology, California State University Los Angeles, Los Angeles, CA, United States
On global, regional, and local scales, sea level histories and paleoshoreline reconstructions are critical to understanding the deep history of human adaptations in island and coastal settings. The distance of any individual site from the coast strongly influences decisions about the transport of coastal resources and has a direct impact on human settlement and resources procurement strategies. Our ability, then, to identify relic productive habitats, such as wetlands, that were subaerial during time periods relevant to human occupation, is critical to models of human settlement and resource patterning that guide our search to identify cultural resources. Accurate location of productive habitats becomes more critical when searching for terminal Pleistocene sites submerged by postglacial marine transgression. While paleoshoreline reconstructions and sea level histories can provide a baseline for identifying drowned and ancient coastal ecosystems, post-transgressive sediment deposited on the seafloor can skew accurate paleoshoreline location. To correct for this, we used sub-bottom profiling data from the southern California Coast to determine revised paleoshoreline locations and to identify sonar signatures indicative of paleogeographic contexts that may harbor wetland environments. These data were used to define core sample locations that resulted in the identification of submerged, preserved paleosols. The paleosols data, presented here for the first time, have provided information on ancient landscapes and relic habitats that were subaerial prior to postglacial sea level rise. In our study area on the continental shelf off the California Channel Islands archipelago, the paleosols correspond to a critical period of shifting habitats, evolving landscapes, species extinctions, and the arrival of humans into a rapidly changing ecosystem.
1 Introduction
The Pleistocene-Holocene transition and the preceding millennia were turbulent periods worldwide. The end of the last glacial cycle was ushered in ∼20,000 years ago with a warming climate, melting glaciers and ice sheets, rising seas, and reemerging habitats adjusting to the thaw. Globally, the ten millennia following the Last Glacial Maximum was a time of human dispersal into, and adaptations to, new and changing landscapes. This was particularly true for the coastal regions of the Americas. Beginning at least 14,000 years ago, but potentially several millennia earlier (Dillehay et al., 2008; Dillehay et al., 2012; Erlandson et al., 1996; McLaren et al., 2018), Homo sapiens settled across these coastal regions, taking advantage of thriving marine and coastal ecosystems. That coastal habitats harbor some of the earliest evidence for habitation in the Americas should come as no surprise. Ocean and coastal ecosystems and the habitats and resources they support have been intertwined with the human story for over 100,000 years. Whether providing food for subsistence (Singer and Wymer 1982; Cortés-Sánchez et al., 2011; Marean et al., 2007; Steele and Álvarez-Fernández 2011), seagrasses and seaweeds for twine (Connolly et al., 1995), shells for artistic expression (Joordens et al., 2015), or dispersal routes into new lands (Erlandson 2001; O’Connor et al., 2011; Erlandson and Braje 2015), aquatic spaces have impacted both the biological and cultural development of our species (Henderson 2019). The deep time history of these spaces make them integral for understanding our past.
Clarifying the role that coastlines played in the millennia after the last glacial cycle is complex. As ice sheets melted and seas rose, over 9.5 million km2 of land was inundated from 20,000–6,000 years ago (see Dobson 2014). In some regions not completely submerged, the amount of land lost to sea level rise is equal to or greater than the subaerial landscape of today; the islands in the Southern California Bight (SCB), a geographic region extending from Point Conception to San Diego in Southern California (Figure 1), are a prime example. The amount of inundated paleolandscape in this region is a key factor in considering coastal social science research focused on the time period during eustatic sea level rise. Rather than honing efforts only on the subaerial portion of the landscape, the regional seascape must also be considered an integral factor in coastal archaeological research. By integrating data from the land- and sea-scape, researchers consider a maritime cultural landscape (Westerdahl 1992), that deemphasizes a modern waterline divide that has shifted through time. The recognition that landscapes and seascapes shift over time and associated habitats are not static, is critical to understanding human-environmental dynamics and interactions (Ford 2011). The importance of maritime cultural landscape studies that consider submerged paleolandscapes is particularly salient in the SCB, which holds some of the earliest evidence for human use of coastal resources in the Americas (Erlandson et al., 2011; Gusick and Erlandson 2019), but has been significantly impacted by post glacial marine transgression.
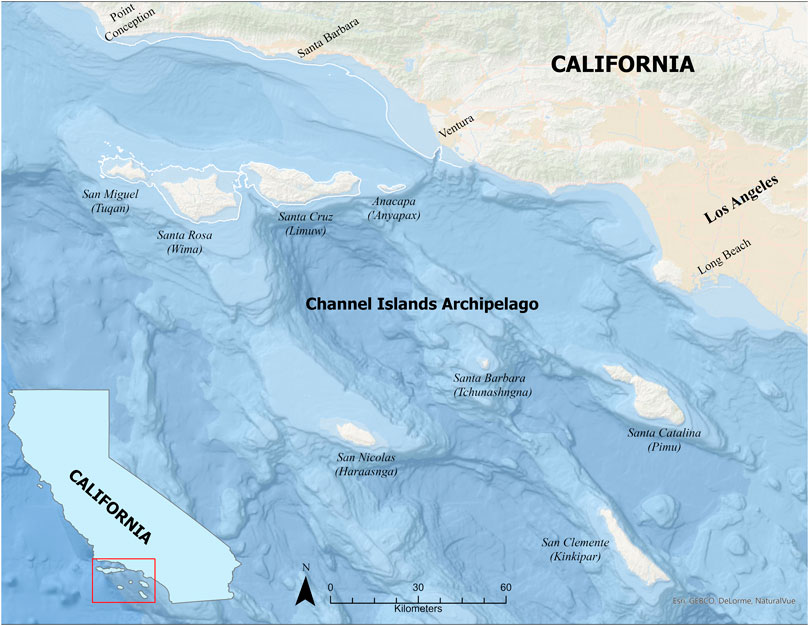
FIGURE 1. Map of the northern portion of the Southern California Bight and the Channel Islands archipelago showing both the southern and northern groups. White boundary around the NCI is the 70 m isobath showing the general extent of Santarosae and the adjacent mainland ∼13,000 years ago.
The impact of postglacial submergence is acutely evident when considering Santarosae, a maritime cultural landscape located within the SCB. Santarosae includes the subaerial Northern Channel Island (NCI) landmasses and the continental shelf that surrounds them. Early human habitation on these islands is apparent in the 20 or so archaeological sites dating to 11,000 cal BP and earlier identified on the subaerial landscape (Gusick and Erlandson 2019). Directly after ∼11,000 cal BP, there is evidence for a growing population on the islands (Braje et al., 2020), with over 120 sites dating to the Early Holocene (11,000–8,000 cal BP). The variation in number between the terminal Pleistocene and Early Holocene sites on Santarosae suggest that many more pre-11,000 cal BP sites are present on the more than 200 km2 of land submerged after the Last Glacial Maximum. To search for evidence of a more extensive terminal Pleistocene occupation, and to clarify the timing of habitation in the region, researchers must consider the maritime cultural landscape of Santarosae and integrate what is known from the current subaerial portion of the landscape with the submerged paleolandscape. Important in this endeavor is identifying areas on the continental shelf that present preservation potential. While modelling can help focus research on areas that likely contain preserved sections of the terminal Pleistocene subaerial landscape, actual identification of preserved paleosols through sampling is critical to reconstructing past landscapes and environments and possibly identifying preserved terminal Pleistocene archaeological sites on the continental shelf. Because paleosols form on past landscapes, they can be analyzed to reconstruct the paleoenvironment present during their formation. Submerged preserved paleosols are also markers of sections of the ancient landscape that survived marine transgression intact. This is particularly important in areas such as the SCB where high tidal and wave energy erode the continental shelf, destroying any archaeological sites it may have contained.
The SCB, and Santarosae in particular, has been a primary focus of submarine mapping and coring efforts of the Archaeological and Biological Assessment of Submerged Landforms off the Pacific Coast project (Braje et al., 2021). Funded by the Bureau of Ocean Energy Management, this broad and multidisciplinary study occurred from 2012–2020 and included submerged landscapes around the NCI and off the coast of Oregon. Project partners included federal agencies, universities, museums and tribal nations and included a variety of specialists ranging from archaeologists to, marine geologists, marine biologists, marine geophysicists, and tribal knowledge caretakers. Designed to better understand the paleoenvironment and paleolandscape of Santarosae, this project included initial data synthesis of archaeological and environmental records combined with geological and geomorphic seabed data to develop a model to guide field research (Braje et al., 2021). Areas selected for the field investigation had a high likelihood of preservation of cultural material and/or may present paleolandscapes or paleohabitat data to help clarify the extent of land and the nature of terminal Pleistocene-aged Santarosae. Field investigations included broad-scale sonar survey for submerged paleolandscape characterization followed by tightly gridded sonar surveys from which core locations were selected. Twenty-six cores were collected from the submerged landscape of Santarosae and preserved paleosols were identified in three of the core samples. This is the first known collection of preserved paleosols from the submerged section of Santarosae and they have provided data to consider the evolution of the paleolandscape and the paleoenvironment that existed on the island prior to its partial inundation. The identification of the preserved paleosols is a critical development in the ongoing efforts to identify regions where archaeological sites may be preserved on submerged landscapes in the SCB.
2 Materials and methods
2.1 Study area
The NCI are part of the 257-km-long Channel Islands archipelago located in the SCB (Figure 1). The eight islands that form the chain are typically divided into two groups: the southern Channel Islands, Santa Barbara (Tongva name: Tchunashngna), San Clemente (Kinkipar), San Nicolas (Haraasnga), and Catalina (Pimuu’nga); and the NCI, San Miguel (Chumash Name: Tuqan), Santa Rosa (Wima), Santa Cruz (Limuw), and Anacapa (‘Anyapax). These islands have the cool wet winters and warm summers typical of the Mediterranean climate in Southern California, which supports a surprising richness of vascular plants (Gill et al., 2019). The Channel Islands archipelago is in one of five world regions with Mediterranean-type ecosystems—California, Central Chile, the Mediterranean Basin, the Cape Region of South Africa, and Southwestern and South Australia—that collectively cover only about 2 percent of the world’s landmass, but comprise 20 percent of the world’s vascular plant species (Esler et al., 2018). The marine ecosystems within these regions also tend to be rich, making them ecological “hot spots” that have long been attractive for human habitation. The waters surrounding the NCI contain a rich marine ecosystem supported by seasonal nutrient rich upwelling and extensive kelp forests (Graham et al., 2009; Erlandson and Braje 2015). This combination supports a biodiversity that is among the richest in the world (Steneck et al., 2002), where kelp forests support shellfish, fish, marine mammals, sea birds, and seaweeds. This region is part of the proposed “Kelp Highway” that extends from Japan to Baja California where the richness of kelp forest resources may have supported a terminal Pleistocene coastal migration of humans into the Americas (Erlandson et al., 2007).
The NCI are located in the western Transverse Ranges Province. This physiographic province is restrained on the west by the Big Bend in the San Andreas Fault, resulting in transpression and associated faulting and folding, and creating a structural orientation that is more east/west than the typical northwest/southeast structural trend of the Pacific-North American plate boundary (Atwater, 1987). While the landscapes of the four islands vary, coastal areas on the islands are characterized by rocky shores and sea cliffs, separated by sandy pocket beaches and dunes (Schoenherr et al., 2003). These coastal areas transition into emergent marine terrace and mountainous areas with varying topography among the islands. The marine terraces on the NCI have a long history of study, and formed through a combination of uplift and oscillating sea level through glacial and interglacial stages of the past two million years (Muhs et al., 2014). During periods of relative sea level stability, broad, and gently seaward dipping abrasion platforms were created by wave-base erosion at the coastline. On uplifting margins, the abrasion platforms created at sea-level highstands can be preserved above sea level, forming the step like landscape, with broad flat marine terraces that characterize the California coast and the NCI (Sorlien 1994; Dickinson 2001; Pinter et al., 2003; Muhs et al., 2014). Abrasion platforms created at times of lower sea level have been submerged by rising seas, but their morphology may be preserved (Klotsko et al., 2015), and several have been identified in geophysical data surrounding the NCI (Emery 1958; Chaytor et al., 2008; Laws et al., 2019). These submerged platforms can record the history of coastal landscape and paleoshoreline changes around the islands.
Around the NCI, there are five submerged abrasion platforms between ∼15 and 130 m below sea level (mbsl) evident on bathymetric profiles (Emery 1958). More recently, Chaytor et al. (2008) used high-resolution multibeam bathymetry to map five submerged abrasion platforms at depths ranging from ∼22 to 90 mbsl offshore from Santa Cruz Island. If ancient shorelines on these terraces are preserved, they can be identified by an increase in slope on the landward edge of the wave-cut abrasion platform. The base of the slope is known as the shoreline angle, and represents the location of a preserved ancient shoreline. The locations and dates of these paleoshoreline angles are critical in clarifying proposed relative sea level rise and uplift rates, which informs understanding of paleolandscape formation (Lambeck et al., 2002; Chaytor et al., 2008; Muhs et al., 2014; Laws et al., 2019). This is particularly important because sea level rise following the last deglaciation significantly transformed the offshore landmasses. Although never connected to the mainland, the NCI once formed a super island called Santarosae located ∼7 km from the mainland continental coast at the end of the last ice age (Orr 1968). Chumash descendant and linguistic scholar Matt Vestuto has suggested a Chumash name for Santarosae, Shamalaḿa, meaning “it was one.” As glacier meltwater poured into the oceans starting around 20,000 years ago, sea level rose 100–110 m until stabilization about 7,000 years ago (Clark et al., 2014). During this massive sea level rise, roughly 75 percent of the landmass of Santarosae was inundated, severing the land bridges between the islands and creating the four NCI present today. The inundation of the archipelago affected shoreline length and island size, greatly restructuring marine habitats, and significantly increasing the distance between the NCI and the mainland (Kinlan et al., 2005; Reeder-Myers et al., 2015). The timing of this inundation is significant as it occurred during a period relevant to human dispersal onto the island.
Human remains dated to approximately 13,000 years old were identified in 1959 on northwestern Santa Rosa Island in Arlington Canyon (Orr 1962; Johnson et al., 2002), and represent the oldest remains identified to date on the Pacific Coast of the Americas. Far from being an isolated case of early occupation, the subaerial remnants of the partially submerged Santarosae contain some of the most extensive evidence for Paleocoastal occupation (13,000–8,000 cal BP) in the Americas (Erlandson et al., 2011; Gusick and Erlandson 2019). More than 120 Paleocoastal sites have now been recorded on the NCI, including more than 11 that have material radiocarbon dated to 11,000 cal BP and older, and at least 19 additional sites that have no organic datable material, but contain diagnostic technology suggesting a probable terminal Pleistocene age (Braje et al., 2013; Braje and Erlandson 2008; Erlandson et al., 2008; Erlandson et al., 2016; Rick, 2008; Rick, 2009; Rick et al., 2005a; Rick et al., 2013; Rick and Erlandson 2012). The peoples who arrived on Santarosae prior to 11,000 years ago would have adapted to one large landmass with abundant pines and numerous streams and wetland environments transitioning into sandy beaches, rocky shores, and productive intertidal and subtidal habitats. As seas rose and inundated the land bridges between the islands during the Pleistocene-Holocene transition (Reeder-Myers et al., 2015) the changes to regional coastal ecology would have been significant with shifting intertidal to subtidal habitats, inundation of river valleys, and flooding and shifting of estuaries and lagoons (Masters and Aiello 2007:40). Today there are no extant estuaries on the NCI, but archaeological evidence suggests that estuaries were present during times of lowered sea levels and were exploited by island groups (Erlandson 1994; Erlandson et al., 2011; Rick et al., 2005b; Erlandson et al., 2019).
2.1.1 Santa Cruz Passage and Crescent Bay
The Santa Cruz Passage is the body of water between Santa Cruz and Santa Rosa islands. This portion of the drowned landscape of Santarosae has been a focus of efforts to document the paleolandscape and paleoecological transitions that occurred when rising seas inundated this region. The margins of this passage, represented by the currently subaerial landscapes of western Santa Cruz and eastern Santa Rosa islands, hold clusters of Paleocoastal sites (Gusick, 2012; Gusick, 2013; Erlandson et al., 2016; Erlandson et al., 2019; Gusick and Erlandson 2019; Gill et al., 2021). The entire roughly 15 km span between the current shorelines on the margins of the Santa Cruz Passage was subaerial until ∼11,000 years ago, with broad plains traversed by several large drainages that likely formed a mix of wetland habitats and sandy beaches. The biological richness in these habitats are hot spots for human exploitation, replete with abundant shellfish, sea birds, waterfowl, and pinnipeds. Considering the paleogeography of this region along with the Paleocoastal sites led Erlandson et al. (2016) to identify a large south-facing, semi-protected paleo-embayment in this region that he dubbed Crescent Bay. Several of the largest drainages on Santarosae likely flowed into this large (∼8 km wide) embayment and then into the large offshore Santa Cruz Submarine Canyon (see Figure 3). Recent research suggests that this area also contained a paleovalley (Maloney et al., 2017; Johnson 2020) and the semi-protected nature of Crescent Bay—protected from the dominant winds and swells that battered the north coast of Santarosae—provides a setting where submerged archaeological sites are most likely to have been protected from marine erosion as sea levels rose. It is clear that one or more estuaries existed around the margins of Crescent Bay, the only examples known from California’s Channel Islands. Two shell middens (CA-SCRI-857 and CA-SRI-708) located above current sea level around the margins of the bay provide some of the earliest evidence for human harvesting of estuarine clams (Chione spp.) in North America, between ∼11,400 and 11,200 cal BP (Erlandson et al., 2019; J. Erlandson, personal communication, 8 May 2022).
Aside from productive habitats, Crescent Bay may also have been the location of two tar seeps that have been recorded near the western Santa Cruz Island coastline (Roberts 1991; Braje et al., 2005). Asphaltum (bitumen/tar) is a natural substance that has been used by humans for 70,000 years (Boëda et al., 2008), and in this region, there is evidence of its use dating back more than 9,000 years (Erlandson et al., 2008; Hodgson 2004; McCawley 1996; Salwen 2011). Importantly, it was used as an adhesive in technologies, such as hafting projectile points to shafts, and as a water-proofing agent for woven water bottles and watercraft that were integral to the maritime hunter-gathers cultures in the region (Connan 1999; Fauvelle 2014; Oron et al., 2015). Some of the larger known tar seeps on the adjacent mainland have extensive archaeological sites near them (Moore et al., 2007; Gamble 2008), and archaeological sites on the NCI contain evidence of asphaltum use throughout human occupation of the islands (Braje et al., 2005; Brown 2016). Such tar seeps were another resource that made the Crescent Bay area attractive for groups settling the islands in the terminal Pleistocene and earliest Holocene.
The Santa Cruz Passage and Crescent Bay have been a focus of the Archaeological and Biological Assessment of Submerged Landforms off the Pacific Coast project, which to date has resulted in numerous publications and graduate theses (Braje et al., 2019; Braje et al., 2022; Gusick et al., 2021; Johnson 2020; Laws et al., 2019; Tahiry 2019), based off the data generated by both broad and tight-gridded sonar surveys conducted with an Edgetech 512i (0.5–16 kHz) Chirp system. Particularly relevant to the selection of core locations are three studies that clarified paleodrainage morphology and evolution (Tahiry 2019), locations of paleoshorelines and uplift rates (Laws et al., 2019; Braje et al., 2022), and extent of marine sedimentation (Johnson 2020). In the Crescent Bay area off the western coast of Santa Cruz Island, a wide paleovalley (up to ∼3.5 km wide and ∼16 m deep) was identified within the Chirp data (Figure 2) (Maloney et al., 2017; Tahiry 2019; Johnson, 2020). This paleovalley contained smaller, nested channel features, and was filled with an acoustic unit exhibiting unique signatures consisting of high-amplitude, wavy, sub-parallel, discontinuous reflectors with transparent to chaotic signature between reflectors. This unit was interpreted to be characteristic of fluvial-estuarine deposits, filling the paleovalley feature. Closer to shore the acoustic signature is more chaotic and stratigraphy is difficult to observe. This unique signature is predominately imaged within Santa Cruz Passage, with most of its distribution directly offshore from Santa Cruz Island.
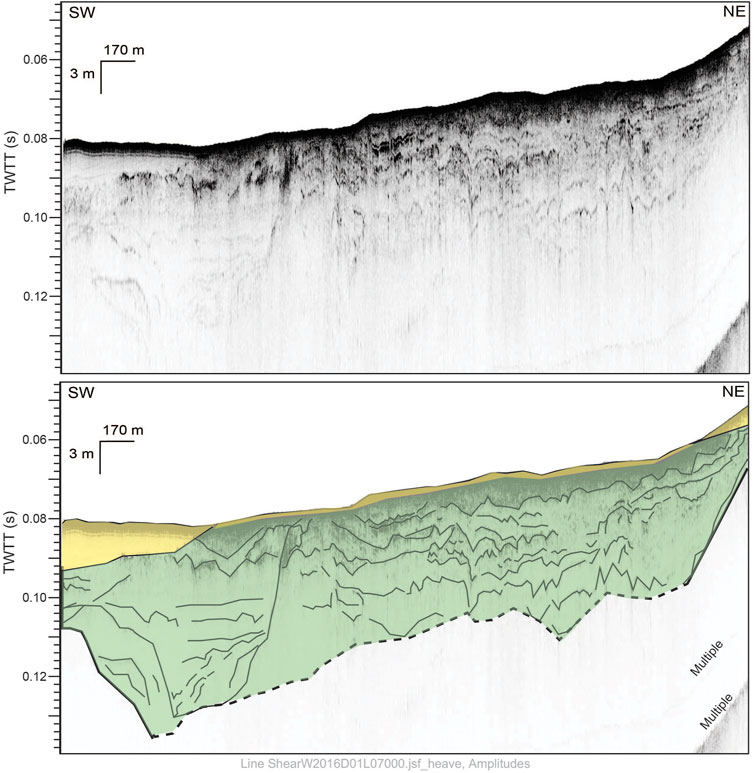
FIGURE 2. Uninterpreted (top) and interpreted (bottom) seismic line from area off Santa Cruz Island where Box 6 is located. Transparent yellow unit is interpreted as marine. Green unit with wavy reflectors is interpreted as terrestrial. High amplitude reflectors are traced in interpretation to show sedimentary structure. See Figure 3 for location (adapted from Johnson 2020).
Using these same Chirp data in the section of Crescent Bay near to Santa Cruz Island, Laws et al. (2019) mapped six paleoshoreline angles identified at 39, 40, 41, 59, 60, and 61 mbsl (rounded to the nearest whole number). The identification of paleoshorelines angles is significant as elevation and ages of preserved paleoshorelines on marine terraces can be used to reconstruct sea level, uplift, and deformation histories (Kern and Rockwell 1992; Kelsey and Bockheim 1994; Muhs et al., 2012; Muhs et al., 2014; Haaker et al., 2016), which are commonly used in marine archaeological (and other) research to focus survey where there is a high likelihood to recover target data. For example, based on a glacial-isostatic adjustment model, Clark et al. (2014) suggest the Last Glacial Maximum lowstand was 106 mbsl. Using this sea level curve, researchers may model the extent of Santarosae landmass at that time by identifying the 106 m isobaths that surround the NCI; however, isobaths measures distance from the current sea level to the sea floor. It does not account for post sea-level rise deposition of sediments offshore, which can be extensive in the SCB region (Erlandson 2021). The marine sediment that lies atop the actual exposed surface that existed during lower sea level (e.g., the subaerial surface) can impact the accuracy of modelled paleoshoreline depth. In fact, results from Laws et al. (2019) suggest that at the Last Glacial Maximum, Santarosae was not as large as predicted using paleoshoreline models from modern bathymetry.
The significance of identifying paleoshorelines angles to update models of paleoshorelines locations and their relevance to identification of submerged archaeological site location is highlighted in Braje et al. (2022). Using the Laws et al. (2019) paleoshoreline angles identified off Santa Cruz Island, Braje et al. (2022) recalculated distance to paleoshorelines, compared to bathymetric models, for sites occupied along the margins of the Santa Cruz Passage. Measuring distance from a cluster of Early Holocene-aged sites (CA-SCRI-547, -549, and -798) dated between ∼9,000 and 8,500 cal BP, the 40 m bathymetric contour was 2.44 km further from the sites than the Chirp identified 40 m paleoshoreline angle (Braje et al., 2022; Figure 4C). This suggests that the shoreline during occupation of the sites was significantly closer to the sites than initially modelled. This significant difference in the two modelling methods is likely because this is a geologically complex area, where several large drainages empty into the ocean (Schumann and Pigati 2017). This context may have caused more extensive marine sedimentation that deeply buried the abrasion platform and paleodrainages. When considering archaeological histories and ancient land use in this region, distance to shoreline is significant. Proximity to coastal resources and adjacent productive habits is critical in estimating settlement and subsistence patterns and possible site locations (Erlandson 2001). A variation of 2.0–2.5 km could shift modelling efforts when considering prioritization of habitats, creating an inaccurate model for settlement patterns. When searching on the continental shelf for possible preserved archaeological sites, a difference of 2.0–2.5 km may result in collection of data that was not subaerial during the time period of interest.
2.2 Methods
The Chirp data analyses described above were critical in selection of core locations. An initial broad landscape survey offshore of the NCI included collection of 65 regional survey lines over ∼190 linear km, including 21 sonar lines collected over ∼95 linear km within the Santa Cruz Passage. Data were collected from NOAA’s R/V Shearwater towing an Edgetech 512i (0.5–16 kHz) Chirp with line spacing varying between 350 and 700 m. The Chirp subbottom profiler provided submeter vertical resolution and ∼40 m sub-seafloor imaging. Chirp data were processed using SIOSEIS (Henkart 2006) and Seismic Unix software (Cohen and Stockwell 1999) to remove heave artifacts and adjust gains. Data were imported into IHS Kingdom and ESRI ArcGIS v. 10.5 (www.esri.com) for interpretation. Kingdom was used to calculate layer thicknesses and the depth to acoustic reflectors. A nominal sound velocity of 1,500 m/s was used to convert time to depth.
These initial broad geophysical surveys were used to investigate large swaths of the seafloor and identify features such as paleochannels, canyons, dune fields, paleoshorelines, faults, and terraces, as well as buried geological units. Based on these data, four 1 km2 areas were selected for targeted, tight-grid spacing (25 m) geophysical surveys. Data were collected from the R/V Point Loma using an Edgetech 512i profiler at 1–15 kHz. These surveys focused on small sections of the landscape deemed to have high potential to yield paleolandscape and paleoenvironmental data during the sampling phase of the project. One of these 1 km2 areas, identified as Box 6, was located just west of Santa Cruz Island, and was selected due to the interpreted paleovalley (Maloney et al., 2017; Tahiry 2019) and unique deposition (Johnson 2020) identified in the Chirp data and described above. Within Box 6, 41 survey lines were collected covering ∼41 linear km (Figure 3).
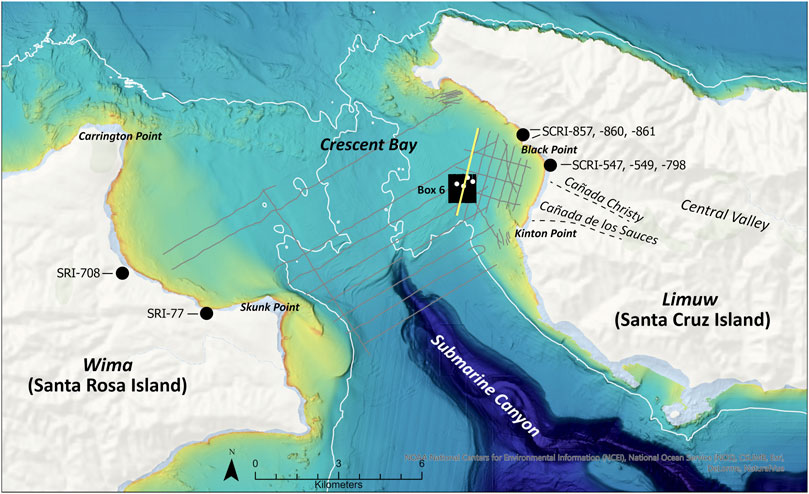
FIGURE 3. Santa Cruz Passage and Crescent Bay research area. Grey lines are transects from broad Chirp survey. Yellow line is the seismic line where the paleovalley was identified (shown in Figure 2). Black lines are the tight-grid spaced Chirp transect within the 1 km2 box 6. White dots are the core locations within Box 6. Black circles are general locations of archaeological sites mentioned in the text. Dashed black lines indicate locations of canyons mention in text. White line is the 40 m bathymetric contour, showing assumed extent of Santarosae at ∼9,000 years ago.
2.2.1 Coring
The sampling phase of the project was conducted aboard the R/V Sally Ride using a Rossfelder P-5 vibracore system. Core tubes were 4.5 m long and 10 cm in diameter and were vibrated into the sea floor until an impenetrable geologic unit was encountered. Twenty-six cores were collected from the submerged shelf around the NCI. Selection of targets for the coring regime focused on four core locations within each of the 1 km2 survey boxes. The locations of Box 6 cores were selected based on acoustic signals interpreted as high amplitude anomalies and/or laminations with a shallow buried transgressive surface (Table 1; Figure 4). These core locations were near to the larger paleovalley that appeared to contain shallow channel-like features and relatively thin marine sedimentation that could be penetrated by coring (Maloney et al., 2017). Intended targets in the core locations were preserved sediments indicative of a wetland environment that likely existed prior to inundation of this section of Sanatrosae, and consistent with the early Holocene-aged sites located on the adjacent terrestrial portion of the island. Water depths at Box 6 coring locations were similar, varying from 34 to 36 mbsl. Based on relative sea level data, sea levels 35 m lower than today occurred at ∼9,250 cal BP (Muhs et al., 2014).
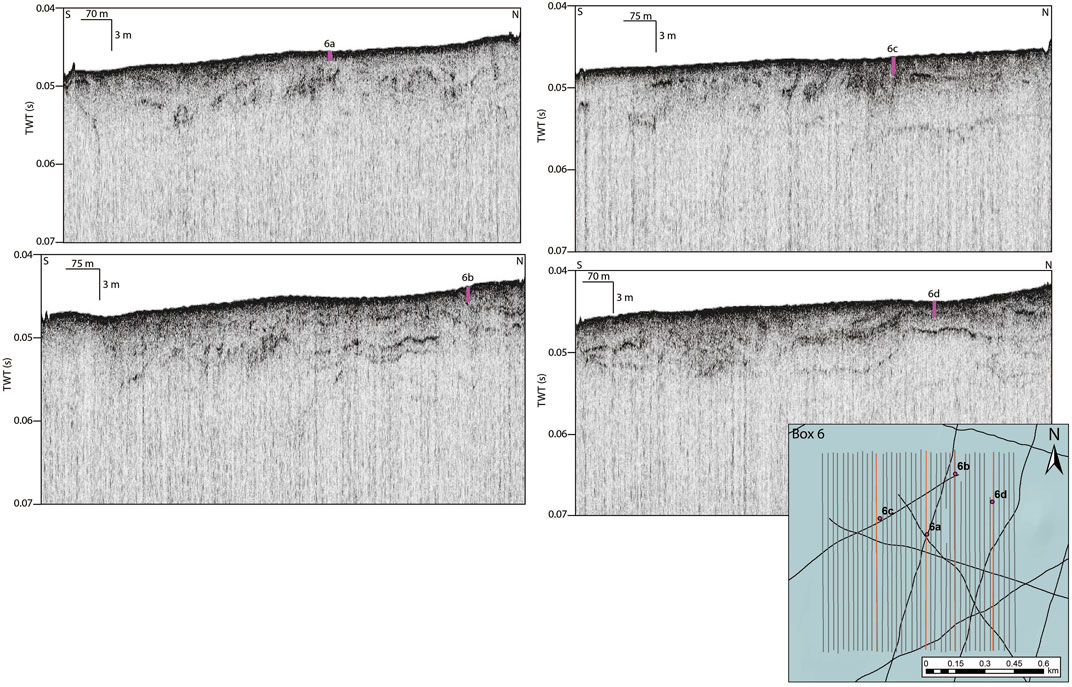
FIGURE 4. Chirp profiles within Box 6 showing locations of cores. Core locations and depth penetrated into the sea floor shown as pink bars overlain on the Chirp profiles. Inset box shows position of the cores within Box 6, and the corresponding Chirp profiles in orange. High-resolution Chirp data shown at 1 km scale to provide context for the depositional environment shown by the reflectors.
After collection, all cores were transported to Oregon State University (OSU) Marine and Geology Repository core facility for processing and analysis. Core analyses included whole core computerized tomography (CT) scans using the OSU College of Veterinary Medicine’s Toshiba Aquilion 64 Slice medical CT scanner. The CT scans were viewed in 3D using OsriX Lite software and images from the center of each core were captured and saved as png files. Scale bars were fit to the CT scan images using Adobe Illustrator and the recorded total length of the cores. Cores longer than 1.5 m were sectioned due to instrument constraints. Core section images were stitched together using scaling, image overlay, and color corrections in Adobe Illustrator to create whole core images.
Once split, a geological description of each core, including sediment character, shell content, color, and stratigraphic features was completed. Marine shell samples were collected from cores that had stratigraphy suggestive of a change in depositional environment (e.g., changes in sediment characteristics down the length of the core). Shells were identified to the genus or species level and pre-treated for dating at the University of Oregon, Museum of Natural and Cultural History. Pre-treatment included etching in a 10% HCl solution and rinsing with deionized water. Some samples were powdered with a drill, but smaller samples were kept whole. All samples were sent to DirectAMS Radiocarbon Dating Services in Bothell, WA for analysis (www.DirectAMS.com). Samples were prepared and analyzed by DirectAMS using their National Electrostatics Corporation 1.5 SDH Compact Pelletron Accelerator Mass Spectrometer. Results were reported by DirectAMS as uncalibrated radiocarbon ages, corrected for isotopic fractionation with an unreported δ13C value measured on the prepared carbon by the accelerator. The radiocarbon ages were calibrated using OxCal version 4.3 (Bronk Ramsey, 2009) and the Marine13 calibration curve (Reimer et al., 2013). All samples were also adjusted using a reservoir age of 261 ± 21 years (Ingram and Southon 1996).
3 Results
All four cores in Box 6 (6A, 6B, 6C, and 6D) penetrated past the transparent upper unit into the unit below with wavy, discontinuous reflectors (see Figure 4), at which point a stark change in sediment composition is observed from overlying gray sands with marine shells to distinct reddish, oxidized, angular sand, rocks, and mud that lack marine shells. In all four cores, just above the contact, the upper unit includes a high density of large shells, and subrounded-subangular pebbles. There were variable colors and dominant muddy grain size observed across all of the cores’ lower units that were interpreted to represent possible deposition within a floodplain, as the sinuosity of the area’s rivers changed with changing base levels.
All of the cores were capped with green marine sands or muds containing marine or estuarine molluscs, representing subtidal sands. Eight radiocarbon samples were collected from the Box 6 cores, all from the upper marine unit. The dates range from ∼9,900 to 5,800 cal BP (Table 2). The ages from near the base of cores 6A and 6C are consistent with the model of relative sea-level for the islands, but dates from the base of cores 6B and 6D are younger than expected for their given depths (Figure 5).
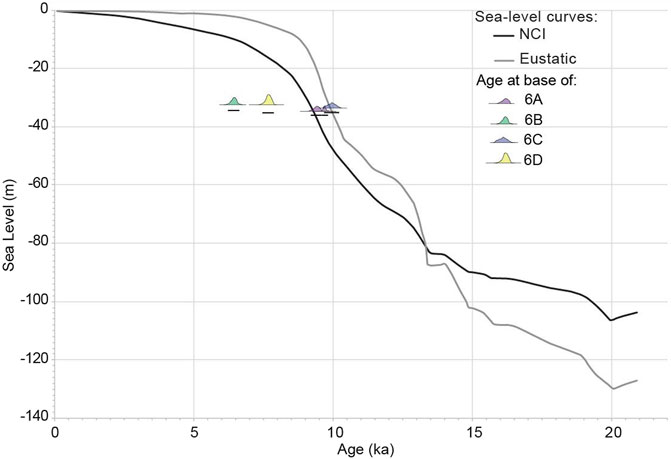
FIGURE 5. Dates closest to the base of the upper marine unit for each core plotted on a sea-level curve showing Age (ka) vs. Sea Level (m). NCI sea level curve based on Clark et al. (2014).
The lower portions of all cores include terrestrial sediments, with deposits in cores 6A, 6B, and 6D identified as sequences of preserved paleosols (described below). These paleosols were recognized primarily from carbonaceous root traces, tapering and branching downward, as well as rhizomes of sedges. Good examples of these features are noted in the paleosol images in Figures 8, 9. Paleosol intervals had distinctive soil structures, such as crumb peds and a sharp top under sedimentary cover, with organic A-horizons gradational downward to B-horizons with high chroma mottles. Munsell color was measured on each horizon and testing with dilute (0.1 M) HCl showed that all horizons were non-calcareous. The individual paleosols were compared with the closest modern soil series (Table 3) mapped on the Channel Islands (United States Department of Agriculture, Natural Resources Conservation Service, 2007). Cores from Box 6 were examined in the Marine Geology Repository of OSU. A graphic log was taken of the cores containing paleosols (Figure 6) and individual paleosols photographed.
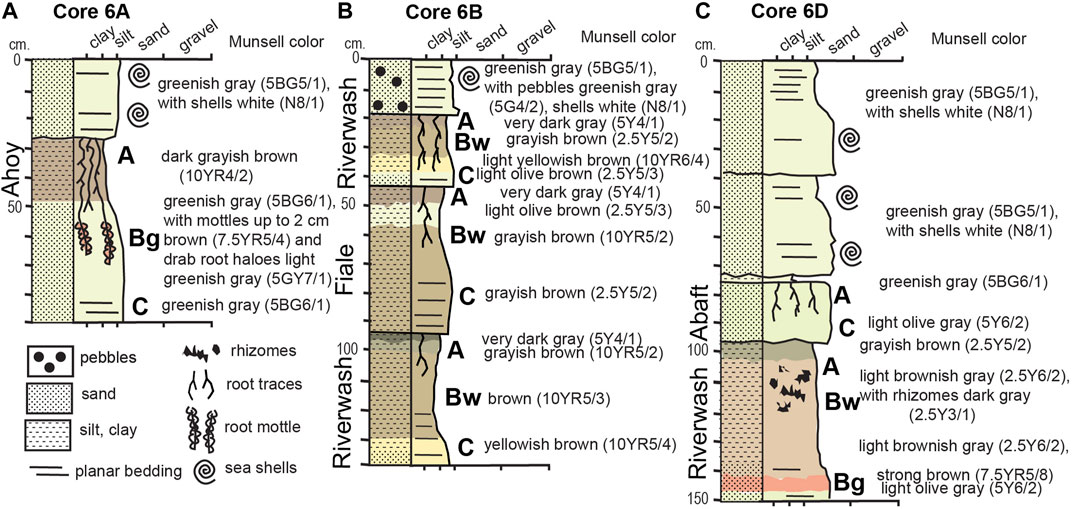
FIGURE 6. Graphic log of cores 6A, 6B, and 6D showing positon and type of paleosols in each core and the location of features such as root traces, root mottle, and rhizomes.
3.1 Core 6A
Core 6A was located at what was interpreted as the edge of a channel feature in Chirp data. The 27–28 cm of marine sediments capping a Xeroll (Ahoy) soil (Figure 7) produced single fragments of two discrete butter clam (Saxidomus spp.) shells at 11–15 cm below core surface that date to ∼9,600 cal BP. These appear to have been articulated clam shells in a thin and discrete lens, suggesting no reworking and a likely age for the underlying soil of >9,600 cal BP. The Xeroll soil has high organic content in the surface (A) horizon which also has distinctive crumb ped structure. Root trces and root mottle was apparent in the subsurface (Bg) horizon. This was a grassland soil comparable with the modern Ahoy Series of the Channel Islands (United States Department of Agriculture, Natural Resources Conservation Service, 2007).
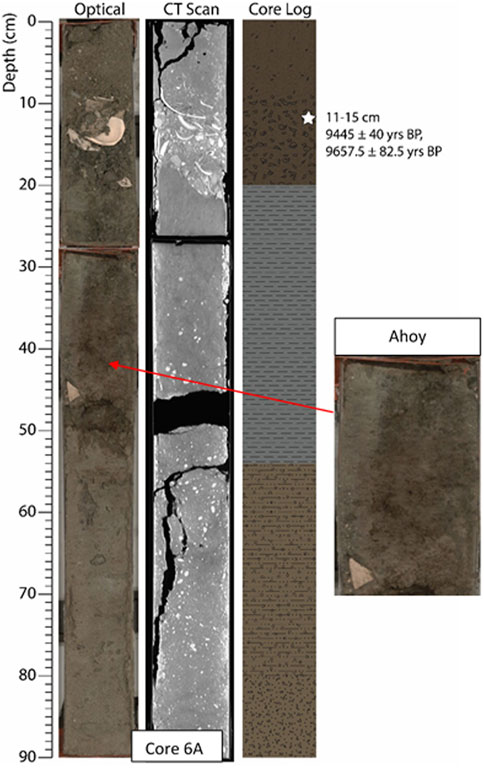
FIGURE 7. Optical, CT, and core log images of Core 6A with photograph inset of Ahoy (Xeroll) paleosols identified in core. White star on core log shows location of radiocarbon sample and depth and age are noted. Red arrow shows location of paleosol.
3.2 Core 6B
The location of core 6B was chosen to reach the acoustic unit with wavy, laminated reflectors. There is 18 cm of marine sediments overlying three stacked Ochrept (Fiale) and Fluvent (Riverwash) soils between 18 and 150 cm (Figure 8). Fluvent soils are more or less freely drained Entisols that form in recent water-deposited sediments on flood plains, fans, and deltas along rivers and small streams. They show little alteration of the parent sediment other than penetration by roots. Most Fluvents are frequently flooded, and stratification of the materials is normal. The Ochrept profiles have more organic matter, minor leaching in the surface (A) horizon, and clay enrichment in the subsurface (Bw) horizon that falls short of the amount needed for an argillic horizon. All three paleosols showed root traces in the surface (A) horizons continuing into the subsurface (Bw) horizons. A California cone (Conus californianus) shell fragment at 10–12 cm in marine sand above the paleosols dated to ∼6,450 cal BP. The Ochrept is a coastal shrubland soil comparable with the modern Fiale Series of the Channel Islands and the Fluvent is a coastal scrub and marsh soil comparable with the modern Riverwash Series of the Channel Islands (United States Department of Agriculture, Natural Resources Conservation Service, 2007).
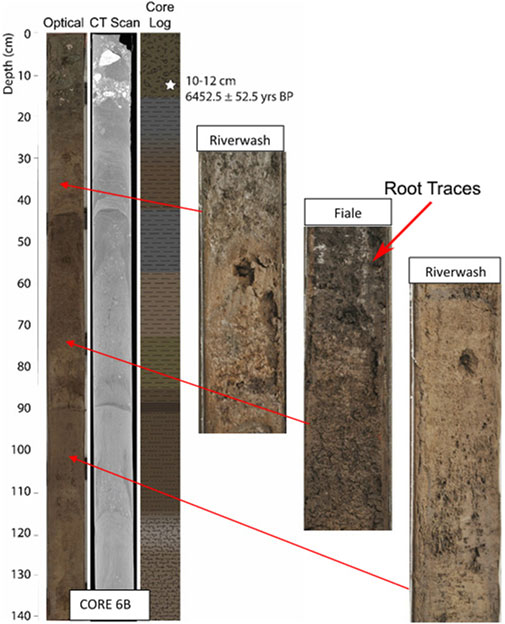
FIGURE 8. Optical, CT, and core log images of Core 6B with photograph insets of two Riverwash (Fluvent) and one Fiale (Xerept) paleosol. White star on core log shows location of radiocarbon sample and depth and age are noted. Red arrow shows location of paleosol. Distinct root traces are noted in the Fiale.
3.3 Core 6D
Core 6D was located where the wavy, laminated unit appeared uplifted toward the seafloor by faulting and folding. This allowed for sampling of deeper units that were unreachable in other locations. In the core, 72 cm of marine sediments are overlying two stacked Psamment (Abaft) and a Fluvent (Riverwash) soils between 72 and 150 cm (Figure 9). Three single fragments of Trachycardium, Crepidula, and Modiolus shell at 42–45 cm were AMS dated to ∼5,800, 6,050, and 7,650 cal BP, respectively. The Psamment profiles are eolian sand with root traces like the modern Abaft Series of the Channel Islands which form under dune binding grasses (United States Department of Agriculture, Natural Resources Conservation Service, 2007). In the lower Fluvent soil comparable with the modern Riverwash Series (United States Department of Agriculture, Natural Resources Conservation Service, 2007), dark tubular masses appear to be carbonaceous plant rhizomes (perhaps Tule reed).
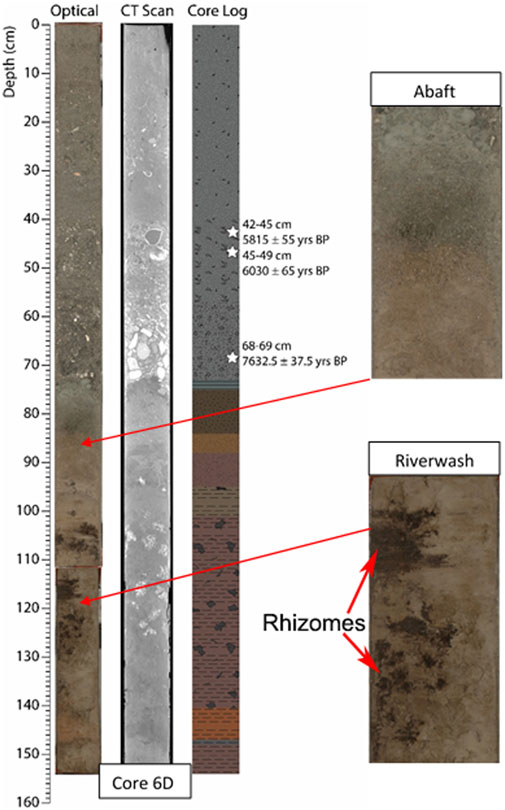
FIGURE 9. Optical, CT, and core log images of Core 6D with photograph insets of Riverwash (Fluvent) and Abaft (Psamment) paleosols. White star on core log shows location of radiocarbon sample and depth and age are noted. Red arrow shows location of paleosol. Rhizomes features are noted in the Riverwash.
4 Discussion
Crescent Bay has become a critical submerged landscape to consider for defining the paleolandscape and paleoecological evolution of Santarosae, and in the search for preserved cultural heritage on the sea floor. Together with the adjacent subaerial potion of western Santa Cruz Island between Black Point and Kinton Point (see Figure 3), there is an archaeologically sensitive landscape that shows terminal Pleistocene through Early Holocene archaeological evidence around what may have been a sizable floodplain with adjacent wetland habitats. Geologically this is a complex area located at the western end of the flat Central Valley where two major island drainages, Cañada de los Sauces and Cañada Christy, terminate at the ocean. The Santa Cruz Island Fault also runs just north of Cañada Christy, adjacent to Black Point. The canyons in this area are strongly controlled by changes in sea level, as this constitutes the local base level for the island’s river systems. When this base level lowers, the streams incise and erode upland areas, carrying sediment to the shoreline, creating a “lowstand delta” from the upland sediment (Schumann and Pigati 2017:522). With rising seas, streams aggrade and create sediment build up from the shoreline into the canyons. The effects of this sediment transfer during sea level changes alters coastal and shelf morphology, making identification of terminal Pleistocene land surfaces and habitats that have survived marine transgression difficult; however, accurate locations of preserved paleosols can aid in defining the spatial and diachronic evolution of terminal Pleistocene habitats that may have been foraging and habitation locales for Paleocoastal peoples. The locations of resources rich habitats, such as floodplains and wetlands, strongly influenced human settlement decisions and archaeological sites tend to cluster on landscapes with easy access to fresh water and wetland resources. These habitats are known from archaeological evidence to have existed on Santarosae (Erlandson et al., 2011; Erlandson et al., 2019; Rick et al., 2013), and research from the California mainland suggests that Paleocoastal habitation focused on rich wetland habitats, as well (Erlandson 1994; Byrd 1996; Reddy and Byrd 1997; York et al., 1999; Byrd and Raab 2007; Wahoff 2012; York 2012). Additionally, estuary deposits in particular are rich in organics and can provide data for understanding regional paleoecology (Watson et al., 2011). Estuaries contain rich biological resources that continually adapt to changes in environments and are ideal for helping interpret shifting climatic conditions (Cole and Liu 1994).
Due to their importance in paleoenvironmental modelling and data potential, the preserved paleosols identified as part of the current research are a significant step forward in the goal to understand and define the paleoenvironment of Santarosae and better understand where on the continental shelf cultural resources may be preserved. Although pollen analyses are needed on the paleosols, based on the closest modern soil series mapped on the Channel Islands (Table 3), the paleosols identified in cores 6A, 6B, and 6D represent a mosaic of shrubland, grassland, marsh, and dune-binding vegetation of a coastal plain. Cores 6B and 6D both had Riverwash (Fluvent) soils, which occur on flood plains, fans, and deltas along rivers and small streams. Although there were no peaty paleosols characteristic of permanently waterlogged wetlands, localized wetland patches are expected on a coastal plain. In fact, localized wetland patches are currently present at the mouths of several of Santa Cruz Island’s canyons, including Cañada de los Sauces, which is directly onshore from the coring site. The Riverwash paleosol in core 6D has stout carbonized rhizomes comparable with those of tule reed (Schoenoplectus californicus), which colonizes shallow lakes and river banks, suggesting that localized areas of wetland habitat were present prior to ∼7,600 years ago, the minimum age for the paleosols in 6D based on radiocarbon dating of the upper marine unit. Core 6D also has an Abaft (Psamment) paleosol that overlays the Riverwash. Abaft soil are found in dune fields with a typical vegetation of beach and dune plant communities. On the Northern Channel Islands, Abaft soils are common on the stabilized dunes on the eastern shore of Santa Rosa Island, directly across the passage from Santa Cruz Island and on the western edge of Crescent Bay. Core 6A contains an Ahoy (Xeroll) paleosol, which forms on stable marine terraces and supports native and nonnative grasses. These coastal terrace landforms with grassland vegetation are prominent features on Santa Rosa and Santa Cruz islands (Schumann et al., 2014), and can be seen directly onshore from the coring site on the western sector of Santa Cruz Island. The fourth identified palesol, Fiale (Xerept), is an Inceptisol common on side slopes of hills and interfluves. This soil is common on the eroded slopes of the river cut terraces on the western sector of Santa Cruz Island. One Fiale series paleosol is between two Riverwash paleosols in Core 6B and additional analysis is needed to determine the temporal relationship between these three units. The Abaft, Ahoy, and Fiale paleosols had high chroma mottles indicating a seasonally high water table, but were well drained for most of the year.
As the base of the upper marine units in Cores 6A, 6B, and 6D represent the initial transgression and rise of sea level, the underlying surface is the wave transgressive surface, which was subaerially exposed prior to flooding (Laws et al., 2019). Therefore, although only the bases of the upper marine units were dated, we can determine minimum ages for the underlying paleosol sequences and infer the paleogeographic processes that allowed the soils to form. The base of the marine unit in Core 6A is dated ∼9,600 cal BP. Below this unit is the undated Ahoy Series, suggesting a subaerial coastal plain was present prior to 9,600 cal BP. This older date for a subaerial terrace landform is supported by models of the Santa Cruz Passage beginning to form around 9,000–10,000 years ago, as sea level rise began separating Santa Cruz and Santa Rosa islands (Clark et al., 2014; Reeder-Myers et al., 2015). The Riverwash Series with possible tule reed rhizomes and the Abaft Series in Core 6D developed prior to 7,650 cal BP. These soils that form along waterways and among dunes, respectively, show the environmental shift in this section of Santarosae as seas continued to rise, creating rapidly changing shallow water environments and drowning river mouths, encouraging evolution of estuary systems (Graham et al., 2003). This paleogeographic process has been identified on the western section of the Passage, where a small paleoestuary on eastern Santa Rosa Island (Cole and Liu 1994; Anderson et al., 2010) attracted people throughout much of the terminal Pleistocene and early Holocene (Rick et al., 2005b). Coastal dune fields are also present on eastern Santa Rosa Island, most extensively at Skunk and Carrington points (see Figure 3). These dune fields formed during glacial periods when seas were lower, exposing the large submarine shelf, allowing the prevailing northwest wind to deflated carbonate sand from the shelf, and blow them on the land surface (Schumann et al., 2014). While the dune fields on Santa Rosa Island stabilized when sea level rose and inundated the shelf (Muhs et al., 2009), dunes did form in other localized areas across the now submerged shelf (Gusick et al., 2019: 152–153). The Abaft Series paleosol identified in Core 6D provides an indication of a localized area where dunes formed on the eastern section of Crescent Bay. Lastly, Core 6B also contains two Riverwash paleosols that formed earlier than 6,450 cal BP, and provides additional data with which to define spatial and diachronic evolution of localized wetland patches within Crescent Bay as marine transgression continued to flood the lowland terrace.
While gently sloping nearshore shelves, such as those within the Santa Cruz Passage, may have encouraged development of estuarine and marsh ecosystems at the mouths of larger streams, their full development and productivity may have been halted by rapid changes in sea level (Reeder-Myers et al., 2015). Although more analyses are needed on the paleosols to understand the development of coastal and intertidal ecosystems along the margins of Crescent Bay, the baseline paleosol data indicating there were areas of localized dune and wetland patches can be considered with Paleocoastal habitation data known from the subaerial portion of the island. This combination can help determine if the shifting wetland habitats suggested by the preserved paleosols were present during habitation and a target for subsistence strategies during the Paleocoastal period.
Archaeological and paleoecological data show that an estuary existed on eastern Santa Rosa Island between 8,500 and 5,000 years ago (Anderson et al., 2010; Rick et al., 2005a), but Venus clam (Chione undatella) shells dating to ∼11,000 and more than 600 birdbone fragments from waterfowl (geese/ducks) recovered from SRI-708 show that another, earlier estuary existed likely near to this site on the western margin of Crescent Bay (Erlandson et al., 2019). Associated with these faunal remains were chipped-stone crescents, transverse projectile point technology used for hunting birds and found primarily in sites dated to 11,000 cal BP and older, commonly adjacent to wetland environments (Erlandson et al., 2011; Rick et al., 2013; Sanchez et al., 2017). Another nearby site, SRI-77, also produced a Chione undatella shell dating to ∼7,450 cal BP (Rick 2020), suggesting that estuary deposits were forming and sustaining resources on this western section of Crescent Bay during the postglacial marine transgression that was flooding the lowland terrace connecting Santa Cruz and Santa Rosa islands.
Across Crescent Bay on western Santa Cruz Island, and near to the core locations, there are two Paleocoastal site clusters (see Figure 3). On a high southwest-facing bluff at the mouth of Black Point Canyon and on the bluff across Black Point Canyon lie two lithic scatter sites (CA-SCRI-860 and CA-SCRI-861) that have produced chipped stone crescents, similar to SRI-708, that are suggestive of a terminal Pleistocene or earliest Holocene occupation and bird hunting (Erlandson et al., 2016; Sanchez et al., 2017). The position of CA-SCRI-860 and CA-SCRI-861 with hunting technology high above Crescent Bay suggests they may have been “look out” sites (Rick et al., 2013), possibly for tracking waterfowl in wetlands or pinnipeds hauled out on sandy beaches. Near to these lithic sites is one recently identified terminal Pleistocene site (CA-SCRI-857) dated to ∼11,400 cal BP from which an estuarine calm was recovered (J. Erlandson, personal communication, 8 May 2022) suggesting an estuarine habitat in the region. Further south in the curve between Black Point and Kinton Point is a cluster of sites dating to the Early Holocene (CA-SCRI-547, CA-SCRI-549, and CA-SCRI-798) located above what is now Christy Beach. A total of five radiocarbon dates derived from well-preserved California Mussel (Mytilus californianus) shells provide a date range of 8,660–8,015 cal BP for the three sites. All are single thin shell lenses located down an erosional face of a coastal grassland terrace, and buried under 6–8 m of alluvium. In contrast to the terminal Pleistocene Black Point sites, these Early Holocene sites are mainly comprised of California Mussel and Black Abalone (Haliotis cracherodii) shells with a small amount of Pismo clam (Tivela stultorum) and Littleneck clam (Protothaca staminea), sandy shore species that are not widespread on the islands (Gusick, 2012; Gusick, 2013). The 3,000-years timespan and the varying technology and mollusks present in both clusters of Paleocoastal sites, likely reflect a rapidly evolving littoral ecosystem impacted by rising seas.
The dates and constituents of these sites support the core data that indicate wetland ecosystems were present across Crescent Bay. The ages of the known onshore sites and the cores show the wetland habitats within Crescent Bay were a target for exploitation from the terminal Pleistocene through the Early Holocene. The terminal Pleistocene sites on the western edge of Crescent Bay (Santa Rosa Island) have yielded estuarine shell as well as technology and faunal material indicating wetland bird hunting. Considered together, the terminal Pleistocene-aged Black Point sites on the eastern edge of Crescent Bay (Santa Cruz Island)—and closest to the core locations—also contained estuarine shell and the same bird-hunting technology as the contemporaneous sites across the bay. Though not on the margins of Crescent Bay, another terminal Pleistocene-aged site, CA-SRI-512, on the north coast of Santa Rosa Island produced crescents and hundreds of waterfowl bones indicating estuary and marsh exploitation during this same terminal-Pleistocene time period (Erlandson et al., 2011; Erlandson et al., 2019). The wide spread consistency of site constituents during this early habitation period suggests that wetland habitats were likely present in various areas around Santarosae and a draw for Paleocoastal peoples during the terminal Pleistocene on the island. The early Holocene-aged (∼8,800 cal BP) sites on Santa Cruz Island yielded sandy beach species and no crescents, perhaps indicating a shift in availability or productivity of local wetland habitats as seas continued to rise and flood lowland areas. The search for terminal Pleistocene and early Holocene-aged sites on the subaerial portion of Santa Cruz Island has not been as extensive as on Santa Rosa Island and there may be more Paleocoastal-aged sites on this eastern edge of Crescent Bay that have not yet been identified.
5 Conclusion
While additional research and analyses are needed, the discovery of intact terrestrial soils preserved beneath the transgressive marine sediments on the current floor of the Santa Cruz Passage provides a “proof of concept” that: 1) terrestrial soils survived the effects of rising seas and active transgression that included strong submarine currents and an often turbulent Northeast Pacific surf zone; and 2) the south-facing Crescent Bay area that was a focus of settlement by Paleocoastal peoples and contains preserved terrestrial soils, likely contains intact submerged terrestrial sites of Early Holocene and terminal Pleistocene age. This is an important step in the search for submerged cultural material on the maritime cultural landscape of Santarosae. By focusing on features that were attractive to early maritime hunter-gatherers, such as wetland environments, we can narrow search parameters to identify archaeological “hot spots” on the continental shelf. These data are crucial in our ability to identify sensitive maritime cultural landscapes and eventually identify, document, and preserve underwater cultural heritage resources. Our current research has increased our understanding of the long-term ecology of Santarosae, of which little is known (Anderson et al., 2010), and expanded our knowledge of the paleolandscape in relation to the terminal Pleistocene peopling of the Americas. The seismic and core data have also contributed to the broad scale mapping of the Northern Pacific continental shelf and definition of regional archaeological and biologically sensitive landscapes (Braje et al., 2016; Braje et al., 2021). Collectively, these efforts help to understand how Pleistocene landscapes in the SCB evolved during rising seas, tectonic activity, and the regional shift to a drier climate that occurred during the Pleistocene-Holocene transition. This evolution has implications not only for early human habitation of the area, but also for addressing key questions in the ecological, biological, and geological disciplines.
Data availability statement
The original contributions presented in the study are included in the article/supplementary material, further inquiries can be directed to the corresponding author/s.
Author contributions
AG participated in all data collection and analysis and wrote the manuscript. JM participated in sonar and core data collection and analysis and manuscript writing. TB participated in all data analysis and manuscript writing. GR analyzed core data and contributed to manuscript writing. LJ participated in sonar and core data collection and analysis and manuscript writing. SK participated in sonar and core data collection, processing and analysis, and manuscript writing. AA participated in archaeological site data collection, analyzed faunal material, prepped samples, and contributed to manuscript writing. JME participated in archaeological site data collection, analysis of all data, and contributed to manuscript writing. All authors contributed to manuscript revisions, and read and approved the submitted version.
Funding
This work was supported by a Bureau of Land Management Cooperative Agreement Awarded (No. M15AC00012) to San Diego State University.
Acknowledgments
We would like to express our gratitude to our home institutors and the Bureau of Ocean Energy Management for supporting this research. We would like to recognize Neal Driscoll at Scripps Institution of Oceanography and the crews of the R/V Shearwater and R/V Sally Ride who were critical in helping us collect geophysical and core data. We also thank the Oregon State University (OSU) Marine and Geology Repository staff for assistance curating the cores. Thank you to the editors for inviting us to participate in this special issue, along with reviewers and editorial and production teams at Frontier in Earth Science for their constructive feedback and help with the publication of our manuscript.
Conflict of interest
The authors declare that the research was conducted in the absence of any commercial or financial relationships that could be construed as a potential conflict of interest.
Publisher’s note
All claims expressed in this article are solely those of the authors and do not necessarily represent those of their affiliated organizations, or those of the publisher, the editors and the reviewers. Any product that may be evaluated in this article, or claim that may be made by its manufacturer, is not guaranteed or endorsed by the publisher.
References
Anderson, R. S., Starratt, S., Jass, R. M. B., and Pinter, N. (2010). Fire and vegetation history on Santa Rosa Island, Channel Islands, and long‐term environmental change in southern California. J. Quat. Sci. 25 (5), 782–797. doi:10.1002/jqs.1358
Atwater, B. F. (1987). Evidence for great Holocene earthquakes along the outer coast of Washington State. Science 236 (4804), 942–944. doi:10.1126/science.236.4804.942
Boëda, E., Bonilauri, S., Connan, J., Jarvie, D., Mercier, N., Tobey, M., et al. (2008). Middle palaeolithic bitumen use at umm el Tlel around 70,000 BP. Antiquity 82, 853e861. doi:10.1017/s0003598x00097623
Braje, T. J., Erlandson, J. M., and Timbrook, J. (2005). An asphaltum coiled basket impression, tarring pebbles, and middle Holocene water bottles from san miguel island, California. J. Calif. Gt. Basin Anthropol. 25, 207–213. doi:10.2307/27825804
Braje, T. J., and Erlandson, J. M. (2008). Early maritime technology from western San Miguel Island, California. Current Research in the Pleistocene 25, 61–63
Braje, T. J., Erlandson, J. M., and Rick, T. C. (2013). “Points in space and time: The distribution of Paleocoastal points and crescents on California’s Northern Channel Islands” in California’s Channel Islands: The Archaeology of Human-Environment Interactions. Editors C. S. Jazwa, and J. E. Perry (Salt Lake City: University of Utah Press), 72–106.
Braje, T. J., Maloney, J., Ball, D., Davis, L., Driscoll, N., Dugan, J., et al. (2016). “Mapping the submerged landscapes of southern California and Oregon: Archaeological, biological, and geological implications,” in Paper Presented at the 8th California Islands Symposium. Oxnard.
Braje, T. J., Maloney, J. M., Gusick, A. E., Erlandson, J. M., Nyers, A., and Davis, L. (2019). Working from the known to the unknown: Linking the Subaerial Archaeology and the Submerged landscapes of Santarosae Island, Alta California, USA. Open Quaternary 5 (1), 10. doi:10.5334/oq.66
Braje, T. J., Erlandson, J. M., Rick, T. C., Davis, L., Dillehay, T., Fedje, D. W., et al. (2020). Fladmark + 40: What have we learned about a potential pacific coast peopling of the Americas? Am. Antiq. 85 (1), 1–21. doi:10.1017/aaq.2019.80
Braje, T. J., Maloney, J., Davis, L., Driscoll, N., Dugan, J., Erlandson, J. M., et al. (2021). Archaeological and biological assessment of submerged landforms off the pacific coast of California and Oregon, USA. Report on file at the BOEM pacific OCS region.
Braje, T. J., Maloney, J., Gusick, A. E., Erlandson, S., and Klotsko, J. M. (2022). Re-evaluating terminal Pleistocene and Early Holocene settlement patterns with Chirp subbottom data from around California's Northern Channel Islands. World Archaeol., 1–15. Press. doi:10.1080/00438243.2022.2077825
Brown, K. M. (2016). Asphaltum (bitumen) production in everyday life on the California Channel Islands. J. Anthropol. Archaeol. 41, 74–87. doi:10.1016/j.jaa.2015.11.003
Byrd, B. F. (1996). Coastal archaeology of las flores creek and horno canyon, Camp Pendleton, California. San Diego: San Diego State University. Unpublished report on file at the South Coastal Information Center.
Byrd, B. F., and Raab, L. M. (2007). “Recent advances in coastal archaeology along the southern California Bight,” in California prehistory: Colonization, culture, and complexity. Editors T. L. Jones, and K. A. Klar (Walnut Creek: Altamira Press), 215–228.
Chaytor, J. D., Goldfinger, C., Meiner, M. A., Huftile, G. J., Romsos, C. G., and Legg, M. R. (2008). Measuring vertical tectonic motion at the intersection of the Santa cruz–catalina ridge and Northern Channel islands platform, California continental borderland, using submerged paleoshorelines. Geol. Soc. Am. Bull. 120 (7-8), 1053–1071. doi:10.1130/b26316.1
Clark, J., Mitrovica, J. X., and Alder, J. (2014). Coastal paleogeography of the California-Oregon-Washington and bering sea continental shelves during the latest Pleistocene and Holocene: Implications for the archaeological record. J. Archaeol. Sci. 52, 12–23. doi:10.1016/j.jas.2014.07.030
Cohen, J. K., and Stockwell Jr., J. W. (1999). CWP/SU: Seismic unix release 33: A free package for Seismic research and processing. Center for Wave Phenomena, Colorado School of Mines.
Cole, K. L., and Liu, G. W. (1994). Holocene paleoecology of an estuary on Santa Rosa island, California. Quat. Res. 41 (3), 326–335. doi:10.1006/qres.1994.1037
Connan, J. (1999). Use and trade of bitumen in antiquity and prehistory: Molecular archaeology reveals secrets of past civilizations. Phil. Trans. R. Soc. Lond. B 354 (1379), 33–50. doi:10.1098/rstb.1999.0358
Connolly, T. J., Erlandson, J. M., and Norris, S. E. (1995). Early Holocene basketry and cordage from daisy cave san miguel island, California. Am. Antiq. 60 (2), 309–318. doi:10.2307/282142
Cortés-Sánchez, M., Morales-Muñiz, A., Simón-Vallejo, M. D., Lozano-Francisco, M. C., Vera-Peláez, J. L., Finlayson, C., et al. (2011). Earliest known use of marine resources by Neanderthals. PloS one 6 (9), e24026. doi:10.1371/journal.pone.0024026
Dickinson, W. R. (2001). Paleoshoreline record of relative Holocene sea levels on Pacific Islands. Earth-Science Rev. 55, 191–234. doi:10.1016/s0012-8252(01)00063-0
Dillehay, T. D., Ramírez, C., Pino, M., Collins, M. B., Rossen, J., and Pino-Navarro, J. D. (2008). Monte verde: Seaweed, food, medicine, and the peopling of South America. Science 320 (5877), 784–786. doi:10.1126/science.1156533
Dillehay, T. D., Bonavia, D., Goodbred, S. L., Pino, M., Vásquez, V., and Tham, T. R. (2012). A late Pleistocene human presence at Huaca Prieta, Peru, and early Pacific Coastal adaptations. Quat. Res. 77 (3), 418–423. doi:10.1016/j.yqres.2012.02.003
Dobson, J. E. (2014). Aquaterra incognita: Lost land beneath the sea. Geogr. Rev. 104 (2), 123–138. doi:10.1111/j.1931-0846.2014.12013.x
Emery, K. O. (1958). Shallow submerged marine terraces of southern California. Geol. Soc. Am. Bull. 69, 39–60. doi:10.1130/0016-7606(1958)69[39:ssmtos]2.0.co;2
Erlandson, J. (1994). Early hunter-gatherers of the California coast. Springer Science & Business Media.
Erlandson, J., Kennett, D., Ingram, B., Guthrie, D., Morris, D., Tveskov, M., et al. (1996). An archaeological and paleontological chronology for Daisy Cave (CA-SMI-261), San Miguel Island, California. Radiocarbon 38 (2), 355–373. doi:10.1017/S0033822200017689
Erlandson, J. M. (2001). The archaeology of aquatic adaptations: Paradigms for a new millennium. J. Archaeol. Res. 9, 287–350. doi:10.1023/a:1013062712695
Erlandson, J. M., Graham, M. H., Bourque, B. J., Corbett, D., Estes, J. A., and Steneck, R. S. (2007). The kelp highway hypothesis: Marine ecology, the coastal migration theory, and the peopling of the Americas. J. Island Coastal Archaeol. 2, 161–174. doi:10.1080/15564890701628612
Erlandson, J. M., Moss, M. L., and Des Lauriers, M. (2008). Life on the edge: early maritime cultures of the Pacific Coast of North America. Quaternary Sci. Rev. 27 (23–24), 2232–2245. doi:10.1016/j.quascirev.2008.08.014
Erlandson, J. M., Rick, T. C., Braje, T. J., Casperson, M., Culleton, B., Fulfrost, B., et al. (2011). Paleoindian seafaring, maritime technologies, and coastal foraging on California’s Channel Islands. Science 331 (6021), 1181–1185. doi:10.1126/science.1201477
Erlandson, J. M. (2021). “Conclusion:,” in The archaeology of island colonization: Global approaches to initial human settlement. Editors M. F. Napolitano, J. H. Stone, and R. J. DiNapoli (Gainesville: University Press of Florida), 352–360. doi:10.2307/j.ctv1m9x2s3.18
Erlandson, J. M., and Braje, T. J. (2015). Coasting out of Africa: The potential of mangrove forests and marine habitats to facilitate human coastal expansion via the Southern Dispersal Route. Quat. Int. 382, 31–41. doi:10.1016/j.quaint.2015.03.046
Erlandson, J. M., Gill, K. M., Glassow, M. A., and Gusick, A. E. (2016). Three paleocoastal lithic sites on Santa Cruz island, California. PaleoAmerica 2 (1), 52–55. doi:10.1080/20555563.2016.1141741
Erlandson, J., Rick, T., Ainis, A., Braje, T., Gill, K., and Reeder-Myers, L. (2019). Late Pleistocene estuaries, palaeoecology and humans on north America's pacific coast. Antiquity 93 (372). doi:10.15184/aqy.2019.185
Esler, K. J., Jacobsen, A. L., and Pratt, R. B. (2018). The biology of Mediterranean-type ecosystems. Oxford University Press.
Fauvelle, M. (2014). Acorns, asphaltum, and asymmetrical exchange: Invisible exports and the political economy of the Santa Barbara channel. Am. Antiq. 79 (3), 573–575. doi:10.7183/0002-7316.79.3.573
Ben Ford (Editor) (2011). The archaeology of maritime landscapes (Vol. 2). Springer Science Business Media.
Gamble, L. H. (2008). The Chumash world at European contact: Power, trade, and feasting among complex hunter-gatherers. Santa Barbara: Univ of California Press.
Gill, K. M., Fauvelle, M., and Erlandson, J. M. (2019). An archaeology of abundance: Reevaluating the marginality of California's islands. Gainsville, FL: University Press of Florida.
Gill, K. M., Braje, T. J., Smith, K., and Erlandson, J. M. (2021). Earliest evidence for geophyte use in North America: 11,500-year-old archaeobotanical remains from California’s Santarosae island. Am. Antiq. 86 (3), 625–637. doi:10.1017/aaq.2021.31
Graham, M. H., Dayton, P. K., and Erlandson, J. M. (2003). Ice ages and ecological transitions on temperate coasts. Trends Ecol. Evol. 18 (1), 33–40. doi:10.1016/s0169-5347(02)00006-x
Graham, M. H., Kinlan, B. P., and Grosberg, R. K. (2009). Post-glacial redistribution and shifts in productivity of giant kelp forests. Proc. R. Soc. B 277 (1680), 399–406. doi:10.1098/rspb.2009.1664
Gusick, A. E. (2012). Behavioral adaptations and mobility of early Holocene hunter-gatherers, Santa Cruz island, California. Santa Barbara: Dissertation on file. University of California.
Gusick, A. E. (2013). “Early maritime hunter-gatherer occupation, Santa Cruz island,” in Small islands, Big implications: The California Channel Islands and their archaeological contribution. Editors J. Perry, and C. Jazwa (Salt Lake City: University of Utah Press), 40–59.
Gusick, A. E., and Erlandson, J. M. (2019). “Paleocoastal landscapes, marginality, and early human settlement of the California islands,” in An archaeology of abundance: Re-Evaluating the marginality of California's islands. Editors K. Gill, J. Erlandson, and M. Fauvelle (Gainesville: University of Florida Press), 59–97. doi:10.5744/florida/9780813056166.003.0003
Gusick, A. E., Dodds, T., Jaffke, D., Meniketti, M., and Ball, D. (2019). Defining maritime cultural landscapes in California. Calif. Archaeol. 11 (2), 139–164. doi:10.1080/1947461x.2019.1659720
Gusick, A. E., Braje, J., Erlandson, T., Maloney, J. M., and Ball, D. (2021). “Above and below the waves:,” in The archaeology of island colonization: Global approaches to initial human settlement. Editors M. Napolitano, J. Stone, and B. Dinapoli (Gainesville: University Press of Florida), 105–131. doi:10.2307/j.ctv1m9x2s3.10
Haaker, E. C., Rockwell, T. K., Kennedy, G. L., Ludwig, L. G., Freeman, S. T., Zumbro, J. A., et al. (2016). Style and rate of long-term uplift of the southern California coast between San Diego and Newport Beach with potential implications for assessing blind thrust models. Appl. Geol. Calif. 26, 679–719.
Henderson, J. (2019). Oceans without history? Marine cultural heritage and the sustainable development agenda. Sustainability 11 (18), 5080. doi:10.3390/su11185080
Henkart, P. (2003). SIOSEIS software. La Jolla, CA: Scripps Institution of Oceanography. Available at: http://sioseis.ucsd.edu
Hodgson, S. F. (2004). California Indians, Aartisans of oil (No. 53). Sacramento, CA: California Department of Conservation Division of Oil Gas and Geo.
Ingram, B. L., and Southon, J. R. (1996). Reservoir ages in eastern Pacific coastal and estuarine waters. Radiocarbon 38, 573–582. doi:10.1017/s0033822200030101
Johnson, J. R., Stafford, T. W., Ajie, H. O., and Morris, D. P. (2002). “Arlington springs revisited,” in Proceedings of the fifth California Islands symposium. Editors D. Brown, K. Mitchell, and H. Chaney (Santa Barbara (CA): Santa Barbara Museum of Natural History), 541–545.
Johnson, L. (2020). Paleolandform reconstruction offshore of the Channel Islands using sediment cores and high-resolution seismic data. MA Thesis. San Diego, CA: San Diego State University, Department of Geology.
Joordens, J. C., d’Errico, F., Wesselingh, F. P., Munro, S., de Vos, J., Wallinga, J., et al. (2015). Homo erectus at Trinil on Java used shells for tool production and engraving. Nature 518 (7538), 228–231. doi:10.1038/nature13962
Kelsey, H. M., and Bockheim, J. G. (1994). Coastal landscape evolution as a function of eustasy and surface uplift rate, Cascadia margin, southern Oregon. Geol. Soc. Am. Bull. 106, 840–854. doi:10.1130/0016-7606(1994)106<0840:cleaaf>2.3.co;2
Kern, P., and Rockwell, T. K. (1992). “Chronology and deformation of quaternary marine shorelines, san Diego county, California,” in Quaternary coasts of the United States: Marine and lacustrine systems (Society of Economic Paleotologists and Mineralogists), 377–382. doi:10.2110/pec.92.48.0377
Kinlan, B. P., Graham, M. H., Erlandson, J. M., Garcelon, D. K., and Schwemm, C. A. (2005). “Late quaternary changes in the size and shape of the California Channel Islands: Implications for marine subsidies to terrestrial communities,” in Proceeding of the sixth California islands symposium, ventura, California, december 1-3, 2003. Editors D. K. Garcelon, and C. A. Schwemm (Arcata: Institute for Wildlife Studies), 131–142.
Klotsko, S., Driscoll, N., Kent, G., and Brothers, D. (2015). Continental shelf morphology and stratigraphy offshore San Onofre, California: The interplay between rates of eustatic change and sediment supply. Mar. Geol. 369, 116–126. doi:10.1016/j.margeo.2015.08.003
Lambeck, K., Yokoyama, Y., and Purcell, T. (2002). Into and out of the last glacial Maximum: sea-level change during oxygen isotope stages 3 and 2. Quat. Sci. Rev. 21, 343–360. doi:10.1016/s0277-3791(01)00071-3
Laws, A., Maloney, J., Klotsko, S., Gusick, A., Braje, T., and Ball, D. (2019). Submerged paleoshoreline mapping using high-resolution CHIRP sub-bottom data, Northern Channel Islands platform, California, USA. Quat. Res. 93, 1–22. doi:10.1017/qua.2019.34
Maloney, J. M., Klotsko, S. A., Gusick, A. E., Braje, T. J., Ball, D., Davis, L., et al. (2017). Paleodrainage morphology during glacial-interglacial cycles in the Northern Channel islands, California. New Orleans, LA: AGU Fall Meeting.
Marean, C. W., Bar-Matthews, M., Bernatchez, J., Fisher, E., Goldberg, P., Herries, A. I. R., et al. (2007). Early human use of marine resources and pigments in South Africa during the Middle Pleistocene. Nature 449, 905–908. doi:10.1038/nature06204
Masters, P. M., and Aiello, I. (2007). “Postglacial evolution of coastal environments,” in California prehistory: Colonization, culture, and complexity. Editors T. L. Jones, and K. A. Klar (New York: Altamira Press), 35–51.
McCawley, W. (1996). The first angelinos. Banning and novato. Banning, CA: Malki Museum Press/Ballena Press Cooperative Publication.
Muhs, D. R., Skipp, G. A. R. Y., Schumann, R. R., Johnson, D. L., McGeehin, J. P., Beann, J., et al. (2009). “The origin and paleoclimatic significance of carbonate sand dunes deposited on the California Channel Islands during the last glacial period,” in Proceedings of the 7th California Islands Symposium, Arcata, CA (Arcata, CA: Institute for Wildlife Studies), 3–14.
Muhs, D. R., Simmons, K. R., Schumann, R. R., Groves, L. T., Mitrovica, J. X., and Laurel, D. (2012). Sea level history during the last interglacial complex on San Nicolas Island, California: Implications for glacial isostatic adjustment processes, paleozoogeography and tectonics. Quat. Sci. Rev. 37, 1–25. doi:10.1016/j.quascirev.2012.01.010
Muhs, D. R., Simmons, K. R., Schumann, R. R., Groves, L. T., DeVogel, S. B., Minor, S. A., et al. (2014). Coastal tectonics on the eastern margin of the pacific rim: Late quaternary sea-level history and uplift rates, Channel Islands national park, California, USA. Quat. Sci. Rev. 105, 209–238. doi:10.1016/j.quascirev.2014.09.017
O’Connor, S., Ono, R., and Clarkson, C. (2011). Pelagic fishing at 42,000 years before the present and the maritime skills of modern humans. Science 334, 1117–1121. doi:10.1126/science.1207703
Oron, A., Galili, E., Hadas, G., and Klein, M. (2015). Early maritime activity on the dead sea: Bitumen harvesting and the possible use of reed watercraft. J. Mari Arch. 10 (1), 65–88. doi:10.1007/s11457-015-9135-2
Orr, P. C. (1962). The Arlington spring site, Santa Rosa island, California. Am. Antiq. 27 (3), 417–419. doi:10.2307/277806
Orr, P. C. (1968). Prehistory of Santa Rosa island. Santa Barbara (CA): Santa Barbara Museum of Natural History.
Pinter, N., Sorlien, C. C., and Scott, A. T. (2003). Fault-related fold growth and isostatic subsidence, California Channel Islands. Am. J. Sci. 303 (4), 300–318. doi:10.2475/ajs.303.4.300
Reddy, S. N., and Byrd, B. F. (1997). A Window to the past: Designing archaeological study on Camp Pendleton, California. San Diego: Report on file at South Coastal Information Center.
Reeder-Myers, L., Erlandson, J. M., Muhs, D. R., and Rick, T. C. (2015). sea level, paleogeography, and archeology on California's Northern Channel islands. Quat. Res. 83 (2), 263–272. doi:10.1016/j.yqres.2015.01.002
Reimer, P. J., Bard, E., Bayliss, A., Beck, J. W., Blackwell, P. G., Bronk Ramsey, C., et al. (2013). IntCal13 and Marine13 radiocarbon age calibration curves 0–50,000 years cal BP. Radiocarbon 55 (4).
Rick, T. C. (2008). An arena point and crescent from Santa Rosa Island, California. Curr. Res. Pleistocene 25, 140–142.
Rick, T. C. (2009). “8000 years of human settlement and land use in old ranch canyon, Santa Rosa island, California,” in Proceedings of the seventh California islands symposium (Arcata, CA: Institute for Wildlife Studies), 21–31.
Rick, T. C. (2020). Early to Middle Holocene estuarine shellfish collecting on the islands and mainland coast of the Santa Barbara Channel, California, USA. Open Quat. 6 (1), 9. doi:10.5334/oq.86
Rick, T. C., and Erlandson, J. M. (2012). “Kelp forests, coastal migrations, and the younger dryas: Late Pleistocene and earliest Holocene human settlement, subsistence, and ecology on California’s Channel islands,” in Hunter-gatherer behavior: Human response during the younger dryas. Editor M. I. Eren (Walnut Creek: Left Coast Press), 79–110.
Rick, T. C., Erlandson, J. M., Vellanoweth, R. L., and Braje, T. J. (2005a). From Pleistocene mariners to complex hunter-gatherers: The archaeology of the California Channel Islands. J. World Prehist. 19 (3), 169–228. doi:10.1007/s10963-006-9004-x
Rick, T. C., Kennett, D. J., and Erlandson, J. M. (2005b). “Preliminary report on the archaeology and paleoecology of the Abalone rocks estuary, Santa Rosa island, California,” in Proceedings of the sixth California islands symposium (Arcata, CA: Institute for Wildlife Studies), 55–63.
Rick, T. C., Erlandson, J. M., Jew, N. P., and Reeder-Myers, L. A. (2013). Archaeological survey, paleogeography, and the search for late Pleistocene paleocoastal peoples of Santa Rosa island, California. J. Field Archaeol. 38, 324–331. doi:10.1179/0093469013z.00000000065
Roberts, L. W. (1991). San Miguel island: Santa barbara's fourth island west. Carmel, CA: Cal Rim Books.
Salwen, S. A. (2011). Asphaltum exchange and development of political complexity among the Chumash. Los Angeles, CA: Unpublished Master’s Thesis, UCLA.
Sanchez, G. M., Erlandson, J. M., and Tripcevich, N. (2017). Quantifying the association of chipped stone crescents with wetlands and paleoshorelines of Western North America. North Am. Archaeol. 38 (2), 107–137. doi:10.1177/0197693116681928
Schoenherr, A. A., Feldmeth, C. R., and Emerson, M. J. (2003). Natural history of the islands of California, 61. Oakland, CA: Univ of California Press.
Schumann, R. R., Minor, S. A., Muhs, D. R., and Pigati, J. S. (2014). Landscapes of Santa Rosa island, Channel Islands national park, California. Monogr. West. North Am. Nat. 7 (1), 48–67. doi:10.3398/042.007.0108
Schumann, R. R., and Pigati, J. S. (2017). Late quaternary fluvial history of Santa Cruz island, California, USA. West. North Am. Nat. 78 (4), 511–529. doi:10.3398/064.078.0401
Singer, R., and Wymer, J. J. (1982). The middle stone age at klasies river mouth in South Africa. Chicago: University of Chicago Press.
Sorlien, C. C. (1994). “Faulting and uplift of the Northern Channel islands, California,” in The fourth California Islands symposium: Update on the status of resources. Editors W. L. Halvorson, and G. L. Maender (Santa Barbara (CA): Santa Barbara Museum of Natural History), 281–296.
Steele, T. E., and Álvarez-Fernández, E. (2011). “Initial investigations into the exploitation of coastal resources in North Africa during the Late Pleistocene at Grotte des Contrebandiers, Morocco,” in Trekking the shore: Changing coastlines and the antiquity of coastal settlement. Editors N. F. Bicho, J. A. Haws, and L. G. Davis (New York: Springer), 383–403. doi:10.1007/978-1-4419-8219-3_16
Steneck, R. S., Graham, M. H., Bourque, B. J., Corbett, D., Erlandson, J. M., Estes, J. A., et al. (2002). Kelp forest ecosystems: Biodiversity, stability, resilience and future. Envir. Conserv. 29 (4), 436–459. doi:10.1017/s0376892902000322
Tahiry, H. (2019). Characterization of paleochannels offshore from the Northern Channel islands. California, USA. MA Thesis: San Diego State University, Department of Geology.
United States Department of Agriculture (USDA), Natural Resources Conservation Service (2007). Soil survey of Channel Islands national park, California. Accessible online at: http://soils.usda.gov/survey/printed_surveys/.
Wahoff, T. (2012). in Report on file at Camp Pendleton, AC/S environmental security (Oceanside, CA: Archaeological Resources Branch).Archaeological monitoring and evaluation report for the BEQ package 7 project marine corps base Camp Pendleton san Diego county, California.
Watson, E. B., Wasson, K., Pasternack, G. B., Woolfolk, A., Van Dyke, E., Gray, A. B., et al. (2011). Applications from paleoecology to environmental management and restoration in a dynamic coastal environment. Restor. Ecol. 19 (6), 765–775. doi:10.1111/j.1526-100X.2010.00722.x
Westerdahl, Christer (1992). The maritime cultural landscape. Int. J. Naut. Archaeol. 21 (1), 5–14. doi:10.1111/j.1095-9270.1992.tb00336.x
York, A. (2012). in Report on file at Camp Pendleton, AC/S environmental security (Oceanside, CA: Archaeological Resources Branch).Historic properties treatment plan for modifications to TAPS 12 in support of MILCON P-1043 and P-1093, marine corps base Camp Pendleton, California.
Keywords: paleolandscape, paleoecology, marine archaeology, paleosol, terminal Pleistocene, Early Holocene
Citation: Gusick AE, Maloney J, Braje TJ, Retallack GJ, Johnson L, Klotsko S, Ainis A and Erlandson JM (2022) Soils and terrestrial sediments on the seafloor: Refining archaeological paleoshoreline estimates and paleoenvironmental reconstruction off the California coast. Front. Earth Sci. 10:941911. doi: 10.3389/feart.2022.941911
Received: 12 May 2022; Accepted: 11 July 2022;
Published: 01 September 2022.
Edited by:
Rik Tjallingii, GFZ German Research Centre for Geosciences, GermanyReviewed by:
Alexander Ray Simms, University of California, Santa Barbara, United StatesMichael O’Leary, University of Western Australia, Australia
Copyright © 2022 Gusick, Maloney, Braje, Retallack, Johnson, Klotsko, Ainis and Erlandson. This is an open-access article distributed under the terms of the Creative Commons Attribution License (CC BY). The use, distribution or reproduction in other forums is permitted, provided the original author(s) and the copyright owner(s) are credited and that the original publication in this journal is cited, in accordance with accepted academic practice. No use, distribution or reproduction is permitted which does not comply with these terms.
*Correspondence: Amy E. Gusick, agusick@nhm.org
†These authors have contributed equally to this work and share first authorship