Engineering surveys of Sri Lankan schools exposed to tsunami
- 1EPICentre, University College London, London, United Kingdom
- 2Department of Civil Engineering, University of Moratuwa, Moratuwa, Sri Lanka
- 3Department of Civil Engineering, South Eastern University, Oluvil, Sri Lanka
- 4Department of Civil Engineering, University of Peradeniya, Kandy, Sri Lanka
- 5Department of Civil, Maritime and Environmental Engineering, University of Southampton, Southampton, United Kingdom
- 6Department of Structures for Engineering and Architecture, University of Naples Federico II, Napes, Italy
The 2004 Indian Ocean tsunami affected 5% of Sri Lanka’s schools, severely damaging 108 and destroying 74. The catastrophe highlighted the critical role of schools in providing educational continuity during community recovery. Sri Lanka has since rehabilitated and rebuilt most of the destroyed schools along the coastline. However, there is a limited understanding of current levels of school exposure to tsunami. This hampers preparedness and risk reduction interventions that can improve community and educational tsunami resilience. This paper presents a multi-disciplinary school exposure database relevant to both vulnerability and loss modelling. The repository includes data on 38 schools and 86 classroom buildings, surveyed across the coastal districts of Ampara, Batticaloa and Galle in Sri Lanka, which were heavily affected by the 2004 tsunami. A new engineering rapid visual survey tool is presented that was used to conduct the physical assessment of schools for the exposure repository. School damage mechanisms observed in past tsunami inform the survey forms, which are designed to capture information at both school compound and building levels. The tsunami engineering survey tools are universally applicable for the visual assessment of schools exposed to tsunami. The surveys show that most Sri Lankan school buildings can be classified into three building archetypes. This means that future risk assessments can be conducted considering a small number of index buildings that are based on these archetypes with differing partition arrangements and structural health conditions. The surveys also raise three significant concerns. Firstly, most schools affected by the 2004 tsunami remain in the same exposed locations without any consideration for tsunami design or strengthening provisions. Secondly, Sri Lankan schools are fragile to tsunami loading and many of the schools in the Galle district suffer from severe corrosion, which will further affect their tsunami vulnerability. Thirdly, schools do not appear prepared for tsunami, and do not have adequate tsunami warnings nor evacuation protocols in place. These observations raise the urgent need to mitigate tsunami risk, including a holistic plan for tsunami retrofitting and for interventions to improve the tsunami preparedness of schools in Sri Lanka.
1 Introduction
School facilities are vital community assets during and after natural disasters, including tsunami. They can provide emergency vertical refuge and play a vital role in education recovery. The UNISDR Comprehensive School Safety (CSS) initiative aims to safeguard students and educators from physical harm and ensure educational continuity in case of a disaster. The framework rests on three pillars: safe learning facilities; school disaster management; and risk reduction and resilience education (UNISDR and GADRRRES, 2017). Each pillar requires a comprehensive understanding of the risks facing the education system. Risk, in turn, requires the understanding of the system’s exposure to hazards.
The 26 December 2004 Indian Ocean tsunami, the deadliest geophysical disaster in Sri Lanka’s history, clearly demonstrated the vital role of schools. The disaster claimed over 35,000 casualties and missing persons, and internally displaced over one million people (Pomonis et al., 2006). The tsunami inflicted acute stresses on the education sector across Sri Lanka’s coastal regions. It affected 5% of the country’s schools, severely damaging 108 and destroying 74. It displaced 94,000 students and 3,000 teachers. Some 458 schools–but estimated by others as 287 (Bitter and Edirisinghe, 2013) - served as internally displaced persons (IDP) camps, causing disruption to education for a further 300,000 students (TERM, 2009; Bitter and Edirisinghe, 2013). Despite the immense shock to the country’s education system, classes resumed in January 2005. By March of that year, affected schools functioned either through double shift arrangements (teachers taught day and evening classes), student transfers or through the construction of temporary shelters. School enrolment also recovered. Overall dropout rates were low the year following the disaster in the most affected regions (UNICEF Evaluation Office, 2009). The legacy of the school rehabilitation process provides a window into today’s school exposure and vulnerability to tsunami. The Education Rehabilitation Monitor (TERM), a non-government organization set up immediately following the 2004 event by the Ministry of Education (MoE), coordinated and oversaw school rehabilitation and reconstruction efforts. By 2008, under the direction of TERM, the MoE, provincial Ministries and Departments of Education and a host of national and international agencies had rehabilitated 70% of the 182 destroyed and damaged schools. The civil war, between the Sri Lankan government and Liberation Tigers of Tamil Eelam (LTTE) forces, obstructed the reconstruction of a further 20 schools (UNICEF Evaluation Office, 2009). A key observation is that schools, NGOs, and local authorities mostly used a set of pre-approved architectural designs to speed up the approval process (De Mel et al., 2021). These designs did not consider the tsunami performance of schools and most schools were rebuilt in the same hazard-prone locations.
Sri Lanka remains susceptible to tsunami generated by megathrust subduction earthquakes in the Northern Andaman-Myanmar, Northern Sumatra-Andaman, and Southern Sumatra trenches. This is in addition to the Makran Trench in the Northern Arabian Sea (Wijetunge, 2010). A recent study conducted in the coastal provinces of Galle, Ampara and Batticaloa by Cels et al. (2022), showed that school principals rank tsunami as the most highly perceived threat facing schools. At the same time, the study found that tsunami are the hazard schools consider they are the least prepared for and that there is a general lack of preparedness and coordination between schools and government, heightening the level of risk to pupils and teachers.
Given the importance of school assets to communities, it is imperative to form an understanding of tsunami risks to the education system. This paper aims to define and classify the exposure of the school building stock of coastal Sri Lanka, enabling the assessment of their structural vulnerability to tsunami. The paper first presents a brief overview of existing survey tools and building classification systems relevant to schools affected by natural hazards. It then presents a new engineering rapid visual survey (RVS) tool for schools exposed to tsunami. The tool captures information at both school compound and individual building levels, allowing for the detailed collection of data for structural fragility and vulnerability analyses. The RVS was deployed in the survey of 38 school compounds (86 buildings in total) in three coastal districts in Sri Lanka between June and November 2020. The results of the survey show that a large portion of the school buildings are built according to three main archetypes or “type-plans”. These are presented in the following text, and the identified archetypes can be used to construct model index buildings for future physical vulnerability studies. The results of the school surveys in terms of structural properties of the school buildings (including the geometric configuration, infill configurations and member details) are also presented in this paper. The final school exposure database and an improved version of the rapid visual survey tool are available in the supplementary materials of this paper.
2 Existing engineering survey and classification methods for school buildings
Within risk assessment frameworks, Rapid Visual Survey tools (RVS) can be used to collect data on the attributes of a physical asset, such as a building, to assess their performance under given hazards. RVS are mostly used where large portfolios of buildings need to be assessed and are not appropriate for a site specific, highly detailed structural assessment. As such, RVS aim to collect the minimum number of attributes necessary to inform a vulnerability assessment and to reduce the time required for the survey of any single asset (hence they are “rapid”). Past studies have used RVS to assign buildings to a vulnerability class, for the development of relative risk indices (e.g., Baiguera et al., 2021). They have also been used to identify building archetypes for the development of representative index buildings that are then analysed to develop fragility or vulnerability functions (e.g., D’Ayala et al., 2020).
Structural classification systems provide the link between exposure data collected in the field and fragility or vulnerability models (Lang et al., 2018). Many such classification systems exist and are present in international building codes and risk assessment platforms, such as FEMA 256 (FEMA, 1994), HAZUS (FEMA, 2003), and PAGER (Jaiswal and Wald, 2008), among others. However, only a few classification systems consider tsunami in determining exposure characteristics and most do not focus on schools.
Schools have several distinct structural and non-structural characteristics that make them susceptible to damage from natural hazards. In addition to distinct architectural layout arrangements, school buildings can sometimes include enhanced structural design provisions (such as seismic provisions) due to being classed as high occupancy structures in building codes (e.g., Eurocode-8, 1998).
A well-established example of RVS to capture building earthquake exposure in seismic areas is FEMA P-154 (Lizundia et al., 2014), which a few studies have adapted for tsunami (e.g., Park et al., 2017 and; Park et al., 2019; Rahman and Rafique, 2018). RVS developed specifically for school infrastructure include the Safer Communities through Safer Schools (SCOSSO) mobile App (Nassirpour et al., 2018). The SCOSSO RVS collects data on structural and non-structural components/fittings in schools and has been applied to a case study consisting of 115 schools in the Philippines (D’Ayala et al., 2020; Nassirpour et al., 2017). This App considers several hazards, but not tsunami.
A study which explicitly developed a RVS for a tsunami vulnerability assessment of schools is the INSPIRE study (INdonesia School Programme to Increase REsilience). INSPIRE was developed for schools in Indonesia exposed to earthquakes and tsunami. Tsunami vulnerability is evaluated using the Papathoma Tsunami Vulnerability Assessment model (PTVA) (Dall’Osso et al., 2016; Papathoma and Dominey-Howes, 2003). The PTVA considers high level attributes that affect tsunami vulnerability and assigns weight factors to each attribute to produce an index, which has been validated against field data from the Indian Ocean tsunami (Dominey-Howes and Papathoma, 2006). The limitations of the INSPIRE RVS and of the PTVA model is that they miss some of the detailed (but key) attributes that make buildings susceptible to tsunami and are necessary for detailed analytical fragility analysis. They also neglect important damage mechanisms like scour and debris impact, or potential tsunami mitigation mechanisms like shielding from flow due to large barriers, which are based on observations of buildings’ surroundings. The Hospital Engineering Assessment for Resilience to Tsunami and Storm Surge in Sri Lanka (HEARTS-SL) RVS presented in Baiguera et al. (2021), does include the level of structural detail and information on building orientation and surroundings that is missing from the INSPIRE approach. It also extends traditional RVS by considering energy and other systems that are critical to determining the functionality of hospital units post-disaster. However, this RVS is highly bespoke to hospital units and not immediately transferable to schools.
3 Method
This paper presents a new RVS tool, called the ReSCOOL RVS tool after the sponsoring research project. The ReSCOOL RVS is designed to evaluate the tsunami exposure of school infrastructure. Its effectiveness is demonstrated through the engineering surveys of 86 school buildings across Sri Lanka by multiple teams of trained engineers.
The ReSCOOL RVS tool collects structural and non-structural exposure information related to tsunami resistance for each building within a school compound, allowing for the modelling of buildings towards their assessment under tsunami loading. It also allows for each structure to be classified according to existing building taxonomies, such as the Global Library Of School Infrastructure (GLOSI, World Bank Group, 2019) and the Global Exposure Database for Multi-Hazard Risk Analysis (GED4ALL, Silva et al., 2018). The RVS also collects information on building and resource arrangements within the school compound including school energy, water sources and preparedness for tsunami. This information allows the development of multi-disciplinary repositories of exposure information, aimed at improving disaster risk reduction efforts for evacuation and for maintaining/restoring school functionality after natural hazards. Although the new RVS tool was developed specifically for Sri Lanka, it can be applied worldwide for on-site rapid collection of data on school exposure to tsunami. The following sections describe the ReSCOOL RVS tool in detail.
3.1 The new ReSCOOL RVS tool for evaluating tsunami exposure of school infrastructure
The ReSCOOL RVS tool enables the collection of sufficient data on.
1) Structural and non-structural characteristics relevant to tsunami performance assessment and for the definition of index buildings for tsunami fragility analyses,
2) Elements of the school surroundings needed to identify susceptibility to debris and scour induced hazards as well as reduction in tsunami loads due to sheltering effects,
3) Occupancy, disaster preparedness, energy and communication systems to support educational resilience studies.
ReSCOOL RVS incorporates some of the school exposure characteristics defined in the SCOSSO project (D’Ayala et al., 2020; Nassirpour et al., 2017) and is hence adequate for characterising school exposure to earthquakes. However, the forms are added to and modified to explicitly consider damage mechanisms from tsunami inundation and associated secondary hazards (e.g., scour and debris impact). These damage mechanisms are informed by post-tsunami field surveys including Dias and Mallikarachchi (2006), Dias et al. (2006), Lagesse et al. (2019), Pomonis et al. (2006) and Rossetto et al. (2007).
The ReSCOOL RVS tool consists of two survey forms, as illustrated in Figure 1. The first form provides a high-level overview of the school compound and supporting infrastructure (referred to hereafter as the ‘school-level form’). The second form captures the detailed structural characteristics of each school building within the school complex (referred to hereafter as the ‘building-level form’). The ReSCOOL RVS tool is fully compatible with GLOSI and GED4ALL taxonomy systems.
The ReSCOOL RVS tool is provided in the supplementary materials (Supplementary Table S1); this has been improved since performing the actual surveys presented herein as a case-study.
3.2 School-level form
The first section of the school-level form captures key attributes such as the school’s name and student years taught; address and geolocation; and school type–whether it is a government, religious or private school. Part of the school-level form can be completed in advance of the field survey using data available online; remotely sensed images (e.g., from Google Earth); and school censuses, among other sources. Doing this research in advance can assists surveyors when they complete the rest of the RVS as they will be able to contextualise the school within a wider urban and coastal setting. The field survey can be used to complete the form and validate the desk-based observations.
The second section of the school-level form gathers data on the site characteristics of the school complex, including local soil conditions and the presence of perimeter walls. The former is key to the occurrence of scouring. Foundation scouring, as depicted in Figure 2B, was one of the main school building failure mechanisms observed in Sri Lanka (Dias et al., 2006). Scouring effectively washes away the soil underneath a structure (typically starting around the corners), undermining the foundations, and eventually leading to its collapse. Perimeter walls can act to block or divert the water flow so long as the wall does not fail. Urban conditions influence localized tsunami flow conditions, including the potential sheltering of one structure by another or as sources of debris (ASCE/SEI 7-16, 2017; Chock, 2016). To this end, the ReSCOOL RVS proposes the use of indices to characterise the level of urban density and vegetation surrounding the school complex.
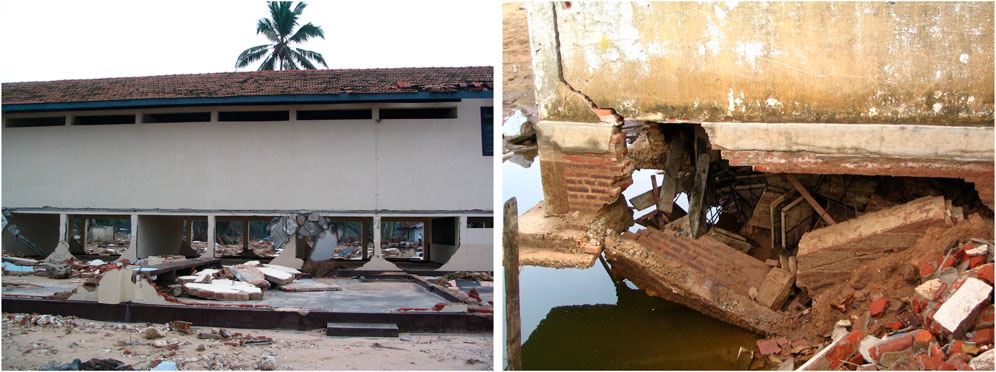
FIGURE 2. (A) Washed away building in front and washed away infills at the rear. (B) Foundation scouring of a corner.
The third section provides a referencing system to locate the buildings and assets on the complex alongside their primary classification parameters, including the asset type; number of storeys; structure type; footprint area; and orientations to the coastline. Building orientation with respect to the coast is a significant determinant of the net tsunami drag forces induced on a structure, as drag forces are a function of the surface area exposed to the tsunami flow (ASCE/SEI 7-16, 2017; Chock, 2016).
The third section of the school-level form collects any information available on the impact of previous hazard events and their intensity measure (e.g., inundation height in case of tsunami) that may have affected the school. Information on past hazard events is best obtained from discussions with school principals and staff on site. The school’s functional loss ratio and functional downtime are used as proxies for the impact on the school. The ReSCOOL RVS also gathers data on the individual level of damage of affected buildings, in addition to the cost and time-taken of any measures to reconstruct or rehabilitate them. These parameters are intended to enable an empirical evaluation of vulnerability and recovery for a particular hazard event.
The ReSCOOL RVS also includes a section for recording functional dependencies and their backup systems (e.g., water, power, telecommunication). Note that these aspects were not covered in the actual survey carried out and described below. Many schools in Sri Lanka rely primarily on water wells, many of which were contaminated or destroyed following the 2004 tsunami (Ratnasooriya et al., 2007).
The last section of the school-level form collects data on school tsunami preparedness, including any information on emergency tsunami protocol. Examples of this could be whether schools regularly engage in tsunami evacuation drills, or if they have designated evacuation routes or refuges.
3.3 Building-level form
The building-level form captures data on the structural and non-structural features of each school building in the school compound. Structural features include the building geometry; construction materials; and typical structural member details of the primary structure, such as foundations, columns, floor slabs and roof systems. Non-structural features focus primarily on the materials and configurations of non-load-bearing infill walls. All collected data is granular enough to enable the identification of building classes and the numerical modelling of school buildings.
Of particular importance to the tsunami performance of buildings are the physical attributes of columns, slabs, and infill walls. The horizontal tsunami loads on columns are strongly associated with the integrity of masonry infills and their out-of-plane resistance. This is because infills create a surface area on which the tsunami flow acts, thereby increasing the total drag forces acting on the structure. Once the infills break away, as depicted in Figure 2A, the reduction in surface area reduces the pressure on the columns and structure (Chock, 2016; Del Zoppo et al., 2021a; Del Zoppo et al., 2021b). Furthermore, infills that act in-plane to the tsunami flow contribute to the lateral strength and stiffness of the structure, at least initially before the infill materials degrade or columns fail. However, partial height infill walls can create a ‘short column’ effect that can precipitate column shear failure. This re-enforces the importance of capturing details on infill geometry, strengths, connections to the structure and openings.
Recent research has highlighted the importance of floor slabs due to the substantial influence that vertical buoyancy forces can have on a structure’s performance in a tsunami. As stipulated by the ASCE 7-16, Chapter 16 (ASCE/SEI 7-16, 2017), when the inundation rises to the first-floor slab, air pockets underneath the slab result in the formation of upward pressure. These upward forces reduce the axial forces on the columns below and thus their lateral resistance capacity (Del Zoppo et al., 2022). The roof plays a smaller role in tsunami vulnerability mainly because a structure is likely to suffer severe damage by the time the inundation reaches the roof level (with the caveat that the roof system contributes to gravity loads and lateral stiffness). Rather, roof attributes are collected to provide data for cyclone, storm, and high wind exposure. The role of staircases is twofold, on the one hand, a staircase can stiffen the structure laterally or in torsion. The asymmetric location of staircases can, for example, lead to eccentric stiffness and a non-uniform load pattern, causing irregular exertion of lateral forces. On the other hand, staircases serve as routes for vertical evacuation. Their location and accessibility are thus critical factors for evacuation protocols. The building-level form collects additional information on the foundation system which becomes especially relevant to tsunami vulnerability if the structure is susceptible to foundation scouring, as discussed under the school-level form.
The building-level form collects data on the building age and any strengthening measures or building alterations. Building age is useful information to contextualize structures in relation to building codes and practices. It also puts structures in context with respect to possible exposure to past hazard events. Finally, surveyors assess the general structural health of the building, capturing any existing damage or degradation, such as the corrosion of structural members. Significant material degradation such as concrete spalling and steel corrosion, particularly of columns, will affect the structure’s ability to withstand tsunami (or gravity) loads.
4 Case-study: Sri Lankan schools
4.1 Sri Lankan school exposure to tsunami
Following the 2004 Indian Ocean tsunami, the Ministry of Education (MoE) and others sought to address the fundamental question of school exposure to a tsunami. The MoE made attempts to relocate the affected schools further inland, thereby reducing school tsunami exposure and risk. These efforts were only partially successful. Due to difficulties in procuring land for school relocation and resistance from local communities, few schools were relocated outside the coastal buffer zone. The buffer zone was initially set at minimum of 100-m from the coast to encourage reconstruction further inland. But it was soon reduced to local setback distances specified by the Coast Conservation Department based on coastal erosion (usually in the range of 35–120 m form the Mean High-Water Line) (Coast Conservation Department, 2021).
Figures 3A,B, show the recovery funds allocated to 187 of the severely affected schools according to the tsunami reconstruction and rehabilitation program (TERM, 2009). Figure 3B shows that the allocated recovery funds (aggregated by district) have an almost linear relationship to the number of students in each district. It also highlights that the three most heavily affected regions in terms of the number of pupils and reconstruction fund allocations are Batticaloa, Galle and Ampara. These three regions were highlighted by post-tsunami field surveys to have sustained some of the greatest losses (Jayasuriya et al., 2005; Palliyaguru et al., 2007; Rossetto et al., 2007) and largest levels of tsunami inundation following the 2004 event (Goff et al., 2006; Wijetunge, 2012). They are the regions of focus for the RESCOOL RVS implementation.
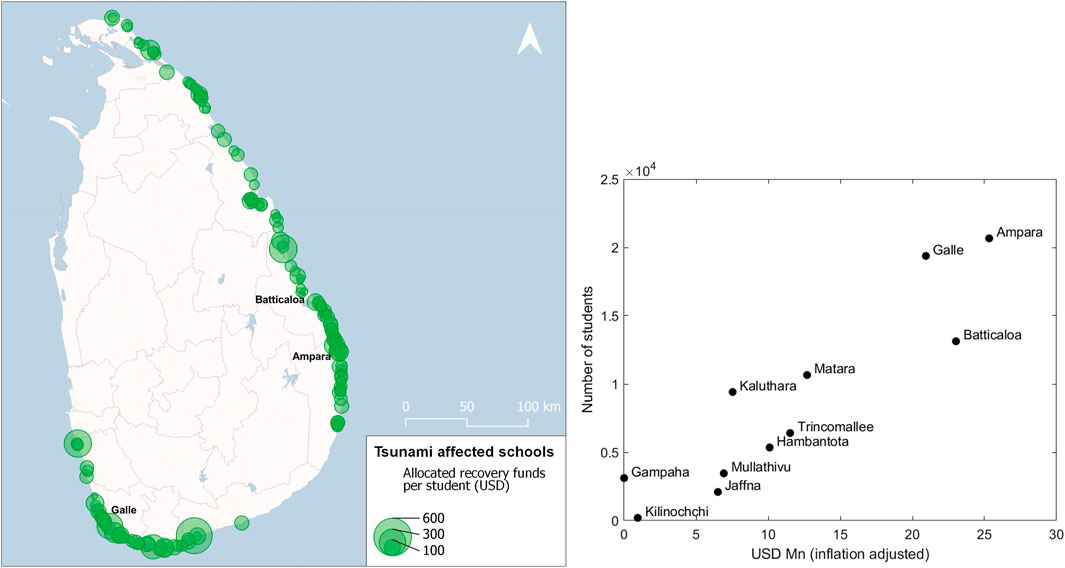
FIGURE 3. (A) School recovery funds allocated per student. (B) Total number of students by district versus allocated funds. Data from TERM, 2009.
Simulations of nine maximum probable tsunami scenarios reveal that a seismic event in the Northern Sumatra–Andaman segment of the Sunda Arc would cause the greatest inundation amplitudes in Sri Lanka (Wijetunge, 2012). The Sri Lankan Disaster Management Centre (DMC) uses the 2004 event’s inundation footprint generated using the earthquake source in Wijetunge, (2012) as the basis for Sri Lanka’s hazard definition (DMC, 2012; Wijethunga and Piyasiri, 2012). At the time of writing, there are 430 coastal schools in Sri Lanka that lie within the DMC tsunami inundation zone, potentially affecting over 193,000 pupils according to the 2019 school census (DMC, 2012; Ministry of Education, 2019). Figure 4 shows the schools (orange circles scaled by pupil population size) that lie within the DMC tsunami hazard zones. The DCM characterises the tsunami hazard zones into three inundation height ranges: low (less than 0.5 m), medium (between 0.5m and 2 m), and high (greater than 2 m).
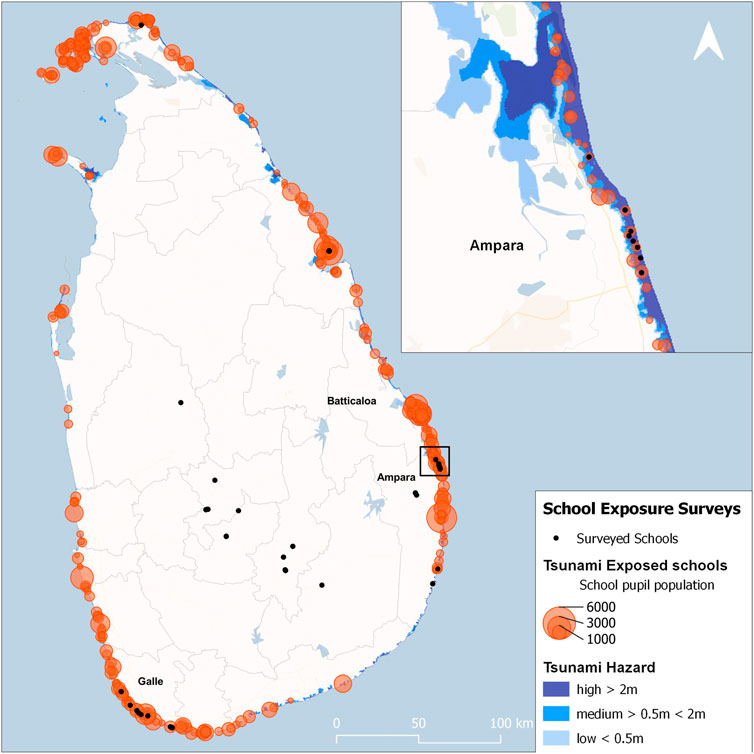
FIGURE 4. Schools exposed to tsunami and schools surveyed; enlarged window of the Ampara district. Data from the DMC, 2012 and Ministry of Education, 2019.
4.2 Surveyed schools
ReSCOOL RVS were conducted on 38 school compounds, comprising 86 individual school buildings, as depicted in Figure 4. The three primary districts of focus were Ampara, with 12 school compounds and 34 school buildings surveyed; Batticaloa, with four school compounds and eight buildings; and Galle, with eight school compounds and 21 buildings. In addition to this core set of coastal schools, students from the South Eastern University of Sri Lanka expanded the database and surveyed schools in their home districts using the same forms. This expands the database to include 14 school compounds and 23 school buildings in the Trincomalee, Jaffna, Kandy, Badulla, Kurunegala and Monaragala Districts. Although not all these schools are within the tsunami hazard zone, they are included in the database (Supplementary Table S2) as they help characterize school building architypes and exposure to other hazard. The surveys did not include single storey structures as they have limited resistance capacity to tsunami and cannot serve as vertical evacuation refuges.
5 Survey findings
The survey data collected using the ReSCOOL RVS tool are presented in the following sections. The findings relate to the typical structural and architectural designs of the surveyed schools, as well as their infill configurations and structural health conditions. In addition, the state of school tsunami preparedness is discussed. This data is valuable for the vulnerability and risk assessment of schools in Sri Lanka.
5.1 School archetypes
The surveys reveal that most schools in Sri Lanka follow one of three standardized layout arrangements, here denoted as Type 1, 2 and 3 and shown in Figures 5–7, respectively. Type 1 schools have two rows of columns at the perimeter aligned with the longer dimension of the building. Type 2 schools have a similar layout to Type 1 but have an additional row of columns aligned with the corridor. This additional row of columns may improve their seismic and tsunami performance, as they enhance the lateral load resistance of the building. Type 1 and Type 2 schools are typically two to four storeys tall, and their architectural arrangement comprises a central staircase with two or three classrooms on either side connected by a longitudinal corridor. A classroom typically spans two bays, each of which is about 3 m (although classrooms sometimes span three or four bays). Most Type 1 and Type 2 schools have nine bays, i.e., four classrooms. However, the number of bays ranges from seven to 16, i.e., two to eight classrooms. The transverse width of the structure is typically around 7.5–10 m for both Type 1 and Type 2 schools. Type 3 schools, much fewer in number, have a central staircase with four adjoining classrooms, which are used as lab rooms, libraries, staff rooms, or rooms for other supporting functions, see Figure 7. Type 3 school buildings are typically two to three storeys high.
The MoE’s use of pre-approved architectural and structural designs provides the best explanation for the prevalence of the three identified typologies amongst the Sri Lankan school building stock, both pre-and post-tsunami. The surveys reveal that there are no significant differences in terms of architectural type plans, design and construction practices among the investigated districts. Figure 8 shows the year of construction (completion) of the entire dataset of coastal school buildings surveyed, classified by building type. Table 1 provides the breakdown of building type by district. It is noted that information about the year of construction is not available for 18 schools. Although Type 1 is the most prevalent architype, a higher proportion of Type 2 schools were built following the tsunami, indicating that there may have been an awareness that buildings needed greater resistance to lateral loads as Type 2 ones have an additional row of columns; Figure 8 also clearly shows a large spike in the numbers of newly constructed school buildings in tsunami-stricken regions over a period of 2 years following the tsunami, implying a 2-year average reconstruction time. A report by the Education Rehabilitation Monitor further substantiates this; it indicates that most affected schools received the full amount of their allocated reconstruction funds by this time. Schools that did not were mostly within conflict zones (TERM, 2009).
It is important to note that a 2-year reconstruction time for school buildings does not equate to a ‘downtime’ period for education provision. Building functionality is a poor proxy for school or educational downtime as this negates the measures schools and relief efforts can take to resume classes in a disaster’s aftermath. In the case of Sri Lanka, classes mostly resumed between 2 weeks and 2 months after the tsunami, with school attendance effectively fully recovered by the end of 2005. This was achieved through student transfers to undamaged/functional schools and the erection of temporary teaching facilities (UNICEF, 2009). Hence, school recovery and resilience require a more nuanced understanding of school functionality than merely reconstruction times.
5.2 Structural designs
Sri Lanka does not have national design codes for buildings, or specific regulations for schools. Instead, Sri Lankan engineers adopt a range of building standards including Eurocodes, (predominantly) British Standards and (occasionally) Australian design standards (Rossetto et al., 2019). None of the building codes adopted consider tsunami loading, and seismic design is not considered in Sri Lanka. Hence, school design in Sri Lanka is dominated by gravity load design, which is covered in similar ways by the predominantly used building codes, leading to a general uniformity in element sizes and detailing. All school buildings covered by the survey are Reinforced Concrete (RC) moment resisting frames with masonry brick infills. They tend to have one column type, one beam type for the short spans between bays in the longitudinal direction, and a different beam design for the long spans in the transverse direction. Figure 9 depicts the nominal structural member section details for Type 1, Type 2 and Type 3 archetypes. School structural drawings from the MoE School Works Division stipulate the use of BS CP110:1972 and BS 8110: 1985, which specify a characteristic strength concrete of 20 or 25 MPa, a steel yield strength of 460 MPa for longitudinal reinforcement, and a mild steel strength of 250 MPa for stirrups.
No significant differences in structural design have been observed among the surveyed districts. All school buildings surveyed have RC floor slabs which are mostly 125 mm in depth. Most school buildings have gable pitched roofs, supported by either steel (75%), timber (20%), or mixed RC and timber (4%) truss systems, except for one school building with a flat RC roof. Roof coverings are either clay tiles (55%), metal sheets (10%) or asbestos sheets (35%). Most surveyed school buildings have shallow brick strip foundations for walls and pad footings for columns, built on sandy soils, owing to their coastal proximity. This paper thus classifies the foundations as flexible, with an elevated risk of scour, supported by evidence during the 2004 tsunami and depicted in Figure 2B (Dias et al., 2006).
5.3 Masonry infill configurations
The presence of infills can have a substantial effect on the building response during tsunami inundation. The ReSCOOL RVS therefore collects information on infill type, material properties, their connection to the surrounding frames, and opening configurations. The masonry infills are either made with clay bricks, with nominal dimensions of 200 × 100 × 50 mm, or (increasingly) cement blocks, with nominal dimensions of 300 × 100 × 150 mm to 300 × 200 × 150 mm (length × width × height), respectively. Wall plaster (∼10 mm thickness) is made of a cement-sand mortar mix ratio of 1:5. The characteristic strength of infill masonry walls depends on the properties of the individual constitutive materials (i.e., masonry unit and mortar). The material strength and quality of clay bricks and cement blocks vary in Sri Lanka because construction materials are not fully standardised and controlled. An investigation into material strengths of brick and block samples indicated that these have low strength, i.e., less than 10 MPa (Thamboo and Dhanasekar, 2020). Mortar in Sri Lanka is typically made using a cement-to-sand ratio of 1:5, corresponding to a strength class of 4 MPa as per EN 1996-1-1 (2005). The compressive strength of masonry assemblages varies in the range of 1.4 MPa–7.3 MPa according to recent experiments from South Eastern University, Sri Lanka (Thamboo and Dhanasekar, 2019; Thamboo, 2020).
The infills and partitions of all the surveyed schools are mostly unconfined masonry. The surveys show that the infill configurations can be grouped into eight classes, (three classes for the front elevations and five classes for the rear elevations), as depicted in Figure 10. Schools typically have a uniform combination of one front and one rear infill configuration across all bays along the longitudinal direction of the structure. The thicknesses of the infills vary between 125 mm and 230 mm, corresponding to masonry brick (solid clay) or block type used and their bonded thickness (half- and full-brick masonry walls). The gable end walls and the two internal walls abutting the staircase are mostly of full-brick masonry construction; and in some cases, the gable end walls constitute masonry confined on all four sides. Classroom partitions and external walls in the longitudinal direction are typically half-brick masonry.
5.4 Structural health and maintenance
Several schools, mostly in the Galle district, exhibit severe structural and non-structural degradation. The surveys found multiple instances of severe cracking and concrete spalling due to reinforcement corrosion in columns, beams, and floor slabs. Figure 11A depicts examples of severe spalling and reinforcement corrosion observed in some ground floor columns of surveyed school buildings. Typical non-structural degradation was in the form of broken roof sheets or tiles, and water leaks and ingress. In addition, in several school buildings the steel roof trusses displayed significant signs of corrosion and rust, as shown in Figure 11B.
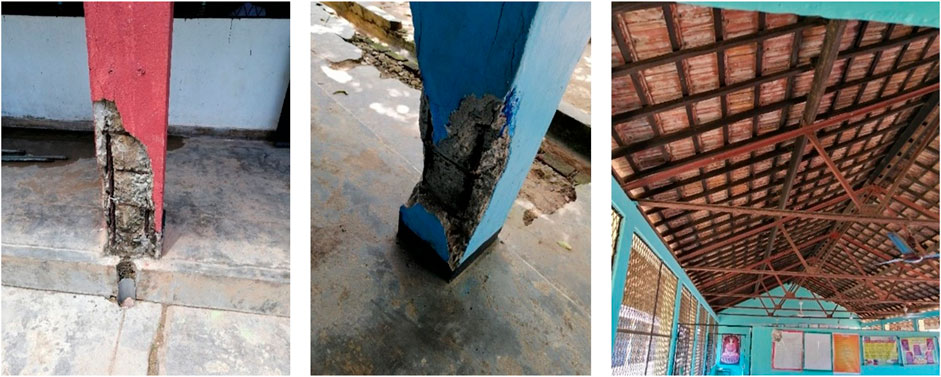
FIGURE 11. (A) Left and centre, Structural degradation: Severe corrosion of columns (B) Right, Roof degradation: Corrosion of steel trusses.
Figure 12 plots the distribution of the surveyed buildings according to their structural health conditions and building age. As expected, age is a reasonable predictor of condition. None of the buildings constructed after 2005 are in a poor condition. A poor structural health condition denotes significant degradation to the structure and threatens the structural integrity of the building if not addressed (like in Figure 11A). A fair health condition denotes minor degradation to structural members (hairline cracks), and some lack of maintenance to non-structural members. Multiple factors may explain the elevated levels of building degradation of such a young building stock - ‘young’ because the mean year of construction of the surveyed buildings in both the Galle and Ampara districts is 2003. One plausible factor is the proximate locations of the schools to the coast. There is some indication, for example, that the proximity to the coast may influence the level of corrosion of RC members and steel roof trusses. This is especially the case in the Galle district, where four schools have poor structural health conditions, and the others have fair conditions. Conversely, in Ampara only one school is in a poor state of structural health, while 27 have fair and six have excellent conditions. In Batticaloa and the other surveyed districts, only one school has a poor structural health condition, 26 have fair conditions and four have excellent conditions. The discrepancy between the Galle and the other districts may be due to regional differences in construction materials, building construction and maintenance practices.
The lack of systematic maintenance, however, is likely to be the main contributing factor to the poor structural health of the building stock. Several school principals expressed concerns during the building surveys regarding the lack of funds available to conduct maintenance works. Moreover, multiple schools contain abandoned and severely dilapidated buildings on school grounds. The principals of these schools indicated that they had not obtained the funds for their demolition and stressed that they constitute a safety hazard (which, objectively, they do). In an independent investigation that looked at the level of preparedness of schools to tsunami, principals (unprompted) highlighted building degradation as posing a considerable danger to the safety of students and staff (Cels et al., 2022). These interviews tell a similar story of difficulties in obtaining funds for repair works. In some cases, school principals have attempted to raise funds themselves or have partially or entirely closed off buildings in fear of spalling material.
5.5 Tsunami and other hazard preparedness
The schools surveyed showed a low level of preparedness for tsunami and other natural hazards for all the districts. None of the schools have any firefighting equipment or practice fire drills, suggesting a low baseline level of preparedness. None of the schools in this survey actively conduct regular tsunami evacuation drills. Only two surveyed schools have identified emergency assembly points and evacuation routes. One of these two schools has designated the upper storeys of two and three storeyed schools as refuges. A tsunami fragility study of a two storey Type 1 school in Sri Lanka by Del Zoppo et al. (2021b) raises the question of whether schools should designate two or three storeyed school buildings as vertical evacuation refuges, given their high level of fragility to tsunami. Furthermore, if the tsunami inundation simulations of Goff et al. (2006) and Siriwardana et al. (2005) are considered, onshore inundation could reach heights up to 12–15 m. Inundation of this height would make three storey school structures unusable as safety refuges since the structure would be fully submerged.
Discussions with school principals during the surveys also highlighted the progressive loss of human capital with respect to tsunami preparedness. For example, multiple principals noted that teacher training in tsunami preparedness, along with practice drills, have significantly diminished in recent years and are not systematic. Many teachers that did receive training have since left the schools. This leaves many schools without any trained staff in tsunami evacuation protocol.
6 Conclusion
Since Sri Lanka remains highly exposed to tsunami and given the vital role of schools in community resilience, assessing the coastal hazard, exposure and vulnerability of schools is of critical importance to ensuring the resilience of coastal communities in Sri Lanka. This paper presents a new engineering rapid visual survey tool for schools exposed to tsunami, ReSCOOL RVS, which is provided in the supplementary material. The ReSCOOL RVS tool is designed to capture key parameters that affects a school’s susceptibility to tsunami (and earthquake) damage. This includes the structural and non-structural characteristics of school buildings and information on the school compound and its surroundings. Moreover, the tool includes sections for recording information on the hazard preparedness of schools and functional dependencies (e.g., water and energy sources). The ReSCOOL RVS was deployed in the survey of 38 school compounds (consisting of 86 buildings), in three districts most severely hit by the 2004 Indian Ocean tsunami in Sri Lanka, namely, the Ampara, Batticaloa and Galle districts. This paper presents a database of the school exposure data collected in the supplementary material, where each school building is also classified according to the GLOSI and GED4ALL taxonomies for wider applicability. The school surveys reveal that there are three prevalent school building archetypes in Sri Lanka, which range from two to four storeys (note that single storey structures were not surveyed due to their limited tsunami resistance). In addition, several combinations of non-structural partitions were also identified. The configurations and properties of these three school types are presented with the view of enabling future detailed numerical fragility assessments of the school archetypes which will, in turn, enable tsunami vulnerability assessments of schools in Sri Lanka.
Overall, this study has found that the tsunami exposure of the school system in Sri Lanka remains high since the 2004 tsunami. With reference to the 2019 school census and the tsunami hazard as defined by Sri Lanka’s Disaster Management Centre, there are currently 430 schools that are within the 2004 Indian Ocean tsunami inundation zone. The engineering surveys corroborate that most schools were rebuilt to the same pre-2004 designs and have not been refitted with tsunami strengthening measures. Past studies performed by the authors on the tsunami performance of one of the archetypes highlights the high fragility of the Type 1 school building design. Furthermore, the survey presented in this paper highlights the lack of systematic maintenance of schools, particularly those in the Galle district. Unless rectified, this deterioration will further compromise school building performance under future tsunami. These factors increase the burden on tsunami preparedness and evacuation to mitigate the tsunami risk to life and to the education network of Sri Lanka. However, the survey reveals a concerning lack of preparedness for tsunami and other hazards, with no schools with any firefighting equipment, and very few conducting regular evacuation drills. Improving the tsunami resilience of Sri Lanka’s school network will require a holistic and comprehensive approach. One that reduces physical exposure and fragility to tsunami where possible, and crucially, one that ensures schools are prepared and able to effectively evacuate.
Data availability statement
The original contributions presented in the study are included in the article/Supplementary Materials; further inquiries can be directed to the corresponding author.
Author contributions
TR was project lead; TR and PD directed and coordinated research activities. JC prepared the manuscript, charts, and figures with contributions from all co-authors. Photos are courtesy of PD, JT, and KW. JT and KW and their researchers conducted the rapid visual surveys, JC processed the survey data and prepared the school exposure database with contributions from PD, JT, and KW. MB, JC, and MZ co-authored the ReSCOOL Rapid Visual Survey tool, JC improved the tool following lessons learned from the field surveys.
Funding
The research presented in this paper was funded by the United Kingdom Global Challenges Research Fund project ReSCOOL (Resilience of Schools to Extreme Coastal FlOOding Loads), awarded to TR by Research England; award 177813.
Acknowledgments
We would like to acknowledge the significant contributions and efforts the following students at the South Eastern University and the University of Peradeniya in Sri Lanka who assisted JT and KW for the building surveys: M. Sathurshan, M.I. Sifan Mohammed, EMSD Jayasooriya, HMSS Hippola.
Conflict of interest
The authors declare that the research was conducted in the absence of any commercial or financial relationships that could be construed as a potential conflict of interest.
Publisher’s note
All claims expressed in this article are solely those of the authors and do not necessarily represent those of their affiliated organizations, or those of the publisher, the editors and the reviewers. Any product that may be evaluated in this article, or claim that may be made by its manufacturer, is not guaranteed or endorsed by the publisher.
Supplementary material
The Supplementary Material for this article can be found online at: https://www.frontiersin.org/articles/10.3389/feart.2023.1075290/full#supplementary-material
References
ASCE/SEI 7-16 (2017). “Minimum design loads and associated criteria for buildings and other structures,” in Minimum design loads and associated criteria for buildings and other structures (America: ASCE). doi:10.1061/9780784414248
Baiguera, M., Rossetto, T., Palomino, J., Dias, P., Lopez-Querol, S., Siriwardana, C., et al. (2021). A new relative risk index for hospitals exposed to tsunami. Front. Earth Sci. 9. doi:10.3389/feart.2021.626809
Bitter, P., and Edirisinghe, S. (2013). “Education in conflict and emergencies introduction of disaster safety in the Sri Lankan education system: Factors for success,” in UKFIET International Conference on Education and Development – Education and Development Post, UK, October 2013.
Cels, J., Rossetto, T., Little, A. W., and Dias, P. (2022). Tsunami preparedness within Sri Lanka’s education system. Int. J. Disaster Risk Reduct. 84, 103473. doi:10.1016/J.IJDRR.2022.103473
Chock, G. Y. K. (2016). Design for tsunami loads and effects in the ASCE 7-16 standard. J. Struct. Eng. (United States) 142 (11), 1–12. doi:10.1061/(ASCE)ST.1943-541X.0001565
Coast Conservation Department (2021). Setback standards for development activities in the coastal zone by Segment. Available at: https://www.coastal.gov.lk/images/pdf/Coastal_Setback.pdf.
Dall’Osso, F., Dominey-Howes, D., Tarbotton, C., Summerhayes, S., and Withycombe, G. (2016). Revision and improvement of the PTVA-3 model for assessing tsunami building vulnerability using “international expert judgment”: Introducing the PTVA-4 model. Nat. Hazards 83 (2), 1229–1256. doi:10.1007/s11069-016-2387-9
D’Ayala, D., Galasso, C., Nassirpour, A., Adhikari, R. K., Yamin, L., Fernandez, R., et al. (2020). Resilient communities through safer schools. Int. J. Disaster Risk Reduct. 45, 101446. doi:10.1016/j.ijdrr.2019.101446
De Mel, T., and Sivagnanam, M. (2021). Interview: Sri Lanka’s education sector following the 2004 Indian Ocean tsunami Interview.
del Zoppo, M., Ludovico, M. d., and Prota, A. (2021a). Methodology for assessing the performance of RC structures with breakaway infill walls under tsunami inundation. J. Struct. Eng. 147 (2), 04020330. doi:10.1061/(ASCE)ST.1943-541X.0002900
del Zoppo, M., Rossetto, T., Ludovico., Md., and Prota, A., (2022). Effect of buoyancy loads on the tsunami fragility of reinforced concrete frames including consideration of blow-out slabs. doi:10.21203/rs.3.rs-1178101/v1
del Zoppo, M., Wijesundara, K., Rossetto, T., Dias, P., Baiguera, M., di Ludovico, M., et al. (2021b). Influence of exterior infill walls on the performance of RC frames under tsunami loads: Case study of school buildings in Sri Lanka. Eng. Struct. 234, 1. doi:10.1016/j.engstruct.2021.111920
Dias, P., Dissanayake, R., and Chandratilake, R. (2006). Lessons learned from tsunami damage in Sri Lanka. Proc. Institution Civ. Eng. Civ. Eng. 159 (2), 74–81. doi:10.1680/cien.2006.159.2.74
Dias, W. P. S., and Mallikarachchi, H. M. Y. C. (2006). Tsunami - planning and design for disaster mitigation. Struct. Eng. 84 (11), 25–29.
Dominey-Howes, D., and Papathoma, M. (2006). Validating a tsunami vulnerability assessment model (the PTVA model) using field data from the 2004 Indian Ocean tsunami. Nat. Hazards 40 (11), 113–136. doi:10.1007/S11069-006-0007-9
FEMA (2003). HAZUS ®MH M R4 technical manual. Washington, D.C.: Federal Emergency Management Agency.
FEMA (1994). Seismic rehabilitation of federal buildings: A benefit/cost model volume 1-A user’s manual. Washington, D.C.: Federal Emergency Management Agency, 1.
Goff, J., Liu, P. L. F., Higman, B., Morton, R., Jaffe, B. E., Fernando, H., et al. (2006). Sri Lanka field survey after the December 2004 Indian Ocean tsunami. Earthq. Spectra 22 (3), 155–172. doi:10.1193/1.2205897
Jaiswal, K., and Wald, D. J. (2008). Creating a global building inventory for earthquake loss assessment and risk management. Available at: https://earthquake.usgs.gov/static/lfs/data/pager/Jaiswal_Wald_(2008)_14WCEE_PAGER_Inventory.pdf.
Jayasuriya, S., Weerakoon, D., Knight-John, M., and Arunatilake, N. (2005). Post-tsunami recovery: Issues and challenges in Sri Lanka. Bank Institute (ADBI) (Issue October)Available at: http://www.adbi.org/files/2006.06.rp71.tsunami.recovery.english.pdf.
Lagesse, R., Rossetto, T., Raby, A., Brennan, A., Rovertson, D., and Adhikari, R. K. (2019). "Observations from the EEFIT-TDMRC mission to Sulawesi, Indonesia to investigate the 28th September 2018 central sulawesi earthquake," in In SECED 2019 Conference: Earthquake Risk and Engineering towards a Resilient World. London, UK: Society for Earthquake and Civil Engineering Dynamics (SECED).
Lang, D. H., Kumar, A., Sulaymanov, S., and Meslem, A. (2018). Building typology classification and earthquake vulnerability scale of Central and South Asian building stock. J. Build. Eng. 15, 261–277. doi:10.1016/J.JOBE.2017.11.022
Lizundia, B., Durphy, S., Griffin, M., Hortacsu, A., Kehoe, B., Porter, K., et al. (2014). “Rapid visual screening of buildings for potential seismic hazards: FEMA 154 and FEMA 155 updates,” in NCEE 2014 - 10th U.S. National Conference on Earthquake Engineering: Frontiers of Earthquake Engineering, United States, 08 September 2022 (NCEE). doi:10.4231/D3M90238V
Ministry of Education (2019). Annual school census of Sri Lanka. Available at: http://www.statistics.gov.lk/Education/StaticalInformation/SchoolCensus/2019.
Nassirpour, A., Adhikari, R. K., Galasso, C., and D’Ayala, D. (2017). Safer communities through safer schools rapid visual survey. UCL EPICentre 3, 20170.
Nassirpour, A., Galasso, C., and D’Ayala, D. (2018). A mobile application for multi-hazard physical vulnerability prioritization of schools. 16th European conference on earthquake engineering (ECEE). Available at: https://discovery.ucl.ac.uk/id/eprint/10053915/.
Palliyaguru, R. S., Amaratunga, D., and Haigh, R. (2007). "Effects of post disaster infrastructure reconstruction on disaster management cycle and challenges confronted: The case of Indian Ocean tsunami in Sri Lanka," in 7th international postgraduate conference in the built and human environment, Salford Quays, UK.
Papathoma, M., and Dominey-Howes, D. (2003). Tsunami vulnerability assessment and its implications for coastal hazard analysis and disaster management planning, Gulf of Corinth, Greece. Nat. Hazards Earth Syst. Sci. 3 (6), 733–747. doi:10.5194/NHESS-3-733-2003
Park, H., Alam, M. S., Cox, D. T., Barbosa, A. R., and van de Lindt, J. W. (2019). Probabilistic seismic and tsunami damage analysis (PSTDA) of the Cascadia Subduction Zone applied to Seaside, Oregon. Int. J. Disaster Risk Reduct. 35, 101076. doi:10.1016/J.IJDRR.2019.101076
Park, H., Cox, D. T., and Barbosa, A. R. (2017). Comparison of inundation depth and momentum flux based fragilities for probabilistic tsunami damage assessment and uncertainty analysis. Coast. Eng. 122, 10–26. doi:10.1016/J.COASTALENG.2017.01.008
Pomonis, A., Rossetto, T., Wilkinson, S., Re, D., Gallocher, S., Peiris, N., et al. (2006). The Indian Ocean tsunami of 26 december 2004: Mission findings in Sri Lanka and Thailand. 44 Available at: http://www.istructe.org/webtest/files/74/74b66946-020a-430d-a684-0f71ff0d2a23.pdf.
Rahman, S. M. S., and Rafique, F. (2018). “A review on seismic vulnerability assessment of the existing buildings of chittagong, the port city of Bangladesh,” in International Conference on Research and Innovation in Civil Engineering (ICRICE 2018), Bangladesh, January 2018 (ICRICE).
Ratnasooriya, H. A. R., Samarawickrama, S. P., and Imamura, F. (2007). Post tsunami recovery process in Sri Lanka. J. Nat. Disaster Sci. 29 (1), 21–28. doi:10.2328/JNDS.29.21
Rossetto, T., Peiris, N., Pomonis, A., Wilkinson, S. M., Del Re, D., Koo, R., et al. (2007). The Indian Ocean tsunami of december 26, 2004: Observations in Sri Lanka and Thailand. Nat. Hazards 42 (1), 105–124. doi:10.1007/s11069-006-9064-3
Rossetto, T., Twigg, J., Dias, P., Shanaka, S., Baiguera, M., and Robinson, D. (2019). Sri Lanka building regulatory capacity assessment (issue 1258029). Washington D.C.: The World Bank.
Silva, V., Yepes-Estrada, C., Dabbeek, J., Martins, L., and Brzev, S. (2018). GED4ALL - global exposure database for multi-hazard risk analysis - multi-hazard exposure taxonomy. In GEM Technical Report.
Siriwardana, C. H. E. R., Ranasinghe, N., Weerakoon, D. P. R., Ratnayake, A. M. M., Jayakody, A. S., and Fernando, W. I. S. (2005). "Final report on the tsunami mapping program (TMP) conducted in the eastern, southern and western coastal regions of Sri Lanka," in Geological survey and mines Bureau, Colombo.
TERM (2009). "Reconstruction and rehabilitation Program of tsunami affected schools," in The education rehabilitation monitor, Colombo, October.
Thamboo, J. A., and Dhanasekar, M. (2020). Assessment of the characteristics of lime mortar bonded brickwork wallettes under monotonic and cyclic compression. Constr. Build. Mater. 261, 120003. doi:10.1016/j.conbuildmat.2020.120003
Thamboo, J. A., and Dhanasekar, M. (2019). Correlation between the performance of solid masonry prisms and wallettes under compression. J. Build. Eng. 22, 429–438. doi:10.1016/j.jobe.2019.01.007
Thamboo, J. A. (2020). Material characterisation of thin layer mortared clay masonry. Constr. Build. Mater. 230, 116932. doi:10.1016/j.conbuildmat.2019.116932
UNICEF (2009). Children and the Indian Ocean tsunami: Evaluation of UNICEF’s response in Indonesia, Sri Lanka and Maldives (2005-2008). November 14, 27. Available at: http://www.unicef.org/evaldatabase/files/Indonesia_Final_for_Print__20_Jan.pdf.
UNICEF Evaluation Office. (2009). Children and the 2004 Indian Ocean tsunami: Evaluation of UNICEF’s response in Sri Lanka. Country Synthesis Report (Issue November). New York: United Nations Childrens' Fund.
UNISDR and GADRRRES (2017). Comprehensive school safety: A global framework in support of the global Alliance for disaster risk Reduction and Resilience in the education Sector and the worldwide Initiative for safe schools (issue march). Global Alliance for Disaster Risk Reduction and Resilience in the Education Sector. Available at: https://www.undrr.org/publication/comprehensive-school-safety.
Wijethunga, J., and Piyasiri, S. (2012). Hazard profiles of Sri Lanka: Tsunami. Available at: http://www.dmc.gov.lk/hazard/hazard/Report.html.
Wijetunge, J. (2010). Assessment of potential tsunamigenic seismic hazard to Sri Lanka. Int. J. Disaster Resil. Built Environ. 1 (2), 207–220. doi:10.1108/17595901011056659
Wijetunge, J. (2012). Nearshore tsunami amplitudes off Sri Lanka due to probable worst-case seismic scenarios in the Indian Ocean. Coast. Eng. 64, 47–56. doi:10.1016/j.coastaleng.2012.02.005
World Bank Group (2019). GLOSI the global library of school infrastructure GPSS. Avilable at: https://gpss.worldbank.org/en/glosi/library.
Keywords: tsunami school exposure, tsunami engineering rapid visual survey, Sri Lanka tsunami, Indian Ocean tsunami 2004, school typologies, school tsunami vulnerability
Citation: Cels J, Rossetto T, Dias P, Thamboo J, Wijesundara K, Baiguera M and Del Zoppo M (2023) Engineering surveys of Sri Lankan schools exposed to tsunami. Front. Earth Sci. 11:1075290. doi: 10.3389/feart.2023.1075290
Received: 20 October 2022; Accepted: 07 February 2023;
Published: 20 February 2023.
Edited by:
Anawat Suppasri, Tohoku University, JapanReviewed by:
Prasanthi Ranasinghe, Lanka Hydraulic Institute Ltd., Sri LankaPanon Latcharote, Mahidol University, Thailand
Copyright © 2023 Cels, Rossetto, Dias, Thamboo, Wijesundara, Baiguera and Del Zoppo. This is an open-access article distributed under the terms of the Creative Commons Attribution License (CC BY). The use, distribution or reproduction in other forums is permitted, provided the original author(s) and the copyright owner(s) are credited and that the original publication in this journal is cited, in accordance with accepted academic practice. No use, distribution or reproduction is permitted which does not comply with these terms.
*Correspondence: Jonas Cels, jonas.cels.19@ucl.ac.uk; Tiziana Rossetto, t.rossetto@ucl.ac.uk