Taxonomic and Functional Diversity of Aquatic Macroinvertebrate Assemblages and Water Quality in Rivers of the Dry Tropics of Costa Rica
- 1Ciencias Naturales, EARTH University, San José, Costa Rica
- 2Escuela de Biología, Universidad de Costa Rica, San José, Costa Rica
- 3Escuela de Producción, Universidad Nacional de Río Negro, Bariloche, Argentina
- 4Centro de Investigación en Ciencias del Mar y Limnología, CIMAR, and Centro de Investigación en Biodiversidad y Ecología Tropical, CIBET, Universidad de Costa Rica, San José, Costa Rica
One of Costa Rica’s driest areas is the province of Guanacaste, in the Pacific Northwest, with almost no rain during the dry season from November to April. Due to this marked seasonality, the area is covered by dry tropical deciduous forest, considered the most threatened and least known tropical ecosystem in this area. This study analyzes and characterizes the assemblages of aquatic macroinvertebrates in water bodies within the Tempisque basin. Biological water quality was measured using the BMWP′-CR index. Macroinvertebrate assemblages were analyzed using abundance, richness, and functional feeding group approaches (FFG). Partial least square (PLS) analyses were performed, and the relationships between environmental factors and macroinvertebrate assemblages are also discussed. Macroinvertebrate assemblages were dominated numerically by mayflies, caddisflies, flies, and beetles. The BWMP′-CR index showed varying biological water quality, ranging from “very bad” to “excellent,” depending on rainfall and site management. Results suggest that tropical Mesoamerican rivers contradict the “river continuum concept” because predators and scrapers displace shredders in numbers. On the other hand, the study area shows a notable high richness of the Coleoptera genera. The class Rhynchocoela (Nemertea) is reported for the first time in Central America. The results indicate that the dry forest river ecosystem shows staggering biodiversity despite the surrounding agricultural land use, probably because of their older origin concerning tropical rain forests in Central America.
Introduction
Located in southern Central America, between Nicaragua and Panamá, Costa Rica has a wide variety of biogeographical features, making it part of the Neotropical region, but still receiving some Nearctic influence. Due to this isthmic and intercontinental location, and despite its small terrestrial surface of just 51,180 km2 (0.03 per cent of the surface of the planet), Costa Rica is estimated to possess about five per cent of the world biodiversity (Obando, 2002; Valerio, 2006), with so far almost 200,000 species described (Avalos, 2019). The percentage of described species in Costa Rica may vary drastically depending on the taxonomic group, varying from 100% for vertebrates to only 0.14–0.19% for insects (Avalos, 2019). Especially remarkable is its species density (number of species per surface area; Obando, 2002); using this measure, Costa Rica could probably occupy first place in the world (Obando, 2002, Obando, 2007; Valerio, 2006). For these reasons, Costa Rica is considered one of the 20 countries with the most biodiversity in the world (Mittermeier et al., 2004).
The country’s position between two oceans and a central mountain system crossing from northwest to southeast results in high environmental heterogeneity levels and influences rainfall distribution patterns, ranging from only 600 mm of rain per year to over 7,000 mm (Herrera, 2016). One of Costa Rica’s driest areas is Guanacaste, in the Pacific Northwest, with almost no rain during the dry season from November to April. During the rainy season, many rivers cause floods, while during the dry season, many dry up completely (Arrieta Chavarría, 1999). Due to this marked seasonality, this area is covered mainly by dry tropical deciduous forest and forms part of a continuous biome that stretches from Northwest Costa Rica to the southern fringes of the Mexican state of Sinaloa along the Pacific coast (Jiménez et al., 2016). Other vegetation types throughout the country include moist forest, wet forest, rain forest, cloud forest, and páramo (alpine vegetation). However, the semi-arid area of Costa Rica deserves special attention since the tropical dry forest is considered the most threatened and least known tropical ecosystem (Janzen and Hallwachs, 2016). In the past, this ecosystem has suffered intensive logging for livestock and agricultural expansion, often using fires to remove native vegetation and control natural succession. Although the research group of Daniel Janzen has extensively studied terrestrial dry tropical environments during the past 5 decades in Guanacaste, the aquatic environments are least known.
As an essential component of freshwater biodiversity (Macadam and Stockan, 2015), aquatic macroinvertebrates have received special attention from researchers and stakeholders interested in biomonitoring and environmental studies during the past decades. This situation is authentic for Latin America, including Costa Rica, and the state of knowledge on aquatic macroinvertebrates for the country has been summarized by Springer et al. (2014). Some analyses have already been made in the Guanacaste region concerning freshwater macroinvertebrates in human-impacted and natural areas.
One of these studies concerns macroinvertebrates in Palo Verde’s wetlands (Trama et al., 2009). This essential wetland in this region was studied using artificial substrates. The study found the presence of 18 orders and 57 families. The highest abundances were represented by the Conchostraca and the Chironomidae (Insecta: Diptera), representing almost half of all specimens collected. Interestingly, the number of individuals and species was higher at lower water levels (dry season). A second analysis was done by Rizo-Patrón et al. (2013), where macroinvertebrate communities were used as bioindicators of water quality in conventional and organic irrigated rice fields in Guanacaste. Macroinvertebrate and insect mean abundances were both higher in organic than in conventional treatments. Fourteen orders and 39 macroinvertebrate families were found in the organic rice, whereas only ten orders and 30 families could be found under conventional farming. It was also found that several genera of macroinvertebrates could be used as good bioindicators of water quality.
In the last decades, the traditional rice production in this area has been switching to sugarcane, and sugarcane production has increased in Costa Rica. According to LAICA (Industrial Agricultural Sugarcane League), the number of independent sugarcane growers has grown from six thousand, at the beginning of the ‘90s, to twelve thousand in 2008. The province of Guanacaste alone produces around 50% of the total national production, and the increase of these sugarcane plantations in Guanacaste has also raised pressure on the watersheds, especially the Tempisque basin, the second largest watershed in Costa Rica. Extensive sugarcane plantations are located along riverbanks, like the Cañas, Colorado, Las Palmas, and the lower basin of the Tempisque river. Although many studies have been carried out in Costa Rica on freshwater macroinvertebrates (Springer et al., 2014), little is known from dry tropical rivers within agricultural landscapes, especially under sugarcane production.
Therefore, this study aimed to characterize the assemblages of freshwater macroinvertebrates in a rural agro-environment within the lower Tempisque basin, especially the Colorado and Las Palmas rivers. For this purpose, individual abundance, taxa richness, and functional feeding groups analyses and correlations with environmental variables, were considered. Water quality was determined through the biotic index BMWP-CR (“Biological Monitoring Working Party”, modified for Costa Rica) based on the families of macroinvertebrates found at each site (MINAE, 2007). Finally, the ecological assemblages and diversity of aquatic macroinvertebrates in the Tempisque basin were analyzed and compared with other tropical dry forest localities in Mexico (Ruiz-Picos et al., 2016) and Panama (Sánchez-Argüello et al., 2010a) and a similar study from a tropical rain forest river at the Caribbean slope from Costa Rica (Kohlmann et al., 2015).
Materials and Methods
Study Area and Period
This study was conducted between November 2009 and July 2010 within the Tempisque basin (Figure 1) in Guanacaste province, Costa Rica. The rainfall pattern is typical for this region, with no rain from December to March, then a rainy season starting in April and ending in November, with a typical bimodal rain peak in June and October or a unimodal peak in July-August during a “La Niña” event (Brenes and Bonilla, 2012). The Tempisque river begins its flow (as the Tempisquito) at the Orosí volcano’s foothills and is the third-longest one in the country (approx. 144 km in length). It drains from the Guanacaste Cordillera at an elevation of approximately 900 masl towards the north-western Pacific lowlands of the Guanacaste province, flowing into the Gulf of Nicoya. It has a watershed covering approximately 3,354 km2 (IMN, 2011) and represents its second-biggest basin after the Grande de Térraba. The Tempisque basin is divided into three sections: the upper reaches, covered with forests, located in the Volcanic Cordillera of Guanacaste; the middle reaches, represented by an undulating terrain dedicated to agriculture between Liberia and Cañas; and the lower reaches, composed of plains and marshes subject to flooding in many areas.
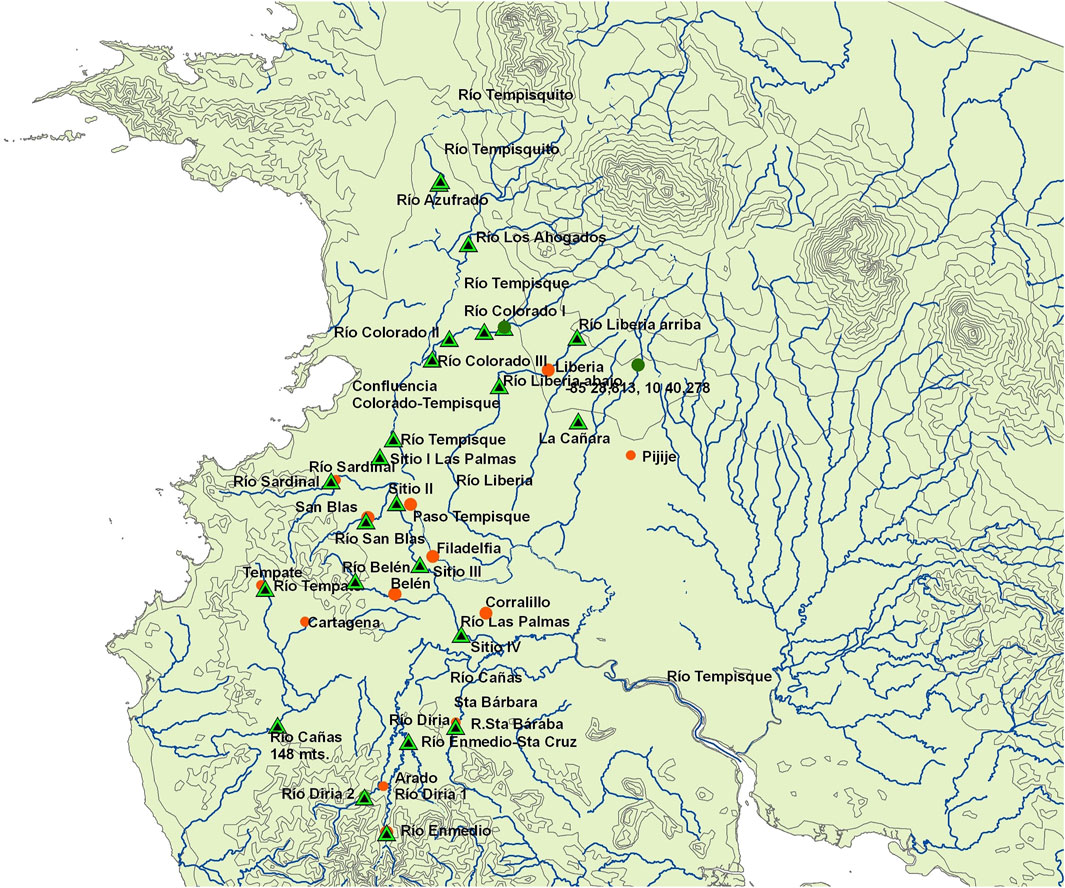
FIGURE 1. Map of the Tempisque basin study area showing rivers, towns (orange circles), and collecting areas (black-green triangles).
The Tempisque river has 36 km that are navigable for small ships and boats at its lower reaches and used to be the primary transportation system before the construction of the Interamerican Highway around 1960; the Spanish conquerors already used it as an invasion route for subjugating the local Chorotega cultures (Peters, 2001). The name of the river, Tempisque, is a word of Nahuatl origin for a tree (Sapotaceae, Sideroxylum capiri), meaning “templi” or “river border” and “ixqui” or “guardian” or “caregiver” (Peters, 2001). The underlying geology is mainly represented by quaternary sediments with Miocene-Pliocene volcanic rocks in its northern fringes, with Cenozoic-Mesozoic sedimentary rocks in its central part areas with ophiolites in its southern fringes (Alvarado and Cárdenes, 2016). Sugarcane, rice, melon, and watermelon plantations have been developed on this watershed and extensive livestock production. Sugarcane has been a continuous activity that was started already in the XVI century by the Spanish conqueror Pedrarias Dávila (Mateo-Vega, 2001). The study area is characterized by a dry tropical climate with mean annual temperatures ranging from 26 to 28°C, annual average evapotranspiration values ranging from 1,800 to 1,900 mm, and annual precipitation averages ranging from 1,500 to 2,000 mm with a pronounced dry season (IMN, 1985). The Tempisque and Las Palmas rivers are so prone to flooding that Arrieta Chavarría (1999) compiled the inhabitants’ stories about these events.
This study was centered on two rivers within an agricultural landscape, the Colorado river (Figure 2) and the Las Palmas river (Figure 3), with three and four sampling sites, respectively. These two rivers were sampled monthly during the whole collecting campaign. Also, 17 additional sites at 15 other rivers of the Tempisque basin were sampled once during the same study period to obtain general information on this area’s water quality. These rivers with the month and year of collection in parentheses are Azufrado (05/10), Belén (02/10), Cañas (02/10), Diriá (05/10), Enmedio (05/10), La Caraña (05/10), Liberia (05/10), Los Ahogados (03/10), Pijije (04/10), San Blas (11/09), Santa Bárbara (05/10), Sardinal (11/09), Tempate (05/10), Tempisque (05/10), and Tempisquito (03/10). All these localities are indicated in the map presented in Figure 1.
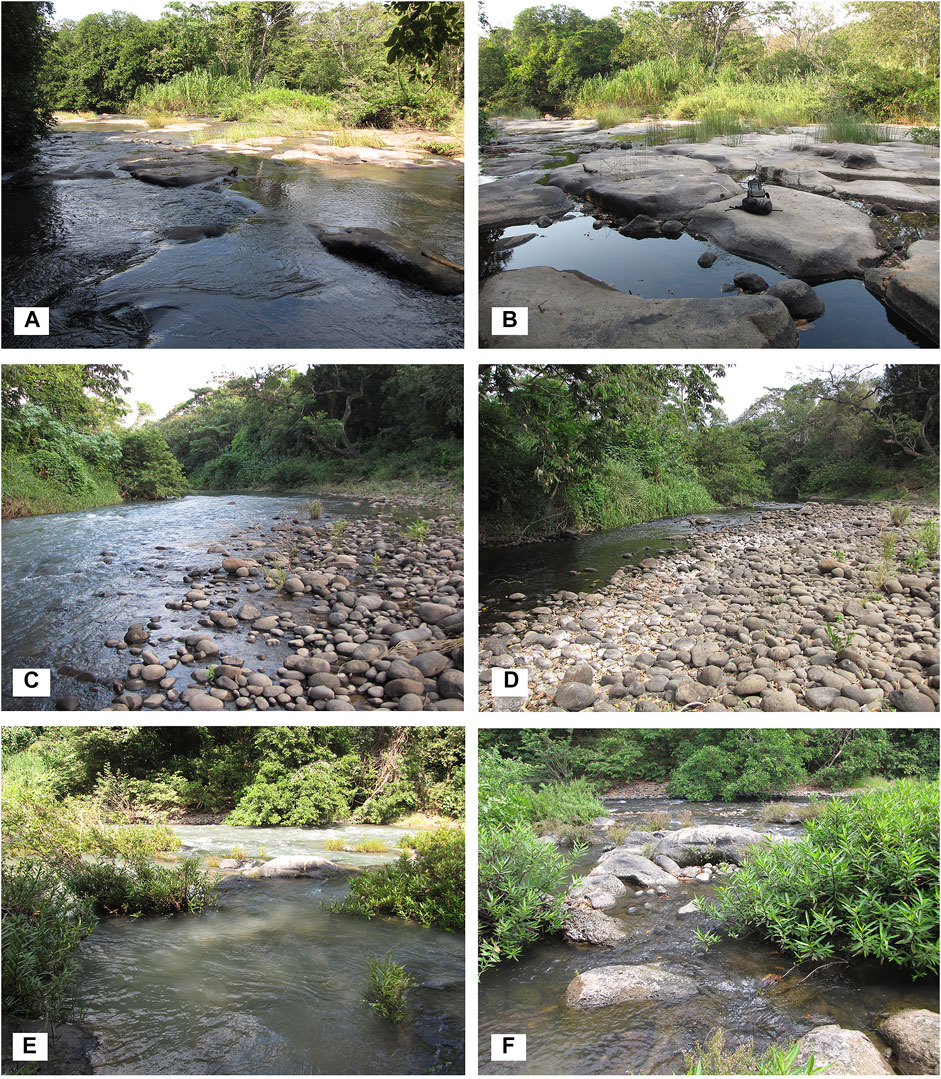
FIGURE 2. Colorado river (A) Site-1 November 2009; (B) Site-1 February 2010; (C) Site-2 November 2009; (D) Site-2 February 2010; (E) Site-3 November 2009; (F) Site-3 February 2010. [(A–F): from left to right and from above to below].
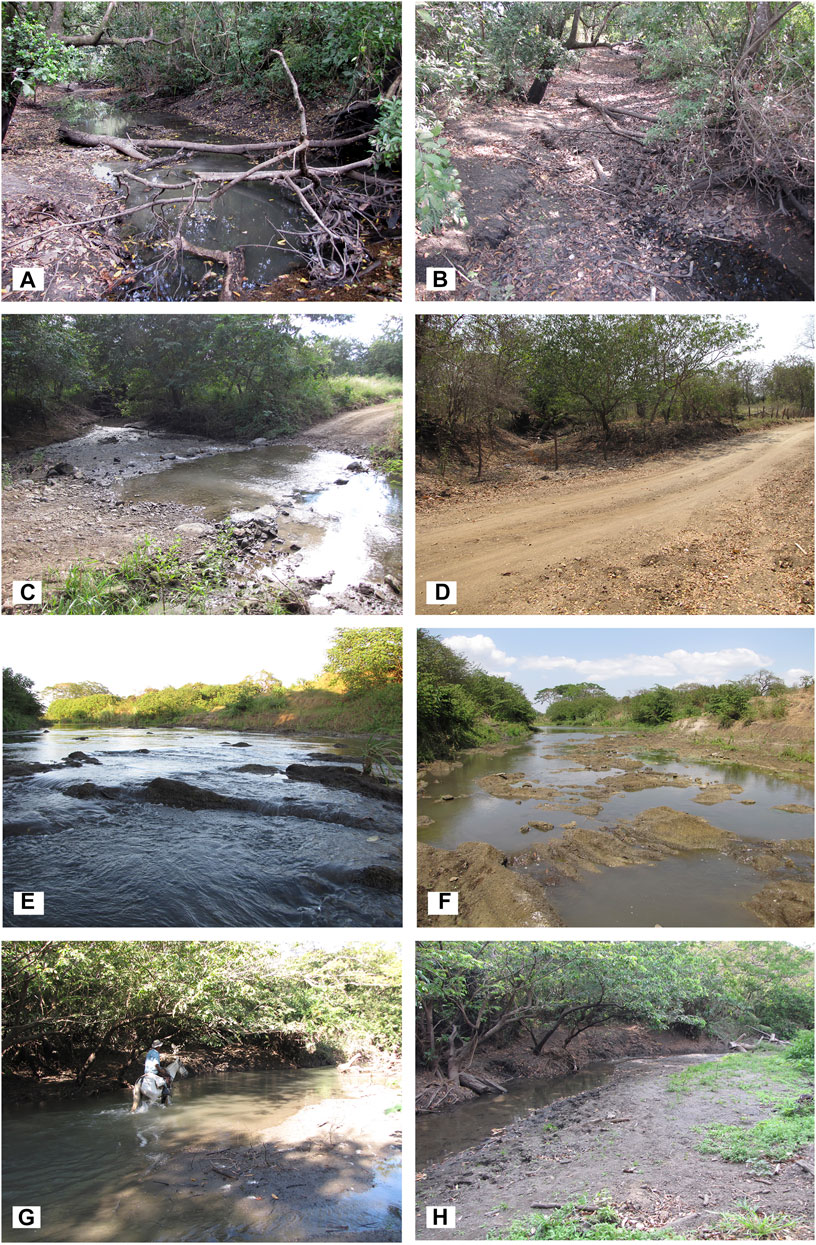
FIGURE 3. Las Palmas river (A) Site-1 December 2009; (B) Site-1February 2010; (C) Site-2 December 2009; (D) Site-2 February 2010; (E) Site-3 December 2009; (F) Site-3 February 2010; (G) Site-4 December 2009; (H) Site-4 February 2010 [(A–H): from left to right and from above to below].
Colorado River
The Colorado river begins at the Rincón de la Vieja volcano’s foothills, at around 800 m altitude, and has a length of 14.8 km before flowing into the Tempisque river. It flows a few kilometres north of the Daniel Oduber International Airport. Once the Colorado river joins the Tempisque, the alluvial valley of the Tempisque river starts. The study area for this permanent river is surrounded by sugarcane and melon plantations. All three study sites have a rocky substrate of different sizes (Table 1) and a mean water temperature of 25°C for July. As shown in Figure 2, the river’s water level can strongly fluctuate in a short period going from November to February, but all sites have permanent water flow. Figure 1 shows the sampling localities.
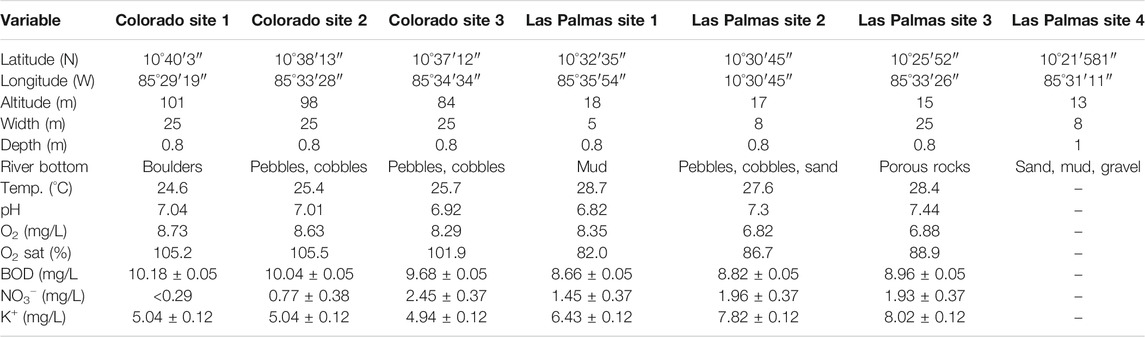
TABLE 1. Geographical, habitat and physical-chemical characteristics at each sampling site of the Colorado and Las Palmas rivers, Guanacaste Province, Costa Rica. Mean values of physical-chemical variables with their standard deviations used for the PLS analysis are included for six collecting sites (July 2010). PO43− is not included in the table and the analyses because it consistently reported a value below the threshold detection limit of < 0.64 mg/L.
The Rincón de la Vieja volcano has been historically active since 1700 (Aguilar and Alvarado, 2020). The volcanic activity is constant but small, with fumes and ashes emitted along the Caribbean and western flanks. It is reported that during 1966 and 1967, the Colorado river changed its colouration under phreatomagmatic activity. The fumes and ashes have acidified the neighbouring rivers, including Colorado, eliminating the original presence of fishes near the volcano (G. Alvarado, pers. comm.). However, the sampling sites (Table 1) did not reflect any acidification during the study period.
Site 1 is characterized by having a river bottom formed of rocks up to 3 m in length. These boulders project themselves over the water level, and it is common to observe plants and shrubs growing on top of them and litter packages accumulating between the rock crevices. A strong current characterizes this site. Several trees of “Sotacaballo” (Fabaceae, Zygia longifolia) project their shade onto the river forming a 15 m barrier on the left bank separating the river from sugarcane on the right margin; a similar vegetation band separates the river from a melon plantation. Site 2 has a bottom formed by small to medium-sized rounded rocks (pebbles and cobbles). Vegetation is also composed of Sotacaballo trees, but taller than at site 1 and with denser tree cover. Site 3 is situated 800 m downstream the confluence with the Santa Inés river. This site also has a rocky substrate surrounded by rich vegetation, where the Sotacaballo is the dominant species. Aquatic vegetation is also present. The main characteristics of the three sites are summarized in Table 1.
Las Palmas River
The Las Palmas river begins at an altitude of 28 m near the town of Nancital and has a length of 13.8 km. Its drainage area covers 400 km2. This intermittent river has a long history of causing floods during the rainy season, most probably caused by its sedimentary nature (Espinoza Rivera, 2018). Sugarcane plantations surround the study area for this river. The study sites vary in the substrate from the muddy bottom of fine sediments to rocky bottom (Table 1) with a mean water temperature of 28°C for July. As shown in Figure 3, the river’s water level can strongly fluctuate in a short period going from December to February, and some sites dried out entirely between February and April. During May, with the beginning of the rainy season, extensive inundations occurred within the whole area, and sampling was impossible in most sites. Sampling localities are indicated in Figure 1.
Site 1 is near the river source, with a small irrigation dam about 500 m upstream and precisely between a sugarcane plantation. There is a protective vegetation barrier of around 15 m at each riverside, giving shadow to the river. The river bottom is muddy. This site went almost completely dry during February, but in March, the site presented high flow due to the surrounding sugarcane plantations’ irrigation activities. In general, the river is almost stagnant and full of organic matter, especially tree trunks and leaf bundles. Site 2 has a rocky substrate (pebbles and cobbles) with sandy areas and is bereft of vegetation because it is a ford for horses, thus receiving the sun’s full impact. This site went dry during February, March, and April and could not be sampled; in May, sampling was also impossible, but this time due to flooding. Site 3 was dredged some months before this study and showed an extensive rocky bottom of porous rocks. The area is used for wading cattle. Shrubs and grasses are the dominant riparian vegetation with a few scattered trees. There are many habitats in this area of the lentic type, algae being commonly present. In March and April, this site presented almost no flow, and pools were the only microhabitat present; in May, sampling was only possible from the riverbank due to the high water level. Site 4 has a bottom formed by sand, mud, and gravel with significant quantities of submerged leaves, branches, tree trunks; secondary vegetation is present along the river margins, with some shade provided by a few trees, the land is covered with sugarcane plantations. In March, sampling was done about 500 m downstream because the original site went completely dry. Due to flooding, this site could not be sampled in May, June, and July.
Macroinvertebrate Sampling, Identification, and BMWP′-CR Index
At each sampling site, a plastic strainer with a 20 cm and 0.5 mm mesh size diameter was used to remove the substrate in the river, and macroinvertebrates were collected directly with tweezers and fixed immediately in 70% alcohol. The main criterion for this semi-quantitative collecting method is the standardized time effort; there were no defined sampling areas. All types of microhabitats present at a particular site were examined equally for macroinvertebrates during a total sampling time of 1 hour at each site. Exact details of the sampling methodology can be found in Stein et al. (2008), Kohlmann et al. (2018a), Kohlmann et al. (2018b), and Maestroni et al. (2018). This same method has been successfully used in a similar study in the Dos Novillos river, from the Caribbean tropical rainforest in Costa Rica (Kohlmann et al., 2015).
All preserved organisms were transported to the laboratory for sorting and taxonomic identification with the aid of a stereomicroscope and specialized taxonomic literature and keys (e.g., Roldán-Pérez, 1996; Merritt et al., 2008; Domínguez and Fernández, 2009; Springer et al., 2010). Doubtful identifications were verified by consulting specialists and recently published neotropical literature (Hamada et al., 2019; Damborenea et al., 2020). All samples are deposited at the Aquatic Entomology Collection of the Zoological Museum of the University of Costa Rica (MZUCR).
A modified “Biological Monitoring Working Party” index for Costa Rica (BMWP′-CR) was used to determine water quality, which is part of the country’s water quality monitoring regulation (MINAE, 2007). The BMWP index was initially developed in 1970 in England as a quick, easy, and cheap method for evaluating water quality using macroinvertebrate families as bioindicators for organic pollution in rivers and streams (Hawkes, 1997). Each family has a previously assigned sensitivity value, ranging from 1 to 10, reflecting tolerance to organic pollution, with lower numbers reflecting high tolerance. Each family’s values found at a given site are summed up to calculate the index, independently from abundance and generic or species richness. For the BMWP′-CR index used in this study, the tolerance values for each family are specified in the respective regulation (MINAE, 2007). Total scores higher than 120 points indicate undisturbed aquatic ecosystems, while low values indicate severe contamination (primarily organic) of the aquatic environment. Based on this index, a biomonitoring field-guide was developed for the Tempisque river basin (Vásquez et al., 2010) as part of the present study.
Physical-Chemical Variables and Data Analysis
The analyzed data comprise the values of the physical-chemical water quality variables (Table 1) (July 2010) and macroinvertebrate assemblage composition during a collecting period of 8 months (November 2009–June 2010). The following physical-chemical variables were measured: temperature, pH, dissolved oxygen, oxygen saturation (%), NO3−, PO4−, K+, and Biological Oxygen Demand (BOD). Potassium was chosen as a research variable because almost nothing is known about this element in Costa Rica. No salinity analyses were undertaken for this study; however, Newbold et al. (1995) analyzed the concentration and export of solutes of six streams that are part of the Tempisque basin and found that Ca2+, Mg2+, and Na+ showed a strong positive correlation with runoff and decreased with streamflow (0.4–2.25 meq/L versus normalized streamflow) in the Tempisquito and Tempisquito Sur rivers and Kathia brook, while K+ (1.38 mg/L for Tempisquito Sur) showed little response to streamflow.
Water samples were also analyzed for multiresidue pesticides by the Research Centre for Environmental Pollution (CICA) of the University of Costa Rica, using liquid-liquid extraction with dichloromethane, followed by gas chromatography in water samples, with a 95% confidence level. The following agrochemicals associated with sugarcane management were tested: Ametryn, Bifenthrin, Bromacil, Cadusaphos, Captane, Cyhalotrin, Cypermethrin, Chlorothalonil, Chlorpyrifos, Deltamethrin, Diazinon, Dichlorvos, Dimethoate, Diuron, Endosulfan α, Endosulfan β, Ethion, Ethoprophos, Fenamiphos, Forate, Imazalil, Isazophos, Malathion, Methyl Parathion, Oxadiazon, Oxyfluorfen, PCNB, Permethrin, Terbuphos, Terbutryn, Tetradiphon, Thiabendazole, Triadimephon, and Triazophos. However, no traces could be detected in the river water samples, although they were taken in July when the collecting campaign’s highest runoff occurred (Figure 4). Finding no residues does not come as a surprise because to monitor pesticides, widespread sampling would be required to detect peak concentrations during pesticide application periods (Liess et al., 2003), whereas low concentrations are very difficult to detect.
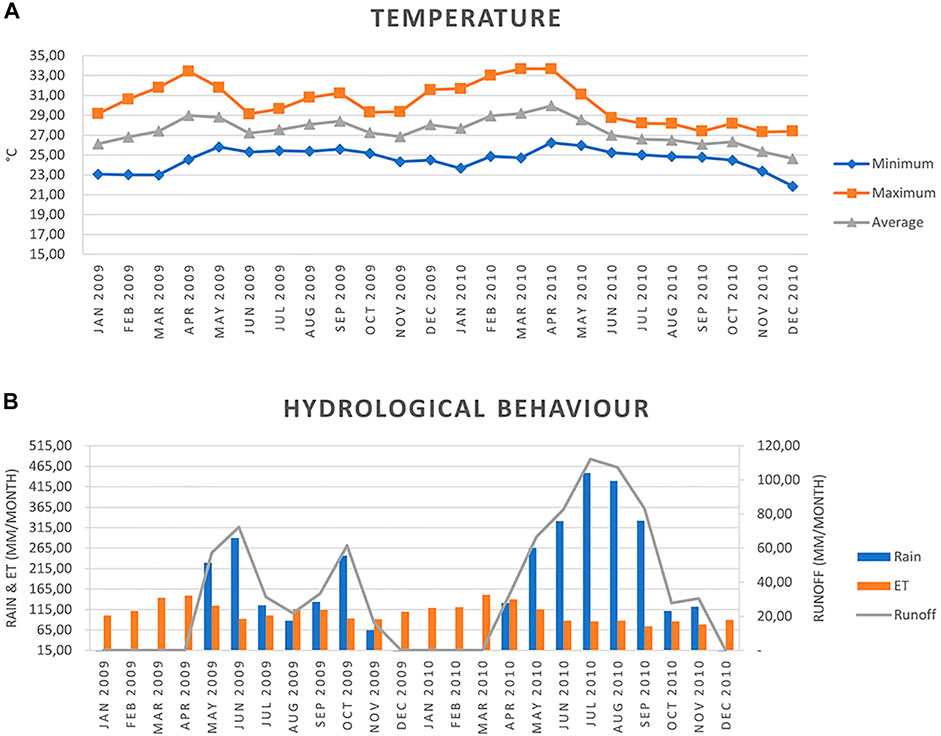
FIGURE 4. (A) Temperature variation and (B) hydrological behaviour (rainfall, evapotranspiration, and runoff) January 2009-December 2010 for the sampled area of the Colorado and Las Palmas rivers (0.5° × 0.5°grid cell at 10.66°N and −85.53°W).
The calculations for the hydrological behaviour (Figure 4) were done using the POWER Project (2020) program from NASA Langley Research Center (LaRC) (https://power.larc.nasa.gov/) using a 0.5° × 0.5° grid cell system at 10.66° N, and-85.53 W. ET was calculated using Hargreaves’s method (Allen et al., 2006), and the runoff followed the method of the United States Service of Soil Conservation (FAO, 1992; Cleves et al., 2016).
Different macroinvertebrate assemblage metrics are considered in this study: relative abundances, taxa richness, and functional feeding groups (FFG). For assigning the FFG groups to each taxon, the classification developed by Ramírez and Gutiérrez-Fonseca (2014) was used. This analysis is based on macroinvertebrates’ two critical aspects: morphological characteristics related to obtaining food resources (e.g., mouthparts and related structures) and behavioural mechanisms (e.g., feeding behaviour). FFG is a valuable tool that provides valuable information on ecosystem functioning, facilitating stream ecosystem comparisons, and avoiding gut content analysis traps, which is more appropriate for assigning trophic guilds (Ramírez and Gutiérrez-Fonseca, 2014). Finally, the correlation of different genera and functional feeding groups with environmental variables to define taxa as bioindicators of specific environmental variables was also analyzed.
Statistical Analysis
Taxonomic grouping and FFG analysis were compared using abundances between sites. To this effect, a simple random sample of individuals of each order was drawn separately from two different rivers (Colorado and Las Palmas). We consider each individual as a unit sample, where n-independent, identical trials (n = 5,607) can have an outcome in any cij category (i = Colorado, Las Palmas; j = 1,...,12 orders or j = 1,..., 6 FFG). The total sample size is fixed, but the row and column totals are not. Like any significance tests, chi-squared tests of independence have limited usefulness and they require large samples. They merely indicate the degree of evidence of association; therefore, we investigated here the nature of the association by using standardized Pearson residuals to identify the lack of independence fit of the taxonomic grouping or the FFG category in relation to the river category. Standardized Pearson residuals that exceed a sample odds ratio of 3 in absolute value indicates lack of independence (Agresti, 2002; p81).
A one-way analysis of variance model was adjusted to identify significant variations between sites in terms of physical-chemical variables, the abundance of macroinvertebrates, and the BMWP′-CR index (ANOVA; α = 0.05). The abundances were transformed by square root to comply with the normality of the error. The one-way ANOVA fixed model assumes homogeneity of variance within the groups it compares. When this assumption was not fulfilled, a heteroscedastic model was adjusted (Pinheiro and Bates, 1996). The selection of the best model was carried out using the Akaike information criterion (AIC) (Akaike, 1973; Burnham and Anderson, 1998; Arroyo, 2008; Zuur et al., 2009). The use of AIC is little taught in statistics classes and is far less understood in the applied sciences than should be the case (Burnham and Anderson, 1998), although recently its use in ecology and ethology has begun to become more general (Salomão et al., 2019; Souza et al., 2020; Lira et al., 2021). When the model detected significant differences, a DGC (Di Rienzo–González–Casanoves) statistical test was performed to compare means (Di Rienzo et al., 2002).
Partial Least Squares (PLS) regression is a technique that combines Principal Component Analysis and Linear Regression (Balzarini et al., 2008). It is applied when desired to predict a set of dependent variables (y), in this case, the abundance of macroinvertebrate genera and FFG abundances and the BMWP′-CR index values, from a set of predictor variables (x), in this case, physical-chemical variables. PLS is particularly suited when the matrix of predictors has more variables than observations (overfitting, as is the case here), and when there is multicollinearity among X values (the case also), as they are usually ordered in environmental gradients (Carrasco et al., 2009). Moreover, Carrasco et al. (2009) consider that this technique is more reliable than others when identifying relevant variables and their magnitudes of influence; it is commonly used in chemistry, but it has been overlooked in ecology, where it is especially appropriate. It has also been successfully applied to morphometrics (Ismail, 2018) and food science (Risoluti et al., 2020). Tobias (1995) considers it a robust form of redundancy analysis (RDA). As Carrasco et al. (2009) (p. 682) indicate: “The applicability of this method will likely be higher for inductive approaches in which the aim is the definition of patterns of variation among large sets of usually related variables.” In this analysis, a Triplot graph was superimposed on a Biplot graph (Gabriel, 1971), correlating all variables. Then, the observations (sites) appear ordered in a Triplot graph, depending on the values of the dependent variables (macroinvertebrate and FFG abundances) and their correlation with the predictor variables (physical-chemical water-quality variables). For the macroinvertebrate genera PLS analysis, out of the 195 collected genera, the multimetric analysis included only the 53 genera collected during July 2010 for the Colorado and Palmas rivers. These 53 genera, composed of 595 individuals, showed high projection values on the first two principal components. All statistical analyses were done using the InfoStat program (Di Rienzo et al., 2020).
Results
Physical-Chemical Parameters
The mean values of the physical-chemical characters are indicated in Table 1. The analysis was done on samples taken during the month with the highest rainfall and runoff values and one of the lowest evapotranspiration rates. Las Palmas river evinced higher temperature (above the mean monthly temperature) and potassium values than the Colorado river. Oxygen concentration and saturation levels, and BOD values are notably higher in the Colorado river than in the Las Palmas river. Nitrate values vary from low to high in the Colorado river, whereas they remain relatively stable in the Las Palmas river. Phosphates remained at low values in all sampling sites. Levels of pH (around 7) did not vary much between both rivers. No traces could be detected in the river water samples for agrochemicals.
Diversity and Composition of Macroinvertebrate Assemblages
The study collected a total of 7,534 specimens. The Colorado river registered 3,223 collected specimens, the Las Palmas river 2,384 specimens, while 1,788 specimens were collected from the other 15 rivers within the Tempisque basin. Collected specimens belong to the following nine classes: Insecta, Arachnida, Malacostraca, Clitellata (Oligochaeta), Rhabditophora (Turbellaria), Gastropoda, Bivalvia, Hirudinea, Rhynchocoela (Nemertea). Within these classes, 76 families and 194 genera were collected from 20 orders (number of specimens in parentheses): Ephemeroptera (2,510), Trichoptera (1,219), Diptera (1,006), Coleoptera (853), Odonata (667), Basommatophora (588), Hemiptera (202), Lepidoptera (106), Megaloptera (71), Sphaeriida (70), Plecoptera (37), Decapoda (19), Lumbriculida (15), Rhynchobdellida (15), Tricladida (10), Trombidiformes (7), Blattodea (1), Eumonostilifera (1), Isopoda (1), Unionoida (1).
The total abundance analysis (Figure 5) shows that Ephemeroptera, Trichoptera, Diptera, and Coleoptera are the most prominent groups, comprising 75.2% of all collected organisms. Ephemeroptera is the most numerous taxa, with almost 34% of all individuals collected, followed by Trichoptera, Diptera, and Coleoptera with 16.5%, 13.6%, and 11.5%, respectively. Figure 6 shows the number of genera per order at each one of the three sampled river systems. The Coleoptera are the most genera-rich group, followed by the Ephemeroptera, Odonata, and Diptera.
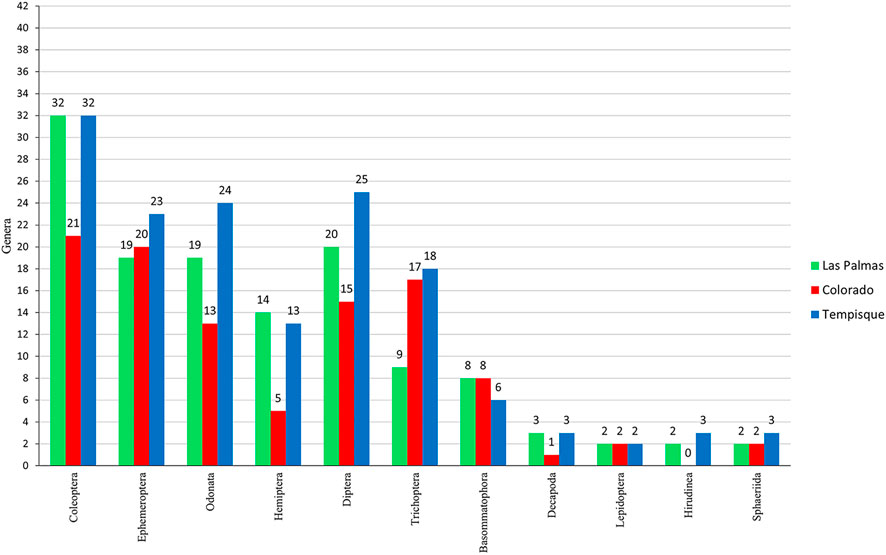
FIGURE 6. Bar-graph is indicating the number of genera per order collected in the three river systems.
Comparisons Between Sampling Sites
The family, genera, EPT richness, mean number of specimens, and mean BMWP′-CR values with resulting water quality for the Colorado and Las Palmas rivers and all other sites within the Tempisque basin are summarized in Table 2. The total family and EPT taxa richness do not show significant frequency differences between sampling sites. An ANOVA analysis was done for the number of specimens and the BMWP′-CR values for the Colorado and Las Palmas rivers. No significant differences were found in both cases. Tempisque localities were sampled only once and, therefore cannot be analyzed using an ANOVA. Water quality was regular in the Colorado and Las Palmas rivers, whereas it varied from bad to excellent in the Tempisque basin depending on the locality. Figure 7 depicts the different BMWP′-CR values for the different sampled localities in the Tempisque Basin. As can be seen, the BMWP′-CR values vary throughout the year for the same localities of the Colorado and Las Palmas rivers, ranging between regular and excellent and between very bad and excellent, respectively. Colorado showed overall better quality since all sites reached excellent water quality at least during one sampling event according to the BMWP′-CR index.
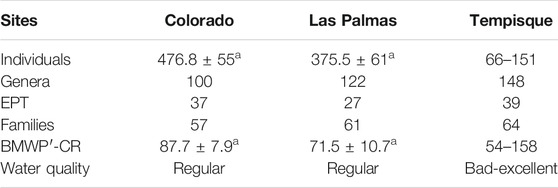
TABLE 2. Mean number of individuals, total number of genera, EPT genera (Ephemeroptera + Plecoptera + Trichoptera), total amount of families, and mean values for the BMWP′-CR index with resulting biological water quality in the different collecting sites. Means in the same row with the same lettering are not significantly different (ANOVA, homocedastic model, DGC-Test, p > 0.05). Tempisque localities were sampled only once and cannot be analyzed using an ANOVA.
Evident changes in the abundance structure of macroinvertebrate assemblages can be observed among the two rivers (Figure 8A). Standardized Pearson residuals show that Plecoptera, Lepidoptera, and others are independent of the river category. The Colorado river shows a very distinct taxonomic structure. Many orders showed non-independence from river category. In the Colorado river, we can cite the highest collected numbers of Ephemeroptera, Trichoptera, Megaloptera, and Diptera. The Las Palmas river is characterized by having the highest numbers of Odonata, Sphaeriida, Basommatophora, Hemiptera, and especially Coleoptera, around fifteen times more than what estimated odds would consider.
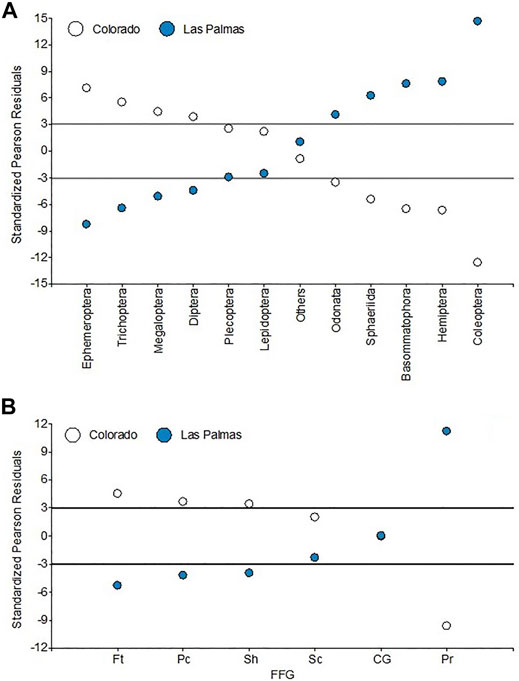
FIGURE 8. Standardized Pearson residuals for the independence tests for (A) orders and (B) FFG. Lines indicate the standardized Pearson residuals 3 and −3.
Functional Feeding Group Analysis
The FFG analysis shows (Supplementary Figure S1) that collector-gatherers are the dominant group at all sites, with around 35% of all individuals collected. They are followed by the predators and scrapers, with each around 20% of all individuals collected, and the filterers at fourth, with around 18% of all individuals collected. Again, there are differences in the different areas’ functioning structures. Standardized Pearson residuals show (Figure 8B) that the Colorado river has the highest numbers of filterers, piercers, and shredders. The Las Palmas river has the highest number of predators, around twelve times more than what estimated odds would consider. Collector-gatherers and scrapers are independent of river category.
The FFG abundance PLS (Figure 9A) presents high variation explanatory values, with factor 1 explaining 60.0% of the total variance and factor 2 explaining 24.4%. The variables potassium and scraper abundances mainly separate the different sites on the horizontal projection, as do the variables pH and piercer and predator abundances on the vertical projection. The FFG most closely allied to the Colorado river sites, and presumably, a good indicator of a lower anthropogenic impact is scraper abundance. On the other hand, the most closely allied variables to the Las Palmas sites and presumably good indicators of anthropogenically impacted areas is the variable predator abundance.
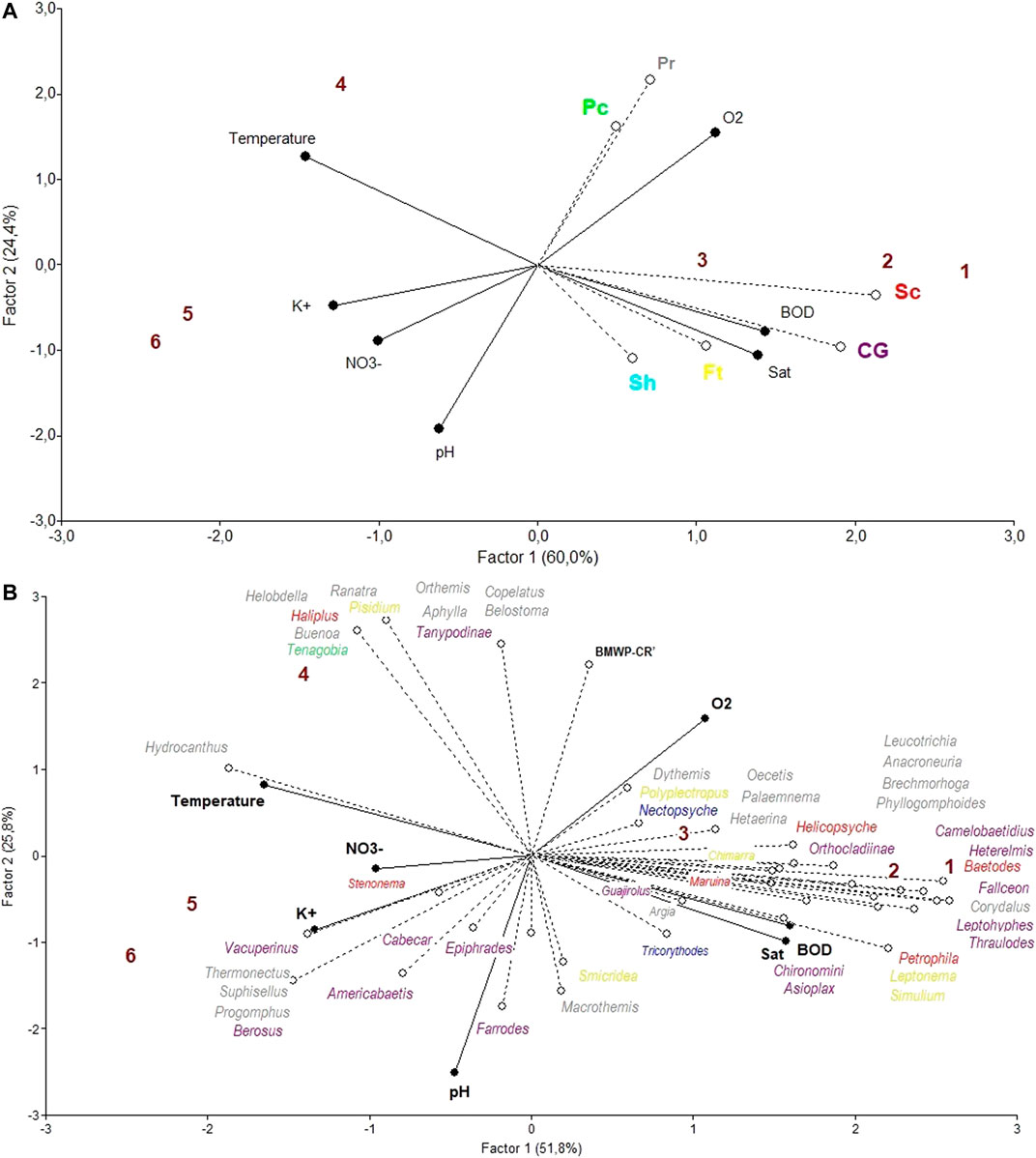
FIGURE 9. Site ordination for the Colorado river (1–3) and the Las Palmas river (4–6) with a triplot PLS analysis using physical-chemical values as independent variables and (A) functional feeding group abundances as dependent variables. CG = Collector-Gatherers, Ft = Filterers, Pc = Piercers, Pr = Predators, Sc = Scrapers, and Sh = Shredders; (B) generic macroinvertebrate abundance and the BMWP′-CR index as dependent variables (FFG categories: purple = collector-gatherers, yellow = filterers, green = piercers, grey = predators, red = scrapers, blue = shredders).
Relationship Between Macroinvertebrate Assemblages, Feeding Group Approaches, Physical-Chemical Parameters, and Sampling Sites.
The macroinvertebrate abundance partial least square (PLS) analysis (Figure 9B) presents high variation explanatory values, with factor 1 explaining 51.8% of the total variance and factor 2 explaining 25.8%. The variables temperature, NO3− and to a lesser degree K+ in one direction, and BOD and oxygen saturation in the other direction, separate mainly the different sites on the horizontal projection, as does pH on the vertical one. The Colorado river localities seem to be associated with higher BOD and oxygen saturation values, whereas the Las Palmas river localities are associated with higher temperature, K+, and NO3− values. The aquatic insect genera Corydalus, Fallceon, Heterelmis, Leptohyphes, and Thraulodes, seem to be most closely related to the Colorado river and could therefore be considered indicators of less human-impacted areas. These genera are all scrapers except for the first, which is a predator. On the other hand, the most closely allied genera to the more agriculturally impacted areas, sites 4, 5, and 6, are the beetle genera Berosus, Hydrocanthus, Suphisellus, Thermonectus, and the dragonfly Progomphus, representing most likely indicators of human-impacted areas. These genera are predators except for the first, which is a collector-gatherer.
Discussion
Physical-Chemical Parameters
The results recorded in this study (Table 1) can be compared with the ambient water quality criteria emitted by the Regulation for the Evaluation and Classification of Water Bodies (MINAE, 2007) for Costa Rica, as general guidelines designed to protect freshwater life, aesthetics, and recreation, which may be expected from the presence of pollutants in any body of water. For temperature, no values are given for the national legislation. The Colorado river’s relatively lower temperature values are probably a consequence of these collecting sites’ higher altitudes and permanent flow. Nitrates are an indicator of nutrient enrichment and a potential human health indicator in drinking water, the reported values < 5 identify a class 1 (no pollution) water quality, meaning the best possible ambient water quality class, ranging on a scale from 1 (best) to 5 (worst). The same applies for the pH values, where class 1 ranges from 6.5 to 8.5, and this range is considered best for fisheries; both rivers fall within this range, although the Las Palmas river deviates more from the value of 7. The Costa Rican regulations use the Dutch System for Evaluating the Physical-Chemical Water Quality for the following environmental parameters, oxygen saturation, BOD, and ammonia. Following this system and according to the oxygen saturation levels, the Colorado river falls in class 1 (no pollution), whereas the Las Palmas river falls in class 2 (incipient pollution), although the Las Palmas values are approaching the class 4 category.
Regarding BOD, which measures the degradation of natural or introduced organic matter into water, the Colorado river falls in class 4 (severe pollution), and the Las Palmas river falls in class 3 (moderate pollution). Potassium, phosphates, and oxygen are not contemplated in the Costa Rican water legislation. In our study, phosphate was always below a detection value of < 0.64 mg/L. Phosphate is essential for plant growth, but too much can damage the river’s ecology, generating eutrophication (Trodd and O’Boyle, 2018). Sewage, industrial discharges, organic and inorganic fertilizers can produce an increase of phosphate in freshwater systems. Average concentrations of < 0.025 mg/L are considered unpolluted waters (Trodd and O’Boyle, 2018). Oxygen, which is a natural characteristic of clean waters, has an inverse relationship with temperature. It is generally considered that oxygen levels below 5 mg/L dangerous conditions for fishes start to set in; below 2 mg/L fish and macroinvertebrate will show great mortality rates. The Las Palmas river has lower oxygen levels than the Colorado river, especially sites 2 and 3, probably due to its higher water temperature levels and stagnant water.
It is interesting to note here the high BOD and oxygen values for the Colorado sites. These sites are surrounded by a lush gallery forest (Figure 2), producing a rain of organic matter into the river. At the same time, there is a slope in the river generating rapids. These two conditions explain the high values for both variables. This situation is contrary to the sites at the Las Palmas river, with almost no slope and much-reduced gallery vegetation (except site 1), dominated by grasses. According to Costa Rican environmental laws, rivers flowing through plantations should be separated from them by 15 m of trees, although this condition can vary in practical terms.
Finally, potassium, a neglected nutrient, does not have a substantial health significance, except at gross levels. Skowron et al. (2018) indicate that potassium concentrations can limit the distribution of larger crustaceans. However, it is an indicator of anthropogenic activities, including agricultural use (Bu et al., 2010). It is usually found in natural surface waters under 5 mg/L (Skowron et al., 2018) and between 2 and 3 mg/L in rivers (Lenntech, 2020); the values found in both rivers are higher than that, indicating the use and pollution of freshwater by artificial fertilizers with the Las Palmas river showing higher potassium values than the Colorado river (Table 1). Potassium can be used as an indicator of surface runoff because it is very soluble and can be washed by rainfall into water bodies depending on rainfall intensity and unlike nitrogen, there are no gaseous losses of potassium (Alfaro et al., 2008; Alfaro et al., 2017; Goulding et al., 2020). This potassium runoff is exceptionally high in tropical regions (Acharya et al., 2007). Considering that the Las Palmas river area is much less covered by vegetation, this suggests a more significant agricultural runoff during rainfall and higher potassium values found in this river.
In general, the Las Palmas river evinces more pollution categories (6) than the Colorado river (1), reflecting a more intense agricultural land use impact. The Colorado river’s permanent flow could also positively impact its water quality due to a dilution effect, contrary to the intermittent Las Palmas river, which reduces its flow considerably during the dry season.
Diversity and Taxonomic Composition of Macroinvertebrate Assemblages
The diversity of macroinvertebrates obtained during this study for the Tempisque watershed, with nine classes, 20 orders, 76 families, and 194 genera, seems remarkably high if compared with other studies. A similar analysis, undertaken in a rainforest river basin of the Costa Rican Caribbean slope, the Dos Novillos, which used the same sampling method, although during a more extended sampling period (2 years versus 6 months), recorded six classes, 16 orders, 53 families, and 101 genera (Kohlmann et al., 2015). A bar graph analysis of order abundance between the Tempisque and Dos Novillos basins (Figure 10) indicates differences between the two systems, where the absence of Sphaeriida at the Dos Novillos is notorious. The present results also compare well with the diversity found at a similar watershed that ranges from pristine to human-affected areas, the Capira basin on the Pacific coast of Panama, also having a mixed evergreen and a deciduous tropical forest, where Sánchez-Argüello et al. (2010a) reported the existence of 17 orders, 69 families, and 120 genera. Another similar comparison can be made with the results reported by Ruiz-Picos et al. (2016) for two sub-watersheds, Apatlaco and Chalma-Telmembe, part of the Balsas watershed along the Mexican Pacific coast. The river systems flow through pristine to human-impacted areas, covering caducifolious, conifer, and oak forests, and registered 21 orders and 66 families.
This high diversity in rivers from tropical dry areas could have a variety of possible explanations. One is the possible origin of this ecosystem. The dry tropical forest is considered an old type of ecosystem that originated in Mexico’s northwest area during the Miocene (Axelrod 1975, Axelrod 1979), expanding into Central America from Mexico around 2.5 Mya (Graham and Dilcher, 1995; Becerra, 2005). Simultaneously, the tropical rainforest is considered a recent invasion from South America into the Central and North America area, dating around 10,000 years ago (Toledo, 1982; Colinvaux, 1997). Kohlmann and Halffter (1990) have shown, in the case of the genus Canthon (Coleoptera: Scarabaeidae) and related genera of dung beetles in Mexico, that speciation processes in these older Miocene ecosystems resulted in much higher biodiversity than the one found from the recently arrived South American ecosystems, like the tropical rainforest. This situation could account for this difference in the diversity collected in Costa Rica, where dry tropical forests already had an older established history than the tropical rainforest (Colinvaux, 1997). A second possible reason for this high diversity could lay that many dry forest rivers are intermittent and change from flowing to stagnant conditions, allowing different taxa to establish during different times of the year.
Comparison Between Sampling Sites
The macroinvertebrate abundance analysis (Table 2) did not show any significant differences, probably because the Colorado and Las Palmas rivers are both under anthropogenic impact. Studies by Paaby et al. (1998), Lorion and Kennedy (2009), and Kohlmann et al. (2015) have registered greater macroinvertebrate abundances in pristine Neotropical environments than in sites under the human impact, although Sánchez-Argüello et al. (2010a) have found the opposite.
There is no statistically significant difference between the Colorado and Las Palmas rivers in the number of families and genera. However, the Tempisque basin is significantly different at the generic level, probably due to a greater collecting area with greater habitat diversity (higher number of different rivers) and a lower anthropological impact effect. Sánchez-Argüello et al. (2010a) have found that pristine areas have the highest taxonomic richness. This richness can be appreciated in Figure 6, where the Tempisque basin shows greater or equal richness in genera.
The EPT taxa richness did not show significant differences, although there seems to be a declining gradient from the least impacted area (Tempisque) to the most impacted one (Las Palmas), where the numbers dropped from 39 to 27. A similar trend was reported by Lorion and Kennedy (2009) for rivers from the Caribbean lowlands of Costa Rica, following a forest-forest buffer - pasture gradient. An analogous tendency in EPT taxa richness was also observed by Kohlmann et al. (2015), going from a forest-pasture-village-banana gradient, with EPT values diminishing from 14 to 9.
The Colorado river showed excellent BMWP′-CR values during the dry season and regular water quality values during the rainy season, whereas the Las Palmas river varied considerably from site to site and between seasons. The Colorado river results are in line with the results found by Sánchez-Argüello et al. (2010b) in Panama, where the runoff produced by the rainy season ends up changing the chemistry and polluting the rivers, therefore lowering the BMWP′-CR values. The Colorado river results also agree with the study by Kampa et al. (2000), where runoffs of anthropogenic origin affect the biological quality of water and the river capacity for self-purification. Finally, macroinvertebrate densities and taxonomic richness get diluted during the rainy season (Ramírez and Pringle, 2001; Sánchez-Argüello et al., 2010a). However, local agronomic practices can impact this clear distinction, as the Las Palmas river results suggest.
Another possibility is the hydrological differences between both rivers; whereas the Colorado river maintains a continuous, albeit low, water flow during the dry season, the Las Palmas river tends to form isolated ponds that concentrate pollutants. Although no chemical water analyses were done during the dry season, both rivers’ taxonomic composition might shed some light (Figure 8A) in this situation. The Las Palmas river has the highest abundance for Coleoptera, Basommatophora, Hemiptera, Sphaeriida, and Odonata. In contrast, the Colorado river has the highest abundance for Ephemeroptera, Trichoptera, Mehaloptera, and Diptera, and the lowest abundance values for Coleoptera. Sánchez-Argüello et al. (2010b) record a total absence of mollusks in a pristine environment while registering high abundances in human-impacted ones, as is the case with the Las Palmas river, thus suggesting high biological pollution in this river.
On the other hand, the high Coleoptera and Hemiptera abundances in the Las Palmas river correlate well with the existence of confined water ponds that form in the Las Palmas river during the dry season, and these good flying groups can quickly colonize them. These Coleoptera abundances are supported by the fact that instead of pertaining mostly to Elmidae, as reported in other studies (Sánchez-Argüello et al., 2010a; Kohlmann et al., 2015), the majority of the Coleoptera in the present study belong to the Dytiscidae and Hydrophilidae families. Akünal and Aslan (2017) found that hydrophilids thrived well in river waters with high temperatures (28–45°C) in western Turkey, possibly a reflection that higher water temperatures may allow more rapid completion of larval stages (Fairchild et al., 2003). This information coincides because the Las Palmas river sites’ mean temperatures are 2–3°C higher than those from the Colorado sites. On the other hand, Burghelea et al. (2011) indicate that Dytiscidae is a common colonizer of natural and managed aquatic environments. These studies seem to support the observed behavior of the Dytiscidae and Hydrophilidae as rapid colonizers of isolated aquatic environments.
Functional Feeding Group Analysis
FFG relative abundances change depending on human impact conditions on the river. Starting with less impacted areas, such as the Tempisque basin in general, one can observe that collector-gatherers tend to be more numerous (Supplementary Figure S1). In the second place, under more anthropologically impacted conditions, such as in the Colorado river (Figure 8B), filterers and scrapers tend to be dominant, whereas predators diminish. Lastly, under more disturbed conditions, such as at the Las Palmas river, piercers and shredders attain their lowest numbers. Comparing these results with a similar one done for the Dos Novillos river on the Caribbean coast of Costa Rica (Kohlmann et al., 2015), similar results were reported. For instance, predators and scrapers had higher abundances, and collector-gatherers and piercers lower abundances in the more organically polluted localities, whereas more pristine environments had greater piercer and lower filterer abundances. Another study, conducted by Granados-Ramírez et al. (2017) in the upper region of the Balsas basin on the Mexican Pacific coast, an area encompassing pristine to human-impacted areas, also found similar results with those obtained for the Tempisque basin. The highest percentages were recorded for collector-gatherers and predators in both studies, while shredders and piercers obtained only around or less than 5%. It would seem then that the dry tropical forest’s aridity environment has no influence over the functional feeding groups’ percentages, but long-term studies, especially from sites with none or little human impact, would be necessary to confirm this statement.
Regarding community composition and according to the seminal paper of Vannote et al. (1980) on “the River Continuum Concept”, they propose the following statements regarding the general macroinvertebrate functional groups–shredders, collectors, scrapers (grazers), and predators–in temperate areas. Vannote et al. (1980) conceptualize that scrapers’ dominance follows shifts in primary production, maximized in mid-sized rivers. It is hypothesized that shredders are codominant with collectors in headwaters, reflecting the riparian zone’s importance. As a stream increases in size and a general reduction in detrital particle size sets in, collectors should increase in importance, becoming dominant in large rivers. Finally, predators change little in relative dominance with stream order. This situation is not the case for tropical rivers in Mesoamerica, as seen in this study and Kohlmann et al. (2015). As predicted, collectors maintain dominance in the community assemblages, but no further similarities were found with the River Continuum Concept. Predators become an influential group, shredders do not attain any importance, and scrapers vie with predators for a second-place dominance. The dominance of collectors and scrapers can result from the presence of different pollution sources, as reported by Van Echelpoel et al. (2018). As pointed out by Wantzen and Wagner (2006), the low abundance of shredders in tropical streams and rivers has been reported by various authors. It seems, therefore, that the functional group assemblage in tropical forests is different from the one hypothesized by Vannote et al. (1980) for temperate rivers, where predators seem to play here a much more critical role than shredders and compete against scrapers for dominance. Other studies from neotropical rivers have also reported that, in general, predators are highly abundant, while shredders are only limitedly present (Van Echelpoel et al., 2018). Predation would seem to be an important driving force in tropical rivers.
Conclusion
The present study shows that macroinvertebrate abundances, taxonomic structure, and functional feeding groups are invaluable tools for taking the pulse of freshwater community assemblages. Interesting is to mention the enormous diversity of Coleoptera at the genus level at more anthropologically impacted conditions, which also have higher water temperatures and form isolated stagnant pools during the dry season.
The study shows considerably high taxonomic richness, especially at the family and genus level, probably derived from the fact that tropical dry forest colonized the area, expanding into Central America from Mexico around 2.5 Mya. In contrast, the tropical rainforest is a recent newcomer, only 10,000 years old. The intermittent nature of rivers and streams in the dry tropical forest could also partially explain this remarkable diversity. Unexpected discoveries were made, such as the first record of Prostoma, the first freshwater nemertean to be reported for the Central American region, suggesting that much of this region’s biodiversity is still waiting to be discovered.
The results found in dry tropical forests and tropical rain forests of Costa Rica contradict the “River Continuum Concept” of Vannote et al. (1980). This model hypothesizes for temperate rivers that shredders and collectors are codominant in rivers, whereas predators maintain a low profile. This situation is not the case in Mesoamerican tropical rivers, where collectors are certainly dominant, but predators and scrapers vie for second place in dominance, and shredders have low abundance importance. Filterers, shredders, and piercers are an important constant in tropical Mesoamerican rivers. Further studies are necessary for rivers from protected tropical dry areas with little or no anthropogenic influence to confirm these findings.
The use of the BMWP′-CR index revealed anthropogenic impacts on different sites and sampling dates. This method evinced changes in macroinvertebrate assemblages associated with anthropologically impacted areas, even when analytical methods could not detect the presence of pesticides in river water in the present study. As expected, the BMWP′-CR index had high scores towards the different rivers’ headwaters and then started a downstream decline. Besides the growing anthropogenic impact, this could also be the effect of a longitudinal gradient in macroinvertebrate composition or a downstream decline in taxon richness as described by Vannote et al. (1980) and Connolly et al. (2007). This situation opens new research questions, especially for freshwater ecosystems in tropical dry regions, which have been underrepresented in tropical freshwater research.
The use of PLS analyses represents a powerful tool for studying ecological data. This technique is more reliable than others when identifying relevant variables and their magnitudes of influence (Carrasco et al., 2009); it is commonly used in chemistry, but it has been overlooked in ecology, where it is especially appropriate. As Castillo et al. (2006) and Rizo-Patrón et al. (2013) have found, multivariate analysis is a more sensitive tool for detecting human impacts than toxicity tests. Therefore, multivariate analyses should be incorporated as an approach for future analyses of tropical freshwater systems.
Data Availability Statement
The raw data supporting the conclusions of this article will be made available by the authors, without undue reservation.
Author Contributions
All authors conceived, wrote, edited, reviewed, and approved this manuscript. DV performed field sampling, sorting, taxonomic identification, and took the pictures of the organisms; AA performed statistical analyses, and BK drafted the first manuscript.
Funding
This material is based upon work supported by the Department of Energy (National Nuclear Security Administration) under Award Number DE-FG36-08GO88044.
Conflict of Interest
The authors declare that the research was conducted in the absence of any commercial or financial relationships that could be construed as a potential conflict of interest.
Publisher’s Note
All claims expressed in this article are solely those of the authors and do not necessarily represent those of their affiliated organizations, or those of the publisher, the editors and the reviewers. Any product that may be evaluated in this article, or claim that may be made by its manufacturer, is not guaranteed or endorsed by the publisher.
Acknowledgments
Temperature and hydrological data were obtained from the NASA Langley Research Center (LaRC) POWER Project funded through the NASA Earth Science/Applied Science Program (https://power.larc.nasa.gov/). We are also in debt with Eng. Gregory Guevara, for his help in calculating the temperature and hydrological variables presented in this study. We thank Ashley Salazar, librarian at EARTH University, for her tireless work in finding literature wholeheartedly. Jeffrey Sibaja from the University of Costa Rica confirmed the first identification of the ribbon worm for Costa Rica as a Nemertean.
Supplementary Material
The Supplementary Material for this article can be found online at: https://www.frontiersin.org/articles/10.3389/fenvs.2021.660260/full#supplementary-material
Supplementary Figure S1 | (A) Percentage abundances of the most common taxonomic groups at the three different sampling areas. (B) Percentage abundances of the functional feeding groups (FFG) at the three different sampling areas. CG = Collector-Gatherers, Ft = Filterers, Pc = Piercers, Pr = Predators, Sc = Scrapers, and Sh = Shredders.
References
Acharya, G. P., McDonald, M. A., Tripathi, B. P., Gardner, R. M., and Mawdesley, K. J. (2007). Nutrient Losses from Rain-Fed Bench Terraced Cultivation Systems in High Rainfall Areas of the Mid-hills of Nepal. Land Degrad. Dev. 18, 486–499. doi:10.1002/ldr.792
Agresti, A. (2002). Categorical Data Analysis. Hoboken, New Jersey: John Wiley & Sons. doi:10.1002/0471249688
Akaike, H. (1973). Information Theory and an Extension of the Maximum Likelihood Principle. 2nd International Symposium On Information Theory. Tsahkadsor. Armenian SSR.
Akünal, A., and Aslan, E. G. (2017). Ecological Investigations on Hydrophilidae and Helophoridae (Coleoptera) Specimens Gathered from Several Water Bodies of Western Turkey. Knowl. Manag. Aquat. Ecosyst. 418, 43. doi:10.1051/kmae/2017035
Alfaro, M., Rosolem, C., Goulding, K., Johnston, J., and Wang, H. (2017). Potassium Losses and Transfers in Agricultural and Livestock Systems. Frontiers of Potassium Science Conference, 25–27 January. Rome: Italy, O166–O173.
Alfaro, M., Salazar, F., Iraira, S., Teuber, N., Villarroel, D., and Ramírez, L. (2008). Nitrogen, Phosphorus and Potassium Losses in a Grazing System with Different Stocking Rates in a Volcanic Soil. Chilean J. Agric. Res. 68, 146–155. doi:10.4067/S0718-58392008000200004
Allen, R. G., Pereira, L. S., Raes, D., and Smith, M. (2006). Evapotranspiración del cultivo: Guías para la determinación de los requerimientos de agua de los cultivos. Nº56. Roma: FAO. Available at: http://www.fao.org/3/x0490s/x0490s00.htm.
Arrieta Chavarría, O. (1999). Gentes e inundaciones en los pueblos del Tempisque. Revista Geográfica de América Cent. 37, 109–126.
Arroyo, A. T. (2008). Modelos mixtos en el análisis de loci de caracteres cuantitativos y su aplicación en mejoramiento vegetal. PhD thesis. Córdoba, Argentina: Universidad Nacional de Córdoba.
Avalos, G. (2019). “Still Searching the Rich Coast: Biodiversity of Costa Rica, Numbers, Processes, Patterns, and Challenges,” in Global Biodiversity, Volume 4: Selected Countries in the Americas and Australia. Editor T. Pullaiah (Ontario and New Jersey: Apple Academic Press), 101–138.
Axelrod, D. I. (1979). Age and Origin of Sonoran Desert Vegetation. Occ. Pap. Calif. Acad. Sci. 132, 1–74.
Axelrod, D. I. (1975). Evolution and Biogeography of Madrean-Tethyan Sclerophyll Vegetation. Ann. Mo. Bot. Garden 62, 280–334. doi:10.2307/2395199
Balzarini, M. G., González, L., Tablada, M., Casanoves, F., Di Rienzo, J. A., and Robledo, C. W. (2008). Infostat. Manual del Usuario. Córdoba: Editorial Brujas.
Becerra, J. X. (2005). From the Cover: Timing the Origin and Expansion of the Mexican Tropical Dry forest. Proc. Natl. Acad. Sci. 102, 10919–10923. doi:10.1073/pnas.0409127102
Brenes, A., and Bonilla, A. (2012). La Niña 2010-2012. Estudio de Caso Costa RicaThe United Nations Office for Disaster Risk Reduction, GAR 2013. Santiago de Cali: Corporación OSSO.
Bu, H., Tan, X., Li, S., and Zhang, Q. (2010). Temporal and Spatial Variations of Water Quality in the Jinshui River of the South Qinling Mts., China. Ecotoxicology Environ. Saf. 73, 907–913. doi:10.1016/j.ecoenv.2009.11.007
Burghelea, C. I., Zaharescu, D. G., Hooda, P. S., and Palanca-Soler, A. (2011). Predatory Aquatic Beetles, Suitable Trace Elements Bioindicators. J. Environ. Monit. 13, 1308–3310. doi:10.1039/c1em10016e
Burnham, K. P., and Anderson, D. R. (1998). Model Selection and Multimodel Inference. A Practical Information-Theoretic Approach. New York, Berlin, Heidelberg: Springer-Verlag. doi:10.1007/978-1-4757-2917-7
Carrasco, L. M., Galván, I., and Gordo, O. (2009). Partial Least Square Regression as an Alternative to Current Regression Methods Used in Ecology. Oikos 118, 681–690. doi:10.1111/j.1600-0706.2008.16881.x
Castillo, L. E., Martínez, E., Ruepert, C., Savage, C., Gilek, M., Pinnock, M., et al. (2006). Water Quality and Macroinvertebrate Community Response Following Pesticide Applications in a Banana Plantation, Limon, Costa Rica. Sci. Total Environ. 367, 418–432. doi:10.1016/j.scitotenv.2006.02.052
Cleves, J. A., Toro, J., and Martínez, L. F. (2016). Los balances hídricos agrícolas en modelos de simulación agroclimáticos. Una revisión analítica. Rev. Col. Cien. Hort. 10, 149–163. doi:10.17584/rcch.2016v10i1.4460
Colinvaux, P. (1997). “The History of Forests on the Isthmus from the Ice Age to the Present,” in Central America - A Natural and Cultural History. Editor A. G. Coates (New Haven and London: Yale University Press), 123–136.
Connolly, N. M., Pearson, B. A., and Pearson, R. G. (2007). “Macroinvertebrates as Indicators of Ecosystem Health in Wet Tropics Streams,” in Biological Indicators of Ecosystem Health in Wet Tropic Streams, Final Report Task 3. Catchment to Reef Research Program. Cooperative Research Centre for Rainforest Ecology & Management and Cooperative Research Centre for the Great Barrier Reef World Heritage Area). Editors A. H. Arthington, and R.G. Pearson (Townsville: James Cook University), 129–176.
Damborenea, C., Roger, D. C., and Thorp, J. H. (2020). Thorp and Covich's Freshwater Invertebrates, Volume 5: Keys to Neotropical and Antarctic Fauna. Cambridge, Massachusetts: Academic Press.
Di Rienzo, J. A., Casanoves, F., Balzarini, M. G., Gonzalez, L., Tablada, M., and Robledo, C. W. (2020). InfoStat versión 2020. Centro de Transferencia InfoStat. Argentina: FCA, Universidad Nacional de Córdoba. Available from: http://www.infostat.com.ar.
Di Rienzo, J. A., Guzmán, A. W., and Casanoves, F. (2002). A Multiple Comparisons Method Based on the Distribution of the Root Node Distance of a Binary Tree. J. Agr. Biol. Env. Stat. 7, 1–14. doi:10.1198/10857110260141193
Domínguez, E., and Fernández, H. R. (2009). Macroinvertebrados Bentónicos Sudamericanos: Sistemática y Biología. Tucumán: Fundación Miguel Lillo.
Espinoza Rivera, Y. L. (2018). Modelación hidrodinámica del río Las Palmas y un trayecto del río Tempisque para determinar áreas de afectación en eventos extremos, permitiendo visualizar su influencia sobre la red de canales del proyecto Paacume. B.Sc. Thesis. Cartago: Tecnológico de Costa Rica.
Fairchild, G. W., Cruz, J., Faulds, A. M., Short, A. E. Z., and Matta, J. F. (2003). Microhabitat and Landscape Influences on Aquatic Beetle Assemblages in a Cluster of Temporary and Permanent Ponds. J. North Am. Benthological Soc. 22, 224–240. doi:10.2307/1467994
FAO (1992). CropWat, a Computer Program for Irrigation Planning and Management. FAO Irrigation and Drainage Paper 26. Rome: FAO.
Gabriel, K. R. (1971). The Biplot Graphic Display of Matrices with Application to Principal Component Analysis. Biometrika 58, 453–467. doi:10.1093/biomet/58.3.453
Goulding, K., Murrell, T. S., Mikkelsen, R. L., Rosolem, C., Johnston, J., Wang, H., et al. (2020). “Outputs: Potassium Losses from Agricultural Systems,” in “Outputs: Potassium Losses from Agricultural Systems” in Improving Potassium Recommendations for Agricultural Crops. Editors T. S. Murrell, R. L. Mikkelsen, G. Sulewski, R. Norton, and M. L. Thompson (Cham, Switzerland: Springer Nature), 75–97. doi:10.1007/978-3-030-59197-7_3
Graham, A., and Dilcher, D. (1995). “The Cenozoic Record of Tropical Dry forest in Northern Latin America and the Southern United States,” in The Cenozoic Record of Tropical Dry forest in Northern Latin America and the Southern United States" in Seasonally Dry Tropical Forests. Editors S. H. Bullock, H. A. Mooney, and E. Medina (New York: Cambridge University Press), 124–145. doi:10.1017/cbo9780511753398.006
Granados-Ramírez, J. G., Rueda-Gómez, A. K., and Vázquez Servín, K. A. (2017). Entomofauna acuática predominante en cinco ríos de la parte alta del Balsas, entre Morelos y Puebla. México. Entomol. Mex. 4, 108–114.
Hamada, N., Thorp, J. H., and Rogers, D. C. (2019). Thorp and Covich's Freshwater Invertebrates. Keys to Neotropical Hexapoda, Vol. 3. Cambridge, Massachusetts, USA: Academic Press.
Hawkes, H. A. (1997). Origin and Development of the Biological Monitoring Working Party Score System. Water Res. 32 (3), 964–968. doi:10.1016/s0043-1354(97)00275-3
Herrera, W. (2016). “Climate of Costa Rica,” in Costa Rican Ecosystems. Editor M. Kappelle (Chicago and London: The University of Chicago Press), 19–29.
IMN (Instituto Meteorológico Nacional) (1985). Atlas Climatológico de Costa Rica. San José, CR: IMN.
IMN (Instituto Meteorológico Nacional) (2011). Atlas de las Cuencas Hidrográficas de Costa Rica. San José, CR: IMN.
Ismail, T. G. (2018). Effect of Geographic Location and Sexual Dimorphism on Shield Shape of the Red Sea Hermit Crab Clibanarius Signatus Using Geometric Morphometric Approach. Canad. J. Zool. 96, 667–679. doi:10.1016/b978-0-12-809324-5.21964-0
Janzen, D., and Hallwachs, W. (2016). “Biodiversity conservation and future in Costa Rica: The case of Área de Conservación Guanacaste (ACG),” in Costa Rican Ecosystems. Editor M. Kappelle (Chicago: The University of Chicago Press), 290–341.
Jiménez, Q. M., Carrillo, E., and Kappelle, M. (2016). “The Northern Pacific lowland Dry Seasonal Forests of Guanacaste and the Nicoya Peninsula,” in Costa Rican Ecosystems. Editor M. Kappelle (Chicago and London: The University of Chicago Press), 247–289.
Kampa, E., Artemiadou, V., and Lazaridou, M. (2000). Ecological Quality of the River Axios during spring and Summer. J. Zool. 130, 21–77.
Kohlmann, B., Arroyo, A., Macchi, P. A., and Palma, R. (2018b). “Biodiversity and Biomonitoring Indexes,” in Biodiversity and Biomonitoring Indexes" in Integrated Analytical Approaches For Pesticide Management. Editors B. Maestroni, and A. Cannavan (Elsevier Publishers/Academic Press and Joint FAO/IAEA Division), 83–106. doi:10.1016/b978-0-12-816155-5.00006-3
Kohlmann, B., Arroyo, A., Springer, M., and Díaz, D. (2015). “Agrorural Ecosystem Effects on the Macroinvertebrate Assemblages of a Tropical River,” in Biodiversity in Ecosystems-Linking Structure and Function. Editors Y.-H. Lo, J. A. Blanco, and S. Roy (Rijeka: INTECH Publishers), 317–351.
Kohlmann, B., and Halffter, G. (1990). Reconstruction of a Specific Example of Insect Invasion Waves: The Cladistic Analysis of Canthon (Coleoptera: Scarabaeidae) and Related Genera in North America. Quaest. Entomol. 26, 1–10.
Kohlmann, B., Palma, R., and Macchi, and. P. (2018a). “Method 29. Biomonitoring of Water Quality Using Macroinvertebrates as Bioindicators,” in Analytical Methods for Agricultural Contaminants. Editors B. Maestroni, V. Ochoa, and A. Cannavan (Joint FAO/IAEA Division and Elsevier Publishers/Academic Press), 157–168.
Lenntech (2020). Potassium (K) and Water. Available at: https://www.lenntech.com/periodic/water/potassium/potassium-and-water.htm (Accessed December 5, 2020).
Liess, M., Brown, C., Dohmen, P., Hart, A., and Heimbach, F. (2003). “Effects of Pesticides in the Field,” in Le Croisic, France: EU and SETAC Europe Workshop (Le Croisic: Society of Environmental Toxicology and Chemistry).
Lira, A. F. A., de Almeida, F. N. A., Salomão, R. P., and de Albuquerque, C. M. R. (2021). Effects of Habitat Quality on Body Size of the Litter Dwelling Scorpion Tityus Pusillus in Fragmented Rainforests of Brazil. J. Arachnology 48, 295–299. doi:10.1636/joa-s-19-081
Lorion, C. M., and Kennedy, B. P. (2009). Relationships between Deforestation, Riparian forest Buffers and Benthic Macroinvertebrates in Neotropical Headwater Streams. Freshw. Biol. 54, 165–180. doi:10.1111/j.1365-2427.2008.02092.x
Macadam, C. R., and Stockan, J. A. (2015). More Than Just Fish Food: Ecosystem Services provided by Freshwater Insects. Ecol. Entomol. 40 (S1), 113–123. doi:10.1111/een.12245
Maestroni, B., Ferris, I. G., Correll, R., Kohlmann, B., Nordgaard, A., Kookana, R. S., et al. (2018). “Generic Guidelines in Integrated Analytical Approaches to Assess Indicators of Pesticide Management Practices at a Catchment Scale. Black-Box Monitoring and the Laboratory's Role in Fostering Good Agricultural Practice,” in Integrated Analytical Approaches for Pesticide Management. Editors B. Maestroni, and A. Cannavan (Elsevier Publishers/Academic Press and Joint FAO/IAEA Division), 7–27.
Mateo-Vega, J. (2001). “Características Generales de la Cuenca del Río Tempisque,” in La Cuenca del Río Tempisque. Perspectivas para un Manejo Integrado. Editors J. A. Jiménez, and E. González (San José, Costa Rica: Organización para Estudios Tropicales), 32–73.
Merritt, K., Cummins, W., and Berg, M. B. (2008). In An Introduction to the Aquatic Insects of North America. 4th Ed. (Dubuque: Kendall/Hunt Publ).
MINAE (Ministerio Nacional de Ambiente y Energía, CR) (2007). Reglamento para la Evaluación y Clasificación de la Calidad de Cuerpos de Agua Superficiales. Decreto Ejecutivo Nº 33903-MINAE-S. Alcance No. 8 a La Gaceta No. 78, lunes 17 de septiembre del 2007. San José, CR: Imprenta Nacional. Available at: http://historico.gaceta.go.cr/pub/2007/09/17/COMP_17_09_2007.html.
Mittermeier, R. A., Robles Gil, P., Hoffman, M., Pilgrim, J., Brooks, T., Mittermeier, C. G., et al. (2004). Hotspots Revisited. Mexico City: CEMEX.
Newbold, J. D., Sweeney, B. W., Jackson, J. K., and Kaplan, L. A. (1995). Concentrations and export of Solutes from Six Mountain Streams in Northwestern Costa Rica. J. North Am. Benthological Soc. 14, 21–37. doi:10.2307/1467722
Obando, V. (2007). Biodiversidad de Costa Rica en cifras. Santo Domingo de Heredia, Costa Rica: Instituto Nacional de Biodiversidad.
Obando, V. (2002). Biodiversidad de Costa Rica, Estado del Conocimiento y Gestión. Santo Domingo de Heredia, Costa Rica: Instituto Nacional de Biodiversidad.
Paaby, P., Ramírez, A., and Pringle, C. M. (1998). The Benthic Macroinvertebrate Community in Caribbean Costa Rican Streams and the Effect of Two Sampling Methods. Rev. Biol. Trop. 46, 185–199.
Peters, G. (2001). “Características Generales de la Cuenca del Río Tempisque,” in La Cuenca del Río Tempisque. Perspectivas para un Manejo Integrado. Editors J. A. Jiménez, and E. González (San José, Costa Rica: Organización para Estudios Tropicales), 1–21.
Pinheiro, J. C., and Bates, D. M. (1996). Unconstrained Parametrizations for Variance-Covariance Matrices. Stat. Comput. 6, 289–296. doi:10.1007/bf00140873
POWER Project (2020). NASA Langley Research Center (LaRC). Available at: https://power.larc.nasa.gov/(Accessed November 5, 2020). Prediction of Worldwide Energy Resource.
Ramírez, A., and Gutiérrez-Fonseca, P. E. (2014). Functional Feeding Groups of Aquatic Insect Families in Latin America: a Critical Analysis and Review of Existing Literature. Rbt 62 (Suppl. 2), 155–167. doi:10.15517/rbt.v62i0.15785
Ramírez, A., and Pringle, C. M. (2001). Spatial and Temporal Patterns of Invertebrate Drift in Streams Draining a Neotropical Landscape. Freshw. Biol. 46, 47–62. doi:10.1046/j.1365-2427.2001.00636.x
Risoluti, R., Gullifa, G., and Materazi, S. (2020). Assessing the Quality of Milk Using a Multicomponent Analytical Platform MicroNIR/Chemometric. Front. Chem. 8, 614718. doi:10.3389/fchem.2020.614718
Rizo-Patrón, F., Kumar, A., McCoy Colton, M. B., Springer, M., and Trama, F. A. (2013). Macroinvertebrate Communities as Bioindicators of Water Quality in Conventional and Organic Irrigated rice fields in Guanacaste, Costa Rica. Ecol. Indic. 29, 68–78.
Roldán-Pérez, G. (1996). Guía para el estudio de los macroinvertebrados acuáticos del Departamento de Antioquia. Bogotá: Fondo FEN-Colombia-Colciencias.
Ruiz-Picos, R. A., Sedeño-Díaz, J. A., and López-López, E. (2016). Ensambles de macroinvertebrados acuáticos relacionados con diversos usos del suelo en los ríos Apatlaco y Chalma-Tembembe (cuenca del Río Balsas), México. Hidrobiológica 26, 443–458.
Salomão, R. P., Favila, M. E., González‐Tokman, D., and Chamorro‐Florescano, I. A. (2019). Contest Dynamics for Food and Reproductive Resources Are Defined by Health Condition in a Dung Beetle. Ethology 125, 343–350. doi:10.1111/eth.12858
Sánchez-Argüello, R., Cornejo, A., Pearson, R. G., and Boyero, L. (2010a). Spatial and Temporal Variation of Stream Communities in a Human-Affected Tropical Watershed. Ann. Limnol. - Int. J. Lim. 46, 149–156. doi:10.1051/limn/2010019
Sánchez Argüello, R., Cornejo, A., Boyero, L., and Santos Murgas, A. (2010b). Evaluación de la calidad del agua en la Cuenca del río Capira, Panamá. Tecnociencia 12, 57–70.
Skowron, P., Sworońska, M., Bronowicka-Mielniczuk, U., Filipek, T., Igras, J., Kowalczyk-Juśko, A., et al. (2018). Anthropogenic Sources of Potassium in Surface Water: The Case Study of the Bystrzyca River Catchment, Poland. Agr. Ecosyst. Env. 265, 454–460. doi:10.1016/j.agee.2018.07.006
Souza, T. B., França, F. M., Barlow, J., Dodonov, P., Santos, J. S., Faria, D., et al. (2020). The Relative Influence of Different Landscape Attributes on Dung Beetle Communities in the Brazilian Atlantic forest. Ecol. Indicators 117, 106534. doi:10.1016/j.ecolind.2020.106534
Springer, M., Echeverría, S., and Gutiérrez, P. (2014). “Costa Rica,” in Diversidad, conservación y uso de los macroinvertebrados dulceacuícolas de México, Centroamérica, Colombia, Cuba y Puerto Rico. Editors P. Alonso-EguíaLis, J. M. Mora, and B. Campbell (Springer), 119–155.
Springer, M., Ramírez, A., and Hanson, P. (2010). Macroinvertebrados de agua dulce de Costa Rica I: Introducción a los grupos de macroinvertebrados, métodos, biomonitoreo, Ephemeroptera, Odonata, Plecoptera, Trichoptera. Rev. Biol. Trop. 58 (Suppl. 4), 1–200. doi:10.15517/rbt.v58i4
Stein, H., Springer, M., and Kohlmann, B. (2008). Comparison of Two Sampling Methods for Biomonitoring Using Aquatic Macroinvertebrates in the Dos Novillos River, Costa Rica. Ecol. Eng. 34, 267–275. doi:10.1016/j.ecoleng.2007.06.010
Tobias, R. (1995). An Introduction to Partial Least Squares regressionSUGI Proceedings. Cary, NC: SAS Institute.
Toledo, V. M. (1982). “Pleistocene Changes of Tropical Vegetation in Mexico,” in Biological Diversification in the Tropics. Editor G. T. Prance (New York: Columbia Univ. Press), 93–111.
Trama, F. A., Rizo Patrón, V., and Springer, M. (2009). Macroinvertebrados bentónicos del humedal de Palo Verde, Costa Rica. Rev. Biol. Trop. 57, 275–284.
Trodd, W., and O’Boyle, S. (2018). Water Quality in 2017: An Indicators Report. Wexford: Environmental Protection Agency. doi:10.4324/9781315190846
Valerio, C. E. (2006). Costa Rica. Ambiente Y Sociedad. Santo Domingo de Heredia, Costa Rica: Instituto Nacional de Biodiversidad.
Van Echelpoel, W., Forio, M. A. E., Van Butsel, J., Lock, K., Utreras, J. A. D., Dominguez-Granda, L. E., et al. (2018). Macroinvertebrate Functional Feeding Group Structure along an Impacted Tropical River: The Portoviejo River (Ecuador). Limnologica 73, 12–19. doi:10.1016/j.limno.2018.10.001
Vannote, R. L., Minshall, G. W., Cummins, K. W., Sedell, J. R., and Cushing, C. E. (1980). The River Continuum Concept. Can. J. Fish. Aquat. Sci. 37, 130–137. doi:10.1139/f80-017
Vásquez, D., Springer, M., Castro, A., and Kohlmann, B. (2010). Indicadores de la Calidad del Agua. Cuenca del Río Tempisque. San José, Costa Rica: EARTH-UCR.
Keywords: freshwater fauna, biodiversity, tropical dry forest, functional feeding groups, Tempisque basin, water quality, tropical rain forest
Citation: Kohlmann B, Vásquez D, Arroyo A and Springer M (2021) Taxonomic and Functional Diversity of Aquatic Macroinvertebrate Assemblages and Water Quality in Rivers of the Dry Tropics of Costa Rica. Front. Environ. Sci. 9:660260. doi: 10.3389/fenvs.2021.660260
Received: 29 January 2021; Accepted: 19 July 2021;
Published: 06 August 2021.
Edited by:
Eugenia López-López, Instituto Politécnico Nacional de México (IPN), MexicoReviewed by:
Fernando Mayer Pelicice, Federal University of Tocantins, BrazilRaphael Ligeiro, Federal University of Pará, Brazil
Copyright © 2021 Kohlmann, Vásquez, Arroyo and Springer. This is an open-access article distributed under the terms of the Creative Commons Attribution License (CC BY). The use, distribution or reproduction in other forums is permitted, provided the original author(s) and the copyright owner(s) are credited and that the original publication in this journal is cited, in accordance with accepted academic practice. No use, distribution or reproduction is permitted which does not comply with these terms.
*Correspondence: Bert Kohlmann, bkohlman@earth.ac.cr