Conversion of Mangroves Into Rice Cultivation Alters Functional Soil Microbial Community in Sub-Humid Tropical Paddy Soil
- ICAR-National Rice Research Institute, Cuttack, India
Conversion of mangrove vegetation into rice cultivation is considerably enhanced nowadays which adversely affects ecological sustainability. Soil microbial community is one of the key indicators to monitor soil health in mangroves. Studies on the variations in the microbial community within mangroves are plenty, whereas reports in mangrove-converted paddy soils are scarce. Therefore, Biolog® eco-plate-based technique was used in this study to assess soil microbial community in the Bhitarkanika (MB) and Sundarban (MS) sub-humid tropical mangroves-converted paddy soil. The results showed that significantly lower soil microbial biomass carbon and enzyme activities were recorded in MB and MS compared to the NRRI (National Rice Research Institute) paddy soil where continuous rice cultivation is being practiced conventionally since 1946 under the sub-humid tropical region. Biolog®-based average well color development (AWCD) was found significantly lower in MS and MB compared to NRRI. Shannon–Weaver and McIntosh indices followed the similar trends of AWCD. A biplot analysis indicated the positive correlation of pH, available phosphorus, actinomycetes population, and phenolic compound utilization under MS, whereas EC and phosphate-solubilizing bacteria were positively correlated under MB. Compared to MS and MB, NRRI paddy soil harbored more carbohydrate-utilizing microbes and showed a positive correlation with fluorescin-diacetate, dehydrogenase, and acid phosphatase. Overall, the present study suggested that the conversion of the Sundarban and Bhitarkanika mangroves into rice cultivation adversely affected the microbial diversity, thereby altering natural sustainability.
Introduction
Mangrove ecosystems constitute a very rich biodiversity of microorganisms which play vital roles in nutrient cycling and regulating the physico-chemical equilibrium in these environments (Alongi et al., 1993; Holguin et al., 1999; Borrell et al., 2016; Atwood et al., 2017). Organic carbon (C) stocks of mangrove ecosystems are measured to have equal or higher than terrestrial tropical forests (Mcleod et al., 2011) and are considered as one of the most important C-sinks in the biosphere (Twilley et al., 1992; Chmura et al., 2003; Nellemann and Corcoran, 2009; and Donato et al., 2011). Besides the natural reservoir of C stocks, the mangrove ecosystem also houses a diverse group of beneficial microbial communities (Holguin et al., 2001; Maria and Sridhar, 2002; and Thatoi et al., 2013). The major microbial community harbored in Indian mangroves is marine algae (60.1%), fungi (11.2%), and bacteria (7.5%) (Sahu et al., 2015). However, these reservoirs are being lost due to several factors that despoiled the mangrove ecosystem around the world. In recent estimates, nearly 0.1 million ha of the world’s mangrove forests was lost from 2001 to 2012 at the rate of 0.7–7% annually, which was around four times higher than that of rainforests (Strong and Minnemeyer, 2015; Thomas et al., 2017). India covers around 7% of the world’s mangroves (Kathiresan 2000; Banerjee et al., 2012a) which is being lost at the rate of 2.8% since the last century (Kumar, 2000; Sahu et al., 2015). It is mostly due to the conversion of mangrove forests into agriculture practices (rice cultivation), aquaculture (shrimp farming), and several anthropogenic activities such as intense boating and fishing, dredging, tourism, and port activities (Duarte et al., 2004; Bouillon et al., 2008; Duarte et al., 2010; Kennedy et al., 2010; United Nations Framework on Climate Change, 2012).
Past reports mainly emphasized the microbial communities under the mangrove ecosystem worldwide (Maria and Sridhar, 2002; Thatoi et al., 2013), however, reports related to the functional diversity of the microbial community under mangrove-converted paddy soil are limited. A paddy cultivated soil ecosystem represents one of the best-studied models for soil microbial ecology (Kumar et al., 2020a; Kumar et al., 2021). Rice can be grown in a wide range of soil, climatic, and hydrological conditions including mangrove ecosystems (Bouillion et al., 2008; Kennedy et al., 2010). Variations of the microbial community under paddy soil are drastically influenced by the application of fertilizers (Kumar et al., 2017; Kumar et al., 2018) and pesticides and have tremendous effects on the nutrient dynamics in the soil. For quantification of microbial community and diversity, researchers are currently using many methods under diverse ecosystems including the rice rhizosphere (Kumar et al., 2018; Manjunath et al., 2018). Among these methods, Biolog® eco-microplates are commonly used to assess the functional diversity of soil microbial communities under different ecosystems including rice (Zak et al., 1994; Li S. et al., 2013; Kumar et al., 2017; Pradhan et al., 2019; Kumar et al., 2020b).
Bhitarkanika and Sundarban are two sub-humid tropical mangroves situated in eastern India and have huge reservoirs of the microbial community (Thatoi et al., 2013; Dutta et al., 2015; Arya and Syriac, 2018). Reports indicated that the mangrove forest area of the Bhitarkanika (Chauhan et al., 2017) and Sundarban (Ramesh et al., 2019) have been significantly converted into agricultural land (∼50 and ∼6% of total area of Bhitarkanika and Sundarban mangroves, respectively) over the last few decades, which is a threat to environmental sustainability with more C-emissions such as methane, thereby reducing the cost-effectiveness of the same land (Priyadarshini, 2015; Chauhan et al., 2017). Moreover, the reclaimed mangroves, including Sundarban, are highly populated and have fallen victim to various adverse effects of climate change and also impacting the salinity regime (Chowdhury et al., 2021a) which might affect the microbial community of that region (Chambers et al., 2016).
Numerous studies indicated the alteration of soil microbial community in Bhitarkanika (Thatoi et al., 2013; Dangar et al., 2016; Behera et al., 2019) and Sundarban (Banerjee et al., 2012b; Das et al., 2012; Bhattacharyya et al., 2019), however, reports on alteration of the microbial community under mangrove-converted paddy soils are limited (Tripathi et al., 2016). Hence, an attempt was made to analyze the significant changes in functional microbial diversity using Biolog® eco-micro plates, chemical, and enzymatic properties of soils of the mangrove-converted paddy soil and compared with NRRI paddy soil where paddy cultivation is being practiced conventionally since 1946. We hypothesized that the conversion of sub-humid tropical mangrove vegetation into rice cultivation may alter its soil microbial community.
Materials and Methods
Soil Sampling and Chemical Analyses
Rhizosphere soil (panicle initiation stage of rice crop) samples were collected from two sub-humid tropical mangrove-converted paddy soils from Bakkhali, Sundarban (MS), West Bengal (21° 33′ N, 88° 15′ E) and Dangamal, Bhitarkanika (MB), Odisha (20° 75′ N 86° 85′ E), and ICAR-National Rice Research Institute (NRRI), Cuttack, India (20° 25′ N, 85° 55′ E). A composite sample was made after collecting five soil samples from each location. A portion of the composite soil was air-dried, powdered, and passed through a sieve of 2 mm. This sample was used to analyze the soil pH, electrical conductivity, organic carbon (SOC), and total nitrogen (TN) using an elemental analyzer (Flash 2000; Thermo Scientific), available-P (Bray and Kurtz 1945), and available-K (Piper and CS, 1966) as per standard protocols. The remaining composite soil sample was stored in a refrigerator at 4°C for biochemical analysis. Microbial biomass C was estimated by using chloroform fumigation extraction methods adopted from Vance et al. (1987). The soil enzyme parameters viz., dehydrogenase (DHA), acid phosphatase (ACP), alkaline phosphatase (ALP), and urease were determined using methods referred by Acosta-Martinez and Tabatabai (2000) and Kumar et al. (2018).
Determination of Different Groups of Soil Microbial Population
10 g of soil was suspended in 0.85% sterile saline distilled water (90 ml) and dilutions were prepared from 10−2 to 10−6 levels. A suitable sterile medium was prepared for each particular group as per the methods referred by Burnett et al. (1957). By using the spread plate technique, 100 µl of soil supernatants were spread uniformly on Petri plates in triplicates and the plates were incubated for 3–7 days at 30 ± 0.1°C and the appeared colonies were counted as colony-forming unit (CFU) g−1 soil (Kumar et al., 2017).
For the determination of heterotrophic aerobic bacterial population (HAB), a nutrient agar medium was prepared and diluted suspensions on plates were spread plated and kept for incubation for 4 days at 30°C. The colonies (bacterial) were counted and recorded as CFU g−1 soil. Similarly, for the enumeration of fungi and actinomycetes, the same process was carried out in Rose Bengal and actinomycetes isolation agar medium, respectively (Kutzner and Buchenauer, 1986).
Winogrodsky’s medium (Nitrosomonas medium) was used to determine the nitrifier (NH4+ oxidizing) population (Sharma and Dangar, 2016). Each Petri plate was kept for incubation at 30°C for 25–30 days and then a sulfanilic acid reagent was flooded into it to observe the pink colonies. Similarly, denitrifier (NO3− reducing) bacterial population was counted by using Winogrodsky’s medium where sodium or potassium nitrate was used instead of ammonium sulfate. Each Petri plate was incubated for 3 days at 30°C and sulfanilic acid reagent was poured into it to observe the appearance of pink colonies and these were counted.
The population of phosphate-solubilizing bacteria (PSB) was counted by using a calcium phosphate agar medium. The diluted soil samples were spread plated on plates and kept for incubation for 3 days at 30°C and the halo zone colonies were counted. A Thiobacillus medium was used to determine the population of sulfur-oxidizing bacteria after incubating the plate with the diluted soil sample for 7 or more days at 30°C. Black/brown colonies that appeared on the plate were counted.
Oligotrophic (OL) and copiotrophic (CO) bacterial populations were determined using tryptone soya yeast extract medium (Ochs et al., 1995) and copiotrophic isolation medium, respectively. Petri-plates were spread plated with diluted soil samples and kept for incubation for 3 days at 30°C. The brownish color colonies were counted as CFU g−1 soil. Jensen’s medium was used to assess the asymbiotic nitrogen-fixing bacterial population after inoculating the plates with diluted soil samples and then kept for incubation for 4 days at 30°C. The colonies that appeared transparent on the plates were counted and transformed into CFU g−1 soil.
Community-Level Substrate Utilization Patterns Analysis Using Biolog-Ecoplate
In a 250 ml flask, fresh soil, equivalent to 10 g dry weight was taken from each MS, MB, and NRRI paddy soils. Then, 100 ml of distilled water was added and placed on a shaker at 250 rpm for 30 min. Final diluted (10−3) soil suspension (150 µl) was added in 93 wells of Biolog ecoplate® (Biolog, Hayward, CA, United States), containing 31 kinds of C sources with three replications out of 96 wells. Whereas, 150 µl of sterile water was added in the remaining 3 wells (did not contain any C-source), designated as control. The eco plates were then incubated at 25°C for 24, 48, 72, 96 h, and the change of color that developed after the utilization of C sources by microorganisms was measured at an absorbance of 590 nm in Microlog 4.01 (Biolog, Hayward, CA, United States).
Determination of the Total Metabolic Activity of Soil Microorganisms
Average well color development (AWCD) for all C-sources was calculated to evaluate the total microbial activity in MS, MB, and NRRI paddy soils (Li F. et al., 2013; Kumar et al., 2017; Manjunath et al., 2018; Pradhan et al., 2019; and Kumar et al., 2020b).
Where C represents the OD (optical density) value of 31 reaction wells, and R represents the OD value of the control. The AWCD reflects the utilizing trend of different C-sources by microorganism (Choi and Dobbs, 1999).
Analysis of Utilization Pattern of Carbon Sources and Functional Diversity of Soil Microorganisms
Determination of the functional soil microbial diversity index utilizing C-sources expressed by Shannon–Weaver index (H), which is used to calculate diversity indices of the microbial community and was quantified by the formula:
Where pi is the ratio of the relative OD (absorbance) value (C-R) of No. i hole to the sum of relative OD values of all holes of the Biolog Eco-plates.
McIntosh index (U) was calculated by the formula:
By subtracting the OD value of the control well from each of the C-source, the relative absorbance value i.e., ni is derived (Magurran, 1988; Wei et al., 2011; Gu et al., 2018).
Determination of Metabolic Fingerprints of Soil Microorganisms
Principal component analysis (PCA) was used to analyze the metabolic activities of soil microorganisms. Optical density (OD) values recorded in 31 kinds of C-sources utilized by the soil microbial community were used to assess the multi-element vectors (Ma et al., 2006). Six major groups of C-utilizing microbial communities were separated as per the methodology of Insam et al. (1996) and then analyzed for biplot-PCA to correlate with other soil parameters.
Statistical Analysis
Online statistical computing software of NARS, Indian Agricultural Statistics Research Institute, New Delhi, India, was used to analyze the data (http://www.iasri.res.in/sscnars). Descriptive statistics like one-way ANOVA was used to access the variance between means of analyzed parameter among the sampling sites and where significant F value was observed. Difference between individual means was tested using Tukey HSD (Honestly significant difference) at a 5% level of significance. The principal coordinate analysis (PCoA) by Bray–Curtis distance-based redundancy analysis (dbRDA) while the Vegan package in R software version 3.5.0 was used to assess the soil parameters with different treatments. The sample size was taken and analyzed as per Chowdhury et al. (2021b).
Results
Soil pH and Nutrient Contents
Soil pH was significantly (p < 0.05) higher in mangrove-converted paddy soils of MS (7.55) and MB (6.9) compared to NRRI paddy soil (NRRI) (6.5) (Table 1) and natural mangroves of MS and MB (Supplementary Table S1). Similarly, soil organic carbon (SOC) was significantly (p < 0.05) lower in MS (0.52%) and MB (0.38%) compared to NRRI (0.66%) (Table 1) and the natural mangroves of MS and MB (Supplementary Table S1). EC was recorded higher in natural mangroves of MS and MB compared to MS, MB, and NRRI paddy soils (Table 1; Supplementary Table S1). Whereas, available phosphorus (AP) and available potassium (AK) were significantly (p < 0.05) higher in mangrove-converted paddy soils (AP higher in MS and AK higher in MB) compared to NRRI (Table 1).
Microbial Biomass Carbon and Soil Enzymatic Activities
Soil microbial biomass carbon (MBC) ranged from 223.9 μg g−1 to 615.9 μg g−1 in all three studied locations. MBC was significantly (p < 0.05) higher in NRRI than MS and MB. The dehydrogenase activity (DHA) in NRRI was 43.5 μg g−1, whereas it was 24.7 µg g−1 and 11.3 μg g−1 in MS and MB, respectively. An almost similar trend in the result found in other soil enzyme activities such as urease (U), acidic phosphatase (ACP) and alkaline phosphatase (ALP), and these were recorded higher in NRRI paddy soil (144.4 mg g−1, 73.3 μg g−1, and 48.8 μg g−1, respectively) compared to MS and MB (Table 2).

TABLE 2. Microbial biomass carbon and enzyme activities of soil from conventional and mangrove-converted rice areas.
Soil Microbial Population Dynamics
All soil microbial populations (Heterotrophic aerobic bacteria, asymbiotic nitrogen-fixing bacteria, nitrifying bacteria, denitrifying bacteria, phosphate solubilizing bacteria, sulfur-oxidizing bacteria, copiotrophs, and oligotrophs) except actinomycetes and fungi were found non-significant among NRRI, MS, and MB sites. Significantly (p < 0.05) higher actinomycete counts were obtained in MS followed by MB and NRRI (MS > MB > NRRI), whereas the fungal population was recorded highest in NRRI followed by MS and MB (NRRI > MS > MB) (Table 3). In undisturbed mangrove soils of MS and MB, the population of HAB, NI and PSB were found lower compared to converted MS and MB, whereas the population of AC showed dominance under undisturbed MS (Supplementary Table S1).

TABLE 3. Microbial populations (Log CFU g−1) of soil from conventional and mangrove-converted rice areas.
Soil Microbial Community and Functional Diversity
A significantly (p < 0.05) lower value of AWCD and rate of AWCD were found in MB compared to MS and NRRI (MB < MS < NRRI) during the 24–96 h incubation period (Figure 1). Similarly, the diversity indices such as Shannan–Weaver and McIntosh indices were also found lower in MB compared to MS and NRRI (NRRI > MS > MB), whereas the Simpson index showed the opposite trend of Shannan–Weaver and McIntosh indices (NRRI < MS < MB) (Figure 2).
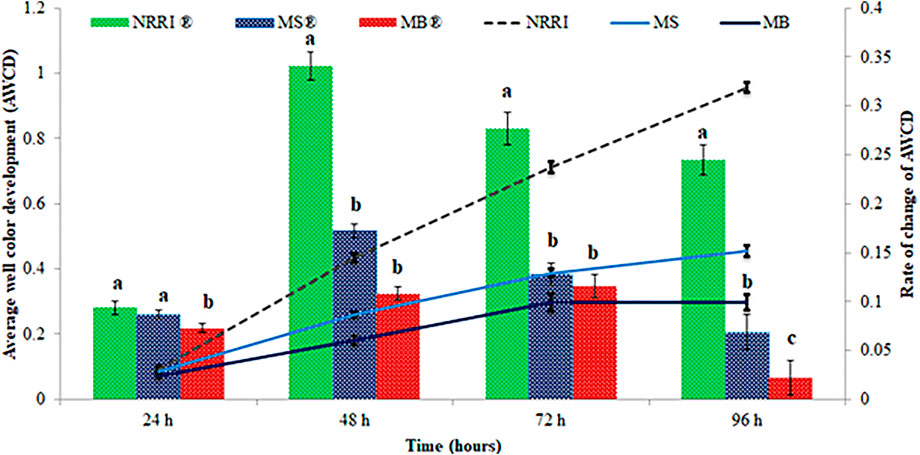
FIGURE 1. Variation of average well color development (AWCD) and rate of AWCD in mangrove-converted and conventional paddy soils. NRRI: National Rice Research Institute, Cuttack, Odisha; MS: Bakkhali, Sundarban, West Bengal; MB: Dangamal, Bhitarkanika, Odisha. ® indicates the rate of change of AWCD. Lines above the bars represent means ± standard deviation. Different letters above the bars indicate significant among the treatments at Tukey HSD (p ≤ 0.05).
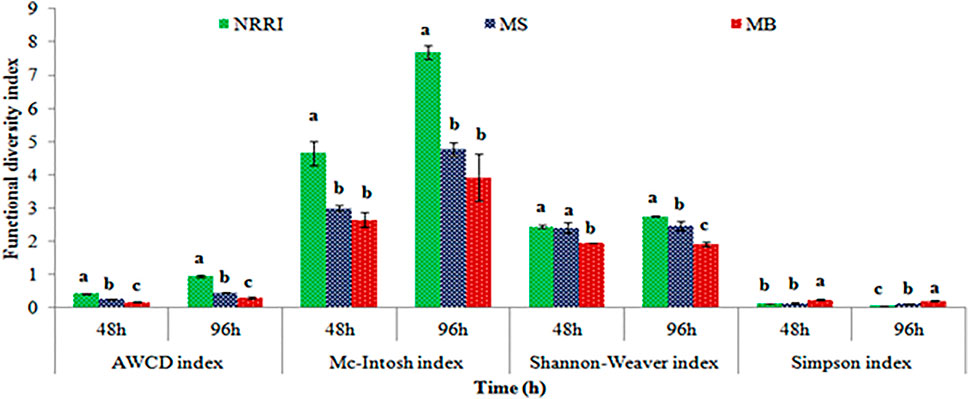
FIGURE 2. Variation of different functional diversity indices in mangrove-converted and conventional paddy soils. NRRI: National Rice Research Institute, Cuttack, Odisha; MS: Bakkhali, Sundarban, West Bengal; MB: Dangamal, Bhitarkanika, Odisha. Lines above the bars represent means ± standard deviation. Different letters above the bars indicate the treatments at Tukey HSD (p ≤ 0.05).
Utilization Pattern of C- Sources by Soil Microbes in NRRI, MS, and MB
A biplot analysis of the parameters obtained from NRRI, MS, and MB soils was shown in the ordination plane (Figure 3), in which the PCA explained a total variance of 97.22%, with principal component 1 (PC1) representing 62.37% and principal component 2 (PC2) representing 34.85% variances, respectively. The PCA showed that the corresponding projective points of six major C groups namely phenolic compounds, polymers, amines, and amino acids; carbohydrates; carboxylic compounds lay at the first, second, and fourth quadrant, respectively (Figure 3), out of which carbohydrates consumption was found highest in NRRI soils compared to MS and MB (Figure 4). The phenolic compounds were positively correlated with MS whereas the carboxylic compound was positively correlated with MB (Figure 4). Polymer compounds were utilized efficiently in the MS soil (Figure 4).
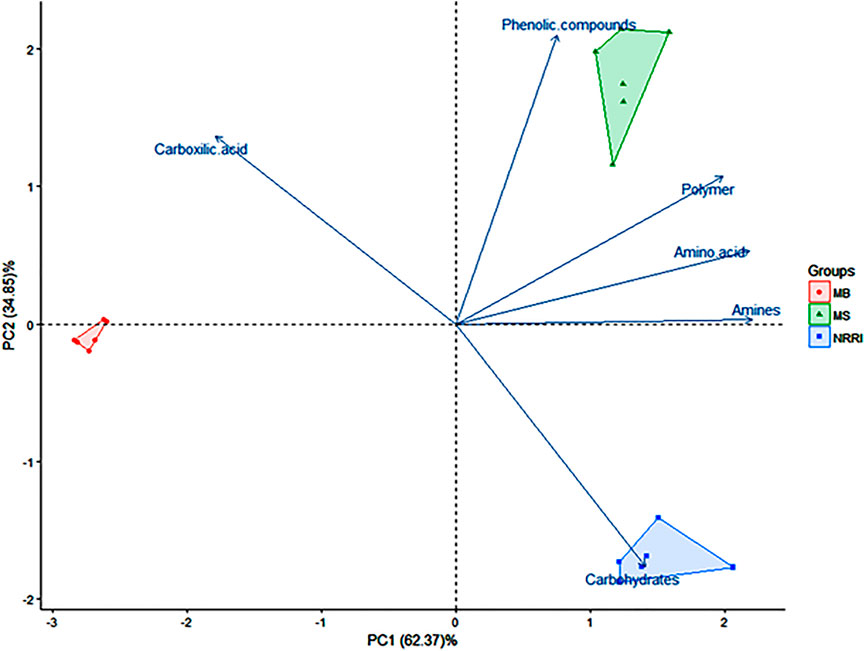
FIGURE 3. Biplot analysis based on 48 h biolog data indicating separation of three treatments of mangrove-converted and conventional paddy soils. NRRI: National Rice Research Institute, Cuttack, Odisha; MS: Bakkhali, Sundarban, West Bengal; MB: Dangamal, Bhitarkanika, Odisha. Values in parentheses indicate percentage contribution by PC1 and PC2.
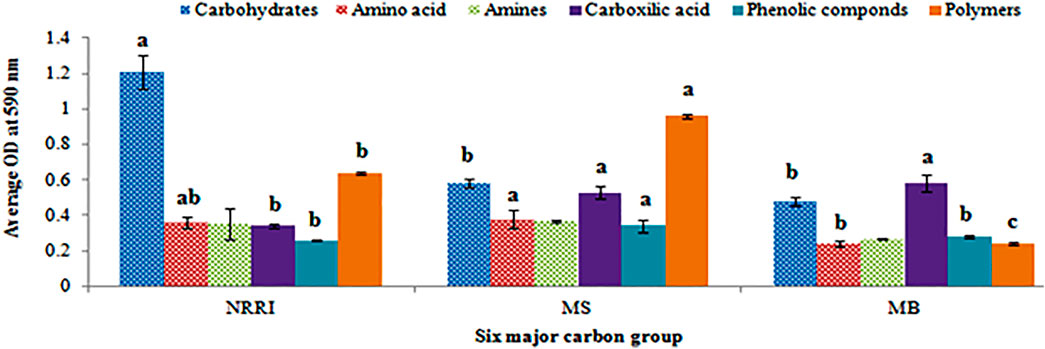
FIGURE 4. Microbial consumption of six major groups of carbon sources of soil in mangrove-converted and conventional paddy soils. NRRI: National Rice Research Institute, Cuttack, Odisha; MS: Bakkhali, Sundarban, West Bengal; MB: Dangamal, Bhitarkanika, Odisha. Line on bars represent means ± standard deviation. Different letters indicate the significant differences in the six major groups of carbon sources utilized by the microbial community among the treatments at Tukey HSD (p ≤ 0.05).
A heat map analysis showed the differentiation of MS and MB from NRRI soil based on the utilization pattern of 31 kinds of C-source by soil microbes. Three C-sources (D-Cellobiose, Glucose-1-phosphate, and α-Methyl-D-Glucoside) were consumed very little by soil microbes present in MS and MB, whereas efficient consumption of these three C-sources was observed in NRRI (Figure 5).
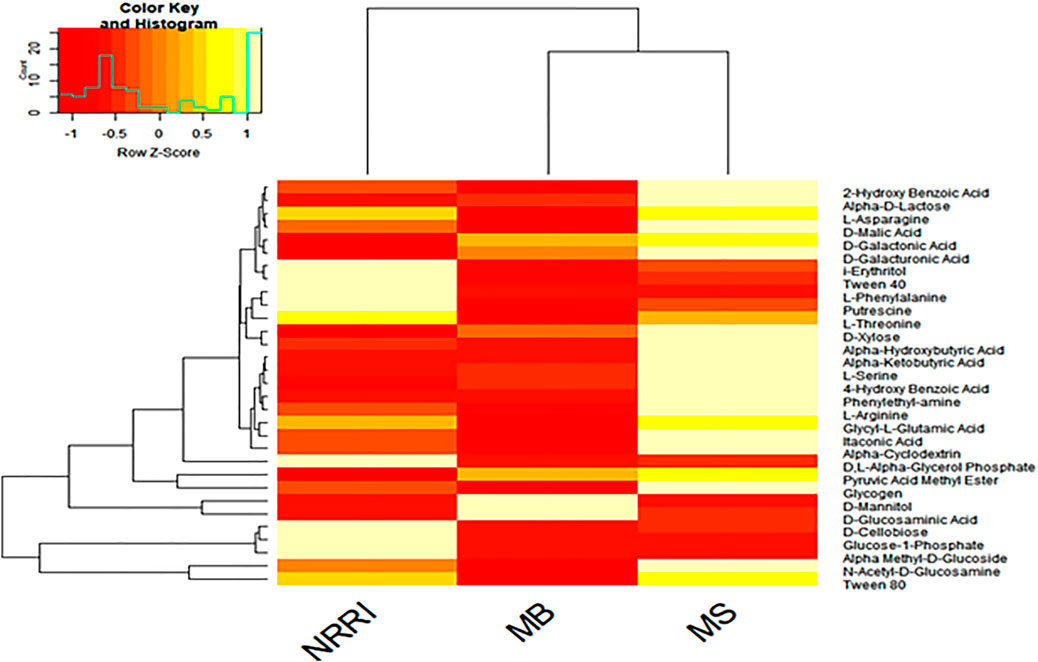
FIGURE 5. Heatmap of carbon source utilization by the microbial community in conventional paddy soil (NRRI) and mangrove-converted paddy soil (MS and MB). NRRI: National Rice Research Institute, Cuttack, Odisha; MS: Bakkhali, Sundarban, West Bengal; MB: Dangamal, Bhitarkanika, Odisha. Light yellow color shows higher and dark red color show lower carbon utilized by the soil microbial community.
Correlation of MS, MB, and NRRI Based on Physico-Chemical and Biological Parameters
A biplot analysis of the parameters obtained from NRRI, MS, and MB was shown in the ordination plane (Figure 6), in which the PCA explained a total variance of 84.17%, with the principal component 1 (PC1) representing 61.80% and principal component 2 (PC2) representing 22.37% variances, respectively. Correlation among the quantified variables demarcated the lines in the same direction being more closely related. Biplot depicts FDA, ACP, and DHA were positively correlated with NRRI (Figure 6). AP, AC, and pH were positively correlated with MS, whereas EC and PSB were positively correlated with MB (Figure 6).
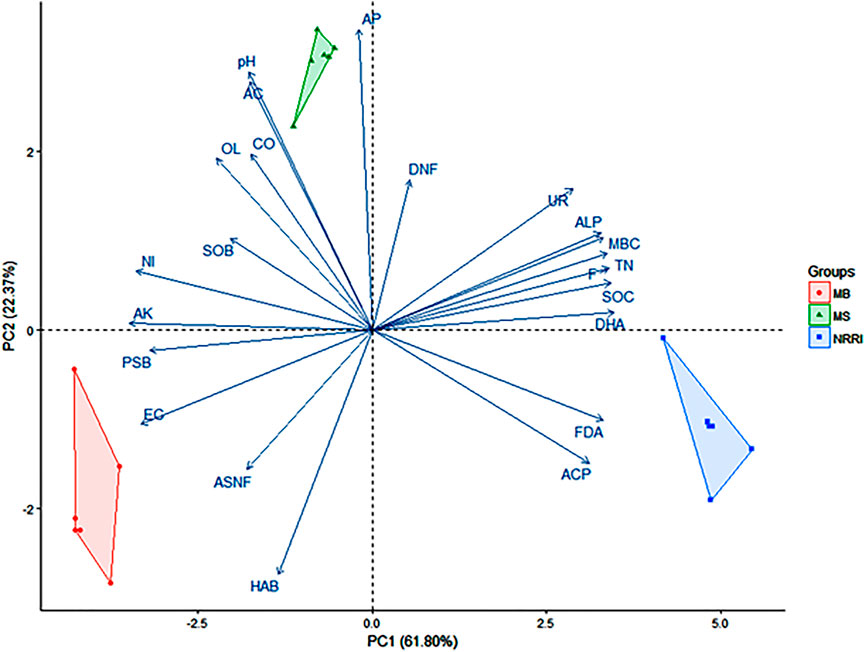
FIGURE 6. Biplot analysis of physico-chemical and biological factors in mangrove-converted and conventional paddy soils. NRRI: National Rice Research Institute, Cuttack, Odisha; MS: Bakkhali, Sundarban, West Bengal; MB: Dangamal, Bhitarkanika, Odisha; MBC: microbial biomass carbon; DHA: dehydrogenase; FDA: fluorescein diacetate; UR: urease; ALP: alkaline phosphatase; ACP: acidic phosphatase; TN: total nitrogen; SOC: soil organic carbon; AK: available potassium; AP: available phosphorus; F: fungi; ASNF: asymbiotic nitrogen fixers; HAB: heterotrophic aerobic bacteria; PSB: phosphate solubilizing bacteria; NI: nitrifiers; SOB: sulfur oxidizing bacteria; OL: oligotrophs; CO: copiotrophs; AC: actinomycetes; DNF: denitrifiers. Values in parentheses indicate percentage contributions by PC1 and PC2.
Discussion
Individual reports on the variation of the soil microbial community under sub-humid tropical mangroves and conventional rice ecology were plenty; however, studies on the conversion of mangrove vegetation into rice cultivation and its effect on the soil microbial community are very limited. Therefore, the present study was initiated to understand the functional microbial community of paddy soil under these habitats. The findings of the present investigation suggested the lower functional microbial diversity (AWCD and rate of change of AWCD) in MS and MB compared to conventionally grown rice under sub-humid tropical conditions. Out of six major C-sources, the majority of the microbes’ under NRRI utilized carbohydrates (a simpler form of sugars) compared to MS and MB, which might be one of the reasons to have a higher diversity of the microbial community in this condition (Huang et al., 1998; Li et al., 2007; Haque et al., 2013; Kumar et al., 2017). In contrast, MS and MB harbored microbial communities consumed complex C-sources such as phenolic compounds, carboxylic compounds, and polymers. Most of the microbes might show a metabolic versatility which permits their existence and survivability under mangrove-converted paddy compared to conventional paddy soil. Carbon utilization pathways in microbes have been investigated for the last 60 years in order to better understand the choice of preferential C-sources utilized by certain bacterial communities in relation to the shift of environmental conditions (Guimarães, 2012; Beisel and Afroz, 2016) as indicated in our study.
Shannon–Weaver and McIntosh indices also showed similar trends of microbial diversity (NRRI > MS > MB), whereas the opposite trend was found in the case of the Simpson index. Shannon–Weaver and McIntosh’s indices are powerful tools to determine the species’ richness and evenness in diverse ecosystems (Shannon and Weaver, 1949; McIntosh et al., 1967; DeJong, 1975; Kennedy and Smith, 1995; Harch et al., 1997). In the present study, lower species’ richness and evenness were found in MS and MB compared to NRRI, and for that reason, the functional diversity of soil microbes was significantly lower in MS and MB. Another possible reason to increase microbial richness in NRRI might be due to the frequent application of organic manures and fertilizers (Dong et al., 2014; Kumar et al., 2017). Besides, in NRRI, rice cultivation was being practiced since 1946 which promoted to build up more organic C at NRRI than MS and MB, possibly favoring a higher species’ richness of bacteria, particularly the carbohydrate utilizers in this condition (Danovaro and Fraschetti, 2002; Pinhey and Tebbs, 2022; Underwood et al., 2022). The Simpson index indicates the dominance of the species community in a particular ecosystem which is inversely proportional to the McIntosh index which is corroborated with our findings.
PCA analysis shows that enzyme activities such as acid phosphatase, dehydrogenase, and fluorescein diacetate are positively correlated with NRRI compared to MS and MB. Suitable soil pH (slightly acidic) favors the production of acidic phosphatase (Dick and Tabatabai, 1984; Gu et al., 2009) in NRRI which helps to increase heterotrophic aerobic bacteria (HAB) by utilizing the different carbohydrate sources in this condition (Kavadia et al., 2007; Shu et al., 2012; Roper and Gupta, 2016). Fluorescein diacetate also showed a positive correlation with NRRI, indicating higher microbial richness as FDA hydrolysis correlates with microbial indices such as ATP content, biomass, and cell density (Jiang et al., 2016). Previous reports indicated that FDA hydrolysis has been found to be significantly correlated with microbial biomass in pure and mixed microbial cultures, pastures, and cultivated soils, and soil amended with municipal refuse, and could be used as an alternative estimate of the content and size of soil microflora (Green et al., 2006; Sánchez-Monedero et al., 2008; Wang et al., 2018).
MS harbored higher CFU of actinomycetes, oligotrophs, sulfur-oxidizing bacteria, and nitrifiers which showed a positive response to available phosphorus (AP), urease, and soil pH. Alkaline pH and higher AP in MS primarily favored the growth of these microbes (Bandyopadhyay and Sarkar, 1987; Das et al., 2011; Halder et al., 2015). The present study also showed the higher CFU of phosphate-solubilizing bacteria in MB compared to MS and NRRI. Reports indicated that the higher phosphate solubilizing microbial diversity occurred in mangrove soil containing a lower amount of AP (Adnan et al., 2017). Researchers also revealed that P was the limiting factor of the microbial diversity and productivity of mangroves ecosystems (Reich and Oleksyn, 2004; Lovelock et al., 2007; Krauss et al., 2008). The present study showed that MB was positively correlated with electrical conductivity (EC). Interestingly, EC was found higher in undisturbed MS and MB which might have hampered the growth of microbial populations such as HAB, NI, and PSB compared to converted MS and MB (Mishra et al., 2012; Chatterjee et al., 2015). The population of AC was more in undisturbed MS than converted MS, possibly due to being halophilic in nature (Ballav et al., 2015). The possibility of high EC might be due to less saline soil as alkalinity is inversely proportional to EC (Mohd-Aizat et al., 2014). Moreover, salinity levels might alter the microbiome under mangrove and mangrove-converted paddy soils, consequently influencing mangrove production (Chambers et al., 2016).
The converted mangroves of both MS and MB showed a negative response to FDA and DHA, this might be due to poor microbial diversity as microbial indices such as biomass, cell density, and ATP content are positively correlated with FDA which might have decreased due to anthropogenic activities in the wetlands of the mangrove ecosystem (Jiang et al., 2016). Conjoining all the results, we found that all the three sites viz., paddy soils of NRRI and converted mangrove paddy soils of MS and MB are distinct ecosystems that differ from each other in terms of microbial diversity, enzymatic, and soil physico-chemical parameters. However, a detailed quantification of biotic (plant taxonomy, anatomy, and sediment fauna) and abiotic (temperature, salinity, tidal amplitude and frequency, and pollution level) variables that can influence the composition and functions of the microbiome is required to achieve a mechanistic understanding under MS and MB compared to NRRI. Overall, our results indicated that rice cultivation under MS and MB alters the microbial diversity compared to NRRI, which might be due to severe anthropogenic activities that gradually destroyed these habitats.
Conclusion
The present study concludes that anthropogenic practices in the coastal wetlands of the mangroves of Sundarban and Bhitarkanika have hindered the microbial diversity of the mangrove ecosystem. However, NRRI, which is a completely rice–rice system where cultivation of rice is being carried out over 70 consecutive years, showed increased microbial richness along with enzymatic and physico-chemical parameters than MS and MB. The decreased concentrations of these parameters in MS and MB might be due to the conversion of these areas into rice cultivating lands. Therefore, the findings of this study suggested the deterioration in the rich mangrove diversity might be due to its conversion to rice cultivation by anthropogenic activities.
Data Availability Statement
The original contributions presented in the study are included in the article/Supplementary Material, further inquiries can be directed to the corresponding author.
Author Contributions
UK framed the notion and was overall in charge of this manuscript preparation. UK and MK collected literature, analyzed data, and drafted the manuscript. PP and AN edited the manuscript. All contributors discussed the outcomes and added them to the final document. All authors have studied and approved the in-print version of the article.
Conflict of Interest
The authors declare that the research was conducted in the absence of any commercial or financial relationships that could be construed as a potential conflict of interest.
Publisher’s Note
All claims expressed in this article are solely those of the authors and do not necessarily represent those of their affiliated organizations, or those of the publisher, the editors, and the reviewers. Any product that may be evaluated in this article, or claim that may be made by its manufacturer, is not guaranteed or endorsed by the publisher.
Acknowledgments
The first author is extremely thankful to the Director, NRRI, Cuttack (Odisha), India for providing financial assistance in the form of institute project and laboratory facility for carrying out this study.
Supplementary Material
The Supplementary Material for this article can be found online at: https://www.frontiersin.org/articles/10.3389/fenvs.2022.858028/full#supplementary-material
References
Acosta-Martínez, V., and Tabatabai, M. A. (2000). Enzyme Activities in a Limed Agricultural Soil. Biol. Fertil. Soils 31 (1), 85–91. doi:10.1007/s003740050628
Adnan, M., Shah, Z., Fahad, S., Arif, M., Alam, M., Khan, I. A., et al. (2017). Phosphate-solubilizing Bacteria Nullify the Antagonistic Effect of Soil Calcification on Bioavailability of Phosphorus in Alkaline Soils. Sci. Rep. 7 (1), 16131. doi:10.1038/s41598-017-16537-5
Alongi, D. M., Christoffersen, P., and Tirendi, F. (1993). The Influence of forest Type on Microbial-Nutrient Relationships in Tropical Mangrove Sediments. J. Exp. Mar. Biol. Ecol. 171 (2), 201–223. doi:10.1016/0022-0981(93)90004-8
Alongi, D. M. (2002). Present State and Future of the World's Mangrove Forests. Envir. Conserv. 29 (3), 331–349. doi:10.1017/s0376892902000231
Arya, S. R., and Syriac, E. K. (2018). Wetlands: The Living Waters-A Review. Agric. Rev. 39 (2). doi:10.18805/ag.r-1717
Atwood, T. B., Connolly, R. M., Almahasheer, H., Carnell, P. E., Duarte, C. M., Ewers Lewis, C. J., et al. (2017). Global Patterns in Mangrove Soil Carbon Stocks and Losses. Nat. Clim Change 7 (7), 523–528. doi:10.1038/nclimate3326
Ballav, S., Kerkar, S., Thomas, S., and Augustine, N. (2015). Halophilic and Halotolerant Actinomycetes from a marine Saltern of Goa, India Producing Anti-bacterial Metabolites. J. Biosci. Bioeng. 119 (3), 323–330. doi:10.1016/j.jbiosc.2014.08.017
Bandyopadhyay, A. K., and Sarkar, D. (1987). Occurrence of Acid saline Soils in Coastal Sundarbans Area of West Bengal. J. Indian Soc. Soil Sci. 35, 542–544.
Banerjee, K., Roy Chowdhury, M., Sengupta, K., Sett, S., and Mitra, A. (2012b). Influence of Anthropogenic and Natural Factors on the Mangrove Soil of Indian Sundarbans Wetland. Arch. Environ. Sci. 6, 80–91.
Banerjee, K., Senthilkumar, B., Purvaja, R., and Ramesh, R. (2012a). Sedimentation and Trace Metal Distribution in Selected Locations of Sundarbans Mangroves and Hooghly Estuary, Northeast Coast of India. Environ. Geochem. Health 34 (1), 27–42. doi:10.1007/s10653-011-9388-0
Behera, P., Mohapatra, M., Kim, J. Y., Adhya, T. K., Pattnaik, A. K., and Rastogi, G. (2019). Spatial and Temporal Heterogeneity in the Structure and Function of Sediment Bacterial Communities of a Tropical Mangrove forest. Environ. Sci. Pollut. Res. 26 (4), 3893–3908. doi:10.1007/s11356-018-3927-5
Beisel, C. L., and Afroz, T. (2016). Rethinking the Hierarchy of Sugar Utilization in Bacteria. J. Bacteriol. 198 (3), 374–376. doi:10.1128/jb.00890-15
Bhattacharyya, A., Haldar, A., Bhattacharyya, M., and Ghosh, A. (2019). Anthropogenic Influence Shapes the Distribution of Antibiotic Resistant Bacteria (ARB) in the Sediment of Sundarban Estuary in India. Sci. Total Environ. 647, 1626–1639. doi:10.1016/j.scitotenv.2018.08.038
Borrell, A., Tornero, V., Bhattacharjee, D., and Aguilar, A. (2016). Trace Element Accumulation and Trophic Relationships in Aquatic Organisms of the Sundarbans Mangrove Ecosystem (Bangladesh). Sci. Total Environ. 545-546, 414–423. doi:10.1016/j.scitotenv.2015.12.046
Bouillon, S., Borges, A. V., CastañedaMoya, E., Diele, K., Dittmar, T., Duke, N. C., et al. (2008). Mangrove Production and Carbon Sinks: a Revision of Global Budget Estimates. Glob. Biogeoch Cycles 22 (2). doi:10.1029/2007gb003052
Bray, R. H., and Kurtz, L. T. (1945). Determination of Total, Organic, and Available Forms of Phosphorus in Soils. Soil Sci. 59 (1), 39–46. doi:10.1097/00010694-194501000-00006
Burnett, G. W., PelczarJr, M. J., and Conn, H. J. (1957). Preparation of media. Man. Microbiol. Methods 2, 37–63.
Campbell, C. D., Grayston, S. J., and Hirst, D. J. (1997). Use of Rhizosphere Carbon Sources in Sole Carbon Source Tests to Discriminate Soil Microbial Communities. J. Microbiol. Methods 30 (1), 33–41. doi:10.1016/s0167-7012(97)00041-9
Chambers, L. G., Guevara, R., Boyer, J. N., Troxler, T. G., and Davis, S. E. (2016). Effects of Salinity and Inundation on Microbial Community Structure and Function in a Mangrove Peat Soil. Wetlands 36 (2), 361–371. doi:10.1007/s13157-016-0745-8
Chatterjee, S. N., Mukhopadhyay, P. R., and Dangar, T. K. (2015). Population Dynamics, Diversity and Characterization of Soil Bacteria in Some South-Eastern Regions of the Sundarbans West Bengala, India. Int. J. Pharma Bio Sci. 6, 353–361.
Chauhan, R., Datta, A., Ramanathan, A., and Adhya, T. K. (2017). Whether Conversion of Mangrove forest to rice Cropland Is Environmentally and Economically Viable? Agric. Ecosyst. Environ. 246, 38–47. doi:10.1016/j.agee.2017.05.010
Chmura, G. L., Anisfeld, S. C., Cahoon, D. R., and Lynch, J. C. (2003). Global Carbon Sequestration in Tidal, saline Wetland Soils. Glob. Biogeochem Cycles 17 (4). doi:10.1029/2002gb001917
Choi, K.-H., and Dobbs, F. C. (1999). Comparison of Two Kinds of Biolog Microplates (GN and ECO) in Their Ability to Distinguish Among Aquatic Microbial Communities. J. Microbiol. Methods 36 (3), 203–213. doi:10.1016/s0167-7012(99)00034-2
Chowdhury, A., Naz, A., Bhattacharyya, S., and Sanyal, P. (2021a). Dynamics of Salinity Intrusion in the Surface and Ground Water of Sundarban Biosphere Reserve, IndiaIOP Conference Series: Earth and Environmental Science. IOP Conf. Ser. Earth Environ. Sci. 944 (1), 012061. doi:10.1088/1755-1315/944/1/012061
Chowdhury, A., Naz, A., and Maiti, S. K. (2021b). Bioaccumulation of Potentially Toxic Elements in Three Mangrove Species and Human Health Risk Due to Their Ethnobotanical Uses. Environ. Sci. Pollut. Res. 28 (25), 33042–33059. doi:10.1007/s11356-021-12566-w
Danovaro, R., and Fraschetti, S. (2002). Meiofaunal Vertical Zonation on Hard-Bottoms: Comparison with Soft-Bottom Meiofauna. Mar. Ecol. Prog. Ser. 230, 159–169. doi:10.3354/meps230159
Das, S., De, M., Ray, R., Ganguly, D., Kumar Jana, T., and De, T. K. (2011). Salt Tolerant Culturable Microbes Accessible in the Soil of the Sundarban Mangrove forest, India. Open J. Ecol. 1 (2), 35. doi:10.4236/oje.2011.12004
Das, S., De, M., Ray, R., Chowdhury, C., Jana, T. K., and De, T. K. (2012). Microbial Ecosystem in Sunderban Mangrove forest Sediment, North-East Coast of Bay of Bengal, India. Geomicrobiology J. 29 (7), 656–666. doi:10.1080/01490451.2011.605988
DeJong, T. M. (1975). A Comparison of Three Diversity Indices Based on Their Components of Richness and Evenness. Oikos 26, 222–227. doi:10.2307/3543712
Der, G., and Everitt, B. S. (2008). A Handbook of Statistical Analyses Using SAS. Chapman and Hall/CRC.
Dick, W. A., and Tabatabai, M. A. (1984). Kinetic Parameters of Phosphatases in Soils and Organic Waste Materials. Soil Sci. 137 (1), 7–15. doi:10.1097/00010694-198401000-00002
Donato, D. C., Kauffman, J. B., Murdiyarso, D., Kurnianto, S., Stidham, M., and Kanninen, M. (2011). Mangroves Among the Most Carbon-Rich Forests in the Tropics. Nat. Geosci 4 (5), 293–297. doi:10.1038/ngeo1123
Dong, W.-Y., Zhang, X.-Y., Dai, X.-Q., Fu, X.-L., Yang, F.-T., Liu, X.-Y., et al. (2014). Changes in Soil Microbial Community Composition in Response to Fertilization of Paddy Soils in Subtropical China. Appl. Soil Ecol. 84, 140–147. doi:10.1016/j.apsoil.2014.06.007
Duarte, C. M., Marbà, N., Gacia, E., Fourqurean, J. W., Beggins, J., Barrón, C., et al. (2010). Seagrass Community Metabolism: Assessing the Carbon Sink Capacity of Seagrass Meadows. Glob. Biogeochem Cycles 24 (4). doi:10.1029/2010gb003793
Duarte, C. M., Middelburg, J. J., and Caraco, N. (2004). Major Role of marine Vegetation on the Oceanic Carbon Cycle. Biogeosci Discuss. 1 (1), 659–679.
Dutta, M. K., Mukherjee, R., Jana, T. K., and Mukhopadhyay, S. K. (2015). Biogeochemical Dynamics of Exogenous Methane in an Estuary Associated to a Mangrove Biosphere; the Sundarbans, NE Coast of India. Mar. Chem. 170, 1–10. doi:10.1016/j.marchem.2014.12.006
Green, V. S., Stott, D. E., and Diack, M. (2006). Assay for Fluorescein Diacetate Hydrolytic Activity: Optimization for Soil Samples. Soil Biol. Biochem. 38 (4), 693–701. doi:10.1016/j.soilbio.2005.06.020
Gu, S., Guo, X., Cai, Y., Zhang, Z., Wu, S., Li, X., et al. (2018). Residue Management Alters Microbial Diversity and Activity without Affecting Their Community Composition in Black Soil, Northeast China. PeerJ 6, e5754. doi:10.7717/peerj.5754
Gu, Y., Wang, P., and Kong, C. H. (2009). Urease, Invertase, Dehydrogenase and Polyphenoloxidase Activities in Paddy Soil Influenced by Allelopathic rice Variety. Eur. J. Soil Biol. 45 (5-6), 436–441. doi:10.1016/j.ejsobi.2009.06.003
Guimarães, L. H. S. (2012). Carbohydrates from Biomass: Sources and Transformation by Microbial Enzymes. Carbohydrates-Comprehensive Stud. Glycobiology Glycotechnology 20, 441–456. Intech: London, UK.
Halder, D., Dutta, P., Mondal, A., and Basu, M. (2015). Isolation and Characterization of Halophilic Bacteria from Sundarban Soil. Int. J. Life Sci. Scientific Res. 2 (4).
Haque, M. M., Kim, S. Y., Pramanik, P., Kim, G.-Y., and Kim, P. J. (2013). Optimum Application Level of winter Cover Crop Biomass as green Manure under Considering Methane Emission and rice Productivity in Paddy Soil. Biol. Fertil. Soils 49 (4), 487–493. doi:10.1007/s00374-012-0766-2
Harch, B. D., Correll, R. L., Meech, W., Kirkby, C. A., and Pankhurst, C. E. (1997). Using the Gini Coefficient with BIOLOG Substrate Utilisation Data to Provide an Alternative Quantitative Measure for Comparing Bacterial Soil Communities. J. Microbiol. Methods 30 (1), 91–101. doi:10.1016/s0167-7012(97)00048-1
Holguin, G., Bashan, Y., Mendoza-Salgado, R. A., Amador, E., Toledo, G., Vazquez, P., et al. (1999). Microbiology of Mangroves, Forests in the Frontier between Land and Sea. Ciencia Desarrollo 25 (144), 26–35.
Holguin, G., Vazquez, P., and Bashan, Y. (2001). The Role of Sediment Microorganisms in the Productivity, Conservation, and Rehabilitation of Mangrove Ecosystems: an Overview. Biol. Fertil. Soils 33 (4), 265–278. doi:10.1007/s003740000319
Huang, Y., Sass, R. L., and Fisher, Jr, F. M. (1998). A Semi‐empirical Model of Methane Emission from Flooded rice Paddy Soils. Glob. Change Biol. 4 (3), 247–268. doi:10.1046/j.1365-2486.1998.00129.x
Insam, H., Amor, K., Renner, M., and Crepaz, C. (1996). Changes in Functional Abilities of the Microbial Community during Composting of Manure. Microb. Ecol. 31 (1), 77–87. doi:10.1007/BF00175077
Jiang, S., Huang, J., Lu, H., Liu, J., and Yan, C. (2016). Optimisation for Assay of Fluorescein Diacetate Hydrolytic Activity as a Sensitive Tool to Evaluate Impacts of Pollutants and Nutrients on Microbial Activity in Coastal Sediments. Mar. Pollut. Bull. 110 (1), 424–431. doi:10.1016/j.marpolbul.2016.06.031
Kathiresan, K. (2000). A Review of Studies on Pichavaram Mangrove, Southeast India. Hydrobiol 430 (1-3), 185–205. doi:10.1023/a:1004085417093
Kavadia, A., Vayenas, D. V., Pavlou, S., and Aggelis, G. (2007). Dynamics of Free-Living Nitrogen-Fixing Bacterial Populations in Antagonistic Conditions. Ecol. Model. 200 (1-2), 243–253. doi:10.1016/j.ecolmodel.2006.07.037
Kennedy, A. C., and Smith, K. L. (1995). Soil Microbial Diversity and the Sustainability of Agricultural Soils. Plant Soil 170 (1), 75–86. doi:10.1007/bf02183056
Kennedy, H., Beggins, J., Duarte, C. M., Fourqurean, J. W., Holmer, M., Marbà, N., et al. (2010). Seagrass Sediments as a Global Carbon Sink: Isotopic Constraints. Glob. Biogeochem Cycles 24 (4). doi:10.1029/2010gb003848
Krauss, K. W., Lovelock, C. E., McKee, K. L., López-Hoffman, L., Ewe, S. M. L., and Sousa, W. P. (2008). Environmental Drivers in Mangrove Establishment and Early Development: a Review. Aquat. Bot. 89 (2), 105–127. doi:10.1016/j.aquabot.2007.12.014
Kumar, U., Kaviraj, M., Panneerselvam, P., Priya, H., Chakraborty, K., Swain, P., et al. (2019b). Ascorbic Acid Formulation for Survivability and Diazotrophic Efficacy of Azotobacter ChroococcumAvi2 (MCC 3432) under Hydrogen Peroxide Stress and its Role in Plant-Growth Promotion in rice (Oryza Sativa L.). Plant PhysiolBiochem. doi:10.1016/j.plaphy.2019.04.003
Kumar, U., Kaviraj, M., Rout, S., Chakraborty, K., Swain, P., Nayak, P. K., et al. (2021). Combined Application of Ascorbic Acid and Endophytic N-Fixing Azotobacter Chroococcum Avi2 Modulates Photosynthetic Efficacy, Antioxidants and Growth-Promotion in rice under Moisture Deficit Stress. Microbiol. Res. 250, 126808.
Kumar, U., Nayak, A. K., Panneerselvam, P., Kumar, A., Mohanty, S., Shahid, M., et al. (2019a). Cyanobiont Diversity in Six Azolla Spp. And Relation to Azolla-nutrient Profiling. Planta 249, 1435–1447. doi:10.1007/s00425-019-03093-7
Kumar, U., Nayak, A. K., Sahoo, S., Kumar, A., Kaviraj, M., and Shahid, M. (2020b). Combined Effects of Elevated CO2, N Fertilizer and Water Deficit Stress on Diazotrophic Community in Sub-humid Tropical Paddy Soil. Appl. Soil Ecol. 155, 1–12. doi:10.1016/j.apsoil.2020.103682
Kumar, U., Behera, S., Saha, S., Das, D., Guru, P. K., Kaviraj, M., et al. (2020a). Non-target Effect of Bispyribac Sodium on Soil Microbial Community in Paddy Soil. Ecotoxicology Environ. Saf. 189, 110019. doi:10.1016/j.ecoenv.2019.110019
Kumar, U., Kumar Nayak, A., Shahid, M., Gupta, V. V. S. R., Panneerselvam, P., Mohanty, S., et al. (2018). Continuous Application of Inorganic and Organic Fertilizers over 47 Years in Paddy Soil Alters the Bacterial Community Structure and its Influence on rice Production. Agric. Ecosyst. Environ. 262, 65–75. doi:10.1016/j.agee.2018.04.016
Kumar, U., Shahid, M., Tripathi, R., Mohanty, S., Kumar, A., Bhattacharyya, P., et al. (2017). Variation of Functional Diversity of Soil Microbial Community in Sub-humid Tropical rice-rice Cropping System under Long-Term Organic and Inorganic Fertilization. Ecol. Indicators 73, 536–543. doi:10.1016/j.ecolind.2016.10.014
Kutzner, B., and Buchenauer, H. (1986). Effect of Various Triazole Fungicides on Growth and Lipid Metabolism of Fusarium Moniliforme as Well as on Gibberellin Contents in Culture Filtrates of the Fungus. J. Plant Dis. Protect, 597–607.
Li, F., Liu, M., Li, Z., Jiang, C., Han, F., and Che, Y. (2013a). Changes in Soil Microbial Biomass and Functional Diversity with a Nitrogen Gradient in Soil Columns. Appl. Soil Ecol. 64, 1–6. doi:10.1016/j.apsoil.2012.10.006
Li, L., Zhang, X., Zhang, P., Zheng, J., and Pan, G. (2007). Variation of Organic Carbon and Nitrogen in Aggregate Size Fractions of a Paddy Soil under Fertilisation Practices from Tai Lake Region, China. J. Sci. Food Agric. 87 (6), 1052–1058. doi:10.1002/jsfa.2806
Li, S., Lü, T., Zhang, X., Gu, G., and Niu, Y. (2013b). Effect of Trichoderma Longbrachiatum T2 on Functional Diversity of Cucumber Rhizomicrobes. J. Environ. Biol. 34 (2), 293–299.
Lovelock, C. E., Feller, I. C., Ball, M. C., Ellis, J., and Sorrell, B. (2007). Testing the Growth Rate vs. Geochemical Hypothesis for Latitudinal Variation in Plant Nutrients. Ecol. Lett. 10 (12), 1154–1163. doi:10.1111/j.1461-0248.2007.01112.x
Ma, Y., Chang, Z. Z., Zhao, Z. T., Wang, X. M., Huang, H. Y., Ye, X. M., et al. (2006). Study on Microbial Communities Characteristics at Germination Stage of Cucumber Seeds. Plant Nutr. Fertilizer Sci. 12, 109–114.
Magurran, A. E. (1988). “Choosing and Interpreting Diversity Measures,” in Ecological Diversity and its Measurement (Dordrecht: Springer), pp61–80. doi:10.1007/978-94-015-7358-0_4
Manjunath, M., Kumar, U., Yadava, R. B., Rai, A. B., and Singh, B. (2018). Influence of Organic and Inorganic Sources of Nutrients on the Functional Diversity of Microbial Communities in the Vegetable Cropping System of the Indo-Gangetic plains. Comptes Rendus Biologies 341, 349–357. doi:10.1016/j.crvi.2018.05.002
Maria, G. L., and Sridhar, K. R. (2002). Richness and Diversity of Filamentous Fungi on Woody Litter of Mangroves along the West Coast of India. Curr. Sci., 573–1580.
McIntosh, R. P. (1967). An index of Diversity and the Relation of Certain Concepts to Diversity. Ecology 48 (3), 392–404.
Mcleod, E., Chmura, G. L., Bouillon, S., Salm, R., Björk, M., Duarte, C. M., et al. (2011). A Blueprint for Blue Carbon: toward an Improved Understanding of the Role of Vegetated Coastal Habitats in Sequestering CO 2. Front. Ecol. Environ. 9 (10), 552–560. doi:10.1890/110004
Mishra, R. R., Swain, M. R., Dangar, T. K., and Thatoi, H. (2012). Diversity and Seasonal Fluctuation of Predominant Microbial Communities in Bhitarkanika, a Tropical Mangrove Ecosystem in India. Revista de biologia Trop. 60 (2), 909–924. doi:10.2307/1932674
Mohd-Aizat, A., Mohamad-Roslan, M. K., Sulaiman, W. N. A., and Karam, D. S. (2014). The Relationship between Soil pH and Selected Soil Properties in 48 Years Logged-Over forest. Int. J. Environ. Sci. 4 (6), 1129.
Nellemann, C., and Corcoran, E. (2009). Blue Carbon: The Role of Healthy Oceans in Binding Carbon: a Rapid Response Assessment. UNEP/Earthprint.
Ochs, C. A., Cole, J. J., and Likens, G. E. (1995). Population Dynamics of Bacterioplankton in an Oligotrophic lake. J. Plankton Res. 17 (2), 365–391. doi:10.1093/plankt/17.2.365
Piper and CS (1966). Soil and Plant Analysis; A Laboratory Manual of Methods for the Examination of Soils and the Determination of the Inorganic Constituents of Plants. Bombay: Hans Publications.
Pradhan, S. K., Kumar, U., Singh, N. R., and Thatoi, H. (2019). Functional Diversity and Metabolic Profile of Microbial Community of Mine Soils with Different Levels of Chromium Contamination. Int. J. Environ. Health Res. 30, 461–473. doi:10.1080/09603123.2019.1601686
Priyadarshini, S. (2015). Climate Change Pushing Sundarban Farmers into ‘awkward Jobs. Nat. India. doi:10.1038/nindia.2015
Ramesh, R., Lakshmi, A., Sappal, S. M., S.R., B., Suganya, M. D., Ganguly, D., et al. (2019). “Integrated Management of the Ganges Delta, India,” in Coasts and Estuaries (Elsevier), 187–211. doi:10.1016/b978-0-12-814003-1.00011-3
Reich, P. B., and Oleksyn, J. (2004). Global Patterns of Plant Leaf N and P in Relation to Temperature and Latitude. Proc. Natl. Acad. Sci. U.S.A. 101 (30), 11001–11006. doi:10.1073/pnas.0403588101
Roper, M. M., and Gupta, V. V. S. R. (2016). Enhancing Non-symbiotic N2 Fixation in Agriculture. Open Agric. J. 10 (1). doi:10.2174/1874331501610010007
Sahu, S. C., Suresh, H. S., Murthy, I. K., and Ravindranath, N. H. (2015). Mangrove Area Assessment in India: Implications of Loss of Mangroves. J. Earth Sci. Clim. Chang 6 (5), 1.
Sánchez-Monedero, M. A., Mondini, C., Cayuela, M. L., Roig, A., Contin, M., and De Nobili, M. (2008). Fluorescein Diacetate Hydrolysis, Respiration and Microbial Biomass in Freshly Amended Soils. BiolFertSoils 44 (6), 885–890.
Sharma, A., and Dangar, T. K. (2016). Studies on the Microbial Diversity on the Soil of Ooty Botanical Garden. J. Sci Lett 1 (3), 96–101.
Shu, W., Pablo, G. P., Jun, Y., and Danfeng, H. (2012). Abundance and Diversity of Nitrogen-Fixing Bacteria in Rhizosphere and Bulk Paddy Soil under Different Duration of Organic Management. World J. Microbiol. Biotechnol. 28 (2), 493–503. doi:10.1007/s11274-011-0840-1
Strong, A., and Minnemeyer, S. (2015). Satellite Data Reveals State of the World’s Mangrove Forests. World Resources Institute. Retrieved from http://www.wri.org/blog/2015/02/satellite-data-reveals-state-world’s-mangrove-forests.
Subbaiah, B. V. (1956). A Rapid Procedure for Estimation of Available Nitrogen in Soil. Curr. Sci. 25, 259–260.
Thatoi, H., Behera, B. C., Mishra, R. R., and Dutta, S. K. (2013). Biodiversity and Biotechnological Potential of Microorganisms from Mangrove Ecosystems: a Review. Ann. Microbiol. 63 (1), 1–19. doi:10.1007/s13213-012-0442-7
Thomas, N., Lucas, R., Bunting, P., Hardy, A., Rosenqvist, A., and Simard, M. (2017). Distribution and Drivers of Global Mangrove forest Change, 1996-2010. PLoS One 12 (6), e0179302. doi:10.1371/journal.pone.0179302
Tripathi, R., Shukla, A. K., Shahid, M., Nayak, D., Puree, C., Mohanty, S., et al. (2016). Soil Quality in Mangrove Ecosystem Deteriorates Due to rice Cultivation. Ecol. Eng. 90, 163–169. doi:10.1016/j.ecoleng.2016.01.062
Twilley, R. R., Chen, R. H., and Hargis, T. (1992). Carbon Sinks in Mangroves and Their Implications to Carbon Budget of Tropical Coastal Ecosystems. Water Air Soil Pollut. 64 (1-2), 265–288. doi:10.1007/978-94-011-2793-6_15
Underwood, G. J. C., Dumbrell, A. J., McGenity, T. J., McKew, B. A., and Whitby, C. (2022). The Microbiome of Coastal Sediments. The Mar. Microbiome, 479–534. doi:10.1007/978-3-030-90383-1_12
United Nations Framework on Climate Change (2012). Land-Use Change and Forestry. online. Available: http://unfccc.int/methods_and_science/lulucf/items/3060.php (accessed on June 12, 2012).
Vance, E. D., Brookes, P. C., and Jenkinson, D. S. (1987). An Extraction Method for Measuring Soil Microbial Biomass C. Soil Biol. Biochem. 19 (6), 703–707. doi:10.1016/0038-0717(87)90052-6
Wang, T., Yuan, Z., and Yao, J. (2018). A Combined Approach to Evaluate Activity and Structure of Soil Microbial Community in Long-Term Heavy Metals Contaminated Soils. Environeng Res. 23 (1), 62–69.
Wei, Y., Yu, L.-F., Zhang, J.-C., Yu, Y.-C., and Deangelis, D. L. (2011). Relationship between Vegetation Restoration and Soil Microbial Characteristics in Degraded Karst Regions: a Case Study. Pedosphere 21, 132–138. doi:10.1016/s1002-0160(10)60088-4
Keywords: mangrove, biolog ECO plates®, microbial community, soil enzymes, rice
Citation: Kumar U, Kaviraj M, Panneerselvam P and Nayak AK (2022) Conversion of Mangroves Into Rice Cultivation Alters Functional Soil Microbial Community in Sub-Humid Tropical Paddy Soil. Front. Environ. Sci. 10:858028. doi: 10.3389/fenvs.2022.858028
Received: 19 January 2022; Accepted: 28 March 2022;
Published: 05 May 2022.
Edited by:
Jehangir Bhadha, University of Florida, United StatesReviewed by:
Amit Kumar, Nanjing University of Information Science and Technology, ChinaAbhiroop Chowdhury, O.P. Jindal Global University, India
Copyright © 2022 Kumar, Kaviraj, Panneerselvam and Nayak. This is an open-access article distributed under the terms of the Creative Commons Attribution License (CC BY). The use, distribution or reproduction in other forums is permitted, provided the original author(s) and the copyright owner(s) are credited and that the original publication in this journal is cited, in accordance with accepted academic practice. No use, distribution or reproduction is permitted which does not comply with these terms.
*Correspondence: Upendra Kumar, ukumarmb@gmail.com, orcid.org/0000-0002-9246-3920