Hedge and Alder-Based Agroforestry Systems: Potential Interventions to Carbon Sequestration and Better Crop Productivity in Indian Sub-Himalayas
- 1ICAR-Indian Institute of Rice Research, Hyderabad, India
- 2ICAR-Indian Institute of Soil Science, Bhopal, India
- 3Bihar Agricultural University, Bhagalpur, India
- 4KVK, Balumath, Latehar, India
- 5Acharya Narendra Deva University of Agriculture and Technology, Ayodhya, India
- 6ICAR-National Rice Research Institute, Cuttack, India
Agroforestry systems (AFSs) have potential to combat climate change and to ensure food security. AFSs can sequester carbon and amend the organic matter, thereby enhancing the crop productivity. Carbon sequestration depends on the type of AFSs, climate, cropping pattern, and management practices. The aim of this study was to evaluate different AFSs for their potential to sequester carbon and impact on soil organic matter (SOM) in the eastern sub-Himalayas, India. Hedge-, alder-, and guava-based AFSs were established along with control (without any tree), and the maize–mustard–potato cropping pattern was followed in each AFS. Soil samples were collected after the fifth crop cycle and further analyzed. The results showed that crop productivity was significantly higher in all the AFSs than control. On average, soil organic carbon (SOC) was found to be significantly higher by 62 and 64% in hedge-based AFSs as compared to guava-based AFSs and control, respectively, and at par with alder-based AFSs. Particulate organic carbon (POC) was higher in all the three AFSs than in the control. For microbial biomass carbon (MBC) and microbial biomass nitrogen (MBN) contents, the trend of AFSs was expressed as alder-based AFS > hedge-based AFS > guava-based AFS > control. Hedge- and alder-based AFSs had higher SOC stocks than guava-based AFSs and control. Carbon dioxide equivalent (CO2 eq.) emissions were greater in control than hedge-based AFSs (35.2 Mg ha−1), followed by alder-based AFSs (28.6 Mg ha−1), and the lowest was observed in guava-based AFSs. On an average, hedge species accumulated more nitrogen (N), phosphorus (P), and potassium (K), which were 60, 12, and 28 kg ha−1 yr−1, respectively. This conclusively proved that AFSs were significantly affecting SOM pools and crop productivity and had a significant role in carbon retention in the soil. Overall, hedge- and alder-based AFSs retained higher soil carbon, and hence, hedge- and alder-based AFSs may be promoted to achieve climate-smart agriculture practices in the acid soils of the Indian sub-Himalayan region.
1 Introduction
The Indian sub-Himalayas occupy around 25 million hectares (m ha), representing 8% of the total land area of India (IFSR, 2019; Nath et al., 2021). The region is environmentally sensitive where landscape, hydrology, and fertility are threatened by climate change and human population (Saini, 2008). Moreover, exploitation of natural resources and shifting cultivation have caused severe land degradation, resulting in a sharp decline in crop productivity (Laskar et al., 2020; Nath et al., 2020; Nath et al., 2021). Several strategies have been recommended for achieving ecological stability and resources conservation together with sustainable crop production. In this direction, agroforestry interventions have been found to bear immense potential and proven with capacity to reduce soil erosions, conserve SOM, improve soil physical characteristics, and further enhance nutrient cycling (Bhatt and Bujarbaruah, 2006; Ramachandran Nair et al., 2010; Ollinaho and Kröger, 2021). AFSs are believed to have a higher potential to sequester carbon and climate change mitigation options (Murthy et al., 2013; Feliciano et al., 2018). Figure 1 shows the carbon stocks in AFSs under different states of India. The sub-Himalayan region has lower carbon stocks in AFSs than the southern states, and there is a huge potential to encourage carbon stocks in AFSs in the sub-Himalayan region.
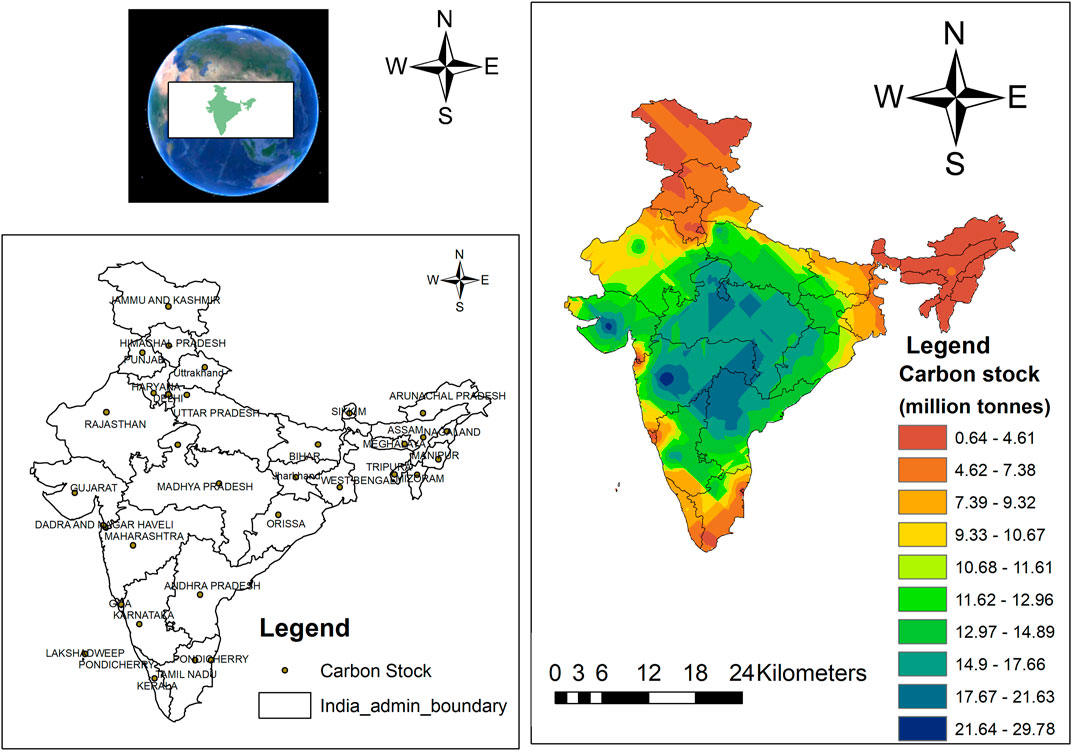
FIGURE 1. Region-wise carbon stocks under Indian agroforestry systems (Source: FSI, 2013).
AFSs are one of the effective land use systems that ensure food and nutritional security (MEA, 2005; Dagar et al., 2020), resilience to climate change and environmental security (Albrecht and Kandji, 2003), and restoration of degrading landscapes (Dagar et al., 2020). Additionally, AFSs enhance the soil quality (Ramos et al., 2015) and conserve ecosystem and biodiversity (Asbjornsen et al., 2014; Hernández et al., 2014). Currently, India is focusing on increasing acreage under crops and forests, and hence, increasing the agroforestry area to 53 m ha by 2050 by utilizing fallow lands, problematic soils (acid soils, alkali soils, saline soils, calcareous soils, acid sulfate soils, and degraded soils), and pasture lands (Dhyani et al., 2013; Dagar and Tewari, 2016; Dagar and Tewari, 2017; Nath et al., 2021). To implement AFSs as a practical tool for utilizing problematic soils and degraded landscape, there is a need for appropriate policies, strategies, national plans, programs, and viable long-term projects.
Alder (Alnus nepalensis)-based AFSs are an age-old ecological agricultural practice which are highly remunerative and grow well in the sub-Himalayan region, but improper handling can result in low crop productivity of the land (Yano and Lanusosang, 2013; Kehie and Khamu, 2018). Different types of fruit trees are used under AFSs. Guava-based AFSs have been found as an appropriate agroforestry model for both Charland and plainland ecosystems (Ahmed et al., 2018). However, trees in the AFSs can be replaced with hedges. In rainfed agriculture, hedge species have suitably helped in soil conservation and sustainable crop production (Kiepe, 1995; Angima et al., 2000). All such benefits that can be derived from hedge species and multipurpose trees have made them integral components of hill farming systems (Bray and Gorham, 1964; Staelens et al., 2003; Bhattacharyya et al., 2009a; Bhattacharyya et al., 2009b).
Improper soil management and land use have led to SOC loss and can cause carbon emissions and affect soil quality in the Indian region (Lal, 2004; Padbhushan et al., 2015; Padbhushan et al., 2016a; Padbhushan et al., 2016b; Rakshit et al., 2018; Sharma et al., 2019; Padbhushan et al., 2021; Padbhushan et al., 2022). Conversely, proper soil management and land use can increase the SOC and further improve soil quality that can partly mitigate the increase in CO2 in the atmosphere (Paustian et al., 1997; Lal and Bruce, 1999; Lal, 2004; Padbhushan et al., 2020; Juhi et al., 2022). Land use systems such as AFS practices and technologies and their service functions have several benefits and hence were adopted as the integral components of mountain farming (Majumdar et al., 2004). However, to better understand the benefits from AFSs, it is imperative to know their potential to augment carbon and N contents in different soil depths since they will provide the baseline information on supplementation of nutrients to crops of varying durations. Furthermore, studies on carbon and N contents as affected by different AFSs would give a valuable insight on the utilization of hedgerow species for soil nutrient enrichment and leaf mulching. Understanding the magnitude of improvement in SOM pools due to AFSs and soil management in subtropical environments, especially the sub-Himalayan region, is limited. Therefore, the impact of land use and microclimate needs to be monitored explicitly to understand the SOM pools and land suitability for different AFSs (Smith, 2010; Padbhushan et al., 2020). This is particularly true for an acid soil of the Indian Himalayas as the information on the impacts of different AFSs on SOC retention is very low under that sort of agro-ecological conditions. Furthermore, the mechanisms of carbon sequestration can reveal important information on differences in carbon fractions under different land use practices (Six et al., 2002; Padbhushan et al., 2020; Padbhushan et al., 2022 and Kumar et al., 2022). A rapid accumulation of carbon from POC has been observed in different land use management systems, which may be an early indicator of change in carbon dynamics (Franzluebbers and Stuedemann, 2009; Sahoo et al., 2019).
Keeping this fact in view, this study was designed to establish the impact of growing various AFSs on SOM pools and carbon stocks in the eastern part of the Indian sub-Himalayas. Presuming that the biomass accumulated by the trees and hedges in the AFSs, it was hypothesized that AFSs would have a considerably higher SOC content and active pools forming the soil profile. More specific objectives of this study are to 1) identify suitable AFSs that can be implemented in the potential interventions for the sub-Himalayan region in India, 2) quantify SOM pools in different AFSs and carbon sequestration, and 3) quantify crop productivity in different AFSs.
2 Materials and Methods
2.1 Experimental Location
The study was conducted in the research farm located in the eastern sub-Himalayan region with 25o40′N latitude to 91°60′E longitude and altitude ranging from 900 to 950 m above mean sea level (Figure 2). The climate of this region is humid subtropical, and mean rainfall is around 240 cm annually. About 90% of the overall precipitation is received during months of April–October. The minimum and the maximum temperature prevailed in the region are during the month of January (6.1°C) and June (29.2°C), respectively. The soil of the experimental site is sandy loam textured (acidic Alfisol) and deficient in phosphorus (Majumdar et al., 2004). The AFS interventions were introduced in about 10 ha fallow area. The average soil depth was >1.0 m, and the slope of the area ranged from 6.4 to 6.8%. Contour bunding was followed for conserving soil and water along the slope into terraces at a vertical interval of 3 m (Singh et al., 1990).
2.2 Experimental Details
Out of the total areas of the 10 ha land, a 1.35 ha portion was selected and divided into small plots, each of 500 m2 area. A randomized block design (RBD) for 27 plots with nine sets of management and three replications each was prepared. These nine sets included six hedge species, namely, Cajanus cajan, Indigofera tinctoria, Desmodium rensonii, Crotalaria tetragona, Flemingia microphylla, and Tephrosia candida; one multipurpose tree (Himalayan alder; Alnus nepalensis); one fruit tree (Guava; Psidium guajava cv. Allahabad safeda); and one control (without tree) (Supplementary Figure S1). Hedge species were planted in thick rows on contour bunds, and Himalayan alder and guava were planted on contours across the slope at spacing 5 m × 5 m. Plots were made at a similar slope of an average of 6.6% for proper light exposure.
Plant density for hedge species ranged from 773.0 ± 10.5 to 833.0 ± 12.5 hedge plot−1 with highest numbers of Crotalaria tetragona and lowest numbers of Tephrosia candida. For Himalayan alder and guava trees, 30 seedlings were accommodated in each plot. Initially, at the time of planting, a uniform basal dose of 0.5 kg diammonium phosphate and 10.0 kg farmyard manure (FYM) were applied in each pit (60 cm × 60 cm × 75 cm). Three plots were left for sole cropping (without a tree) to serve as control plots.
2.3 Crop Cultivation
Maize (Zea mays; cultivar Vijay composite) was grown in the kharif (rainy) season and was followed by the cultivation of mustard (Brassica campestris; cultivar M-27) and potato (Solanum tuberosum; cultivar Kufri Jyoti) in the rabi (winter) season in all plots, including the control plots for year-round cultivation throughout the period of experiment. Conventional tillage (plowing up to 15 cm soil layer) was practiced in all plots before the sowing of crops. Maize was sown in the last week of April in each year (spacing 50 cm × 25 cm) and harvested by the last week of September. The recommended dose of NPK (kg ha−1) for maize crop was 80, 60, and 40, respectively. Thereafter, mustard was sown in the same plots (spacing 30 cm × 10 cm) in the second week of October and harvested in last week of December. The recommended fertilizer dose for mustard crop was 60 kg N ha−1, 60 kg P ha−1, and 40 kg K ha−1, respectively. Potato was sown in the second week of January (spacing 60 cm × 15 cm) and harvested in the first week of March every year. Recommended fertilizer dose (NPK, kg ha−1) for potato crop was 100, 100 and 150, respectively. Whole plot yield was considered to calculate yield per hectare (Semwal and Maikhuri, 1996). Thus, the crop productivity of the initial years was discarded. In each plot of guava and alder, crop productivity of the first 10 plants from any border was discarded to avoid the border effects (Puri and Nair, 2004). Vegetation growth was estimated using the quadrat estimation method, as proposed by Hanley (1978) and Bonham (1989). Biological yield was calculated after the harvest of the crop as per the standards of yield parameter calculations.
2.4 Soil Sample and Analysis
The soil sampling was done for all six hedge species from all the plots in three replications from soil depth (0–30 cm, at the depths 10-cm interval). Thus, a total of 18 (6 hedges species × 3 replications × 1 location) soil samples were collected from each soil depth layer in the plots under hedge-based AFSs. For statistical analysis, composite soil samples were collected from six hedge species treated as a replication. In a similar way, soil samples were collected from six locations in each land use system (hedge-based AFS, alder-based AFS, guava-based AFS, and control). The distances between the places where samples were collected and the tree trunks were 60–80 cm. The soils collected from every land use were mixed thoroughly, and representative soil samples were used for analysis. The representative soil samples were air-dried, sieved (through a 2-mm sieve), mixed, and then stored in sealed plastic jars. Various physical and chemical properties of representative subsamples were determined using standard methods (Page et al., 1982). Soil pH was estimated by using the Jackson method (1973). SOC was estimated by Walkley and Black (1934). Available N, P, and K in the soil were determined by using the method suggested by Page et al. (1982). Bulk density was estimated by using the core sampler method of Piper (1950). Available K in the soil was measured by using a flame photometer, and N by using the Kjeldahl method. Total N (TN) was estimated by Kjeldahl (1883). Mean (for three soil samples) initial values for soil pH, Walkley–Black carbon (WBC), available N, P, K, Ca, and Mg were 4.45 ± 0.10, 1.65 ± 0.12%, 275.0 ± 6.50 kg ha−1, 3.20 ± 0.21 kg ha−1, 297.0 ± 5.94 kg ha−1, 0.51 ± 0.04 meq 100 g−1, and 0.39 ± 0.01 meq 100 g−1, respectively.
2.5 Soil Organic Matter Fractionation Study
2.5.1 Microbial Biomass Carbon (MBC) and Microbial Biomass Nitrogen (MBN)
Witt et al. (2000) proposed the modified chloroform fumigation extraction method to determine the MBC in fresh soil samples. The standard equation used to calculate MBC (mg kg−1) is given as follows:
MBN was determined by using the fumigation method as proposed by Joergensen and Olfs (1998). The standard 2equation used to calculate MBN (mg kg−1) is given as follows:
2.5.2 Particulate Organic Matter Carbon
A little modification (Six et al., 2002) was made to the method described by Cambardella and Elliott (1992) in order to determine POC and particle size distribution.
2.5.3 Capacity Level of Soil
The capacity level of soils to preserve carbon associated with silt and clay particles was proposed by Hassink (1997). The soil sample was sieved through an 8 mm mesh sieve; roots and stubbles were removed, and further sample was analyzed. The soil sample weighing 50 g was suspended in 250 ml distilled water for 24 h. The sample was treated ultrasonically for 15 min. Dispersed soil suspension was dried for 24 h in an oven at 105°C and ground for analyzing total carbon.
Other formulas used in this study were as follows:
1) SOC stocks (Mg ha−1) (Datta et al., 2015)
2) Microbial quotient (MQ, %) (McGonigle and Turner, 2017)
3) SOC stocks loss (Mg ha−1), CO2 eq. emission (Mg ha−1) and relative SOC stocks loss (%) (Padbhushan et al., 2020)
4) Soil carbon sequestration (Mg C ha−1) is given by
2.6 Nutrient Content in Leaf Litter
Leaf litter samples were collected from AFSs (hedge-, alder-, and guava-based AFSs). Biomass production was determined using the quadrat method (Hanley, 1978; Bonham, 1989). A total of forty 0.1 m2 (0.2 m × 0.5 m) quadrats, with systematic spacing at an interval of 1.5 m along two parallel 30 m lines which are 3 m apart, were considered under this method. The canopy coverage was calculated within 6-cover classes (0–5%, 5–25%, 25–50%, 50–75%, 75–95%, and 95–100%) and the mid-point of every class was used to estimate the average. This method minimizes the error in biomass yield estimation. The nutrient content (NPK) was determined in the dried leaf litter sample, as proposed by Jackson (1973). Nutrient components in dried leaf litter leads to evaluation of nutrient accumulation.
2.7 Statistical Analysis
All soil and plant parameters were analyzed by using analysis of variance (ANOVA) for a randomized block design (RBD). Tukey’s honestly significant difference test (Tukey’s HSD test) was used as a post hoc mean separation test using software Statistical Analysis System 9.1 (SAS Institute, United States) for representing the significance of ANOVA at p < 0.05. Linear regression and correlation were done to understand the relationship within and between carbon fractions and soil properties.
3 Results
3.1 Growth Performance and Crop Productivity of Agroforestry Systems in the Indian Sub-Himalayas
Survival percentage in the guava-based AFS was significantly (p < 0.05) higher than that in the alder-based AFS and non-significantly at par with the hedge-based AFS (Table 1). Plant density per hectare was significantly higher in the hedge-based AFS than in alder- and guava-based AFSs (Table 1). On comparing plant height, its maximum height was obtained in the alder-based AFS which was significantly higher than the hedge-based AFS and non-significantly at par the guava-based AFS (Table 1). The collar diameter was significantly lower in hedge- and guava-based AFSs.
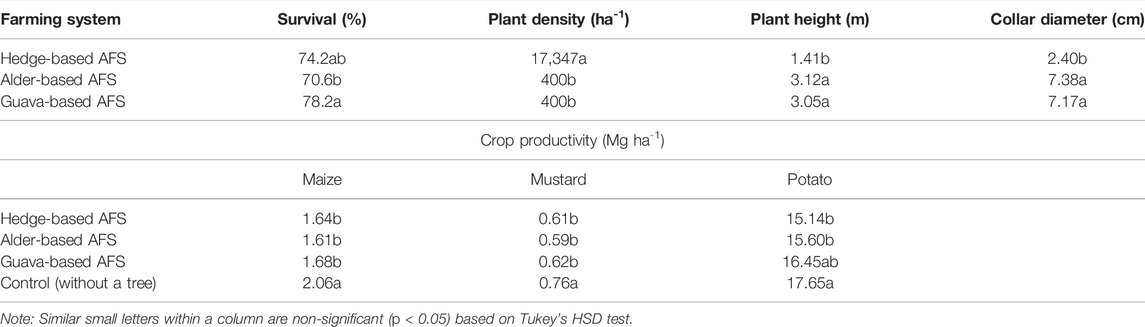
TABLE 1. Growth performance and crop productivity in different agroforestry systems of the Indian sub-Himalayas.
The maize yield was significantly (p < 0.05) higher in the control (plots without a tree) than in all AFS-treated plots, which were statistically at par with one other (Table 1). Compared to AFSs, on average, control had 16, 22, and 18% more maize yield than hedge-, alder-, and guava-based AFSs, respectively. Similar trend was found in the crop productivity of mustard. Compared to AFSs, on average, the control had 20, 22, and 18% more mustard yield than hedge-, alder-, and guava-based AFSs, respectively. Crop productivity of potato was significantly higher in the control than in the hedge- and alder-based AFSs but was statistically at par with the guava-based AFS (Table 1). The yield of potato in hedge-, alder-, and guava-based AFSs was statistically similar (Table 1). The potato yield was 14, 12, and 7% more in the control than in hedge-, alder-, and guava-based AFSs, respectively.
3.2 Soil Properties in Different Agroforestry Systems
All the land use systems have strong acidity in soil reaction. No significant effect was observed in soil pH under different AFSs compared to control (Table 2). The available N content was significantly higher in the hedge- and alder-based AFSs than in the control. The guava-based AFS was at par with control treatment (Table 2). The available P content was similar in the treated alder-based AFS and control but statistically at par with the hedge-based AFS, however significantly higher than the guava-based AFS. The alder-based AFS had approximately 25% higher available P than the guava-based AFS (Table 2). The available K content was the highest under the hedge-based AFS in comparison with the control; however, other AFSs (alder- and guava-based AFSs) were statistically at par with one another (Table 2). The total N was found to be greater in hedge- and alder-based AFSs and control than in the guava-based AFS. Among treatments, the range of total N was 1.4–1.9 g kg−1 (Table 2).

TABLE 2. Effect of various agro-forestry systems on soil properties in the soil depth (0–30 cm) Indian sub-Himalayas.
3.3 Soil Organic Carbon and Its Stocks and Organic Matter Fractions in Different Agroforestry Systems
The hedge-based AFS had significantly higher SOC than the guava-based AFS and control plot (Figure 3). SOC in the hedge-based AFS was increased by 62 and 64% in comparison to the guava-based AFS and control plots, respectively. Alder- and hedge-based AFSs had a similar SOC content. SOC in the alder-based AFS was increased by 61 and 59% in comparison to the guava-based AFS and control plots, respectively. SOC stocks showed a similar trend to SOC among AFSs (Figure 4). SOC stocks were 10 and 8 Mg ha−1 higher in the hedge- and alder-based AFSs, respectively, than in the control. Supplementary Figure S2 showed that SOC stocks were 21 and 22% higher in the subsurface layer (20–30 cm) than in the surface layer (0–10 cm) in case of hedge- and alder-based AFSs, respectively. Supplementary Figure S2 also showed cumulative SOC stocks, and it was more in the hedge-based AFS, followed by the alder-based AFS, than in the guava-based AFS and control (without a tree).
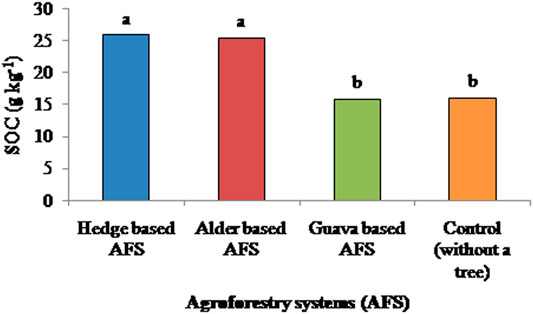
FIGURE 3. Effects of various agroforestry systems on soil organic carbon (SOC) in the soil depth of 0–30 cm of the Indian sub-Himalayas. (Means of different land use systems within a column followed by similar lowercase letters are not significant at p < 0.05 based on Tukey’s HSD test.)
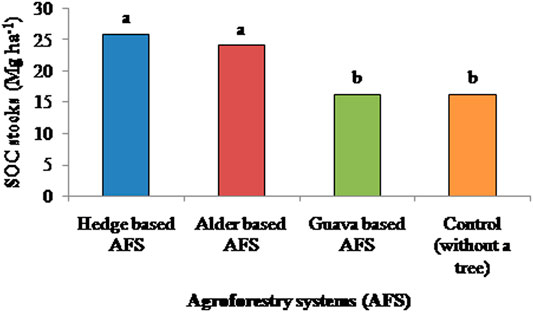
FIGURE 4. Effects of various agroforestry systems on soil organic carbon stocks (SOC stocks) in the soil depth of 0–30 cm of the Indian sub-Himalayas. (Means of different land use systems within a column followed by similar lowercase letters are not significant at p < 0.05 based on Tukey’s HSD test.)
Among organic matter fractions, the MBC content was found significantly higher in all the AFSs than in control (Figure 4). The alder-based AFS had 71 and 28% higher MBC content than the control and hedge-based AFS, respectively. Higher MBC under the alder-based AFS may be an indication of higher biological activity (than the control plots), which helps in the transformation of various essential nutrients and their availability. However, the guava-based AFS and control had similar MBC content. The MBN content was similar to the MBC content. The trend of the MBN content was mentioned as alder-based AFS > hedge-based AFS > guava-based AFS > control. Hedge- and alder-based AFSs were observed to have significantly higher MBC content than the control (Figure 5). POC values were observed to be significantly higher in alder- and hedge-based AFSs than the in guava-based AFS and control (Figure 5). The guava-based AFS and control had a similar POC content.
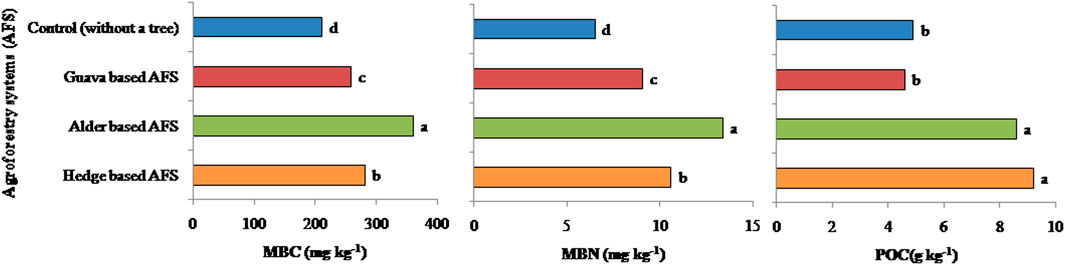
FIGURE 5. Effect of various agroforestry systems on organic matter fractions in the soil depth 0–30 cm of the Indian sub-Himalayas. (MBC, microbial biomass carbon; MBN, microbial biomass nitrogen; and POC, particulate organic carbon. Means of different land use systems within a column followed by similar lowercase letters are not significant at p < 0.05 based on Tukey’s HSD test.)
Ratios of SOM forms were found to be affected by AFS, as shown in Table 3. The SOC/TN (C:N) ratio was significantly higher in hedge-, alder-, and guava-based AFSs than in control. The maximum C:N was observed in the guava-based AFS, followed by hedge- and alder-based AFSs. Supplementary Figure S3 showed depth-wise C:N in the soil, and it was significantly higher in the soil depth of 10–20 cm for the hedge-based AFS than that in the soil depth 0–10 cm, while other land use systems recorded more C:N in the soil depth of 0–10 cm than in the soil depth of 10–20 cm. The ratio of MBC/SOC showed the MQ in different land use systems, and it was higher in guava- and alder-based AFSs than in the hedge-based AFS. The ratio of MBN/TN showed a similar trend to MBC/SOC, except that the lowest ratio was observed in the control. However, the ratio of MBC/SOC was the lowest in the hedge-based AFS, and the ratio of MBC/MBN was the highest in control. Moreover, all the three AFSs were statistically at par with one another. POC/SOC was more in the hedge-based AFS and statistically at par with the alder-based AFS.
3.4 Relative Soil Organic Carbon Stocks Loss and (CO2) eq. Emissions
Results for relative SOC stocks loss and CO2 eq. emissions showed significant changes due to different AFSs (Figure 6). Relative SOC stocks loss was higher in control (without a tree) over hedge-based AFS, followed by control over alder-based AFS, than control over guava-based AFS; however, it was negative for control over guava-based AFS, showing greater SOC stocks in control than the guava-based AFS. Relative SOC stocks loss was 59.9% higher under control over hedge-based AFS and 47.8% higher under control over alder and guava-based AFSs, which was more or less similar to the control treatment. A similar trend was recorded for CO2 eq. emission. It was the highest under control over hedge-based AFS (35.2 Mg ha−1), followed by control over alder-based AFS (28.6 Mg ha−1), and the lowest trend was observed under control over guava-based AFS.
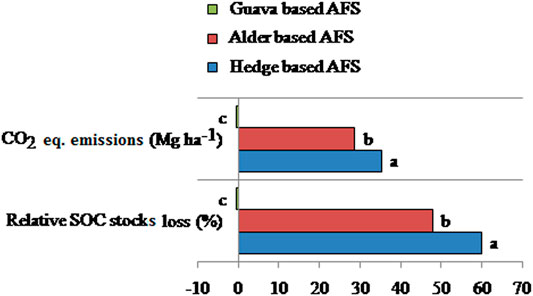
FIGURE 6. Effects of land use systems on relative SOC loss (%) and CO2 equivalent emission in the soil depth of 0–30 cm of the Indian sub-Himalayas. (Means of different land use systems within a column followed by similar lowercase letters are not significant at p < 0.05 based on Tukey’s HSD test.)
3.5 Interrelation Among SOM Pools
SOC had positive and highly significant relationship with MBC and MBN at p < 0.01 (Table 4). SOC was also positively and significantly correlated to available N at p < 0.01 and available K at p < 0.05. MBC demonstrated a significantly positive relationship with MBN at p < 0.01. MBC was also highly positively correlated with available N and negatively correlated with available P, directly affecting nutrient transformation and nutrient availability (Table 4).
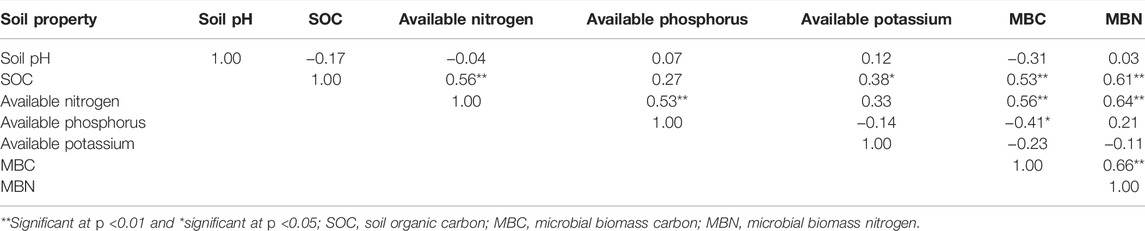
TABLE 4. Interrelationships (Pearson’s correlation coefficient “r”) among properties of all land use systems.
SOM parameters in AFS were related to each other and represented by linear regression equations given as follows (Table 5):
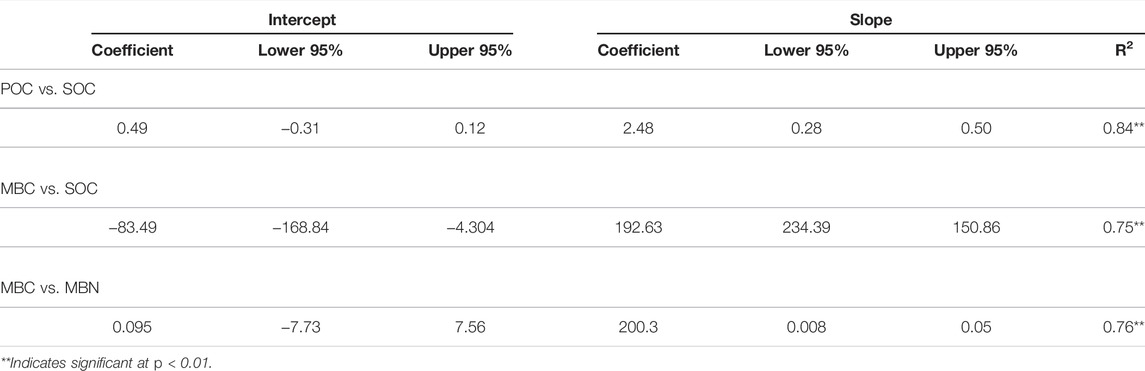
TABLE 5. Confidence interval (95%) of the regression equations showing the relationship among soil organic matter fractions in different agroforestry systems of the Indian sub-Himalayas.
3.6 Annual Nutrient Accumulation by Different Agroforestry Systems
Leaf biomass was significantly higher in the hedge-based AFS, followed by guava-based AFS, in than the alder-based AFS (Table 6). N was accumulated higher in the hedge-based AFS than in alder- and guava-based AFSs was at par with the alder-based AFS (Table 6). P accumulation showed the trend followed as hedge-based AFS > guava-based AFS > alder-based AFSs (Table 6). K accumulation was greater in the hedge-based AFS, followed by guava-based AFS, than the alder-based AFS (Table 6).

TABLE 6. Biomass production and annual nutrient accumulation by agroforestry system in the Indian sub-Himalayas.
4 Discussion
Agroforestry is one of the old farming practices that combine multipurpose trees with various shrubs, cropping systems, and livestock in the land use system that supports life sustenance and ecological biodiversity, maintains soil and water systems, and sequester carbon above or below ground biomass (Gebre, 2016). Agroforestry land use has been found efficient for soil conservation and minimizing surface runoff in the Indian Himalayan region (Nath et al., 2021). Although practiced worldwide, this farming system depends on nature, climate, and geographical conditions of the region, which vary from country to country. Also culture, demand and socioeconomic conditions govern this farming system. As AFSs are more complex in nature than monoculture, they require serious effort, economic reliability, and technical expertise to successful adoption in the potential region. Indian sub-Himalayas are such probable region whose potential can be increased by adopting proper AFSs. Several researchers have used different AFSs and cropping systems, but more reliable information is required to support the farming community economically. In this study, one of the important existing cropping system maize–mustard–potato was used under different multipurpose trees including alder, guava, and different hedge species. The present study revealed that the productivity of maize and mustard was significantly higher in the control plots than in the plots under AFS, indicating unfavorable growth of light-demanding food crops in the understory of AFS. Bhatt and Misra (2003) also recorded higher crop yield in control plots (without a tree) than in the agri–silvi system in Meghalaya, India. Crop productivity observed in the present investigation was in line with the yields reported by Dhyani and Tripathi (1999) for maize; Bhatt et al. (2001) for maize and mustard; and Bhatt et al. (2016) for maize, mustard, and potato in selected AFS in Meghalaya, India.
Studies on the impact of land use change in the acid soils of the sub-Himalayan region to SOC retention have been extensively done, and several interventions were proposed by several workers related to tillage, fertilizer management, and organic amendments (Kundu et al., 2007; Bhattacharyya et al., 2012; Padbhushan et al., 2020; Padbhushan et al., 2021). Also, some of the studies reported the effect of AFSs on the SOC content and SOM pool in the acid soils of the sub-Himalayan region, India (Brahma et al., 2018). In view of the previous studies, the present study was planned to identify the best-suited AFS for SOC retention and climate smart agriculture practices for combating changes in agriculture practices in the region.
A significant influence of AFS in improving the SOC content indicated that the intervention of agroforestry increased the soil carbon content over the plots without any trees (control). The accumulation of pruned biomass in the AFS treatments increased SOC that improved microbial activity and further increased the MBC content of soils. The SOC content in the AFS, especially hedge-based AFS, was higher on average than the control, suggesting more carbon addition due to more biomass accumulation, higher C:N, and slower mineralization process in the soil system. More biomass production releases more root exudates, which ultimately leads to improved soil carbon content (Halvorson et al., 1999; Kundu et al., 2007; Bhattacharyya et al., 2009b; Dignac et al., 2017; Canarini et al., 2019). The alder-based AFS showed a similar carbon content to the hedge-based AFS, while the guava-based AFS had a lower SOC content to the hedge-based AFS but greater than the control plot. Similar findings were in agreement with Somarriba et al. (2013) who reported that the cocoa-based AFS accumulated higher carbon in the soil system in Central America. Malhi et al. (2002) have reported that the hedge-based AFS increased the SOC content by 114% in the surface soil compared to adjacent cultivated land in Canada. Control plots had higher root biomass inputs than the AFS system that probably minimizes the SOC and N contents in the surface soil. Moreover, in the subsurface layer, the accumulation of biomass and litter was higher in the AFS than in control, which ensured a higher SOC content in AFSs over the control plot. The guava-based AFS has less biomass and litter accumulation than alder- and hedge-based AFSs and resulted in lower SOC retention in the acid soil.
Microbial biomass carbon (MBC) is the most active portion of SOM pool. It is one of the important soil quality indicators that change rapidly on throughput interventions and technology (Padbhushan et al., 2016a; Padbhushan et al., 2021). AFSs are considered as a strategic intervention to improve the MBC content in the soil (Rodrigues et al., 2015). In this study, alder- and hedge-based AFSs have shown a higher MBC content over the control plots that are without any tree due to more leaf litter addition and biomass accumulation. AFSs provided favorable conditions for the growth of microorganisms, mainly soil bacteria, resulting in a higher MBC content (Lepcha and Devi, 2020). Researchers have showcased a linear correlation between SOC and MBC (Jenkinson and Ladd, 1981; Leita et al., 1999), and a similar finding was obtained in this study, showing AFSs are contributing toward MBC similar to SOC.
Soil organic matter (SOM) pool has a major portion of POC that serves as a useful parameter for the soil quality indicator (Gregorich et al., 1994; Bhattacharyya et al., 2012; Awale et al., 2017; Bongiorno et al., 2019). The portion of carbon is obtained from slow decomposition of SOM (Cambardella and Elliott, 1992). The present study showed higher POC in the alder- and hedge-based AFSs than in the guava-based AFS and control. This is due to the accumulation of more SOC in POC fractions in hedge- and alder-based AFSs in acid soils than in the guava-based AFS and control. Similar results were in agreement with several research studies that revealed the sensitivity of POC to land use management and its significance in SOM pool (Carter et al., 2003; Liang et al., 2003; Su and Zhao, 2003). The hedge-based AFS is a long growing season AFS which has more extension roots than other AFSs and crops; this yields more carbon sequestration with residue. This results in more accumulation of POC and SOC in the soil. Moreover, soil erosion due to wind decreases POC and SOC contents in control plots compared to the hedge-based AFS (Su et al., 2004).
The capacity level for carbon, which represents the protected carbon associated with clay and silt particles, was found to be 9–12 g carbon kg−1 soil for the control plot that is without any tree; 11–15 g carbon kg−1 soil for the guava-based AFS; 19–25 g carbon kg−1 soil for the alder-based AFS; and 31–36 g carbon kg−1 soil for the hedge-based AFS.
According to our results, soil carbon sequestration was significantly higher under the hedge-based AFS, followed by the alder-based AFS than control (without a tree). The guava-based AFS had more or less similar soil carbon sequestration than control in the soil depth 0–30 cm cumulatively. The value of soil carbon sequestered was 9.58 and 7.81 Mg ha−1 higher in the hedge and alder-based AFSs than in the control. This study confirmed that hedges and multipurpose trees (Alder) increased the SOC content in the soil. This study was in agreement with several studies, which showed incorporating trees improves in SOC stocks (Haile et al., 2008; Ramachandran Nair et al., 2009; De Stefano et al., 2017). This is probably due to higher litter inputs and root residue addition in hedge and alder-based AFSs than the control (Jobbágy and Jackson, 2000; Bhatt et al., 2016). Less soil cover and less litter inputs in the control (without a tree) resulted in lower SOC stocks (Kumar et al., 2021). Soil characteristics also play an important role in carbon sequestration due to humification, aggregation, and translocation of biomass into subsoil (Lal, 2001). In this study, a similar result was found, showing an increase in the SOC stocks in the subsurface for hedge and alder-based AFSs. Proper land use conversion is important for ecological and environmental stabilization. Ramachandran Nair et al. (2009) ranked SOC stocks and reported that AFSs sequestered more carbon than arable crops. Similar results were found in this study; however, the guava-based AFS did not contribute to carbon sequestration and hence is not suitable for regions like Indian sub-Himalayas. This was probably due to no difference in input addition between the control (without a tree) and guava-based AFS in the region.
The available P status was mostly lower due to very strong acidic nature of soils. Short-term management of soils by AFSs did not affect soil pH. However, available N, P, and K contents were significantly improved in the plots under hedge and agri–silvi-based AFSs, mainly due to the positive effects of agroforestry. The experimental soils were known to be 1:1 kaolinite type of clay minerals, which adds a substantial amount of K after weathering, and hence making the soils of the surface layer rich in the available K content. Still, the plots under the hedge-based AFS had a significantly higher available K content as the K accumulation in these plots are 3- to 4-fold higher than other plots (Bhatt et al., 2016).
The potential nutrient returns in the form of leaf litter are in the order of 80–120 kg N, 8–12 kg P, 40–120 kg K, and 20–60 kg Ca for humid tropics of India, as observed by Lupwayi and Haque (1998). In this investigation, 59.68 kg N, 11.95 kg P, and 28.31 kg K were contributed by hedges. Since the material decomposed within 7 months, we could estimate the total amount of each nutrient that would accumulate in the soil. Agroforestry therefore promotes closer nutrient cycling, as evident from the present investigation. Among the hedges, foliage of C. tetragona added maximum N (88.65 kg ha−1), P (18.65 kg ha−1), and K (44.81 kg ha−1) to the soil. Nutrient release followed the order N > K > P for both hedge- and alder-based AFSs. A high initial content of N of hedges reflects its relative suitability as better substrate for microflora action.
5 Conclusion
Converting fallow lands into hedge- and alder-based AFSs resulted in more soil carbon retention and nutrient accumulation in the acid soils of the Indian sub-Himalayas. Higher crop productivity obtained in hedge- and alder-based AFSs ensured more food security than in the guava-based AFS and control (crop without any tree). A significant increase in SOM pool (MBC, MBN, and POC) and carbon stocks was obtained in hedge- and alder-based AFSs compared to guava-based AFS and control plots. Due to more biomass accumulation and leaf litter, a higher SOC content was observed in the subsurface layer than in the surface layer in hedge- and alder-based AFSs, while in the guava-based AFS and control plots, a higher SOC content was found in the surface layer than in the subsurface layer. Nutrient accumulation was higher in hedge- and alder-based AFSs than in the guava-based AFS and control plots. This is one of the rare studies that evaluated the rate of soil carbon retention by different AFSs in the Indian Himalayas. Thus, hedge- and alder-based AFSs may be promoted to achieve climate-smart agriculture practices in the acid soils of the sub-Himalayan region.
Data Availability Statement
The original contributions presented in the study are included in the article/Supplementary Material, further inquiries can be directed to the corresponding authors.
Author Contributions
BP framed the notion and was in charge of this manuscript preparation. BP, AV and AK reviewed the literature, analyzed data, and drafted the manuscript. RP and UK also helped in drafting the manuscript. RK prepared the map. UK, RP, MK, RK, BK and SG edited the manuscript. All contributors discussed the outcomes and added points to the final document. All authors have studied and approved the in print version of the manuscript.
Conflict of Interest
The authors declare that the research was conducted in the absence of any commercial or financial relationships that could be construed as a potential conflict of interest.
Publisher’s Note
All claims expressed in this article are solely those of the authors and do not necessarily represent those of their affiliated organizations, or those of the publisher, the editors, and the reviewers. Any product that may be evaluated in this article, or claim that may be made by its manufacturer, is not guaranteed or endorsed by the publisher.
Acknowledgments
We are grateful to all the involved different institutes for providing necessary fund and facilities for this study.
Supplementary Material
The Supplementary Material for this article can be found online at: https://www.frontiersin.org/articles/10.3389/fenvs.2022.858948/full#supplementary-material
Supplementary Figure S1 | Representative figures of hedge (A), alder (B) and guava (C) agroforestry systems and compared with maize-mustard-potato (D) cropping system under sub-Indian Himalayas.
Supplementary Figure S2 | Effects of various agroforestry systems on soil organic carbon stocks (SOC stocks) in the different soil depths (0-10, 10-20 and 20-30 cm) of the Indian sub-Himalayas. (Means of different land use systems within a column followed by similar lowercase letters are not significant at p < 0.05 based on Tukey’s HSD test.)
Supplementary Figure S3 | Effect of various agroforestry systems on C:N in the Indian sub-Himalayas. (Means of different land use systems within a column followed by similar lowercase letters are not significant at p < 0.05 based on Tukey’s HSD test.)
References
Ahmed, F., Monika, A. U. H., Hossain, M. A., Wadud, M. A., and Rahman, G. M. M. (2018). Performance of Guava Fruit Tree-Based Agroforestry Practice during Summer Season in Charland and Plainland Ecosystems. J. Agrofor. Environ. 12 (1 & 2), 1–8.
Albrecht, A., and Kandji, S. T. (2003). Carbon Sequestration in Tropical Agroforestry Systems. Agric. Ecosyst. Environ. 99, 15–27. doi:10.1016/s0167-8809(03)00138-5
Angima, S., O’Neill, M., Onwega, A. K., and Stott, D. (2000). Use of Tree/grass Hedges for Soil Erosion Control in the Central Kenyan highlands. J. Soil Water Conserv. 55, 478–482.
Asbjornsen, H., Hernandez-Santana, V., Liebman, M., Bayala, J., Chen, J., Helmers, M., et al. (2014). Targeting Perennial Vegetation in Agricultural Landscapes for Enhancing Ecosystem Services. Renew. Agric. Food Syst. 29 (2), 101–125. doi:10.1017/S1742170512000385
Awale, R., Emeson, M. A., and Machado, S. (2017). Soil Organic Carbon Pools as Early Indicators for Soil Organic Matter Stock Changes under Different Tillage Practices in Inland Pacific Northwest. Front. Ecol. Evol. 5, 96. doi:10.3389/fevo.2017.00096
Bhatt, B., and Bujarbaruah, K. M. (2006). Complementary of Livestock and Agroforestry for Sustainable Agriculture in North East. Agroforestry in North East India: Opportunities and Challenges. Umiam, Meghalaya: ICAR Research Complex for NEH region, 581–591.
Bhatt, B. P., and Misra, L. K. (2003). Production Potential and Cost-Benefit Analysis of Agrihorticulture Agroforestry Systems in Northeast India. J. Sustain. Agric. 22, 99–108. doi:10.1300/j064v22n02_07
Bhatt, B. P., Singh, R., Misra, L. K., Tomar, J. M. S., Singh, M., Chauhan, D. S., et al. (2001). “Agroforestry Research and Practices: an Overview,” in Steps towards Modernization of Agriculture in NEH Region. ICAR Research Complex for NEH Region. Editors N.D. Verma, and B.P. Bhatt (Umiam, Meghalaya, India), 365–392.
Bhatt, B. P., Parmar, B., Bordoloi, L. J., Benjamin , , and Bhattacharyya, R. (2016). Impact of Agroforestry Systems on Soil and Nutrient Conservation in the Eastern Himalayas, India. Int. J. Bio-Res. Stress Manage. 7, 575–581. doi:10.5958/0976-4038.2016.00091.9
Bhattacharyya, R., Prakash, V., Kundu, S., Pandey, S. C., Srivastva, A. K., and Gupta, H. S. (2009a). Effect of Fertilisation on Carbon Sequestration in Soybean - Wheat Rotation under Two Contrasting Soils and Management Practices in the Indian Himalayas. Soil Res. 47, 592–601. doi:10.1071/sr08236
Bhattacharyya, R., Prakash, V., Kundu, S., Srivastva, A. K., and Gupta, H. S. (2009b). Soil Properties and Their Relationships with Crop Productivity after 30 Years of Different Fertilization in the Indian Himalayas. Arch. Agron. Soil Sci. 55, 641–661. doi:10.1080/03650340902718615
Bhattacharyya, R., Tuti, M. D., BishtBhatt, J. K. J. C., Bhatt, J. C., and Gupta, H. S. (2012). Conservation Tillage and Fertilization Impact on Soil Aggregation and Carbon Pools in the Indian Himalayas under an Irrigated Rice-Wheat Rotation. Soil Sci. 177, 218–228. doi:10.1097/ss.0b013e3182408f1e
Bongiorno, G., Bünemann, E. K., Oguejiofor, C. U., Meier, J., Gort, G., Comans, R., et al. (2019). Sensitivity of Labile Carbon Fractions to Tillage and Organic Matter Management and Their Potential as Comprehensive Soil Quality Indicators across Pedoclimatic Conditions in Europe. Ecol. Ind. 99, 38–50. doi:10.1016/j.ecolind.2018.12.008
Brahma, B., Pathak, K., Lal, R., Kurmi, B., Das, M., Nath, P. C., et al. (2018). Ecosystem Carbon Sequestration through Restoration of Degraded Lands in Northeast India. Land Degrad. Dev. 29, 15–25. doi:10.1002/ldr.2816
Bray, J. R., and Gorham, E. (1964). Litter Production in Forests of the World. Adv. Ecol. Res. 2, 101–157. doi:10.1016/s0065-2504(08)60331-1
Cambardella, C. A., and Elliott, E. T. (1992). Particulate Soil Organic‐Matter Changes across a Grassland Cultivation Sequence. Soil Sci. Soc. Am. J. 56, 777–783. doi:10.2136/sssaj1992.03615995005600030017x
Canarini, A., Kaiser, C., Andrew, M., Richter, A., and Wanek, W. (2019). Root Exudation of Primary Metabolites: Mechanisms and Their Roles in Plant Responses to Environmental Stimuli. Front. Pl. Sci. 10, 157. doi:10.3389/fpls.2019.00157
Carter, M. R., Kunelius, H. T., Sanderson, J. B., Kimpinski, J., Platt, H. W., and Bolinder, M. A. (2003). Productivity Parameters and Soil Health Dynamics under Long-Term 2-year Potato Rotation in Atlantic Canada. Soil Till. Res. 72, 153–168. doi:10.3389/fpls.2019.00157
J.C. Dagar, and J.C. Tewari (Editors) (2016). Agroforestry Research Developments (New York, USA: Nova Science Publishers, Inc), pp578.
Dagar, J. C., and Tewari, V. P. (2017). “Evolution of Agroforestry as a Modern Science,” in Evolution of Agroforestry as a Modern Science. Editors J.C. Dagar, and V.P. TewariAgroforestry (Singapore: Springer Nature), 13–90. doi:10.1007/978-981-10-7650-3_2
J. C. Dagar, S. R. Gupta, and D. Teketay (Editors) (2020). Agroforestry for Degraded Landscapes: Recent Advances and Emerging Challenges (Springer Nature Singapore, Pte Ltd.), Vols. 1 and 2, 1-475–1-554.
Datta, A., Basak, N., Chaudhari, S. K., and Sharma, D. K. (2015). Soil Properties and Organic Carbon Distribution under Different Land Uses in Reclaimed Sodic Soils of North-West India. Geoderma Reg. 4, 134–146. doi:10.1016/j.geodrs.2015.01.006
De Stefano, A., Jacobson, M. G., and Jacobson, M. G. (2017). Soil Carbon Sequestration in Agroforestry Systems: a Meta-Analysis. Agroforest Syst. 92, 285–299. doi:10.1007/s10457-017-0147-9
Dhyani, S. K., and Tripathi, R. S. (1999). Tree Growth and Crop Yield under Agrisilviculture Practices in north-east India. Agroforestry Syst. 44, 1–12.
Dhyani, S. K., Tewari, R. K., Tiwari, R., Singh, R., and Srivastava, S. R. (2013). Production and Guidance. Retrieved from: http://cafri.res.in/html/CAFRI-AR/AR(2013-14)_English.pdf Accessed: February 6, 2014.
Dignac, M.-F., Derrian, D., Barré, P., Barot, S., Cécillon, L., Chenu, C., et al. (2017). Increasing Soil Carbon Storage: Mechanisms, Effects of Agricultural Practices and Proxies. A Review. Agron. Sustain. Dev. 37, 14. doi:10.1007/s13593-017-0421-2
Feliciano, D., Ledo, A., Hillier, J., and Nayak, D. R. (2018). Which Agroforestry Options Give the Greatest Soil and above Ground Carbon Benefits in Different World Regions? Agric. Ecosyst. Environ. 254, 117–129. doi:10.1016/j.agee.2017.11.032
Franzluebbers, A. J., and Stuedemann, J. A. (2009). Soil-profile Organic Carbon and Total Nitrogen during 12 Years of Pasture Management in the Southern Piedmont USA. Agric. Ecosyst. Environ. 129, 28–36. doi:10.1016/j.agee.2008.06.013
FSI (2013). Trees in Agroforestry Systems in India (Chapter 7). Available at: https: // fsi.nic.in / cover_2013 / treesinggroforestry.pdf.
Gebre, A. B. (2016). Potential Effects of Agroforestry Practices on Climate Change Mitigation and Adaptation Strategies: a Review. J. Nat. Sci. Res. 6 (15), 83–89.
Gregorich, E. G., Carter, M. R., Angers, D. A., Monreal, C. M., and Ellert, B. H. (1994). Towards a Minimum Data Set to Assess Soil Organic Matter Quality in Agricultural Soils. Can. J. Soil Sci. 74, 367–385. doi:10.4141/cjss94-051
Haile, S. G., Nair, P. K. R., and Nair, V. D. (2008). Carbon Storage of Different Soil‐Size Fractions in Florida Silvopastoral Systems. J. Environ. Qual. 37, 1789–1797. doi:10.2134/jeq2007.0509
Halvorson, A. D., Reule, C. A., and Follett, R. F. (1999). Nitrogen Fertilization Effects on Soil Carbon and Nitrogen in a Dryland Cropping System. Soil Sci. Soc. Am. J. 63, 912–917. doi:10.2136/sssaj1999.634912x
Hanley, T. A. (1978). A Comparison of the Line-Interception and Quadrat Estimation Methods of Determining Shrub Canopy Coverage. J. Range Manage. 31, 60–62. doi:10.2307/3897638
Hassink, J. (1997). The Capacity of Soils to Preserve Organic C and N by Their Association with clay and silt Particles. Plant Soil 191, 77–87. doi:10.1023/a:1004213929699
Hernández, D. E. A., Shivlani, M., and Sabat, A. M. (2014). Ecosystem-Based and Community-Based Model Integration to Designate Coral Reef No-Take Marine Protected Areas: A Case Study from Puerto Rico. Nat. Resour. 5, 538–560. doi:10.4236/nr.2014.510049
IFSR (2019). India State of Forest Report. Forest Survey of India, Ministry of Environment, Forest and Climate Change, Government of India.
Jenkinson, D. S., and Ladd, J. N. (1981). “Microbial Biomass in Soil: Measurement and Turnover,” in Soil Biochemistry. Editors E. A. Paul, and J. N. Ladd (New York: Marcel Dekker), 415–471.
Jobbágy, E. G., and Jackson, R. B. (2000). The Vertical Distribution of Soil Organic Carbon and its Relation to Climate and Vegetation. Ecol. Appl. 10 (2), 423–436. doi:10.2307/264110410.1890/1051-0761(2000)010[0423:tvdoso]2.0.co;2
Joergensen, R. G., and Olfs, H. W. (1998). The Variability between Different Analytical Procedures and Laboratories for Measuring Soil Microbial Biomass C and Biomass N by the Fumigation Extraction Method. Z. Pflanzenernaehr. Bodenk. 161, 51–58. doi:10.1002/jpln.1998.3581610109
Juhi, , Singh, Y. K., Singh, B., Das, A., Kohli, A., Kumar, R., et al. (2022). Crop Yields and Soil Organic Matter Pools in Zero-Till Direct-Seeded rice-based Cropping Systems as Influenced by Fertigation Levels in the Indo-Gangetic plains in India. Carbon Manage. 13 (1), 78–89. doi:10.1080/17583004.2021.2016495
Kehie, M., and Khamu, S. (2018). Indigenous Alder Based Farming Practices in Nagaland, India: A Sustainable Agricultural Model. Jtfp 5. doi:10.25173/jtfp.2017.5.2.75
Kiepe, P. (1995). No Runoff, No Soil Loss: Soil and Water Conservation in Hedgerow Barrier systems. Wageningen, The Netherlands: Agricultural University.
Kjeldahl, J. (1883). Neue Methode zur Bestimmung des Stickstoffs in organischen Körpern. Fresenius, Z. f. anal. Chem. 22 (1), 366–382. doi:10.1007/bf01338151
Kumar, A., Padbhushan, R., Singh, Y. K., Kohli, A., and Ghosh, M. (2021). Soil Organic Carbon under Various Land Uses in Alfisols of Eastern India. Indian J. Agril. Sci. 91 (7), 975–979.
Kumar, G., Kumari, R., Shambhavi, S., Kumar, S., Kumari, P., and Padbhushan, R. (2022). Eight-year Continuous Tillage Practice Impacts Soil Properties and Forms of Potassium under Maize-based Cropping Systems in Inceptisols of Eastern India. Commun. Soil Sci. Plant Anal. 53, 602–621. doi:10.1080/00103624.2021.2017961
Kundu, S., Bhattacharyya, R., Prakash, V., Ghosh, B., and Gupta, H. (2007). Carbon Sequestration and Relationship between Carbon Addition and Storage under Rainfed Soybean-Wheat Rotation in a sandy Loam Soil of the Indian Himalayas. Soil Tillage Res. 92, 87–95. doi:10.1016/j.still.2006.01.009
Lal, R., and Bruce, J. P. (1999). The Potential of World Cropland Soils to Sequester C and Mitigate the Greenhouse Effect. Environ. Sci. Pol. 2, 177–185. doi:10.1016/s1462-9011(99)00012-x
Lal, R. (2001). “Fate of Eroded Soil Carbon: Emission or Sequestration,” in Soil Carbon Sequestration and the Greenhouse Effect. Editor R. Lal (Madison, WI: Soil Science Society of America Special Publication), 57, 173–181.
Lal, R. (2004). Soil Carbon Sequestration in India. Clim. Change 65, 277–296. doi:10.1023/b:clim.0000038202.46720.37
Laskar, S. Y., Sileshi, G. W., Pathak, K., Debnath, N., Nath, A. J., Laskar, K. Y., et al. (2021). Variations in Soil Organic Carbon Content with Chronosequence, Soil Depth and Aggregate Size under Shifting Cultivation. Sci. Total Environ. 762, 143114. doi:10.1016/j.scitotenv.2020.143114
Leita, L., De Nobili, M., Mondini, C., Muhlbachova, G., Marchiol, L., Bragato, G., et al. (1999). Influence of Inorganic and Organic Fertilization on Soil Microbial Biomass, Metabolic Quotient and Heavy Metal Bioavailability. Biol. Fertil. Soils 28, 371–376. doi:10.1007/s003740050506
Lepcha, N. T., and Devi, N. B. (2020). Effect of Land Use, Season, and Soil Depth on Soil Microbial Biomass Carbon of Eastern Himalayas. Ecol. Process. 9 (1), 65. doi:10.1186/s13717-020-00269-y
Liang, X., Xie, Z., and Huang, M. (2003). A New Parameterization for Surface and Groundwater Interactions and its Impact on Water Budgets with the Variable Infiltration Capacity (VIC) Land Surface Model. J. Geophys. Res. 108, 8613–8615. doi:10.1029/2002jd003090
Lupwayi, N. Z., and Haque, I. (1998). Mineralization of N, P, K, Ca and Mg from Sesbania and Leucaena Leaves Varying in Chemical Composition. Soil Biol. Biochem. 30, 337–343. doi:10.1016/s0038-0717(97)00132-6
Majumdar, B., Kailash, K., Venkatesh, M. S., and PatiramBhatt, B. P. (2004). Effect of Different Agroforestry Systems on Soil Properties in Acid Alfisols of Meghalaya. J. Hill Res. 17, 1–5.
Malhi, Y., Meir, P., and Brown, S. (2002). Forests, Carbon and Global Climate. Philos. Trans. R. Soc. Lond. Ser. A: Math. Phys. Eng. Sci. 360, 1567–1591. doi:10.1098/rsta.2002.1020
McGonigle, T., and Turner, W. (2017). Grasslands and Croplands Have Different Microbial Biomass Carbon Levels Per Unit of Soil Organic Carbon. Agriculture 7, 57. doi:10.3390/agriculture7070057
MEA (2005). Millennium Ecosystem AssessmentEcosystems and Human Well-Being: Biodiversity Synthesis. Washington, DC: World Resources Institute.
Murthy, I. K., Gupta, M., Tomar, S., Munsi, M., Tiwari, R., Hegde, G. T., et al. (2013). Carbon Sequestration Potential of Agroforestry Systems in India. J. Earth Sci. Clim. Change 4, 131. doi:10.4172/2157-7617.1000131
Nath, A. J., Sahoo, U. K., Giri, K., Sileshi, G. W., and Das, A. K. (2020). “Incentivizing Hill Farmers for Promoting Agroforestry as an Alternative to Shifting Cultivation in Northeast India,” in Incentivizing hill Farmers for Promoting Agroforestry as an Alternative to Shifting Cultivation in Northeast India. Agroforestry for Degraded Landscapes. Editor J.C. Dagar (Springer Nature Singapore Pte Ltd), 425–444. doi:10.1007/978-981-15-4136-0_14
Nath, A. J., Kumar, R., Devi, N. B., Rocky, P., Giri, K., Sahoo, U. K., et al. (2021). Agroforestry Land Suitability Analysis in the Eastern Indian Himalayan Region. Environ. Challenges 4, 100199. doi:10.1016/j.envc.2021.100199
Ollinaho, O. I., and Kröger, M. (2021). Agroforestry Transitions: The Good, the Bad and the Ugly. J. Rural Stud. 82, 210–221. doi:10.1016/j.jrurstud.2021.01.016
Padbhushan, R., Rakshit, R., Das, A., and Sharma, R. P. (2015). Assessment of Long-Term Organic Amendments Effect on Some Sensitive Indicators of Carbon under Subtropical Climatic Condition. The Bioscan 10, 1237–1240.
Padbhushan, R., Rakshit, R., Das, A., and Sharma, R. P. (2016a). Effects of Various Organic Amendments on Organic Carbon Pools and Water Stable Aggregates under a Scented rice-potato-onion Cropping System. Paddy Water Environ. 14, 481–489. doi:10.1007/s10333-015-0517-8
Padbhushan, R., Das, A., Rakshit, R., Sharma, R. P., Kohli, A., and Kumar, R. (2016b). Long-Term Organic Amendment Application Improves Influence on Soil Aggregation, Aggregate Associated Carbon and Carbon Pools under Scented Rice-Potato-Onion Cropping System after the 9th Crop Cycle. Commun. Soil Sci. Plant Anal. 47, 2445–2457. doi:10.1080/00103624.2016.1254785
Padbhushan, R., Sharma, S., Rana, D. S., Kumar, U., Kohli, A., and Kumar, R. (2020). Delineate Soil Characteristics and Carbon Pools in Grassland Compared to Native Forestland of India: A Meta-Analysis. Agronomy 10, 1969. doi:10.3390/agronomy10121969
Padbhushan, R., Sharma, S., Kumar, U., Rana, D. S., Kohli, A., Kaviraj, M., et al. (2021). Meta-Analysis Approach to Measure the Effect of Integrated Nutrient Management on Crop Performance, Microbial Activity, and Carbon Stocks in Indian Soils. Front. Environ. Sci. 9, 724702. doi:10.3389/fenvs.2021.724702
Padbhushan, R., Kumar, U., Sharma, S., Rana, D. S., Kumar, R., Kohli, A., et al. (2022). Impact of Land-Use Changes on Soil Properties and Carbon Pools in India: A Meta-Analysis. Front. Environ. Sci. 9, 794866. doi:10.3389/fenvs.2021.794866
Page, A. L., Miller, R. H., and Keeny, D. R. (1982). Methods of Soil Analysis. Part 2. Second edition. Madison, USA: Wiscosin, 425.
Paustian, K., Collins, H. P., and Paul, E. A. (1997). “Management Controls on Soil Carbon,” in Soil Organic Matter in Temperate Agroecosystems. Editors E. A. Paul, K. Paustian, E. T. Elliott, and C.V. Cole (Boca Raton, FL: CRC Press), 15–49.
Piper, C. S. (1950). Soil and Plant Analysis. Adelaide, Australia: The University of Adelaide Press, 368–369.
Puri, S., and Nair, P. K. R. (2004). Agroforestry Research for Development in India: 25 Years of Experiences of a National Program. Agroforestry Syst. 61-62, 437–452. doi:10.1023/b:agfo.0000029014.66729.e0
Rakshit, R., Das, A., Padbhushan, R., Sharma, R. P., Sushant, S., and Kumar, S. (2018). Assessment of Soil Quality and Identification of Parameters Influencing System Yield under Long-Term Fertilizer Trial. Jour. Indian Socie. Soil Scie. 66, 166–171. doi:10.5958/0974-0228.2018.00021.x
Ramachandran Nair, P. K., Mohan Kumar, B., and Nair, V. D. (2009). Agroforestry as a Strategy for Carbon Sequestration. Z. Pflanzenernähr. Bodenk. 172 (1), 10–23. doi:10.1002/jpln.200800030
Ramachandran Nair, P. K., Nair, V. D., Mohan Kumar, B., and Showalter, J. M. (2010). Carbon Sequestration in Agroforestry Systems. Adv. Agron. 108, 237–307. doi:10.1016/S0065-2113(10)08005-3
Ramos, N. C., Gastauer, M., de Almeida Campos Cordeiro, A., Meira-Neto, J. A. A., and Augusto Alves, João. (2015). Environmental Filtering of Agroforestry Systems Reduces the Risk of Biological Invasion. Agroforest Syst. 89 (2), 279–289. doi:10.1007/s10457-014-9765-7
Rodrigues, R. C., Araújo, R. A., Costa, C. D., Lima, A. J. T., Oliveira, M. A., José, M. A., et al. (2015). Soil Microbial Biomass in an Agroforestry System of Northeast Brazil. Trop. Grasslands - Forrajes Tropicales 3 (3), 4141–4148. doi:10.17138/TGFT
Sahoo, U. K., Singh, S. L., Gogoi, A., Kenye, A., and Sahoo, S. S. (2019). Active and Passive Soil Organic Carbon Pools as Affected by Different Land Use Types in Mizoram, Northeast India. PLoS One 14 (7), e0219969. doi:10.1371/journal.pone.0219969
Saini, H. S. (2008). Climate Change and its Future Impact on the Indo-Gangetic Plain (IGP). Earth Sci. India 301 (3), 138–147.
Semwal, R. L., and Maikhuri, R. K. (1996). Structure and Functioning of Traditional Hill Agroecosystems of Garhwal Himalaya. Biol. Agric. Hortic. 13 (3), 267–289. doi:10.1080/01448765.1996.9754784
Sharma, S., Padbhushan, R., and Kumar, U. (2019). Integrated Nutrient Management in Rice-Wheat Cropping System: An Evidence on Sustainability in the Indian Subcontinent through Meta-Analysis. Agronomy 9, 71. doi:10.3390/agronomy9020071
Singh, G., Venkataramanan, C., Sastry, G., and Joshi, B. P. (1990). Manual of Soil and Water Conservation Practices. New Delhi, India: Oxford and IBH Co, 52.
Six, J., Feller, C., Denef, K., Ogle, S. M., de Moraes, J. C., and Albrecht, A. (2002). Soil Organic Matter, Biota and Aggregation in Temperate and Tropical Soils - Effects of No-Tillage. Agronomie 22, 755–775. doi:10.1051/agro:2002043
Smith, J. (2010). Agroforestry: Reconciling Productivity with Protection of the Environment: A Synopsis of Research Literature. Elm Farm: The Organic Research Centre, 1–24.
Somarriba, E., Cerda, R., Orozco, L., Cifuentes, M., Dávila, H., Espin, T., et al. (2013). Carbon Stocks and cocoa Yields in Agroforestry Systems of Central America. Agric. Ecosyst. Environ. 173, 46–57. doi:10.1016/j.agee.2013.04.013
Staelens, J., Nachtergale, L., Luyssaert, S., and Lust, N. (2003). A Model of Wind-Influenced Leaf Litterfall in a Mixed Hardwood forest. Can. J. For. Res. 33, 201–209. doi:10.1139/x02-174
Su, Y. Z., and Zhao, H. l. (2003). Soil Properties and Plant Species in an Age Sequence of Caragana Microphylla Plantations in the Horqin Sandy Land, north China. Ecol. Eng. 20, 223–235. doi:10.1016/s0925-8574(03)00042-9
Su, Y.-Z., Zhao, H.-L., Zhang, T.-H., and Zhao, X.-Y. (2004). Soil Properties Following Cultivation and Non-grazing of a Semi-arid sandy Grassland in Northern China. Soil Tillage Res. 75, 27–36. doi:10.1016/s0167-1987(03)00157-0
Walkley, A., and Black, I. A. (1934). An Examination of the Degtjareff Method for Determining Soil Organic Matter, and a Proposed Modification of the Chromic Acid Titration Method. Soil Sci. 37, 29–38. doi:10.1097/00010694-193401000-00003
Witt, C., Cassman, K. G., Olk, D. C., Biker, U., Liboon, S. P., Samson, M. I., et al. (2000). Crop Rotation and Residue Management Effects on Carbon Sequestration, Nitrogen Cycling and Productivity of Irrigated rice Systems. Plant Soil 225, 263–278. doi:10.1023/a:1026594118145
Keywords: agroforestry systems, carbon sequestration, carbon stocks, soil organic carbon, microbial biomass carbon, CO2 equivalent emission
Citation: Parmar B, Vishwakarma A, Padbhushan R, Kumar A, Kumar R, Kumari R, Kumar Yadav B, Giri SP, Kaviraj M and Kumar U (2022) Hedge and Alder-Based Agroforestry Systems: Potential Interventions to Carbon Sequestration and Better Crop Productivity in Indian Sub-Himalayas. Front. Environ. Sci. 10:858948. doi: 10.3389/fenvs.2022.858948
Received: 20 January 2022; Accepted: 11 March 2022;
Published: 14 April 2022.
Edited by:
Rosa Francaviglia, Council for Agricultural and Economics Research (CREA), ItalyReviewed by:
Bal Ram Singh, Norwegian University of Life Sciences, NorwayGaurav Mishra, Indian Council of Forestry Research and Education, India
Arun Jyoti Nath, Assam University, India
Copyright © 2022 Parmar, Vishwakarma, Padbhushan, Kumar, Kumar, Kumari, Kumar Yadav, Giri, Kaviraj and Kumar. This is an open-access article distributed under the terms of the Creative Commons Attribution License (CC BY). The use, distribution or reproduction in other forums is permitted, provided the original author(s) and the copyright owner(s) are credited and that the original publication in this journal is cited, in accordance with accepted academic practice. No use, distribution or reproduction is permitted which does not comply with these terms.
*Correspondence: Rajeev Padbhushan, rajpd01@gmail.com; Upendra Kumar, ukumarmb@gmail.com