Removing Malachite Green and Leucomalachite Green From Freshwater and Seawater With Four Water Treatment Agents
- 1Department of Aquaculture, National Taiwan Ocean University, Keelung City, Taiwan
- 2Department of Aquaculture, National Pingtung University of Science and Technology, Pingtung county, Taiwan
Malachite green (MG) residue in aquatic environments can adversely affect food chains. To solve this problem, many adsorbents have been developed to remove MG from aqueous solutions. These adsorbents exhibit benefits in different aspects such as low cost, nontoxicity, and environment friendliness. Zeolite, oyster shell powder (OSP), humic acid (HA), and Chloride dioxide (ClO2) are widely used agents for water purification, indicating their potential to remove MG from both freshwater and seawater. Liquid chromatography tandem mass spectrometry was performed as a valid analytical method for measuring MG and its metabolite leucomalachite green (LMG). The analysis examined linearity, specificity, accuracy, precision, and limit of quantification. We found that three agents—zeolite, OSP, and HA—have high affinity for MG and LMG in both freshwater and seawater because of their physicochemical properties; ClO2 exhibited even greater efficiency for MG and LMG degradation due to its oxidation capacity. The MG removal efficiency of zeolite, OSP and HA in freshwater was 100%, 87.52%, and 100%, respectively. In the MG-containing seawater, the removal efficiency of zeolite, OSP and HA was 95.24%, 6.57%, and 89.36%, respectively. The LMG removal efficiency of zeolite, OSP and HA in freshwater was 100%, 53.56%, and 100%, while in seawater, the LMG removal efficiency of zeolite, OSP and HA was 100%, 62.70%, and 84.65%, respectively. ClO2 treatments in both freshwater and seawater expressed 100% removal efficiency. The adsorption capacity of three water agents in MG-containing freshwater decreased in the order: zeolite = HA > OSP; in MG-containing seawater: zeolite > HA > OSP. Whereas the adsorption capacity of three water agents in LMG-containing freshwater decreased in the order: zeolite = HA > OSP; in LMG-containing seawater: zeolite > HA > OSP. In this study, four water treatment agents are reported to remove MG and LMG from freshwater and seawater, indicating their potential for reducing the amount of hazardous MG and LMG residue in aquatic environments.
Introduction
Water pollution, in the form of hazardous artificial substances, directly or indirectly influences biological systems and the environment. Malachite green (MG) is traditionally known as a dye, also a synthetic parasiticide, used in the aquaculture industry. It can kill protozoa and fungi and to prevent the economic losses associated with parasites (Alderman, 1985; Srivastava et al., 2004). However, MG is an illegal parasiticide because the use of one of its metabolites—leucomalachite green (LMG)—harms aquatic animals, including damaging immune and reproductive systems and promoting carcinogenesis (Bills and Hunn, 1976; Meyer and Jorgenson, 1983; Mitrowska et al., 2005).
Although MG has been banned in aquaculture, its residue and metabolite LMG remain in freshwater and seawater present a substantial challenge because MG is a recalcitrant compound that resists normal biodegradation. According to the 2002/657/EC, a minimum required performance limit (MRPL) of 2 µg/kg was established for both MG and LMG in aquaculture products (Chain, 2016). However, the residue and metabolite of MG in the water environment are neglected by people, it remains to be confined that the concentration and limits of MG and LMD in the freshwater or seawater by food control authorities. Studies have developed various methods, including biodegradation and the use of nanoparticles or natural materials (Baek et al., 2010; Wang et al., 2014; Zhou et al., 2015; Lima et al., 2017; Li et al., 2018), to partially remove MG. For instance, polymer composites have been used in MG removal, such as sodium alginate/SBAC composite beads, polymer-marine biomass based nanocomposite, and lignocellulose biomass composite bio-sorbent, which perform high removal efficiency in solution (Khan et al., 2019; Das et al., 2020; Sarojini et al., 2022). Although such methods reduce MG in various aqueous solutions, whether they can be applied to freshwater or seawater to remove MG remains unclear. Besides the challenges in the removal efficiency of freshwater or seawater, the cost, price, and eco-friendly materials are still under-investigated.
MG removal presents challenges in aquatic environments, which are complex because of their composition, pH, and temperatures; this is especially true in high-salinity seawater. Water treatment agents, such as zeolite, oyster shell powder (OSP), and humic acid (HA), have been frequently used in water purification because they are environment friendly, nontoxic, inexpensive (Wu et al., 2010; Zhang et al., 2015; Wei et al., 2018); such qualities indicate the potential of these adsorbents for removing MG from both freshwater and seawater.
This study developed novel approaches for the removal of MG from both freshwater and seawater; these approaches employ four water treatment agents, zeolite, OSP, HA, and ClO2. The advantages of these four agents are as follows:
(1) Zeolite is a polar adsorbent with a high affinity for bacteria (Milenkovic et al., 2017), and a multicavity substance with strong electrostatic force for adsorption (Arbuznikov et al., 1998; Rhodes, 2016). Zeolite is abundant with aluminosilicates. The adsorbent properties of zeolite have made it useful for removing heavy metal ions and dyes (Wang and Ariyanto, 2007; Han et al., 2010). The adsorption kinetics of malachite green by zeolite have been proposed, which are pseudo-first-order, pseudo-second-order, and diffusion models (Wang and Ariyanto, 2007). Han et al. (2010) also demonstrated that spent zeolite could be reused after microwave irradiation for 10 min at 160 W, suggesting that zeolite is a low cost and eco-friendly material to remove the malachite green.
(2) Oyster shell powder (OSP) is commonly used as a fertilizer for soil improvement in agriculture and as a filter material for water recycling and aquaculture (Huh et al., 2016). OSP can adsorb heavy metals such as Cd and Cr (Wu et al., 2014; Alidoust et al., 2015; Fan et al., 2015; Jung et al., 2016). OSP (CaCO3 microparticles) absorbs metal ions through ion exchange in three steps: 1) absorption of metal ions on the porous surface; 2) precipitation of the metal ions on the surface; and 3) formation of heavy metal complex nucleation and crystals on the surface (Xu et al., 2021). In addition to metal ions, calcined oyster shells are also used as an eco-friendly and low-cost effective adsorbent for anionic dye removal from water (Inthapanya et al., 2019). However, it remains unclear whether OSP has potential function to remove malachite green.
(3) Humic acid (HA) can be used for chelation to react with cations and remove heavy metals used in aquaculture (Musani et al., 1980; Livens, 1991a; Zhou et al., 2015). It is composed of an aromatic nucleus surrounded by carboxylic and phenolic substitutes arranged in a motif. Due to its hydrophobic structure and abundance of hydrophilic groups, HA has a high affinity for organic compounds, such as cationic dyes, because of hydrophobic interactions, ion exchange, π−π interactions, and hydrogen bonds (Luo et al., 2007). It has been demonstrated the adsorption kinetics, equilibrium, and adsorption thermodynamics for the removal of cationic dye (methylene blue (MB) by using HA-modified expanded perlite (HA/EP) (Luo et al., 2014).
(4) Chlorine Dioxide (ClO2) is an oxidant, which used for disinfection in water purification because of its capacity for strong oxidation reactions (Volk et al., 2002). MG has been reported to react with some oxyanions, suggesting its potential to be degraded through ClO2 oxidation (Alderman and Clifton-Hadley, 1993; Mohammed et al., 2010). ClO2 has been demonstrated to degrade the triaryl methane dye-Brilliant Blue-R by its oxidation capacity (Nadupalli et al., 2019), leading to a possibility that MG (N-methylated diaminotriphenylmethane industrial dye) might be degraded by ClO2.
To our knowledge, no research has employed liquid chromatography tandem mass spectrometry (LC-MS/MS) to analyze the capacity of these four agents to remove MG and LMG from freshwater and seawater. Our study is the first to report the use of these alternative water treatment agents for the successful removal of MG and LMG.
Materials and Methods
Experimental Water
To assess the removal efficiency of MG and LMG in water samples without any interferes, the double distilled water from Ultra Analytic; ELGA, pH7.4) was used as freshwater samples (salinity 0‰). Seawater (pH 8.4; salinity 34 ± 1‰) was collected from the aquatic animal culture room of the Department of Aquaculture at National Taiwan Ocean University. The seawater was filtrated by 0.22 µm filter and autoclaved under 121°C for 20 min prior to experiments. Both water samples were examined and no detectable MG and LMG in water.
Experimental Water Treatment Agents
White zeolite was purchased from Gih-Hwa Enterprise, Taiwan. The composition of zeolite is 68.9% SiO2, 13.5% Al2O3, 0.2% MgO, 4.26% CaO, 1.6% Na2O, 2.2% K2O, and 0.1% P2O5. OSP is consisted by 95.99% CaCO3, 0.69% SiO2, 0.42% Al2O3, 1.83% Fe2O3, 0.52% SO3, and 0.54% MgO. It was purchased from a commercial store. HA is consisted by 71.2% HA, 9.6% fulvic acid, 10.0% ash, and 9.2% water. It was purchased from Gih-Hwa Enterprise, Taiwan. Finally, the ClO2 was purchased from Taiwan Pulp & Paper Corporation.
Analytical Method Validation
The technique of analytical method validation was adapted from those used in previous studies (Halme et al., 2004; Mitrowska et al., 2005; Halme et al., 2007) and included analyses of linearity, specificity, accuracy, precision, and limit of quantification (LOQ). All procedures for measuring MG and LMG residue in freshwater and seawater were evaluated in accordance with the guidelines of the Taiwan Food and Drug Administration (https://www.fda.gov.tw/).
Chemicals and Reagents
MG (C46H50N4·3C2H2O4, purity: 96.9%) was purchased from Sigma-Aldrich. LMG (C23H26N2, purity: 98%) was purchased from Sigma-Aldrich. MG-d5 (C29H22D5N5O7, purity: 99.6%) and LMG-d5, (C23H21D5N2, purity: 99.8%) were purchased from Witega and were used as the internal standards for MG and LMG, respectively.
Zeolite, Oyster Shell Powder, and Humic Acid Treatment for Malachite Green and Leucomalachite Green Removal From Freshwater and Seawater
Three water treatment agents (200 mg/L), zeolite, OSP, and HA, were added individually to 50 ml freshwater or seawater-containing 1 mg/L MG or LMG, vortex until dissolved and then stand in bench at room temperature. 20 µl of sample was collected at day 0 as control and at day 1, 2, 4, 7, 14, 30, 60, and 90 from each treatment group. The collected samples were analyzed by LC-MS/MS and MG or LMG concentration were calculated by the following formula. Cinitial and Cfinal indicate the concentration of control and treatment water samples, respectively. The adsorption capacity was expressed as follows:
AC is the adsorption capacity, Ci (mg/l) and Cf (mg/l) are the concentration of MG or LMG before and after treatment, respectively, V (l) is the solution volume of MG or LMG, and Ws (g) is the concentration of three water agents (200 mg/L).
Liquid Chromatography Tandem Mass Spectrometry
A high-performance liquid chromatography tandem mass spectrometer consisting of an Agilent 1100 Series HPLC (Agilent, Germany) and an API 4000 Q-Trap mass spectrometer (Applied Biosystems, Canada) with electrospray ionization was employed for analysis. MG, LMG, MG-d5, and LMG-d5 were detected through LC-MS/MS using multiple reaction monitoring for mass transitions of m/z 329.5→313.4, 331.2→239.3, 334.0→318.3, and 336.0→239.2, respectively. After optimization, the working MS parameters were as follows: flow rate of 800 μL/min, positive polarity, curtain gas pressure of 10 psi, ion source gas pressure of 50 psi, ion spray voltage of 5500 V, and temperature of 500°C. All data were analyzed using Analyst 1.4 software.
Statistical Analyses
Data were analyzed through one-way analysis of variance with a subsequent Scheffe test, with statistical significance was defined as (p < 0.05).
Results
Analytical Method Validation for Malachite Green and Leucomalachite Green
The method of analyzing MG and LMG was validated in accordance with the guidelines of the Taiwan Food and Drug Administration and was applied to all LC-MS/MS measurements, those of linearity, specificity, accuracy, and precision, and to the LOQ by LC-MS/MS. All of the measurements are presented in the Supplementary Figures S1, S2 and Supplementary Tables S1–S5.
Zeolite, Oyster Shell Powder, and Humic Acid for Malachite Green Removal From Freshwater and Seawater
To test whether zeolite, OSP and HA could efficiently remove MG from freshwater, we treated MG containing freshwater (initial MG conc. 1300.0 ± 70.0 μg/L) with 200 μl of each water agents and PBS as control group. Then samples were collected at 1, 2, 4, 7, 14, 30, 60, and 90 days post treatment. In day 1, the conc. of MG is significantly reduced (zeolite: 19.3 ± 2.3 μg/L; OSP: 675 ± 16.4 μg/L; HA: 165.6 ± 4.9 μg/L), compare to control group (1002.6 ± 70.0 μg/L, p < 0.05; Table 1). At day 30, the concentration of MG is not detectable in Zeolite and HA group. In total, After 90 days, 100%, 87.52%, and 100% of the MG had been removed by the zeolite, SOP, and HA, respectively (Table 2). As shown in Supplementary Figure S3, the adsorption capacity of three water agents in MG-containing freshwater were calculated as following: 65.0 mg/g for zeolite, 60.9 mg/g for OSP, and 65.0 mg/g for HA. These results suggest that zeolite and HA have a good adsorption capacity for MG in freshwater among the three tested agents.
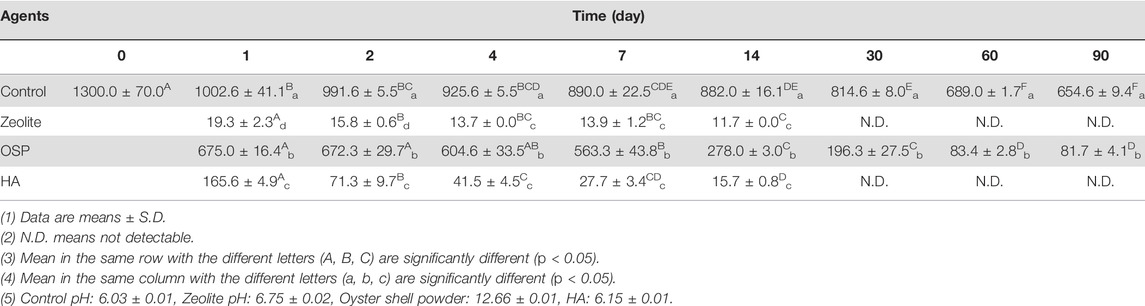
TABLE 1. Concentrations of MG (μg/L) in freshwater after treating with three agents (200 mg/L) at observation point during 90 days.
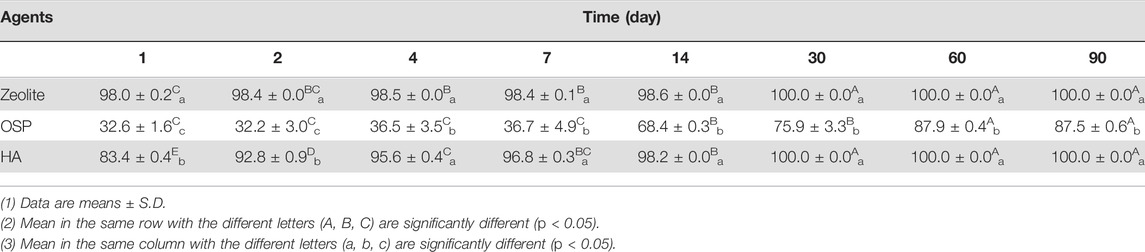
TABLE 2. The removal efficiency (%) of 1000 μg/L of MG in freshwater treated with three agents (200 mg/L) at observation point during 90 days.
In the seawater samples, we treated MG-containing seawater (initial MG conc. 1100.0 ± 20.0 μg/L) with 200 μl of each water agents and PBS as control group. Then samples were collected after 1, 2, 4, 7, 14, 30, 60, and 90 days. In day 1, the conc. MG in seawater is significantly reduced by zeolite (265.0 ± 7.0 μg/L) and HA (421.0 ± 11.1 μg/L), compare to control group (772.3 ± 42.7 μg/L; Table 3). After 90 days, the MG removal rates of zeolite, OSP, and HA were 95.2%, 6.57%, and 89.36%, respectively (Table 4). As shown in Supplementary Figure S3, the adsorption capacity of three water agents in MG-containing seawater were calculated as following: 54.6 mg/g for zeolite, 47.1 mg/g for OSP, and 54.1 mg/g for HA. This suggests that the adsorption ability of zeolite and HA is poorer in high-salinity seawater than in freshwater; by contrast, OSP efficiently removes MG from seawater.
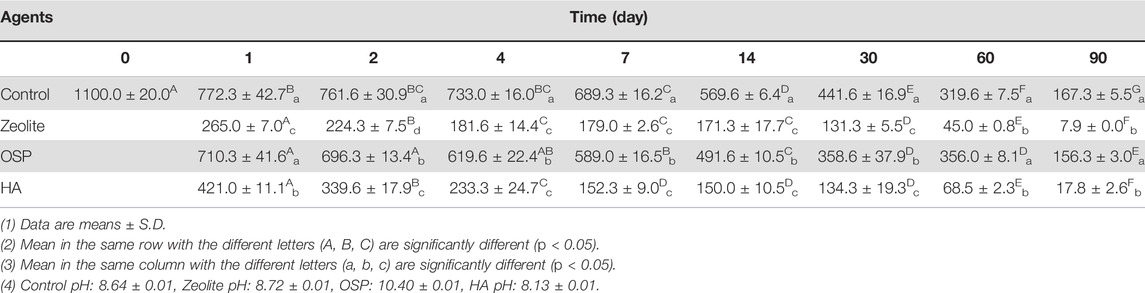
TABLE 3. Concentrations of MG (μg/L) in seawater after treating with three agents (200 mg/L) at observation point during 90 days.
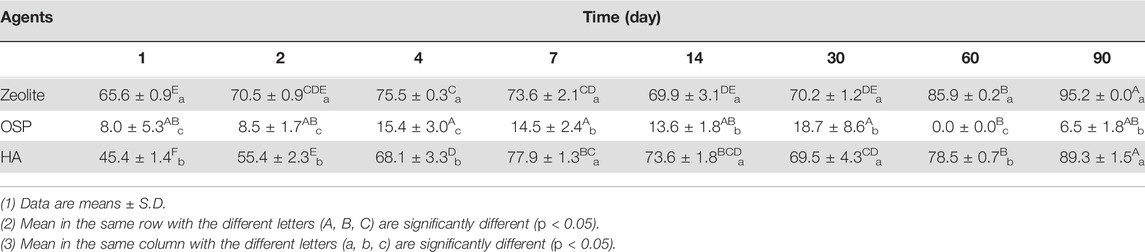
TABLE 4. The removal efficiency (%) of 1000 μg/L of MG in seawater treated with three agents (200 mg/L) at observation point during 90 days.
Zeolite, Oyster Shell Powder, and Humic Acid for Leucomalachite Green Removal From Freshwater and Seawater
To investigate whether zeolite, OSP, and HA could effectively remove LMG from freshwater, freshwater containing 836.3 ± 25.5 μg/L LMG was treated with the agents. We collected samples at 1, 2, 4, 7, 14, 30, 60, and 90 days after treatment. As shown in Table 5, in the zeolite-treated samples, the amount of residual LMG had significantly decreased after 30 days. After 60 days, the concentration of LMG was lower than the limit of detection. Moreover, OSP reduced the concentration of LMG to 836.3 ± 25.5 μg/L by day 1 and 1.6 ± 0.1 μg/L by day 90. HA also gradually reduced the concentration of LMG over 90 days. A comparison of the efficiency of the three agents (Table 6) revealed that zeolite was the most effective at removing LMG from freshwater, followed by OSP and then HA. As shown in Supplementary Figure S3, the adsorption capacity of three water agents in LMG-containing freshwater were calculated as following: 41.8 mg/g for zeolite, 41.7 mg/g for OSP, and 41.8 mg/g for HA. The results suggest that zeolite, OSP, and HA may be effective treatment agents for removing LMG from freshwater.
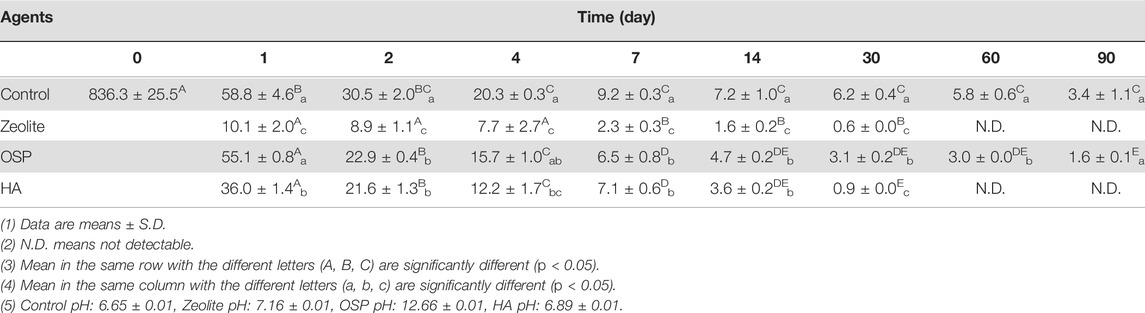
TABLE 5. Concentrations of LMG (μg/L) in freshwater after treating with three agents (200 mg/L) at observation point during 90 days.
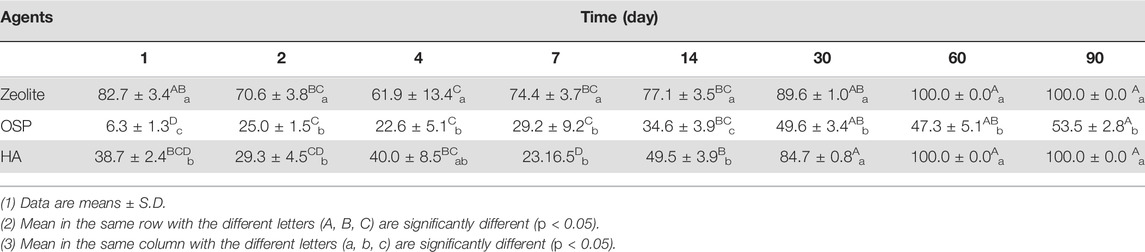
TABLE 6. The removal efficiency (%) of 1000 μg/L of LMG in freshwater treated with three agents (200 mg/L) at observation point during 90 days.
In the seawater test, we added LMG at a concentration of 847.3 ± 16.9 μg/L to seawater before treatment with zeolite, OSP, or HA. We collected samples at 1, 2, 4, 7, 14, 30, 60, and 90 days after treatment. Table 7 indicates that, compared with the negative control, zeolite, OSP, and HA resulted in significantly less LMG in seawater; the removal rates are listed in Table 8. As shown in Supplementary Figure S3, the adsorption capacity of three water agents in LMG-containing seawater were calculated as following: 42.3 mg/g for zeolite, 42.3 mg/g for OSP, and 42.3 mg/g for HA.
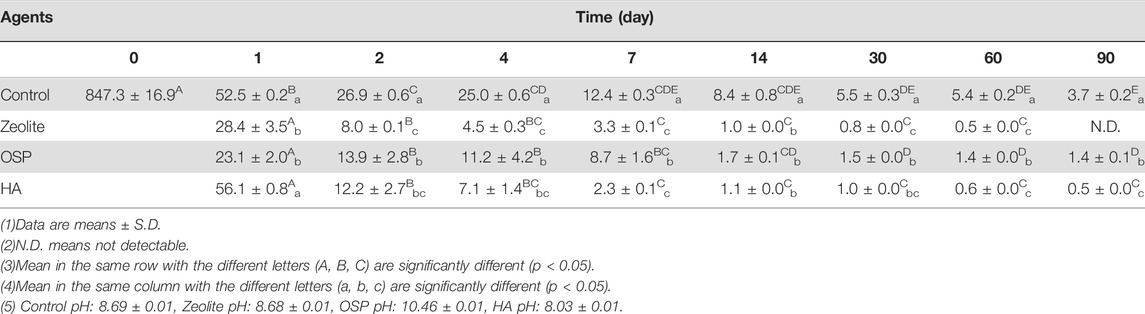
TABLE 7. Concentrations of LMG (μg/L) in seawater after treating with three agents (200 mg/L) at observation point during 90 days.
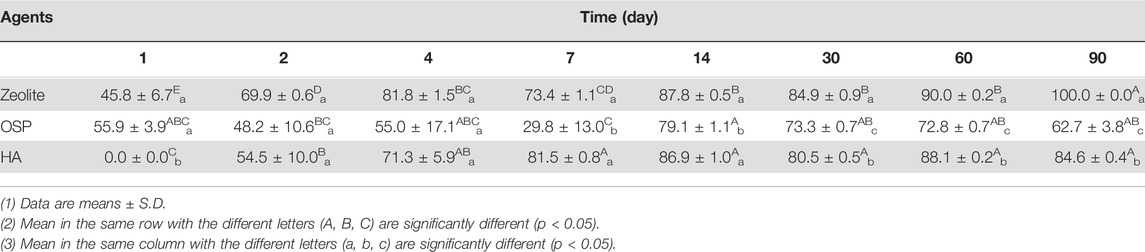
TABLE 8. | The removal efficiency (%) of 1000 μg/L of LMG in seawater treated with three agents (200 mg/L) at observation point during 90 days.
Chloride Dioxide for Malachite Green Degradation From Freshwater and Seawater
ClO2 is a common disinfectant used in drinking-water processing. To investigate whether ClO2 could degrade MG, we added MG to samples of freshwater at a concentration of 1253.3 ± 15.2 μg/L before treatment with various ClO2 (1, 5, 10, or 25 mg/L) concentrations. We then collected freshwater samples at 1, 2, 4, 7, and 14 days after treatment. As shown in Table 9A, the concentration of MG is detectable when water sample treated with 1 mg/ClO2, and gradually diminished over 14 days. Once samples treated above 5 mg/L ClO2, the MG is degraded and is lower than the limit of detection limit. A comparison of MG removal rates indicates that in the concentrations of ClO2 (5, 10, 25 mg/L) result in a greater capacity for MG degradation from freshwater (Supplementary Table S6).
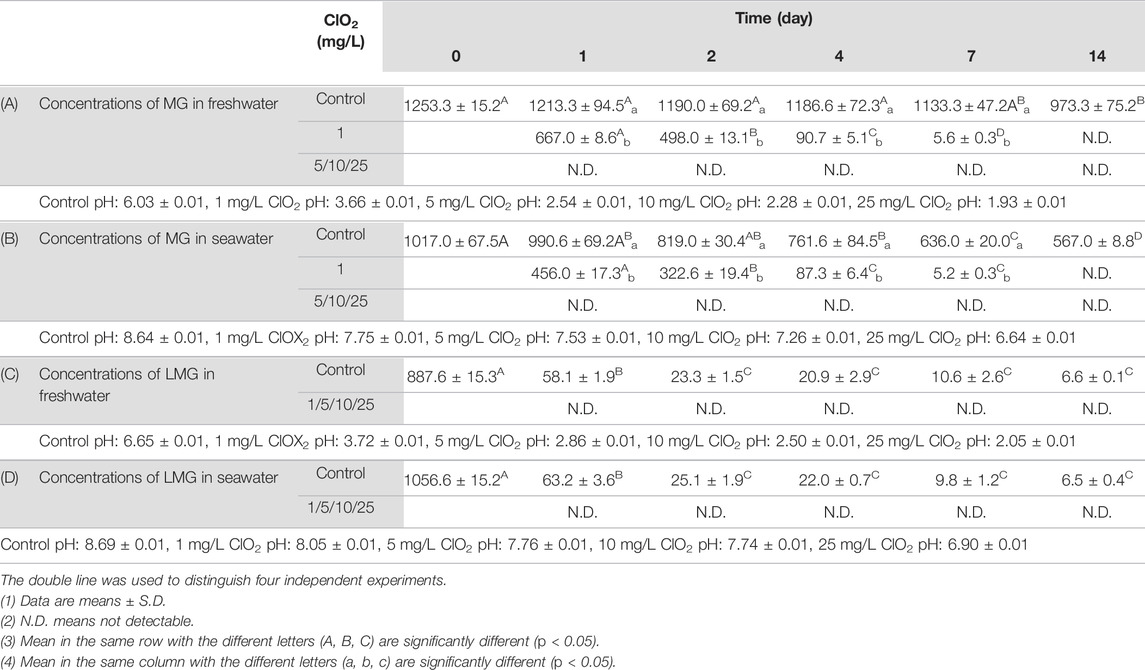
TABLE 9. Concentrations of MG (μg/L) in freshwater after treating with various concentrations of ClO2 at observation point during 14 days.
In the seawater test, we treated seawater containing 1017.0 ± 67.5 μg/L MG with various concentrations of ClO2 (1, 5, 10, or 25 mg/L) and then collected samples at 1, 2, 4, 7, and 14 days after treatment. Similar to those in the freshwater tests, the samples treated with 1 mg/L ClO2 exhibited less MG after 14 days, and in the other samples (5, 10, and 25 mg/L ClO2), the concentration decreased below the limit of detection (Table 9B and Supplementary Table S7). These results indicate that ClO2 efficiently degrades MG from seawater.
Chloride Dioxide for Leucomalachite Green Degradation From Freshwater and Seawater
To further determine whether ClO2 could also degrade LMG from freshwater and seawater, the LMG -containing freshwater (887.6 ± 15.3 μg/L) and seawater (1056.6 ± 15.2 μg/L) were treated with various concentrations of ClO2 (1, 5, 10, or 25 mg/L). Samples were collected at 1, 2, 4, 7, and 14 days after treatment. In the freshwater and seawater samples, the concentration of LMG was lower than the limit of detection at every time point (Table 9C,D), indicating that ClO2 degrades LMG from freshwater and seawater highly effectively (Supplementary Tables S8, S9).
Discussion
Performance of Zeolite, Oyster Shell Powder, and Humic Acid in Adsorbing Malachite Green and Leucomalachite Green
Adsorbents are considered effective for removing MG and LMG from aquatic environments. Many adsorbents, such as magnetic nanocomposites, coffee waste, and chitosan, can efficiently eliminate MG from wastewater and aquaculture water (Baek et al., 2010; Wang et al., 2014; Lima et al., 2017; Li et al., 2018; Arumugam et al., 2019).
The adsorption capacity of zeolite depends on its cavity structures and the charges of its inner and outer surfaces (Altın et al., 1998). Crini (2006) demonstrated that zeolite has ion-exchange capacity. Wu et al. (2010) indicated while the influent concentration of MG was 50 mg/L, the maximum adsorption quantity reached 23.55 mg/g by using a fixed-bed column. In our study, zeolite removed 100.00% of MG from freshwater within 30 days and 95.24% of MG from seawater within 90 days. Moreover, zeolite removed 100.00% of LMG from freshwater within 60 days and 100.00% of LMG from seawater within 90 days. These results demonstrate that zeolite is highly effective in adsorbing MG or LMG; this effectiveness may be due to MG being a cationic dye and LMG being adsorbed by the surface cavities of the zeolite (Arbuznikov et al., 1998; Rhodes, 2016).
OSP has a cavity structure and exhibits mild alkaline properties in aqueous solutions; thus, it can react with water contaminants to form coordination complexes or precipitants for water purification (Boyd and Massaut, 1999). We discovered that OSP was less effective at removing MG and LMG than were zeolite and HA, but this might have been caused by OSP’s high affinity for anionic dye (Inthapanya et al., 2019), resulting in a low affinity to cationic dye MG; however, LMG might be adsorbed by the cavities in the structure of the OSP.
HA consists of anionic functional groups, such as carboxylic group and phenolic groups, which become polyanionic in aqueous solutions to form stable complexes with metals (Thanabalasingam and Pickering, 1986; Livens, 1991b; Bowley et al., 2016). Zanini et al. (2006) proposed that MG is adsorbed because of the hydrophobicity and electrostatic force of HA. In our study, HA removed 100.00% of the MG from freshwater within 30 days and 89.3% of the seawater MG within 90 days. Moreover, zeolite removed 100.00% of the LMG from freshwater within 60 days, and 84.6% from seawater in 90 days. These results indicate that HA is efficient at removing MG and LMG for both freshwater and seawater and that such efficiency may be mediated by its physicochemical properties, such as its polyanionicity, hydrophobicity, and electrostatic force.
Inexpensive, nontoxic adsorbents are optimal for removing MG and LMG from water. In this study, we examined three agents—zeolite, OSP, and HA; these agents can effectively remove both MG and LMG, exhibit favorable adsorption efficiency, and cost little. Nevertheless, their effectiveness may be limited by their saturation capacity or their ability to be recycled; studies are currently investigating the recycling of such adsorbents (Han et al., 2010).
Oxidation by ClO2 Is Effective for Malachite Green and Leucomalachite Green Degradation From Freshwater and Seawater
ClO2 is often used for disinfection in water purification (Volk et al., 2002) and degrades pesticide residues from vegetables or fruits through its oxidation capacity (Wei et al., 2018; Calvo et al., 2019). During oxidation, ClO2 is photodegraded into nontoxic metabolites, including H2O, NaCl, ClO2, and carbohydrates, and it is beneficial for environmental protection (Costilow et al., 1984). We propose that ClO2 can be used for the degradation of MG or LMG from freshwater and seawater in aquiculture because of its oxidation capacity. Our results indicate that, compared with zeolite, OSP, and HA, ClO2 treatment degrades MG and LMG with higher efficiency, even at low concentrations.
Effect of Seawater Salinity on Malachite Green and Leucomalachite Green Removal
The salinity of freshwater and seawater affects MG removal efficiency. Seawater salinity is complex, and cations such as Na+, K+, Ca2+, Mg2+, and Sr2+ and anions such as Cl−, SO42−, Br−, HCO3 (CO32−), and F− are present. We found that the efficiency of OSP for removing MG from seawater (6.57% ± 1.83%) was lower than that from freshwater (<limit of detection), suggesting that the complexity of the salinity of seawater may reduce the adsorption capacity of OSP. Moreover, the pH of the OSP–treated seawater increased, possibly because of the dissociation of the powder, thereby influencing adsorption efficiency. Moreover, OSP reacts with ions in seawater to form coordination complexes or precipitants, resulting in low MG removal efficiency (Xu et al., 2021). Furthermore, zeolite and HA demonstrate great efficiency for removing MG from freshwater than from seawater. Compared with HA and OSP, zeolite is most efficient in removing LMG from both freshwater and seawater. These results suggest that, in various aquatic environments, MG and its metabolite LMG differ in their affinities for adsorbents. In sum, the determinants of the efficiency of MG and LMG removal from seawater are ion concentrations and pH values.
Reversible Malachite Green–Leucomalachite Green Reaction After Treatment With Three Agents
MG was detected after days 7 in seawater containing LMG and zeolite, 14 days in seawater containing LMG and OSP, and 7 days in seawater containing LMG and HA. This suggests that LMG may be oxidized by the air within the zeolite cavities, the HCO3− (CO32−) of OSP, or a reaction with an oxygen-containing group of HA, thereby reverting to MG. By contrast, no MG was detected in freshwater containing LMG, suggesting that the high ion exchanges of seawater affect the removal efficiency of MG; by contrast, complete removal occurs in freshwater. In summary, these findings suggest that a functional composite material comprising zeolite, OSP, HA, and ClO2 could be used to remove MG and LMG from various aquatic environments.
Conclusion
In this study, we demonstrated that four agents: zeolite, OSP, HA, and ClO2, can remove MG and LMG from both freshwater and seawater; ClO2 is the most effective, followed by zeolite, HA, and OSP. Our results suggest that these agents can be used in water purification treatment to reduce the concentrations of hazardous MG or LMG residue in both freshwater and seawater.
Data Availability Statement
The original contributions presented in the study are included in the article/Supplementary Material, further inquiries can be directed to the corresponding author.
Author Contributions
Y-RL: methodology, formal analysis, and manuscript writing; Y-FH: formal analysis and manuscript revision; C-YH, H-TH, Z-HL, A-TL, and Y-SW: methodology and formal analysis; and F-HN: supervision.
Conflict of Interest
The authors declare that the research was conducted in the absence of any commercial or financial relationships that could be construed as a potential conflict of interest.
Publisher’s Note
All claims expressed in this article are solely those of the authors and do not necessarily represent those of their affiliated organizations, or those of the publisher, the editors, and the reviewers. Any product that may be evaluated in this article, or claim that may be made by its manufacturer, is not guaranteed or endorsed by the publisher.
Acknowledgments
We would like to thank Wallace Academic Editing for editing and proofreading this manuscript.
Supplementary Material
The Supplementary Material for this article can be found online at: https://www.frontiersin.org/articles/10.3389/fenvs.2022.906886/full#supplementary-material
References
Alderman, D. J., and Clifton-Hadley, R. S. (1993). Malachite Green: a Pharmacokinetic Study in Rainbow Trout, Oncorhynchus mykiss (Walbaum). J. Fish. Dis. 16 (4), 297–311. doi:10.1111/j.1365-2761.1993.tb00864.x
Alderman, D. J. (1985). Malachite Green: a Review. J. Fish. Dis. 8 (3), 289–298. doi:10.1111/j.1365-2761.1985.tb00945.x
Alidoust, D., Kawahigashi, M., Yoshizawa, S., Sumida, H., and Watanabe, M. (2015). Mechanism of Cadmium Biosorption from Aqueous Solutions Using Calcined Oyster Shells. J. Environ. Manag. 150, 103–110. doi:10.1016/j.jenvman.2014.10.032
Altın, O., Özbelge, H. Ö., and Doğu, T. (1998). Use of General Purpose Adsorption Isotherms for Heavy Metal-Clay Mineral Interactions. J. Colloid Interface Sci. 198 (1), 130–140. doi:10.1006/jcis.1997.5246
Arbuznikov, A., Vasilyev, V., and Goursot, A. (1998). Relationships between the Structure of a Zeolite and its Adsorption Properties. Surf. Sci. 397 (1), 395–405. doi:10.1016/S0039-6028(97)00760-7
Arumugam, T. K., Krishnamoorthy, P., Rajagopalan, N. R., Nanthini, S., and Vasudevan, D. (2019). Removal of Malachite Green from Aqueous Solutions Using a Modified Chitosan Composite. Int. J. Biol. Macromol. 128, 655–664. doi:10.1016/j.ijbiomac.2019.01.185
Baek, M.-H., Ijagbemi, C. O., O, S.-J., and Kim, D.-S. (2010). Removal of Malachite Green from Aqueous Solution Using Degreased Coffee Bean. J. Hazard. Mater. 176 (1-3), 820–828. doi:10.1016/j.jhazmat.2009.11.110
Bills, T. D., and Hunn, J. B. (1976). Changes in the Blood Chemistry of Coho Salmon Exposed to Malachite Green. Progressive Fish-Culturist 38 (4), 214–216. doi:10.1577/1548-8659(1976)38[214:citbco]2.0.co;2
Bowley, H. E., Young, S. D., Ander, E. L., Crout, N. M. J., Watts, M. J., and Bailey, E. H. (2016). Iodine Binding to Humic Acid. Chemosphere 157, 208–214. doi:10.1016/j.chemosphere.2016.05.028
Boyd, C. E., and Massaut, L. (1999). Risks Associated with the Use of Chemicals in Pond Aquaculture. Aquac. Eng. 20 (2), 113–132. doi:10.1016/S0144-8609(99)00010-2
Calvo, H., Redondo, D., Remón, S., Venturini, M. E., and Arias, E. (2019). Efficacy of Electrolyzed Water, Chlorine Dioxide and Photocatalysis for Disinfection and Removal of Pesticide Residues from Stone Fruit. Postharvest Biol. Technol. 148, 22–31. doi:10.1016/j.postharvbio.2018.10.009
Chain, E. P. o. C. i. t. F. (2016). Malachite Green in Food. Efs2 14 (7), e04530. doi:10.2903/j.efsa.2016.4530
Costilow, R. N., Uebersax, M. A., and Ward, P. J. (1984). Use of Chlorine Dioxide for Controlling Microorganisms during the Handling and Storage of Fresh Cucumbers. J Food Sci. 49 (2), 396–401. doi:10.1111/j.1365-2621.1984.tb12431.x
Crini, G. (2006). Non-conventional Low-Cost Adsorbents for Dye Removal: A Review. Bioresour. Technol. 97 (9), 1061–1085. doi:10.1016/j.biortech.2005.05.001
Das, L., Das, P., Bhowal, A., and Bhattachariee, C. (2020). Treatment of Malachite Green Dye Containing Solution Using Bio-Degradable Sodium alginate/NaOH Treated Activated Sugarcane Baggsse Charcoal Beads: Batch, Optimization Using Response Surface Methodology and Continuous Fixed Bed Column Study. J. Environ. Manag. 276, 111272. doi:10.1016/j.jenvman.2020.111272
Fan, L., Zhang, S., Zhang, X., Zhou, H., Lu, Z., and Wang, S. (2015). Removal of Arsenic from Simulation Wastewater Using Nano-Iron/oyster Shell Composites. J. Environ. Manag. 156, 109–114. doi:10.1016/j.jenvman.2015.03.044
Halme, K., and Lindfors *, K. (2004). Determination of Malachite Green Residues in Rainbow Trout Muscle with Liquid Chromatography and Liquid Chromatography Coupled with Tandem Mass Spectrometry. Food Addit. Contam. 21 (7), 641–648. doi:10.1080/02652030410001721457
Halme, K., Lindfors, E., and Peltonen, K. (2007). A Confirmatory Analysis of Malachite Green Residues in Rainbow Trout with Liquid Chromatography-Electrospray Tandem Mass Spectrometry. J. Chromatogr. B 845 (1), 74–79. doi:10.1016/j.jchromb.2006.07.048
Han, R., Wang, Y., Sun, Q., Wang, L., Song, J., He, X., et al. (2010). Malachite Green Adsorption onto Natural Zeolite and Reuse by Microwave Irradiation. J. Hazard. Mater. 175 (1-3), 1056–1061. doi:10.1016/j.jhazmat.2009.10.118
Huh, J.-H., Choi, Y.-H., Lee, H.-J., Choi, W. J., Ramakrishna, C., Lee, H.-W., et al. (2016). The Use of Oyster Shell Powders for Water Quality Improvement of Lakes by Algal Blooms Removal. J. Korean Ceram. Soc. 53 (1), 1–6. doi:10.4191/kcers.2016.53.1.1
Inthapanya, X., Wu, S., Han, Z., Zeng, G., Wu, M., and Yang, C. (2019). Adsorptive Removal of Anionic Dye Using Calcined Oyster Shells: Isotherms, Kinetics, and Thermodynamics. Environ. Sci. Pollut. Res. 26 (6), 5944–5954. doi:10.1007/s11356-018-3980-0
Jung, S., Heo, N. S., Kim, E. J., Oh, S. Y., Lee, H. U., Kim, I. T., et al. (2016). Feasibility Test of Waste Oyster Shell Powder for Water Treatment. Process Saf. Environ. Prot. 102, 129–139. doi:10.1016/j.psep.2016.03.004
Khan, M. R., Wabaidur, S. M., Busquets, R., Khan, M. A., Siddiqui, M. R., and Azam, M. (2019). Identification of Malachite Green in Industrial Wastewater Using Lignocellulose Biomass Composite Bio-Sorbent and UPLC-MS/MS: a Green Environmental Approach. Process Saf. Environ. Prot. 126, 160–166. doi:10.1016/j.psep.2019.04.008
Li, B., Gan, L., Owens, G., and Chen, Z. (2018). New Nano-Biomaterials for the Removal of Malachite Green from Aqueous Solution via a Response Surface Methodology. Water Res. 146, 55–66. doi:10.1016/j.watres.2018.09.006
Lima, D. R., Klein, L., and Dotto, G. L. (2017). Application of Ultrasound Modified Corn Straw as Adsorbent for Malachite Green Removal from Synthetic and Real Effluents. Environ. Sci. Pollut. Res. 24 (26), 21484–21495. doi:10.1007/s11356-017-9802-y
Livens, F. R. (1991a). Chemical Reactions of Metals with Humic Material. Environ. Pollut. 70 (3), 183–208. doi:10.1016/0269-7491(91)90009-L
Livens, F. R. (1991b). Chemical Reactions of Metals with Humic Material. Environ. Pollut. 70 (3), 183–208. doi:10.1016/0269-7491(91)90009-l
Luo, D., Yu, Q.-W., Yin, H.-R., and Feng, Y.-Q. (2007). Humic Acid-Bonded Silica as a Novel Sorbent for Solid-phase Extraction of Benzo[a]pyrene in Edible Oils. Anal. Chim. Acta 588 (2), 261–267. doi:10.1016/j.aca.2007.02.016
Luo, W.-J., Gao, Q., Wu, X.-L., and Zhou, C.-G. (2014). Removal of Cationic Dye (Methylene Blue) from Aqueous Solution by Humic Acid-Modified Expanded Perlite: Experiment and Theory. Sep. Sci. Technol. 49 (15), 2400–2411. doi:10.1080/01496395.2014.920395
Meyer, F. P., and Jorgenson, T. A. (1983). Teratological and Other Effects of Malachite Green on Development of Rainbow Trout and Rabbits. Trans. Am. Fish. Soc. 112 (6), 818–824. doi:10.1577/1548-8659(1983)112<818:taoeom>2.0.co;2
Milenkovic, J., Hrenovic, J., Matijasevic, D., Niksic, M., and Rajic, N. (2017). Bactericidal Activity of Cu-, Zn-, and Ag-Containing Zeolites toward Escherichia coli Isolates. Environ. Sci. Pollut. Res. 24 (25), 20273–20281. doi:10.1007/s11356-017-9643-8
Mitrowska, K., Posyniak, A., and Zmudzki, J. (2005). Determination of Malachite Green and Leucomalachite Green in Carp Muscle by Liquid Chromatography with Visible and Fluorescence Detection. J. Chromatogr. A 1089 (1), 187–192. doi:10.1016/j.chroma.2005.07.004
Mohammed, Y., Iyun, J. F., and Idris, O. S. (2010). Studies into the Kinetics and Mechanism of the Redox Reaction of Malachite Green and Nitrite Ions in Aqueous Acidic Medium. J. Of Chem. Soc. Of Niger. 35.
Musani, L., Valenta, P., Nürnberg, H. W., Konrad, Z., and Branica, M. (1980). On the Chelation of Toxic Trace Metals by Humic Acid of Marine Origin. Estuar. Coast. Mar. Sci. 11 (6), 639–649. doi:10.1016/S0302-3524(80)80015-6
Nadupalli, S., Dasireddy, V. D. B. C., Koorbanally, N. A., and Jonnalagadda, S. B. (2019). Kinetics of Oxidation of Triaryl Methane Dye, Brilliant Blue-R with Chlorine Dioxide. S. Afr. J. Chem. 72, 40–46. doi:10.17159/0379-4350/2019/v72a6
Rhodes, C. J. (2016). Electric Fields in Zeolites: Fundamental Features and Environmental Implications‡‡. Chem. Pap. 70(1), 4–21. doi:10.1515/chempap-2015-0160
Sarojini, G., Venkatesh Babu, S., Rajamohan, N., and Rajasimman, M. (2022). Performance Evaluation of Polymer-Marine Biomass Based Bionanocomposite for the Adsorptive Removal of Malachite Green from Synthetic Wastewater. Environ. Res. 204, 112132. doi:10.1016/j.envres.2021.112132
Srivastava, S., Sinha, R., and Roy, D. (2004). Toxicological Effects of Malachite Green. Aquat. Toxicol. 66 (3), 319–329. doi:10.1016/j.aquatox.2003.09.008
Thanabalasingam, P., and Pickering, W. F. (1986). Arsenic Sorption by Humic Acids. Environ. Pollut. Ser. B, Chem. Phys. 12 (3), 233–246. doi:10.1016/0143-148X(86)90012-1
Volk, C. J., Hofmann, R., Chauret, C., Gagnon, G. A., Ranger, G., and Andrews, R. C. (2002). Implementation of Chlorine Dioxide Disinfection: Effects of the Treatment Change on Drinking Water Quality in a Full-Scale Distribution System. J. Environ. Eng. Sci. 1 (5), 323–330. doi:10.1139/s02-026
Wang, H., Yuan, X., Zeng, G., Leng, L., Peng, X., Liao, K., et al. (2014). Removal of Malachite Green Dye from Wastewater by Different Organic Acid-Modified Natural Adsorbent: Kinetics, Equilibriums, Mechanisms, Practical Application, and Disposal of Dye-Loaded Adsorbent. Environ. Sci. Pollut. Res. 21 (19), 11552–11564. doi:10.1007/s11356-014-3025-2
Wang, S., and Ariyanto, E. (2007). Competitive Adsorption of Malachite Green and Pb Ions on Natural Zeolite. J. Colloid Interface Sci. 314 (1), 25–31. doi:10.1016/j.jcis.2007.05.032
Wei, J., Chen, Y., Tiemur, A., Wang, J., and Wu, B. (2018). Degradation of Pesticide Residues by Gaseous Chlorine Dioxide on Table Grapes. Postharvest Biol. Technol. 137, 142–148. doi:10.1016/j.postharvbio.2017.12.001
Wu, Q., Chen, J., Clark, M., and Yu, Y. (2014). Adsorption of Copper to Different Biogenic Oyster Shell Structures. Appl. Surf. Sci. 311, 264–272. doi:10.1016/j.apsusc.2014.05.054
Wu, X., Wang, Y., Liu, J., Ma, J., and Han, R. (2010). Study of Malachite Green Adsorption onto Natural Zeolite in a Fixed-Bed Column. Desalination Water Treat. 20 (1-3), 228–233. doi:10.5004/dwt.2010.1212
Xu, Z., Valeo, C., Chu, A., and Zhao, Y. (2021). The Efficacy of Whole Oyster Shells for Removing Copper, Zinc, Chromium, and Cadmium Heavy Metal Ions from Stormwater. Sustainability 13 (8), 4184. doi:10.3390/su13084184
Zanini, G. P., Avena, M. J., Fiol, S., and Arce, F. (2006). Effects of pH and Electrolyte Concentration on the Binding between a Humic Acid and an Oxazine Dye. Chemosphere 63 (3), 430–439. doi:10.1016/j.chemosphere.2005.08.053
Zhang, H., Yin, M., Shi, J., and Wang, Y. (2015). Quest for the Binding Mode of Malachite Green with Humic Acid. J. Mol. Struct. 1081, 268–273. doi:10.1016/j.molstruc.2014.10.021
Keywords: malachite green (MG), leucomalachite green (LMG), adsorbent, chlorine dioxide (ClO2), liquid chromatography tandem mass spectrometry (LC-MS/MS)
Citation: Lin Y-R, Hu Y-F, Huang C-Y, Huang H-T, Liao Z-H, Lee A-T, Wu Y-S and Nan F-H (2022) Removing Malachite Green and Leucomalachite Green From Freshwater and Seawater With Four Water Treatment Agents. Front. Environ. Sci. 10:906886. doi: 10.3389/fenvs.2022.906886
Received: 29 March 2022; Accepted: 11 May 2022;
Published: 15 June 2022.
Edited by:
Dunia E. Santiago, University of Las Palmas de Gran Canaria, SpainReviewed by:
Risfidian Mohadi, Sriwijaya University, IndonesiaSadish Oumabady, Tamil Nadu Agricultural University, India
Rosmawani Mohammad, Universiti Malaysia Kelantan, Malaysia
Copyright © 2022 Lin, Hu, Huang, Huang, Liao, Lee, Wu and Nan. This is an open-access article distributed under the terms of the Creative Commons Attribution License (CC BY). The use, distribution or reproduction in other forums is permitted, provided the original author(s) and the copyright owner(s) are credited and that the original publication in this journal is cited, in accordance with accepted academic practice. No use, distribution or reproduction is permitted which does not comply with these terms.
*Correspondence: Fan-Hua Nan, fhnan@mail.ntou.edu.tw