Identification and Toxicity Evaluation of Water-Soluble Chemicals Generated by the Photooxidative Degradation of Expanded Polystyrene
- 1Department of Chemistry, Kyungpook National University, Daegu, South Korea
- 2Department of Food Science and Biotechnology, Graduate School, Kyungpook National University, Daegu, South Korea
- 3Food and Bio-Industry Research Institute, Inner Beauty/Antiaging Center, Kyungpook National University, Daegu, South Korea
- 4Mass Spectrometry Convergence Research Center and Green-Nano Materials Research Center, Daegu, South Korea
- 5BK21 FOUR Community-Based Intelligent Novel Drug Discovery Education Unit, College of Pharmacy and Research Institute of Pharmaceutical Sciences, Kyungpook National University, Daegu, South Korea
- 6Knu BnC, Daegu, South Korea
In this study, expanded polystyrene (EPS) was photodegraded in vitro, and its water-soluble degradation products were extracted using solid-phase extraction. An in vivo test using the MTT assay showed that EPS exhibited increased toxicity upon photodegradation. This clearly demonstrates the necessity of investigating the degradation products at a molecular level, and thus, we conducted analyses using high-resolution mass spectrometry coupled with liquid chromatography. Overall, 68 compounds were detected, of which 13 were identified after comparing the retention times and tandem mass spectra of the extracted compounds with those of standards. The toxicity of the degraded EPS was studied by examination of the detected compounds combined with published reports. In addition, the quantification of the most abundant compounds showed that discarded EPS can generate a significant amount of chemicals by UV degradation. The data in this study show that degraded EPS can pose a significant environmental threat as a source of potentially hazardous chemicals. This study provides a fundamental basis for the evaluation of the fate and toxicity of photodegraded EPS.
Introduction
Plastics are essential in modern society and reports suggest that the annual production is >300 million tons worldwide (Scarr and Hernandez, 2019), of which only 10% are recycled, whereas ∼8 million tons are thrown into the ocean (Picó and Barceló, 2019). The potential plastic pollution problem has recently attracted public attention (Alliance to End Plastic Waste, 2020) since discarded plastic is decomposed and degraded by ultraviolet (UV) rays from the Sun, with the photooxidation mechanisms being complex (ter Halle et al., 2016; Song et al., 2017). In addition, the plastic waste being further degraded into microplastics (Naik et al., 2020) with physical breaks, weakening, and weathering are caused by environmental factors, such as wind and waves (Eo et al., 2018; van Sebille et al., 2020).
Microplastics are present in coastal areas, seas, rivers, soil, and air (Bakir et al., 2014; Davis and Murphy, 2015; Jang et al., 2017; Prata, 2018). It has been reported that microplastics were detected in the stomachs of the carcasses of marine animals washed up ashore (Nelms et al., 2019) and in the intestines of deep-sea shrimps that live in Peru–Chile and Mariana Trench, which are the deepest locations in the world (Jamieson et al., 2019). Microplastics are also present in the human environment and have been detected in drinking water and seafood (Kosuth et al., 2018; Garrido Gamarro et al., 2020). Global efforts on reducing plastic usage have led to the enforcement of policies and campaigns (Xanthos and Walker, 2017), however, the amount of plastic waste has increased since the coronavirus outbreak. The increased demand for sanitation, healthcare, and hygiene products has increased the use of disposable plastic (Prata et al., 2020; Patrício Silva et al., 2021). Globally, the rise of the delivery industry due to the outbreak of the pandemic has led to increasing the use of single-use products (Chu et al., 2020).
Expanded polystyrene (EPS) is one of the most widely used plastics (e.g., construction, packaging, and food containers) and according to Statista, 26.56 million metric tons of EPS were used in 2020 (Statista, 2021). Even though EPS is relatively light in weight compared to other household wastes, it occupies a large volume and is the most ubiquitous waste along the coast (Davis and Murphy, 2015). It is one of the most frequently found microplastics on South Korean beaches, with a size of 1–5 mm per unit area (Eo et al., 2018). EPS is used in packaging and shipping of frozen food as well as in buoys in aquaculture (Jang et al., 2016; Grandviewresearch, 2021), which are often lost or discarded into the sea. EPS can be easily blown away by wind because of its lightweight, thus, it often serves as a carrier of pollutants (Bakir et al., 2014; Hüffer and Hofmann, 2016).
The styrene bond in EPS can be degraded by sunlight via photocatalytic oxidation (Yousif and Haddad, 2013; Gewert et al., 2015) and fragmented into smaller particles (Song et al., 2020). Fragmentation increases the surface area of the EPS, thereby accelerating its photodegradation. Plastic surfaces can be a source of radical formation and inherent phototransformation (Naik et al., 2020) since the benzyl carbon in styrene can form free radicals after UV exposure and react with oxygen, water, or other molecules (Gewert et al., 2015). The photodegradation of EPS generates dissolved organic carbon via complex degradation pathways (Ward et al., 2019) making EPS a significant source of chemical pollution (Rochman et al., 2013; Jang et al., 2016).
Several studies have reported the generation of micro/nanoplastics from the degradation of EPS (Song et al., 2020), and the effects of EPS leachate (Thaysen et al., 2018). However, only a few have conducted extensive analyses for the comprehensive identification of the EPS photodegradation chemicals (Gewert et al., 2018).
High-resolution mass spectrometry (HRMS) has been widely used to study environmental pollutants (Cho et al., 2019; Solihat et al., 2019) since it can identify unknown compounds (Schymanski et al., 2014). Electrospray ionization (ESI)-mass spectrometry is the most widely used method in environmental analyses since it is suitable for the analysis of polar molecules (Kim et al., 2019; Vanini et al., 2020).
In this study, a toxicity test was performed for the water-soluble compounds generated from the UV degradation of EPS, and the products were thoroughly investigated at a molecular level using ESI coupled with HRMS. The data of the identified compounds were then combined to explain the observed toxicity.
Materials and Methods
Sample Preparation
Water and methanol were purchased from J.T. Baker (Phillipsburg, NJ, United States) and were used without further purification. The standard compounds listed in Supplementary Table S1 were purchased from TCI (Tokyo, Japan) and Sigma-Aldrich (St. Louis, MO, United States). An EPS box was cut into a size weighing 0.5 g as described in a previous study (ter Halle et al., 2016). The samples were placed in a transparent quartz crucible containing 100 ml of purified water. The output of the UV lamp (6,258 Xenon arc lamp, Newport Corporation, Irvine, CA, United States of America) was at 300 W, and the exposure time of the lamp corresponds to the sum of the annual cumulative amount of UV A and B in Korea.
Based on data published by the Korea Meteorological Administration (National Institute of Meteorological Sciences, 2018), the annual cumulative amount of UV A and B in Korea is 267.27 MJ/m2 (National Institute of Meteorological Sciences, 2018). During the UV exposure, the sample was stirred using a magnetic stirring bar. After UV exposure, the isolation of the compounds dissolved in water was performed with solid-phase extraction (SPE) using an Oasis PRiME HLB extraction cartridge (Waters, Milford, MA, United States). The cartridge was washed with 20 ml water and activated with 20 ml methanol. Blank samples were prepared by irradiating the solvent with UV radiation without EPS. The obtained solutions were treated using the aforementioned method.
In Vitro Toxicity Test
Human keratinocytes (HaCaT; AddexBio Technologies, San Diego, CA, United States) were used to evaluate the toxicity of the prepared samples. Cells were maintained in a Dulbecco’s modified essential medium supplemented with 100 units/ml penicillin–streptomycin and 10% heat-inactivated fetal bovine serum (Grand Island, NY, United States) in a humidified atmosphere of 5% CO2 at 37°C. The MTT assay was performed to evaluate the cellular toxicity of the prepared samples (Alam et al., 2021). Briefly, cells (1 × 105 cells/ml) were seeded in a 96-well black clear bottomed plate (Corning Inc., Corning, NY, United States) and cultured at 37°C with 5% CO2 for 24 h. The prepared samples (6 µl) were then treated with the cells and incubated for 24 h. Subsequently, the medium was suctioned, 10% of MTT solution was added, and the preparation was incubated for 1 h. Colored formazan crystals dissolved in 100% dimethyl sulfoxide were added to the previously incubated preparation, and the optical density of the mixture was measured at 590 nm using a microplate reader (Victor3, PerkinElmer, Waltham, MA, United States).
Mass Spectrometry and Instrumentation
The SPE extract was analyzed using an ESI-Q-Exactive Orbitrap mass spectrometer (Thermo Fisher Scientific, Waltham, MA, United States) in the negative mode. The extracted sample was introduced into the mass spectrometer using a syringe pump (Hamilton Co., NV, United States) at a flow rate of 5 μl/min for direct injection analysis and into an ACQUITY ultrahigh-performance liquid chromatography (UPLC) system (Waters, Milford, MA, United States) at 300 μl/min. The capillary temperature, spray voltage, and S-lens were maintained at 300°C, 3.2 V, and 50, respectively. The automatic gain control target was set at 5 × 105 for direct injection and 2 × 105 for liquid chromatography–mass spectrometry (LC–MS) and tandem mass spectrometry (MS/MS). Sheath, aux, and sweep gas flow rates were set at 5, 2, and 0 (arbitrary units) for direct injection analysis and 50, 15, and 2 for LC–MS and MS/MS analysis, respectively. MS/MS was set to one loop count and 0.4 mass-to-charge ratio (m/z) isolation window. Chromatographic separations were performed with an ACQUITY 1.7-µm BEH C18 column (2.1 × 100 mm) attached to an ACQUITY 1.7-µm BEH C18 guard cartridge (Waters, Milford, MA, United States). The column was maintained at 40°C and eluted with an A/B gradient, where A = water/0.1% formic acid (v/v) and B = acetonitrile/0.1% formic acid (v/v). The gradient of LC for the compound separation is shown in Supplementary Table S2.
A Xevo TQ-S micro triple-quadrupole (TQ) mass spectrometer coupled with an ACQUITY UPLC system was used for quantitative analysis. The TQ parameters were set to 3.50 kV capillary and a 5 V cone for source voltage at an operating multiple reaction monitoring mode, with the desolvation and source temperatures set at 300 and 120°C, respectively. The desolvation and source gas flow rates were 650 and 10 L nitrogen/h, respectively. Argon was used as the collision gas for the MS/MS analysis.
Data Processing
Xcalibur software (version 4.0; Thermo Fisher Scientific, Waltham, MA, United States) was used to collect and process the data obtained using the Orbitrap mass spectrometer. Peaks with S/N ratios greater than 30 and an abundance greater than 1 × 105 were selected and processed. Molecular compositions were assigned using the Composer64 version 1.5.6 software (Sierra Analytics Inc., Modesto, CA, United States). The chemical formula CcHhOo was assigned with constraints of C (6–50), H (6–200), and O (0–10), and the calibration was performed according to a previously described method (Cho et al., 2021). The chemical structures were assigned to the chemical formulas based on the interpretation of the MS/MS spectra and database search using METLIN (Smith et al., 2005) and mzCloud (https://www.mzcloud.org, HighChem LLC, Bratislava, Slovakia). The MassLynx V4.2 software (Waters, Milford, MA, United States) was used for data processing in quantitative analysis.
The toxicity database was retrieved from Tox-Info provided by the National Institute of Food and Drug Safety Evaluation under the Ministry of Food and Drug Safety (www.nifds.go.kr/toxinfo), and the hazardous substances databank was retrieved from PubChem under the National Center for Biotechnology Information (pubchem.ncbi.nlm.nih.gov).
Results and Discussion
EPS floating on water was exposed to UV light, as described in the experimental section. The irradiated EPS samples produced physically fragmented fine particles rendering the water turbid and yellowish. The turbid water was filtered, and the dissolved organic compounds were extracted using SPE.
The potential cellular toxicity of the prepared samples was evaluated using an MTT assay after SPE. The UV-exposed EPS samples were prepared in triplicate whereas the control sample was prepared without UV exposure. The cell viability results obtained from the MTT assay are shown in Figure 1. The EPS solutions without UV irradiation did not show any potential toxicity towards the HaCaT cells; however, those with UV irradiation showed significant cellular toxicity in a concentration-dependent manner compared to the non-UV irradiated EPS solutions. Approximately 80% of the cells survived in 50 ppm of the EPS samples. The cell viability dropped to approximately 70% in the 200 ppm EPS samples. Overall, the cell viability in the EPS solutions with UV irradiation was lower than that without UV irradiation. It has been reported that the leachate of polystyrene can be toxic (Gunaalan et al., 2020), with hexabromocyclododecanes and styrene oligomers playing an important role in its toxicity (De-la-Torre et al., 2020). It was thus concluded that the photodegradation products of EPS by UV irradiation can exhibit cellular toxicity toward HaCaT cells.
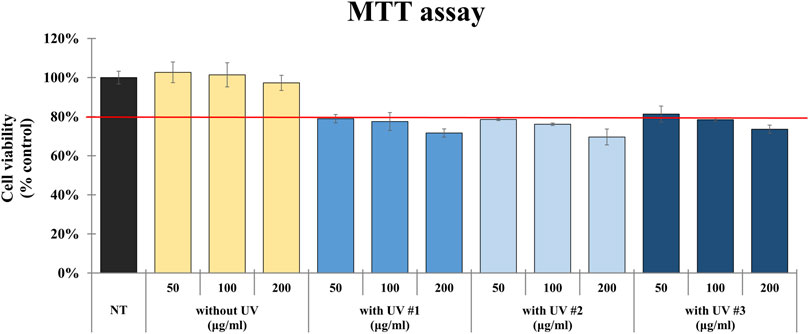
FIGURE 1. MTT assay toxicity evaluation of EPS degradation. NT indicates 100% viability of the control group, whereas the red line indicates 80% viability of the cells compared with the ontrol. Values below 80% cell viability suggest cytotoxicity, which results in cell death.
To identify the chemicals generated by the UV irradiation of EPS, the SPE eluted solution was analyzed using LC coupled with HRMS and the obtained chromatogram is shown in Figure 2. Positive-mode ESI–MS was also performed. However, the peaks in the spectra showed low abundance and no significant peaks were observed. Overall, 68 compounds were detected (Supplementary Table S3) and the elemental formulas were assigned to the peaks based on mass accuracy. Schymanski et al. (2014) proposed a confidence level for identifying small molecules with HRMS which was used in this study. The observed compounds corresponding to level 1 are listed in Table 1. The confirmed structures, based on the reference standard compounds, were assigned in level 1. Tentative candidates were obtained based on the exact mass, and the tandem mass spectra were designated as level 3. The compounds that were not found in the database or in the MS/MS spectra were marked as level 4. The chemical structures of the compounds with confidence levels of 3 and 4 were tentative.
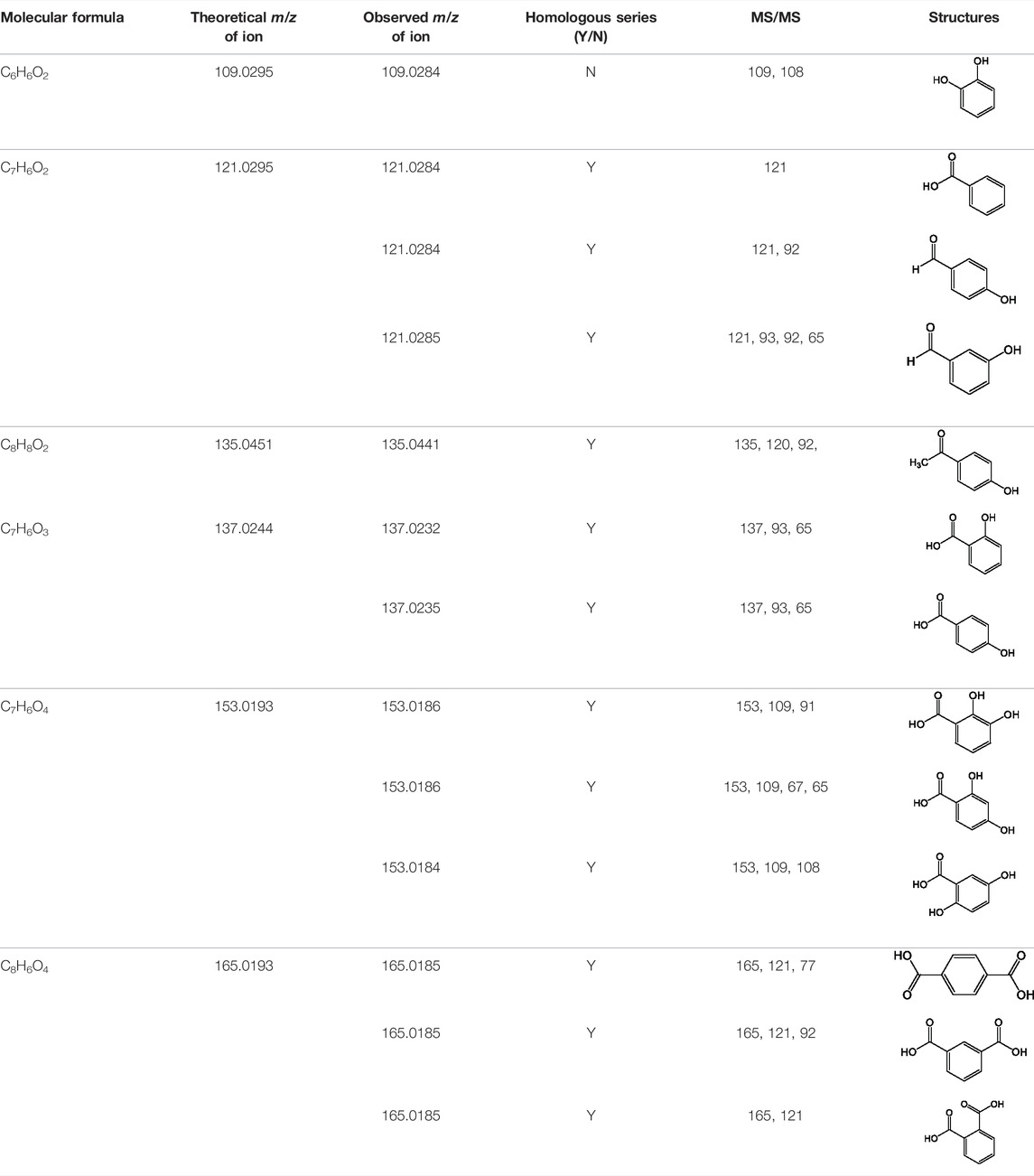
TABLE 1. List of the EPS photodegradation products identified by LC–MS. Compounds are listed in order to m/z values of ions in the negative mode.
To confirm the chemical structures of the observed compounds, standard reference compounds were purchased and analyzed by LC–MS. The obtained tandem mass spectra and LC retention times of the standard compounds were compared with those of the SPE eluents. Overall, 13 compounds were identified using this combined approach. The confirmed structures are listed in Table 1. In a previous study, C11H12O4 was assigned to the structure listed in Supplementary Table S3 with a confidence level of 1 (Gewert et al., 2018). C9H10O3, C17H18O3, and C25H26O3 have been proposed as homologous series in a previous study. In this study, 13 additional homologous series were identified.
Based on the MS data and previous studies on styrene degradation (Sheldrick and Vogl, 1976; Geuskens et al., 1978; Weir, 1978; Mailhot and Gardette, 1992), the reaction pathways to generate the compounds listed in Table 1 and Supplementary Table S3 are very complex, including a series of oxidation, β-scission, H abstraction, rearrangement, and isomerization reactions. Furthermore, various reaction products, including isomers, can be generated depending on the part of the polystyrene molecule that is cut by UV photolysis.
The toxicity of the identified compounds was examined using data from literature. Catechol, also known as pyrocatechol or 1,2-dihydroxybenzene, is used as a corrosion inhibitor and colorant in various industrial applications and it can cause eczematous dermatitis and irritation of the skin and eyes (Powell et al., 2001; Williams, 2013). In addition, catechol is classified as a human carcinogen corresponding to group 2 B by the IARC carcinogen classification (International Agency for Research on Cancer, 2021). Benzoic acid is a commonly used antimicrobial preservative in food and beverages. Although contact allergy symptoms may take several days to appear, exposure to benzoic acid causes allergic symptoms such as mild conjunctival congestion and in rare cases, it can lead to severe anaphylactic shock (Pevny and Danhauser, 1981; Johnson et al., 2017). Salicylic acid is used as an anti-inflammatory and topical antibacterial agent. It causes hypoglycemia by remarkably decreasing glucose neogenesis and increasing glucose utilization (Raschke et al., 1991), irritation of the nose and throat, nausea, vomiting, tinnitus, dizziness, headache, stuffiness, confusion, sweating, rapid pulse and breathing, and skin rash (Inchem, 1998). Severe exposure causes delirium, hallucinations, convulsions, coma, respiratory failure, and cardiovascular collapse (Chiaretti et al., 1997; Fiume, 2003). 4-hydroxybenzoic acid is used as a preservative and antioxidant. At low concentrations, it has no significant effects, whereas exposure to high concentrations causes irritation of the respiratory tract and has potential toxic effects on the liver and blood [European Chemicals Agency (ECHA), 2021]. Phthalic acid, terephthalic acid, and isophthalic acid exhibit reproductive and developmental toxicity (Bang et al., 2011). Therefore, the list of the observed compounds and their reported toxicity strongly supports the finding that photodegradation can increase the toxicity of EPS.
Quantitative analysis was conducted for the three most abundant compounds found in the LC–MS spectra of the degradation products and the results are presented in Figure 3. The limit of detection (LOD) and limit of quantitation (LOQ) obtained from the m/z peaks are listed in Table 2. The raw data used to obtain the information in Table 2 are provided in Supporting Information (Supplementary Table S4).
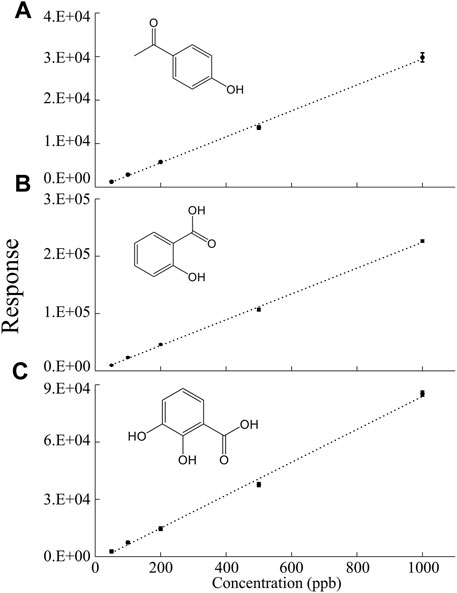
FIGURE 3. Calibration curves for the quantitative analysis of (A) 4-hydroxyacetophenone, (B) salicylic acid, and (C) 2,3-dihydroxybenzoic acid.
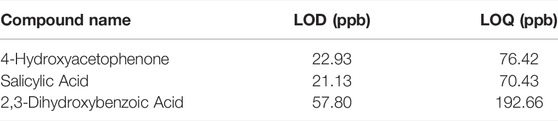
TABLE 2. Limit of detection (LOD) and limit of quantitation (LOQ) of the calibration curve for the quantitative analysis of the obtained compounds.
Based on the calibration curve presented in Figure 3, the amounts of 4-hydroxyacetophenone, salicylic acid, and 2, 3-dihydroxybenzoic acid generated from EPS (0.5 g) after 1 year of UV exposure were calculated. 8.1 ± 0.77 µg of 4-hydroxyacetophenone, 110 ± 7.6 µg of salicylic acid, and 12. ± 1.9 µg of 2,3-dihydroxybenzoic acid were estimated to be generated and the detailed calculations are shown in the Supporting Information (Supplementary Table S4). As stated in the previous section, only a small percentage of EPS is recycled [U.S. Environmental Protection Agency (EPA), 2018] and this study revealed that EPS quantities as low as 0.5 g were able to produce micrograms of chemicals. Therefore, considering the enormous amount of EPS produced and the low recycling rate, the data clearly shows that the total amount of chemicals generated by EPS can pose a significant environmental threat.
Conclusion
Compounds generated by the degradation of EPS were thoroughly investigated by LC coupled with HRMS. The MS data revealed the presence of various water-soluble chemicals, including hydrocarbon molecules of styrene monomers and oxidized degradation products. The results revealed 68 compounds of which 13 were identified using reference standards. The toxicity of EPS increased after photodegradation, indicating the necessity to examine the water-soluble compounds generated from photodegradation at a molecular level. Our study showed that the photodegradation products of EPS should be considered equally threatening as the micro- or nanosized EPS particles and additives. Considering the enormous amount of EPS produced and the low recycling rate, it is expected that a large amount of potentially hazardous chemicals will be generated by the degradation of EPS. Therefore, this study indicates the urgency of monitoring chemicals in degraded EPS, with the results offering a fundamental basis for the evaluation of the fate and toxicity of photodegraded EPS.
Data Availability Statement
The original contributions presented in the study are included in the article/Supplementary Material, further inquiries can be directed to the corresponding author.
Author Contributions
SL: Conceptualization, Methodology, Data curation, Writing—Original draft preparation, Visualization, Investigation, Validation, and Formal analysis. MA: Visualization, Investigation, Validation, and Resources. M-JJ: Conceptualization, Methodology, Writing—Review and Editing. SL: Conceptualization, Methodology, and Writing—Review and Editing. K-HL: Conceptualization, Methodology, and Writing—Review and Editing. S-HL: Formal analysis, Validation, Resources, and Writing—Review and Editing. SK: Writing—Reviewing and Editing, Supervision, Project administration, and Funding acquisition.
Funding
This study was supported by the Korea Basic Science Institute (KBSI), National Research Facilities and Equipment Center (NFEC) grant funded by the Korean government (Ministry of Education) (2019R1A6C1010001), and “Establishing National Metrology Infrastructure for Reliable Measurement of Particulate Matters” funded by the Korea Research Institute of Standards and Science (KRISS–2019–GP2019-0010). This research was supported by “Land and sea based input and fate of microplastics in the marine environment” of Korea Institute of Marine Science and Technology Promotion (KIMST) funded by the Ministry of Oceans and Fisheries (20220357).
Conflict of Interest
The authors declare that the research was conducted in the absence of any commercial or financial relationships that could be construed as a potential conflict of interest.
Publisher’s Note
All claims expressed in this article are solely those of the authors and do not necessarily represent those of their affiliated organizations, or those of the publisher, the editors and the reviewers. Any product that may be evaluated in this article, or claim that may be made by its manufacturer, is not guaranteed or endorsed by the publisher.
Supplementary Material
The Supplementary Material for this article can be found online at: https://www.frontiersin.org/articles/10.3389/fenvs.2022.938120/full#supplementary-material
References
Alam, R., Ardiati, F. C., Solihat, N. N., Alam, M. B., Lee, S. H., Yanto, D. H. Y., et al. (2021). Biodegradation and Metabolic Pathway of Anthraquinone Dyes by Trametes Hirsuta D7 Immobilized in Light Expanded Clay Aggregate and Cytotoxicity Assessment. J. Hazard. Mater. 405, 124176. doi:10.1016/j.jhazmat.2020.124176
Alliance to End Plastic Waste (2020). Progress Report 2020 – it All Starts with Collaboration [Online]. Available at: https://www.lyondellbasell.com/globalassets/advancing-possible-stories/leadership-corner/aepw-progress-report-2020-lyondellbasell.pdf ([Accessed].
Bakir, A., Rowland, S. J., and Thompson, R. C. (2014). Transport of Persistent Organic Pollutants by Microplastics in Estuarine Conditions. Estuar. Coast. Shelf Sci. 140, 14–21. doi:10.1016/j.ecss.2014.01.004
Bang, D.-Y., Lee, I.-K., and Lee, B.-M. (2011). Toxicological Characterization of Phthalic Acid. Toxicol. Res. 27 (4), 191–203. doi:10.5487/TR.2011.27.4.191
Chiaretti, A., Wismayer, D. S., Tortorolo, L., Piastra, M., and Polidori, G. (1997). Salicylate Intoxication Using a Skin Ointment. Acta Paediatr. 86 (3), 330–331. doi:10.1111/j.1651-2227.1997.tb08902.x
Cho, E., Riches, E., Palmer, M., Giles, K., Ujma, J., and Kim, S. (2019). Isolation of Crude Oil Peaks Differing by M/z ∼0.1 via Tandem Mass Spectrometry Using a Cyclic Ion Mobility-Mass Spectrometer. Anal. Chem. 91 (22), 14268–14274. doi:10.1021/acs.analchem.9b02255
Cho, E., Solihat, N. N., Kim, Y. H., and Kim, S. (2021). Comprehensive Lists of Internal Calibrants for Ultrahigh-Resolution Mass Spectrometry Analysis of Crude Oil and Natural Organic Matter and Their Preparation Recipes. J. Am. Soc. Mass Spectrom. 32 (2), 590–596. doi:10.1021/jasms.0c00456
Chu, J., Liu, H., and Salvo, A. (2020). Air Pollution as a Determinant of Food Delivery and Related Plastic Waste. Nat. Hum. Behav. 5, 212–220. doi:10.1038/s41562-020-00961-1
Davis, W., and Murphy, A. G. (2015). Plastic in Surface Waters of the inside Passage and Beaches of the Salish Sea in Washington State. Mar. Pollut. Bull. 97 (1), 169–177. doi:10.1016/j.marpolbul.2015.06.019
De-la-Torre, G. E., Dioses-Salinas, D. C., Pizarro-Ortega, C. I., and Saldaña-Serrano, M. (2020). Global Distribution of Two Polystyrene-Derived Contaminants in the Marine Environment: A Review. Mar. Pollut. Bull. 161, 111729. doi:10.1016/j.marpolbul.2020.111729
Eo, S., Hong, S. H., Song, Y. K., Lee, J., Lee, J., and Shim, W. J. (2018). Abundance, Composition, and Distribution of Microplastics Larger Than 20 μm in Sand Beaches of South Korea. Environ. Pollut. 238, 894–902. doi:10.1016/j.envpol.2018.03.096
European Chemicals Agency (ECHA) (2021). 4-hydroxybenzoic Acid. Available at: https://echa.europa.eu/registration-dossier/-/registered-dossier/15944/7/1 (Accessed February 23, 2022).
Fiume, M. Z. (2003). Safety Assessment of Salicylic Acid, Butyloctyl Salicylate, Calcium Salicylate, C12-15 Alkyl Salicylate, Capryloyl Salicylic Acid, Hexyldodecyl Salicylate, Isocetyl Salicylate, Isodecyl Salicylate, Magnesium Salicylate, MEA-Salicylate, Ethylhexyl Salicylate, Potassium Salicylate, Methyl Salicylate, Myristyl Salicylate, Sodium Salicylate, TEA-Salicylate, and Tridecyl Salicylate. Int. J. Toxicol. 22 (3_Suppl. l), 1–108. doi:10.1177/1091581803022S303
Garrido Gamarro, E., Ryder, J., Elvevoll, E. O., and Olsen, R. L. (2020). Microplastics in Fish and Shellfish - A Threat to Seafood Safety? J. Aquatic Food Prod. Technol. 29 (4), 417–425. doi:10.1080/10498850.2020.1739793
Geuskens, G., Baeyens-Volant, D., Delaunois, G., Lu-Vinh, Q., Piret, W., and David, C. (1978). Photo-oxidation of Polymers-I. Eur. Polym. J. 14 (4), 291–297. doi:10.1016/0014-3057(78)90051-4
Gewert, B., Plassmann, M. M., and MacLeod, M. (2015). Pathways for Degradation of Plastic Polymers Floating in the Marine Environment. Environ. Sci. Process. Impacts 17 (9), 1513–1521. doi:10.1039/C5EM00207A
Gewert, B., Plassmann, M., Sandblom, O., and MacLeod, M. (2018). Identification of Chain Scission Products Released to Water by Plastic Exposed to Ultraviolet Light. Environ. Sci. Technol. Lett. 5 (5), 272–276. doi:10.1021/acs.estlett.8b00119
Grandviewresearch (2021). Global Expanded Polystyrene Market Size Report, 2021-2028 [Online]. Available at: https://www.grandviewresearch.com/industry-analysis/expanded-polystyrene-eps-market (Accessed February 23, 2022).
Gunaalan, K., Fabbri, E., and Capolupo, M. (2020). The Hidden Threat of Plastic Leachates: A Critical Review on Their Impacts on Aquatic Organisms. Water Res. 184, 116170. doi:10.1016/j.watres.2020.116170
Hüffer, T., and Hofmann, T. (2016). Sorption of Non-polar Organic Compounds by Micro-sized Plastic Particles in Aqueous Solution. Environ. Pollut. 214, 194–201. doi:10.1016/j.envpol.2016.04.018
Inchem (1998). International Programme on Chemical Safety (IPCS) “INCHEM Internationally Peer Reviewed Chemical Safety Information” [Online]. Available at: https://inchem.org/documents/pims/pharm/pim642.htm (Accessed February 23, 2022).
International Agency for Research on Cancer (IARC) (2021). Agents Classified by the IARC Monographs, Volumes 1–130. Lyon: IARC. [Online]. Available at: http://monographs.iarc.fr/ENG/Classification/index.php (Accessed February 23, 2022).
Jamieson, A. J., Brooks, L. S. R., Reid, W. D. K., Piertney, S. B., Narayanaswamy, B. E., and Linley, T. D. (2019). Microplastics and Synthetic Particles Ingested by Deep-Sea Amphipods in Six of the Deepest Marine Ecosystems on Earth. R. Soc. open Sci. 6 (2), 180667. doi:10.1098/rsos.180667
Jang, M., Shim, W. J., Han, G. M., Rani, M., Song, Y. K., and Hong, S. H. (2016). Styrofoam Debris as a Source of Hazardous Additives for Marine Organisms. Environ. Sci. Technol. 50 (10), 4951–4960. doi:10.1021/acs.est.5b05485
Jang, M., Shim, W. J., Han, G. M., Rani, M., Song, Y. K., and Hong, S. H. (2017). Widespread Detection of a Brominated Flame Retardant, Hexabromocyclododecane, in Expanded Polystyrene Marine Debris and Microplastics from South Korea and the Asia-Pacific Coastal Region. Environ. Pollut. 231, 785–794. doi:10.1016/j.envpol.2017.08.066
Johnson, W., Bergfeld, W. F., Belsito, D. V., Hill, R. A., Klaassen, C. D., Liebler, D. C., et al. (2017). Safety Assessment of Benzyl Alcohol, Benzoic Acid and its Salts, and Benzyl Benzoate. Int. J. Toxicol. 36 (3_Suppl. l), 5S–30S. doi:10.1177/1091581817728996
Kim, D., Jung, J.-H., Ha, S. Y., An, J. G., Shankar, R., Kwon, J.-H., et al. (2019). Molecular Level Determination of Water Accommodated Fraction with Embryonic Developmental Toxicity Generated by Photooxidation of Spilled Oil. Chemosphere 237, 124346. doi:10.1016/j.chemosphere.2019.124346
Kosuth, M., Mason, S. A., and Wattenberg, E. V. (2018). Anthropogenic Contamination of Tap Water, Beer, and Sea Salt. PLOS ONE 13 (4), e0194970. doi:10.1371/journal.pone.0194970
Mailhot, B., and Gardette, J. L. (1992). Polystyrene Photooxidation. 2. A Pseudo Wavelength Effect. Macromolecules 25 (16), 4127–4133. doi:10.1021/ma00042a013
Naik, R. A., Rowles, L. S., Hossain, A. I., Yen, M., Aldossary, R. M., Apul, O. G., et al. (2020). Microplastic Particle versus Fiber Generation during Photo-Transformation in Simulated Seawater. Sci. Total Environ. 736, 139690. doi:10.1016/j.scitotenv.2020.139690
National Institute of Meteorological Sciences (2018). Report of Global Atmosphere Watch 2018. Republic of Korea. [Online]. Available at: http://www.climate.go.kr/home/cc_data/2018_report_of_Global_atmosphere_watch.pdf [Accessed].
Nelms, S. E., Barnett, J., Brownlow, A., Davison, N. J., Deaville, R., Galloway, T. S., et al. (2019). Microplastics in Marine Mammals Stranded Around the British Coast: Ubiquitous but Transitory? Sci. Rep. 9 (1), 1075. doi:10.1038/s41598-018-37428-3
Patrício Silva, A. L., Prata, J. C., Walker, T. R., Duarte, A. C., Ouyang, W., Barcelò, D., et al. (2021). Increased Plastic Pollution Due to COVID-19 Pandemic: Challenges and Recommendations. Chem. Eng. J. 405, 126683. doi:10.1016/j.cej.2020.126683
Pevny, I., and Danhauser, I. (1981). Anaphylactic Shock during General Anaesthesia Due to Fentanyl and Alloferin in a Patient with Positive Skin Test (Author's Transl). Anaesthesist 30 (8), 400–404.
Picó, Y., and Barceló, D. (2019). Analysis and Prevention of Microplastics Pollution in Water: Current Perspectives and Future Directions. ACS Omega 4 (4), 6709–6719. doi:10.1021/acsomega.9b00222
Powell, C. H., Bingham, E., and Cohrssen, B. (2001). Patty's Toxicology. New York: John Wiley & Sons.
Prata, J. C. (2018). Airborne Microplastics: Consequences to Human Health? Environ. Pollut. 234, 115–126. doi:10.1016/j.envpol.2017.11.043
Prata, J. C., Silva, A. L. P., Walker, T. R., Duarte, A. C., and Rocha-Santos, T. (2020). COVID-19 Pandemic Repercussions on the Use and Management of Plastics. Environ. Sci. Technol. 54 (13), 7760–7765. doi:10.1021/acs.est.0c02178
Raschke, R., Arnold-Capell, P. A., Richeson, R., and Curry, S. C. (1991). Refractory Hypoglycemia Secondary to Topical Salicylate Intoxication. Archives Intern. Med. 151 (3), 591–593. doi:10.1001/archinte.1991.0040003012102210.1001/archinte.151.3.591
Rochman, C. M., Hoh, E., Kurobe, T., and Teh, S. J. (2013). Ingested Plastic Transfers Hazardous Chemicals to Fish and Induces Hepatic Stress. Sci. Rep. 3 (1), 3263. doi:10.1038/srep03263
Scarr, S., and Hernandez, M. (2019). Drowning in Plastic: Visualizing the World’s Addiction to Plastic Bottles. [Online]. Available at: https://graphics.reuters.com/ENVIRONMENT-PLASTIC/0100B275155/index.html (Accessed February 23, 2022).
Schymanski, E. L., Jeon, J., Gulde, R., Fenner, K., Ruff, M., Singer, H. P., et al. (2014). Identifying Small Molecules via High Resolution Mass Spectrometry: Communicating Confidence. Environ. Sci. Technol. 48 (4), 2097–2098. doi:10.1021/es5002105
Sheldrick, G. E., and Vogl, O. (1976). Induced Photodegradation of Styrene Polymers: A Survey. Polym. Eng. Sci. 16 (2), 65–73. doi:10.1002/pen.760160202
Smith, C. A., Maille, G. O., Want, E. J., Qin, C., Trauger, S. A., Brandon, T. R., et al. (2005). Metlin. Ther. Drug Monit. 27 (6), 747–751. doi:10.1097/01.ftd.0000179845.53213.39
Solihat, N. N., Acter, T., Kim, D., Plante, A. F., and Kim, S. (2019). Analyzing Solid-phase Natural Organic Matter Using Laser Desorption Ionization Ultrahigh Resolution Mass Spectrometry. Anal. Chem. 91 (1), 951–957. doi:10.1021/acs.analchem.8b04032
Song, Y. K., Hong, S. H., Eo, S., Han, G. M., and Shim, W. J. (2020). Rapid Production of Micro- and Nanoplastics by Fragmentation of Expanded Polystyrene Exposed to Sunlight. Environ. Sci. Technol. 54 (18), 11191–11200. doi:10.1021/acs.est.0c02288
Song, Y. K., Hong, S. H., Jang, M., Han, G. M., Jung, S. W., and Shim, W. J. (2017). Combined Effects of UV Exposure Duration and Mechanical Abrasion on Microplastic Fragmentation by Polymer Type. Environ. Sci. Technol. 51 (8), 4368–4376. doi:10.1021/acs.est.6b06155
Statista (2021). Expanded Polystyrene Global Market Value 2020-2028. San Francisco, CA: Grand View Research. Available at: https://www.statista.com/statistics/942737/expanded-polystyrene-global-market-value/ (Accessed February 28, 2022).
ter Halle, A., Ladirat, L., Gendre, X., Goudouneche, D., Pusineri, C., Routaboul, C., et al. (2016). Understanding the Fragmentation Pattern of Marine Plastic Debris. Environ. Sci. Technol. 50 (11), 5668–5675. doi:10.1021/acs.est.6b00594
Thaysen, C., Stevack, K., Ruffolo, R., Poirier, D., De Frond, H., DeVera, J., et al. (2018). Leachate from Expanded Polystyrene Cups Is Toxic to Aquatic Invertebrates (Ceriodaphnia Dubia). Front. Mar. Sci. 5 (71). doi:10.3389/fmars.2018.00071
U.S. Environmental Protection Agency (EPA) (2018). Advancing Sustainable Materials Management: Facts and Figures Report. [Online]. Available at: https://www.epa.gov/sites/default/files/2021-01/documents/2018_tables_and_figures_dec_2020_fnl_508.pdf (Accessed March 31, 2022).
van Sebille, E., Aliani, S., Law, K. L., Maximenko, N., Alsina, J. M., Bagaev, A., et al. (2020). The Physical Oceanography of the Transport of Floating Marine Debris. Environ. Res. Lett. 15 (2), 023003. doi:10.1088/1748-9326/ab6d7d
Vanini, G., Barra, T. A., Souza, L. M., Madeira, N. C. L., Gomes, A. O., Romão, W., et al. (2020). Characterization of Nonvolatile Polar Compounds from Brazilian Oils by Electrospray Ionization with FT-ICR MS and Orbitrap-MS. Fuel 282, 118790. doi:10.1016/j.fuel.2020.118790
Ward, C. P., Armstrong, C. J., Walsh, A. N., Jackson, J. H., and Reddy, C. M. (2019). Sunlight Converts Polystyrene to Carbon Dioxide and Dissolved Organic Carbon. Environ. Sci. Technol. Lett. 6 (11), 669–674. doi:10.1021/acs.estlett.9b00532
Weir, N. A. (1978). Reactions of Hydroxyl Radicals with Polystyrene. Eur. Polym. J. 14 (1), 9–14. doi:10.1016/0014-3057(78)90144-1
Williams, M. (2013). The Merck Index: An Encyclopedia of Chemicals, Drugs, and Biologicals, 15th Edition Edited by M.J.O'Neil, Royal Society of Chemistry, Cambridge, UK ISBN 9781849736701; 2708 Pages. April 2013, $150 with 1-year Free Access to the Merck Index Online. Drug Dev. Res. 74 (5), 339. doi:10.1002/ddr.21085
Xanthos, D., and Walker, T. R. (2017). International Policies to Reduce Plastic Marine Pollution from Single-Use Plastics (Plastic Bags and Microbeads): A Review. Mar. Pollut. Bull. 118 (1), 17–26. doi:10.1016/j.marpolbul.2017.02.048
Keywords: microplastics, photodegradation, expanded polystyrene, high-resolution mass spectrometry, toxicity
Citation: Lee S, Alam MB, Jung M-J, Lee S, Liu K-H, Lee S-H and Kim S (2022) Identification and Toxicity Evaluation of Water-Soluble Chemicals Generated by the Photooxidative Degradation of Expanded Polystyrene. Front. Environ. Sci. 10:938120. doi: 10.3389/fenvs.2022.938120
Received: 07 May 2022; Accepted: 07 June 2022;
Published: 04 July 2022.
Edited by:
Andrew Turner, University of Plymouth, United KingdomReviewed by:
Stéphane Bouchonnet, Polytechnic university, FranceCassandra Rauert, The University of Queensland, Australia
Copyright © 2022 Lee, Alam, Jung, Lee, Liu, Lee and Kim. This is an open-access article distributed under the terms of the Creative Commons Attribution License (CC BY). The use, distribution or reproduction in other forums is permitted, provided the original author(s) and the copyright owner(s) are credited and that the original publication in this journal is cited, in accordance with accepted academic practice. No use, distribution or reproduction is permitted which does not comply with these terms.
*Correspondence: Sunghwan Kim, sunghwank@knu.ac.kr