Fluoxetine induces photochemistry-derived oxidative stress on Ulva lactuca
- 1MARE - Marine and Environmental Sciences Centre and ARNET – Aquatic Research Infrastructure Network Associate Laboratory, Faculdade de Ciências da Universidade de Lisboa, Lisbon, Portugal
- 2cE3c, Centre for Ecology, Evolution and Environmental Changes, Faculdade de Ciências, Universidade de Lisboa, Lisboa, Portugal
- 3Departamento de Biologia Vegetal, Faculdade de Ciências da Universidade de Lisboa, Lisboa, Portugal
- 4BioISI - Biosystems and Integrative Sciences Institute, Plant Functional Genomics Group, Departamento de Biologia Vegetal, Faculdade de Ciências da Universidade de Lisboa, Lisboa, Portugal
- 5Centro de Estudos Geográficos, Instituto de Geografia e Ordenamento do Território, Universidade de Lisboa, Lisboa, Portugal
- 6Laboratório Associado Terra, Lisboa, Portugal
- 7INOV-INESC, Lisboa, Portugal
- 8CeFEMA, Universidade de Lisboa, Lisboa, Portugal
- 9MARE - Marine and Environmental Sciences Centre and ARNET – Aquatic Research Infrastructure Network Associate Laboratory c/o Department of Zoology, Faculty of Sciences and Technology, University of Coimbra, Coimbra, Portugal
- 10MARE – Marine and Environmental Sciences Centre and ARNET – Aquatic Research Infrastructure Network Associate Laboratory, ESTM, Politécnico de Leiria, Peniche, Portugal
- 11Southern Seas Ecology Laboratories, School of Biological Sciences, The University of Adelaide, Adelaide, SA, Australia
- 12Departamento de Biologia Animal, Faculdade de Ciências da Universidade de Lisboa, Lisboa, Portugal
Emerging pollutants impose a high degree of stress on marine ecosystems, compromising valuable resources, the planet and human health. Pharmaceutical residues often reach marine ecosystems, and their input is directly related to human activities. Fluoxetine is an antidepressant, and one of the most prescribed selective serotonin reuptake inhibitors globally and has been detected in aquatic ecosystems in concentrations up to 40 μg L−1. The present study aims to evaluate the impact of fluoxetine ecotoxicity on the photochemistry, energy metabolism and enzyme activity of Ulva lactuca exposed to environmentally relevant concentrations (0.3, 0.6, 20, 40, and 80 μg L−1). Exogenous fluoxetine exposure induced negative impacts on U. lactuca photochemistry, namely on photosystem II antennae grouping and energy fluxes. These impacts included increased oxidative stress and elevated enzymatic activity of ascorbate peroxidase and glutathione reductase. Lipid content increased and the altered levels of key fatty acids such as hexadecadienoic (C16:2) and linoleic (C18:2) acids revealed strong correlations with fluoxetine concentrations tested. Multivariate analyses reinforced the oxidative stress and chlorophyll a fluorescence-derived traits as efficient biomarkers for future toxicology studies.
Introduction
The ongoing increase in human population with increasing access to therapeutic substances, allied to the inefficient or incomplete removal of anthropogenic-related by-products in wastewater treatment plants (WWTPs), have led to the detection of emerging pollutants (EP) in marine ecosystems (Fatta-Kassinos, Meric and Nikolaou, 2011; Gavrilescu et al., 2015; Kurwadkar et al., 2015; Tang et al., 2019). As reported by EUROSTAT (2017), between 2002 and 2011, over 50% of the manufactured chemicals were considered harmful to ecosystems, with 70% of them having environmental impacts. The toxicology assessment of Pharmaceuticals and Personal Care Products (PPCPs) is of utmost importance due to their environmental persistence and capability of inducing results at lower concentrations, not only in humans but also in an array of other species, from diatoms (Duarte et al., 2020; Feijão et al., 2020; Silva et al., 2020) to molluscs (Fong and Ford, 2014; Moreno-González et al., 2016) and fish (Brooks et al., 2003; Corcoran et al., 2010; Bell et al., 2011; Duarte et al., 2019). PPCPs not only easily dissolve in water but also tend to show characteristics such as high lipophilicity, low biodegradability rate, high bioaccumulation potential, and do not evaporate at normal aquatic temperature and pressure (Kurwadkar et al., 2015). Over the last decade and following the EU’s Task Group for the Marine Strategy Framework Directive implementation, recommendations for monitoring emerging contaminants and their effects on marine organisms increased. However, research on PPCPs exposure effects on many marine groups, namely macroalgae is still lacking (Law et al., 2010; Leston et al., 2011, 2014; Wiklund et al., 2011).
Due to the COVID-19 pandemic, an increase in the incidence of mental disorders, such as anxiety and depression, has been reported [e.g., in Canada (Uthayakumar, Kitchen and Gomes, 2021) and in France (Levaillant, 2021)], leading to increased consumption of anti-depressants. Fluoxetine is an antidepressant that selectively inhibits serotonin reabsorption (SSRI) and increases its concentration in the synaptic cleft (Brooks et al., 2003). It is often prescribed to treat various conditions and disorders, such as the abovementioned depression, but also anorexia, nervous bulimia, obsessive-compulsive or panic disorders (El-Bassat et al., 2011). The half-life of fluoxetine in humans and aquatic ecosystems is about 1–4 days (Lancaster and Gonzalez, 1989; Johnson et al., 2005; Sawyer and Howell, 2011) and, given its widespread use, it is frequently detected in aquatic environments (Vasskog et al., 2008; Mezzelani, Gorbi and Regoli, 2018; Reis-Santos et al., 2018). Concentrations can be as high as 40 μg L−1 (Ortiz, 2010; aus der Beek et al., 2016) and toxicity to various organisms has been reported (Fent, Weston and Caminada, 2006; Corcoran, Winter and Tyler, 2010). Since pharmaceuticals are designed to achieve results at the lowest possible concentrations in humans (Ebele, Abou-Elwafa Abdallah and Harrad, 2017) it is of the utmost importance to evaluate the consequences of their environmental presence on non-targeted species.
Being at the base of marine ecosystems, macroalgae are responsible for up to 50% of the global oxygen production and are a food and habitat source for numerous marine organisms (Areco, Salomone and Afonso, 2021). Found in the most various regions, from tropical to polar climates, the green macroalga Ulva lactuca can grow up to 30 cm wide, attached, sessile or free-floating and is known to form floating communities called green tides (Dominguez and Loret, 2019; Raj, Anandan and Mathew, 2020; Areco, Salomone and Afonso, 2021). Additionally, these macroalgae are used as compost or as an enriching agent in agriculture, as a food source in aquaculture, whereas its by-products (e.g. pigments, lipids and highly charged sulphated polysaccharides, known as Ulvan) find their application in cosmetic and pharmaceutical industries (Alam et al., 2016). From an environmental perspective, U. lactuca has been harnessed for biomonitoring Duarte et al., 2021a) and bioremediation of heavy metals (Trinelli, Areco and Dos Santos Afonso, 2013; Trinelli et al., 2019). Its cosmopolitan distribution, fast growth rates and tolerance to abiotic stresses, such as salinity (Bews et al., 2021) and heavy metals (Bonanno, Veneziano and Piccione, 2020; Areco, Salomone and Afonso, 2021), make this species an exceptional model in ecotoxicological studies.
In this context, the objective of this study was to evaluate fluoxetine exposure effects on the photochemistry, energy metabolism, and antioxidant biomarkers of the green macroalga Ulva lactuca, as well as to identify valuable and efficient early stress biomarkers of environmental contamination. To evaluate fluoxetine exposure-induced stress on U. lactuca photosynthesis, non-invasive high-throughput screening tools, such as pulse amplitude modulated (PAM) fluorometry and laser-induced fluorescence (LIF), were performed, which have been widely used in ecotoxicological studies (Cabrita et al., 2016; Gameiro et al., 2016; Duarte et al., 2021b). The extensive data produced by PAM fluorometry gives insight on light absorption, energy utilization and dissipation, and the state of the photosynthetic machinery, whilst LIF evaluates changes in the fluorescence peak in the red (685 nm) and far-red (735 nm) regions of the spectrum (Lavrov et al., 2012). The fluorescence peak ratio Fred/far-red depends mainly on the chlorophyll content of the sample but is also associated with fatty acid composition variations and other metabolic adaptations (Gameiro, Utkin and Cartaxana, 2015). To further investigate the fluoxetine-induced stress effects on U. lactuca, fatty acid composition and membrane saturation parameters were also assessed. Finally, stress resistance-related machinery such as carotenoid content (β-carotene and xanthophylls), total energy reserves quantification, mitochondrial electron transport system activity, and antioxidant enzyme activity were also evaluated following fluoxetine exposure.
Methods
Ulva lactuca growth conditions and ecotoxicological exposure
Ulva lactuca (Ulvaceae, Chlorophyta) was supplied by ALGAplus aquaculture (Ílhavo, Portugal) and maintained in filtered seawater enriched with Provasoli’s Enriched Seawater (PES) medium, under constant aeration (Leston et al., 2014). Cultures were maintained in a FytoScope FS 130 growth chamber (Photon Systems Instruments, Drásov, Czech Republic) using a sinusoidal function to simulate sunrise and sunset conditions. Light intensity was set at 40 μmol photons m−2 s−1 and temperature at 18 ± 1°C (Sand-Jensen, 1988; Feijão et al., 2017; Bonanno, Veneziano and Piccione, 2020).
At the beginning of the experiment, Ø1.5 cm algae discs were cut and placed in 24-well transparent plates with 3 ml PES medium for 48 h to allow algal recovery after cut-induced stress. After the first 24 h, the algal medium was replaced.
At the end of the 48-h acclimation period, the discs were separated into one control group and five treatment groups (N = 30 samples per group). The samples were exposed to several fluoxetine concentrations (0, 0.3, 0.6, 20, 40, and 80 μg L−1) that not only mimic environmental conditions, but also allow the assessment of future effects throughout a relevant gradient (Johnson et al., 2007; El-Bassat et al., 2011; Minguez et al., 2014; aus der Beek et al., 2016; Grzesiuk, Wacker and Spijkerman, 2016; Grzesiuk et al., 2018; Feijão et al., 2020). The exposure lasted 48 h to avoid nutrient deficiency and salinity increase according to Han et al. (2007). At the end of the exposure period, samples were monitored non-invasively using bio-optical methods (see next section) and immediately flash-frozen in liquid nitrogen for further analysis.
Photochemical assessment
At the end of the 48-h trial, all samples were dark-adapted for 15 min prior to PAM fluorometry measurements. To evaluate fluoxetine-exposure related effects on photochemistry, namely light absorption, chemical (potential) energy utilization and energy dissipation, the pre-programmed OJIP protocol available in the FluorPen FO100 (Photon System Instruments, Drásov, Czech Republic) was used. This standard protocol involves exposing the sample to short-duration modulated saturating light at 3500 μmol photons m−2 s−1 to induce a fluorescence curve known as the Kautsky Effect (Zhu et al., 2005; Feijão et al., 2020). From this analysis, a set of variables is obtained through the FluorPen1.1 software (Table 1).
Laser-Induced Fluorescence (LIF) spectra of U. lactuca discs were obtained through a frequency-doubled pulsed Nd:YAG laser Quantel Ultra 532-30-20-H-N and an optical receiver based on a commercial palm-size Ocean Optics USB4000 spectrometer as described by Gameiro et al. (2016). Reflected fluorescence was collected by a light-gathering optical train based on optomechanical elements from Thorlabs Inc. (Lavrov et al., 2012; Utkin et al., 2013; Gameiro et al., 2016). The spectrometer was tuned and calibrated using a mercury argon calibration source Mikropack CAL-2000 to demonstrate high sensitivity (approximately 55 photons per count) and resolution (0.19 nm per channel).
The analysis consists of exposing the sample to 30 mJ, 7 ns monochromatic (532 nm) radiation pulses, generated at approximately 20 Hz rate. To avoid disturbing effects due to reaction centre (RC) closure and excitation annihilation, the pulsed radiation was kept at a sufficiently low single-pulse fluence (Rosema et al., 1998) regulated by the dimension of the laser spot on the targeted surface. LIF-derived parameters such as the Fred/FFar-red (F685/F735) ratio and maximum fluorescence (Fmax) were obtained.
Pigment profiling
To assess fluoxetine-induced changes in pigment abundance, samples were freeze-dried for 48 h and then extracted with 3 ml of pure acetone for 24 h in the dark at −20°C to prevent pigment degradation (Cabrita et al., 2016, 2017; Feijão et al., 2020). Following centrifugation (4000 × g for 15 min at 4°C), supernatants were analysed using a UV-1603 spectrophotometer (Shimadzu, Kyoto, Japan) and read from 350 to 750 nm (0.5 nm steps). The obtained absorbance spectrums were introduced in the Gauss-Peak Spectra (GPS) fitting library and analysed through the algorithm developed by Küpper et al. (2007), enabling the detection of the most abundant pigments such as chlorophyll a (chl a) and b, pheophytin a (phaeo a), β-Carotene, zeaxanthin, antheraxanthin, violaxanthin, and auroxanthin.
Fatty acid analysis
Direct trans-esterification of U. lactuca discs was performed by adding freshly prepared methanol sulfuric acid (97.5:2.5, v/v) and subjecting the samples to 70°C for 60 min. Petroleum ether and Milli-Q water were added to recover the fatty acids methyl esters (FAMEs). Followed by a brief homogenization using the vortex and 5 min centrifugation (5000 × g), the upper phase was dried under a nitrogen flow in an SBH200D/2 sample concentrator (Stuart, Staffordshire, United Kingdom) at 30°C (Duarte et al., 2021a). FAMEs were resuspended in an appropriate amount of hexane and analysed in a Varian 430-GC gas chromatograph (Varian, Inc., CA, United States) equipped with a hydrogen flame ionization detector (FID) set at 300°C. The temperature of the injector was set to 270°C, with a split ratio of 50. The fused-silica capillary column (50 m × 0.25 mm; WCOT Fused Silica, CP-Sil 88 for FAME; Varian, Inc.) was maintained at a constant nitrogen flow of 2.0 ml min−1 and the oven set at 190°C. Fatty acid identification was performed by comparison of retention times with standards (Sigma-Aldrich), and chromatograms were analysed by the peak surface method, using the Galaxy software. The internal standard used was pentadecanoic acid (C15:0) (Feijão et al., 2017). The saturation-derived variables (saturated fatty acids, SFA; unsaturated fatty acids, UFA; monounsaturated fatty acids, MUFA; Polyunsaturated fatty acids, PUFA; SFA/UFA; PUFA/SFA) were determined and the double bond index (DBI) was calculated as follows:
Oxidative stress biomarkers
Fluoxetine-exposed and control U. lactuca discs were homogenized in a mortar and pestle using liquid nitrogen. The soluble protein fraction was extracted at 4°C in 500 µl of a previously mixed solution consisting of 50 mM sodium phosphate buffer (pH 7.6) with 0.1 mM Na-EDTA. Following sample centrifugation at 15,000 g for 10 min at 4°C (Sigma 2–16K, Sigma Laborzentrifugen GmbH, Germany), the supernatant was collected. To assess the enzymatic activity of catalase (CAT, EC 1.11.1.6), ascorbate peroxidase (APx, EC 1.11.1.11), superoxide dismutase (SOD, EC 1.15.1.1) and glutathione reductase (GR, EC 1.8.1.7), measurements were performed at 25°C in an Epoch 2 Microplate Reader (BioTek Instruments, VA, United States). Measurement of CAT activity was performed according to Teranishi et al. (1974) by monitoring H2O2 consumption and consequent decrease in absorbance at 240 nm (ε = 39.4 M−1 cm−1). Determination of APx activity was performed according to Tiryakioglu et al. (2006) through measurement of ascorbate oxidation observed as a decrease in absorbance at 290 nm (ε = 2.8 mM−1 cm−1). Following the protocol of Marklund and Marklund (1974), SOD activity was assayed by monitoring pyrogallol reduction shown as an absorbance increase at 325 nm. Pyrogallol autoxidation was read without enzymatic extract throughout the reading interval to allow the comparison of results. To determine GR activity, glutathione-dependent oxidation of NADPH and its decrease in absorbance at 340 nm (ε = 6.22 mM−1 cm−1) were monitored according to Edwards, Rawsthorne and Mullineaux (1990). The oxidative ratio was determined as:
To evaluate changes in lipid peroxidation, U. lactuca discs were homogenized in 1 ml of fresh solution containing 0.5% of Thiobarbituric acid (TBA) and 20% Trichloroacetic acid (TCA). Samples were placed at 90°C for 30 min in an Accublock Digital Dry Bath (Labnet Inc., CA, United States). After stopping the reaction on ice and the following centrifugation at 15,000 g for 10 min at 4°C, samples were analysed in a UV-1603 spectrophotometer (Shimadzu, Kyoto, Japan). Absorbances were measured at 532 and 600 nm and the concentration of malondialdehyde (MDA) was determined according to Heath and Packer (1968) using the molar extinction coefficient, 155 mM−1 cm−1.
Energy balance
Energy available
Using 1 ml of Mili-Q water (18.2 MΩ cm), discs were homogenized by mortar and pestle. Aliquots from each sample were used to assess lipid, carbohydrate and protein contents and electron transport system (ETS) activity. Mili-Q water was used as a reaction blank. The spectrophotometric measurements were performed at 25°C in a synergy H1 Hybrid Multi-Mode microplate reader (Biotek® Instrument, Vermont, United States).
To assess energy available (Ea), the results obtained regarding lipid, carbohydrate, and protein content (following the methodologies described in De Coen and Janssen (1997), were transformed into energetic equivalents. The conversion was performed using the respective combustion energies as reported by Gnaiger (1983): 17,500 mJ mg carbohydrates−1, 24,000 mJ mg protein−1, and 39,500 mJ mg lipid−1.
Energy consumption
The consumption and metabolism of cellular oxygen are directly linked to mitochondrial ETS activity. Therefore, energy consumption (Ec) was determined using ETS data (for each 2 μmol of INT-formazan formed, 1 μmol of O2 was consumed), converting the result into energetic equivalents using the specific oxyenthalpic equivalents for an average lipid, protein and carbohydrate mixture (480 kJ mol O2−1) reported by Gnaiger (1983). ETS assessment protocol was performed as described by King and Packard (1975) with major modifications (Aderemi et al., 2018).
Cellular energy allocation
The cellular energy allocation (CEA), standardized to mg−1 FW, was determined based on the previous lipid, carbohydrate and protein content measurements and respective ETS activity (Verslycke, Ghekiere and Janssen, 2004).
Statistical analysis
Spearman correlation coefficients and statistical significance among biophysical and biochemical parameters were obtained. Non-parametric Kruskal–Wallis tests with Bonferroni post hoc comparisons were also performed. These analyses were performed in R studio 1.4.1717 via packages “corrplot” (Wei and Simko, 2021) and “agricolae” (De Mendiburu and Simon, 2015). Canonical Analysis of Principal Coordinates (CAP) was performed to evaluate the ability of biochemical and biophysical traits to successfully classify discs according to fluoxetine concentration exposure. The benefit of this multivariate approach is its robustness in analysing heterogeneous data, which is why it is frequently used to compare different sample groups based on their intrinsic characteristics (Duarte et al., 2021c; Pires et al., 2021). Multivariate statistical analysis procedures were conducted using Primer 6 software (Clarke and Gorley, 2006).
Results
Fluoxetine exposure impact on U. lactuca photochemistry
Regarding the Kautsky curve fluorescence data points, it is possible to observe that all samples exposed to fluoxetine reveal higher fluorescence emission and curve shape changes compared to the control samples (Figure 1A). The oxidized quinone pool (area) was lower in samples exposed to the two highest fluoxetine concentrations (40 and 80 μg L−1), with no statistical difference between them (Figure 1B). The energy needed to close all reaction centres (Figure 1C, SM) decreased in samples exposed up to 0.6 μg L−1 of fluoxetine, then increased, registering the highest value at 80 μg L−1. The turnover rate of dark-adapted reaction centres (N) and the probability of an electron moving from quinone B (QB) to the Photosystem I (PSI) end-electron acceptor (δR0) followed the same pattern (Figures 1D,E). The dysconnectivity between two PSII antennae increased, as evidenced by an increase in grouping probability (PG) in samples exposed to the two highest fluoxetine concentrations tested (Figure 1F).
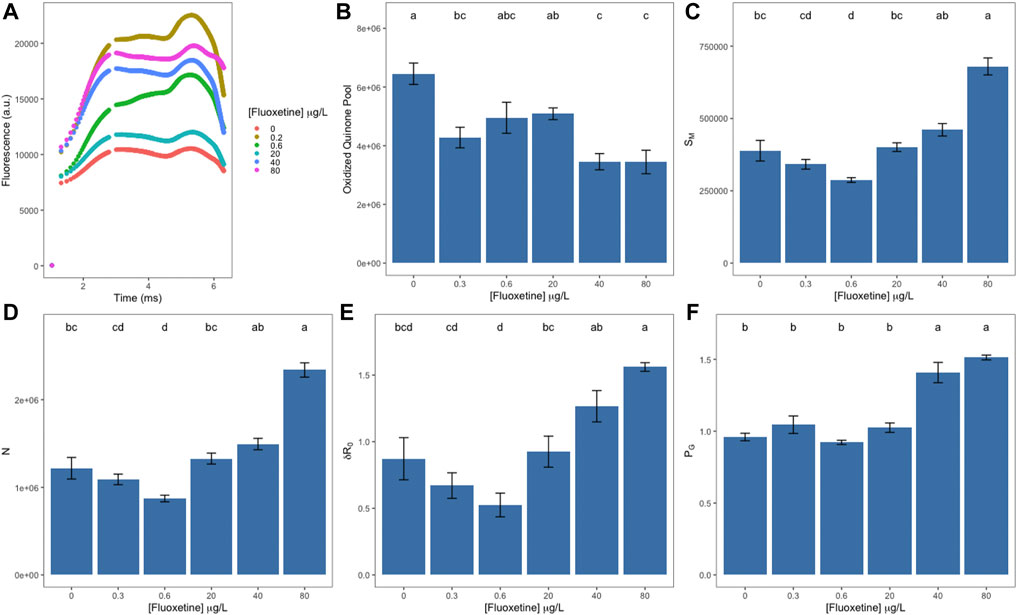
FIGURE 1. OJIP-derived parameters of Ulva lactuca following a 48-h exposure to different fluoxetine concentrations. (A) Kautsky chlorophyll a fluorescence induction curves. (B) Oxidized quinone pool. (C) Energy needed to close all reaction centres (SM). (D) Reaction centre turnover rate (N). (E) Probability of moving an electron from quinone B (QB) to the PSI end-electron acceptor (δR0). (F) Grouping probability (PG). Average ± standard error, N = 5; different letters indicate significant differences at p < 0.05.
When exposed to increasing concentrations of fluoxetine, U. lactuca suffered a decrease in energy absorption (ABS/CS, Figure 2A) starting at 0.3 μg L−1, with a maximum decrease observed at the two highest fluoxetine concentrations tested (40 and 80 μg L−1). Samples exposed to these highest concentrations also revealed a strong decrease in trapped (TR/CS) and transported (ET/CS) energy (Figures 2B,C). Dissipated energy flux (DI/CS, Figure 2D) decreased when U. lactuca was exposed to low fluoxetine concentrations (0.3 and 0.6 μg L−1), returning to control levels at higher concentrations (Figure 2D). Oxidized reaction centre density (RC/CS) was significantly depleted in samples exposed to the two highest fluoxetine concentrations (40 and 80 μg L−1), registering the lowest value at 80 μg L−1 (Figure 2E).
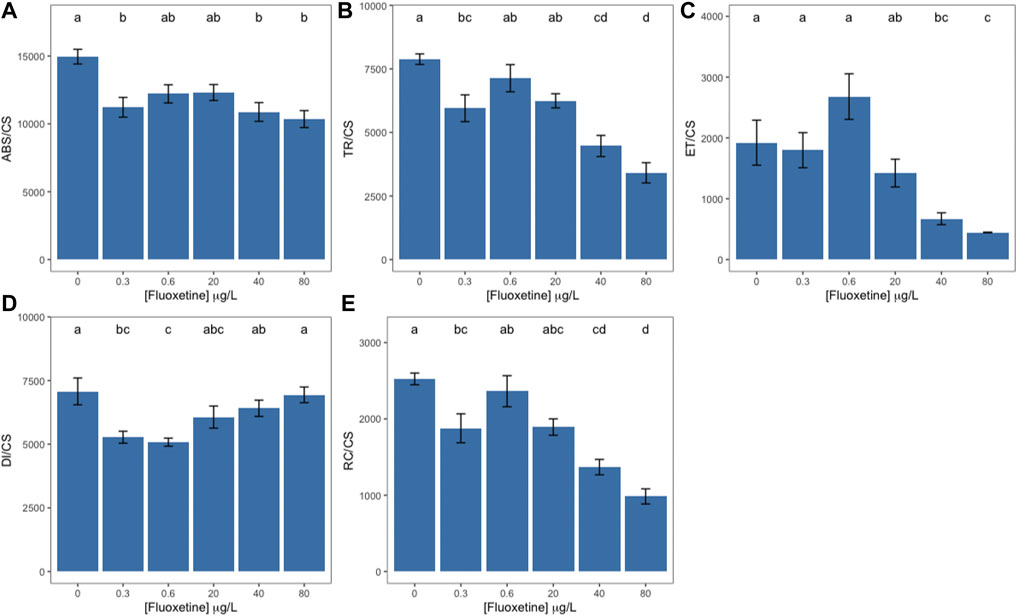
FIGURE 2. Ulva lactuca phenomological energy fluxes following a 48-h exposure to different fluoxetine concentrations. (A) Energy absorption per cross section (ABS/CS). (B) Trapped energy per cross section (TR/CS). (C) Electron transport per cross section (ET/CS). (D) Energy dissipation per cross section (DI/CS). (E) Number of reaction centres available per cross-section (RC/CS). Average ± standard error, N = 5; different letters indicate significant differences at p < 0.05.
The obtained fluorescence spectra of fluoxetine-exposed U. lactuca samples revealed that exposure to the two lowest fluoxetine concentrations (0.3 and 0.6 μg L−1) induced a fluorescence increase at 685 nm when compared to unexposed samples, registering the highest value in samples exposed to 0.3 μg L−1 of fluoxetine (Figure 3A). Fluoxetine concentrations above 20 μg L−1 induced an abrupt decrease at the same wavelength (Figure 3A). Maximum absorbance at the red region (Figure 3B) revealed a slight increase in samples exposed to the lowest concentrations, followed by a statistically significant decrease in those exposed to the highest concentrations (40 and 80 μg L−1) while within the far-red region (Figure 3C) fluoxetine-exposed samples only showed a statistically significant decrease at 20 μg L−1. Wavelength deviation where maximum red and far-red fluorescence was attained (Figures 3D,E respectively) presented an overall sinusoidal shape, with positive values in samples exposed to lower concentrations and negative in samples exposed to the highest concentrations of fluoxetine. The F685/F735 ratio slightly decreased with increasing concentrations, registering the lowest value in samples exposed to the highest fluoxetine concentration (Figure 3F). Maximum fluorescence presented a statistically significant decrease in samples exposed to fluoxetine concentrations above 20 μg L−1 (Figure 3G). Maximum wavelength and its deviation did not show any statistically significant differences between control and fluoxetine-exposed samples (Figures 3H,I).
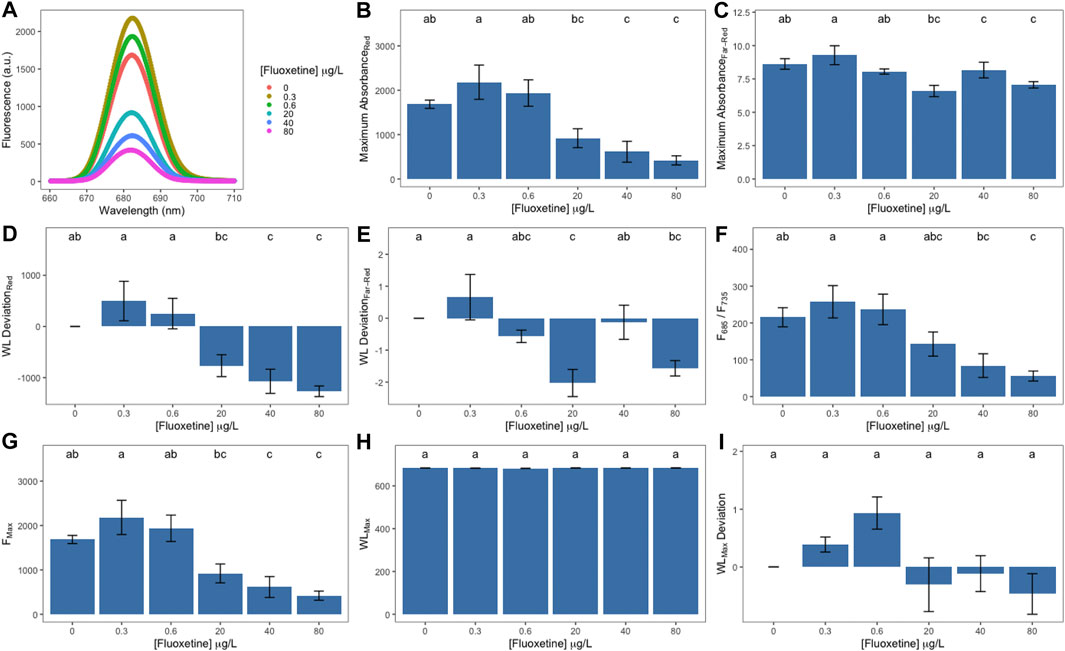
FIGURE 3. LIF-derived parameters of Ulva lactuca following a 48-h exposure to different fluoxetine concentrations. (A) Relative fluorescence. (B) Maximum absorbance at the red region. (C) Maximum absorbance at the far-red region. (D) Wavelength deviation at the red region. (E) Wavelength deviation at the far-red region. (F) Red/Far-red fluorescence ratios (F685/F735). (G) Maximum fluorescence (Fmax). (H) Maximum wavelength (WLMax). (I) Maximum wavelength deviation (WLMax Deviation). Average ± standard error, N = 5; different letters indicate significant differences at p < 0.05.
Photosynthetic pigments and carotenoid concentration
Photosynthetic pigments such as chlorophyll a and b (chl a and chl b) as well as phaeophytin a (phaeo a), a degradation product of chl a, did not show any statistically different variation induced by fluoxetine exposure (Supplementary Figures S1A–C). Moreover, the xanthophyll cycle-related pigments and the de-epoxidation state did not show any statistically significant variation (Supplementary Figures S1D–I).
Fatty acid composition and membrane fluidity
The most abundant fatty acids found in U. lactuca are the unsaturated palmitic acid (C16:0), the polyunsaturated linolenic acid (C18:3), followed by the hexadecatrienoic (C16:3) and monounsaturated oleic (C18:1) acids (Supplementary Figure S2A). With the exception of increased oleic acid concentration, fluoxetine exposure did not significantly affect the fatty acid relative abundance, double bond index (DBI) and unsaturation ratios (SFA/UFA and PUFA/SFA) of exposed macroalgae (Supplementary Figures S2B–D).
Oxidative stress biomarkers
Samples exposed to the highest fluoxetine concentrations revealed reduced catalase and increased ascorbate peroxidase activities (Figures 4A,B, respectively). When exposed to 40 and 80 μg L−1 of fluoxetine, samples registered a steep decrease in superoxide dismutase activity, with a simultaneous decrease of the oxidative ratio to zero (Figures 4C,D). Glutathione reductase activity tended to increase with increasing exogenous fluoxetine concentrations (>0.3–80 μg L−1) (Figure 4E). Lipid peroxidation products also increased significantly registering the highest value in samples exposed to 40 μg L−1 (Figure 4F).
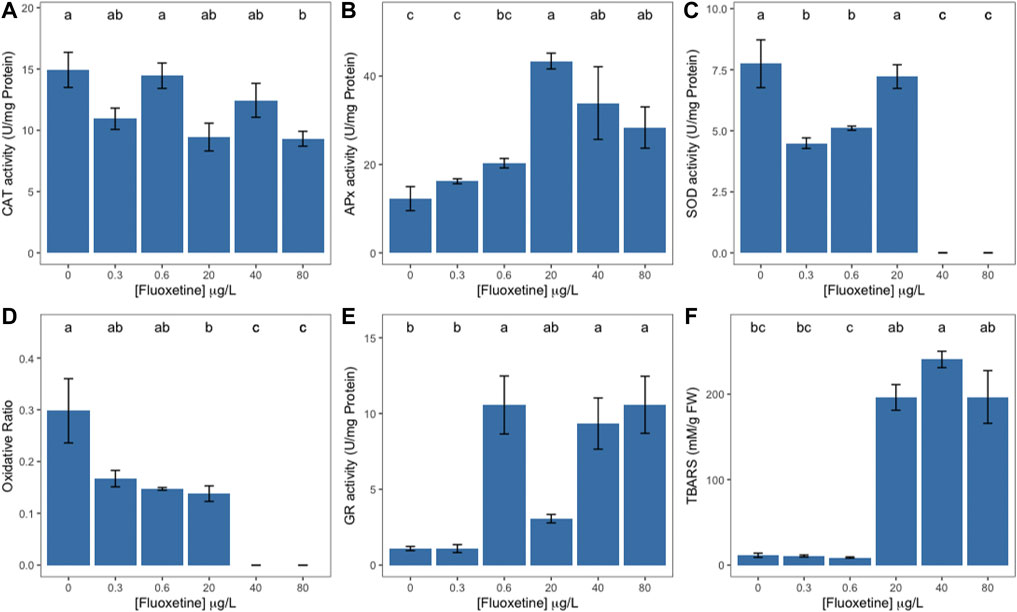
FIGURE 4. Ulva lactuca oxidative stress enzyme activity and lipid peroxidation products following a 48-h exposure to different fluoxetine concentrations. (A) Catalase (CAT). (B) Ascorbate Peroxidase (APx). (C) Superoxide Dismutase (SOD). (D) Oxidative ratio. (E) Glutathione reductase (Gr). (F) Thiobarbituric Acid Reactive Substances (TBARS). Average ± standard error, N = 3; different letters indicate significant differences at p < 0.05.
Energy balance
Although no statistically significant change in the carbohydrate content was observed, macroalgae discs showed an increase in lipid content starting at the exogenous fluoxetine concentration of 0.6 μg L−1 and peaking at 40 μg L−1 (Figures 5A,B). Protein content registered a significant decrease in samples exposed to intermediate fluoxetine concentrations (0.6–40 μg L−1), whilst samples exposed to 80 μg L−1 showed no statistically significant difference (Figure 5C). The mitochondrial electron transport (ETS) revealed a tendency to decrease in fluoxetine-exposed samples (Figure 5D) while the available energy (Ea) and cellular energy allocation did not vary significantly (Figures 5E,F).
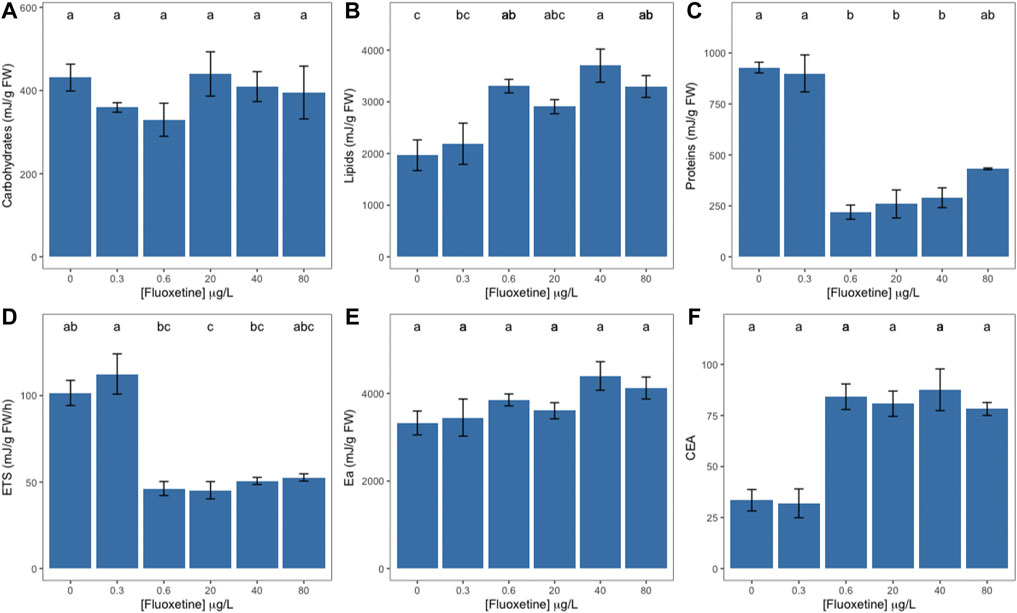
FIGURE 5. Ulva lactuca energetic metabolism at the mitochondria level of Ulva lactuca following a 48-h exposure to different fluoxetine concentrations. (A) Carbohydrates. (B) Lipids. (C) Proteins. (D) Electron Transport System (ETS). (E) Available energy (Ea). (F) Cellular energy allocation (CEA). Average ± standard error, N = 3; different letters indicate significant differences at p < 0.05.
Biomarker integrated profiles
To analyse the relationship between the biophysical and biochemical traits, Spearman correlations are presented in Figure 6. Exogenous exposure to fluoxetine induced strong negative correlations among the fluorometric data, namely the LIF-derived parameters and the OJIP-derived energy fluxes, except for the dissipated energy flux. The strong negative trend was also evident for the oxidative enzymatic activities, specifically for catalase and superoxide dismutase activities. Although no statistically significant variations were observed in pigment abundance, the highest positive Spearman correlations were registered in the xanthophyll cycle. Similarly, to the latter, despite the lack of statistical differences in the fatty acid-derived data, a strong positive correlation between the exogenous fluoxetine concentration and membrane fluidity, here evaluated via the double bond index (DBI), was observed. Additionally, several correlations were found between other analysed traits namely, strong negative correlations between the xanthophyll cycle pigments and fluorometric variables (the F685/F735 ratio and energy fluxes), and strong positive correlations between the F685/F735 ratio and fatty acids (C16:2, C18:2, DBI), enzyme activity (CAT and SOD), lipids, proteins, and therefore Ea and CEA.
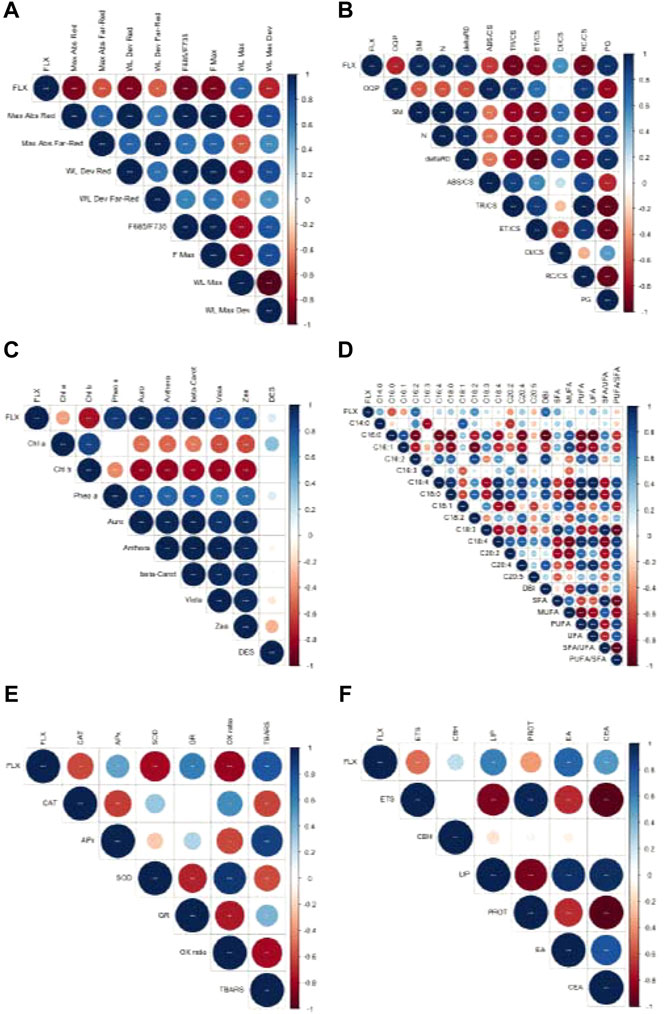
FIGURE 6. Ulva lactuca Spearman correlations with fluoxetine exposure. (A) LIF-derived variables. (B) PAM-derived variables. (C) Pigment concentration variables. (D) Fatty acid analysis variables. (E) Oxidative stress variables. (F) Energy metabolism variables. Shades of blue indicate positive correlations while shades of red indicate negative correlations between parameters. N = 30 (fluorometric data); N = 3 (pigments, oxidative stress and energetic biomarkers and fatty acids); asterisks indicate statistically significant differences at p < 0.05 (*), 0.01 (**) and 0.001 (***).
Multivariate Canonical Analysis of Principal Coordinates
The multivariate Canonical Analysis of Principal Coordinates (CAP) allowed the evaluation of the efficiency of the various photochemical and biochemical datasets to be used as biomarkers to accurately separate the different fluoxetine exposure groups. Biochemical and biophysical traits were analysed in groups, namely PAM and LIF (Figures 7A,B), fatty acids relative abundance (Figure 7C), pigment concentration (Figure 7D) and the oxidative stress data (Figure 7E). Oxidative stress biomarkers had the highest classification accuracy (>80%) in distinguishing the different exposure groups, followed by the Kautsky fluorescence data (>70%) (Figure 7F). Fatty acids relative abundance presented the lowest accuracy when classifying U. lactuca samples according to the fluoxetine exposure to which they were subjected (<25%).
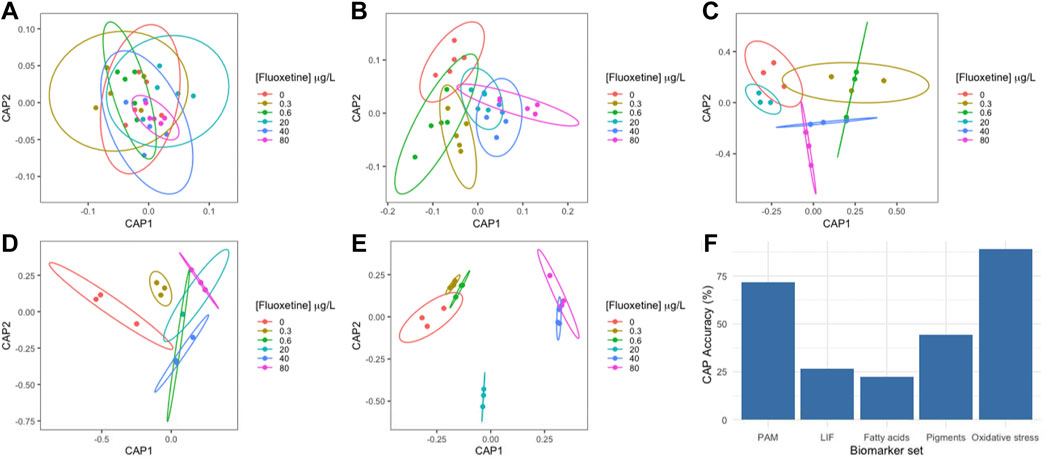
FIGURE 7. Ulva lactuca Canonical Analysis of Principal components (CAP) following a 48-h exposure to different fluoxetine concentrations. (A) PAM-derived variables. (B) LIF-derived variables. (C) Fatty acid profile. (D) Pigment profile. (E) Oxidative stress biomarkers. (F) CAP accuracy. N = 5 (PAM and LIF); N = 3 (Fatty acids, pigments, and oxidative stress).
Discussion
Early detection of xenobiotic toxicity in marine environments throughout the trophic web is paramount given the constant re-introduction, persistence, unknown interactions with non-targeted species, and synergistic effects of emerging pollutants such as PPCPs (Bi et al., 2018; Duarte et al., 2021b; Pires et al., 2021). Not only is it valuable to have the possibility of efficiently assessing toxicity through non-destructive high-throughput physical techniques, such as PAM fluorometry and LIF, but these methods allow for fast insights on known chemical pathways, due to the intrinsic correlation between energy conversion, oxidative stress, and biomass production.
In humans, the mode of action of SSRIs, such as fluoxetine has been extensively detailed and is reported to induce a serotonin concentration increase at the synaptic cleft due to its reabsorption inhibition (Brooks et al., 2003). However, non-targeted organisms, such as unicellular microalgae or multicellular macroalgae may respond differently to fluoxetine exposure when compared to other organisms that are structurally, chemically and genetically more complex (Fent, Weston and Caminada, 2006; Minguez et al., 2014; Bi et al., 2018). In the present study, U. lactuca exposed to increasing exogenous fluoxetine concentrations showed a significant dose-related decrease in energy absorption and trapped energy flux. A similar response has already been reported in the microalgae Phaeodactylum tricornutum, exposed to the same fluoxetine concentrations (Feijão et al., 2020), which is often associated with lower efficiency in light-harvesting and exciton energy generation from captured photons (Duarte et al., 2021d) and commonly reported in microalgal exposure to other PPCPs (Silva et al., 2020) and pesticides (de Carvalho et al., 2020). The photosynthetic response seems to mirror the metabolic changes occurring in P. tricornutum, namely a decrease in the oxidized quinone pool and RC density, with a significantly increased quinone turnover and energy needed to close all RCs. The connectivity between two PSII antennae gradually decreased (inversely to PG) as exogenous fluoxetine concentrations increased (Feijão et al., 2020). The outcome of PSII antenna dysconnectivity lies in the inevitable electron transport impairment (Duarte et al., 2017), and is associated with the abovementioned reduced quinone pool and the turnover rate causing quinone overreduction and increased electron leakage to O2 and subsequent superoxide radical (O2·−) formation (Cleland and Grace, 1999; Takagi et al., 2016; Ivanov, Borisova-Mubarakshina and Kozuleva, 2018). However, due to the cessation of SOD activity observed in the macroalgae samples exposed to the highest fluoxetine concentrations, quinone overreduction might have occurred due to the increased content in lipid peroxidation products, despite generally increased antioxidant enzymes activities (APx, GR). This often leads to the activation of photoprotective mechanisms such as β-carotene mobilization to quench singlet oxygen (1O2) resulting from lipid degradation (Telfer et al., 2002; Telfer, 2014; Pospíšil, 2016). While β-carotene content did not increase, a strong positive correlation was observed with exogenous fluoxetine concentration, indicating that at higher fluoxetine concentrations U. lactuca might prioritize carotenoid production to counteract photosynthesis-derived oxidative stress.
Regarding the other H2O2-scavenging enzyme, namely CAT, fluoxetine exposure appears to have a more prominent effect on its activity at higher organizational levels when comparing the response of P. tricornutum to U. lactuca. Catalase activity seemed to be less affected in P. tricornutum, where it remained stable than in macroalgae like U. lactuca which showed a significant decrease in CAT activity at the highest exogenous fluoxetine concentration of 80 μg L−1 (Feijão et al., 2020). In P. tricornutum, APx, which has a higher affinity for H2O2 (Das and Roychoudhury, 2014), revealed a significant increase at the highest exogenous fluoxetine concentration, while in U. lactuca it was the only H2O2-scavenging enzyme that increased its activity when exposed to fluoxetine concentrations above 20 μg L−1 (Feijão et al., 2020). GR is a versatile enzyme with high reductive potential involved in the reduction of glutathionedisulfide to glutathione which can scavenge numerous ROS (H2O2, 1O2, O2·−) and has been reported to be involved in multiple cellular processes, including xenobiotic detoxification (Mullineaux and Rausch, 2005). In U. lactuca, GR activity increased and showed a strong positive correlation with exogenous fluoxetine concentration, highlighting the occurrence of fluoxetine-induced stress.
Variations in chl content have been reported in photosynthetic organisms following exposure to fluoxetine and other SSRIs, such as freshwater plankton colonizing quartz rocks (Yang et al., 2019), algal biofilms (Richmond et al., 2019), and diatoms (Feijão et al., 2020). In this study, while chl a content did not vary, the FRed/FFar-Red which often detects chl a content variations (Chappelle et al., 1984; Hák, Lichtenthaler and Rinderle, 1990) and is highly sensitive to stressors such as dehydration in plants and macroalgae, did decrease in samples exposed to higher fluoxetine concentrations revealing possible underlying molecular changes in known metabolic pathways such as anthocyanin content and lipids (Bartošková et al., 1999; Gameiro et al., 2016). This decrease in the FRed/FFar-Red ratio was mostly due to a more pronounced decrease in fluorescence in the red region which is often related to changes in PSII electronic transitions. However, a decrease in fluorescence was also observed in fluoxetine-exposed samples in the far-red region which implies changes in vibrational energy sublevels (Govindjee, 1995; Franck, Juneau and Popovic, 2002).
The total lipid content was significantly increased in fluoxetine-exposed samples at most concentrations above 0.6 μg L−1, showing a strong negative correlation with the FRed/FFar-Red ratio. While available energy and CEA did not show any significant changes, both revealed strong positive correlations with exogenous fluoxetine concentration, implying a bigger net energy budget for plant growth and division (Verslycke, Ghekiere and Janssen, 2004). However, given the low photosynthetic ET/CS and negative trend in the mitochondrial ETS, in energetic terms, it seems that fluoxetine exposure might be reducing both autotrophic energy production and respiratory activity (Duarte et al., 2020).
Neutral lipids (NL) which include triacylglycerol (TAG) seem to be a big component (55%) of some Chlorophyta such as Ulva armoricana (Kendel et al., 2015) and often increase under stress conditions in macroalgae and higher plants (Klok et al., 2014; Du and Benning, 2016; Ferreira et al., 2021), so it cannot be excluded the possibility that the lipid content increase might be associated with NL content increase reinforcing the prevalence of fluoxetine-induced stress. Although lipid profiling was not assessed, the strong positive correlations between key fatty acids and fluoxetine exposure should be highlighted, namely the C16:2 and C18:2 fatty acids. Digalactosyldiacylglycerol (DGDG) was found to be the only polar lipid with C16:2 in U. lactuca from the Adriatic and Sea of Japan making this fatty acid a possible biomarker for DGDG content variations (Kostetsky et al., 2018). Similarly, although not exclusive from this lipid class, C18:2 was found mostly present in DGDG in U. lactuca (Barkina et al., 2020). The galactolipids DGDG and monogalatosyldiacylglycerol (MGDG) are the most abundant lipids in plant thylakoid membranes and they are reported to be responsible for photosystem stabilization and electron modulation at the quinone level (Sakurai et al., 2007; Kern and Guskov, 2011; Mizusawa and Wada, 2012). More importantly, DGDG is also known to protect against Mn cluster dissociation in the O2-evolving complexes and therefore negative impacts on its content can impair PSII activity (Sakurai et al., 2007). Although membrane fluidity (DBI) did not change, a strong positive correlation was observed with fluoxetine exposure reinforcing the hypothesis that U. lactuca might be prioritizing membrane integrity over photosynthesis stabilization.
Aside from all the physiological meaning in terms of a possible mode of action of fluoxetine on non-target organisms, namely macroalgae, it is also important to highlight the possibility of using some of these metabolic features as potential fluoxetine exposure biomarkers. Overall, the biomarker CAP analysis demonstrated that chlorophyll a fluorescence (PAM: >70% correct classification accuracy) and oxidative stress-derived biomarkers (Oxidative stress: 100%) are the best non-invasive and invasive techniques, respectively, to assess fluoxetine exposure in U. lactuca. Bio-optical techniques such as PAM have the advantage to allow performing a non-invasive evaluation of the organisms, producing a high amount of data that can efficiently act as biomarker variables or be integrated into multivariate biomarker indexes (Duarte, et al., 2021c; Duarte et al., 2021d; Lemos, 2021), and used for exposure assessment. The assessed oxidative stress biomarkers have been demonstrated to be reliable from diatoms to plants exposed to stressors such as heavy metals, pharmaceuticals and pesticides (de Carvalho et al., 2020; Pires et al., 2021). Thus, bio-optical and oxidative stress-derived biomarkers are highlighted as efficient approaches for future fluoxetine exposure toxicity assessments in U. lactuca.
Conclusion
It is clear from this study that exogenous exposure to fluoxetine causes significant stress on the energy metabolism of U. lactuca. The negative impacts led to increased oxidative stress and activation of photoprotective pathways such as antioxidant enzyme activity. Non-invasive high-throughput techniques like PAM and LIF have the potential to detect signs of fluoxetine-induced stress before its classical tangible markers such as chl a content and fatty acid abundance suffered abrupt declines or variations. The present study highlights the effects of fluoxetine exposure on a non-target organism that are concomitant with the effects that SSRI has on other organisms ranging from diatoms to fish. Therefore, the potential impact of fluoxetine on the trophic chain is of concern. Given the negative bearings observed after a short-term exposure analysed in this work, it could be expected that long-term fluoxetine exposure and possible synergistic effects with other EPs commonly found in aquatic environments, may potentiate fluoxetine toxicity to macroalgae and other marine biota, affecting not only macroalgae communities but also the trophic webs and ecosystems of which they are part.
Data availability statement
The raw data supporting the conclusions of this article will be made available by the authors, upon reasonable request.
Author contributions
BD, VF, and PR-S conceived and designed the experiments. EF, RC, ID, AU, and SN performed the experiments and data processing. EF wrote the manuscript. AM, MC, AU, IC, JM, and ML provided technical and editorial assistance. All authors contributed to the article and approved the submitted version.
Funding
The authors would like to thank Fundação para a Ciência e a Tecnologia (FCT) for funding the research at MARE (UIDB/04292/2020 and UIDP/04292/2020), ARNET-Aquatic Research Infrastructure Network Associated Laboratory (LA/P/0069/2020), BioISI (UID/MULTI/04046/2019) and via project grant PTDC/CTA-AMB/30056/2017 (OPTOX). Work was also funded by the Integrated Programme of SR&TD SmartBioR (reference Centro-01-0145-FEDER-000018), co-funded by Centro 2020 program, Portugal 2020, European Union, through the European Regional Development Fund. BD and VF were supported by researcher contracts (CEEC-IND/00511/2017 and 2021.00244.CEECIND). MC and SN is supported by a DL-57 investigation contract.
Acknowledgments
EF and Rd would like to thank Sara Šariri and Lauren Snauwaert for their help and technical assistance.
Conflict of interest
The authors declare that the research was conducted in the absence of any commercial or financial relationships that could be construed as a potential conflict of interest.
Publisher’s note
All claims expressed in this article are solely those of the authors and do not necessarily represent those of their affiliated organizations, or those of the publisher, the editors and the reviewers. Any product that may be evaluated in this article, or claim that may be made by its manufacturer, is not guaranteed or endorsed by the publisher.
Supplementary material
The Supplementary Material for this article can be found online at: https://www.frontiersin.org/articles/10.3389/fenvs.2022.963537/full#supplementary-material
References
Aderemi, A. O., Novais, S. C., Lemos, M. F., Alves, L. M., Hunter, C., and Pahl, O. (2018). Oxidative stress responses and cellular energy allocation changes in microalgae following exposure to widely used human antibiotics. Aquat. Toxicol. 203, 130–139. doi:10.1016/j.aquatox.2018.08.008
Alam, S. S., Abd El-Kader, H. A. M., Abd El-Rahim, A. H., Hamed, S. M., and Saber, A. A. (2016). The protective role of Ulva lactuca against genotoxic and biochemical effects induced by γ-irradiation in rats. Int. J. Pharm. Sci. Rev. Res. 37 (2), 40–48.
Areco, M. M., Salomone, V. N., Afonso, M., and dos, S. (2021). Ulva lactuca: A bioindicator for anthropogenic contamination and its environmental remediation capacity. Mar. Environ. Res. 171, 105468. doi:10.1016/j.marenvres.2021.105468
aus der Beek, T., Weber, F. A., Bergmann, A., Hickmann, S., Ebert, I., Hein, A., et al. (2016). Pharmaceuticals in the environment-Global occurrences and perspectives. Environ. Toxicol. Chem. 35 (4), 823–835. doi:10.1002/etc.3339
Barkina, M. Y., Pomazenkova, L. A., Chopenko, N. S., Velansky, P. V., Kostetsky, E. Y., and Sanina, N. M. (2020). Influence of warm-acclimation rate on polar lipids of ulva lactuca. Russ. J. Plant Physiol. 67 (1), 111–121. doi:10.1134/S1021443720010021
Bartošková, H., Nauš, J., and Výkruta, M. (1999). The arrangement of chloroplasts in cells influences the reabsorption of chlorophyll fluorescence emission. The effect of desiccation on the chlorophyll fluorescence spectra of Rhizomnium punctatum leaves. Photosynth. Res. 62 (2), 251–260. doi:10.1023/a:1006333411167
Bell, K. Y., Wells, M. J. M., Traexler, K. A., Pellegrin, M-L., Morse, A., and Bandy, J. (2011). Emerging pollutants. Water Environ. Res. doi:10.2175/106143011x13075599870298
Bews, E., Booher, L., Polizzi, T., Long, C., Kim, J. H., and Edwards, M. S. (2021). Effects of salinity and nutrients on metabolism and growth of Ulva lactuca : Implications for bioremediation of coastal watersheds. Mar. Pollut. Bull. 166, 112199. doi:10.1016/j.marpolbul.2021.112199
Bi, R., Zeng, X., Mu, L., Hou, L., Liu, W., Li, P., et al. (2018). Sensitivities of seven algal species to triclosan, fluoxetine and their mixtures. Sci. Rep. 8 (1), 1–10. doi:10.1038/s41598-018-33785-1
Bonanno, G., Veneziano, V., and Piccione, V. (2020). The alga Ulva lactuca (Ulvaceae, Chlorophyta) as a bioindicator of trace element contamination along the coast of Sicily, Italy. Sci. Total Environ. 699, 134329. doi:10.1016/j.scitotenv.2019.134329
Brooks, B. W., Foran, C. M., Richards, S. M., Weston, J., Turner, P. K., Stanley, J. K., et al. (2003). Aquatic ecotoxicology of fluoxetine. Toxicol. Lett. 142 (3), 169–183. doi:10.1016/S0378-4274(03)00066-3
Cabrita, M. T., Gameiro, C., Utkin, A. B., Duarte, B., Cacador, I., and Cartaxana, P. (2016). Photosynthetic pigment laser-induced fluorescence indicators for the detection of changes associated with trace element stress in the diatom model species Phaeodactylum tricornutum. Environ. Monit. Assess. 188 (5), 285. doi:10.1007/s10661-016-5293-4
Cabrita, M. T., Padeiro, A., Amaro, E., dos Santos, M. C., Leppe, M., Verkulich, S., et al. (2017). Evaluating trace element bioavailability and potential transfer into marine food chains using immobilised diatom model species Phaeodactylum tricornutum, on King George Island, Antarctica. Mar. Pollut. Bull. 121 (1–2), 192–200. doi:10.1016/j.marpolbul.2017.05.059
Chappelle, E. W., Wood, F. M., McMurtrey, J. E., and Newcomb, W. W. (1984). Laser-induced fluorescence of green plants. 1: A technique for the remote detection of plant stress and species differentiation. Appl. Opt. 23 (1), 134–138. doi:10.1364/AO.23.000134
Clarke, K., and Gorley, R. N. (2006). PRIMER v6: User manual/tutorial, 29. Plymouth’: PRIMER-E, 1060–1065.
Cleland, R. E., and Grace, S. C. (1999). Voltammetric detection of superoxide production by photosystem II. FEBS Lett. 457 (3), 348–352. doi:10.1016/S0014-5793(99)01067-4
Corcoran, J., Winter, M. J., and Tyler, C. R. (2010). Pharmaceuticals in the aquatic environment: A critical review of the evidence for health effects in fish. Crit. Rev. Toxicol. 40 (4), 287–304. doi:10.3109/10408440903373590
Das, K., and Roychoudhury, A. (2014). Reactive oxygen species (ROS) and response of antioxidants as ROS-scavengers during environmental stress in plants. Front. Environ. Sci. 2 (DEC), 1–13. doi:10.3389/fenvs.2014.00053
de Carvalho, R. C., Feijao, E., Matos, A. R., Cabrita, M. T., Novais, S. C., Lemos, M. F. L., et al. (2020). Glyphosate-based herbicide toxicophenomics in marine diatoms: Impacts on primary production and physiological fitness. Appl. Sci. Switz. 10 (21), 7391. doi:10.3390/app10217391
De Coen, W. M., and Janssen, C. R. (1997). The use of biomarkers in Daphnia magna toxicity testing. IV. Cellular energy allocation: A new methodology to assess the energy budget of toxicant-stressed Daphnia populations. J. Aquatic Ecosyst. Stress Recovery 6 (1), 43–55. doi:10.1023/A:1008228517955
De Mendiburu, F., and Simon, R. (2015). Agricolae - ten years of an open source statistical tool for experiments in breeding, agriculture and biology. PeerJ Prepr. 3, e1404v1. doi:10.7287/peerj.preprints.1404v1
Dominguez, H., and Loret, E. P. (2019). Ulva lactuca, A source of troubles and potential riches. Mar. Drugs 17 (6), 1–20. doi:10.3390/md17060357
Du, Z.-Y., and Benning, C. (2016). “Triacylglycerol accumulation in photosynthetic cells in plants and algae,” in Lipids in plant and algae development. Editors Y. Nakamura, and Y. Li-Beisson (Cham: Springer International Publishing), 179–205. doi:10.1007/978-3-319-25979-6_8
Duarte, B., Carreiras, J., Feijao, E., de Carvalho, R. C., Matos, A. R., Fonseca, V. F., et al. (2021d). Potential of asparagopsis armata as a biopesticide for weed control under an invasive seaweed circular-economy Framework. Biology 10, 1321. doi:10.3390/biology10121321
Duarte, B., Carreiras, J., Feijao, E., Reis-Santos, P., Cacador, I., Matos, A. R., et al. (2021a). Fatty acid profiles of estuarine macroalgae are biomarkers of anthropogenic pressures: Development and application of a multivariate pressure index. Sci. Total Environ. 788, 147817. doi:10.1016/j.scitotenv.2021.147817
Duarte, B., Durante, L., Marques, J. C., Reis-Santos, P., Fonseca, V. F., and Cacador, I. (2021c). Development of a toxicophenomic index for trace element ecotoxicity tests using the halophyte Juncus acutus: Juncus-TOX. Ecol. Indic. 121, 107097. doi:10.1016/j.ecolind.2020.107097
Duarte, B., Feijao, E., Cruz de Carvalho, R., Duarte, I. A., Silva, M., Matos, A. R., et al. (2020). Effects of propranolol on growth, lipids and energy metabolism and oxidative stress response of phaeodactylum tricornutum. Biology 9 (12), 1–21. doi:10.3390/biology9120478
Duarte, B., Feijao, E., Cruz de Carvalho, R., Franzitta, M., Carlos Marques, J., Cacador, I., et al. (2021b). Unlocking Kautsky’s dark box: Development of an optical toxicity classification tool (OPTOX index) with marine diatoms exposed to emerging contaminants. Ecol. Indic. 131, 108238. doi:10.1016/j.ecolind.2021.108238
Duarte, B., Pedro, S., Marques, J. C., Adao, H., and Cacador, I. (2017). Zostera noltii development probing using chlorophyll a transient analysis (JIP-test) under field conditions: Integrating physiological insights into a photochemical stress index. Ecol. Indic. 76, 219–229. doi:10.1016/j.ecolind.2017.01.023
Duarte, I. A., Pais, M. P., Reis-Santos, P., Cabral, H. N., and Fonseca, V. F. (2019). Biomarker and behavioural responses of an estuarine fish following acute exposure to fluoxetine. Mar. Environ. Res. 147, 24–31. doi:10.1016/j.marenvres.2019.04.002
Ebele, A. J., Abou-Elwafa Abdallah, M., and Harrad, S. (2017). Pharmaceuticals and personal care products (PPCPs) in the freshwater aquatic environment. Emerg. Contam. 3 (1), 1–16. doi:10.1016/j.emcon.2016.12.004
Edwards, E. A., Rawsthorne, S., and Mullineaux, P. M. (1990). Subcellular distribution of multiple forms of glutathione reduetase in leaves of pea Pisum sativum L .. Germany: Planta, 278–284.
El-Bassat, R. A., Touliabah, H., Harisa, G., and Sayegh, F. (2011). Aquatic toxicity of various pharmaceuticals on some isolated plankton species. Int. J. Med. Med. Sci. 3 (6), 170–180. Available at: http://www.academicjournals.org/journal/IJMMS/article-abstract/D03211B147.
EUROSTAT (2017). Available at: 655 http://epp.eurostat.ec.europa.eu/tgm/table.do?tab=table&init= 656 1&plugin=1&language=en&pcode=ten00011.
Fatta-Kassinos, D., Meric, S., and Nikolaou, A. (2011). Pharmaceutical residues in environmental waters and wastewater: Current state of knowledge and future research. Anal. Bioanal. Chem. 399 (1), 251–275. doi:10.1007/s00216-010-4300-9
Feijão, E., Cruz de Carvalho, R., Duarte, I. A., Matos, A. R., Cabrita, M. T., Novais, S. C., et al. (2020). Fluoxetine arrests growth of the model diatom phaeodactylum tricornutum by increasing oxidative stress and altering energetic and lipid metabolism. Front. Microbiol. 11, 1803–1817. doi:10.3389/fmicb.2020.01803
Feijão, E., Gameiro, C., Franzitta, M., Duarte, B., Caçador, I., Teresa Cabrita, M., et al. (2017). Heat wave impacts on the model diatom Phaeodactylum tricornutum : Searching for photochemical and fatty acid biomarkers of thermal stress. Ecol. Indic. 95, 1026–1037. doi:10.1016/j.ecolind.2017.07.058
Fent, K., Weston, A. A., and Caminada, D. (2006). Ecotoxicology of human pharmaceuticals. Aquat. Toxicol. 76 (2), 122–159. doi:10.1016/j.aquatox.2005.09.009
Ferreira, D., Figueiredo, J., Laureano, G., Machado, A., Arrabaca, J. D., Duarte, B., et al. (2021). Membrane remodelling and triacylglycerol accumulation in drought stress resistance: The case study of soybean phospholipases A. Plant Physiology Biochem. 169, 9–21. doi:10.1016/j.plaphy.2021.10.033
Fong, P. P., and Ford, A. T. (2014). The biological effects of antidepressants on the molluscs and crustaceans: A review. Aquat. Toxicol. 151, 4–13. doi:10.1016/j.aquatox.2013.12.003
Franck, F., Juneau, P., and Popovic, R. (2002). Resolution of the Photosystem I and Photosystem II contributions to chlorophyll fluorescence of intact leaves at room temperature. Biochimica Biophysica Acta - Bioenergetics 1556 (2–3), 239–246. doi:10.1016/S0005-2728(02)00366-3
Gameiro, C., Utkin, A. B., and Cartaxana, P. (2015). Characterisation of estuarine intertidal macroalgae by laser-induced fluorescence. Estuar. Coast. Shelf Sci. 167, 119–124. doi:10.1016/j.ecss.2015.11.010
Gameiro, C., Utkin, A., Cartaxana, P., Silva, J. M. d., and Matos, A. (2016). The use of laser induced chlorophyll fluorescence (LIF) as a fast and non-destructive method to investigate water deficit in Arabidopsis. Agric. Water Manag. 164, 127–136. doi:10.1016/j.agwat.2015.09.008
Gavrilescu, M., Demnerova, K., Aamand, J., Agathos, S., and Fava, F. (2015). Emerging pollutants in the environment: Present and future challenges in biomonitoring, ecological risks and bioremediation. New Biotechnol. 32 (1), 147–156. doi:10.1016/j.nbt.2014.01.001
Gnaiger, E. (1983). Calculation of energetic and biochemical equivalents. Aquatic Physiological Appl., 337–345. Polarographic Oxygen Sensors. doi:10.1007/978-3-642-81863-9_30
Govindjee, G. (1995). Sixty-three years since Kautsky: Chlorophyll a fluorescence. Funct. Plant Biol. 22, 131–160. doi:10.1071/pp9950131
Grzesiuk, M., Spijkerman, E., Lachmann, S. C., and Wacker, A. (2018). Environmental concentrations of pharmaceuticals directly affect phytoplankton and effects propagate through trophic interactions. Ecotoxicol. Environ. Saf. 156, 271–278. doi:10.1016/j.ecoenv.2018.03.019
Grzesiuk, M., Wacker, A., and Spijkerman, E. (2016). Photosynthetic sensitivity of phytoplankton to commonly used pharmaceuticals and its dependence on cellular phosphorus status. Ecotoxicology 25 (4), 697–707. doi:10.1007/s10646-016-1628-8
Hák, R., Lichtenthaler, H. K., and Rinderle, U. (1990). Decrease of the chlorophyll fluorescence ratio F690/F730 during greening and development of leaves. Radiat. Environ. Biophys. 29 (4), 329–336. doi:10.1007/BF01210413
Han, Y.-S., Brown, M. T., Park, G. S., and Han, T. (2007). Evaluating aquatic toxicity by visual inspection of thallus color in the green macroalga ulva: Testing a novel bioassay. Environ. Sci. Technol. 41 (10), 3667–3671. doi:10.1021/es062158a
Heath, R. L., and Packer, L. (1968). Photoperoxidation in isolated chloroplasts: I. Kinetics and stoichiometry of fatty acid peroxidation. Archives Biochem. Biophysics 125 (1), 189–198. doi:10.1016/0003-9861(68)90654-1
Ivanov, B. N., Borisova-Mubarakshina, M. M., and Kozuleva, M. A. (2018). Formation mechanisms of superoxide radical and hydrogen peroxide in chloroplasts, and factors determining the signalling by hydrogen peroxide. Funct. Plant Biol. 45 (1–2), 102–110. doi:10.1071/FP16322
Johnson, D. J., Sanderson, H., Brain, R. A., Wilson, C. J., Bestari, K. J. T., and Solomon, K. R. (2005). Exposure assessment and microcosm fate of selected selective serotonin reuptake inhibitors. Regul. Toxicol. Pharmacol. 42 (3), 313–323. doi:10.1016/j.yrtph.2005.05.010
Johnson, D. J., Sanderson, H., Brain, R. A., Wilson, C. J., and Solomon, K. R. (2007). Toxicity and hazard of selective serotonin reuptake inhibitor antidepressants fluoxetine, fluvoxamine, and sertraline to algae. Ecotoxicol. Environ. Saf. 67 (1), 128–139. doi:10.1016/j.ecoenv.2006.03.016
Kendel, M., Wielgosz-Collin, G., Bertrand, S., Roussakis, C., Bourgougnon, N., and Bedoux, G. (2015). Lipid composition, fatty acids and sterols in the seaweeds ulva armoricana, and solieria chordalis from brittany (France): An analysis from nutritional, chemotaxonomic, and antiproliferative activity perspectives. Mar. Drugs 13 (9), 5606–5628. doi:10.3390/md13095606
Kern, J., and Guskov, A. (2011). Lipids in photosystem II: Multifunctional cofactors. J. Photochem. Photobiol. B Biol. 104 (1), 19–34. doi:10.1016/j.jphotobiol.2011.02.025
King, F. D., and Packard, T. T. (1975). Respiration and the activity of the respiratory electron transport system in marine zooplankton1. Limnol. Oceanogr. 20 (5), 849–854. doi:10.4319/lo.1975.20.5.0849
Klok, A. J., Lamers, P., Martens, D., Draaisma, R., and Wijffels, R. (2014). Edible oils from microalgae: Insights in TAG accumulation. Trends Biotechnol. 32 (10), 521–528. doi:10.1016/j.tibtech.2014.07.004
Kostetsky, E., Chopenko, N., Barkina, M., Velansky, P., and Sanina, N. (2018). Fatty acid composition and thermotropic behavior of glycolipids and other membrane lipids of ulva lactuca (Chlorophyta) inhabiting different climatic zones. Mar. Drugs 16 (12), 494. doi:10.3390/md16120494
Küpper, H., Seibert, S., and Parameswaran, A. (2007). Fast, sensitive, and inexpensive alternative to analytical pigment HPLC: Quantification of chlorophylls and carotenoids in crude extracts by fitting with Gauss peak spectra. Anal. Chem. 79 (20), 7611–7627. doi:10.1021/ac070236m
Kurwadkar, S., Struckhoff, G., Mishra, P., Modha, N., Murga, E., Amaral, B., et al. (2015). Emerging micro-pollutants in the environment: Occurrence. fate, distribution, 97–112. American Chemical Society. doi:10.1021/bk-2015-1198.ch005
Lancaster, S. G., and Gonzalez, J. P. (1989). Lofepramine: A review of its pharmacodynamic and pharmacokinetic properties, and therapeutic efficacy in depressive illness. Drugs 37 (2), 123–140. doi:10.2165/00003495-198937020-00003
Lavrov, A., Utkin, A. B., Marques da Silva, J., Vilar, R., Santos, N. M., and Alves, B. (2012). Water stress assessment of cork oak leaves and maritime pine needles based on LIF spectra. Opt. Spectrosc. 112 (2), 271–279. doi:10.1134/S0030400X12020166
Law, R., Hanke, G., Angelidis, M., Batty, J., Bignert, A., Dachs, J., et al. (2010). Marine Strategy Framework directive: Task group 8 report contaminants and pollution effects. Jt. Res. Centre. European Commission. doi:10.2788/85887
Lemos, M. F. L. (2021). Biomarker studies in stress biology: From the gene to population, from the organism to the application. Biology 10 (12), 1340. doi:10.3390/biology10121340
Leston, S., Nunes, M., Viegas, I., Lemos, M. F., Freitas, A., Barbosa, J., et al. (2011). The effects of the nitrofuran furaltadone on Ulva lactuca. Chemosphere 82 (7), 1010–1016. doi:10.1016/j.chemosphere.2010.10.067
Leston, S., Nunes, M., Viegas, I., Nebot, C., Cepeda, A., Pardal, M. A., et al. (2014). The influence of sulfathiazole on the macroalgae ulva lactuca. Chemosphere 100, 105–110. doi:10.1016/j.chemosphere.2013.12.038
Levaillant, M. (2021). Impact of COVID-19 pandemic and lockdowns on the consumption of anxiolytics , hypnotics and antidepressants according to age groups : A French nationwide study. Psychol. Med., 1–7. doi:10.1017/s0033291721004839
Marklund, S., and Marklund, G. (1974). Involvement of the superoxide anion radical in the autoxidation of pyrogallol and a convenient assay for superoxide dismutase. Eur. J. Biochem. 47 (3), 469–474. doi:10.1111/j.1432-1033.1974.tb03714.x
Mezzelani, M., Gorbi, S., and Regoli, F. (2018). Pharmaceuticals in the aquatic environments: Evidence of emerged threat and future challenges for marine organisms. Mar. Environ. Res. 140, 41–60. doi:10.1016/j.marenvres.2018.05.001
Minguez, L., Di Poi, C., Farcy, E., Ballandonne, C., Benchouala, A., Bojic, C., et al. (2014). Comparison of the sensitivity of seven marine and freshwater bioassays as regards antidepressant toxicity assessment. Ecotoxicology 23 (9), 1744–1754. doi:10.1007/s10646-014-1339-y
Mizusawa, N., and Wada, H. (2012). The role of lipids in photosystem II. Biochimica Biophysica Acta (BBA) - Bioenergetics 1817 (1), 194–208. doi:10.1016/j.bbabio.2011.04.008
Moreno-González, R., Rodriguez-Mozaz, S., Huerta, B., Barcelo, D., and Leon, V. (2016). Do pharmaceuticals bioaccumulate in marine molluscs and fish from a coastal lagoon? Environ. Res. 146, 282–298. doi:10.1016/j.envres.2016.01.001
Mullineaux, P. M., and Rausch, T. (2005). Glutathione, photosynthesis and the redox regulation of stress-responsive gene expression. Photosynth. Res. 86 (3), 459–474. doi:10.1007/s11120-005-8811-8
Ortiz, A. J. C. (2010) ‘Determinación de la presencia de naproxeno, fluoxetina, atorvastatina, enalapril y acetaminofeno en el lago Guayo y el lago Lucchetti en el sur de Puerto Rico’.
Pires, V. L., Novais, S. C., Lemos, M. F. L., Fonseca, V. F., and Duarte, B. (2021). Evaluation of multivariate biomarker indexes application in ecotoxicity tests with marine diatoms exposed to emerging contaminants. Appl. Sci. Switz. 11 (9), 3878. doi:10.3390/app11093878
Pospíšil, P. (2016). Production of reactive oxygen species by photosystem II as a response to light and temperature stress. Front. Plant Sci. 7 (DECEMBER2016), 1–12. doi:10.3389/fpls.2016.01950
Raj, R., Anandan, R., and Mathew, S. (2020). Chemical science review and letters nutritional and human healthcare aspects of ulva lactuca. Chem. Sci. Rev. Lett. 9 (34), 541–545. doi:10.37273/chesci.CS205101130
Reis-Santos, P., Pais, M., Duarte, B., Cacador, I., Freitas, A., Vila Pouca, A. S., et al. (2018). Screening of human and veterinary pharmaceuticals in estuarine waters: A baseline assessment for the tejo estuary. Mar. Pollut. Bull. 135, 1079–1084. doi:10.1016/j.marpolbul.2018.08.036
Richmond, E. K., Rosi, E. J., Reisinger, A. J., Hanrahan, B. R., Thompson, R. M., and Grace, M. R. (2019). Influences of the antidepressant fluoxetine on stream ecosystem function and aquatic insect emergence at environmentally realistic concentrations. J. Freshw. Ecol. 34 (1), 513–531. doi:10.1080/02705060.2019.1629546
Rosema, A., Snel, J., Zahn, H., Buurmeijer, W., and Van Hove, L. (1998). The relation between laser-induced chlorophyll fluorescence and photosynthesis. Remote Sens. Environ. 65 (2), 143–154. doi:10.1016/S0034-4257(98)00020-0
Sakurai, I., Mizusawa, N., Wada, H., and Sato, N. (2007). Digalactosyldiacylglycerol is required for stabilization of the oxygen-evolving complex in photosystem II. Plant Physiol. 145 (4), 1361–1370. doi:10.1104/pp.107.106781
Sand-Jensen, K. (1988). Minimum light requirement for growth in Ulva lactuca. Mar. Ecol. Prog. Ser. 50, 187–193. doi:10.3354/meps050187
Sawyer, E. K., and Howell, L. L. (2011). Pharmacokinetics of fluoxetine in rhesus macaques following multiple routes of administration. Pharmacology 88 (1–2), 44–49. doi:10.1159/000329417
Silva, M., Feijao, E., da Cruz de Carvalho, R., Duarte, I. A., Matos, A. R., Cabrita, M. T., et al. (2020). Comfortably numb: Ecotoxicity of the non-steroidal anti-inflammatory drug ibuprofen on Phaeodactylum tricornutum. Mar. Environ. Res. 161, 105109. doi:10.1016/j.marenvres.2020.105109
Takagi, D., Takumi, S., Hashiguchi, M., Sejima, T., and Miyake, C. (2016). Superoxide and singlet oxygen produced within the thylakoid membranes both cause photosystem I photoinhibition. Plant Physiol. 171 (3), 1626–1634. doi:10.1104/pp.16.00246
Tang, Y., Yin, M., Yang, W., Li, H., Zhong, H., Mo, L., et al. (2019). Emerging pollutants in water environment: Occurrence, monitoring, fate, and risk assessment. Water Environ. Res., 984–991. doi:10.1002/wer.1163
Telfer, A., et al. (2002). What is β-carotene doing in the photosystem II reaction centre? Phil. Trans. R. Soc. Lond. B 357 (1426), 1431–1440. doi:10.1098/rstb.2002.1139
Telfer, A. (2014). Singlet oxygen production by PSII under light stress: Mechanism, detection and the protective role of β-carotene. Plant Cell. Physiol. 55 (7), 1216–1223. doi:10.1093/pcp/pcu040
Teranishi, Y., Tanaka, A., Osumi, M., and Fukui, S. (1974). Catalase activities of hydrocarbon-utilizing candida yeasts. Agric. Biol. Chem. 38 (6), 1213–1220. doi:10.1080/00021369.1974.10861301
Tiryakioglu, M., Eker, S., Ozkutlu, F., Husted, S., and Cakmak, I. (2006). Antioxidant defense system and cadmium uptake in barley genotypes differing in cadmium tolerance. J. Trace Elem. Med. Biol. 20 (3), 181–189. doi:10.1016/j.jtemb.2005.12.004
Trinelli, M. A., Areco, M. M., and Dos Santos Afonso, M. (2013). Co-biosorption of copper and glyphosate by Ulva lactuca. Colloids Surfaces B Biointerfaces 105, 251–258. doi:10.1016/j.colsurfb.2012.12.047
Trinelli, M. A., Cantera, C. G., Areco, M. M., and Afonso, M. D. S. (2019). Glyphosate photodegradation: Stoichiometry, kinetic and catalytic effects. Int. J. Environ. Health 9 (4), 306–326. doi:10.1504/IJENVH.2019.108663
Uthayakumar, S., Kitchen, S. A., and Gomes, T. (2021). The effects of COVID ‐ 19 on the dispensing rates of antidepressants and benzodiazepines in Canada. Depress. Anxiety. 39 (2), 156–162. doi:10.1002/da.23228
Utkin, A. B., Vieira, S., Marques da Silva, J., Lavrov, A., Leite, E., and Cartaxana, P. (2013). Compact low-cost detector for in vivo assessment of microphytobenthos using laser induced fluorescence. Opt. Spectrosc. 114 (3), 471–477. doi:10.1134/S0030400X13030259
Vasskog, T., Anderssen, T., Pedersen-Bjergaard, S., Kallenborn, R., and Jensen, E. (2008). Occurrence of selective serotonin reuptake inhibitors in sewage and receiving waters at Spitsbergen and in Norway. J. Chromatogr. A 1185 (2), 194–205. doi:10.1016/j.chroma.2008.01.063
Verslycke, T., Ghekiere, A., and Janssen, C. R. (2004). Seasonal and spatial patterns in cellular energy allocation in the estuarine mysid Neomysis integer (Crustacea: Mysidacea) of the Scheldt estuary (The Netherlands). J. Exp. Mar. Biol. Ecol. 306 (2), 245–267. doi:10.1016/j.jembe.2004.01.014
Wiklund, A. K. E., Oskarsson, H., Thorsen, G., and Krumblad, L. (2011). Behavioural and physiological responses to pharmaceutical exposure in macroalgae and grazers from a Baltic Sea littoral community. Aquat. Biol. 14 (1), 29–39. doi:10.3354/ab00380
Yang, Z., Lu, T., Zhu, Y., Zhang, Q., Zhou, Z., Pan, X., et al. (2019). Aquatic ecotoxicity of an antidepressant, sertraline hydrochloride, on microbial communities. Sci. Total Environ. 654, 129–134. doi:10.1016/j.scitotenv.2018.11.164
Zhu, X. G., Govindjee, ., Baker, N. R., deSturler, E., Ort, D. R., and Long, S. P. (2005). Chlorophyll a fluorescence induction kinetics in leaves predicted from a model describing each discrete step of excitation energy and electron transfer associated with Photosystem II. Planta 223 (1), 114–133. doi:10.1007/s00425-005-0064-4
Keywords: antidepressants, macroalgae, oxidative stress, pharmaceuticals, photobiology
Citation: Feijão E, Cruz de Carvalho R, Duarte IA, Matos AR, Cabrita MT, Utkin AB, Caçador I, Marques JC, Novais SC, Lemos MFL, Reis-Santos P, Fonseca VF and Duarte B (2022) Fluoxetine induces photochemistry-derived oxidative stress on Ulva lactuca. Front. Environ. Sci. 10:963537. doi: 10.3389/fenvs.2022.963537
Received: 07 June 2022; Accepted: 03 August 2022;
Published: 24 August 2022.
Edited by:
Pedro Neves Carvalho, Aarhus University, DenmarkReviewed by:
M Oves, King Abdulaziz University, Saudi ArabiaAnuradha Patel, Allahabad University, India
Copyright © 2022 Feijão, Cruz de Carvalho, Duarte, Matos, Cabrita, Utkin, Caçador, Marques, Novais, Lemos, Reis-Santos, Fonseca and Duarte. This is an open-access article distributed under the terms of the Creative Commons Attribution License (CC BY). The use, distribution or reproduction in other forums is permitted, provided the original author(s) and the copyright owner(s) are credited and that the original publication in this journal is cited, in accordance with accepted academic practice. No use, distribution or reproduction is permitted which does not comply with these terms.
*Correspondence: Eduardo Feijão, emfeijao@fc.ul.pt