A comparing vision of the lakes of the basin of Mexico: from the first physicochemical evaluation of Alexander von Humboldt to the current condition
- 1Instituto Politécnico Nacional, Escuela Nacional de Ciencias Biológicas, Prol. de Carpio y Plan de Ayala, CDMX, Ciudad de México, México
- 2University of Siegen, Geography, School of Science and Technology, Siegen, Germany
- 3Instituto Politécnico Nacional, Coordinación Politécnica para la Sustentabilidad, Av. IPN S/n Esq, Wilfrido Massieu, CDMX, Ciudad de México, México
- 4Department of Chemistry and Biology, School of Science and Technology, University of Siegen, Siegen, Germany
The Basin of Mexico is an endorheic lacustrine basin with an outstanding ecological and social history. There is evidence that it hosted human settlers since the late Pleistocene. This basin was home to great antique civilizations and many endemic species of flora and fauna. The main lake in the Basin was the Great Lake of Mexico, which was divided into five lakes and provided goods and services to the native communities. After the Spanish conquest, a rule was established to drain the lakes to prevent flooding in the city. The naturalist Alexander von Humboldt visited Mexico City in the early 1800s, and carried out the first formal scientific water quality analysis of the lakes of the basin. The Basin of Mexico gone through serious modifications due to urbanization and changes of land use reducing the lacustrine area to the virtual extinction of the lakes. The lakes are currently reduced to wetlands accounting for only 2.83% of the former lake and receiving mainly treated wastewater discharges. We carried out a comparative study between Humboldt’s results and the current characteristics of water from these lake remnants analyzed with the same methods that he used. In addition, we assessed several morphometric parameters and performed water quality assessments using modern methods. Changes in water quality characteristics and ionic composition were detected, with Xochimilco being the lake with the highest water quality score and Texcoco and Chalco showing major alterations. The drastic reduction in the area of the remaining water bodies and the modifications in their water quality are discussed.
1 Introduction
The Basin of Mexico (also known as the Anahuac Basin), located south of the Mexican Plateau and at the center of the Trans-Mexican Volcanic Belt, is bordered by mountain systems that circumscribe it as an endorheic basin. This basin dates from the late Tertiary (Álvarez and Navarro, 1957) and previously harbored a large lake named “The Great Lake of Mexico”. However, during the dry season, this large lake was separated into five lakes which, during pre-Hispanic times, were named Lake Zumpango, Lake Xaltocán, Lake Texcoco, Lake Xochimilco, and Lake Chalco (Legorreta, 2006). Thus, lakes were connected during periods of heavy rainfall and formed a single large water body, while in drought periods, the lakes became separate water bodies.
A sedimentary succession analysis with stable isotopes, diatoms, organic geochemistry, and tephrochronology have been allowed to identify conspicuous changes in the former Lake Texcoco shoreline between the late Pleistocene and the late Holocene. Additional switches included the exchange between aquatic and terrestrial plants (C3 and C4 plants), shifts from saline to alkaline and freshwater conditions, the influence of volcanic activity, marginal reworking of lake sediments, and the inflow of water drained from the basin (Lamb et al., 2009). Evidence has been found that humans settled in this basin since the late Pleistocene. Findings of the first humans in the Basin of Mexico are recorded near Chalco and El Peñón (Texcoco Lake springs), and Tepexpam. The presence of these skeletal remains close to the former lake suggests that this water body offered appealing resources for the development and survival of the first human settlers of the basin (González et al., 2003). Given the presence of humans since those times, is possible that they may have exerted environmental pressure by using the resources available in the basin and the water bodies. Other studies have shown that changes have occurred from saline to alkaline and freshwater conditions (Lamb et al., 2009), and other strong impacts due to volcanic activity and climatic changes, such as the last Glacial period.
The basin was home to several Mesoamerican civilizations. Among them, the most relevant was the Aztec empire, also named Mexicas, who settled on an island at the center of Lake Texcoco. The Aztec empire was at its peak when the Spaniards arrived. Other important civilizations settled in the Basin of Mexico were the Teotihuacans, Colhuas, Xochimilcas (in the littoral of Lake Xochimilco), Chalcas (in the littoral of Lake Chalco), and Xaltocanmecas (in the littoral of Lake Xaltocan), among others (Candiani, 2014; Torres-Alves and Morales-Nápoles, 2020). The lakes provided several ecosystem services to the local inhabitants, including water supply, food, and a means of transportation for the people and goods (Berres, 2000; Biar, 2020). The Aztecs modified the lacustrine system by building infrastructure such as dikes. In addition, they invented the farming system so-called chinampas—an interesting crop system composed by small artificial islands built in strips with sediments from the lake bottom, branches, and decaying vegetation, creating a network of channels serving as the irrigation system, with an average depth of 1.5 m.
The lakes functioned as means of communication: Lake Chalco was fed by freshwater draining from the mountains at the south and from springs. Lake Xochimilco was fed by the large number of springs in the area and by Lake Chalco; Lake Zumpango received water from the Cuautitlán River and fed Lake Xaltocan. Finally, Lake Texcoco, being at the bottom of the basin, received water from all the lakes and rivers (Torres-Alves and Morales-Nápoles, 2020).
Despite the dikes built by pre-Hispanic peoples, the basin maintained its lacustrine areas with changes in level associated with the rainy and dry seasons, suggesting that the original populations sustainably exploited the local resources (León Portilla, 1988). During the Spanish colonial period, the population increased and the urban area located within the lacustrine influence area expanded; consequently, the city was subjected to continuous floods that jeopardized its growth. In the 17th century, a work known as the Tajo de Nochistongo (Gurrfa, 1978) was built to divert the water of the Cuautitlán River, which fed Lake Texcoco. This work, together with the Tequixquiac tunnel, the Grand Canal and the so-called “Deep Drainage”, was undertaken to drain off the lake system that had remained until then. These artificial drainages and urban growth, have caused drastic modifications in the basin and its lake systems (Alcántara and Escalante, 2005). As a result, neither the former lacustrine system nor the Nezahualcoyotl dike remains nowadays (Torres-Alves and Morales Nápoles, 2020; Montero-Rosado et al., 2022).
In the early 1800s, many European naturalists conducted scientific journeys to land still unknown at the time. The purpose of those journeys was not only the discovery of unexplored territories, but also investigation, including the collection of samples, the use of advanced scientific instruments, and the proposal of new taxonomic classification systems (Heck, 2020). The primary aim of Alexander von Humboldt (Berlin, Germany, 1769 – 1859) in his trip to the Americas (1799–1804) was data collection for the development of a science that had not yet been outlined and that was then called “the physics of the world”. This was later called “theory of the Earth” and then Physical Geography (Heck, 2020). In Volume Eight of “The American Travel Journal”, under the heading “Chemical Analysis of the Lakes in the Valley of Mexico”, Humboldt detailed the analyses he carried out in early 1804 (in the dry season), on water samples from Lake Tescuco, Lake Zumpango, Lake Xaltocan, Lake San Cristóbal (the last one, was a water body separated from Lake Xaltocan, possibly by a dike or by the process of drying up of the former lake Texcoco), Lake Chalco, and Lake Xochimilco (Humboldt, 1802–1804), thus providing the first formal scientific analysis of lakes in the Basin of Mexico.
When Alexander von Humboldt visited Mexico City, the local population was only 160000 inhabitants (Humboldt, 2003). He noticed trends of increasing aridity and decreasing soil fertility from south to north (Humboldt, 2003). To date, the basin is home to one of the most densely populated cities in the world, considered a “Megacity” (UNESCO, 2018) with a population size above 20 million inhabitants (INEGI, 2023). According to Tortajada (2008), this huge urban area makes it extremely hard to provide services to the entire population, and generates large-scale environmental issues in the atmosphere and water bodies. This megacity lacks proper management supporting sustainable resource use, which has led to the depletion of some of the water bodies in the Basin of Mexico. Over several centuries, different environmental and social factors have led to the almost total disappearance of the lake system of the Basin of Mexico.
This contribution aims to provide a comparative perspective not only in relation to Humboldt’s analysis of the early 19th century, but also considering a more detailed recent study on water quality. The following particular objectives are derived from this work, a) Compare the results of the characteristics of the lakes of the basin of Mexico with the tests carried out by Humboldt with the results of recent water samples analyzed with the same methods used by Humboldt, b) analyze the significant changes in surface area of the still existing waterbodies concerning the surface area of the whole basin and based on Humboldt´s map, c) characterize water quality and assess a water quality index of the lakes using modern test methods in the dry and rainy seasons to obtain a current diagnosis. The implications regarding the state of conservation and the perspectives this work entails for management purposes are discussed. The implications regarding the state of conservation and the perspectives that this work entails for management purposes are discussed.
2 Methods
2.1 Study area
The endorheic Basin of Mexico (BM), also called the Valley of Mexico Basin (19° 29′52″N, 99° 7′37″W), is located in the central part of the Trans-Mexican Volcanic Belt Province belonging to the Mexican Transition Zone (Morrone et al., 2017; Morrone et al., 2022), with a mean altitude of 2,276 m above sea level. According to Arce et al. (2019) the area of the basin is of 9,620 km2. The basin is delimited by the volcanic ranges of Sierra de las Cruces to the west, Sierra Nevada to the east, Sierra Chichinautzin to the south, and the Apan-Tezontepec volcanic range to the north (Arce et al., 2019; Martínez-Abarca et al., 2021) (Figure 1).
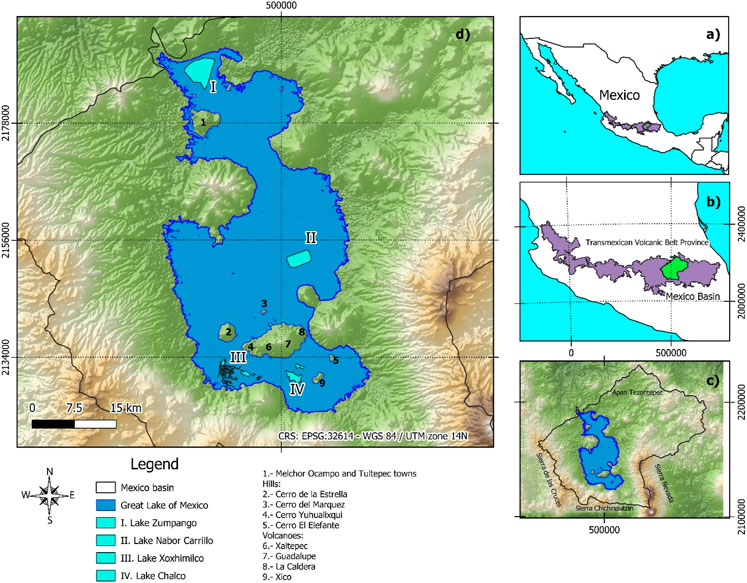
FIGURE 1. Study Area. (A) Reference map of the study area in Mexico. (B) Location of the BM into the Transmexican Volcanic Belt Province. (C) Basin of Mexico. (D) Surface area and shape of the former Great Lake of Mexico based on remote sensing and the location of the four remnant lakes studied.
The predominant soil types in the area of the former Great Lake of Mexico are Phaeozem, Vertisol, and Solonchac; the latter is characterized by a high content of soluble salts (Sedeño-Díaz et al., 2019; CONABIO, 2023) (Supplementary Material; Figure 1A).
Today, the predominant climate in the Basin of Mexico (BM hereafter) is temperate [C (w0), C (w1), and C (w2)] according to Köppen’s classification (García, 2004). Temperature ranges from 13°C to 25°C, with a mean annual temperature of 16°C; the mean annual precipitation varies between 700 mm and 900 mm, with the rainy season in the summer and up to 5% of rainfall in the winter (Alcocer and Williams, 1996; López-López et al., 2016) (Supplementary Material, Figure 1B).
During the Upper Cretaceous and Lower Tertiary, the BM was an open basin, with a surface drainage to the south through two main rivers, which produced large deposits of alluvial material at the bottom of the basin. Subsequently, during the Pleistocene, the basin closed off (Palma et al., 2022). In this sense, the lithological layers in the BM are dominated by alluvial deposits (with sedimentary rocks) and andesite-basalt (Supplementary Material, Figure 2).
The former Great Lake of Mexico was located within the BM (Figure 1) and was split into five lakes during the dry season, namely,: Texcoco, Zumpango, Xaltocan, Chalco, and Xochimilco (Berres, 2000; Montero-Rosado et al., 2022). Nowadays, only four lakes remain: Chalco, Texcoco (Nabor Carrillo reservoir, built in 1983, covering a small area located where the great Texcoco Lake once was), Xochimilco, and Zumpango, all with major modifications (Figure 1). Texcoco and Xochimilco are currently protected natural areas considered wetlands and designated as Ramsar sites (Numbers 2469 and 1,363, respectively); however, both lakes receive the effluents of different wastewater treatment facilities (López-López et al., 2016; Morales-García et al., 2020). For its part, Lake Zumpango, with a storage capacity of 100 million m3, functions like a regulating vessel. During approximately 2 months in the rainy season, it receives part of the water from the Cuautitlán River and the Emisor Poniente through the Santo Tomás canal. The water thus stored is used for agriculture, and the rest is diverted to the “Gran Canal del Desagüe”, which drains into the Pánuco basin. Lake Chalco is mainly filled with rainwater; however, municipal and industrial wastewater treated and untreated is also discharged into it (Ortiz-Zamora and Ortega-Guerrero, 2007). The original connectivity between lakes was lost due to urban settlements in the former lacustrine area.
When Alexander von Humboldt visited Mexico, in addition to analyzing the water of the BM lakes, he drafted a map of the BM, including the lakes, which had already lost a considerable area by that time (the early 1800s). In the present work, we studied the same lakes Humboldt analyzed, except for Lakes Xaltocan and San Cristobal since both became extinct (Figure 2).
2.2 Spatial analysis
We conducted a morphometric analysis of the BM to determine its perimeter, maximum and mean areas, and the compactness coefficient (defined as the ratio between the basin perimeter and the circumference of a circle with an area equal to the basin area, as proposed by Gravelius, 1914). The model designed in this work from the surface of the Great Lake of Mexico was developed using the Digital Elevation Model (DEM) by Hydrosheds.org (2023), with a 3s resolution, and public shapefiles from Mexico’s Institute of Statistics, Geography and Computer Sciences (Instituto Nacional de Estadística, Geografía e Informática; INEGI, 2023). The map entitled Carte de la Vallée de Mexico, drafted by Humboldt and published in 1811, was obtained from the website at David Rumsey Map Collection (2023). This map was georeferenced by the nearest neighbor method, using the landmarks marked in Humboldt’s map to elaborate a new map superimposed on the DEM of Mexico Basin mentioned above. All spatial analyses were performed using the QGIS geographic information system (open-source system).
2.3 Fieldwork
The water bodies studied were monitored in two contrasting seasons: dry (March 2022) and rainy (October 2022). In each sampling period, the following environmental variables were recorded with the use of a Quanta® multiparameter probe (Hydrolab DS5): water and air temperature (°C), dissolved oxygen (DO mg L-1), pH, salinity (UPS), turbidity (NTU), and conductivity (µS cm-1). Additionally, 500 mL water samples were collected in polyethylene bottles, in duplicate. Samples were transferred in the dark and refrigerated (4 °C) for subsequent testing in the laboratory. The following water quality parameters were determined with HACHDR 3900, Hach® spectrophotometer techniques: nitrites (NO2, mg L-1), nitrates (NO3, mg L-1), ammonium (NH4, mg L-1), total nitrogen (TN, mg L-1), orthophosphates (PO4, mg L-1), total phosphorus (mg L-1), alkalinity (mg L-1), biochemical oxygen demand (BOD5, mg L-1), color (U-PtCo), and total suspended solids (TSS mg L-1). Total hardness was quantified by titration with EDTA (CaCO3, mg L-1). Alkalinity (CaCO3, mg L-1) was quantified by titration according to APHA (2005) techniques. In addition, at each study site, 100 mL of water was collected in Whirl-Pak bags for microbiological analyses. These water samples were tested for total and fecal coliforms according to the APHA MPN technique (2005).
Separately, chlorophyll a (Chl a) was quantified as per the standardized APHA technique (APHA, 2005). To this end, 500 mL of water collected from each study site was filtered through Whatman filters (0.45 µm), followed by extraction in acetone (90%) for 24 h in the dark under refrigeration (4 °C) prior to the Chl a analysis.
Another 50 mL water sample was acidified and digested to determine Cl−, SO42-, Na+, K+, Ca2+, and Mg2+ using Inductively Coupled Plasma Optical Emission Spectrometry (ICP OES) techniques (Perkin Elmer Optima 8,000) at the CICATA Legaria laboratory of the Instituto Politécnico Nacional.
2.4 Water quality index
To obtain a single parameter indicating the water quality characteristics of the current lakes, we calculated the water quality index (WQI) proposed by Dinius (1987) for each study lake and period. This index includes 13 environmental variables: dissolved oxygen saturation (%), atmospheric and water temperature, pH, biochemical oxygen demand, alkalinity, nitrates, conductivity, chlorides, hardness, true color, and fecal and total coliforms. The output of this WQI ranges from 0 to 100, which is suitable for a better understanding of water quality and decision-making on water use, and as support of management programs. Mean WQI values were assessed using two different data sets: individual lakes (WQI values for all study periods), and study periods (WQI values for all study lakes for each study period).
2.5 Statistical analysis
All data were assessed for normality and homoscedasticity. Then, ANOVA followed by Tukey’s multiple comparison test or Kruskal–Wallis followed by Duncan’s multiple range test were performed for parametric or non-parametric data, respectively, to test the data for significant differences between lakes and between study periods. Box-plot graphs were constructed to depict the physicochemical and biological variables evaluated, by study lake and study period, using the XLSTAT software (Addinsoft, 2023). Heatmaps were constructed with the mean values and the standard error of the main physicochemical characteristics evaluated. A principal component analysis (PCA) was performed, after a factorial analysis of all the physicochemical factors evaluated, to identify the variables with the greatest contribution to the trends between study sites. The data included the four lakes studied, the factors recorded in the field, and the results of the physicochemical testing carried out in the laboratory; all the variables were transformed to log(x+1). The PCA was performed with Pearson’s correlation using the XLSTAT software (Addinsoft, 2023).
In the case of Na+, Ca2+, K+, Mg2+, Cl−, and HCO3−, a Piper diagram was constructed using the free software Easy Quim V.5 (Vazquez-Suñe and Serrano-Juan, 2012) to classify the lakes according to their ionic composition.
As for the information generated by Alexander von Humboldt, we reviewed his diary (Humboldt, 1802–1804). From it, we obtained his records about the test results of the water samples collected from the center of the lakes (Zumpango, San Cristobal, Texcoco, Xochimilco, and Chalco). These tests and observations included color, odor, taste, density, and the reactions from testing water samples with turmeric Curcuma paper (alkalinity) and the reactants lead acetate (hydrogen sulfide), silver nitrate (chloride), lead nitrate (sulfate), lime water (carbonates), and nitric acid (bicarbonates) (Richter and Engshuber, 2014).
With Humboldt’s results, a table was elaborated to summarize all the test results. Given that the results obtained by Humboldt are semi-quantitative, they were coded to provide quantitative data for a PCA. The coding involved using a 0-to-5 scale, where 0 means no reaction to the test and 5 indicates the maximum reaction reported by Humboldt. Variables such as colour and taste, were considered as categorical. The PCA aims to identify the similarities and differences between lakes, as well as any environmental gradients in the water bodies based on the lake parameters recorded by Alexander von Humboldt. The PCA was performed with Pearson coefficient and using the XLSTAT software (Addinsoft, 2023). The same procedure used for the PCA of our data was used for Humboldt´s data.
3 Results and discussion
3.1 The basin of Mexico
The analysis of basin morphometry indicates that the BM comprises an area of 9,219.3 km2, with an approximate total length of 142.5 km in its main axis, oriented in a southwestern–northeastern direction, a maximum width of 112.7 km, a perimeter of 529.8 km, and a compactness coefficient score of 1.55. According to Faye and Ndiaye (2021), the compactness coefficient (IK) can be used to classify lakes into four different shapes: circular (1), squat (1–1.15), intermediate (1.15–1.5), and elongated (1.5 and above); therefore, the BM has an elongated shape, with its main axis oriented as mentioned above (Table 1). Some of these values differ from those obtained by Arce et al. (2019), namely, 9,620 km2 in area, 100 km in length, and 80 km in width; separately, Alcocer and Williams (1996), based on the work of Alvarez and Navarro (1957), reported a total length of 125 km, 90 km in width, and a mean area of 9,600 km2, which are similar to the figures reported by Arce et al. (2019).

TABLE 1. Results of the main morphometric parameters of the Basin of Mexico obtained with GIS and the digital model elevation.
3.2 Reduction in Lake surface area
In this study, using GIS tools, the Digital Elevation Model (DEM) from Hydrosheds.org (2023), and considering 2,250 masl as the high watermark of the lakes as proposed by Torres-Alves and Morales-Nápoles (2020), we derived a new shape for the Great Lake of Mexico, estimating an area of 1,280 km2 and a perimeter of 644.2 km (Figure 1). Based on this new shape, it is possible to observe that the hills named La Estrella, Yuhualixqui, El Marquez, and El Elefante, and the volcanoes Xico, Guadalupe, La Caldera, and Xaltepec were previously islands located within the Great Lake of Mexico, as well as the area that currently harbors the Tultepec and Melchor Ocampo towns, located in the northern area of the former lake, since this area exceeds 2,250 m asl. This result is consistent with the alluvial deposits in the BM (Supplementary Material, Figure 2). Several authors have proposed models that estimate the surface area of the Great Lake of Mexico. Cruickshank García (1998) estimated that the lake region measured almost 2000 km2; Torres-Alves and Morales-Nápoles (2020) indicated an area of 1,000 km2; and Alcocer and Williams (1996) calculated an area of 7,868 km2.
Therefore, considering the original lacustrine area of 1,280 km2 as the original (baseline) area (100%), the remaining lacustrine area in Humboldt’s time (1803) was 428.26 km2, accounting for 33.46% of the original area. This implies a loss of two-thirds of the original lake area by the early nineteenth century. Today, the remaining lacustrine area is a mere 2.83% of the original area of the Great Lake of Mexico (Table 2). Given its current role as a regulating vessel, Lake Zumpango has increased in area by 7.66% compared to the area shown in Humboldt’s map (Figure 2). The lake with the greatest loss of area from Humboldt’s time to date is Lake Texcoco (95.57%). For its part, Lake Xochimilco represents 11.32% of the area of all lakes in Humboldt’s time; this scenario may be due to the increasing number of chinampas being built in this area. Tussupova et al. (2020) state that many saline lakes around the world are drying up rapidly due to anthropogenic activities, causing local adverse effects on health (lung diseases) air quality (excess dust), and ecological impacts (e.g., biodiversity), among others. In the case of the BM lakes, the decision to drain the lakes and urban growth since the Spanish colonial period has brought about major consequences.
These results highlight the extreme reduction of the lake area in the BM, which has brought with it a shift in the cultural perception of the BM landscape from a lakescape in the pre-Hispanic era to an urban landscape today (Biar, 2020).
3.3 Revisiting Humboldt’s results
The values of the water variables tested in the five lakes in the early 1800s and obtained from Humboldt´s diary (Humboldt, 1802–1804) are summarized in Table 3.
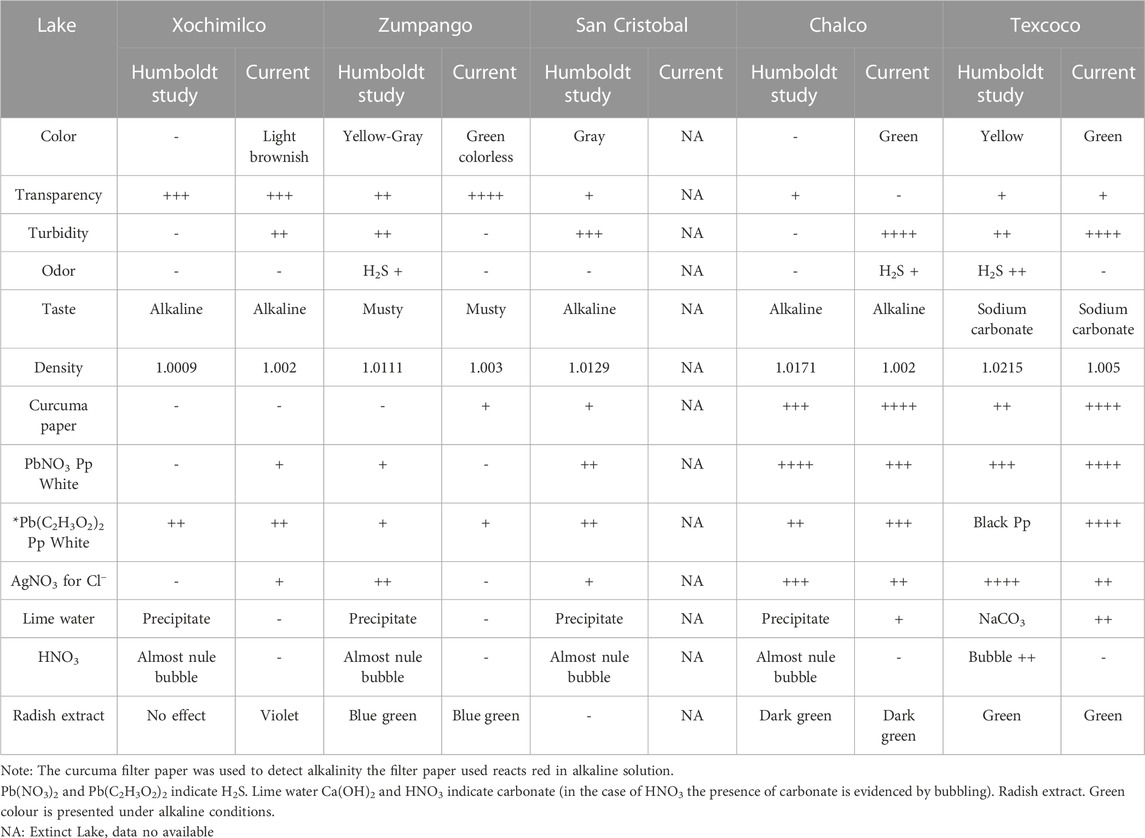
TABLE 3. Summary of the variables assessed by Humboldt in the lakes of the Basin of México during his visit to the Basin of Mexico (1804) and the recent samples in 2022.
Based on Table 3, Lakes Texcoco and Chalco were denser and more saline than the rest of the BM lakes; however, Lake Chalco had colorless clear water (i.e., no turbidity). On the other hand, Lake Xochimilco water was odorless and showed the lowest density. Currently extinct, Lake San Cristobal had turbid water with a density intermediate between Lakes Texcoco and Xochimilco. Contrary to some reports (Berres, 2000; Torres-Alves and Morales-Nápoles, 2020), Lake Chalco was relatively saline, not entirely freshwater. Humboldt recorded this water characteristic in the early 1800s, and Caballero and Ortega-Guerrero (1998) mentioned that Lake Chalco had been saline in some periods of the recent past.
The principal component analysis of the lakes based on the variables evaluated by Humboldt shows an explained variance of 83.23% in its first two components (Figures 3A, B). In the convex hulls diagram, Lakes Texcoco, Chalco, and Xochimilco are separate units, while Lakes Zumpango and San Cristobal are grouped in the same cluster (Figure 3A). The dispersion of the lakes along the environmental gradients formed by the variables tested by Humboldt is notorious. On the far right (Figure 3B), we can see the vectors of the variables that are associated, including density, and several tests such as the turmeric Curcuma paper, Pb(NO3)2, AgNO3, lime water, Pb(Ac)2, HNO3, sodium carbonate taste, hydrogen sulfide odor, and yellowish color, all of which attain the highest values at the far right of the diagram; Lake Texcoco Lake is located at this end of the gradient. In contrast, on the far left of the diagram, the vectors corresponding to transparency, alkaline taste, colorless, greyish color, and musty taste are observed. Lake Xochimilco is located on the far left of the diagram, characterized by its non-turbid, colorless water with an alkaline taste, characteristics that contrast with those of Lake Texcoco. The environmental conditions in Lakes Xochimilco and Texcoco also form a gradient ranging from less dense and less saline water to denser and more saline conditions. Along this gradient, Lakes Zumpango and San Cristobal are positioned on the lower left quadrant, characterized by grayish water with greater turbidity and a musty taste; regarding the tests with reactants, these lakes were less reactive than Texcoco but more than Xochimilco. Finally, Lake Chalco, positioned in the upper right quadrant, was characterized by reacting to turmeric curcuma paper and silver nitrate, and by a higher density than that of Lakes Xochimilco, Zumpango, and San Cristobal, but lower than that of Lake Texcoco. Lake Chalco was positioned opposite to Lakes Zumpango and San Cristobal since its water was more transparent, colorless and with an alkaline taste, and reacted to silver nitrate and turmeric paper (Figure 3B).
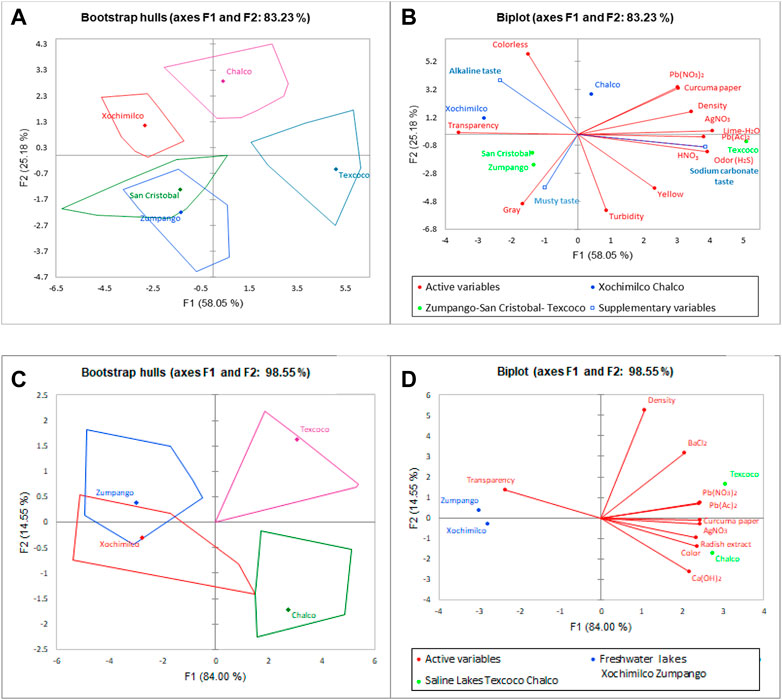
FIGURE 3. Biplot of the lakes of the BM according to the variables studied by Humboldt. (A) Convex hulls of each lake studied in the early 1800s; (B) arrangement of the lakes along environmental gradients, and vectors of the tests carried out by Humboldt; (C) convex hulls of each lake studied in 2022; (D) arrangement of the lakes along environmental gradients, and vectors of the tests carried out in the present study using the same methods as Humboldt.
According to Humboldt’s results, the high Cl−, HCO3−, and CO32- contents are worth noting; together with alkalinity, these results reveal the alkaline soda-lake nature of Lake Texcoco. According to Jones et al. (1998), soda lakes are characterized by the predominance of NaCl, NaHCO3, and Na2CO3, which are consistent with the findings of Humboldt from his qualitative chemical tests. In contrast, Lake Xochimilco turned out to be a freshwater lake with conditions opposite to those of Lake Texcoco, Lake Chalco stood out for its high alkalinity, with carbonate-rich waters, and ranked second in terms of its high density. Lakes Zumpango and San Cristobal showed intermediate characteristics between Lake Texococo (soda lake) and Lake Xochimilco (freshwater lake). Based on the above, the BM lakes showed an environmental gradient ranging from freshwater to saline soda lakes, with a strongly alkaline lake that does not reach a soda-lake state (Lake Chalco). Likewise, the spatial view of the BM is consistent with Humboldt’s perception in the early 1800s that everything was interconnected (Holl, 2018). Humboldt claimed that the lack of water might turn the valley sterile and unhealthy, increasing the salinity and aridity. He observed that the aquatic plants covering the lakes released hydrogen sulfide, which could be perceived when the wind blew across Lake Texcoco (Humboldt, 2003, 256).
3.4 Physicochemical characteristics of the lacustrine remnants of Lake Texcoco
3.4.1 Testing with Humboldt’s methods
The results of our tests of water samples collected from the study lakes using Humboldt’s methods are shown in Table 3. The PCA of these data shows that the first two principal components accounted for 98.55% of the explained variance. The lakes in the bootstrap hulls diagram showed the distribution of the lakes along the gradient of the environmental conditions assessed (Figure 3C). Lakes Zumpango and Xochimilco were grouped in the same cluster positioned on the left side of the biplot, while Lakes Chalco and Texcoco were on the right side of the biplot, although as separate lakes, each with its own particular conditions. The vectors of the tests showed that Lakes Texcoco and Chalco have colorful water of the highest density that reacted with Pb(NO3)2, Pb(Ac)2, AgNO3, curcuma paper, and radish extract. Both lakes reacted with Ca(OH)2, but the reaction was more intense for water from Lake Chalco; furthermore, water from both lakes also reacted with BaCl2, but the reaction was stronger in water from Lake Texcoco (Figure 3D). These results suggest remarkable changes in the chemical composition of the lakes. In contrast to Humboldt’s findings, Lake Chalco is currently more saline, sharing some environmental characteristics with Lake Texcoco, such as high alkalinity, color (green color due to algal blooms), chloride, HS2, sulfates, and, particularly, carbonates.
Another contrast versus Humboldt’s data is that Lakes Xochimilco and Zumpango are currently very similar in chemical composition, both being less saline, more alkaline, and colorless; in general, the reactions tested in water from both lakes were null or less intense than those in water from Lakes Chalco and Texcoco.
3.4.2 Ionic composition
The Piper diagram showed that almost all lakes, except for Lake Xochimilco, were plotted in the [Na+] type field of the lower-left triangle (Figure 4), while Xochimilco lies in the boundary with the [Mg+] and [Na+] type fields, suggesting that the lakes of the Basin of México are dominated by the cation Na+. These conditions are common in areas with arid climates, as stated by Shengbin, et al. (2022) for the water bodies of the Tibetan Plateau. In the case of the major anions, Lakes Xochimilco, Texcoco, and Zumpango are plotted in the [Cl−] type field of the lower-right triangle (Figure 4), and Lake Chalco was plotted in the [HCO3−] type field. This diagram suggests that the Basin of México lakes evolved from the fresh hydrochemical facie of the [HCO3−] type to the saline Cl− type. Furthermore, the central diamond shape of the Piper diagram (Figure 4) also shows that the hydrochemical facies of the Basin of Mexico lakes evolved from the fresh HCO3–Ca type to the saline Cl–Na type. The Basin of México lakes are currently dominated by the saline Cl–Na type, with Lake Chalco being very close to the HCO3–Ca type, and Lake Xochimilco being of a mixed Cl–Mg–Ca type.
3.4.3 Physicochemical characterization
According to the physicochemical results of the water bodies that still persist in the Basin of Mexico, the lakes showed remarkable differences in salinity. Lakes Chalco and Texcoco reached the highest values (1.82 ± 0.77 UPS and 1.72 ± 0.19 UPS, respectively), while Lakes Xochimilco and Zumpango had the lowest (0.51 ± 0.11 UPS and 0.27 ± 0.01 UPS, respectively) (Figure 5A). Accordingly, Lakes Texcoco and Chalco are referred to as saline lakes hereafter. At the same time, Xochimilco and Zumpango are considered freshwater lakes. The color, turbidity, and total suspended solids, total nitrogen, nitrates, alkalinity, sulfates, and Chl a showed the same behavior, where the highest mean values were observed in the following ranking order: Chalco > Texcoco > Xochimilco > Zumpango (Figures 5B–I; Table 4). The pH showed its highest values in Lake Texcoco, followed by Chalco (9.3 ± 0.03 and 9.1 ± 1.08, respectively), and with the lowest values in Lakes Xochimilco and Zumpango (8.19 ± 0.32 and 8.02 ± 0.52, respectively) (Figure 5J; Table 4). Ammonium reached higher values in the saline lakes (6.88 ± 5.53 mg L-1 and 4.65 ± 0.47 mg L-1, corresponding to Chalco and Texcoco, respectively), and values lower than 1 mg L-1 were recorded in Lakes Xochimilco and Zumpango (Figure 5K; Table 4). Finally, chlorides were higher in the saline lakes, with average values of 508.49 ± 112.69 and 547.05 ± 65.02 in Lakes Texcoco and Chalco, respectively, while Lakes Xochimilco and Zumpango showed chloride values of 60.78 and 279 mg L-1, respectively (Figure 5L). To note, all the lakes tested positive for fecal coliforms, with peak values of up to 800 ± 1600 MPN in Lake Chalco.
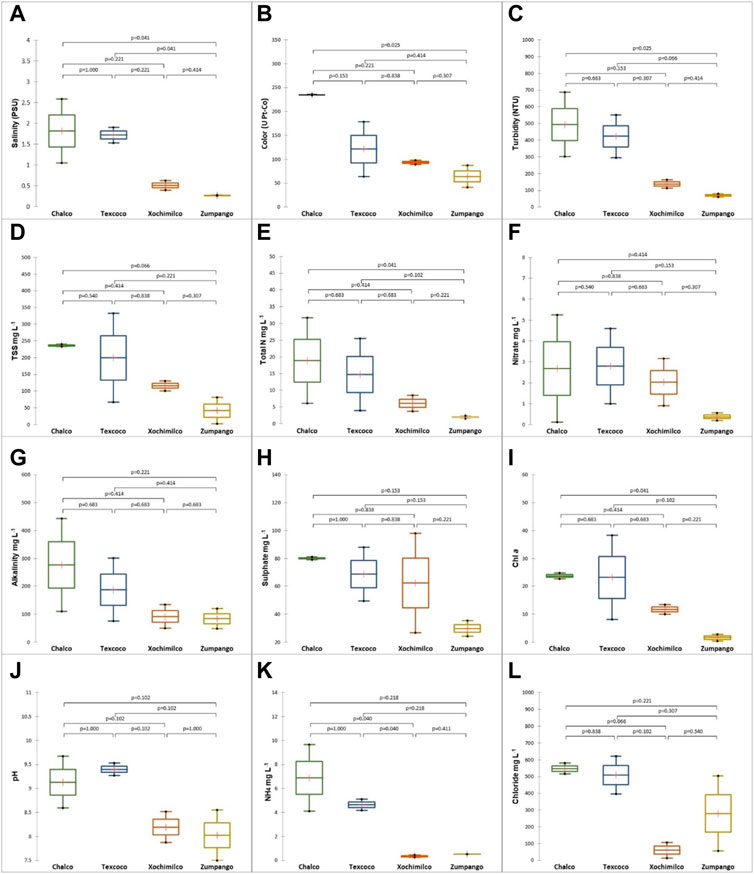
FIGURE 5. Box plots of environmental variables that show marked differences between lakes. (A) Salinity, (B) Color, (C) Turbidity, (D) Total Suspended Solids (TSS), (E) Total Nitrogen, (F) Nitrates, (G) Alkalinity, (H) Sulfates, (I) Chl a, (J) pH, (K) Ammonia, (L) Chloride.

TABLE 4. Heatmap of the Mean values and ± standard error of the main variables showing differences between lakes.
Regarding the differences between study periods, when comparing the mean salinity of saline and freshwater lakes, no significant differences were detected between the dry and rainy seasons (p > 0.05) (Table 5). Seasonal differences (although not significant) in pH were only observed for Lakes Xochimilco and Zumpango, but not for the saline lakes (Figure 6A). As for alkalinity and dissolved oxygen, seasonal differences (although not significant) between the dry and rainy seasons were observed for saline and freshwater lakes (Figures 6B, C). The highest alkalinity values were recorded in the rainy season for both types of lakes; in the case of dissolved oxygen, the highest values were observed in the dry season in both types of lakes (Table 5). On the other hand, for total N, ammonium, and turbidity, higher values were observed in saline lakes during the rainy season (Figures 6D–F). On the other hand, temperature, salinity, total P, orthophosphates, sulfates, color, chlorides, hardness, total suspended solids, BOD5, fecal coliforms, nitrites, and Chl a did not show seasonal differences (p > 0.05) between the dry and rainy seasons.
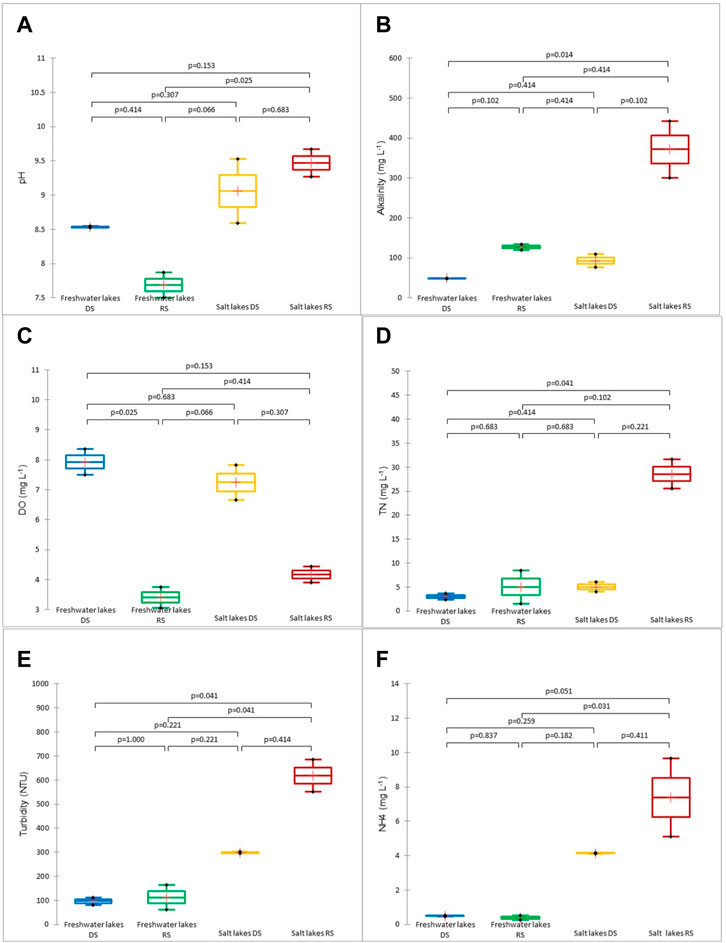
FIGURE 6. Box plots of environmental variables that showed significant differences between study periods for the types of lakes. (A) pH, (B) alkalinity, (C) dissolved oxygen (DO), (D) total nitrogen (TN), (E) turbidity, (F) ammonium.

TABLE 5. Heat map of the mean values and ± standard error of the variables that show differences between seasons (RS: Rainy season, DS: Dry season).
3.4.4 Integration of environmental variables and study periods
The principal component analysis of the study sites and the environmental variables showed an explained variance of 69.06% in its first two components. The diagram shows the dispersion of the study sites along environmental gradients in which clusters can be identified, with the saline lakes (Chalco and Texcoco) positioned on the right quadrants of the diagram (Figure 7A). In general, these lakes are characterized by the highest salinity, turbidity, and total suspended solids, and were also rich in nutrients (N and P) and attained high color scores (Figure 7B). Additionally, during the rainy season, these lakes showed higher ammonium and nitrate levels, as well as the highest Chl a concentration. On the other hand, in the dry season, these lakes had higher values of nitrites, orthophosphates, and chlorides, as well as the highest pH values (Figure 7B). For their part, the freshwater lakes, Xochimilco and Zumpango, were positioned on the left quadrants of the diagram (Xochimilco in the rainy season, on the margin of the upper right quadrant) (Figure 7A). During the dry season, the freshwater lakes, in addition to their lower salinity, attained the lowest alkalinity, nitrites, sulfates, nutrients (TN and TP), and BOD5. For its part, Lake Xochimilco during the rainy season showed high hardness and lower orthophosphates, nitrites, chlorides, and dissolved oxygen, while in the dry season, it showed lower salinity and concentration of nutrients (TN, NH4, nitrates, nitrites, TP, and orthophosphates), as well as the lowest turbidity, total suspended solids, pH, alkalinity, sulfates, color, and BOD5 (Figure 7B).
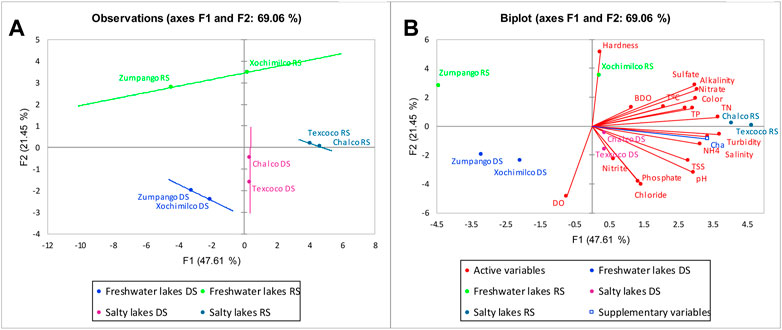
FIGURE 7. Biplot of the PCA with samples of the study lakes in the Basin of Mexico. (A) Groups of lakes by season, (B) arrangement of the lakes along environmental gradients, and vectors of the environmental factors assessed.
The physicochemical factors assessed suggest an environmental gradient: Lakes Chalco and Texcoco are clustered for their high salinity, while Lakes Xochimilco and Zumpango showed the lowest salinity. Saline lakes are also characterized by high alkalinity, nutrient enrichment, and, particularly, high chloride levels.
Despite the geographic proximity of Lake Chalco to Lake Xochimilco (both in the south of the basin), our results show that they currently have contrasting conditions in terms of salinity, chloride content, and alkalinity. All the lakes studied have faced a declining volume of water due to water drainage and extraction from their aquifers (Soto-Coloballes, 2019). The water bodies that were virtually driven to extinction are Lake Chalco (Ortega-Guerrero, et al., 1993) and Lake Texcoco (Soto-Coloballes, 2019). The intense exploitation of the aquifers of the Basin of Mexico has led to the progressive subsidence of this area, which in the case of Lake Chalco amounts to 40 cm/year, giving rise to an extensive plain. This subsidence has led to the formation of a “new Lake Chalco” in this topographic depression, with the water surface 12 m below the original ground level (Ortega-Guerrero, et al., 1993; Ortíz-Zamora and Ortega-Guerrero, 2007). This new Lake Chalco is fed by runoff water and the inflow of streams from mountainous areas, but also by untreated wastewater from adjacent towns and industrial areas that reaches the new lake through canals (Ortíz-Zamora and Ortega-Guerrero, 2007). According to paleolimnological studies, between 39,000 years BP and approximately 6,000 years BP, Lake Chalco has faced several episodes of high salinity and alkalinity alternating with acidity and freshwater conditions (Bradbury, 1989; Caballero and Ortega, 1998). Berres (2000), in his study on the ichthyofauna of the basin of Mexico, pointed out that the southern lakes of the Basin of Mexico, i.e., Lakes Chalco and Xochimilco, are freshwater lakes; however, our results show that Lake Chalco currently has alkaline and saline conditions, indicating a drastic environmental change. These conditions may result from the flooding of the so-called “New Lake Chalco”, thereby incorporating solutes previously precipitated during the draining of the former Lake Chalco. Those sediments were exposed to air and dried up through evaporation, resulting in saline soils; when the New Lake Chalco floods, these solutes are incorporated into the lake water.
Lake Xochimilco was also subjected to water extraction to supply drinking water to the downtown area. The declining inflow from springs led to a reduction in the surface area of the lake; this water body currently receives treated wastewater in addition to untreated domestic wastewater from households adjacent to the canals. According to our results, its waters show freshwater conditions, although with high contents of chloride and fecal coliforms.
Today, Lake Zumpango is an artificial reservoir located in the depression of the former Lake Zumpango and receives an inflow of runoff water from rainfall and treated wastewater. The former Lake Zumpango received the water from its tributary, the Cuautitlán River. Recently, however, this river has been diverted and now discharges its water in the Tajo de Nochistongo to avoid inputs to Lake Zumpango and, from it, to Lake Texcoco. The main water source entering Lake Zumpango is treated wastewater; consequently, the lake is now covered by the aquatic weed Eichornia crassipes. E. crassipeps (Water hyacinth) is an invasive species included among the 100 world´s worst invasive alien species (Lowe et al., 2000). This species reaches a fast growth leading to covering the total surface of lakes which produces high evapotranspiration, prevents the passage of light into the water column (limiting the photosynthetic activity), the gaseous exchange of the atmosphere with the water surface (causing depletion of dissolved oxygen in the water column), and promotes high evapotranspiration, thus represents a risk to remaining native biodiversity and the maintenance of water volume of lakes.
All the lakes studied, remnants of the former Great Lake of Mexico, have been desiccated and currently receive mainly treated wastewater from various treatment plants (PAOT, 2014; Soto-Coloballes, 2019), which brings about major changes in water quality, including nutrient enrichment. Lakes Chalco and Texcoco are indeed facing salinization and eutrophication processes.
Lake Texcoco has evolved from its previous soda-saline condition to a new one characterized by high levels of Na+ and Cl−from evaporation and the concentration of salts from the precipitation of CaCO3. For its part, Chalco, despite being a lake with a lower density than Texcoco that previously showed high alkalinity, today shows signs of a salinization process with a trend towards increasing Na+ and Cl− levels. Our findings are consistent with Martínez-Abarca et al. (2021), who pointed out that Lake Chalco has been reduced to a shallow and subsaline wetland. Both lakes were completely drained off, and the water bodies currently monitored are new. The area previously covered by Lake Texcoco today has systems built for recovering the lake (Soto-Coloballes, 2019), so that its evolution towards conditions with a predominance of Na+ and Cl− is the result of its previous draining and precipitation of calcium and sodium salts. Besides being drained, Lake Chalco, has also subsided due to the intensive exploitation of its groundwater. This groundwater extraction has resulted in the consolidation of its aquitard (Ortiz-Zamora and Ortega Guerrero, 2007) and, consequently, the formation of a depression or basin that has given rise to the “new” Lake Chalco, into which treated and untreated wastewater (Ortíz-Zamora and Ortega-Guerrero, 2007) is also discharged, in addition to runoff from the valley itself.
3.4.5 Water quality index
The Water Quality Index scores calculated in this study agree with the physicochemical analyses. Lake Xochimilco had the highest WQI score, with a mean value of 73.5, while Lakes Chalco and Texcoco attained the lowest WQI scores, with mean values of 64.8 and 62.7, respectively (Figure 8A). WQI scores were not significantly different between lakes.
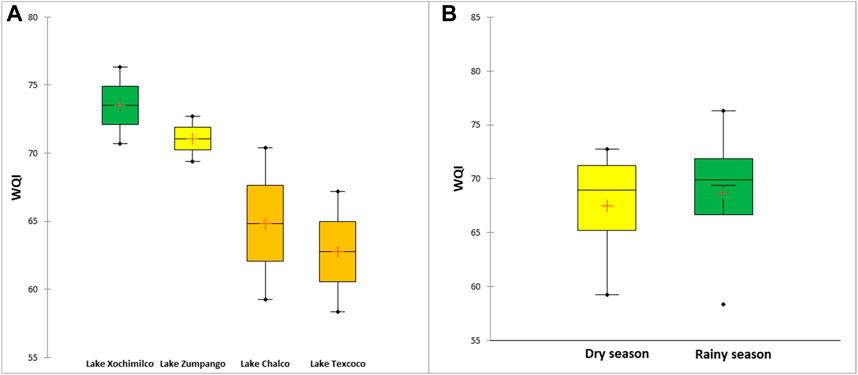
FIGURE 8. Box and whisker plot of (A) WQI score by lake in both study periods, and (B) WQI score by study period, considering all WQI scores for each lake.
Although the differences in WQI between study periods were not statistically significant (p > 0.05), our results show that the mean WQI scores during the dry season are slightly lower than during the rainy season (67.47 vs. 68.61, respectively) (Figure 8B).
Variables such as chlorides, conductivity, and total hardness can bring down the WQI scores, as pointed out by López-López et al. (2019), who recorded low WQI scores in a river with high values of these parameters. Maansi et al. (2022), estimating various water quality indices in Lake Sukhna, in India, found that higher hardness and alkalinity influenced the water quality. In this case, Lakes Chalco and Texcoco showed high values of multiple water parameters such as salinity and concentrations of chlorides, nitrates, sulfates, total nitrogen, and orthophosphates.
The WQI scores make evident that water from the lakes does not have conditions for its use in human supply and its use is limited to other uses such as agriculture and harbor wildlife. In the past, the lakes of the basin of México represented a resource not only for the antique human civilizations, including the first humans in America, but also to populations during the Spanish colonial period, offering several ecosystem services such as water supply, food, water for agriculture, transportation, climate regulation, and maintained high biodiversity.
In addition, given the endorheic nature of the basin, biological richness in the BM was characterized by species considered microendemic to the lakes of the BM, such as the fish Evarra bustamantei (Xochimilco carp), E. tlahuacensis (Tláhuac carp), E. eigenmanni (green carp), and G. viviparus (mexclapique). Unfortunately, the three species of the genus Evarra are extinct (IUCN, 2023), and G. viviparus (a viviparous fish) is currently listed as threatened (Sedeño-Díaz and López-López, 2009; IUCN, 2023). A species that stands out among the endemic amphibians is Ambystoma mexicanum, listed as critically endangered (IUCN, 2023). In all cases, the current conservation status has been associated with human intervention and habitat loss.
Unfortunately, currently, these lakes have received multiple stressors from wastewater pollution and even have faced desiccation resulting from human activities in the basin of one of the largest cities in the world, these conditions evidence improper management limiting the potential ecosystem services of lakes. The current diagnosis shows that the water quality of the lakes in the BM undergoes a eutrophication process more pronounced in saline lakes (Texcoco and Chalco lakes). Furthermore, during the rainy season, a depletion in dissolved oxygen and an increase in nutrient concentration were detected, showing a higher deterioration during this season. In this sense, lakes need urgent attention to diminish the input of pollutants and to carry out appropriate rehabilitation measures for each remnant lake. It is mandatory to conserve the endemic species that still prevail in the basin and promote the rehabilitation of the ecosystem services that these water bodies provide in the past. Furthermore, macroinvertebrates and microalgae species of ancestral human consumption such as Notonecta unifasciata, Krizousacorixa azteca, Corisella texcocana, Cambarellus montezumae, Phormidium tenue, Nostoc commune, and Chrooccocus turgidis, among others (Ortega, 1972), as well as the cyanobacterium Spirulina (Arthorspora) (Grant, 1992) should be recovery.
Future directions are necessary to take into account topics on best practices of wastewater management, rainwater harvesting to conserve aquifers, and aquatic weed management control methods. Likewise, it is important to draw up management programs containing restoration and conservation measures in the declared natural areas and Ramsar sites (Texcoco and Xochimilco), and if necessary, to establish declarations for the conservation of the Zumpango and Chalco lakes. This study establishes the baseline for comparison on the dramatic loss of lake area, which should not be allowed to continue, therefore suggesting increasing surface lake area, and slowing urban growth by setting buffer areas around the remaining lakes.
4 Conclusion
Different environmental and anthropogenic factors have led to the almost total disappearance of the lake system of the Basin of Mexico. The first formal assessment of the lakes was carried out by Alexander von Humboldt and allowed us to infer the state of these lakes in the early nineteenth century and assess the changes that have occurred since then. The former lakes no longer exist; the only water bodies that remain today are shallow wetlands representing only 2.83% of the original lake surface area. These water bodies have received treated wastewater discharges affecting their original water quality and ionic composition, with Lake Xochimilco being the lake with the best water quality. The most critical change is the case of Lake Chalco, which previously was less saline according to Humbodlt´s test results; today, Chalco is almost as saline as Texcoco, although the former is dominated by carbonates while Texcoco is dominated by Na+ and Cl−.
The assessment by Humboldt provides valuable information on the state of the BM lakes in the early 1800s and allows for comparing it versus current data to visualize the contrasting conditions of these lakes today. Population growth, urbanism, and the loss of natural land cover have been the leading factors that led to the current deplorable conditions observed in the present study. These water bodies should be the subject of conservation and recovery programs, as they are the habitat for multiple species that are microendemic to the Basin of Mexico, as well as for migratory birds; furthermore, some of these lakes have been declared as UNESCO World Heritage sites. American Public Health Association, 2023.
Data availability statement
The datasets presented in this article are not readily available because The data will be made available strictly for academic purposes upon justification. Requests to access the datasets should be directed to Dra. EL-L, eulopez@ipn.mx.
Author contributions
Conceptualization, analyzed the data, and wrote the article EL-L methodology, EL-L and JS-D; monitoring EL-L, VH, JS-D, MG, and AR-R; data curation EL-L and JS-D, GIS tools, JS-D review and editing EL-L, VH, JS-D, MG, and AR-R, original draft preparation EL-L and JS-D, validation EL-L, VH, JS-D, and MG, funding acquisition EL-L and JS-D. All authors contributed to the article and approved the submitted version.
Conflict of interest
The authors declare that the research was conducted in the absence of any commercial or financial relationships that could be construed as a potential conflict of interest.
Publisher’s note
All claims expressed in this article are solely those of the authors and do not necessarily represent those of their affiliated organizations, or those of the publisher, the editors and the reviewers. Any product that may be evaluated in this article, or claim that may be made by its manufacturer, is not guaranteed or endorsed by the publisher.
Supplementary material
The Supplementary Material for this article can be found online at: https://www.frontiersin.org/articles/10.3389/fenvs.2023.1217343/full#supplementary-material
References
Addinsoft (2023). XLSTAT statistical and data analysis solution. New York, United States: XLSTAT. https://www.xlstat.com.
Alcántara, J. L., and Escalante, P. (2005). Current threats to the Lake Texcoco globally important bird area. Bird Conservation Implement. Integration Am. 2.
Alcocer, J., and Williams, W. D. (1996). Historical and recent changes in Lake Texcoco, a saline lake in Mexico. Int. J. Salt Lake Res. 5 (1), 45–61. doi:10.1007/BF01996035
Alvarez, J., and Navarro, L. (1957). Los Peces del Valle de México. Comisión Nacional para el Fomento de la Piscicultura Rural México: Comision para el Fomento de la Piscicultura Rural, 62.
American Public Health Association (Apha), (2023). “American water works association, water environment federation,” in Standard methods for the examination of water and wastewater. Editors W. C. Lipps, E. B. Braun-Howland, and T. E. Baxter 24 (Washington DC: APHA Press).
Arce, J. L., Layer, P. W., Macías, J. L., Morales-Casique, E., García-Palomo, A., Jiménez-Domínguez, F. J., et al. (2019). Geology and stratigraphy of the Mexico basin (Mexico city), central trans-Mexican volcanic belt. J. Maps 15 (2), 320–332. doi:10.1080/17445647.2019.1593251
Berres, T. E. (2000). Climatic change and lacustrine resources at the period of initial aztec development. Anc. Mesoam. 11 (1), 27–38. doi:10.1017/S0956536100111101
Biar, A. (2020). A lacustrine cultural landscape in the prehispanic Basin of Mexico. Int. J. Naut. Archaeol. 49 (2), 341–356. doi:10.1111/1095-9270.12424
Bradbury, J. P. (1989). Late quaternary lacustrine paleoenvironments in the cuenca de México. Quat. Sci. Rev. 8, 75–100. doi:10.1016/0277-3791(89)90022-x
Caballero, M., and Guerrero, B. O. (1998). Lake levels since about 40,000 years ago at Lake Chalco, near Mexico city. Quat. Res. 50 (1), 69–79. doi:10.1006/qres.1998.1969
Candiani, V. S. (2014). Dreaming of dry land: Environmental transformation in colonial Mexico city. California, United States: Stanford University Press.
Conabio, (2023). Sistema geoportal de información. Available at: http://www.conabio.gob.mx/informacion/gis/(Accessed March 15, 2023).
Cruickshank Gracia, G. (2003). The recovery of the former Texcoco Lake. Voices of Mexico: UNAM, 111–116.
Dinius, S. H. (1987). Design of an index of water quality 1. JAWRA J. Am. Water Resour. Assoc. 23 (5), 833–843. doi:10.1111/j.1752-1688.1987.tb02959.x
Faye, C., and Ndiaye, M. (2021). Use of geospatial tools in morphometric analysis and prioritisation of sub-catchments of the soungrougrou (casamance basin). Quaest. Geogr. 40 (3), 65–84. doi:10.2478/quageo-2021-0024
García, E. (2004). Modificaciones al sistema de clasificación climática de Köppen. 5. México: Instituto de Geografía. Ciudad de México. Universidad Autónoma de México.
González, S., Jiménez-López, C., Hedges, R., Huddart, D., Ohman, J., Turner, A., et al. (2003). Earliest humans in the Americas: New evidence from México. J. Hum. Evol. 44, 379–387. doi:10.1016/s0047-2484(03)00004-6
Grant, W. D. (1992). “Alkaline environments,” in Encyclopedia of microbiology. Editor J. Lederberg (London: Academic), 73–80.
Gravelius, H. (1914). “Grundrifi der gesamten Gewâsserkunde,” in Band 1: h'lufikunde (compendium of hydrology?, vol. 1: Rivers, in German) (Berlin, Germany: Goschen).
Gurrfa, J. (1978). El Desague del Valle de Mexico Durante la Epoca Novohispana. México. UNAM: Instituto de Investigaciones Historicas, 175.
Heck, V. (2020). “Alexander von Humboldt als meister fächerverbindenden denkens,” in Naturwissenschaftliche Kompetenzen in der Gesellschaft von morgen. Editor S. Habig (Vienna: Gesellschaft für Didaktik der Chemie und Physik), 40, 548–551.
Holl, F. (2018). Alexander von Humboldt und der Klimawandel: Mythen und Fakten. Int. J. Humboldt Stud. 19 (37), 37–56. HiN XIX. doi:10.18443/273
Humboldt, A. von (2003). Reise auf dem Magdalena, durch die Anden und Mexiko. Aus seinen Reisetagebüchern zusammengestellt und erläutert durch Margot Faak, Bd. 2: Berlin. Übersetzung, Anmerkungen, Register, HydroSheds. Available at: https://www.hydrosheds.org/(Accessed March 15, 2023).
Humboldt, A. von (1802-1804). Tagebücher der Amerikanischen Reise VIII, a) 82v [156], b) 83r [157], c) 83r [157] – 83v [158]. Available at: https://digital.staatsbibliothek-berlin.de/werkansicht/?PPN=PPN779884590&PHYSID=PHYS_0001&view=overview-tiles (Accessed March 15, 2023).
INEGI (2023). Instituto nacional de Estadística y Geografía. Available at: https://www.inegi.org.mx/(Accessed March 15, 2023).
IUCN (2023). Red list. Available at: https://www.iucnredlist.org/(Accessed March 15, 2023).
Jones, B. E., Grant, W. D., Duckworth, A. W., and Owenson, G. G. (1998). Microbial diversity of soda lakes. Extremophiles 2, 191–200. doi:10.1007/s007920050060
Lamb, A. L., Gonzalez, S., Huddart, D., Metcalfe, S. E., Vane, C. H., and Pike, A. W. (2009). Tepexpan palaeoindian site, Basin of Mexico: Multi-proxy evidence for environmental change during the late pleistocene–late holocene. Quat. Sci. Rev. 28 (19-20), 2000–2016. doi:10.1016/j.quascirev.2009.04.001
Legorreta, J. (2006). El agua y la ciudad de México. De Tenochtitlán a la megalópolis del siglo XXI. México: Universidad Autónoma Metropolitana. Cd. México. ISBN 970-31-0522-X.
León Portilla, M. (1988). Historia verdadera de la conquista de la Nueva España: Bernal Díaz del Castillo. México: Secretaría de Educación Pública.
López-López, E., Sedeño-Díaz, J. E., Mendoza-Martínez, E., Gómez-Ruiz, A., and Martínez Ramírez, E. (2019). Water quality and macroinvertebrate community in dryland streams: The case of the tehuacán-cuicatlán biosphere reserve (México) facing climate change. Water 11, 1376. doi:10.3390/w11071376
López-López, E., Sedeño-Díaz, J. E., and Ruiz-Picos, R. A. (2016). “Shallow lakes of the Mexican central plateau: Assessing their health condition with oxidative stress biomarkers in sentinel organisms,” in Croatia. Editor M. Nageeb Rashed (London, UK: Intech).
Lowe, S., Browne, M., Boudjelas, S., and De Poorter, M. (2000). 100 of the world’s worst invasive alien species. A selection from the global invasive species database. Auckland, New Zealand: Hollands Printing Ltd.
MaansiJindal, R., and Wats, M. (2022). Evaluation of surface water quality using water quality indices (WQIs) in Lake Sukhna, Chandigarh, India. Appl. Water Sci. 12, 2. doi:10.1007/s13201-021-01534-x
Martínez-Abarca, L. R., Lozano-García, S., Ortega-Guerrero, B., Chávez-Lara, C. M., Torres-Rodríguez, E., Caballero, M., et al. (2021). Environmental changes during MIS6-3 in the Basin of Mexico: A record of fire, lake productivity history and vegetation. J. S. Am. Earth Sci. 109, 103231. doi:10.1016/j.jsames.2021.103231
Montero-Rosado, C., Ojeda-Trejo, E., Espinosa-Hernández, V., Fernández-Reynoso, D., Caballero Deloya, M., and Benedicto Valdés, G. S. (2022). Water diversion in the Valley of Mexico Basin: An environmental transformation that caused the desiccation of Lake Texcoco. Land 11 (4), 542. doi:10.3390/land11040542
Morales-García, S. S., Meza-Olvera, E., Shruti, V. C., and Sedeño-Díaz, J. E. (2020). Assessment of metal contamination and their ecological risks in wetland sediments of the former Texcoco saline lake, Mexico. J. Soils Sediments 20, 2912–2930. doi:10.1007/s11368-020-02613-3
Morrone, J. J., Escalante, T., Rodríguez-Tapia, g., Carmona, A., Arana, M., and Mercado-Gómez, J. D. (2022). Biogeographic regionalization of the Neotropical region: New map and shapefile. Ann. Braz. Acad. Sci. 94 (1), e20211167. doi:10.1590/0001-3765202220211167
Morrone, J. J. (2017). Neotropical biogeography: Regionalization and evolution. Florida, United States: CRC Press.
Ortega, M. M. (1972). Study of the edible algae of the Valley of Mexico. Bot. Mar. 15, 162–166. doi:10.1515/botm.1972.15.3.162
Ortega-Guerrero, A., Cherry, J. A., and Rudolph, D. L. (1993). Large-scale aquitard consolidation near Mexico City. Groundwater 31 (5), 708–718. doi:10.1111/j.1745-6584.1993.tb00841.x
Ortiz-Zamora, D. C., and Ortega Guerrero, M. A. (2007). Origin and evolution of a new lake in the Chalco plain: Implications for land subsidence and flooding hazards to the urban areas of valle de Chalco (state of Mexico) and tláhuac (federal district) (in Spanish). Investigaciones Geográficas, México: Boletín del Instituto de Geografía, UNAM.
Palma, A., Rivera, A., and Carmona, R. (2022). A unified hydrogeological conceptual model of the Mexico Basin aquifer after a century of groundwater exploitation. Water 14, 1584. doi:10.3390/w14101584
Paot, (2014). Procuraduria ambiental y de Ordenamiento territorial de la Ciudad de México. Available at: http://centro.paot.org.mx/documentos/paot/estudios/SIG_XOCH.pdf (Accessed March 15, 2023).
Richter, W., and Engshuber, M. (2014). Alexander von Humboldts messtechnik: Instrumente – methoden – ergebnisse. Berlin: epubli.
Rumsey, David (2023). David Rumsey map collection. Available at: https://www.davidrumsey.com/(Accessed March 15, 2023).
Sedeño-Díaz, J. E., López-López, E., Mendoza-Martínez, E., Rodríguez-Romero, A. J., and Morales-García, S. S. (2019). Distribution coefficient and metal pollution index in water and sediments: Proposal of a new index for ecological risk assessment of metals. Water 12 (1), 29. doi:10.3390/w12010029
Sedeño-Díaz, J. E., and López-López, E. (2009). Threatened fishes of the world: Girardinichthys viviparus (bustamante 1837) (cyprinodontiformes: Goodeidae). Environ. Biol. Fish. 84, 11–12. doi:10.1007/s10641-008-9380-4
Soto-Coloballes, N. V. (2019). Proyectos y obras para el uso de los terrenos desecados del antiguo lago de Texcoco, 1912-1998. Estud. Hist. Mod. Contemp. México (58), 259–287. doi:10.22201/iih.24485004e.2019.58.70695
Torres-Alves, G. A., and Morales-Nápoles, O. (2020). Reliability analysis of flood defenses: The case of the Nezahualcoyotl dike in the aztec city of Tenochtitlan. Reliab. Eng. Syst. Saf. 203, 107057. doi:10.1016/j.ress.2020.107057
Tortajada, C. (2008). Challenges and realities of water management of megacities: The case of Mexico city metropolitan area. J. Int. Aff., 147–166.
Tussupova, K., Anchita, , Hjorth, P., and Moravej, M. (2020). Drying lakes: A review on the applied restoration strategies and health conditions in contiguous areas. Water 12, 749. doi:10.3390/w12030749
UNESCO (2018). World urbanization prospects: The 2018 revision, methodology. New York: United Nations.
Vazquez-Suñe, E., and Serrano-Juan, A. (2012). Easy Quim v5. 0. Grupo de Hidrología subterránea. Available at: https://h2ogeo.upc.edu/es/software-hidrologia-subterrania/11-software-hidrologia-subterrania/42-easy-quim.
Wang, Shengbin, Xie, Zhan, Wang, Fenglin, Zhang, Yuqing, Wang, Wanping, Lui, Kui, et al. (2022). Geochemical characteristics and quality appraisal of groundwater from huatugou of the qaidam basin on the Tibetan plateau. Front. Environ. Sci. Front. Earth Sci., Sec. Geohazards Georisks. 10, 2022. doi:10.3389/feart.2022.874881
Keywords: former great lake of Mexico, contrasting conditions over time, freshwater and saline lakes, water quality index, lake extinction
Citation: López-López E, Heck V, Sedeño-Díaz JE, Gröger M and Rodríguez-Romero AJ (2023) A comparing vision of the lakes of the basin of Mexico: from the first physicochemical evaluation of Alexander von Humboldt to the current condition. Front. Environ. Sci. 11:1217343. doi: 10.3389/fenvs.2023.1217343
Received: 05 May 2023; Accepted: 03 July 2023;
Published: 19 July 2023.
Edited by:
Maria Ilhéu, University of Evora, PortugalReviewed by:
Agustina Cortelezzi, National Scientific and Technical Research Council (CONICET), ArgentinaAna Maria Antão-Geraldes, Instituto Politécnico de Braganča, Portugal
Copyright © 2023 López-López, Heck, Sedeño-Díaz, Gröger and Rodríguez-Romero. This is an open-access article distributed under the terms of the Creative Commons Attribution License (CC BY). The use, distribution or reproduction in other forums is permitted, provided the original author(s) and the copyright owner(s) are credited and that the original publication in this journal is cited, in accordance with accepted academic practice. No use, distribution or reproduction is permitted which does not comply with these terms.
*Correspondence: Eugenia López-López, eulopez@ipn.mx