Research progress and hotspot analysis of soil microplastics: a bibliometrics-based review
- 1Policy Research Center for Environmental and Economic, Ministry of Ecology and Environment of the People’s Republic of China, Beijing, China
- 2Inspur Software Group Ltd., Beijing, China
- 3School of Environment and Natural Resource, Renmin University of China, Beijing, China
Microplastics are ubiquitous in soil ecosystems, and they have attracted widespread attention owing to their accumulation, migration and diffusion in soil environments and their negative effects on the environment and human health. However, previous studies on microplastics have predominantly concentrated on aquatic environments, and their presence in soil ecosystems has seldom been studied systematically. This review presents a systematic analysis of the current research on soil microplastics based on the available literature from 2016 to 2023 using CiteSpace. The most influential journals, authors and countries in this field were identified. Keyword co-occurrence analysis was performed to determine research hotspots, and cluster analysis was employed to provide an accurate summary of this field. Furthermore, burst detection was used to explore research fronts. This review reveals the increasing depth and diversity of research on soil microplastics and discusses potential research fronts for future studies in this field.
1 Introduction
The widespread use of plastic products has greatly facilitated the productivity and daily life of people, but it also poses serious environmental problems. Global plastic production has risen exponentially from 1.5 MT in 1950 to 359 MT in 2018, and it is projected to reach 670 MT in 2040 (Delangiz et al., 2022). However, only 9% of the plastics are recycled each year (Yao et al., 2023). Discarded plastics gradually break down into smaller fragments and particles due to long-term microbial mediation or environmental factors such as wind, ultraviolet radiation, water and ice. Thompson et al. (2004) introduced the term “microplastics” in their paper on plastic debris in seawater and sediments. Microplastics are now typically defined as plastic debris and particles with a diameter of less than 5 mm, and they have attracted significant attention as novel environmental pollutants (Machado et al., 2018a). Microplastics have been detected in various ecosystems, including marine (Zhou et al., 2022), freshwater (Talbot and Chang, 2022), terrestrial (Wang et al., 2022) and airborne (Chandrakanthan et al., 2023). The United Nations Environment Programme (UNEP) has recognised microplastics as a significant emerging pollutant due to their small size, widespread distribution and long-lasting presence in the environment (UNEP, 2010; Su et al., 2019; Selonen et al., 2020).
Soil is a natural resource that is necessary for human survival and is threatened by microplastic pollution. However, while many studies have focused on microplastics in aquatic environments, research on soil microplastics started relatively late and is still in its early stages. Rillig (2012) were one of the first groups to publish an article calling attention to microplastic pollution in terrestrial ecosystems. Further research has led to the development of new technologies for quantifying and identifying microplastics in soil (Elert et al., 2017; Bläsing and Amelung, 2018). Microplastic pollution has been reported to be more prevalent in soil than in the ocean by four to 23 times (Chen et al., 2020). Microplastics may accumulate, migrate and diffuse in soil (Li et al., 2021), and they can affect soil ecosystems by interfering with the carbon and nitrogen cycling (Rillig et al., 2021; Rong et al., 2021). The leaching of additives that may be carcinogenic or endocrine disruptors can also cause toxicological harm (Lambert et al., 2014; Liwarska-Bizukojc, 2021). Microplastics can absorb and act as a vector for organic pollutants, heavy metals, antibiotic-resistant bacteria and antibiotic-resistant genes (ARGs) (Liu et al., 2021; Zhang et al., 2022). Thus, microplastic pollution has gradually become an urgent environmental and health problem.
Research on soil microplastics has been rapidly expanding the understanding of their distribution, identification and toxic effects. However, a systematic analysis of the research progress and hotspots of soil microplastics as a field is still lacking. Such a systematic summary will serve as an important reference for understanding and preventing the negative effects caused by microplastics in soil environments. We conducted a bibliometric analysis on a Web of Science database from 2016 to 2023 to systematically review the current state of the literature on soil microplastics. Based on our analysis, we identified the knowledge structure, development context, hot topics and developing trends in soil microplastics research, and we explored possible opportunities and challenges for the future. We expect our review to provide a theoretical basis for the research and management of soil microplastics.
2 Methodology
2.1 Data collection
The Web of Science Core Collection (WOSCC) was selected as the database for analysis. The search terms were set to TS (Topic Search) = (“microplastics” AND “soil”). Original articles were selected without considering papers from proceedings or conferences and book chapters. The search was conducted on 1 April 2023, and the retrieved papers were published from 2016 to 2023. The data retrieval started in 2016 because there were no results for soil microplastics published before 2015 in WOSCC database. All retrieved papers were manually checked to remove irrelevant documents, and 1,627 papers were kept. All records, including titles, abstracts and cited references, were exported as a pure text file for subsequent analysis.
2.2 Scientometric analysis
CiteSpace is a Java-based scientific visualisation software used for science mapping and scientific domain visualisation designed by Chen et al. (2006). CiteSpace provides various functions for exploring knowledge maps, including identifying the contributing authors, countries and journals, research hotspots and research fronts. We used CiteSpace (Version 6.2. R3) to analyse the frequency of studies on soil microplastics between 2016 and 2023. The time span was set to January 2016–April 2023, the time partition was 1 year, and the selected node types were author, country and keyword. The cropping method was selected as a pathfinder to prune sliced and merged networks. The rest of the settings were left at their default, and co-occurrence and clustering analyses were performed. In the obtained knowledge map, a node represented one item, such as a keyword, journal or reference. The links described the co-citation or co-occurrence between these nodes (Xie, 2015). Each node was represented by tree rings of varying thickness, indicating the number of citations and different colours representing the publication years of the literature cited (i.e., blue for oldest and orange for latest) (Ouyang et al., 2018). Nodes with high centrality were highlighted with a purple ring to better identify pivotal points (Freeman, 2008). The flow diagram of the study design is shown in Figure 1.
3 Results
3.1 Basic characteristics of the publications
3.1.1 Publication dynamics
We analysed the number of papers published each year to determine the general trend of soil microplastics research, as shown in Figure 2. In total, 1,627 papers related to soil microplastics were published between 2016 and 2023. From 2016 to 2018, no more than 50 papers were published each year, which we classified as a slow development stage. Several papers with a high citation frequency were published during this state, which helped the public become aware of the potential harm caused by microplastics in soil (Steinmetz et al., 2016; Machado et al., 2018b; Chae and An, 2018). During this stage, researchers mostly focused on analytical methods (Shan et al., 2018; Zhang SL et al., 2018), occurrence (Liu MT et al., 2018; Scheurer and Bigalke, 2018; Zhou et al., 2018), potential damage to soil organisms (Deng et al., 2017; Lwanga et al., 2017; Zhu D et al., 2018) and soil ecosystems (Machado et al., 2018a). Some papers focused on the interaction of microplastics with other pollutants, but the literature was limited (Hodson et al., 2017; Liu J et al., 2018). From 2019 to 2022, soil microplastics gradually gained significant attention, and the number of papers published each year increased rapidly, which we classified as a rapid development stage. In 2022, 600 papers were published, which is approximately three times the 216 papers published in 2020 and six times the 96 papers published in 2019. These results indicate that research on soil microplastics has entered a stage of rapid growth and diversification.
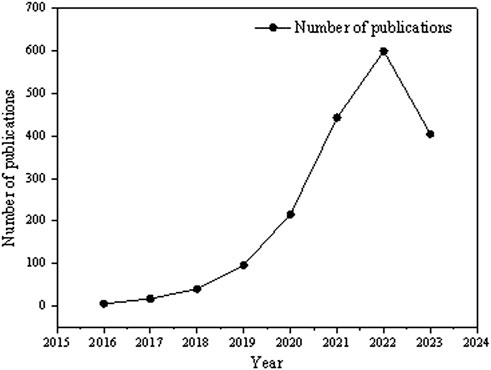
FIGURE 2. Number of papers on soil microplastics published each year in the WOSCC database (2016–2023).
3.1.2 Journal distribution
The key journals of a field can be identified from the distribution of related papers. Table 1 lists the 10 journals that published the most papers related to soil microplastics. Together, these journals published 781 papers or 55.04% of all retrieved papers. The four journals with the most published papers were Science of the Total Environment, Journal of Hazardous Materials, Environmental Pollution and Chemosphere. All these journals had an impact factor of greater than 5. The four journals with the greatest impact factors were the Journal of Hazardous Materials, Water Research, Environmental Science and Technology and Science of the Total Environment. Overall, the key journals for soil microplastics were determined as Science of the Total Environment, Journal of Hazardous Materials, Environmental Pollution and Chemosphere in terms of quantity and influence.
3.2 Cooperative network analysis
Co-author analysis was conducted to identify the major authors and their cooperative relationships. Figure 3 shows the cooperative network among authors. The node size indicates the influence of an author, and the links between nodes indicate a cooperative relationship between authors. A thicker link indicates a closer relationship. The most influential authors in the field of soil microplastics were identified as Matthias C. Rillig (Germany), Violette Geissen (Netherlands) and Luo Yongming (China). Closely cooperating research teams were also identified. The research team, with Matthias C. Rillig as the core and including Kim Shin Woong, mainly studied the effects of microplastics on nematodes in a soil environment (Kim et al., 2021a; Kim et al., 2021b). Violette Geissen and her research team mainly focused on the effects of microplastics on soil microbiomes as well as in agricultural soil. Luo Yongming and his research team, including He Defu, mainly carried out comprehensive reviews on soil microplastics. Overall, the authors in this field were closely linked with frequent communications.
Figure 4 shows the cooperative network among countries, which helps with understanding the geographic distribution of studies on soil microplastics. The ring size indicates the number of papers published by each country, and the links between countries indicate a cooperative relationship. Most research on soil microplastics has taken place in China (frequency = 826), followed by the United States (frequency = 180), Germany (frequency = 132), Australia (frequency = 106) and India (frequency = 81). Moreover, countries have closely cooperated with each other on soil microplastics. For example, the links between China and Germany, the United States and India, Australia and South Korea are relatively close and complex, indicating a closer cooperative relationship between these countries.
3.3 Keywords co-occurrence analysis
Keyword co-occurrence analysis is often used to explore research hotspots in a particular field. Figure 5 shows the results of the keyword co-occurrence analysis on soil microplastics. In total, 487 nodes and 1,509 links were detected. The first paper on soil microplastics was published by Rillig (2012). However, owing to the complexity of soil environments and the limitations of detecting microplastics in soil, research on microplastics has predominantly focused on aquatic environments. This explains the prominence of the keywords “water,” “fresh water” and “marine environment”. Table 2 lists the keywords related to soil microplastics and excludes those irrelevant keywords to identify the research hotspots. The most frequent keywords were “pollution” (frequency = 427), “microplastics” (frequency = 343), “soil” (frequency = 225), “plastics” (frequency = 186) and “particles” (frequency = 186). The centrality measures how much a node affects the shortest path between other nodes, and a higher centrality value indicates more influence (Zeb et al., 2022). The keywords with the highest centrality were “analytical methods” (centrality = 0.20), “organic pollutants” (centrality = 0.19), “community structure” (centrality = 0.19), “organic matter” (centrality = 0.14) and “ingestion” (centrality = 0.14). With regard to trends over time, research on soil microplastics has clearly expanded from an initial focus on pollution, identification and quantification to include fate, exposure toxicity, adsorption, biodegradation and bioaccumulation from 2016 to 2023.
3.4 Cluster analysis
We conducted a cluster analysis on keywords to clarify the different directions adopted by the research on soil microplastics. Figure 6 shows that research on soil microplastics can be divided into 12 clusters. Table 3 lists the clusters and their ID, size, silhouette, cluster label and respective keywords. Clusters #0 and #2 are focused on conventional and biodegradable microplastics. Conventional plastics are widely used in packaging, agricultural films, biomedical materials and 3D printing, but they have caused serious environmental pollution. With the increasing awareness of sustainable development, biodegradable plastics are gaining attention as a potential substitute (Wang et al., 2022). Biodegradable plastics comprise natural or microbial-secreted polymers that can be converted into H2O, CO2 and biomass through biological activity under suitable conditions (e.g., temperature, humidity and microorganisms) (Rujnic-Sokele and Pilipovic, 2017). However, biodegradable plastics have the same lifespan as conventional plastics if the degradation conditions are not met, and they may disintegrate into microplastics more rapidly than conventional plastics. Thus, they have emerged as another threat to the environment (Wei et al., 2021). Moreover, the separation and extraction standards; environmental behaviour and ecological effects; impact on microorganisms, soil fauna and plants; and adsorption and desorption of toxic substances onto biodegradable microplastics remain to be studied.
Clusters #8, #10, and #11 are focused on the distribution characteristics and analytical methods for soil microplastics. Microplastics have been found in soils across all seven continents in diverse environments such as coastlines, farmland, riparian forest buffer zones and cropped areas (Yang et al., 2022). Through a method based on pressurized fluid extraction (PFE), microplastics were detected in 90% of soil samples from an industrial area in Sydney, with concentrations in the range of 300–67,500 mg/kg (Fuller and Gautam, 2016). In Iran and Chile, the microplastic concentrations in farmland soils were 67–400/kg and 600–10,400/kg, respectively (Corradini et al., 2019; Rezaei et al., 2019). The average abundance of microplastics in farmland soils in southeast Germany was (0.34 ± 0.36)/kg (Piehl et al., 2018). As a major producer and user of plastic products, China’s soil is contaminated with microplastics to varying degrees. Microplastics have been found in mulched farmland soils in Shanghai, Liaoning and Shanxi Provinces with abundances as high as 10,586/kg, and the abundance increases with the number of mulching years (Lv et al., 2019; Ding et al., 2020; Li et al., 2022). Huang et al. (2020) investigated microplastics in agricultural soils by analysing 384 soil samples collected from 19 provinces across China. They found that the microplastic concentrations in the soil samples were 0.1–324.5 kg/ha, with an average of 83.6 kg/ha. However, soils is a system with complex composition, and microplastics may interact with soil components such as organic matter, mineral soil and chemical ingredients, posing an adverse effect on the detection of soil microplastics (Zhang et al., 2020). Therefore, while various analytical methods have been developed to study the microplastics in aquatic environments, there is currently no standardised analytical process available for soil microplastics.
Analysing microplastics in soil samples involves extraction, separation, identification and quantification. A method for effectively separating microplastics from soil samples is required. Density separation is commonly used to isolate low-density plastic particles from soil samples, which formed a distinct cluster in the retrieved literature. Sodium chloride (NaCl), which has a density of 1.2 g·cm−3, is commonly used to separate less dense particles from environmental media (Thompson et al., 2004). In addition, high-density solutions such as saturated sodium polytungstate can be used to isolate certain high-density microplastics (Corcoran et al., 2009). After separation, microplastics are commonly identified by microscopy, spectroscopy and thermal analysis (Woo et al., 2021). Light microscopy is commonly used for identifying microplastics with large particle sizes, but its reliability and accuracy are poor. Scanning electron microscopy and transmission electron microscopy have also been used for microplastic identification. Fourier transform infrared (FTIR) spectroscopy can be used to easily identify specific chemical bonds and reveal the polymer composition of microplastics. Raman spectroscopy can generate unique spectra for polymers with different molecular structures and atoms, so it provides an efficient way to explore the chemical structure of microplastics (Witzig et al., 2020). Recently, thermal analyses have been utilised to identify microplastics in samples, and they include techniques such as differential scanning calorimetry (DSC, Arhant et al., 2019), thermogravimetric analysis (TGA, Uheida et al., 2020) and pyrolysisgas chromatography-mass spectrometry (Py-GC-MS, Mizuguchi et al., 2023). Thermal analysisis often complemented by other techniques, such as GC-MS, to overcome its limitations (Bitter and Lackner, 2021). New instruments have been applied to improve the efficiency and accuracy of microplastic identification in recent years, and they are sometimes used in combination to compensate for the shortcomings of individual technologies. Erni-Cassola et al. (2017) combined visual classification with fluorescent staining to identify microplastics. Shim et al. (2016) used Nile Red [9-diethylamino5H-benzo (α) phenoxazine-5-one] to stain microplastics. Atomic force microscopy (AFM) can be combined with infrared and Raman spectroscopy for the analysis of microplastics and even nanoplastics. AFM can achieve nanometer-resolution imaging to reveal the chemical structure of target plastics (Luo et al., 2021a). Near-infrared (NIR) spectroscopy (Paul et al., 2019), vis–NIR spectroscopy (Garaba and Dierssen, 2017) and nano-thermal analysis (Luo et al., 2021b) have all been used for microplastic identification. Overall, analytical methods have been rapidly developed for research on microplastics, but a standardised approach is still lacking. Reliable detection and monitoring methods are urgently needed so that measured data from different sources can be compared.
Clusters #3, #4, and #7 are focused on the composite toxicity of microplastics and other pollutants in soil (e.g., heavy metals, organic pollutants, ARGs). Due to their small size, strong hydrophobicity and large specific surface area, microplastics have strong adsorption properties and can act as vectors for many contaminants in soil. Microplastics have been measured to have pollutant concentrations hundreds of or even thousands of times higher than that of their surrounding environment (Mato et al., 2001; Hirai et al., 2011). The interactions of microplastics with other contaminants may alter their surface properties, environmental behaviour and toxic effects and thus increase their adverse impact on soil ecosystems (Wang F et al., 2022). Heavy metals are a common soil pollutant and easily interact with microplastics through electrostatic attraction, chemical bonding and binding with oxygen-containing functional groups on their surface. Microplastics can also interact with other hydrophobic organic pollutants such as polychlorinated biphenyls, polycyclic aromatic hydrocarbons, petroleum hydrocarbons (PHCs) and organochlorine pesticides (OCPs). ARGs pose a significant risk to human health and have become a major public concern. Microplastics can serve as a host for distinct bacterial communities compared to the surrounding environment and exert selection pressure for the development of ARGs (Liu et al., 2021). In addition, additives (such as plasticizers, flame retardants, photostabilizers, antioxidants, and pigments) are intentionally added into plastics during production to maintain or enhance their properties (Do et al., 2022). Many of these organic additives are hazardous. After entering the soil, microplastics can undergo a series of aging and wear process, and their own additives are gradually released and coexist with microplastics in the soil environment, causing joint pollution to the soil ecosystem. Overall, the risk posed by microplastics combined with coexisting pollutants is still unclear in terms of toxicity and formation and requires further studies.
Cluster #6 is focused on the microplastics from sewage sludge. Microplastics is a common pollutant in wastewater treatment plants (Murphy et al., 2016). Wastewater treatment plants can effectively remove microplastics from wastewater, and over 95% of microplastics in wastewater are removed and concentrated in sewage sludge, which is often applied to fertilize fields as agricultural amendment and soil compost due to its good properties as fertilizer, posing a potential threat for soil health (Li et al., 2018; Berg et al., 2020; Rolsky et al., 2020). According to the review of Bläsing and Amelung. (2018), the concentrations of plastic detected in sludge ranged from 1,500 to 24,000 items kg−1. The high microplastics load of sewage sludge makes it one of the most important input path of microplastic to soil (Nizzetto et al., 2016). The presence of microplastics in sewage sludge derived from wastewater treatment plants has only been studied to a limited extent. The fate of microplastics in sludge treatments, and the effect of microplastics derived from sludge processes to soil system need to be further studied.
Cluster #9 is focused on the impact of microplastics on gut microbiota. Gut microbiota plays an important role in on host health, metabolism and immunity. In addition, it is closely related to soil decomposition processes. Due to the differences between the microbial gut environment and the surrounding soil, gut microbiota of soil organisms is significantly distinct from that in the surrounding soil (Thakuria et al., 2010). However, research on the effects of microplastics on gut microbiota is in its absolute infancy. As an exogenous soil pollutant, microplastics can generally induce gut microbiota dysbiosis in terrestrial organisms. For example, studies on the effects of microplastics on the gut microbiota of soil organisms have shown that microplastics could induce gut microbiota dysbiosis in collembolan Folsomia candida (Zhu et al., 2018), Tenebrio molitor larvae (Urbanek et al., 2020), mice (Jin et al., 2019), and soil oligochaete Enchytraeus crypticus (Zhu et al., 2018). In contrast, Wang et al. (2019) reported that microplastics exposure has little effect on the gut bacterial communities of earthworms Metaphire californica. Different types, sizes, shapes, additives, as well as exposure concentration and time of microplastics may influence the effects of microplastics on the gut microbiota of soil organisms, which should be investigated systematically.
3.5 Hotspots and emerging trends of soil microplastics research
Burst detection is used for monitoring transitions in the research fronts of a field. Table 4 lists the top-15 keywords in terms of burst strength and duration. The red line shows when a keyword was most frequently cited.
Keywords such as “litter,” “ingestion” and “extraction” had burst durations of more than 4 years, which indicates that these topics have always intrigued researchers and have been extensively studied. Between 2016 and 2020, the keyword “litter” had a burst strength of 8.21, which indicates continued concern about the possible threat of microplastics to terrestrial ecosystems and humans. The keyword “ingestion” appeared between 2016 and 2019, indicating concerns about the exposure of organisms, particularly humans, to microplastics through ingestion. Humans can ingest microplastic particles through ingestion, inhalation or skin contact. Cox et al. (2019) suggested that people might ingest 39,000–52,000 microplastic particles annually through food. Microplastics can also enter the human body through the food chain. The keyword “extraction” is an essential analytical procedure for soil microplastics, and it indicates concern over the development of analytical methods. The keyword “synthetic fibers” showed the highest burst strength of 13.33. Synthetic fibres can be considered as a type of microplastics, which can be shed from synthetic fabrics during conventional washing of synthetic fiber clothes, as well as falling out in indoor environments (Falco et al., 2019; Zhang et al., 2020). Nowadays, synthetic fibres have been found in various environmental media, and the additives and dyes contained in synthetic fibres are hazardous to wildlife and humans (Velasco et al., 2022), which have posed widespread concern. In addition, the keywords “abundance” and “persistent organic pollutants” also demonstrated relatively high burst strength. The keyword “abundance” suggests concern over the abundance of microplastics in soil found in different regions of the seven continents (Zhang and Liu, 2018; Zhou et al., 2018; Yang et al., 2022). The keyword “persistent organic pollutants” indicates concern over the interactions between microplastics and persistent organic pollutants.
We defined the latest research front as keywords that emerged from 2020 to 2023, which included “dissolved organic matter,” “deposition” and “knowledge gaps”. The keyword “dissolved organic matter” indicates interest in the role of dissolved organic matter in the fate and transport of microplastics (Cao et al., 2023). The keyword “deposition” indicates concern over microplastics suspended in the atmosphere that can settle on soil surfaces through air transport (Cai et al., 2017; Liu et al., 2019). The keyword “knowledge gaps” indicates an awareness that research on soil microplastics is immature and requires further development. Sampling, extraction, separation, identification and quantification procedures should be standardised. In addition, research is still lacking on the mechanism by which microplastics influence soil properties and their direct or indirect toxicity on plants, fauna, microorganisms and humans. The adsorption mechanism and consequences of microplastics as pollutant vectors should also be further investigated (Rocha-Santos and Duarte, 2015).
4 Discussion
Microplastics have been found in diverse soil types across continents. However, compared with marine and freshwater ecosystems, the soil matrix is complex with numerous factors; hence, research on soil microplastics is still in progress. Accurate monitoring, including extraction, separation, identification and quantification, is essential for improved understanding of soil microplastics. Improved technologies with high sensitivity and precision have been utilised to detect soil microplastics, but assessment methods are still in the developmental phase. Moreover, the absence of standardised procedures for sampling, pre-treatment and quantification of microplastics has impeded the comparison of microplastic pollution across different regions. Soils have complex compositions with diverse pollutants, such as heavy metals, organic pollutants and ARGs, with which microplastics interact readily. The combined effects on soil environment and organisms are also of concern. Researchers have made significant efforts to explore the impact of microplastics on soil biota and health, and much progress has been made in understanding their toxic effects on soil fauna, plants and microorganisms, as well as their direct influence on the physicochemical properties and material circulation of soil. However, research on the mechanism by which microplastics interact with the soil matrix is still in the preliminary stage. Most studies on the toxic effects of microplastics on soil biota have been conducted in laboratories; hence, they do not accurately reflect real-world environments. Microplastics can affect the digestive, respiratory, reproductive and cardiovascular systems of humans through ingestion, inhalation or skin contact. However, research on microplastics has predominantly been conducted on animals, and further studies are needed to explore their effects on humans.
We conducted a bibliometric analysis to clarify the research progress, hotspots and developing trends of soil microplastics. Research on soil microplastics can be divided into two stages. In the slow development stage (2016–2018), researchers noticed the potential harm caused by microplastics in soil and focused on determination methods and their occurrence and accumulation in soil. In the rapid development stage (2019–2023), growing concern on soil microplastics led to diversified research directions that considered their fate, exposure toxicity, adsorption, biodegradation and bioaccumulation. Cluster analysis identified 12 clusters of research on soil microplastics, including biodegradable microplastics, the distribution and analytical methods, the composite toxicity of microplastics with other pollutants and their effect on soil microorganisms. Burst detection indicated that research on soil microplastics has continued to focus on the development of analytical procedures and possible threats to terrestrial ecosystems and humans. Moreover, many knowledge gaps that remain unaddressed in this field are gaining attention in recent times.
We suggest that future research on soil microplastics should focus on the following aspects:
• Develop a standardised analytical procedure and unit for measuring the concentration of microplastics in the soil (e.g., by quantity or weight) and systematically monitor their distribution in soils with different land uses and from different regions.
• Examine the relationship between microplastics and biochemical processes in the soil to clarify the mechanism by which they affect soil properties and their direct or indirect toxicity while they move through the food chain.
• In addition to laboratory-based studies, conduct field trials with doses close to realistic levels in the environment to analyse the potential risks and propose environmental management and restoration strategies.
• Collect more information on how microplastics combine with other environmental pollutants and their toxicological effects on soil biota and health.
• Explore separation and extraction standards for biodegradable microplastics; their environmental behaviour and ecological effects; their impact on microorganisms, fauna and plants; and the adsorption and desorption of toxic substances from biodegradable microplastics as well as investigate the feasibility of using them for sustainable development.
Plastics are necessary to current lifestyles. Instead of banning the use of plastic products, sustainable strategies are required to reduce the microplastic contents in soil. For example, legislation should be proposed to strengthen the control over the use of non-degradable plastics and related products and to increase public awareness for sorting household garbage. More efficient technologies and tighter technical standards should be proposed to improve the recycling rate of plastic waste, providing more theoretical and practical support for plastic waste control.
Bibliometric methods based on CiteSpace can clarify the research context of a certain field. However, compared with traditional reviews, CiteSpace has its limitations. For example, Citespace software is time-consuming and laborious on merging synonyms, which have to be combined manually. In addition, Citespace is presented in a literature statistical manner and lacks the of experimental study support, which may obscure the information of the research itself. In future studies, the combination of bibliometric analysis and other analyses (such as model analysis) may be an effective way to make the results more realistic. Moreover, we should note that while there is no significantly impact on the main conclusions of the work, it is undeniable that some detailed information, such as the quantity, weight and size of microplastics, was omitted when using Citespace for literature analysis. In the future, the combination of Citespace and pluralistic text analysis methods, such as word frequency analysis, entity recognition, relationship analysis, etc., is expected to enrich the discussion of detailed information. We also look forward to developing new software on the basis of citespace to further embed these text screening functions.
Author contributions
JM: Conceptualization, Methodology, Software, Visualization, Writing–original draft, Writing–review and editing. WH: Methodology, Software, Visualization, Writing–original draft. RP: Validation, Writing–review and editing. KZ: Conceptualization, Supervision, Validation, Writing–review and editing.
Funding
The author(s) declare that no financial support was received for the research, authorship, and/or publication of this article.
Conflict of interest
Author WH was employed by Inspur Software Group Ltd.
The remaining authors declare that the research was conducted in the absence of any commercial or financial relationships that could be construed as a potential conflict of interest.
Publisher’s note
All claims expressed in this article are solely those of the authors and do not necessarily represent those of their affiliated organizations, or those of the publisher, the editors and the reviewers. Any product that may be evaluated in this article, or claim that may be made by its manufacturer, is not guaranteed or endorsed by the publisher.
Abbreviations
AFM, Atomic force microscopy; ARG, ntibiotic-resistant genes; DSC, Differential scanning calorimetry; NIR, Near-Infrared; TS, Topic Search; UNEP, United Nations Environment Programme; WOSCC, Web of Science Core Collection.
References
Arhant, M., Le Gall, M., Le Gac, P.-Y., and Davies, P. (2019). Impact of hydrolytic degradation on mechanical properties of PET-Towards an understanding of microplastics formation. Polym. Degrad. Stab. 161, 175–182. doi:10.1016/j.polymdegradstab.2019.01.021
Berg, P. V. D., Huerta-Lwanga, E., Corradini, F., and Geissen, V. (2020). Sewage sludge application as a vehicle for microplastics in eastern Spanish agricultural soils. Environ. Pollut. 261, 114198. doi:10.1016/j.envpol.2020.114198
Bitter, H., and Lackner, S. (2021). Fast and easy quantification of semi-crystalline microplastics in exemplary environmental matrices by differential scanning calorimetry (DSC). Chem. Eng. J. 423, 129941. doi:10.1016/j.cej.2021.129941
Bläsing, M., and Amelung, W. (2018). Plastics in soil: analytical methods and possible sources. Sci. Total Environ. 612, 422–435. doi:10.1016/j.scitotenv.2017.08.086
Cai, L. Q., Wang, J. D., Peng, J. P., Tan, Z., Zhan, Z. W., Tan, X. L., et al. (2017). Characteristic of microplastics in the atmospheric fallout from Dongguan city, China: preliminary research and first evidence. Environ. Sci. Pollut. Res. Int. 24, 24928–24935. doi:10.1007/s11356-017-0116-x
Cao, Y. X., Ma, X. Y., Chen, N., Chen, T. T., Zhao, M. J., Li, H. H., et al. (2023). Polypropylene microplastics affect the distribution and bioavailability of cadmium by changing soil components during soil aging. J. Hazard Mater 443, 130079. doi:10.1016/j.jhazmat.2022.130079
Chae, Y., and An, Y. J. (2018). Current research trends on plastic pollution and ecological impacts on the soil ecosystem: a review. Environ. Pollut. 240, 387–395. doi:10.1016/j.envpol.2018.05.008
Chandrakanthan, K., Fraser, M. P., and Herckes, P. (2023). Airborne microplastics in a suburban location in the desert southwest: occurrence and identification challenges. Atmos. Environ. 298, 119617–122310. doi:10.1016/j.atmosenv.2023.119617
Chen, C. M., Chen, Y., Hou, J. H., and Liang, Y. X. (2006). CiteSpace II: detecting and visualizing emerging trends and transient patterns in scientific literature. J. China Soc. Sci. Tech. Inf. 28, 401–421. doi:10.1002/asi.20317
Chen, H. P., Wang, Y. H., Sun, X., Peng, Y. K., and Xiao, L. (2020). Mixing effect of polylactic acid microplastic and straw residue on soil property and ecological function. Chemosphere 243, 125271. doi:10.1016/j.chemosphere.2019.125271
Corcoran, P. L., Biesinger, M. C., and Grifi, M. (2009). Plastics and beaches: a degrading relationship. Mar. Pollut. Bull. 58, 80–84. doi:10.1016/j.marpolbul.2008.08.022
Corradini, F., Meza, P., Eguiluz, R., Casado, F., Huerta-Lwanga, E., and Geissen, V. (2019). Evidence of microplastic accumulation in agricultural soils from sewage sludge disposal. Sci. Total Environ. 671, 411–420. doi:10.1016/j.scitotenv.2019.03.368
Cox, K. D., Covernton, G. A., Davies, H. L., Dower, J. F., Juanes, F., and Dudas, S. E. (2019). Human consumption of microplastics. Environ. Sci. Technol. 53, 7068–7074. doi:10.1021/acs.est.9b01517
Delangiz, N., Aliyar, S., Pashapoor, N., Nobaharan, K., Lajayer, B. A., and Rodríguez-Couto, S. (2022). Can polymer-degrading microorganisms solve the bottleneck of plastics’ environmental challenges? Chemosphere 294, 133709. doi:10.1016/j.chemosphere.2022.133709
Deng, Y., Zhang, Y., Lemos, B., and Ren, H. (2017). Tissue accumulation of microplastics in mice and biomarker responses suggest widespread health risks of exposure. Sci. Rep. 7, 46687. doi:10.1038/srep46687
Ding, L., Zhang, S. Y., Wang, X. Y., Yang, X. M., Zhang, C. T., Qi, Y. B., et al. (2020). The occurrence and distribution characteristics of microplastics in the agricultural soils of Shaanxi Province, in north-western China. Sci. Total Environ. 720, 137525. doi:10.1016/j.scitotenv.2020.137525
Do, A. T. N., Ha, y, and Kwon, J. H. (2022). Leaching of microplastic-associated additives in aquatic environments: a critical review. Environ. Pollut. 305, 119258. doi:10.1016/j.envpol.2022.119258
Elert, A. M., Becker, R., Duemichen, E., Eisentraut, P., Falkenhagen, J., Sturm, H., et al. (2017). Comparison of different methods for MP detection: what can we learn from them, and why asking the right question before measurements matters. Environ. Pollut. 231, 1256–1264. doi:10.1016/j.envpol.2017.08.074
Erni-Cassola, G., Gibson, M. I., Thompson, R. C., and Christie-Oleza, J. A. (2017). Lost, but found with nile red: a novel method for detecting and quantifying small microplastics (1 mm to 20 μm) in environmental samples. Environ. Sci. Technol. 51, 13641–13648. doi:10.1021/acs.est.7b04512
Falco, F. D., Pace, E. D., Cocca, M., and Avella, M. (2019). The contribution of washing processes of synthetic clothes to microplastic pollution. Sci. Rep. 9, 6633. doi:10.1038/s41598-019-43023-x
Freeman, L. C. (2008). Centrality in social networks conceptual clarification. Soc. Netw. 1, 215–239. doi:10.1016/0378-8733(78)90021-7
Fuller, S., and Gautam, A. (2016). A procedure for measuring microplastics using pressurized fluid extraction. Environ. Sci. Technol. 50, 5774–5780. doi:10.1021/acs.est.6b00816
Garaba, S., and Dierssen, H. (2017). Spectral reference library of 11 types of virgin plastic pellets common in marine plastic debris. Available online: https://ecosis.org/package/spectral-reference-library-of-11-types-of-virgin-plastic-pellets-commonin-marine-plastic-debris (accessed on September 25, 2021).
Hirai, H., Takada, H., Ogata, Y., Yamashita, R., Mizukawa, K., Saha, M., et al. (2011). Organic micropollutants in marine plastics debris from the open ocean and remote and urban beaches. Mar. Pollut. Bull. 62, 1683–1692. doi:10.1016/j.marpolbul.2011.06.004
Hodson, M. E., Duffus-Hodson, C. A., Clark, A., Prendergast-Miller, M. T., and Thorpe, K. L. (2017). Plastic bag derived-microplastics as a vector for metal exposure in terrestrial invertebrates. Environ. Sci. Technol. 51, 4714–4721. doi:10.1021/acs.est.7b00635
Huang, Y., Liu, Q., Jia, W. Q., Yan, C. R., and Wang, J. (2020). Agricultural plastic mulching as a source of microplastics in the terrestrial environment. Environ. Pollut. 260, 114096. doi:10.1016/j.envpol.2020.114096
Jin, Y., Lu, L., Tu, W., Luo, T., and Fu, Z. (2019). Impacts of polystyrene microplastic on the gut barrier, microbiota and metabolism of mice. Sci. Total Environ. 649, 308–317. doi:10.1016/j.scitotenv.2018.08.353
Kim, S. W., Liang, Y., Zhao, T. T., and Rillig, M. C. (2021a). Indirect effects of microplastic-contaminated soils on adjacent soil layers: vertical changes in soil physical structure and water flow. Front. Environ. Sci. 9, 681934. doi:10.3389/fenvs.2021.681934
Kim, S. W., Waldman, W. R., Kim, T. Y., and Rillig, M. C. (2021b). Effects of different microplastics on nematodes in the soil environment: tracking the extractable additives using an ecotoxicological approach. Environ. Sci. Technol. 54, 13868–13878. doi:10.1021/acs.est.0c04641
Lambert, S., Sinclair, C. J., and Boxall, A. B. (2014). Occurrence, degradation and effect of polymer-based materials in the environment. Rev. Environ. Contam. Toxicol. 227, 1–53. doi:10.1007/978-3-319-01327-5_1
Li, S. T., Ding, F., Flury, M., Wang, Z., Xu, L., Li, S. Y., et al. (2022). Macro- and microplastic accumulation in soil after 32 years of plastic film mulching. Environ. Pollut. 300, 118945. doi:10.1016/j.envpol.2022.118945
Li, X., Chen, L., Mei, Q., Dong, B., Dai, X., Ding, G., et al. (2018). Microplastics in sewage sludge from the wastewater treatment plants in China. Water Res. 142, 75–85. doi:10.1016/j.watres.2018.05.034
Li, X. N., Jiang, X., Song, Y., and Chang, S. X. (2021). Coexistence of polyethylene microplastics and biochar increases ammonium sorption in an aqueous solution. J. Hazard Mater 405, 124260. doi:10.1016/j.jhazmat.2020.124260
Liu, J., Ma, Y., Zhu, D. Q., Xia, T. J., Qi, Y., Yao, Y., et al. (2018). Polystyrene nanoplastics-enhanced contaminant transport: role of irreversible adsorption in glassy polymeric domain. Environ. Sci. Technol. 52, 2677–2685. doi:10.1021/acs.est.7b05211
Liu, K., WangFang, X. H. T., Xu, P., Zhu, L. X., and Li, D. J. (2019). Source and potential risk assessment of suspended atmospheric microplastics in Shanghai. Sci. Total Environ. 675, 462–471. doi:10.1016/j.scitotenv.2019.04.110
Liu, M. T., Lu, S. B., Song, Y., Lei, L. L., Hu, J. N., Lv, W. W., et al. (2018). Microplastic and mesoplastic pollution in farmland soils in suburbs of Shanghai, China. Environ. Pollut. 242, 855–862. doi:10.1016/j.envpol.2018.07.051
Liu, Y., Liu, W. Z., Yang, X. M., Wang, J., Lin, H., and Yang, Y. Y. (2021). Microplastics are a hotspot for antibiotic resistance genes: progress and perspective. Sci. Total Environ. 773, 145643. doi:10.1016/j.scitotenv.2021.145643
Liwarska-Bizukojc, E. (2021). Effect of (bio) plastics on soil environment: a review. Sci. Total Environ. 795, 148889. doi:10.1016/j.scitotenv.2021.148889
Luo, H., Xiang, Y., Li, Y., Zhao, Y., and Pan, X. (2021a). Photocatalytic aging process of Nano-TiO2 coated polypropylene microplastics: combining atomic force microscopy and infrared spectroscopy (AFM-IR) for nanoscale chemical characterization. J. Hazard Mater 404, 124159. doi:10.1016/j.jhazmat.2020.124159
Luo, H., Zeng, Y., Zhao, Y., Xiang, Y., Li, Y., and Pan, X. (2021b). Effects of advanced oxidation processes on leachates and properties of microplastics. J. Hazard Mater 413, 125342. doi:10.1016/j.jhazmat.2021.125342
Lv, W. Q., Zhou, W. Z., Lub, S. B., Huang, W. W., Yuan, Q., Tian, M. L., et al. (2019). Microplastic pollution in rice-fish co-culture system: a report of three farmland stations in Shanghai, China. Sci. Total Environ. 652, 1209–1218. doi:10.1016/j.scitotenv.2018.10.321
Lwanga, E. H., Gertsen, H., Gooren, H., Peters, P., Salanki, T., van der Ploeg, M., et al. (2017). Incorporation of microplastics from litter into burrows of Lumbricus terrestris. Environ. Pollut. 220, 523–531. doi:10.1016/j.envpol.2016.09.096
Machado, A. A. D., Kloas, W., Zarfl, C., Hempel, S., and Rillig, M. C. (2018a). Microplastics as an emerging threat to terrestrial ecosystems. Glob. Change Biol. 24, 1405–1416. doi:10.1111/gcb.14020
Machado, A. A. D., Lau, C. W., Till, J., Kloas, W., Lehmann, A., Becker, R., et al. (2018b). Impacts of microplastics on the soil biophysical environment. Environ. Sci. Technol. 52, 9656–9665. doi:10.1021/acs.est.8b02212
Mato, Y., Isobe, T., Takada, H., Kanehiro, H., Ohtake, C., and Kaminuma, T. (2001). Plastic resin pellets as a transport medium for toxic chemicals in the marine environment. Environ. Sci. Technol. 35, 318–324. doi:10.1021/es0010498
Mizuguchi, H., Takeda, H., Kinoshita, K., Takeuchi, M., Takayanagi, T., Teramae, N., et al. (2023). Direct analysis of airborne microplastics collected on quartz filters by pyrolysis-gas chromatography/mass spectrometry. J. Anal. Appl. Pyrolysis 171, 105946. doi:10.1016/j.jaap.2023.105946
Murphy, F., Ewins, C., Carbonnier, F., and Quinn, B. (2016). Wastewater treatment works (WwTW) as a source of microplastics in the aquatic environment. Environ. Sci. Technol. 50 (11), 5800–5808. doi:10.1021/acs.est.5b05416
Nizzetto, L., Futter, M., and Langaas, S. (2016). Are agricultural soils dumps for microplastics of urban origin? Environ. Sci. Technol. 20, 10777–10779. doi:10.1021/acs.est.6b04140
Ouyang, W., Wang, Y. D., Lin, C. Y., He, M. C., Hao, F. H., Liu, H. B., et al. (2018). Heavy metal loss from agricultural watershed to aquatic system: a scientometrics review. Sci. Total Environ. s637, 208–220. doi:10.1016/j.scitotenv.2018.04.434
Paul, A., Wander, L., Becker, R., Goedecke, C., and Braun, U. (2019). High-throughput NIR spectroscopic (NIRS) detection of microplastics in soil. Environ. Sci. Pollut. Res. Int. 26, 7364–7374. doi:10.1007/s11356-018-2180-2
Piehl, S., Leibner, A., Löder, M. G. J., Dris, R., Bogner, C., and Laforsch, C. (2018). Identification and quantification of macro-and microplastics on an agricultural farmland. Sci. Rep. 8, 17950. doi:10.1038/s41598-018-36172-y
Rezaei, M., Riksen, MJPM, Sirjani, E., Sameni, A., and Geissen, V. (2019). Wind erosion as a driver for transport of light density microplastics. Sci. Total Environ. 669, 273–281. doi:10.1016/j.scitotenv.2019.02.382
Rillig, M. C. (2012). Microplastic in terrestrial ecosystems and the soil? Environ. Sci. Technol. 46, 6453–6454. doi:10.1021/es302011r
Rillig, M. C., Leifheit, E., and Lehmann, J. (2021). Microplastic effects on carbon cycling processes in soils. Plos Biol. 19, 3001130. doi:10.1371/journal.pbio.3001130
Rocha-Santos, T., and Duarte, A. C. (2015). A critical overview of the analytical approaches to the occurrence, the fate and the behavior of microplastics in the environment. Trac. Trends Anal. Chem. 65, 47–53. doi:10.1016/j.trac.2014.10.011
Rolsky, C., Kelkar, V., Driver, E., and Halden, R. U. (2020). Municipal sewage sludge as a source of microplastics in the environment. Curr. Opin. Environ. Sustain 14, 16–22. doi:10.1016/j.coesh.2019.12.001
Rong, L. L., Zhao, L. F., Zhao, L. C., Cheng, Z. P., Yao, Y. M., Yuan, C. L., et al. (2021). LDPE microplastics affect soil microbial communities and nitrogen cycling. Sci. Total Environ. 773, 145640. doi:10.1016/j.scitotenv.2021.145640
Rujnic-Sokele, M., and Pilipovic, A. (2017). Challenges and opportunities of biodegradable plastics: a mini review. Waste Manag. Res. 35, 132–140. doi:10.1177/0734242X16683272
Scheurer, M., and Bigalke, M. (2018). Microplastics in Swiss floodplain soils. Environ. Sci. Technol. 52, 3591–3598. doi:10.1021/acs.est.7b06003
Selonen, S., Dolar, A., Kokalj, A. J., Skalar, T., Dolcet, L. P., Hurley, R., et al. (2020). Exploring the impacts of plastics in soil - the effects of polyester textile fibers on soil invertebrates. Environ. Sci. Technol. 700, 134451. doi:10.1016/j.scitotenv.2019.134451
Shan, J. J., Zhao, J. B., Liu, L. F., Zhang, Y. T., Wang, X., and Wu, F. C. (2018). A novel way to rapidly monitor microplastics in soil by hyperspectral imaging technology and chemometrics. Environ. Pollut. 238, 121–129. doi:10.1016/j.envpol.2018.03.026
Shim, W. J., Song, Y. K., Hong, S. H., and Jang, M. (2016). Identification and quantification of microplastics using Nile red staining. Mar. Pollut. Bull. 113, 469–476. doi:10.1016/j.marpolbul.2016.10.049
Steinmetz, Z., Wollmann, C., Schaefer, M., Buchmann, C., David, J., Troeger, J., et al. (2016). Plastic mulching in agriculture. Trading short-term agronomic benefits for long-term soil degradation? Sci. Total Environ. 550, 690–705. doi:10.1016/j.scitotenv.2016.01.153
Su, Y. M., Ashworth, V., Kim, C., Adeleye, A. S., Rolshausen, P., Roper, C., et al. (2019). Delivery, uptake, fate, and transport of engineered nanoparticles in plants: a critical review and data analysis. Environ. Sci. Nano 6, 2311–2331. doi:10.1039/C9EN00461K
Talbot, R., and Chang, H. (2022). Microplastics in freshwater: a global review of factors affecting spatial and temporal variations. Environ. Pollut. 292, 118393. doi:10.1016/j.envpol.2021.118393
Thakuria, D., Schmidt, O., Finan, D., Egan, D., and Doohan, F. M. (2010). Gut wall bacteria of earthworms: a natural selection process. ISME J. 4, 357–366. doi:10.1038/ismej.2009.124
Thompson, R. C., Olsen, Y., Mitchell, R. P., Davis, A., Rowland, S. J., John, A. W. G., et al. (2004). Lost at sea: where is all the plastic? Science 304, 838. doi:10.1126/science.1094559
Uheida, A., Mejía, H. G., Abdel-Rehim, M., Hamd, W., and Dutta, J. (2020). Visible light photocatalytic degradation of polypropylene microplastics in a continuous water flow system. J. Hazard Mater 406, 124299. doi:10.1016/j.jhazmat.2020.124299
United Nations Environment Programme (2010). Unep yearbook 2010: new science and progress in our changing environment. Beijing: China Environmental Science Press.
Urbanek, A. K., Rybak, J., Wróbel, M., Leluk, K., and Mirończuk, A. M. (2020). A comprehensive assessment of microbiome diversity in Tenebrio molitor fed with polystyrene waste. Environ. Pollut. 262, 114281. doi:10.1016/j.envpol.2020.114281
Velasco, A. N., Gentile, S. R., Zimmermann, S., and Stoll, S. (2022). Contamination and removal efficiency of microplastics and synthetic fibres in a conventional drinking water treatment plant. Front. Water 4, 835451. doi:10.3389/frwa.2022.835451
Wang, F., Wang, Q., Adams, C. A., Sun, Y., and Zhang, S. (2022). Effects of microplastics on soil properties: current knowledge and future perspectives. J. Hazard Mater 424, 127531. doi:10.1016/j.jhazmat.2021.127531
Wang, H. T., Ding, J., Xiong, C., Zhu, D., Li, G., Jia, X. Y., et al. (2019). Exposure to microplastics lowers arsenic accumulation and alters gut bacterial communities of earthworm Metaphire californica. Environ. Pollut. 251, 110–116. doi:10.1016/j.envpol.2019.04.054
Wang, Y., Ding, K. Q., Ren, L. X., Peng, A. P., and Zhou, S. D. (2022). Biodegradable microplastics: a review on the interaction with pollutants and influence to organisms. Bull. Environ. Contam. Toxicol. 108, 1006–1012. doi:10.1007/s00128-022-03486-7
Wei, X. F., Bohlén, M., Lindblad, C., Hedenqvist, M., and Hakonen, A. (2021). Microplastics generated from a biodegradable plastic in freshwater and seawater. Water Res. 198, 117123. doi:10.1016/j.watres.2021.117123
Witzig, C. S., Foldi, C., Worle, K., Habermehl, P., Pittroff, M., Muller, Y. K., et al. (2020). When good intentions go bad-false positive microplastic detection caused by disposable gloves. Environ. Sci. Technol. 54, 12164–12172. doi:10.1021/acs.est.0c03742
Woo, H., Seo, K., Choi, Y., Kim, J., Tanaka, M., Lee, K., et al. (2021). Methods of analyzing microsized plastics in the environment. Appl. Sci. 11, 10640. doi:10.3390/app112210640
Xie, P. (2015). Study of international anticancer research trends via co-word and document co-citation visualization analysis. J. Sci. 105, 611–622. doi:10.1007/s11192-015-1689-0
Yang, H., Yumeng, Y., Yu, Y., Yinglin, H., Fu, B., and Wang, J. (2022). Distribution, sources, migration, influence and analytical methods of microplastics in soil ecosystems. Ecotoxicol. Environ. Saf. 243, 114009. doi:10.1016/j.ecoenv.2022.114009
Yao, S., Ni, N., Li, X. N., Wang, N., Bian, Y. G., Jiang, X., et al. (2023). Interactions between white and black carbon in water: a case study of concurrent aging of microplastics and biochar. Water Res. 238, 120006. doi:10.1016/j.watres.2023.120006
Zeb, A., Liu, W., Shi, R. Y., Lian, Y. H., Wang, Q., Tang, J. C., et al. (2022). Evaluating the knowledge structure of micro- and nanoplastics in terrestrial environment through scientometric assessment. Appl. Soil Ecol. 177, 104507. doi:10.1016/j.apsoil.2022.104507
Zhang, B., Yang, X., Chen, L., Chao, J. Y., Teng, J., and Wang, Q. (2020). Microplastics in soils: a review of possible sources, analytical methods and ecological impacts. J. Chem. Technol. Biotechnol. 95, 2052–2068. doi:10.1002/jctb.6334
Zhang, G. S., and Liu, Y. F. (2018). The distribution of microplastics in soil aggregate fractions in southwestern China. Appl. Soil Ecol. 642, 12–20. doi:10.1016/j.scitotenv.2018.06.004
Zhang, Q., Zhao, Y., Du, F., Cai, H., Wang, G., and Shi, H. (2020). Microplastic fallout in different indoor environments. Environ. Sci. Technol. 54, 6530–6539. doi:10.1021/acs.est.0c00087
Zhang, S. L., Yang, X. M., Gertsen, H., Peters, P., Salanki, T., and Geissen, V. (2018). A simple method for the extraction and identification of light density microplastics from soil. Sci. Total Environ. 616, 1056–1065. doi:10.1016/j.scitotenv.2017.10.213
Zhang, T., Jiang, B., Xing, Y., Ya, H., Lv, M. J., and Wang, X. (2022). Current status of microplastics pollution in the aquatic environment, interaction with other pollutants, and effects on aquatic organisms. Environ. Sci. Pollut. Res. Int. 29, 16830–16859. doi:10.1007/s11356-022-18504-8
Zhou, C., Bi, R., Su, C., Liu, W. H., and Wang, T. Y. (2022). The emerging issue of microplastics in marine environment: a bibliometric analysis from 2004 to 2020. Mar. Pollut. Bull. 179, 113712. doi:10.1016/j.marpolbul.2022.113712
Zhou, Q., Zhang, H. B., Fu, C. C., Zhou, Y., Dai, Z. F., Li, Y., et al. (2018). The distribution and morphology of microplastics in coastal soils adjacent to the Bohai Sea and the Yellow Sea. Geoderma 322, 201–208. doi:10.1016/j.geoderma.2018.02.015
Zhu, B. K., Fang, Y. M., Zhu, D., Christie, P., Ke, X., and Zhu, Y. G. (2018). Exposure to nanoplastics disturbs the gut microbiome in the soil oligochaete Enchytraeus crypticus. Environ. Pollut. 239, 408–415. doi:10.1016/j.envpol.2018.04.017
Zhu, D., Chen, Q. L., An, X. L., Yang, X. R., Christie, P., Ke, X., et al. (2018). Corrigendum to “Exposure of soil collembolans to microplastics perturbs their gut microbiota and alters their isotopic composition” [Soil Biology and Biochemistry 116, 302-310]. Soil Biol. Biochem. 124, 277–278. doi:10.1016/j.soilbio.2018.05.031
Keywords: bibliometric analysis, Citespace, microplastics, soil, visualized analysis
Citation: Miao J, Huang W, Pan R and Zhou K (2023) Research progress and hotspot analysis of soil microplastics: a bibliometrics-based review. Front. Environ. Sci. 11:1297646. doi: 10.3389/fenvs.2023.1297646
Received: 20 September 2023; Accepted: 17 November 2023;
Published: 30 November 2023.
Edited by:
Jose Antonio Rodriguez Martin, Instituto Nacional de Investigación y Tecnología Agroalimentaria (INIA), SpainReviewed by:
Zacharias Steinmetz, RPTU Kaiserslautern-Landau, GermanyFrancoise Watteau, Université de Lorraine, France
Copyright © 2023 Miao, Huang, Pan and Zhou. This is an open-access article distributed under the terms of the Creative Commons Attribution License (CC BY). The use, distribution or reproduction in other forums is permitted, provided the original author(s) and the copyright owner(s) are credited and that the original publication in this journal is cited, in accordance with accepted academic practice. No use, distribution or reproduction is permitted which does not comply with these terms.
*Correspondence: Kai Zhou, zhou.kai@prcee.org