Research trends in the use of secondary batteries for energy storage
- 1Facultad de Ingenierías, Instituto Tecnológico Metropolitano, Medellín, Colombia
- 2Escuela de Ingenieria Industrial, Universidad Señor de Sipán, Chiclayo, Peru
- 3Instituto de Investigación y Estudios de la Mujer, Universidad Ricardo Palma, Lima, Peru
- 4Escuela Profesional de Ingeniería Mecánica Eléctrica, Universidad Señor de Sipán, Chiclayo, Peru
- 5Escuela de posgrado, Universidad Señor de Sipán, Chiclayo, Peru
Introduction: This study addresses the use of secondary batteries for energy storage, which is essential for a sustainable energy matrix. However, despite its importance, there are still important gaps in the scientific literature. Therefore, the objective is to examine the research trends on the use of secondary batteries for energy storage and to assess their development and direction.
Methods: A bibliometric analysis is used, following the PRISMA-2020 guidelines for the analysis of secondary sources. 98 studies were identified and analyzed in the bibliometric evaluation category. Although this is a review of different research documents and different types of batteries are addressed, the study focuses mainly on the identification of the different existing trends in the use of second-use batteries for energy storage.
Results: The study’s findings show a significant increase in interest, particularly in 2023, 2022, and 2021. Key researchers such as Zakeri and Kulcinski, as well as journals like Energy Conversion and Management, have made significant contributions. The significance of the topic is highlighted by the annual production of articles. The leading research in this field comes from the United States and China, with a shift in focus towards specific areas such as thermal assessment and storage. Thematic clusters include life cycle analysis, thermal storage, and lithium-ion batteries. Emerging terms such as ‘smart grid’ and ‘exergy’ indicate a growing interest.
Discussion: Understanding trends in battery research is crucial for developing sustainable energy solutions and addressing global energy challenges.
1 Introduction
The transition to a more efficient and sustainable energy matrix requires energy storage as a fundamental element. The use of rechargeable batteries in this situation has gained increasing attention as a promising method to increase battery life and reduce their environmental impact (Koese et al., 2023). Originally used in electric cars or energy storage systems, these batteries have been taken out of service due to a reduction in their storage capacity, but still have a significant amount of capacity remaining that can be applied to stationary storage applications. To advance in the study, one of the tools to be carried out is a review of the body of literature in the field of science that deals with the use of used batteries as a form of energy storage. In particular, the analysis will focus on studies that discuss the technical, economic and environmental aspects of these emerging technologies. They will be able to identify the trends and improvements in the use of these batteries, as well as the opportunities and challenges associated with their implementation in mass energy storage systems, through an analysis of the scientific literature (Dai et al., 2022).
In one study, vanadium flow batteries and lithium-ion batteries for energy storage were each subjected to a social life cycle assessment (Koese et al., 2023). Making informed decisions about the deployment of energy storage technologies requires this type of analysis to understand the environmental and societal impacts of these technologies. On the other hand. An economic analysis of energy market transactions using a life-cycle cost (Dai et al., 2022). Their findings provide insightful data on the economic viability of used batteries compared to other storage options. In addition, research to extend the life of conventional batteries in electric vehicles will combine supercapacitors and conventional batteries in a hybrid energy storage system to be developed (Hsieh et al., 2021). By significantly increasing battery efficiency and longevity, this type of creative thinking can help reduce maintenance costs and waste production. Finally, differential power control of modules in energy storage systems with used batteries will be investigated (Liu et al., 2020). The results are relevant for managing and optimizing the performance of these batteries in stationary applications and renewable energy research systems.
The creation of more efficient and environmentally friendly energy systems is highly dependent on energy storage. This situation has led to the use of used batteries as a promising tactic to extend battery life and improve battery performance in energy storage applications. The reuse of batteries that have been removed from electric vehicles or other devices offers a particular opportunity to reduce e-waste and other environmental impacts associated with the extraction of materials needed to make new batteries (Hsieh et al., 2021). Because of its potential to provide a viable economic and environmental solution, scientific research on the use of used batteries for energy storage has gained momentum. Economic and environmental lifecycle studies have shed light on the benefits and difficulties of using these batteries in energy storage systems. To determine the viability of various storage technologies, including new and second-use batteries, in electricity markets, they conducted an economic analysis of their life cycles. Their study results show how competitive second-use batteries are and how they can provide various flexibility services in the energy market (Tang and Wang, 2023).
The environmental impact of secondary batteries compared to other storage technologies has been better understood through environmental analysis and life cycle assessment, as well as the economic advantage. Life cycle studies have considered a variety of factors, including resource use, greenhouse gas emissions, and waste generation. For example, a life cycle assessment of vanadium flow batteries and renewable energy storage systems based on lithium-ion batteries was conducted (da Silva Lima et al., 2021). His research helped to develop a deeper understanding of the environmental impacts of secondary batteries and identified areas for performance improvement. In this sense, the toxic compounds in high demand for metals and fossil fuels generated by this type of batteries carry greater negative effects (da Silva Lima et al., 2021).
In summary, there are a number of advantages to using used batteries as energy storage devices that support their importance in the study and creation of sustainable energy systems. These batteries create new opportunities for a more effective and greener energy transition due to their potential to reduce ewaste generation, economic viability, and reduced environmental impact compared to new-use alternatives. The informed adoption of these technologies and the promotion of their incorporation into large-scale energy storage systems have benefited greatly from scientific studies and life cycle assessments (Karasu and Dincer, 2020; Colelli et al., 2022).
The lack of comprehensive studies that fully evaluate the life cycle of these technologies represents one of the major research gaps in the use of secondary batteries for energy storage. There are still opportunities to conduct more comprehensive and comparative analyses of their performance in different applications, although research has been conducted that addresses technical, economic, and environmental aspects (Hayatina et al., 2023). A review of the life cycle assessment of thermal energy storage in building applications is conducted, showing that this topic has received some attention. Comparative studies between different energy storage technologies, including new and used batteries, are another important gap in research in this area. Tidal current energy harvesting technologies are reviewed and a life cycle assessment is performed, but a detailed analysis of how second-use batteries compare to other storage alternatives in terms of efficiency, cost, and sustainability is lacking (Li and Zhu, 2023). Research that examines this comparison across different application contexts and electricity systems would be useful.
In addition, although there are studies examining the application of iron-air batteries in energy storage, there is still a lack of dedicated research on the optimization of secondary batteries to maximize their lifetime and performance in large-scale storage systems (McKerracher et al., 2015). The discovery of techniques and technologies to extend the life of these batteries and increase their efficiency would significantly contribute to their wider adoption in the field of energy storage, as these batteries have been taken out of service due to loss of capacity. Therefore, the objective is to study second-use batteries in order to structure a research agenda that will allow the direction of future research, for which the following research questions are also posed:
RQ1: In which years has the interest in secondary batteries increased?
RQ2: What is the growth rate of scientific articles on secondary batteries?
RQ3: What are the main research references on secondary batteries?
RQ4: What is the thematic evolution derived from the scientific production on second-use batteries?
RQ5: What are the main thematic clusters on used batteries?
RQ6: What are the growing and emerging keywords in the field of second-use battery research?
RQ7: Which topics are positioned as protagonists for the design of a research agenda on second-use batteries?
2 Materials and methods
In the current study, a structured review of the literature on the use of second-use batteries for energy storage was conducted using an exploratory research approach. The methodology was based on a quantitative review of the bibliography, following the guidelines set out in the PRISMA-2020 statement (Page et al., 2021). From secondary research sources, relevant studies related to the topic of interest were identified and evaluated. This method allowed for a global and detailed vision of the directions, developments and research gaps in the field of study, establishing the basis for a rigorous and essential systematic review.
2.1 Inclusion criteria
Within the framework of this bibliometric research focused on the use of used batteries for energy storage, inclusion criteria have been precisely defined to ensure the relevance and completeness of the search. First of all, the titles and keywords are considered as the main metadata to decide the inclusion of the records. In the same way, documents that contain the combination of related terms, such as “energy harvesting,” “energy storage” and “energy storage system,” along with their various synonyms and references relevant to organizations in the field of energy, will be retained.
In order to carry out this bibliometric evaluation, three stages of exclusion have been established in order to guarantee the quality and relevance of the selected records. In the first stage, documents with erroneous indexing are eliminated, which makes it possible to discard inappropriate records that may not be related to the topic of interest. In the second stage, documents without access to the full text are discarded. However, it is important to note that this stage is only applied in the case of systematic literature reviews, since only the available metadata are analyzed in the bibliometric evaluation. Finally, in the third stage of exclusion, conference proceedings, articles with incomplete indexing and texts that do not contribute to the research on the use of used batteries for energy storage will be eliminated. These stages of exclusion will be carried out in a thorough and transparent manner to ensure the quality and accuracy of the results of the bibliometric evaluation.
2.2 Source of information
In this bibliometric evaluation focused on the use of secondary batteries for energy storage, it was decided to choose the Scopus and Web of Science databases, as they are considered the main sources of scientific information today. These databases are recognized for their ability to effectively retrieve and analyze scientific information. They offer extensive coverage of scientific journals, conferences, patents, and other types of academic papers, making them reliable and comprehensive sources for tracking research in various fields of knowledge. Additionally, as they are international and multidisciplinary databases, it is possible to find research related to second-use batteries in different contexts and applications in different countries, which makes them a valuable input for the development of this research. The relevance of using these databases in the field of education is emphasized, since Web of Science and Scopus play a fundamental role in proposing research directions in the context of teaching school history from a contemporary perspective (Guzmán Contreras, 2023).
2.3 Search strategy
In order to carry out an effective search in the selected databases, Scopus and Web of Science, two specialized search equations were developed, adapted both to the inclusion criteria outlined for the bibliometric evaluation and to the search characteristics inherent to each platform. These equations were carefully designed to achieve accurate identification of documents related to the use of used batteries for energy storage. In the search equation for Scopus, keywords related to “energy harvesting,” “energy storage” and “energy storage system” were used, along with phrases related to “used batteries” and “life cycle.” Similarly, the search formulation developed for Web of Science used similar key terms to ensure the completeness of the search in both databases. These specific search formulations allow to obtain a complete and relevant collection of scientific literature on the subject, which is the basis for a solid and well-founded bibliometric evaluation.
For the Scopus database: (TITLE (“energy harvest*” OR “energy storage*” OR “energy storage system*”) AND TITLE (“second life batter*” OR “second life battery*” OR “secondary battery *” OR “secondary battery*” OR “life cycle”)).
For the Web of Science database: (TI= (“energy harvest*” OR “energy storage *” OR “energy storage system*”) AND TI= (“second life batte*” OR “second life battery*” OR “second life battery*” OR “secondary battery*” OR OR “life cycle”)).
2.4 Data management
In order to carry out this bibliometric evaluation on the use of secondary batteries for energy storage, the Microsoft Excel® platform was used to extract, store and process the information derived from each of the selected databases. Microsoft Excel® was chosen to organize the bibliographic data resulting from the research in Scopus and Web of Science, allowing an efficient management of the collected data and facilitating its subsequent analysis and processing. In addition, the free software VOSviewer® in combination with Microsoft Excel® was used for the analysis and visualization of the bibliometric data. VOSviewer® is a widely recognized and powerful tool for network analysis and visualization of bibliometric data (Hirawan et al., 2022). Using VOSviewer®, it was possible to perform a co-citation analysis and visualize the connections between the most influential documents and authors in the field of energy storage using used batteries. This tool, combined with Microsoft Excel®, made it possible to generate graphs and visual representations of various bibliometric indicators, thus facilitating the interpretation and communication of the results in a clear and effective manner.
The choice of Microsoft Excel® for data management and VOSviewer® for the analysis and visualization of the information was essential in order to carry out a solid and well-founded bibliometric evaluation on the topic of interest. This was done in accordance with methodological standards and with a guarantee of precision in the presentation of the results. Through this combination of tools, a comprehensive and detailed analysis of research trends and patterns related to the application of used batteries in energy storage was achieved.
2.5 Selection process
According to the guideline established in the PRISMA 2020 statement, as demonstrated in the study by (12), it is very important that researchers declare whether they used an internal automated classification system to support the study selection phase, as well as whether they carried out an internal or external validation to assess the risk of study omission or misclassification in the bibliometric analysis. This transparency is an essential element in ensuring the integrity and reliability of the literature review process in the field of bibliometrics.
In the context of the present study on the use of rechargeable batteries for energy storage, the automation capabilities of Microsoft Excel® were used as an internal tool to facilitate the study selection process. This tool was designed jointly by all the researchers involved in the study and was used independently by each of them to apply the inclusion and exclusion criteria to the documents identified in the Scopus and Web of. Science. This automated approach helped to reduce the risk of overlooking studies or making incorrect classifications, while at the same time allowing the convergence of results by establishing a uniform and meticulous process in the selection of documents destined for bibliometric analysis.
2.6 Data collection process
In accordance with the guidelines established by (Page et al., 2021) to carry out a bibliometric analysis, it is essential to detail the methods used to collect data from the reports, including aspects related to the process of collecting, validating and confirming the information. In this study on the use of rechargeable batteries for energy storage, the automated tool Microsoft Excel® was used to collect data from the reports obtained from the two selected databases. Scopus and Web of Science. This tool simplified the structuring and organization of the bibliographic data, which allowed an efficient management of the records derived from the bibliometric search.
Similarly, in the data validation process, each of the authors of this study performed the role of reviewer independently. Each author reviewed the records obtained and applied the pre-defined inclusion and exclusion criteria for the bibliometric analysis. This methodology guarantees an exhaustive and rigorous review of the documents to ensure the quality and accuracy of the data collected.
In addition, a collaborative data confirmation process involving all study authors was implemented. During this phase, each author’s individual results were submitted for discussion and review until full consensus was reached on the data collected. This confirmation phase confirmed the coherence and uniformity of the data collection, thus strengthening the validity and reliability of the results obtained in the bibliometric analysis.
2.7 Data elements
For the study in question, which deals with the use of rechargeable batteries for energy storage, searches were carried out with the aim of collecting results corresponding to all those articles that were in line with the research objectives. This meant that a specific search selection had to be carried out for each of the databases in order to identify the articles that dealt specifically with the topic of interest. All articles that were consistent with the defined scope of results were included, considering all relevant measures, time points, and analyses. However, if incomplete or unclear information is found, these texts will be excluded under the category of “non-relevant texts.” The purpose of this exclusion is to maintain the coherence and focus of the study in accordance with the objectives and scope of the research.
2.8 Assessment of the risk of bias of the study
In the context of the current bibliometric research on the use of secondary batteries for energy storage, special emphasis was placed on the assessment of the risk of bias present in the included studies. For this purpose, as in the data collection process, all study authors independently participated in the bias assessment. Using the same automated Microsoft Excel® tool that was used for data collection, an appropriate assessment was performed to ensure the quality and integrity of the identified results. The assessment of bias was performed carefully, taking into account the specifics of each study included in the analysis.
2.9 Effect measures
In carrying out this bibliometric evaluation focused on the use of used batteries for energy storage, it is necessary to point out that, despite the fact that primary research uses effect measures such as risk ratio or difference of means, in this research based on secondary research sources, different indicators have been implemented for the synthesis and presentation of results. Specifically, the number of publications and the number of citations were examined as indicators to assess the relevance and influence of studies in the field of used battery energy storage. In addition, the temporal pattern of the use of each keyword was examined to identify trends and variations in interest in the topic over time. Microsoft Excel® was used to carry out these analyses, allowing the bibliographic data to be monitored and properly processed.
Likewise, the VOSviewer® tool was used to determine the thematic links between the documents by visualizing the nodes present in the knowledge network. This facilitated the identification of the most relevant topics and the grouping of related research into specific areas of study. In this way, the bibliometric evaluation provides a comprehensive and detailed perspective of the research landscape related to the use of used batteries for energy storage, through the analysis of bibliometric indicators and the use of advanced visualization techniques.
2.10 Synthesis methods
In carrying out the current bibliometric evaluation on the use of used batteries for energy storage, careful procedures will be followed to determine the suitability of the studies in each synthesis phase. In order to visually present the results of the studies and of the individual syntheses, tabular and graphical presentation methods were used, which allowed a clear and understandable presentation of the bibliometric data obtained. These procedures were carried out through the use of automated tools, in particular Microsoft Excel®, which used bibliometric indicators of quantity, quality and structure (Durieux and Gevenois, 2010), applied to all those documents that passed the three exclusion phases. Established in the research process.
2.11 Assessment of reporting bias
In the context of this bibliometric evaluation focused on the use of rechargeable batteries for energy storage, it is essential to address the risk of bias that may arise due to the lack of results in the synthesis, often due to reporting bias. It has been observed that there is a bias towards certain synonyms present in thesauri such as the IEEE, which is reflected in the inclusion criteria, in the search strategy and in the data collection itself. This bias could lead to the exclusion of some relevant documents from the analysis, as certain terms may be more common or preferred in the scientific literature, while other less used synonyms may go unnoticed.
In addition, the exclusion of conference proceedings, articles with incomplete indexing, and nonrelevant texts in the exclusion criteria could result in the omission of valuable information and limit the comprehensive construction of knowledge on the topic. It is critical to consider these potential limitations and to take steps to mitigate the risk of bias, such as a thorough review of search terms and consideration of different sources of scientific literature, in order to obtain a more comprehensive and balanced view of the field of study.
2.12 Assessment of certainty
In the context of this bibliometric evaluation focused on the use of used batteries for energy storage, it is crucial to examine the certainty or confidence in the body of evidence obtained for each result. In contrast to primary studies, which focus on the assessment of a specific certainty, it was decided in this bibliometric review to carry out a general assessment of certainty. This approximation was achieved through the independent and rigorous application of the inclusion and exclusion criteria in the selection of the documents to be analyzed. In addition, bibliometric indicators were defined to allow a quantitative and objective assessment of the body of evidence.
At the same time, special attention was paid to the identification of possible limitations, which were predefined in the methodological design of the study, guaranteeing the transparency and reliability of the process. Similarly, in the discussion phase, the weaknesses of the analysis were addressed, recognizing and contextualizing the possible limitations and weaknesses of the analysis carried out. Through this methodology, a rigorous and essential assessment of the certainty of the body of evidence collected in this bibliometric evaluation has been ensured.
At the beginning of this bibliometric analysis focused on the use of used batteries for energy storage, a detailed identification of relevant articles and documents was carried out through the implementation of search strategies designed for each of the selected information sources. In addition, a meticulous elimination of duplicates was carried out in order to ensure the coherence and completeness of the data collected. After this phase, the three stages of exclusion mentioned above were carried out, which allowed the refinement of the sample and the selection of a total of 98 articles that met the predefined inclusion criteria for this bibliometric evaluation. Finally, Figure 1 is presented, through which the PRISMA-2020 statement proposes to consider the entire methodological design.
3 Results
In the present bibliometric study, significant results were obtained in the study of the use of rechargeable batteries for energy storage. Figure 2 shows a cubic polynomial growth of approximately 98.82% in the number of publications over time. The years that stand out the most in terms of the number of publications on the subject are 2020, 2021, 2022 and 2023, which shows that there is a significant increase in interest and research in this field, indicating that the use of second-use batteries in the energy industry is increasing.
With regard to the main authors within the studies on the use of secondary batteries for energy storage, two groups have been identified, as shown in Figure 3. The first group is characterized by authors such as Zakeri, Syri, Kulcinski and Denholm, who have a significant impact in terms of citations received, indicating that their work is known and referenced by other researchers in the field; however, this group of authors shows a low scientific productivity in relation to the number of articles published.
On the contrary, the second group of authors, such as Zhang Y, Li X and Deng Y, are characterized by their high scientific productivity, although their works have not received a significant number of citations, in their works related to the field of second-generation batteries. Use for energy storage.
For this bibliometric study of the main journals publishing documents related to the use of secondary batteries for energy storage, three groups were identified, as shown in Figure 4. The first group includes journals such as Energy Conversion and Management and Journal of Energy Storage, which stand out in terms of scientific productivity and impact.
The second group consists of journals that are important for scientific production but have a lower index of scientific productivity, including Renewable and Sustainable Energy Reviews. On the other hand, the third group is mainly characterized by high scientific productivity, i.e., they present a greater number of articles on the subject, although their works have not received a significant number of citations; leading journals in this group are Energy, Energies, and the Journal of Cleaner Production.
To conclude the analysis in terms of research references, Figure 5 presents three groups of countries that are relevant in terms of scientific production on the use of secondary batteries for energy storage. The first group includes countries such as the United States, the United Kingdom and China, which stand out in terms of both scientific productivity and impact, suggesting that they have a large number of relevant and highly cited publications. The second group is made up of countries that have a large impact on the scientific literature, despite having a lower index of scientific productivity, including Finland and Sweden.
On the other hand, the third group consists of countries such as Australia and France, which are mainly characterized by high scientific productivity, since they have a greater number of publications on the subject, although their works have not received a significant number of citations.
In the present study, an analysis of the thematic evolution in the literature related to the use of secondary batteries for energy storage was carried out, as can be seen in Figure 6, where the most used keyword in each of the years of study, from the year 2000 to the present 2023, is shown. In the year 2000, as a starting point, the appearance of the life cycle concept stands out. Over the years, there has been an evolution in the topics studied, and topics such as Life Cycle Assessment and Thermal Energy Storage stand out as central research topics in recent years, reflecting current research trends in the field.
Figure 7 shows the main keyword co-occurrence network, in this bibliometric study through 9 thematic clusters. The cluster in light blue is characterized by being the most prominent, since it is composed of terms such as Life Cycle Assessment, Thermal Energy Storage, Electrochemistry and Lithium-Ion Batteries. Second is the cluster in red, which includes terms such as Capex, Life Cycle Costing, ICA, Life Cycle Analysis, Renewable Energy, and Emissions. Similarly, other clusters are identified in shades of brown, pink, dark blue, orange, purple, dark green, and lemon green, each representing different conceptual relationships.
This research proposes a new Cartesian plane, where the X-axis measures the frequency of keyword use, while the Y-axis measures the validity of their use over time, as shown in Figure 8.
This approach allows to divide the level into 4 different quadrants. In quadrant 4 are those concepts whose use has decreased over time, where some keywords are identified, such as Life Cycle Assessment. On the other hand, in quadrant 2 are those keywords that are rarely used but highly topical, becoming emerging terms in the field of study; among them are lithium-ion batteries, recycling, exergy and thermochemical energy storage, although it does not really correspond to the topic of the study.
4 Nomenclature
4.1 Resource identification initiative
Capital expenditure (CAPEX).
Energy conversion and management (ECM).
Greenhouse gas (GHG).
Life cycle analysis (LCA).
Life cycle assessment (LCA).
Thermal energy storage (TES).
Used batteries for energy storage (UBES).
5 Discussion
This section offers a detailed analysis of the results obtained in the bibliometric analysis on the UBES, here the most relevant findings are examined in depth, such as the identification of the main authors, journals and countries in the field of study, practical suggestions from the results obtained are also presented, highlighting how this research can contribute to the advancement of knowledge in the field and how it can be useful for decision-making in industry and energy policy.
It also addresses the limitations of the study and discusses how these factors may have influenced the results; it provides a classification of keywords according to their function, highlighting the most used terms and how they have evolved over time; it identifies the main research gaps in the field, highlighting the areas that require further attention in the future; and finally, it presents the main research agenda arising from the results obtained. This agenda identifies the most promising research areas and topics that can be the subject of future studies in the field of secondary batteries for energy storage.
5.1 Analysis of the growth of the scientific literature on the use of secondary batteries for energy storage
In Figure 2, a significant increase in scientific production related to the use of secondary batteries for energy storage was observed in the years 2020–2023. In 2023, an assessment of the social life cycle of vanadium flow batteries and lithium-ion batteries was carried out (Koese et al., 2023), as well as a comparison of greenhouse gas emissions in the life cycle of different energy storage technologies (Han et al., 2023), both studies contributing to the understanding of the environmental and social impacts of battery technologies used for energy storage. In 2022, a lifecycle comparison of lithium-ion batteries and lead-acid batteries for grid applications (Yudhistira et al., 2022) and a technical-economic feasibility study of a zero net emission microgrid integrating energy storage systems with secondary batteries (Bhatt et al., 2022) will provide relevant information for the selection of appropriate energy storage technologies.
In 2021, relevant information on the environmental impact and operational performance of different energy storage technologies will be provided through a life cycle assessment of renewable energy storage systems based on lithium-ion batteries and lithium-ion vanadium batteries (da Silva Lima et al., 2021) and an operational design of centralized charging stations using energy storage systems with secondary batteries (Deng et al., 2021). In 2020, a techno-economic assessment of energy storage systems using life-cycle cost and levelized cost of energy metrics (Mostafa et al., 2020) and the identification of research gaps in the life-cycle assessment of lithium-ion batteries for grid-scale energy storage systems (Pellow et al., 2020) will provide important information for understanding the cost and sustainability of energy storage technologies.
5.2 Analysis of research references on the use of secondary batteries for energy storage
Figure 3 allowed us to observe the most prominent authors in relation to the subject of study, where Zakeri and Syri are research references due to their study on electrical energy storage systems and their comparative analysis of life cycle costs (Zakeri and Syri, 2015). In their research, they provided a comprehensive view of different storage technologies, which is essential for the development of efficient and sustainable strategies in this field. Similarly, Kulcinski and Denholmp are recognized for their study on the life cycle energy demand and greenhouse gas emissions of large-scale energy storage systems (Denholm and Kulcinski, 2004). Their research will provide valuable information. To understand the environmental impact of these technologies and has been a reference in decision-making related to the implementation of energy storage systems. For his part, Zhang Y has been identified as a reference researcher thanks to his contributions in the field of design and operational planning of centralized charging stations using energy storage systems with secondary batteries (Deng et al., 2021). In another study, Zhang carried out optimal planning of the complete life cycle of energy storage systems, which is essential for improving the efficiency and sustainability of these systems (Zhang et al., 2020).
Li X has also stood out as a reference in the field of energy storage, especially in the analysis of the costs and benefits of battery storage systems, and his research on the analysis of the life cycle of innovative energy islands (Li et al., 2018) and the assessment of the sustainability of hydroelectric energy storage (Guo et al., 2020) has been fundamental for decision-making in the development of sustainable energy storage technologies. Finally, Deng Y was recognized for his research in the field of energy storage with second-use batteries and its application in centralized charging systems (Deng et al., 2021) and in the planning of integrated energy systems at the utility level (Guo et al., 2021), their studies have significantly contributed to the development of strategies for a more efficient use of second-use batteries in energy storage systems.
The journals Energy Conversion and Management, Journal of Energy Storage, and Renewable and Sustainable Energy Reviews have been fundamental in advancing knowledge on the use of second-use batteries for energy storage, as shown in Figure 4; Energy Conversion and Management has emerged as a leading journal in the field, publishing important research on life-cycle energy requirements and greenhouse gas emissions of large-scale energy storage systems (Denholm and Kulcinski, 2004) and on technical-economic feasibility studies of microgrids with second-use battery energy storage systems to achieve zero net carbon emissions (Bhatt et al., 2022). On the other hand, the Journal of Energy Storage has been an important platform for the dissemination of relevant research, such as the study evaluating the technological cost and life cycle analysis of energy storage systems (Mostafa et al., 2020). An important paper on cost optimization and life cycle analysis of SOEC-based power-to-gas systems for seasonal energy storage in distributed systems was also published in this journal (Prabhakaran et al., 2019).
As for Renewable and Sustainable Energy Reviews, it has become a valuable source for understanding the comparative analysis of the life cycle costs of electrical energy storage systems (Zakeri and Syri, 2015) and the costbenefit analysis of the life cycle of energy storage systems (Li et al., 2018). These journals have allowed the dissemination of research that has laid the foundation for the development of more efficient and sustainable energy storage technologies, which is essential for the community of scientists and professionals working in the field of energy storage. The countries that have stood out in terms of scientific production on the use of secondary batteries for energy storage have been the United States, the United Kingdom and China, which have stood out in terms of both scientific productivity and impact in the field, as shown in Figure 5.
The United States has been a prominent leader, and its contributions include research on life-cycle energy requirements and greenhouse gas emissions of large-scale energy storage systems (Denholm and Kulcinski, 2004) and research gaps in the environmental life-cycle assessment of lithium-ion batteries for energy storage systems (Pellow et al., 2020); on the other hand, the United Kingdom has conducted significant research on iron-air secondary batteries and their potential for energy storage (McKerracher et al., 2015) and life-cycle cost-benefit analysis of energy storage systems (Li et al., 2018). China also stands out on this topic with research on planning integrated energy use systems at the park level using secondary batteries (Guo et al., 2021) and assessing the sustainability of the life cycle of hydropower storage systems (Guo et al., 2020).
With respect to Finland and Sweden, they are also references in terms of scientific productivity in the field of energy storage, Finland has contributed important research on the comparative analysis of the life cycle costs of electrical energy storage systems (Zakeri and Syri, 2015), while Sweden has conducted comparative life cycle assessment studies of lithium-ion batteries and lead-acid batteries for energy storage in the grid (Yudhistira et al., 2022). These countries have significantly contributed to the development of knowledge in the field of energy storage and its role in the transition to more sustainable and efficient energy.
5.3 Analysis of the thematic evolution on the use of secondary batteries for energy storage
The analysis of the thematic evolution in Figure 6 shows that the concept of “life cycle” played a crucial role in the first years of research on the use of secondary batteries for energy storage. Research on the life-cycle energy requirements and greenhouse gas emissions of large-scale energy storage systems was a milestone in the field, as it provided a comprehensive perspective on the environmental and sustainability issues involved. For this type of technology (Denholm and Kulcinski, 2004), this life-cycle approach was able to understand the environmental and energy requirements from material extraction to final disposal, which was essential for establishing guidelines and strategies for developing a more sustainable use of secondary batteries.
While the concepts of “Life Cycle Assessment” (LCA) and “Thermal Energy Storage” (TES) have gained significant importance in the current state of the issue of using second-use batteries for energy storage, LCA has revolutionized the way the environmental impact and sustainability of energy storage systems are assessed (Ahmadi et al., 2017), by addressing the entire life cycle of batteries and their reuse in energy storage systems. In the case of energy, LCA has enabled a more holistic and accurate assessment of the associated environmental aspects, which has led to a better understanding of the challenges and opportunities to reduce the environmental footprint of batteries and improve their useful life, which in turn has stimulated research and development of more sustainable technologies in this area.
On the other hand, the concept of TES has been fundamental in advancing the efficiency and capacity of energy storage technologies, allowing the storage and controlled release of heat. TES has proven to be a valuable resource in optimizing the performance of solar energy generation systems and in the management of electricity demand (Oró et al., 2012). This has led to improvements in energy management and has opened up new possibilities for the integration of renewable energies into electricity networks, thus improving the stability and reliability of energy supply.
5.4 Analysis of thematic clusters on the use of secondary batteries for energy storage
The main keyword co-occurrence network shown in Figure 7, where the light blue cluster shows a thematic affinity between terms such as “Life Cycle Assessment” (LCA), “Thermal Energy Storage” (TES), “Electrochemistry” and “Lithium-ion batteries,” the set of concepts indicates a close relationship in the scientific literature on the use of second-use batteries for energy storage, focusing on crucial aspects such as life cycle assessment, thermal storage and lithium-ion battery technologies.
Two papers can be cited to further understand this thematic connection; the first paper presents a comparative assessment of the environmental life cycle of conventional energy storage systems and an innovative thermal storage system (David et al., 2021), while the second paper presents an environmental and economic analysis of the life cycle of a thermal energy storage system based on organic phase change materials embedded in open-cell copper foams (Venettacci et al., 2022); both studies highlight the relevance of LCA and TES in the field of energy storage, valuable information for the development of more sustainable and efficient technologies in this field.
The second most important cluster, represented by the color red, is characterized by the co-occurrence of keywords such as “Capex” (Capital Expenditure), “Life Cycle Cost,” “ICA” (Inventory Control Analysis), “Analysis of the Life Cycle,” “Renewable Energies” and “Emissions,” these concepts are related to the economic and environmental evaluation in the field of the use of energy storage with second-rate batteries. To emphasize in this cluster, relevant works can be mentioned where they focus on cost optimization and life cycle analysis of seasonal energy storage systems based on solid oxide electrolysis cells (SOEC) for Power to Gas in decentralized systems (Prabhakaran et al., 2019), and in the analysis of the economic life cycle of thermal energy storage systems using the Capex and Opex (Operating Expenditure) approach (Akbar et al., 2021), these studies have demonstrated the importance of addressing aspects of cost and environmental sustainability in the evaluation of energy storage systems, contributing significantly to the thematic evolution in this field.
5.5 Analysis of the frequency and conceptual validity of the use of second
The Cartesian plane of Figure 8 presented in the bibliometrics shows that the keyword “Life Cycle Assessment” is located in quadrant 4, indicating that its use has decreased compared to previous periods. This suggests that in the past this topic received more attention and relevance in the scientific literature on the use of secondary batteries for energy storage. To understand the historical context of this trend, an assessment of the lifecycle energy demand and greenhouse gas emissions of large-scale energy storage systems is provided (Denholm and Kulcinski, 2004), and a focus on the reuse of lithium-ion batteries from electric vehicles in energy storage systems using a lifecycle cascade (Ahmadi et al., 2017).
However, with the passage of time and due to the growth of other related research areas, the frequency of use of the term “Life Cycle Assessment” has experienced a decrease in recent literature, which could reflect a change in research focus to other relevant aspects of the subject. Quadrant 2 of the Cartesian plane presents emerging concepts that have acquired management in the scientific field on the use of second-rate batteries for energy storage, these concepts are highly valid today and are expected to play a prominent role in the future.
First, “lithium-ion batteries” are one of the main types of batteries used in energy storage applications due to their high energy density and efficiency, some studies have compared the environmental impact and life cycle of these batteries with other types of batteries, demonstrating their importance in the search for sustainable solutions for energy storage in the electrical grid and other applications (Yudhistira et al., 2022; Adeyemo et al., 2023). It also has that the concept of “thermochemical energy storage” although it does not correspond to the type of energy storage addressed in this work, has emerged as a promising option for thermal energy storage, research has analyzed the life cycle and environmental impact of thermochemical energy storage systems, highlighting its potential to contribute to energy efficiency and reduction of emissions in heating and cooling applications in buildings (Colelli et al., 2022; Hayatina et al., 2023).
Another relevant concept is that of “recycling,” which has become a major concern in the context of energy storage, with the growing use of lithium-ion batteries, it is essential to properly address waste management and recycling of these batteries to reduce environmental impact and take advantage of valuable materials, studies address the challenges and opportunities in recycling batteries and other electronic devices, highlighting the importance of the circular approach in the sustainable development of the landscape (Pellow et al., 2020; Sahajwalla and Hossain, 2023). Finally, the concept of “exergy” is relevant in evaluating the efficiency and performance of energy systems, including those that use second-use batteries for storage; a novel approach of a trigeneration system is presented that evaluates both the energy and exergy and life-cycle approaches, showing interest in improving the efficiency of energy storage systems in a broader context of sustainability (Roushenas et al., 2021). To ensure the safety of used lithium-ion batteries at the reuse site, the EPA (United States Environment Protection Agency) recommends primarily following universal waste storage standards and DOT (United States Department of Transportation) transportation standards for lithium batteries. This includes personnel handling, removing and disassembling batteries must be trained to do so. In addition, the battery terminals must be insulated with non-conductive tape, plastic bags or some other technique that maintains the separation between them. It must be guaranteed that the batteries are stored in air-conditioned places with good ventilation, separated from flammable materials, separate the batteries with irreparable damages or defective batteries in appropriate containers. It is also important to install advanced fire detection and extinguishing equipment, perform frequent visual and thermal inspections of the batteries, maintain continuous communication with fire chiefs and first responders in the area and, above all, have an evacuation and action plan in case of an emergency. The above was taken pursuant to the Bipartisan Infrastructure Act of 2021 which mandates EPA to develop best practices for the collection of batteries for recycling (United States Environment Protection Agency, 2024a).
EPA is currently developing a Report to Congress on best practices for battery collection for recycling to be released in 2024. This report will identify existing best practices, describe the current state of battery collection, and outline EPA’s next steps. Following the release of this report, EPA will seek to capture and share new and innovative best practices to complement the report governed by Section 260.43 of the Code of Federal Regulations (United States Code of Federal Regulations, 2024).
The useful life of lithium-ion batteries cannot be accurately predicted, however, 4 factors that ensure the legitimate use and reuse of lithium batteries should be considered in the recycling process (United States Environment Protection Agency, 2024b):
1. The battery provides a useful input to a product: when removed from service, it still can continue to function effectively and safely as a battery in a device or equipment that requires a battery.
2. The reused battery is a useful product: the product can be sold to a third party or used by the recycler as an active substitute for a battery he would otherwise purchase.
3. The battery is handled as a valuable commodity: between decommissioning and reuse, it is managed with proper tracking and safety procedures like newly manufactured battery inventories.
4. The reused battery does not contain hazardous components or manifest hazardous characteristics that an analog product does not have; a battery that is damaged or unsafe may be more likely to be reactive and suffer a thermal runaway than a battery in good condition and should not be reused or sold for reuse.
5.6 Classification of keywords related to the use of secondary batteries for energy storage according to their function
Table 1 has been elaborated with the purpose of carrying out an exhaustive classification of the main emerging and growing keywords related to the topic, this classification has been organized according to the variety of roles that second-use batteries play in the context of energy storage. The table makes it possible to identify and categorize the most important emerging and growing keywords, as well as to provide a clear summary of the most important characteristics and applications associated with each identified characteristic; each characteristic listed in Table 1 is presented with a brief description of its characteristics, accompanied by representative examples of applications in which this function is applied; this structure allows researchers and professionals in the field to quickly identify and understand the emerging and growing areas in which secondary batteries are demonstrating their use in energy storage.
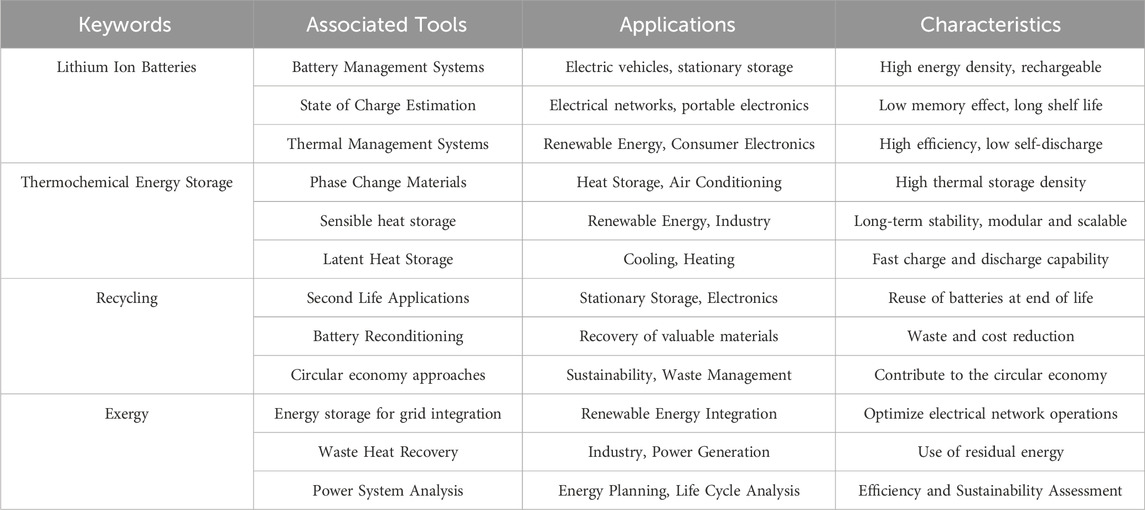
Table 1. Classification of keywords according to their function. Own elaboration from Scopus and Web of Science.
As can be seen in the table above, the classification of the main keywords according to their function is based on the concepts that were classified as emerging in relation to the use of secondary batteries, such as lithium-ion batteries, recycling, exergy and thermochemical energy storage which does not correspond to the objective of this research, however it emerges as a promising research topic in other applications, whose classification could provide a basis for the analyses and that in the future researchers can support their studies based on these key concepts.
5.7 Practical implications
This bibliometrics has yielded a number of valuable practical implications for the scientific community, industry and decision-making in the field of energy. One of the most notable findings is the conceptual transition in the thematic evolution of the studies, the migration from the traditional focus on the life cycle towards a deeper attention in the assessment of the life cycle and thermal energy storage suggests a maturity in the research, where progress is made from the general consideration of life cycles towards the specific assessment and energy efficiency in storage.
The thematic cluster analysis reveals a significant conceptual connection between key terms such as Life Cycle Assessment, Thermal Energy Storage, Electrochemistry and Lithium Ion Batteries, suggesting an increasing convergence of these fields in current research, which has direct implications for interdisciplinary collaboration, as it promotes synergy between scientific and technological disciplines to address complex challenges related to energy storage.
Looking at the frequency and trend of keywords reveals a dynamic landscape in the field, with terms such as Life Cycle Assessment declining in relevance, and emerging concepts such as Lithium Ion Batteries, Recycling and Exergy gaining prominence; such changes may influence the future direction of research and guide resource conservation, as they indicate areas of potential growth and points of critical attention in the search for more sustainable energy solutions.
5.8 Limitations
Despite the valuable perspectives obtained through the application of the PRISMA-2020 methodology in the present bibliometrics on the use of secondary batteries for energy storage, it is essential to recognize certain limitations that could influence the interpretation and generalization of the results; Firstly, the dependence on specific databases such as Scopus and Web of Science could have introduced a certain bias in the selection of publications, excluding possible contributions from sources not indexed on these platforms; in addition, the choice of tools such as Microsoft Excel® and VOSviewer® for bibliometric analysis could have limited the ability to explore more complex and subtle patterns present in the dataset.
Another important limitation lies in the nature of the bibliometric indicators used to measure and quality; although these indicators provide a quantitative and relative vision of scientific production, they do not capture the conceptual depth or innovation in the content of publications; in addition, the focus on growing and emerging keywords, while illuminating, could miss certain relevant terms that may be emerging in the literature but were not considered in the analysis.
Finally, the inherent limitations of any bibliometric study, such as the lack of quality and precision of the metadata in the databases, could have influenced the precision of the results obtained; together, these limitations suggest the importance of interpreting the results with caution and considering the context in which the bibliometrics were carried out, in order to obtain a more complete and nuanced understanding of the evolution of the field of study.
5.9 Gaps in the research
In the context of this research focused on the use of second-use batteries for energy storage, areas of knowledge have been identified that require further investigation and exploration in future research in order to fill the conceptual and technical gaps that currently exist. These research gaps, framed in a personal scientific perspective, delineate the third key points that require further attention and analysis (see Table 2).
Thus, there are a total of 4 gaps, classified into thematic, geographic, interdisciplinary and temporal gaps, which show the variety of perspectives that can be given to future studies that tend to cover existing needs and fill gaps in the field of investigation.
5.10 Research agenda
Figure 9 summarizes the design of the research agenda. This figure incorporates the central concepts identified in the research area that guide the future direction of studies on the use of secondary batteries for energy storage.
The Life Cycle Analysis (LCA) is considered as a fundamental tool to comprehensively assess the environmental impact of the technologies used in the use of batteries, the current importance of LCA lies in its ability to identify the phases of the life cycle with the greatest environmental impact, allowing for informed design and decision-making, for future research, it is essential to explore improved LCA methodologies that consider not only environmental impact, but also social and economic aspects to achieve a more comprehensive and sustainable approach.
The understanding the different negative environmental effects that can be related to secondary batteries is essential for an effective implementation, in the future it can be deepened in the evaluation of specific aspects of the environmental impacts, such as the consumption of natural resources, the generation of waste and the emission of toxic substances, in addition, it is possible to investigate how decisions on the design and operation of secondary batteries can influence their environment over time, the study of emerging technologies that reduce environmental impacts and promote sustainability can also be a relevant approach for future researchers.
Analysis of greenhouse gas (GHG) emissions associated with the use of spent batteries is critical to understanding their contribution to climate change. Future research can include more detailed studies that consider the full life cycle of batteries and their impact on GHG emissions, as well as how seconduse batteries can be integrated into energy systems in a way that reduces net emissions. The exploration of technologies and strategies that minimize GHG emissions during the production, use, and disposal of batteries will be essential to moving toward a more sustainable energy system.
Thermal energy storage (TES) is emerging as an innovative perspective for the topic at hand, and future research may require a thorough evaluation of the thermal storage capacities of these batteries and their effectiveness in specific applications, such as demand management, energy in buildings or integration with heating and cooling systems, it is also crucial to investigate how second-use batteries can be optimized to improve their TES capacity and how they can be effectively integrated into energy systems to achieve greater thermal efficiency.
Lithium-ion batteries are emerging as an essential technology for energy storage; future research could deepen the development of lithium-ion battery materials and technologies specifically adapted for their second use, taking into account factors such as long life and durability. Chemical stability, taking into account that it is crucial to study in detail the aspects of degradation and safety as these batteries move from their first use to their second use, in order to ensure optimal performance and minimize risks.
The recovery of residual heat is an emerging trend in the search for sustainable energy solutions, and it could be explored in the future how secondary batteries can contribute to the efficient recovery of residual heat generated during their operation, turning it into an additional energy source. Furthermore, it is useful to investigate how secondary batteries can be integrated into heat recovery systems in industrial and urban applications to maximize energy efficiency and reduce thermal waste.
The integration of secondary batteries into smart grids has significant potential to improve the stability and efficiency of the energy system, and future research can explore how secondary batteries can contribute to optimizing the operation of smart grids, whether in terms of load balancing, demand response, or integration of intermittent renewable energy sources, and investigate advanced control and management strategies that allow for more effective integration of these batteries into smart energy systems. These research perspectives provide a solid foundation for future studies that can expand and enrich the knowledge on the use of secondary batteries for energy storage, leading to significant advances and valuable contributions to science and technology.
Finally, although they are not included in the study because they were not found among the results of the research analyzed. Post-lithium ion batteries could also be included among the terms for future research on second-use batteries and energy storage. The above is because these batteries respond better than other types of batteries such as; lead-acid, metal hydride and nickel to properties such as energy density, hours of operation in different devices, among other aspects (Hossain et al., 2019). Similarly, there are also Na-ion batteries, which have recently attracted attention in the field of energy storage due to low cost, the use of environmentally friendly precursors and a secure supply of raw materials (Wang et al., 2024). Also those of all-solid-state batteries, the latter are already being manufactured in a Thai company due to their potential for high energy density, which they indicate could generate greater autonomy with a single charge in the automotive industry (INSIDEEVs, 2020; Bhatt et al., 2022). Finally, aqueous alkaline-ion batteries have recently generated interest due to their high energy density, high intrinsic safety, and low cost (Zhang et al., 2019).
6 Conclusion
Based on the results of the bibliometrics on the use of secondary batteries for energy storage, a deeper understanding of several key aspects has been achieved. Firstly, a growing interest in recent years, especially in 2023, 2022 and 2021, has been identified, reflecting the growing relevance and urgency of this issue. In addition, a cubic polynomial pattern is observed in the number of scientific articles. It is concluded that this increase in scientific production demonstrates the importance and potential of techniques for using secondary batteries for energy storage and addressing emerging challenges.
On the other hand, leading researchers such as Zakeri and Syri, Kulcinski and Denholm, and leading journals such as Energy Conversion and Management and the Journal of Energy Storage have consolidated their authority in the field. The United States and China are emerging as prominent leaders, underscoring the importance of global collaboration in secondary battery research.
In addition, the thematic evolution shows a shift from a life cycle focus to specific topics such as life cycle assessment and thermal energy storage. This change reflects the search for more detailed and applicable approaches as the field matures. The thematic clusters identified, such as “Life Cycle Assessment,” “Thermal Energy Storage,” “Electrochemistry,” and “Lithium-Ion Batteries,” highlight the interconnectedness of core concepts that influence a holistic understanding of this technology.
Similarly, the emergence of terms such as “lithium-ion batteries,” “recycling,” and “exergy” indicate evolving research directions that emphasize the importance of battery technology, thermal storage, and sustainability. It is concluded that this bibliometry points to a research agenda that emphasizes the deepening of concepts and the sustained exploration of emerging areas, in line with a field that continues to evolve in the search for advanced and advanced energy solutions.
Data availability statement
The data analyzed in this study is subject to the following licenses/restrictions: The data that supper the findings of this study are available from the corresponding author, upon reasonable request. Requests to access these datasets should be directed to AV-A, valenciajho@uss.edu.pe.
Author contributions
VG-P: Conceptualization, Funding acquisition, Project administration, Validation, Writing–original draft, Writing–review and editing. AV-A: Data curation, Formal Analysis, Funding acquisition, Methodology, Resources, Software, Supervision, Visualization, Writing–original draft, Writing–review and editing. EZ: Conceptualization, Validation, Writing–original draft, Writing–review and editing. GS-S: Writing–original draft, Writing–review and editing. SG-M: Writing–original draft, Writing–review and editing. LP-M: Writing–original draft, Writing–review and editing.
Funding
The author(s) declare financial support was received for the research, authorship, and/or publication of this article. The APC was funded by Universidad Señor de Sipán.
Acknowledgments
The authors thank Universidad Señor de Sipán for the support in the publication process of this work.
Conflict of interest
The authors declare that the research was conducted in the absence of any commercial or financial relationships that could be construed as a potential conflict of interest.
Publisher’s note
All claims expressed in this article are solely those of the authors and do not necessarily represent those of their affiliated organizations, or those of the publisher, the editors and the reviewers. Any product that may be evaluated in this article, or claim that may be made by its manufacturer, is not guaranteed or endorsed by the publisher.
References
Adeyemo, A. A., Alves, E., Marra, F., Brandao, D., and Tedeschi, E. (2023). Suitability assessment of highpower energy storage technologies for offshore oil and gas platforms: a life cycle cost perspective. J. Energy Storage 61, 106643. doi:10.1016/j.est.2023.106643
Ahmadi, L., Young, S. B., Fowler, M., Fraser, R. A., and Achachlouei, M. A. (2017). A cascaded life cycle: reuse of electric vehicle lithium-ion battery packs in energy storage systems. Int. J. Life Cycle Assess. 22 (1), 111–124. doi:10.1007/s11367-015-0959-7
Akbar, A., Majid, MABA, and Mokhtar, A. A. (2021). Life cycle costing of thermal energy storage system using CAPEX and OPEX. J. Mech. Sci. Technol. 35 (7), 3223–3229. doi:10.1007/s12206-021-0642-9
Bhatt, A., Ongsakul, W., and Madhu, M. N. (2022). Optimal techno-economic feasibility study of net-zero carbon emission microgrid integrating second-life battery energy storage system. Energy Convers. Manag. 266, 115825. doi:10.1016/j.enconman.2022.115825
Colelli, G., Chacartegui, R., Ortiz, C., Carro, A., Arena, A. P., and Verda, V. (2022). Life cycle and environmental assessment of calcium looping (CaL) in solar thermochemical energy storage. Energy Convers. Manag. 257, 115428. doi:10.1016/j.enconman.2022.115428
Dai, S., Ye, Z., Wei, W., Wang, Y., and Jiang, F. (2022). Economic analysis of transactions in the energy storage power market: a life-cycle cost approach. Front. Energy Res. 10. doi:10.3389/fenrg.2022.845916
da Silva Lima, L., Quartier, M., Buchmayr, A., Sanjuan-Delmás, D., Laget, H., Corbisier, D., et al. (2021). Life cycle assessment of lithium-ion batteries and vanadium redox flow batteries-based renewable energy storage systems. Sustain. Energy Technol. Assessments 46, 101286. doi:10.1016/j.seta.2021.101286
David, B. R., Spencer, S., Miller, J., Almahmoud, S., and Jouhara, H. (2021). Comparative environmental life cycle assessment of conventional energy storage system and innovative thermal energy storage system. Int. J. Thermofluids 12, 100116. doi:10.1016/j.ijft.2021.100116
Deng, Y., Zhang, Y., Luo, F., and Mu, Y. (2021). Operational planning of centralized charging stations utilizing second-life battery energy storage systems. IEEE Trans. Sustain Energy 12 (1), 387–399. doi:10.1109/tste.2020.3001015
Denholm, P., and Kulcinski, G. L. (2004). Life cycle energy requirements and greenhouse gas emissions from large scale energy storage systems. Energy Convers. Manag. 45 (13–14), 2153–2172. doi:10.1016/j.enconman.2003.10.014
Durieux, V., and Gevenois, P. A. (2010). Bibliometric indicators: quality measurements of scientific publication. Radiology 255 (2), 342–351. doi:10.1148/radiol.09090626
Guo, M., Mu, Y., Jia, H., Deng, Y., Xu, X., and Yu, X. (2021). Electric/thermal hybrid energy storage planning for park-level integrated energy systems with second-life battery utilization. Adv. Appl. Energy 4, 100064. doi:10.1016/j.adapen.2021.100064
Guo, Z., Ge, S., Yao, X., Li, H., and Li, X. (2020). Life cycle sustainability assessment of pumped hydro energy storage. Int. J. Energy Res. 44 (1), 192–204. doi:10.1002/er.4890
Guzmán Contreras, P. (2023). Enseñanza de la disciplina histórica escolar desde un enfoque contemporáneo: una propuesta de agendas investigativas desde la Web of Science y Scopus. RIDE Rev. Iberoam. Investig. el Desarro. Educ. 13 (26). doi:10.23913/ride.v13i26.1398
Han, X., Li, Y., Nie, L., Huang, X., Deng, Y., Yan, J., et al. (2023). Comparative life cycle greenhouse gas emissions assessment of battery energy storage technologies for grid applications. J. Clean. Prod. 392, 136251. doi:10.1016/j.jclepro.2023.136251
Hayatina, I., Auckaili, A., and Farid, M. (2023). Review on the life cycle assessment of thermal energy storage used in building applications. Energies (Basel) 16 (3), 1170. doi:10.3390/en16031170
Hirawan, D., Oktafiani, D., Fauzan, T. A., Luckyardi, S., and Jamil, N. (2022). Research trends in farming system soil chemical: a bibliometric analysis using VOSviewer. Moroc. J. Chem. 10 (3). doi:10.48317/IMIST.PRSM/morjchem-v10i3.33145
Hossain, D. M., Mody, J., Faruque, H. M. R., Haque Sunny, M. S., and Mohammad, N. (2019). A comprehensive review on second-life batteries: current state, manufacturing considerations, applications, impacts, barriers and potential solutions, business strategies, and policies. IEEE Access 7, 73215–73252. doi:10.1109/access.2019.2917859
Hsieh, M. F., Chen, P. H., Pai, F. S., and Weng, R. Y. (2021). Development of supercapacitor-aided hybrid energy storage system to enhance battery life cycle of electric vehicles. Sustainability 13 (14), 7682. doi:10.3390/su13147682
INSIDEEVs (2020). GPSC to build battery plant in Thailand for 24M semi-solid tech. Available at: https://insideevs.com/news/399802/gpsc-solid-state-battery-24m/.
Karasu, H., and Dincer, I. (2020). Life cycle assessment of integrated thermal energy storage systems in buildings: a case study in Canada. Energy Build. 217, 109940. doi:10.1016/j.enbuild.2020.109940
Koese, M., Blanco, C. F., Vert, V. B., and Vijver, M. G. (2023). A social life cycle assessment of vanadium redox flow and lithium-ion batteries for energy storage. J. Ind. Ecol. 27 (1), 223–237. doi:10.1111/jiec.13347
Li, G., and Zhu, W. (2023). Tidal current energy harvesting technologies: a review of current status and life cycle assessment. Renew. Sustain. Energy Rev. 179, 113269. doi:10.1016/j.rser.2023.113269
Li, X., Chalvatzis, K., and Stephanides, P. (2018). Innovative energy islands: life-cycle cost-benefit analysis for battery energy storage. Sustainability 10 (10), 3371. doi:10.3390/su10103371
Liu, C., Gao, N., Cai, X., and Li, R. (2020). Differentiation power control of modules in second-life battery energy storage system based on cascaded H-bridge converter. IEEE Trans. Power Electron 35 (6), 6609–6624. doi:10.1109/tpel.2019.2954577
McKerracher, R. D., Ponce de Leon, C., Wills, R. G. A., Shah, A. A., and Walsh, F. C. (2015). A review of the IronAir secondary battery for energy storage. Chempluschem 80 (2), 323–335. doi:10.1002/cplu.201402238
Mostafa, M. H., Abdel Aleem, S. H. E., Ali, S. G., Ali, Z. M., and Abdelaziz, A. Y. (2020). Techno-economic assessment of energy storage systems using annualized life cycle cost of storage (LCCOS) and levelized cost of energy (LCOE) metrics. J. Energy Storage 29, 101345. doi:10.1016/j.est.2020.101345
Oró, E., Gil, A., de Gracia, A., Boer, D., and Cabeza, L. F. (2012). Comparative life cycle assessment of thermal energy storage systems for solar power plants. Renew. Energy 44, 166–173. doi:10.1016/j.renene.2012.01.008
Page, M. J., McKenzie, J. E., Bossuyt, P. M., Boutron, I., Hoffmann, T. C., Mulrow, C. D., et al. (2021). The PRISMA 2020 statement: an updated guideline for reporting systematic reviews. Int. J. Surg. 88, 105906. doi:10.1016/j.ijsu.2021.105906
Pellow, M. A., Ambrose, H., Mulvaney, D., Betita, R., and Shaw, S. (2020). Research gaps in environmental life cycle assessments of lithium ion batteries for grid-scale stationary energy storage systems: end-oflife options and other issues. Sustain. Mater. Technol. 23, e00120. doi:10.1016/j.susmat.2019.e00120
Prabhakaran, P., Giannopoulos, D., Köppel, W., Mukherjee, U., Remesh, G., Graf, F., et al. (2019). Cost optimisation and life cycle analysis of SOEC based Power to Gas systems used for seasonal energy storage in decentral systems. J. Energy Storage 26, 100987. doi:10.1016/j.est.2019.100987
Roushenas, R., Zarei, E., and Torabi, M. (2021). A novel trigeneration system based on solid oxide fuel cellgas turbine integrated with compressed air and thermal energy storage concepts: energy, exergy, and life cycle approaches. Sustain Cities Soc. 66, 102667. doi:10.1016/j.scs.2020.102667
Sahajwalla, V., and Hossain, R. (2023). Rethinking circular economy for electronics, energy storage, and solar photovoltaics with long product life cycles. MRS Bull. 48 (4), 375–385. doi:10.1557/s43577-023-00519-2
Tang, H., and Wang, S. (2023). Life-cycle economic analysis of thermal energy storage, new and secondlife batteries in buildings for providing multiple flexibility services in electricity markets. Energy 264, 126270. doi:10.1016/j.energy.2022.126270
United States Code of Federal Regulations (2024). 260.43 Legitimate recycling of hazardous secondary materials. Available at: https://www.ecfr.gov/current/title-40/chapter-I/subchapter-I/part-260#260.43.2018May.
United States Environment Protection Agency (2024a). Battery collection best practices and battery labeling guidelines. Available at: https://www.epa.gov/infrastructure/battery-collection-best-practices-and-battery-labeling-guidelines.
United States Environment Protection Agency (2024b). Legitimate hazardous waste recycling versus sham recycling. Available at: https://www.epa.gov/hw/legitimate-hazardous-waste-recycling-versus-sham-recycling.
Venettacci, S., Cozzolino, R., Ponticelli, G. S., and Guarino, S. (2022). Environmental and economic life cycle assessment of thermal energy storage based on organic phase change material embedded in open-cell copper foams. Sustain Prod. Consum. 29, 387–405. doi:10.1016/j.spc.2021.10.026
Wang, Y. J., Deng, M., Zhang, X., Zhang, J., Sui, Y., Sun, K., et al. (2024). Sustainable synthesis of Ni, Mn co-doped FePO4@C cathode material for Na-ion batteries. J. Colloid Interface Sci. 661, 23–32. doi:10.1016/j.jcis.2024.01.198
Yudhistira, R., Khatiwada, D., and Sanchez, F. (2022). A comparative life cycle assessment of lithium-ion and lead-acid batteries for grid energy storage. J. Clean. Prod. 358, 131999. doi:10.1016/j.jclepro.2022.131999
Zakeri, B., and Syri, S. (2015). Electrical energy storage systems: a comparative life cycle cost analysis. Renew. Sustain. Energy Rev. 42, 569–596. doi:10.1016/j.rser.2014.10.011
Zhang, J., Chen, L., Niu, L., Jiang, P., Shao, G., and Liu, Z. (2019). Na superionic conductor-type TiNb(PO4)3 anode with high energy density and long cycle life enables aqueous alkaline-ion batteries. ACS Appl. Mater Interfaces 11 (43), 39757–39764. doi:10.1021/acsami.9b10671
Keywords: sustainability, renewable energy, environmental impact, bibliometric analysis, sustainable energy matrix, PRISMA-2020
Citation: García-Pineda V, Valencia-Arias A, Zapata Ochoa EA, Sánchez-Santos G, Gastiaburú-Morales SY and Palacios-Moya L (2024) Research trends in the use of secondary batteries for energy storage. Front. Environ. Sci. 12:1362706. doi: 10.3389/fenvs.2024.1362706
Received: 28 December 2023; Accepted: 26 March 2024;
Published: 17 April 2024.
Edited by:
Jill A. Engel-Cox, National Renewable Energy Laboratory (DOE), United StatesReviewed by:
Taylor L. Curtis, National Renewable Energy Laboratory (DOE), United StatesShigeto Okada, Kyushu University, Japan
Copyright © 2024 García-Pineda, Valencia-Arias, Zapata Ochoa, Sánchez-Santos, Gastiaburú-Morales and Palacios-Moya. This is an open-access article distributed under the terms of the Creative Commons Attribution License (CC BY). The use, distribution or reproduction in other forums is permitted, provided the original author(s) and the copyright owner(s) are credited and that the original publication in this journal is cited, in accordance with accepted academic practice. No use, distribution or reproduction is permitted which does not comply with these terms.
*Correspondence: Alejandro Valencia-Arias, valenciajho@uss.edu.pe