Biochar application for sustainable soil erosion control: a review of current research and future perspectives
- Department of Agricultural Engineering and Technology, School of Engineering and Technology, Nagaland University, Dimapur, Nagaland, India
Soil erosion is a pressing environmental issue with significant agricultural productivity and ecosystem stability implications. In recent years, biochar, a carbon-rich product of biomass pyrolysis, has emerged as a promising soil amendment tool for erosion control due to its ability to improve soil quality and stability. This review paper aims to comprehensively analyze the effectiveness of biochar role in mitigation of soil erosion and sustainable land management practices. By examining a wide range of research studies, this paper elucidates the impact of biochar on key soil erosion parameters as it directly affects the soil structure, water-holding capacity, and nutrient retention. The paper discusses how biochar interacts with soil particles and aggregates to enhance their stability and resistance to erosive forces. It also assesses the influence of biochar properties, such as feedstock type, pyrolysis temperature, and application rate, on its erosion control efficacy. Furthermore, this review explores the role of biochar in promoting plant growth and root development, thereby reinforcing the vegetation cover and further reducing erosion susceptibility. Finally, an outline of potential challenges and opportunities for the widespread adoption of biochar-based erosion control strategies in different agricultural and environmental contexts is presented. Overall, this review provides valuable insights into the multifacet role of biochar in sustainable soil management and offers recommendations for future research directions on direct and indirect application on soil erosion control.
1 Introduction
Soil erosion is a significant environmental issue affecting various parts of the world. It is the process by which topsoil is removed or displaced by natural forces, such as wind or water flow. This is exacerbated by human activities like deforestation, overgrazing, and improper agricultural practices (Bhattacharyya et al., 2015). The global annual soil loss due to erosion is significant, suggesting approximately 24 billion tons of fertile topsoil loss yearly (Abdel Rahman, 2023). Soil erosion, caused by water, wind, and human activities, severely threatens agricultural productivity, food security, and environmental sustainability. Soil erosion can have various detrimental impacts, including reduced agricultural productivity, water pollution, sedimentation in rivers and lakes, and destruction of natural habitats (Issaka and Ashraf, 2017).
Globally, several regions face extreme soil erosion problems. For example, extensive soil erosion is a critical issue in China, primarily due to deforestation, overgrazing, and intensive farming practices (Wang et al., 2021). It led to sedimentation in rivers, reduced agricultural productivity, and increased desertification. Many African regions, particularly sub-Saharan Africa, experience significant soil erosion. Deforestation, overcultivation, and poor land management further contribute to the problem (Abdel Rahman, 2023). This has severe consequences for agricultural productivity and food security in the region. Countries like Indonesia and the Philippines struggle with soil erosion due to deforestation, unsustainable farming practices, and the expansion of palm oil plantations (Ramdani and Lounela, 2020), which led to the loss of fertile soil, reduced biodiversity, and increased sedimentation in water bodies. Soil erosion is also a concern in the United States, particularly in the Midwest and Great Plains regions, where intensive agriculture developed degradation of soil quality and increased sedimentation in waterways (Chen et al., 2022). In South American countries like Brazil and Argentina, deforestation, unsustainable agricultural practices, and land degradation contribute to significant soil erosion issues by impacting local ecosystems and global climate patterns (Santibañez and Santibañez, 2007).
In India, soil erosion is a pressing issue, particularly in regions with intensive agricultural activities, deforestation, and improper land management practices. Factors such as overgrazing, monsoon rains, and the unsustainable use of land contribute to the problem. They impact the agricultural productivity and fertile topsoil, results into sedimentation in rivers and increases vulnerability to natural disasters like floods and landslides in India (Bhattacharyya et al., 2015). Initiatives such as implementation of watershed management practices, afforestation programs, and sustainable agricultural techniques are being undertaken to mitigate soil erosion and conserve the country’s vital natural resources.
Soil erosion contributes to the loss of essential nutrients and topsoil. As the fertile top layer is gradually washed or blown away, the soil’s ability to support plant growth diminishes, which impacts crop yields and overall agricultural sustainability. The loss of nutrients crucial for plant growth (such as nitrogen, phosphorus, and potassium) further exacerbates the problem, necessitating the increased use of fertilizers, which have environmental and economic implications (Nathan et al., 2022; Zhu et al., 2022; Jiao et al., 2023). Moreover, erosion-induced sedimentation in water bodies can disrupt aquatic ecosystems and water quality by causing eutrophication and additional environmental challenges (Wang et al., 2021). To combat this, implementing measures such as conservation tillage, agroforestry, and cover cropping is crucial in preserving the nutrient-rich topsoil and sustaining agricultural productivity in the long term (Fahad et al., 2022).
So far, several effective practices have been implemented globally to control soil erosion. These practices included the use of conservation tillage, which involves minimizing soil disturbance during planting and establishing buffer zones near water bodies to trap sediment and reduce nutrient runoff (Wen et al., 2023). Additionally, adopting agroforestry techniques, such as planting trees in combination with crops, helped stabilize the soil and mitigate erosion (Fahad et al., 2022). Terracing and contour farming continued to be widely employed on hilly terrain to reduce water runoff and prevent soil loss (Deng et al., 2021). Furthermore, promoting cover cropping and crop rotation strategies contributed to maintaining soil structure and fertility, thereby minimizing erosion risks (Koudahe et al., 2022; Scavo et al., 2022). The emphasis on maintaining soil structure and microbial diversity also contributes to improved water retention and reduced susceptibility to erosion. In addition, the organic farming plays a pivotal role in promoting sustainable agricultural practices and effectively controlling soil erosion (Gamage et al., 2023). Organic farming enhances the capacity to withstand the negative impacts of climate change by fostering resilience within the agroecosystem. It establishes robust and environment friendly farming systems that demonstrate resilience to temperature variations and drought while indirectly prevents soil erosion. The organic farming also advocates for sustainable and eco-friendly management, embraces conservation practices, and supports restoration activities. At the same time, the organic farming practices reduce the risk of water pollution from chemical runoffs, fostering a healthier environment. However, the inorganic fertilizers increased soil erosion and degradation while providing essential nutrients for plant growth (Tripathi et al., 2020). The reliance on these fertilizers often leads to a reduction in microbial habitat and biodiversity within the soil. The integration of all these measures, along with sustainable land management approaches, played a critical role in combating soil erosion and preserving agricultural productivity.
India has been actively implementing various measures as well to combat soil erosion. These include promoting sustainable agricultural practices like conservation tillage and crop rotation and constructing check dams and contour trenches for water conservation (Bhattacharyya et al., 2015). Afforestation programs and watershed management projects have also been crucial in controlling soil erosion and preserving soil fertility (Liu et al., 2020). Educational campaigns to raise awareness about effective soil conservation techniques among farmers and local communities may produce adequate results (Mango et al., 2017).
Biochar, a carbon-rich product of biomass pyrolysis, has shown promise as an effective tool for controlling soil erosion (Wani et al., 2023). Its porous structure and high surface area enhance soil porosity and water retention by reducing runoff and soil loss during heavy rainfall (Kameyama et al., 2012). Biochar-amended soils have improved stability, reduced susceptibility to erosion, and enhanced nutrient retention capacity (Nathan et al., 2022; Vahidi et al., 2022). Furthermore, incorporating biochar into soil has been found to promote microbial activity by fostering a healthier soil ecosystem that further contributes to erosion control (Das et al., 2021; Jia et al., 2024). Biochar application exerts generally positive impacts on different forms of soil erosion, including wind, water, and cultivation erosion. In the context of wind erosion, biochar enhances soil moisture and structure by promoting aggregation and reducing susceptibility to wind-driven soil loss to some extent (Silva et al., 2015). The water erosion is mitigated through biochar application with the improvement in water retention capacity, reduction in surface runoff, as well as enhancement in soil stability (Gholamahmadi et al., 2023). This effectively prevents the soil particles from being transported by water to great extent. In the event of soil disturbance during agricultural practices, biochar’s role in enhancing soil structure and stability also contributes to reduced erosion. Although the specific mechanisms differ for different scenarios, the overarching effect of biochar is a consistent for soil resilience across various erosion types and making it a versatile and effective solution for sustainable land management. Although biochar use for soil erosion control appears promising, further research is necessary to fully understand its long-term effectiveness, optimal application rates, and potential environmental impacts. Nonetheless, biochar holds potential as a sustainable solution for mitigating soil erosion and improving overall soil health.
Besides its effectiveness in reducing soil erosion, biochar offers significant advantages in soil nutrient management. With its high surface area and porosity, biochar exhibits a remarkable ability to retain essential plant nutrients, preventing their leaching and making them available to plants over an extended period (Feng et al., 2022; Nathan et al., 2022). Furthermore, its application contributes to improved soil structure, increased water retention, and enhanced microbial habitat, i.e., fostering nutrient cycling and promoting overall soil health (Kameyama et al., 2012). Its role in mitigation of greenhouse gas emissions by sequestering carbon further highlights its potential as a sustainable solution for agricultural sustainability and environmental conservation (Abhishek et al., 2022).
While biochar offers numerous benefits in soil nutrient management, its application must be carefully managed, considering factors such as feedstock type, production process, and soil characteristics. Its widespread adoption requires further research to determine optimal application rates, the long-term effects on soil health, and potential environmental impacts (Mango et al., 2017). Despite these considerations, biochar shows promise as a sustainable tool for improving soil fertility, enhancing crop productivity, and contributing to climate change mitigation efforts (Abhishek et al., 2022; Zhang et al., 2022a).
Current understanding suggests that biochar can effectively mitigate soil erosion by enhancing soil stability, reducing surface runoff, and improving water infiltration (Kameyama et al., 2012; Xia et al., 2022). However, challenges remain, including the need to establish optimal application rates, as excessive biochar application might alter soil properties and impact plant growth (Gholamahmadi et al., 2023). Additionally, the influence of biochar on specific soil types, climates, and crop varieties requires further investigation for a comprehensive understanding of its effectiveness in diverse agricultural settings. Studies are underway to assess the long-term impacts of biochar application on soil fertility, microbial activity, and ecosystem dynamics. Exploring the synergistic effects of biochar in combination with other soil conservation practices is also a critical area of research, aiming to develop integrated and sustainable approaches for effective soil erosion control in various agricultural landscapes.
Addressing the global soil erosion problem requires a combination of sustainable land management practices, afforestation, soil conservation measures, and responsible agricultural techniques. This review paper encompasses a comprehensive analysis of the current research on using biochar for soil erosion control, emphasizing its effectiveness, mechanisms, and potential applications. It also addresses the challenges and opportunities of adopting biochar-based strategies in sustainable land management.
2 Biochar impact on soil erosion parameters
Biochar has shown considerable potential in positively influencing various soil erosion parameters (Figure 1). Its application significantly reduces soil loss by enhancing soil stability, improving aggregate strength, and promoting the formation of stable soil aggregates (Zhang et al., 2022a; Qiu et al., 2023). The porous structure of biochar aids in increasing water infiltration and reducing surface runoff, thereby minimizing the impact of water on soil erosion (Jien and Wang, 2013). Additionally, biochar-amended soils exhibit improved water-holding capacity, which is likely reducing soil erosion vulnerability during heavy rainfall events (Kameyama et al., 2012). Its ability to enhance soil organic matter content and stimulate microbial activity contributes to developing a more resilient soil structure that likely mitigates erosion risks. Moreover, the long-term stability of biochar in the soil ensures a sustained impact on erosion control, which is making it a valuable tool in promoting sustainable land management practices and preserving soil integrity (Das et al., 2021; Hou, 2021). The current research explores the mechanisms underlying biochar’s influence on soil erosion parameters and optimizes its application for effective erosion control in various environmental settings.
2.1 Impact on soil structure/texture
This is evident that biochar significantly impacts the soil structure. Its introduction to the soil enhances soil aggregation by creating a more stable and porous soil matrix. By improving aggregation, biochar develops the formation of stable soil aggregates with better soil porosity, water infiltration, and retention (Zhang et al., 2022a). The porous nature of biochar allows for increased water and nutrient-holding capacity, which is promoting a favorable environment for root growth and microbial activity. The biochar improves soil structure by reducing soil compaction, particularly in clayey soils, thus enhances the root penetration and nutrient uptake (Blanco-Canqui, 2021). The long-term stability of biochar in the soil contributes to sustained improvements in soil structure, which is crucial for sustainable agricultural practices and ecosystem health (Kameyama et al., 2012). The influence of soil texture on soil-biochar interactions plays a crucial role in determining the effectiveness of biochar applications. Studies have shown that soil texture affects the adsorption capacity, porosity, and nutrient retention of biochar. In sandy soils, biochar often improves water retention and nutrient availability due to its high surface area and porosity, thus enhances the overall soil fertility. Conversely, in clayey soils, biochar alleviates compaction issue, improve drainage, and enhance the soil structure. Further research should be conducted to understand the intricate mechanisms of biochar-soil interactions and to optimize its application for improving soil structure and long-term agricultural sustainability.
2.2 Impact on water holding capacity of soil
Biochar can have a significant impact on the water-holding capacity of soil. Its porous structure and high surface area enable it to hold water effectively, thus enhancing the soil’s ability to retain moisture (Kameyama et al., 2012). When incorporated into the soil, biochar acts as a sponge by absorbing excess water during high precipitation and releasing it slowly during dry periods, which maintains optimal soil moisture level for plant growth. This characteristic is particularly beneficial in arid and semi-arid regions with limited water availability. The improved water-holding capacity provided by biochar also contributes to drought resistance, reduces the need for irrigation, and promotes the survival and growth of crops, which ultimately enhances the agricultural productivity and sustainability (Kameyama et al., 2012). At the same time, the impact of biochar on water retention fluctuates depending on biochar type, application rate, soil type, and environmental conditions, which emphasizes the importance of these factors while utilizing biochar for water management in agricultural systems (as highlighted in Section 4). The influence of biochar on soil water retention for sustainable water resource management and climate-resilient agriculture need to be investigated further.
2.3 Impact of nutrient retention
Biochar has a substantial impact on the retention of nutrients in the soil. Its high surface area and porous structure provide an ideal environment for nutrient adsorption and retention, which prevents leaching and making essential nutrients available to plants over an extended period. By effectively trapping nutrients such as nitrogen, phosphorus, potassium, and various micronutrients, biochar helps to reduce nutrient and pollution release into water bodies by promoting environmental sustainability (Yang et al., 2017a; 2017b; 2019a; Feng et al., 2022; Nathan et al., 2022). Furthermore, biochar in the soil enhances the cation exchange capacity and facilitates the retention and exchange of positively charged ions, which are crucial for plant growth (Domingues et al., 2020). This improved nutrient retention capacity increased the nutrient-use efficiency in agricultural systems and minimized the need for additional fertilizers i.e., reduced the risk of nutrient loss (Zhang et al., 2022a). The specific effects of biochar on nutrient retention can also vary depending on factors such as biochar feedstock, production process, application rate, and soil type, which emphasizes the importance of tailored approaches for optimization of nutrient management in diverse agricultural settings. The intricates mechanisms underlying the interaction between biochar and soil nutrients for sustainable farming practices to maximize nutrient retention and minimize environmental impacts should be a topic of future research.
3 Biochar interaction mechanisms
The interaction between biochar and soil involves complex and multifaceted mechanisms that influence various soil properties and processes (Figure 2). One key mechanism is the physical structure of biochar, which contributes to improved soil porosity, water retention, and aeration, i.e., promoting favorable conditions for plant growth and microbial activity (Ding et al., 2016; Alkharabsheh et al., 2021; Liu et al., 2024). The high surface area and porosity of biochar also facilitate the adsorption and retention of nutrients, organic compounds, and contaminants, which is enhancing soil fertility and reducing the leaching of essential nutrients. Additionally, the chemical properties of biochar, such as its pH and surface charge, influences the soil acidity, nutrient availability, and cation exchange capacity by further impacting soil health and plant nutrition (Domingues et al., 2020). Moreover, the biological interactions between biochar and soil microbiota are crucial in nutrient cycling, organic matter decomposition, and stable soil aggregates, which contributes towards long-term soil fertility and structure development (Zhang et al., 2022a). These intricate mechanisms are essential for optimizing biochar application in diverse agricultural systems for sustainable soil management practices and mitigation of environmental challenges associated with soil degradation and nutrient loss. The complexities of biochar-soil interactions for development of effective and tailored strategies are being explores for maximizing the benefits of biochar in enhancing soil quality and agricultural productivity.
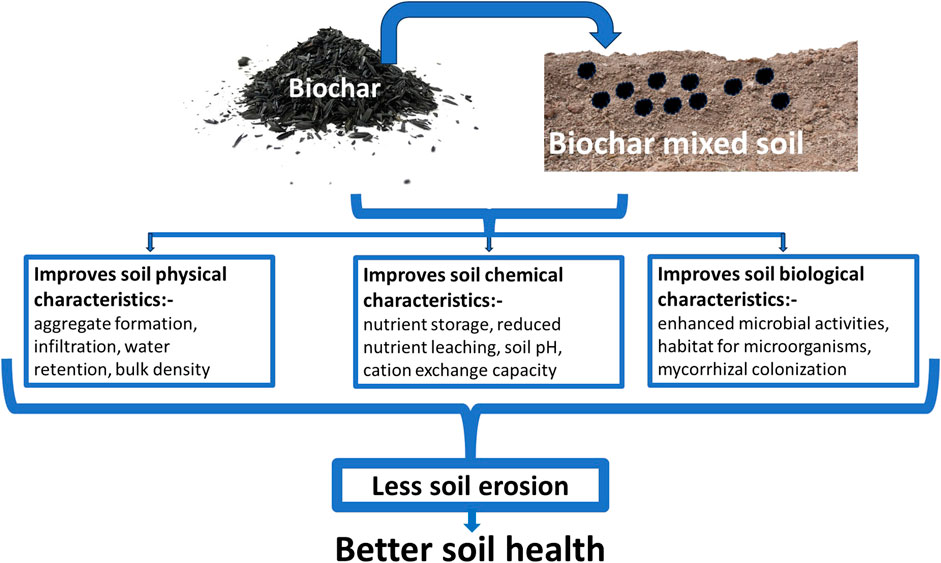
Figure 2. Multifaceted mechanisms affecting soil properties and processes due to the interaction between biochar and soil.
3.1 Interaction with soil particles
The introduction of biochar into soil initiates intricate interactions with soil particles, which influences various physical and chemical properties. Biochar’s porous structure and high surface area enable it to interact with soil particles physically by enhancing the soil aggregation and stability. The biochar forms stable soil aggregates, improves soil porosity, water infiltration, and water-holding capacity, thereby creates a more favorable environment for plant growth (Domingues et al., 2020). Chemically, biochar interacts with soil particles through its surface functional groups, which is affecting the soil pH, nutrient availability, and cation exchange capacity. These interactions also improve the soil fertility, enhance the nutrient retention, and reduce the nutrient leaching, i.e., ultimately benefiting plant health and productivity (Ding et al., 2016). These intricate relations between biochar and soil particles are crucial for optimizing its application to improve soil quality, promote sustainable agriculture, and mitigate environmental degradation. For the future research, the application of biochar would be a valuable tool for soil enhancement and sustainable land management.
3.2 Aggregate formation in the field due to biochar
Biochar has demonstrated a significant potential in promoting aggregate formation in soil (Hua et al., 2014). Biochar stabilizes soil aggregates through its physical and chemical properties, leading to improved soil structure and stability. The porous nature of biochar allows for the creation of microhabitats that facilitate the colonization of beneficial soil microorganisms, which ultimately promoting the soil aggregation. Additionally, biochar serves as a binding agent, helping to cement the soil particles together and enhancing the stable macroaggregate formation (Blanco-Canqui, 2017; Yang et al., 2019b). This enhanced aggregation contributes to better soil porosity, water infiltration, and root penetration, ultimately fostering a more conducive environment for the plant growth (Domingues et al., 2020). Moreover, the persistent nature of biochar in the soil matrix ensures a sustained positive influence on aggregate formation, thereby promoting long-term soil fertility and resilience. The biochar’s role in aggregate formation is considered an important tool to develop a sustainable practices that leverage biochar as a valuable tool for enhancing soil structure and overall agricultural productivity.
3.3 Stability and resistance to erosive forces
Biochar application induces notable change in the stability and resistance of soil to erosive forces. Its incorporation into the soil matrix enhances soil aggregation and structure, which is developing stable soil aggregates that are less prone to breakdown under erosive forces (Herath et al., 2013; Curaqueo et al., 2014). The increased aggregate stability contributes to improved soil structure, reduced susceptibility to compaction, and enhanced resistance to water and wind erosion (Jien and Wang, 2013; Li et al., 2019; Xia et al., 2022). Biochar’s ability to improve soil organic matter content and promote microbial activity further strengthens the soil structure, which is fostering the development of a more resilient soil matrix to withstand erosive pressures. Additionally, the water-holding capacity provided by biochar aids in maintaining optimal soil moisture levels, which reduces the impact of water erosion even during heavy rainfall events (Kameyama et al., 2012). Its long-term persistence in the soil ensures sustained improvements in soil stability, i.e., underscoring its potential as an effective tool for mitigation of soil erosion and development of soil integrity.
4 Biochar properties on erosion intensity
The properties of biochar play a critical role in influencing erosion intensity in various environmental contexts. The fundamental biochar properties, such as particle size, surface area, porosity, and chemical composition, significantly impact its effectiveness in erosion mitigation (Table 1). Biochar with larger particle sizes and increased surface area tends to create a more stable soil structure by reducing the soil susceptibility to erosive forces (Wang et al., 2020). Its high porosity facilitates the water infiltration and retention, which minimizes the surface runoff and soil detachment during rainfall events (Ahmadi et al., 2020). Furthermore, the chemical composition of biochar, including its pH, nutrient content, and surface functional groups, influences soil stability and aggregation, thereby reducing the potential for soil loss due to erosion (Wani et al., 2023). This intricate interplay between biochar properties and erosion intensity is crucial for optimization of its application in erosion-prone areas and sustainable land management practices.
4.1 Feedstock type for biochar
The choice of feedstock/biomass for biochar production significantly influences its effectiveness in reduction of erosion intensity. The microscopic image of biochar synthesized from selected feedstock types is illustrated in Figure 3. They illustrate the unique surface roughness for biochar developed using different biomasses. Various feedstock materials, such as wood chips, agricultural residues, and organic waste, yield biochar with distinct physical and chemical properties that would impact its erosion control capabilities. Feedstocks with high lignin and cellulose content, such as woody biomass, produce biochar with enhanced structural stability and a higher resistance to erosive forces (Wang et al., 2020). This type of biochar effectively strengthens the soil aggregates by reducing soil detachment and erosion risk. The relationship between feedstock type and biochar properties would be essential for the selection of suitable feedstocks to maximize biochar’s efficacy in erosion-prone environments.
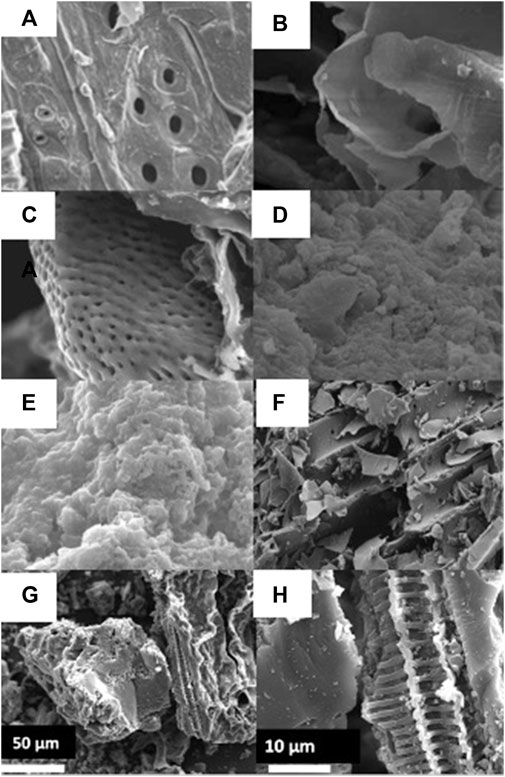
Figure 3. Morphology of biochar produced from different types of feedstock: (A) mesquite wood (Trigo et al., 2016); (B) corn stalk (Ma et al., 2016); (C) chicken manure (Joseph et al., 2010); (D) sewage sludge (Song et al., 2014); (E) anaerobic digestate (Tang et al., 2019); (F) coconut husk (Suman and Gautam, 2017) (G) paddy straw (H) tea residue.
4.2 Pyrolysis temperature for biochar synthesis
The pyrolysis temperature employed in biochar production significantly impacts its effectiveness in mitigating erosion intensity. Different pyrolysis temperatures yield biochar with varying physical and chemical properties that influence its stability and performance in erosion control. Biochar produced at higher pyrolysis temperatures tends to have higher carbon content, increased aromaticity, and a more condensed structure (Yang et al., 2019b), which is improving the resistance to degradation and erosive forces. Such biochar exhibits enhanced stability, higher surface area, and improved water-holding capacity (Wang et al., 2020). It is evident that higher pyrolysis temperatures significantly impact the nutrient and mineral content of feedstocks. When biomass is pyrolyzed at high temperatures, there is a greater likelihood of thermal decomposition, which is leading to the loss of volatile organic compounds and a reduction in the overall nutrient content of the resulting biochar (Yang et al., 2019b). The high temperatures facilitate the breakdown of complex organic compounds, i.e., loss of volatile nitrogen compounds and the transformation of phosphorus into less plant-available forms (Liu et al., 2023; Zhao et al., 2024). The severity of this impact varies based on the feedstock type and the specific pyrolysis conditions. Generally, higher temperatures tend to result in biochar with lower concentrations of nutrients and minerals. Studies have shown that at elevated pyrolysis temperatures, such as above 600°C, biochar tends to have reduced nitrogen and phosphorus content (Zhang et al., 2022b).
While biochar has been extensively studied as a soil amendment, it is crucial to recognize that the implication of high-temperature pyrolysis on nutrient retention might vary across different contexts and applications. The effectiveness of biochar as a soil amendment tool depends on factors such as soil type, climate, and the specific goals of the agricultural or environmental management system. Therefore, interpretations of biochar’s applicability should consider these contextual variations, and cautious consideration is needed when extrapolating findings from one study to another. The impact of pyrolysis temperature on biochar properties is essential for optimizing its production to yield biochar with the desired characteristics for effective erosion control. The future research should explore the relationships between pyrolysis temperature, biochar properties, and erosion intensity systematically in order to develop a tailored pyrolysis protocol for producing biochar which effectively mitigates the erosion and preserve soil quality.
4.3 Application rate on agricultural land
Biochar provides a stable soil structure to agricultural soil. The long-lasting soil amendment of biochar enhances the soil structure, water retention, and nutrient availability; however, the application rate of biochar should be carefully determined based on the specific soil properties, crop types, and local environmental conditions for effective soil erosion control (Gholamahmadi et al., 2023). Optimal application rates typically range from 10 to 50 tons per hectare, depending on the severity of erosion, soil texture, and organic matter content (Bista et al., 2019). The biochar application rate for different soil types is indicated in Table 2. This range allows formation of a stable soil structure and improved water infiltration by reducing surface runoff and effectively minimizing erosion (Wani et al., 2023). However, it is crucial to consider the potential impact of excessive biochar application on soil pH, nutrient availability, and plant growth by emphasizing the need for site-specific assessments and careful monitoring to ensure sustainable agricultural practices and long-term soil health.
5 Biochar impact on plant
Biochar, with its porous structure and high surface area, has a range of positive effects on plant growth and development. It has demonstrated a significant potential to impact the crop production and agricultural sustainability positively (Table 3). Its introduction to the soil enhances the overall soil health and fertility, i.e., improving crop yields and quality. Biochar’s porous structure aids to increase in water retention and reduction in nutrient leaching, thereby promoting more efficient water and nutrient use by crop efforts (Zhang et al., 2022a). By fostering a healthier and more balanced soil microbiome, biochar contributes to better plant nutrient availability and uptake.
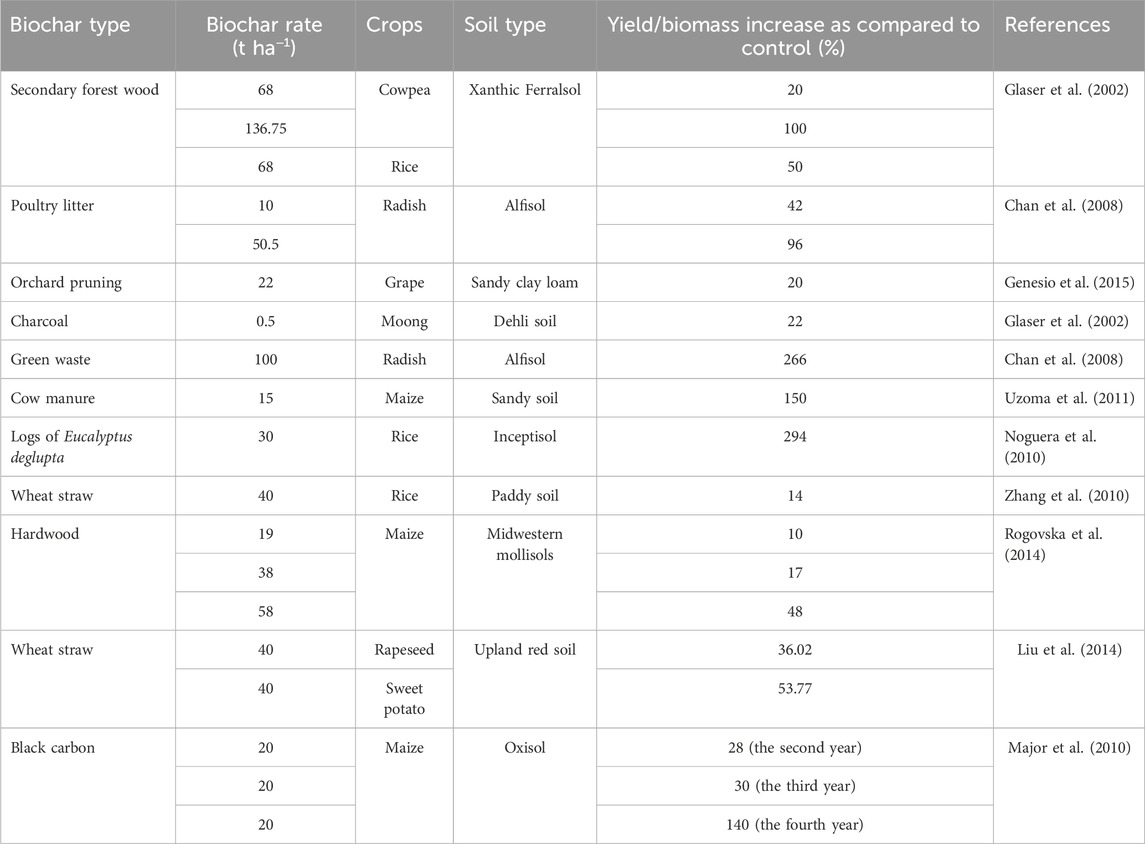
Table 3. Effects of biochar addition on crop yield (adopted from Ding et al., 2016).
Additionally, its influence on soil structure and aeration facilitates root growth and penetration, further supporting the plant development. The biochar’s ability to sequester carbon in the soil also contributes to climate change mitigation and aids in the long-term stability and sustainability of agricultural ecosystems (Abhishek et al., 2022). Nevertheless, while biochar has shown significant promise, its effect on crops is influenced by factors such as the specific crop type, soil conditions, biochar properties, and application methods. Therefore, a careful consideration of these variables is crucial for maximizing the potential benefits of biochar in sustainable crop production system.
5.1 Development and growth of plant roots
The development and growth of plant roots on land, where biochar has been applied, demonstrate several promising effects that contribute to improved plant performance. Biochar amendments enhance the soil’s physical structure by promoting better aeration and water retention, which in turn fosters more extensive and vigorous root systems efforts (Zhang et al., 2022a). The porous nature of biochar provides a conducive habitat for beneficial soil microorganisms by creating a symbiotic relationship that aids in nutrient cycling and availability, thereby facilitating robust root development. Furthermore, the increased water-holding capacity of biochar-amended soil supports sustained root growth, particularly during dry spells by helping the plants to withstand drought stress. Studies also suggest that biochar amendments influence the rhizosphere’s microbial community composition, i.e., potentially improving nutrient acquisition and overall plant health (Liao et al., 2013; Yan et al., 2022). However, the specific effects of biochar on root development may vary depending on factors such as biochar properties, application rates, and soil conditions. Therefore, a comprehensive research is necessary to optimize biochar application strategies for enhanced root growth and sustainable agricultural practices that promote resilient plant-root systems and long-term soil health.
5.2 Reinforcement of vegetation cover
The reinforcement of vegetation cover due to biochar application plays a vital role in promoting ecosystem stability and resilience. Biochar amendments enhance the soil fertility and structure, fostering an environment conducive to robust plant growth and establishment. The improved water-holding capacity of biochar-amended soil helps to mitigate the negative impacts of water stress on vegetation, allowing plants to maintain optimal physiological functions even during periods of limited water availability (Wang et al., 2021). Moreover, biochar’s ability to promote nutrient retention and availability in the soil supports vegetation’s sustained growth and vitality, aiding in establishing dense and healthy vegetation cover (Alkharabsheh et al., 2021; Kochanek et al., 2022). This reinforcement of vegetation cover not only helps to prevent soil erosion but also contributes to the conservation of biodiversity and the overall stability of the ecosystem. However, the effectiveness of biochar in reinforcing vegetation cover may depend on various factors, including the specific biochar properties, application methods, and local environmental conditions. Therefore, a comprehensive understanding of these factors is essential to maximize the benefits of biochar application for sustainable ecosystem management and conservation.
5.3 Reduction of erosion susceptibility
The application of biochar to land is an effective strategy for reduction of erosion susceptibility and soil conservation. Biochar amendments significantly improve the soil structure and stability, enhancing the soil’s resistance to erosive forces such as wind and water (Jien and Wang, 2013; Li et al., 2019). The porous nature of biochar increases the soil porosity and aggregation by creating a more stable soil matrix, i.e., less susceptible to displacement and runoff (Hao et al., 2022). By enhancing water retention and infiltration, biochar helps to maintain soil moisture levels and reduces the likelihood of surface crusting and soil detachment, which are common precursors to erosion (Kameyama et al., 2012; Domingues et al., 2020; Zhang et al., 2022a). Additionally, incorporating biochar into the soil promotes the development of a well-structured root system, which is further anchoring the soil and minimizing the risk of erosion. The improved nutrient retention and microbial activity associated with biochar-amended soil also contribute to the establishment of a healthy vegetative cover by providing additional protection against erosion. However, the effectiveness of biochar in reducing erosion susceptibility may vary depending on factors such as biochar properties, application rates, and soil characteristics. Thus, site-specific assessments and sustainable land management practices are essential for harnessing the full potential of biochar in mitigating erosion and ensuring long-term soil conservation.
6 Potential challenges and opportunities for biochar-based erosion control strategies
While biochar-based erosion control strategies hold significant promise, several challenges and opportunities must be considered for effective implementation. It is important to note that most of these studies are indirect and corelated with their corresponding soil and water properties. The implementation of biochar-based erosion control strategies encounters challenges requiring careful consideration and proactive management. One of the primary challenges lies in the intricate interactions between biochar, soil properties, and local environmental conditions, necessitating site-specific assessments to determine the optimal biochar application rates and methods (Bista et al., 2019). The cost of large-scale biochar implementation can be prohibitive, particularly for resource-limited agricultural communities, thereby hindering widespread adoption.
Furthermore, ensuring the long-term stability of biochar-amended soils and maintaining their erosion control benefits demand continuous monitoring and management, especially in dynamic and variable environmental contexts. The effectiveness of biochar in erosion control is also influenced by factors such as biochar characteristics, soil type, and climatic variability, underscoring the need for comprehensive research and tailored application techniques (Li and Tasnady, 2023). Addressing these challenges requires collaborative efforts between researchers, policymakers, and local communities to develop sustainable and cost-effective biochar-based erosion control strategies that are dependent on specific environmental contexts and socioeconomic constraints. Biochar-based erosion control strategies present a fertile ground for various research opportunities to enhance their effectiveness and practical implementation. Firstly, there is a need for comprehensive field studies to assess the long-term impact of biochar on different soil types and under varying climatic conditions by considering its influence on soil structure, water retention, and vegetation cover (Wang et al., 2021). Soil erosion control through biochar treatments greatly dependent on soil types. The detail of biochar impact and uses on different soil types are highlighted in Table 2. The interaction mechanism between biochar and soil microorganisms provide insights into optimizing its potential for enhancing soil stability and erosion resistance.
Additionally, research focusing on development of cost-effective production techniques and biochar formulations to specific soil erosion challenges facilitate the broader adoption in diverse agricultural settings. Exploring the potential synergies between biochar and other soil conservation practices, such as cover cropping and terracing, could offer integrated and sustainable erosion management solutions (Bhattacharyya et al., 2015). Furthermore, investigating the socioeconomic implications of biochar adoption, including its impact on agricultural productivity and livelihoods, can guide policy development and promote community engagement in erosion control efforts (Rogers et al., 2021; Li and Tasnady, 2023). These research opportunities pave the way for the refinement and widespread application of biochar-based erosion control strategies by contributing to the sustainability and resilience of agricultural landscapes worldwide.
It is evident from this study that the existing research is limited on the direct use of biochar application for soil erosion control. Instead, the majority of references primarily explore the impact of biochar on various soil parameters which influence the soil erosion either directly or indirectly. This gap highlights the need for more targeted investigations specifically addressing the role of biochar in mitigation of soil erosion to better understand its potential contributions for sustainable land management. Addressing these challenges and capitalizing on the opportunities successfully integrate the biochar-based erosion control strategies into sustainable land management practices and promote the resilient agricultural systems and environmental conservation.
7 Conclusion
In conclusion, biochar application for sustainable soil erosion control holds considerable promise, as evidenced by several research findings. Its ability to enhance soil structure, water retention, and vegetation cover underscores its potential as an effective erosion mitigation strategy. However, the complexities of biochar-soil interactions and the diverse environmental and socioeconomic contexts in which erosion occurs emphasize the need for further research and development. Future efforts should focus on conducting long-term field studies to assess the durability and efficacy of biochar in diverse geographical settings. Additionally, exploring innovative production methods, systematic biochar formulations, and integrated erosion management approaches could unlock new opportunities for maximizing its benefits. Furthermore, addressing the economic feasibility and scalability of biochar adoption and understanding its broader implications for sustainable agricultural practices and ecosystem services are crucial for its successful integration into erosion control strategies. By embracing a multidisciplinary approach and fostering collaboration between researchers, policymakers, and local communities, biochar application can emerge as a pivotal tool in promoting sustainable soil conservation and nurturing resilient agricultural landscapes for generations.
Author contributions
PS: Conceptualization, Data curation, Formal Analysis, Investigation, Methodology, Validation, Visualization, Writing–original draft, Writing–review and editing.
Funding
The author(s) declare that no financial support was received for the research, authorship, and/or publication of this article.
Conflict of interest
The author declares that the research was conducted in the absence of any commercial or financial relationships that could be construed as a potential conflict of interest.
The author(s) declared that they were an editorial board member of Frontiers, at the time of submission. This had no impact on the peer review process and the final decision.
Publisher’s note
All claims expressed in this article are solely those of the authors and do not necessarily represent those of their affiliated organizations, or those of the publisher, the editors and the reviewers. Any product that may be evaluated in this article, or claim that may be made by its manufacturer, is not guaranteed or endorsed by the publisher.
Supplementary material
The Supplementary Material for this article can be found online at: https://www.frontiersin.org/articles/10.3389/fenvs.2024.1373287/full#supplementary-material
References
Abdel Rahman, M. A. E. (2023). An overview of land degradation, desertification and sustainable land management using GIS and remote sensing applications. Rendiconti Lincei. Sci. Fis. Nat. 34 (3), 767–808. doi:10.1007/s12210-023-01155-3
Abhishek, K., Shrivastava, A., Vimal, V., Gupta, A. K., Bhujbal, S. K., Biswas, J. K., et al. (2022). Biochar application for greenhouse gas mitigation, contaminants immobilization and soil fertility enhancement: a state-of-the-art review. Sci. Total Environ. 853, 158562. doi:10.1016/j.scitotenv.2022.158562
Abrol, V., Ben-Hur, M., Verheijen, F. G. A., Keizer, J. J., Martins, M. A. S., Tenaw, H., et al. (2016). Biochar effects on soil water infiltration and erosion under seal formation conditions: rainfall simulation experiment. J. Soils Sediments 16, 2709–2719. doi:10.1007/s11368-016-1448-8
Agegnehu, G., Bass, A. M., Nelson, P. N., and Bird, M. I. (2016). Benefits of biochar, compost and biochar–compost for soil quality, maize yield and greenhouse gas emissions in a tropical agricultural soil. Sci. Total Environ. 543, 295–306. doi:10.1016/j.scitotenv.2015.11.054
Ahmadi, S. H., Ghasemi, H., and Sepaskhah, A. R. (2020). Rice husk biochar influences runoff features, soil loss, and hydrological behavior of a loamy soil in a series of successive simulated rainfall events. CATENA 192, 104587. doi:10.1016/j.catena.2020.104587
Alkharabsheh, H. M., Seleiman, M. F., Battaglia, M. L., Shami, A., Jalal, R. S., Alhammad, B. A., et al. (2021). Biochar and its broad impacts in soil quality and fertility, nutrient leaching and crop productivity: a review. Agronomy 11 (5), 993. doi:10.3390/agronomy11050993
Bhattacharyya, R., Ghosh, B., Mishra, P., Mandal, B., Rao, C., Sarkar, D., et al. (2015). Soil degradation in India: challenges and potential solutions. Sustainability 7 (4), 3528–3570. doi:10.3390/su7043528
Bista, P., Ghimire, R., Machado, S., and Pritchett, L. (2019). Biochar effects on soil properties and wheat biomass vary with fertility management. Agronomy 9 (10), 623. doi:10.3390/agronomy9100623
Blanco-Canqui, H. (2017). Biochar and soil physical properties. Soil Sci. Soc. Am. J. 81 (4), 687–711. doi:10.2136/sssaj2017.01.0017
Blanco-Canqui, H. (2021). Does biochar application alleviate soil compaction? Review and data synthesis. Geoderma 404, 115317. doi:10.1016/j.geoderma.2021.115317
Cai, W., Huang, H., Chen, P., Huang, X., Gaurav, S., Pan, Z., et al. (2020). Effects of biochar from invasive weed on soil erosion under varying compaction and slope conditions: comprehensive study using flume experiments. Biomass Conv. bioref. 14, 5771–5790. doi:10.1007/s13399-020-00943-3
Chan, K. Y., Van Zwieten, L., Meszaros, I., Downie, A., and Joseph, S. (2008). Using poultry litter biochars as soil amendments. Aust. J. Soil Res. 46, 437–444. doi:10.1071/SR08036
Chen, L., Rejesus, R. M., Aglasan, S., Hagen, S. C., and Salas, W. (2022). The impact of cover crops on soil erosion in the US Midwest. J. Environ. Manag. 324, 116168. doi:10.1016/j.jenvman.2022.116168
Chen, L., Yu, Z., Xu, H., Wan, K., Liao, Y., and Ma, X. (2019). Microwave-assisted co-pyrolysis of Chlorella vulgaris and wood sawdust using different additives. Bioresour. Technol. 273, 34–39. doi:10.1016/j.biortech.2018.10.086
Curaqueo, G., Meier, S., Khan, N., Cea, M., and Navia, R. (2014). Use of biochar on two volcanic soils: effects on soil properties and barley yield. J. Soil Sci. Plant Nutr., 0. ahead, 0–0. doi:10.4067/S0718-95162014005000072
Das, S., Mohanty, S., Sahu, G., Rana, M., and Pilli, K. (2021). “Biochar: a sustainable approach for improving soil health and environment,” in Soil erosion - current challenges and future perspectives in a changing world. London, United Kingdom: (IntechOpen). doi:10.5772/intechopen.97136
Deng, C., Zhang, G., Liu, Y., Nie, X., Li, Z., Liu, J., et al. (2021). Advantages and disadvantages of terracing: a comprehensive review. Int. Soil Water Conservation Res. 9 (3), 344–359. doi:10.1016/j.iswcr.2021.03.002
Ding, Y., Liu, Y., Liu, S., Li, Z., Tan, X., Huang, X., et al. (2016). Biochar to improve soil fertility. A review. Agron. Sustain. Dev. 36 (2), 36. doi:10.1007/s13593-016-0372-z
Domingues, R. R., Sánchez-Monedero, M. A., Spokas, K. A., Melo, L. C. A., Trugilho, P. F., Valenciano, M. N., et al. (2020). Enhancing cation exchange capacity of weathered soils using biochar: feedstock, pyrolysis conditions and addition rate. Agronomy 10 (6), 824. doi:10.3390/agronomy10060824
Fahad, S., Chavan, S. B., Chichaghare, A. R., Uthappa, A. R., Kumar, M., Kakade, V., et al. (2022). Agroforestry systems for soil health improvement and maintenance. Sustainability 14 (22), 14877. doi:10.3390/su142214877
Feng, Q., Chen, M., Wu, P., Zhang, X., Wang, S., Yu, Z., et al. (2022). Calcium alginate-biochar composite as a novel amendment for the retention and slow-release of nutrients in karst soil. Soil Tillage Res. 223, 105495. doi:10.1016/j.still.2022.105495
Gamage, A., Gangahagedara, R., Gamage, J., Jayasinghe, N., Kodikara, N., Suraweera, P., et al. (2023). Role of organic farming for achieving sustainability in agriculture. Farming Syst. 1 (1), 100005. doi:10.1016/j.farsys.2023.100005
Genesio, L., Miglietta, F., Baronti, S., and Vaccari, F. P. (2015). Biochar increases vineyard productivity without affecting grape quality: results from a four years field experiment in Tuscany. Agric. Ecosyst. Environ. 201, 20–25. doi:10.1016/j.agee.2014.11.021
Gholamahmadi, B., Jeffery, S., Gonzalez-Pelayo, O., Prats, S. A., Bastos, A. C., Keizer, J. J., et al. (2023). Biochar impacts on runoff and soil erosion by water: a systematic global scale meta-analysis. Sci. Total Environ. 871, 161860. doi:10.1016/j.scitotenv.2023.161860
Gholami, L., Karimi, N., and Kavian, A. (2019). Soil and water conservation using biochar and various soil moisture in laboratory conditions. CATENA 182, 104151. doi:10.1016/j.catena.2019.104151
Glaser, B., Haumaier, L., Guggenberger, G., and Zech, W. (2001). The “Terra Preta” phenomenon: a model for sustainable agriculture in the humid tropics. Naturwissenschaften 88 (1), 37–41. doi:10.1007/s001140000193
Glaser, B., Lehmann, J., and Zech, W. (2002). Ameliorating physical and chemical properties of highly weathered soils in the tropics with charcoal—a review. Biol. Fertil. Soils 35, 219–230. doi:10.1007/s00374-002-0466-4
Hao, Z., Dong, Z., Han, S., and Zhang, A. (2022). Effects of biochar and arbuscular mycorrhizal fungi on winter wheat growth and soil N2O emissions in different phosphorus environments. Front. Plant Sci. 13, 1069627. doi:10.3389/fpls.2022.1069627
Herath, H. M. S. K., Camps-Arbestain, M., and Hedley, M. (2013). Effect of biochar on soil physical properties in two contrasting soils: an Alfisol and an Andisol. Geoderma 209–210, 188–197. doi:10.1016/j.geoderma.2013.06.016
Hou, D. (2021). Biochar for sustainable soil management. Soil Use Manag. 37 (1), 2–6. doi:10.1111/sum.12693
Hua, L., Lu, Z., Ma, H., and Jin, S. (2014). Effect of biochar on carbon dioxide release, organic carbon accumulation, and aggregation of soil. Environ. Prog. Sustain. Energy 33 (3), 941–946. doi:10.1002/ep.11867
Issaka, S., and Ashraf, M. A. (2017). Impact of soil erosion and degradation on water quality: a review. Geol. Ecol. Landscapes 1 (1), 1–11. doi:10.1080/24749508.2017.1301053
Jia, Q., Sun, J., Gan, Q., Shi, N., and Fu, S. (2024). Zea mays cultivation, biochar, and arbuscular mycorrhizal fungal inoculation influenced lead immobilization. Microbiol. Spectr., e0342723. doi:10.1128/spectrum.03427-23
Jiao, Y., Zhu, G., Meng, G., Lu, S., Qiu, D., Lin, X., et al. (2023). Estimating non-productive water loss in irrigated farmland in arid oasis regions: based on stable isotope data. Agric. Water Manag. 289, 108515. doi:10.1016/j.agwat.2023.108515
Jien, S.-H., and Wang, C.-S. (2013). Effects of biochar on soil properties and erosion potential in a highly weathered soil. CATENA 110, 225–233. doi:10.1016/j.catena.2013.06.021
Kameyama, K., Miyamoto, T., Shiono, T., and Shinogi, Y. (2012). Influence of sugarcane bagasse-derived biochar application on nitrate leaching in calcaric dark red soil. J. Environ. Qual. 41 (4), 1131–1137. doi:10.2134/jeq2010.0453
Kochanek, J., Soo, R. M., Martinez, C., Dakuidreketi, A., and Mudge, A. M. (2022). Biochar for intensification of plant-related industries to meet productivity, sustainability and economic goals: a review. Resour. Conservation Recycl. 179, 106109. doi:10.1016/j.resconrec.2021.106109
Koudahe, K., Allen, S. C., and Djaman, K. (2022). Critical review of the impact of cover crops on soil properties. Int. Soil Water Conservation Res. 10 (3), 343–354. doi:10.1016/j.iswcr.2022.03.003
Lee, S. S., Shah, H. S., Awad, Y. M., Kumar, S., and Ok, Y. S. (2015). Synergy effects of biochar and polyacrylamide on plants growth and soil erosion control. Environ. Earth Sci. 74, 2463–2473. doi:10.1007/s12665-015-4262-5
Lehmann, J., Pereira da Silva, J., Steiner, C., Nehls, T., Zech, W., and Glaser, B. (2003). Nutrient availability and leaching in an archaeological Anthrosol and a Ferralsol of the Central Amazon basin: fertilizer, manure and charcoal amendments. Plant Soil 249 (2), 343–357. doi:10.1023/A:1022833116184
Li, S., and Tasnady, D. (2023). Biochar for soil carbon sequestration: current knowledge, mechanisms, and future perspectives. C 9 (3), 67. doi:10.3390/c9030067
Li, Y., Zhang, F., Yang, M., Zhang, J., and Xie, Y. (2019). Impacts of biochar application rates and particle sizes on runoff and soil loss in small cultivated loess plots under simulated rainfall. Sci. Total Environ. 649, 1403–1413. doi:10.1016/j.scitotenv.2018.08.415
Liao, R., Gao, B., and Fang, J. (2013). Invasive plants as feedstock for biochar and bioenergy production. Bioresour. Technol. 140, 439–442. doi:10.1016/j.biortech.2013.04.117
Liu, J., He, T., Yang, Z., Peng, S., Zhu, Y., Li, H., et al. (2024). Insight into the mechanism of nano-TiO2-doped biochar in mitigating cadmium mobility in soil-pak choi system. Sci. Total Environ. 916, 169996. doi:10.1016/j.scitotenv.2024.169996
Liu, J., Wang, Y., Li, Y., Peñuelas, J., Zhao, Y., Sardans, J., et al. (2023). Soil ecological stoichiometry synchronously regulates stream nitrogen and phosphorus concentrations and ratios. CATENA 231, 107357. doi:10.1016/j.catena.2023.107357
Liu, Y.-F., Liu, Y., Shi, Z.-H., López-Vicente, M., and Wu, G.-L. (2020). Effectiveness of re-vegetated forest and grassland on soil erosion control in the semi-arid Loess Plateau. CATENA 195, 104787. doi:10.1016/j.catena.2020.104787
Liu, Z., Chen, X., Jing, Y., Li, Q., Zhang, J., and Huang, Q. (2014). Impact of biochar on mineralisation of C and N from soil and willow litter and its relationship with microbial community biomass and structure. Biol. Fertil. Soils 50, 695–702. doi:10.1007/s00374-013-0884-5
Major, J., Rondon, M., Molina, D., Riha, S. J., and Lehmann, J. (2010). Maize yield and nutrition during 4 years after biochar application to a Colombian savanna oxisol. Plant Soil 333 (1–2), 117–128. doi:10.1007/s11104-010-0327-0
Mango, N., Makate, C., Tamene, L., Mponela, P., and Ndengu, G. (2017). Awareness and adoption of land, soil and water conservation practices in the Chinyanja Triangle, Southern Africa. Int. Soil Water Conservation Res. 5 (2), 122–129. doi:10.1016/j.iswcr.2017.04.003
Nathan, O. O., Monicah, M.-M., Jayne, M. N., Isaya, S., George, N., and Daniel, M. N. (2022). Nutrient and organic carbon losses by erosion, and their economic and environmental implications in the drylands of Kenya. Environ. Challenges 7, 100519. doi:10.1016/j.envc.2022.100519
Noguera, D., Rondón, M., Laossi, K. R., Hoyos, V., Lavelle, P., Cruz de Carvalho, M. H., et al. (2010). Contrasted effect of biochar and earthworms on rice growth and resource allocation in different soils. Soil Biol. biochem. 42, 1017–1027. doi:10.1016/j.soilbio.2010.03.001
Novak, J. M., Lima, I., Xing, B., Gaskin, J. W., Steiner, C., Das, K. C., et al. (2009). Characterization of designer biochar produced at different temperatures and their effects on a loamy sand. Ann. Environ. Sci. 3, 195–206.
Nyambo, P., Taeni, T., Chiduza, C., and Araya, T. (2018). Effects of maize residue biochar amendments on soil properties and soil loss on acidic hutton soil. Agronomy 8, 256. doi:10.3390/agronomy8110256
Peng, X., Tong, X., Hao, L., and Wu, F. (2019). Applicability of biochar for limiting interrill erosion and organic carbon export of sloping cropland in a semi-arid area of China. Agric. Ecosyst. Environ. 280, 68–76. doi:10.1016/j.agee.2019.04.021
Qiu, S., Yang, H., Zhang, S., Huang, S., Zhao, S., Xu, X., et al. (2023). Carbon storage in an arable soil combining field measurements, aggregate turnover modeling and climate scenarios. CATENA 220, 106708. doi:10.1016/j.catena.2022.106708
Ramdani, R., and Lounela, A. K. (2020). Palm oil expansion in tropical peatland: distrust between advocacy and service environmental NGOs. For. Policy Econ. 118, 102242. doi:10.1016/j.forpol.2020.102242
Rogers, P. M., Fridahl, M., Yanda, P., Hansson, A., Pauline, N., and Haikola, S. (2021). Socio-economic determinants for biochar deployment in the Southern Highlands of Tanzania. Energies 15 (1), 144. doi:10.3390/en15010144
Rogovska, N., Laird, D. A., Rathke, S. J., and Karlen, D. L. (2014). Biochar impact onBiochar impact on Midwestern Mollisols and maize nutrient availabilityMidwestern Mollisols and maize nutrient availability. Geoderma 230, 340–347. doi:10.1016/j.geoderma.2014.04.009
Sadeghi, S. H., Hazbavi, Z., and Harchegani, M. K. (2016). Controllability of runoff and soil loss from small plots treated by vinasse produced biochar. Sci. Total Environ. 541, 483–490. doi:10.1016/j.scitotenv.2015.09.068
Sadeghi, S. H., Kiani-Harchegani, M., Hazbavi, Z., Sadeghi, P., Angulo-Jaramillo, R., Lassabatere, L., et al. (2020). Field measurement of effects of individual and combined application of biochar and polyacrylamide on erosion variables in loess and marl soils. Sci. Total Environ. 728, 138866. doi:10.1016/j.scitotenv.2020.138866
Santibañez, F., and Santibañez, P. (2007). “Trends in land degradation in Latin America and the Caribbean, the role of climate change,” in Climate and land degradation world meteorological organization (Verlag: Springer), 65–81.
Scavo, A., Fontanazza, S., Restuccia, A., Pesce, G. R., Abbate, C., and Mauromicale, G. (2022). The role of cover crops in improving soil fertility and plant nutritional status in temperate climates. A review. Agron. Sustain. Dev. 42 (5), 93. doi:10.1007/s13593-022-00825-0
Silva, F. C., Borrego, C., Keizer, J. J., Amorim, J., and Verheijen, F. (2015). Effects of moisture content on wind erosion thresholds of biochar. Atmos. Environ. 123 (A), 121–128. doi:10.1016/j.atmosenv.2015.10.070
Tripathi, S., Srivastava, P., Devi, R. S., and Bhadouria, R. (2020). Influence of synthetic fertilizers and pesticides on soil health and soil microbiology, in Agrochemicals detection, treatment and remediation, Editor(s): M. N. Vara Prasad, Butterworth-Heinemann, pp 25–54. doi:10.1016/B978-0-08-103017-2.00002-7
Uzoma, K. C., Inoue, M., Andry, H., Fujimaki, H., Zahoor, A., and Nishihara, E. (2011). Effect of cow manure biochar on maize productivity under sandy soil condition. Soil Use Manag. 27, 205–212. doi:10.1111/j.1475-2743.2011.00340.x
Vahidi, M. J., Zahan, M. H. S., Atajan, F. A., and Parsa, Z. (2022). The effect of biochars produced from barberry and jujube on erosion, nutrient, and properties of soil in laboratory conditions. Soil Tillage Res. 219, 105345. doi:10.1016/j.still.2022.105345
Wang, D., Yuan, Z., Cai, Y., Jing, D., Liu, F., Tang, Y., et al. (2021). Characterisation of soil erosion and overland flow on vegetation-growing slopes in fragile ecological regions: a review. J. Environ. Manag. 285, 112165. doi:10.1016/j.jenvman.2021.112165
Wang, L., Ok, Y. S., Tsang, D. C. W., Alessi, D. S., Rinklebe, J., Wang, H., et al. (2020). New trends in biochar pyrolysis and modification strategies: feedstock, pyrolysis conditions, sustainability concerns and implications for soil amendment. Soil Use Manag. 36 (3), 358–386. doi:10.1111/sum.12592
Wani, I., Narde, S. R., Huang, X., Remya, N., Kushvaha, V., and Garg, A. (2023). Reviewing role of biochar in controlling soil erosion and considering future aspect of production using microwave pyrolysis process for the same. Biomass Convers. Biorefinery 13 (13), 11543–11569. doi:10.1007/s13399-021-02060-1
Wen, X., Zhen, L., Jiang, Q., and Xiao, Y. (2023). A global review of the development and application of soil erosion control techniques. Environ. Res. Lett. 18 (3), 033003. doi:10.1088/1748-9326/acbaac
Xia, R., Shi, D., Ni, S., Wang, R., Zhang, J., and Song, G. (2022). Effects of soil erosion and soil amendment on soil aggregate stability in the cultivated-layer of sloping farmland in the Three Gorges Reservoir area. Soil Tillage Res. 223, 105447. doi:10.1016/j.still.2022.105447
Yan, H., Cong, M., Hu, Y., Qiu, C., Yang, Z., Tang, G., et al. (2022). Biochar-mediated changes in the microbial communities of rhizosphere soil alter the architecture of maize roots. Front. Microbiol. 13, 1023444. doi:10.3389/fmicb.2022.1023444
Yang, W., Bradford, S., Wang, Y., Sharma, P., Shang, J., and Li, B. (2019a). Transport of biochar colloids in saturated porous media in the presence of humic substances or proteins. Environ. Pollut. 246, 855–863. doi:10.1016/j.envpol.2018.12.075
Yang, W., Shang, J., Sharma, P., Li, B., Liu, K., and Flury, M. (2019b). Colloidal stability and aggregation kinetics of biochar colloids: effects of pyrolysis temperature, cation type, and humic acid concentrations. Sci. Total Environ. 658, 1306–1315. doi:10.1016/j.scitotenv.2018.12.269
Yang, W., Wang, Y., Shang, J., Liu, K., Sharma, P., Liu, J., et al. (2017a). Antagonistic effect of humic acid and naphthalene on biochar colloid transport in saturated porous media. Chemosphere 189, 556–564. doi:10.1016/j.chemosphere.2017.09.060
Yang, W., Wang, Y., Sharma, P., Li, B., Liu, K., Liu, J., et al. (2017b). Influence of naphthalene on transport and retention of biochar colloids through saturated porous media. Colloids Surf. A Physicochem. Eng. Asp. 530, 146154. doi:10.1016/j.colsurfa.2017.07.010
Zhang, A., Cui, L., Pan, G., Li, L., Hussain, Q., Zhang, X., et al. (2010). Effect of biochar amendment on yield and methane and nitrous oxide emissions from a rice paddy from Tai Lake Plain, China. Agric. Ecosyst. Environ. 139, 469–475. doi:10.1016/j.agee.2010.09.003
Zhang, J., Zhang, S., Niu, C., Jiang, J., and Sun, H. (2022a). Positive effects of biochar on the degraded forest soil and tree growth in China: a systematic review. Phyton 91 (8), 1601–1616. doi:10.32604/phyton.2022.020323
Zhang, X., Zhao, B., Liu, H., Zhao, Y., and Li, L. (2022b). Effects of pyrolysis temperature on biochar’s characteristics and speciation and environmental risks of heavy metals in sewage sludge biochars. Environ. Technol. Innovation 26, 102288. doi:10.1016/j.eti.2022.102288
Zhao, Y., Hao, Y., Cheng, K., Wang, L., Dong, W., Liu, Z., et al. (2024). Artificial humic acid mediated migration of phosphorus in soil: experiment and modelling. CATENA 238, 107896. doi:10.1016/j.catena.2024.107896
Keywords: biochar, soil erosion, erosion control mechanisms, soil amendment, soil quality
Citation: Sharma P (2024) Biochar application for sustainable soil erosion control: a review of current research and future perspectives. Front. Environ. Sci. 12:1373287. doi: 10.3389/fenvs.2024.1373287
Received: 19 January 2024; Accepted: 15 March 2024;
Published: 27 March 2024.
Edited by:
Sören Thiele-Bruhn, University of Trier, GermanyReviewed by:
Mark Kibblewhite, Cranfield University, United KingdomAnastasiah Ngigi, Multimedia University of Kenya, Kenya
Copyright © 2024 Sharma. This is an open-access article distributed under the terms of the Creative Commons Attribution License (CC BY). The use, distribution or reproduction in other forums is permitted, provided the original author(s) and the copyright owner(s) are credited and that the original publication in this journal is cited, in accordance with accepted academic practice. No use, distribution or reproduction is permitted which does not comply with these terms.
*Correspondence: Prabhakar Sharma, prabhakar.sharma@gmail.com