Opportunities and challenges to improve carbon and greenhouse gas budgets of the forest industry through better management of pulp and paper by-products
- 1Département Science et Technologie, Téluq, Université du Québec, Québec, QC, Canada
- 2Centre d’étude de la Forêt, Université du Québec à Montréal, Montréal, QC, Canada
- 3Centre de Recherche sur les Matériaux Renouvelables, Département des Sciences du bois et de la forêt, Université Laval, Québec, QC, Canada
- 4Canadian Wood Fibre Centre, Canadian Forest Service, Natural Resources Canada, Québec, QC, Canada
- 5Centre d’étude de la forêt, Faculté de Foresterie, de Géographie et de Géomatique, Université Laval, Québec, QC, Canada
Developing land use strategies to optimize carbon sinks and improve carbon footprints involves proposing efficient nature-based solutions that industries and businesses can implement while considering financial and legislative constraints. The pulp and paper industry is associated with significant greenhouse gas (GHG) emissions, primarily due to the substantial carbon dioxide (CO2) footprint of its mills. Also, some forestry operations contribute to the release of carbon to the atmosphere in the form of CO2 and methane (CH4). Conversely, this industry could potentially be a significant ally in the fight against climate change by favoring forestry practices that reduce carbon emissions and increase its sequestration, namely, by adding value to industrial by-products (e.g., biosolids) instead of treating them as wastes and landfilling them. Notably, the pulp and paper industry has been seeking alternative uses of its by-products, such as fertilizers to maximize tree growth. In this paper, we identify opportunities and challenges that exist for the pulp and paper industry in regard to recycling industrial by-products to: 1) lower GHG emissions directly at the mill and 2) improve its GHG budget by increasing carbon sequestration in forests and plantations. We illustrate our analyses by describing a case study of a pulp and paper mill in southern Quebec, Canada, that uses its biosolids and other by-products as fertilizers. This case study highlights that this strategy could not only contribute to the reduction of GHGs but could also create added value and improve economic returns of forest operations.
1 Introduction
Other than innovative technologies such as carbon capture (Kätelhön et al., 2021) and utilization (Jeffry et al., 2021) as well as charge carrier dynamics (Sun et al., 2023), nature-based climate solutions (NCS) are proposed to reduce atmospheric greenhouse gases (GHGs) and mitigate climate change (Griscom et al., 2017; Seddon, 2022). Nature-based climate solutions include efforts that aim to increase the ability of ecosystems to sequester carbon and decrease GHG emissions. Applying NCS may include land-use practices such as protecting forests or proceeding to afforestation of abandoned farmlands, decommissioned mine sites or abandoned industrial fields (Bastin et al., 2019; Lewis et al., 2019; Kaarakka et al., 2021). Nature-based climate solutions may also include more subtle changes in cultural practices such as reducing the use of synthetic fertilizers and using biochar, lime, green crops and crop rotation, and mycorrhizae to reduce nitrous oxide (N2O) emissions, a potent GHG (Forster et al., 2021; Hassan et al., 2022). In agricultural contexts, implementing such NCS could also imply using less intensive tilling practices to reduce carbon loss from soils (Maia et al., 2022). Similarly, the forest industry can modify forest management practices with the goal of sequestering more carbon and lowering GHG emissions (Drever, 2021).
The pulp and paper industry, known for its energy-intensive operations (either as electricity, heat for steam production and fuel), is closely tied to a substantial carbon dioxide (CO2) footprint, contributing to 2% of direct industrial CO2 emissions in 2022 (Szabó et al., 2009; Del Rio et al., 2022; IEA, 2023), even though this industry often self-generates its electricity and heat using its residues as fuel. In the US, for example, Tomberlin et al. (2020) estimated that one metric ton of paper creates a production weighted average of 942 kg of CO2 equivalent, with 50% coming from fuels (Tomberlin et al., 2020). Globally in 2021, the pulp and paper industry emitted an average of 0.45 t of CO2 per tonne of paper produced, whereas projections suggest emissions of 0.31 t CO2 per tonne of paper by 2030 (IEA, 2022).
Moreover, the industry faces emissions from by-products such as paper biosolids (PBs) and de-inking sludges, with landfilling and incineration recognized as sources of CO2 and methane (CH4) emissions (Faubert et al., 2016). With the aim of developing a circular economy and mitigating climate change more efficiently, the pulp and paper industry has been looking at alternatives to landfilling and incineration of PBs. Notably, the industry has been using its biosolids as a soil amendment for silvicultural, agricultural and ecological restoration purposes, as well as for biorefinery products such as wood adhesives and fillers, thermoplastic composites, and sorbent materials (Pervaiz and Sain, 2015; Bilodeau-Gauthier et al., 2022; Chen et al., 2023; Grimond et al., 2023). Recycling of PBs in silviculture could increase carbon sequestration by maximizing tree growth (Bilodeau-Gauthier et al., 2022) and promoting a stable form of carbon in soils (Khlifa et al., 2023), as well as reducing GHG emissions compared to synthetic fertilizers (Chen et al., 2023). Additionally, it could avoid a significant CO2-equivalent flux to the atmosphere associated with the landfilling or incineration of PBs. This avoidance could also be conducted in a cost-effective way, while fast-growing tree plantations receiving PBs could contribute to fibre supply and help conserve habitats and biodiversity (Himes et al., 2022). In this perspective paper, we aim to identify opportunities and challenges for the pulp and paper industry in recycling its PBs to: 1) lower GHG emissions directly at the mill site, 2) improve its GHG budget by increasing carbon sequestration in forests and plantations, and 3) favor a forest land management scheme for the preservation of natural habitats and biodiversity.
2 The world problem of biosolids, with emphasis on paper biosolids
Quantifying global waste generation proves challenging due to varied definitions and methodologies. Current estimates indicate waste production at 19.8 billion tonnes annually, with 15% comprising biomass like solid wood, construction wood, paper and cardboard (Maalouf and Mavropoulos, 2023). Despite uncertainties, projections suggest a global increase to 28 and 46 billion tonnes per year by 2030 and 2050, respectively (Di Giacomo and Romano, 2022). Managing these wastes poses a significant environmental challenge, with 40% being landfilled, 19% recycled or composted, and 11% incinerated (Kaza et al., 2018). As the global population continues to grow, waste management emerges as one of the greatest environmental challenges of the 21st century (Vaverková, 2019).
Effective waste management should be guided by policies that promote reducing at the source, reusing, recycling and recovering (i.e., the 4R principle; Rada et al., 2018). However, more often than not, it involves landfilling or incineration. Because incineration is costly and a direct and significant source of GHGs, landfilling is the preferred method and is expected to increase in the coming decades. Nonetheless, the expansion of landfill sites brings environmental challenges, including increased hydraulic loads of leachates on adjacent watercourses, air quality issues (e.g., odors and suspended particles) and GHG emissions (Wang et al., 2014; Zhang et al., 2019; Siddiqua et al., 2022).
Sewage sludge, a by-product of municipal wastewater treatment, is termed municipal biosolids (MBs) when meeting certain criteria for land application. These must exhibit low levels of pathogens and other contaminants such as metals, although criteria vary from country to country (LeBlanc et al., 2009; Lowman et al., 2013; Popoola et al., 2023). Global production of biosolids is projected to reach 200 million tonnes annually by 2025 (Mohajerani et al., 2017), with the pulp and paper industry being a major contributor. In 2020, 34 countries accounted for 82% of the global paper and paperboard production (402 million tonnes) (FAO, 2023), set to increase to 550 million tonnes by 2050 (Mabee and Roy, 2003). As such, the production of PBs is expected to increase proportionally. In Quebec, Canada, for example, paper mill biosolids amounted to 1 million tonne in 2018 (RECYC-QUÉBEC, 2018). The pulp and paper production process generates wastes and by-products, of which 87% is classified as pulp and paper mill sludge, whereas the remaining is considered as impurities, waste chemicals and gaseous emissions (Turner et al., 2022).
The pulp and paper industry is regulated for wastewater discharge from pulp and paper mills into surface waters. Paper biosolids, similar to MBs, are disposed by landfilling, incineration, or are applied to land for various purposes (e.g., agriculture, forestry, ecological restoration). In addition to environmental impacts, landfilling and incineration of biosolids face limited social acceptance and economic challenges, such as substantial fees. These fees aim, in part, to incentivize industries to adhere to the 4R principle before resorting to elimination (Primeau, 2014; Faubert et al., 2015). While landfilling is cost-effective compared to incineration, cost recovery options (e.g., biogas recovery for energy production) remains challenging. Consequently, the use of MBs and PBs as soil amendments or materials for reconstructing soils in restoration projects is gaining popularity.
3 Landfilling of paper biosolids
Landfilling of PBs is the most widely used disposal method, and the pulp and paper industry generally manages its own landfilling facilities (Haile et al., 2021). In addition to hazardous substances reaching adjacent watercourses and causing air quality issues (Haile et al., 2021; Siddiqua et al., 2022), landfilling sites generate large amounts of GHGs under various conditions (Wang et al., 2014; Zhang et al., 2019). However, estimates of the proportion of landfill sites dedicated to PB disposal relative to landfill sites as a whole and empirical estimates of GHG emissions from them are non-existent, making it difficult to assess their contribution to GHG emissions globally (Primeau, 2014; Faubert et al., 2016; MELCC, 2020). Faubert et al. (2016) suggested from modelling and theory that 2.69 tonnes of CO2 and 0.24 tonne of CH4 could be emitted to the atmosphere for every tonne of PBs that is landfilled. Methane emissions are a primary concern for climate change due to its high global warming potential (GWP) compared to CO2, i.e., 28 to 32 times higher over 100 years as initially suggested by Byrne and Goldblatt (2014). However, a new model (GWP*) has recently been proposed to improve calculations of warming-equivalent emissions since GWP can overestimate the cumulative effect of short-lived climate pollutants, such as CH4, by not properly considering the short-term and long-term effects of these pollutants in the atmosphere (Cain et al., 2019; Lynch et al., 2020). Methane from landfilled PBs is considered the main GHG because most of CO2 emissions could be offset by the main constituent of PBs, i.e., short lignin fibers derived from photosynthesis (Camberato et al., 2006). However, landfilled biosolids can also generate N2O through the cooxidation of ammonia by methanotrophic soil bacteria in cover soils (Zhang et al., 2009).
Methanogenesis occurs during the anaerobic mineralization of organic matter (Le Mer and Roger, 2001). Anaerobic conditions in landfill cells are created over time through the compaction of layers of organic matter (Olivier, 2013). Because PBs are rich in wood fibers and organic matter, they provide a significant carbon source for methanogenic microbes (Camberato et al., 2006). During the fermentation process, methanogenic microbes transform organic matter into CH4 and some CO2 (Le Mer and Roger, 2001). Landfill cells are typically covered with an engineered cap comprising a clay layer to limit water infiltration and gas exchange. This cap is further covered by a sandy mineral soil with low organic matter content (Handel et al., 1997; Fraser-McDonald et al., 2022). A portion of the CH4 produced is then oxidized through methanotrophy to CO2 and water in the cover soil layer, facilitated by its high oxygen levels (Boeckx et al., 1996; Le Mer and Roger, 2001) and low organic matter content (Handel et al., 1997). Methane that remains unaffected in the cover soil layer is released to the atmosphere by diffusion and plant gas exchange (Le Mer and Roger, 2001).
Cover soils thus aim to reduce CH4 emissions by creating oxygenated conditions where CH4 transforms into CO2 and water before reaching the atmosphere. However, fissures, cracks and holes in the mineral cap often form, creating preferential channels for CH4 from the organic layer to bypass oxidation and be released directly to the atmosphere (Schroth et al., 2012). Also, highly porous material can allow CH4 to flow through the cover soil too quickly for oxidation to occur before reaching the atmosphere (Wang et al., 2022). The heterogeneous nature of the cover soil often leads to high spatial variability in CH4 fluxes across a landfill site (Schroth et al., 2012). Estimates of CH4 emissions from PB landfill sites, assuming that 10% of CH4 is oxidized in the cover soil, may therefore be underestimated (Chanton et al., 2009; Schroth et al., 2012). However, membrane gas permeation has been used in recent years to channel biogas for energy use or to transform it into CO2 through flaring (Makaruk et al., 2010; Chmielewski et al., 2019). To our knowledge, however, this method has only been used in municipal landfills.
4 Paper biosolids as fertilizers
Application of MBs and PBs to agricultural soils can positively impact soil fertility and crop yields (Gagnon and Ziadi, 2012; Lu et al., 2012; Ziadi et al., 2013; Abdi et al., 2016; Sharma et al., 2017). Other than providing nitrogen and phosphorus, PBs: 1) are rich in calcium, thus addressing soil acidity issues, 2) enhance soil structure by providing organic matter (or carbon), 3) increase nutrient cycling by improving soil microbial biomass and activity and 4) increase soil cation exchange capacity due to the added organic matter and increased pH. Paper biosolids are less enriched in nitrogen and phosphorus compared to MBs and contain fewer contaminants, thus reducing environmental risks (Charbonneau et al., 2001; Gagnon et al., 2013). The high fiber content of PBs also imparts slow-nitrogen-release fertilizer properties (Gagnon and Ziadi, 2012). In forestry, the use of PBs can be particularly significant for carbon sequestration. It has the potential to stimulate tree growth by improving foliar nutrition and, consequently, accelerating carbon capture through photosynthesis (Lteif et al., 2007; Rodriguez et al., 2018; Bilodeau-Gauthier et al., 2022). However, further work is necessary to assess how fertilization with PBs can influence carbon sequestration of forest plantations under different climates, site conditions (e.g., soils), site (mechanical) preparation and weed management. To our knowledge, no effort was made in synthesizing such data.
Fertilization with PBs can decrease GHG emissions when compared to synthetic fertilizers such as urea (Chen et al., 2023). Furthermore, the use of PBs in agriculture and forestry diverts biosolids from landfills, thereby reducing associated CH4 emissions (Faubert et al., 2019). Considering these factors, repurposing PBs from the pulp and paper industry as fertilizers in silviculture emerges not only as a theoretical concept but also as a practical NCS. To illustrate this potential, we present a case study from southern Quebec. This case study demonstrates how the purposeful use of PBs in silvicultural treatments can effectively contribute to sustainable waste management practices and significantly enhance carbon sequestration efforts (Figure 1).
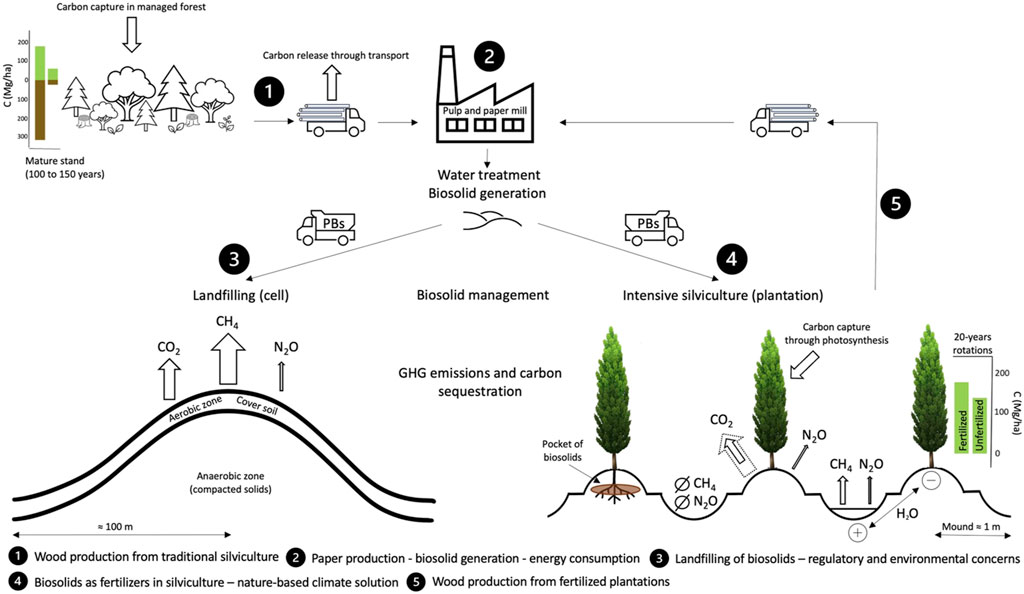
Figure 1. Conceptual diagram of paper biosolids management methods. The size of GHG emissions arrows does not accurately represent differences between disposal methods but indicates variations within each method. Solid arrows refer to greenhouse gases emitted from biosolids, whereas the dotted arrow for CO2 represents emitted by background (natural) soil respiration. Soil and tree biomass carbon stocks from unpublished data for mature managed deciduous forests (K. Lafore) and hybrid poplar plantations (S. Lebel-Desrosiers) in southern Quebec, Canada. For the latter, soil carbon stocks are not yet available.
In this case study, PBs are specifically used to fertilize fast-growing hybrid poplar (e.g., Populus x canadensis × P. maximowiczii) plantations, classified as an intensive silvicultural practice. Trees are planted on mound which are created with an excavator (Figure 1). Using allometric equations from the R software package “allodb” (Gonzalez-Akre et al., 2022), it was estimated that, over a 20-year period, plantations receiving industrial by-products could sequester up to 26.7% more carbon than unfertilized plantations (treated: 171 Mg C ha−1; unfertilized: 134 Mg C ha−1). Achieving a target of 200 m3 ha−1 for merchantable wood could be realized, on average, in about 20 years in fertilized plantations (compared to 140 m3 ha−1 in unfertilized plantations), allowing faster rotations and higher carbon sequestration in the long term. In comparison, wood biomass (aboveground) in twenty-one mature (∼100–150 years) deciduous forests and mixedwood stands in southern Quebec holds between 38 and 184 Mg ha−1 of carbon (K. Lafore, unpublished data).
It could be argued that this wood biomass is destined for paper, a product with a short life span and limited recycling cycles, resulting in fewer benefits in terms of carbon emission mitigation. Yet, augmenting yields in forest plantations destined for paper production also has the added benefit of relieving pressure on other forests for wood supply. This, in turn, leads to the protection of larger swaths of forest landscapes supporting the provision of other ecosystem services (Wang et al., 2022).
Soil carbon stocks are expected to substantially increase after PB application due to their high organic carbon content and carbon:nitrogen ratio (Henry et al., 1994; Gagnon and Ziadi, 2012). At an application rate of about 125 Mg ha−1, the newly added carbon (approximately 50–60 Mg C ha−1) is anticipated to become a stable long-term storage in forest soils, similar to observations in ecological restoration projects, some being afforestation efforts, using MBs for soil reconstruction (Trlica and Teshima, 2011; Carbassa et al., 2020; Khlifa et al., 2023). These afforestation projects can also lead to significant tree growth and carbon sequestration as well (e.g., Grimond et al., 2023; Bélanger et al., 2024). Soil carbon improvement results from ‘pockets’ of PBs trapped at the base of the mounds during their creation and due to limited mixing, along with the aggregation of organic carbon to clay-mineral particulates. The stability of these carbon pools is largely influenced by the chemical signature of the carbon forms in the PBs, particularly the presence of more labile carbohydrates (Gagnon and Ziadi, 2022).
In the mid-term, increased tree growth is expected to further enhance soil carbon stocks via increased litter flux, including roots, to the soil (Thevathasan and Gordon, 1997; Arevalo et al., 2011), promoting forest floor development and carbon accumulation in mineral horizons. Preliminary data suggest that forest floor depth of the southern Quebec case study sites increased from an average of 1.7–5 cm in just 12 years for plantations receiving PBs, while no change in forest floor depth was observed in unfertilized plantations over 14 years. The soil organic content of boreal, mixed and deciduous forest soils in Quebec is, on average, 44 Mg C ha−1 (Tremblay and Ouimet, 2000). Less than 3% of the forests exhibited soil organic content (SOC) above 100 Mg C ha−1 in this study. Similarly, a very large variation in soil carbon stocks (2–315 Mg ha−1) was measured for old-growth forest stands in southern Quebec (K. Lafore, unpublished data). Although estimating the amount of this new carbon is challenging, a forest floor in steady state in these temperate forests typically reaches a depth of 5–15 cm and is 25%–40% of the soil carbon stock. The expectation is that this steady state will be reached more rapidly in the plantations receiving PBs.
Another key, yet complex aspect to consider, is the potential reduction in GHG emissions by diverting PBs from landfills and applying them to plantations. It is anticipated that applying relatively small amounts of PBs at the soil surface, without or with very limited compaction and under well-aerated soil conditions, will significantly lower overall CH4 and N2O emissions compared to landfilling. However, spatial variation in fluxes is expected to be large, correlating with field microtopography associated with mounding. Mounding creates a heterogeneous soil surface with: 1) dry zones characterized by lower litter accumulation (mounds), 2) unaltered areas with soil moisture levels and litter accumulation rates expected from a well-drained forest soil (ground level), and 3) wetter zones with substantial litter accumulation (depressions) that could contribute to CH4 and N2O emissions. In fact, like methanogenesis, denitrification rates are higher in compacted and poorly drained soils (Beare et al., 2009). Considering that wet depressions represent about one-third of the planted landscape, GHG emissions under this microtopography should be assessed. Preliminary summer sampling at the case study site suggests that CH4 fluxes are present in only some depressions and CO2 fluxes were lower in (wet) depressions and on (dry) mounds (287–356 mg m−2 h−1) than on unaltered soil (701 mg m−2 h−1).
The values above suggest no significant change in CO2 fluxes due to mounding or PB application, as they are within the range of those measured in comparable forest stands under the same soil temperatures (Bélanger et al., 2021). Similarly, our preliminary data suggest that PB application does not lead to the production of N2O across the mounding microtopography. Chen et al. (2023) observed the opposite after PB application in hybrid poplar plantations in Alberta, i.e., a 21% and 17% increase in CO2 and N2O emissions, but no change in CH4 emissions. These sites appear to show less microtopography (prepared by one-way cultivation). Measurements of the southern Quebec case study sites were taken 3 years after PB application, while fresh and wet biosolids are known to be significant sources of N2O (Roman-Perez and Hernandez-Ramirez, 2022). The duration of these large emissions is not well known, especially in forest soils; this deserves our full attention because the benefits that could be gained by reducing landfill CH4 emissions and increasing carbon sequestration with the use of PBs in hybrid poplar plantations could be offset if this practice also causes N2O emissions for a prolonged period. Further monitoring of soil conditions and gas efflux is thus required to determine if carbon accumulates in the new soils and to assess GHG dynamics to fully evaluate their potential as a climate solution.
5 Conclusion
Management of waste materials from the pulp and paper industry often involves landfilling. However, our preliminary results offer a promising perspective for the enhanced recovery and recycling of PBs, leading to reduced GHG emissions and improved carbon sequestration when combined with appropriate silvicultural practices. As more field data are collected to better quantify landfill CH4 emissions, diverting PBs from landfills to plantations could prove valuable for the forest sector to contribute to CH4 emissions mitigation targets.
Despite the promising benefits of applying biosolids in forestry, challenges must be addressed. Environmental and regulatory concerns, including the potential for heavy metal accumulation and pathogen transmission, require careful management (Pöykiö et al., 2007; Lu et al., 2012). Among the pollutants of concerns are perfluoroalkylated substances, synthetic chemicals such as perfluorooctane sulfonate and perfluorooctanoic acid (PFAS), which pose health risks (Kirk et al., 2018; Ehrlich et al., 2023; van Larebeke et al., 2023). Communities near landfill sites have raised public health concerns, including quality-of-life impacts due to odors (Lowman et al., 2013). Moreover, it is not yet clear how the wastewater treatment processes impact the concentration of PFAS in sludges and biosolids (Behnami et al., 2024; Gewurtz et al., 2024). Therefore, the development of guidelines and monitoring systems is essential to ensure that the use of biosolids adheres to environmental safety standards and does not adversely affect forest ecosystems or human health.
Data availability statement
The datasets presented in this article are not readily available because this is a perspective paper only and we report preliminary data only. When full data are published, they will be made available publicly. Requests to access the datasets should be directed to nicolas.belanger@teluq.ca.
Author contributions
SL: Conceptualization, Data curation, Formal Analysis, Investigation, Methodology, Writing–original draft, Writing–review and editing. BC: Conceptualization, Formal Analysis, Investigation, Methodology, Writing–original draft, Writing–review and editing. AL: Conceptualization, Investigation, Methodology, Writing–original draft, Writing–review and editing. SL: Conceptualization, Formal Analysis, Investigation, Methodology, Writing–original draft, Writing–review and editing, Data curation. KL: Data curation, Conceptualization, Formal Analysis, Investigation, Methodology, Writing–original draft, Writing–review and editing. ET: Funding acquisition, Project administration, Resources, Supervision, Conceptualization, Formal Analysis, Investigation, Methodology, Writing–original draft, Writing–review and editing. NT: Funding acquisition, Project administration, Resources, Supervision, Conceptualization, Formal Analysis, Investigation, Methodology, Writing–original draft, Writing–review and editing. NB: Validation, Conceptualization, Investigation, Methodology, Writing–original draft, Writing–review and editing, Data curation, Formal Analysis, Funding acquisition, Project administration, Resources, Supervision.
Funding
The authors declare that financial support was received for the research, authorship, and/or publication of this article. This research was funded by the Natural Sciences and Engineering Research Council of Canada through an Alliance grant (reference ALLRP 572865-22) to NB, ET, and NT as well as by the Fonds de recherche du Québec Science et technologie through an Appui aux réseaux d’innovation grant (reference 2020-RI-278290) to NB and NT.
Conflict of interest
The authors declare that the research was conducted in the absence of any commercial or financial relationships that could be construed as a potential conflict of interest.
Publisher’s note
All claims expressed in this article are solely those of the authors and do not necessarily represent those of their affiliated organizations, or those of the publisher, the editors and the reviewers. Any product that may be evaluated in this article, or claim that may be made by its manufacturer, is not guaranteed or endorsed by the publisher.
References
Abdi, D., Ziadi, N., Shi, Y., Gagnon, B., Lalande, R., and Hamel, C. (2016). Residual effects of paper mill biosolids and liming materials on soil microbial biomass and community structure. Can. J. Soil Sci. doi:10.1139/CJSS-2016-0063
Arevalo, C. B. M., Bhatti, J. S., Chang, S. X., and Sidders, D. (2011). Land use change effects on ecosystem carbon balance: from agricultural to hybrid poplar plantation. Agric. Ecosyst. Environ. 141, 342–349. doi:10.1016/j.agee.2011.03.013
Bastin, J.-F., Finegold, Y., Garcia, C., Mollicone, D., Rezende, M., Routh, D., et al. (2019). The global tree restoration potential. Science 365 (6448), 76–79. doi:10.1126/science.aax0848
Beare, M. H., Gregorich, E. G., and St-Georges, P. (2009). Compaction effects on CO2 and N2O production during drying and rewetting of soil. Soil Biol. Biochem. 41 (3), 611–621. doi:10.1016/j.soilbio.2008.12.024
Behnami, A., Zoroufchi Benis, K., Pourakbar, M., Yeganeh, M., Esrafili, A., and Gholami, M. (2024). Biosolids, an important route for transporting poly- and perfluoroalkyl substances from wastewater treatment plants into the environment: a systematic review. Sci. total Environ. 925, 171559. doi:10.1016/j.scitotenv.2024.171559
Bélanger, N., Collin, A., Khlifa, R., and Lebel-Desrosiers, S. (2021). Balsam fir and American beech influence soil respiration rates in opposite directions in a sugar maple forest near its northern range limit. Front. For. Glob. Change 4, 664584. doi:10.3389/ffgc.2021.664584
Bélanger, N., Grimond, L., Khlifa, R., Bilodeau-Gauthier, S., and Rivest, D. (2024). “Afforestation of former asbestos mines in Quebec, Canada: an efficient nature-based climate solution that also leads to gains in biodiversity. Chapter 8,” in Biodiversity and ecosystem services on post-industrial land (New Jersey, U.S: Wiley publisher).
Bilodeau-Gauthier, S., Palma Ponce, G., Miquel, J.-C., Lafleur, B., Brais, S., and Bélanger, N. (2022). Growth and foliar nutrition of a hybrid poplar clone following the application of a mixture of papermill biosolids and lime mud. Can. J. For. Res. 52 (1), 117–128. doi:10.1139/cjfr-2021-0086
Boeckx, P., Cleemput, O. V., and Villaralvo, I. (1996). Methane emission from a landfill and the methane oxidising capacity of its covering soil. Soil Biol. Biochem. 28, 1397–1405. doi:10.1016/S0038-0717(96)00147-2
Byrne, B., and Goldblatt, C. (2014). Radiative forcing at high concentrations of well-mixed greenhouse gases. Geophys. Res. Lett. 41 (1), 152–160. doi:10.1002/2013GL058456
Cain, M., Lynch, J., Allen, M. R., Fuglestvedt, J. S., Frame, D. J., and Macey, A. H. (2019). Improved calculation of warming-equivalent emissions for short-lived climate pollutants. Npj Clim. Atmos. Sci. 2 (1), 29. doi:10.1038/s41612-019-0086-4
Camberato, J. J., Gagnon, B., Angers, D. A., Chantigny, M. H., and Pan, W. L. (2006). Pulp and paper mill by-products as soil amendments and plant nutrient sources. Can. J. Soil Sci. 86 (4), 641–653. doi:10.4141/S05-120
Carabassa, V., Domene, X., Díaz, E., and Alcañiz, J. M. (2020). Mid-term effects on ecosystem services of quarry restoration with Technosols under Mediterranean conditions: 10-year impacts on soil organic carbon and vegetation development. Restor. Ecol. 28 (4), 960–970. doi:10.1111/rec.13072
Chanton, J. P., Powelson, D. K., and Green, R. B. (2009). Methane oxidation in landfill cover soils, is a 10% default value reasonable? J. Environ. Qual. 38 (2), 654–663. doi:10.2134/jeq2008.0221
Charbonneau, H., Hébert, M., and Jaouich, A. (2001) Portrait de la valorisation agricole des Matières résiduelles fertilisantes au Québec – partie 2: contenu en éléments fertilisants et qualité environnementale. VECTEUR environnement, novembre 2000.
Chen, X., Thomas, B. R., Pattison, S., An, Z., and Chang, S. X. (2023). Pulp mill biosolids mitigate soil greenhouse gas emissions from applied urea and improve soil fertility in a hybrid poplar plantation. J. Environ. Manag. 344, 118474. doi:10.1016/j.jenvman.2023.118474
Chmielewski, A., Urbaniak, A., Palige, J., Roubinek, O., Wawryniuk, K., and Dobrowolski, A. (2019). Membrane installation for biogas enrichment – field tests and system simulation. Chem. Process Eng. 40 (2), 235–260. doi:10.24425/cpe.2019.126116
Del Rio, F., Sovacool, B. K., Griffiths, S., Bazilian, M., Kim, J., Foley, A. M., et al. (2022). Decarbonizing the pulp and paper industry: a critical and systematic review of sociotechnical developments and policy options. Renew. Sustain. Energy Rev. 167, 112706. doi:10.1016/j.rser.2022.112706
Di Giacomo, G., and Romano, P. (2022). Evolution and prospects in managing sewage sludge resulting from municipal wastewater purification. Energies 15 (15), 5633. doi:10.3390/en15155633
Drever, C. R., Cook-Patton, S. C., Akhter, F., Badiou, P. H., Chmura, G. L., Davidson, S. J., et al. (2021). Natural climate solutions for Canada. Sci. Adv. 7, eabd6034. doi:10.1126/sciadv.abd6034
Ehrlich, V., Bil, W., Vandebriel, R., Granum, B., Luijten, M., Lindeman, B., et al. (2023). Consideration of pathways for immunotoxicity of per- and polyfluoroalkyl substances (PFAS). Environ. Health 22 (1), 19. doi:10.1186/s12940-022-00958-5
FAO. (2023). Pulp and paper capacities, survey 2022–2024/Capacités de la pâte et du papier, enquête 2022-2024/Capacidades de pulpa y papel, estudio 2022-2024.
Faubert, P., Barnabé, S., Bouchard, S., Côté, R., and Villeneuve, C. (2016). Pulp and paper mill sludge management practices: what are the challenges to assess the impacts on greenhouse gas emissions? Resour. Conservation Recycl. 108, 107–133. doi:10.1016/j.resconrec.2016.01.007
Faubert, P., Bélisle, C. L., Bertrand, N., Bouchard, S., Chantigny, M. H., Paré, M. C., et al. (2019). Land application of pulp and paper mill sludge may reduce greenhouse gas emissions compared to landfilling. Resour. Conservation Recycl. 150, 104415. doi:10.1016/j.resconrec.2019.104415
Faubert, P., Lemay-Bélisle, C., Bertrand, N., and Bouchard, S. (2015). La gestion des biosolides des papetières au Québec: quelle serait la meilleure option pour réduire les émissions de gaz à effet de serre? Vecteur Environ.
Forster, P., Storelvmo, T., Armour, K., Collins, W., Dufresne, J.-L., Frame, D., et al. (2021). “The Earth’s energy budget, climate feedbacks, and climate sensitivity (Chapter 7),” in Climate change 2021: the physical science basis. Contribution of working group I to the sixth assessment report of the intergovernmental panel on climate change (Cambridge: Cambridge University Press).
Fraser-McDonald, A., Boardman, C., Gladding, T., Burnley, S., and Gauci, V. (2022). Methane emissions from trees planted on a closed landfill site. Waste Manag. Res. J. a Sustain. Circular Econ. 40 (11), 1618–1628. doi:10.1177/0734242X221086955
Gagnon, B., and Ziadi, N. (2012). Papermill biosolids and alkaline residuals affect crop yield and soil properties over nine years of continuous application. Can. J. Soil Sci. 92 (6), 917–930. doi:10.4141/cjss2012-026
Gagnon, B., and Ziadi, N. (2022). Soil carbohydrate and aggregation as affected by carbohydrate composition of paper mill biosolids. Can. J. Soil Sci. 102 (2), 371–384. doi:10.1139/cjss-2021-0136
Gagnon, B., Ziadi, N., Robichaud, A., and Karam, A. (2013). Metal availability following paper mill and alkaline residuals application to field crops. J. Environ. Qual. 42 (2), 412–420. doi:10.2134/jeq2012.0310
Gewurtz, S. B., Auyeung, A. S., Silva, De, Teslic, S., and Smyth, S. A. (2024). Per- and polyfluoroalkyl substances (PFAS) in Canadian municipal wastewater and biosolids: recent patterns and time trends 2009 to 2021. Sci. Total Environ. 912, 168638. doi:10.1016/j.scitotenv.2023.168638
Gonzalez-Akre, E., Piponiot, C., Lepore, M., Herrmann, V., Lutz, J. A., Baltzer, J. L., et al. (2022). Allodb: an R package for biomass estimation at globally distributed extratropical forest plots. Methods Ecol. Evol. 13 (2), 330–338. doi:10.1111/2041-210X.13756
Grimond, L., Rivest, D., Bilodeau-Gauthier, S., Khlifa, R., Elferjani, R., and Bélanger, N. (2023). Novel soil reconstruction leads to successful afforestation of a former asbestos mine in southern Quebec, Canada. New For. 55, 477–503. doi:10.1007/s11056-023-09989-3
Griscom, B. W., Adams, J., Ellis, P. W., Houghton, R. A., Lomax, G., Miteva, D. A., et al. (2017). Natural climate solutions. Proc. Natl. Acad. Sci. 114 (44), 11645–11650. doi:10.1073/pnas.1710465114
Haile, A., Gelebo, G. G., Tesfaye, T., Mengie, W., Mebrate, M. A., Abuhay, A., et al. (2021). Pulp and paper mill wastes: utilizations and prospects for high value-added biomaterials. Bioresour. Bioprocess. 8 (1), 35. doi:10.1186/s40643-021-00385-3
Handel, S. N., Robinson, G. R., Parsons, W. F. J., and Mattei, J. H. (1997). Restoration of woody plants to capped landfills: root dynamics in an engineered soil. Restor. Ecol. 5 (2), 178–186. doi:10.1046/j.1526-100X.1997.09721.x
Hassan, M. U., Aamer, M., Mahmood, A., Awan, M. I., Barbanti, L., Seleiman, M. F., et al. (2022). Management strategies to mitigate N2O emissions in agriculture. Life 12 (3), 439. doi:10.3390/life12030439
Henry, C. L., Cole, D. W., Hinckley, T. M., Harrison, R. B., and Features Submission, H. C. (1994). The use of municipal and pulp and paper sludges to increase production in forestry. J. Sustain. For. 1 (3), 41–55. doi:10.1300/J091v01n03_04
Himes, A., Betts, M., Messier, C., and Seymour, R. (2022). Perspectives: thirty years of triad forestry, a critical clarification of theory and recommendations for implementation and testing. For. Ecol. Manag. 510, 120103. doi:10.1016/j.foreco.2022.120103
Jeffry, L., Ong, M. Y., Nomanbhay, S., Mofijur, M., Mubashir, M., and Show, P. L. (2021). Greenhouse gases utilization: a review. Fuel 301, 121017. doi:10.1016/j.fuel.2021.121017
Kaarakka, L., Cornett, M., Domke, G., Ontl, T., and Dee, L. E. (2021). Improved forest management as a natural climate solution: a review. Ecol. Solutions Evid. 2 (3), e12090. doi:10.1002/2688-8319.12090
Kätelhön, A., Meys, R., Deutz, S., Suh, S., and Bardow, A. (2021). Climate change mitigation potential of carbon capture and utilization in the chemical industry. PNAS 116 (23), 11187–11194. doi:10.1073/pnass.1821029116
Kaza, S., Yao, L. C., Bhada-Tata, P., and Van Woerden, F. (2018) What a waste 2.0: a global snapshot of solid waste management to 2050. Washington, DC: World Bank. doi:10.1596/978-1-4648-1329-0
Khlifa, R., Rivest, D., Grimond, L., and Bélanger, N. (2023). Stability of carbon pools and fluxes of a Technosol along a 7-year reclamation chronosequence at an asbestos mine in Canada. Ecol. Eng. 186, 106839. doi:10.1016/j.ecoleng.2022.106839
Kirk, M., Smurthwaite, K., Braunig, J., Trevenar, S., D’Este, C., Lucas, R., et al. (2018) The PFAS health study: systematic literature review [Application/pdf], 256 pages. doi:10.25911/KW6T-7H44
LeBlanc, R. J., Matthews, P., and Richard, R. P. (2009). Global atlas of excreta, wastewater sludge, and biosolids management: moving forward the sustainable and welcome uses of a global resource/. Greater Moncton Sewerage Commission. Available at: https://digitallibrary.un.org/record/660552/files/un_habitat_atlas_excreta_wastewater_sludge.pdf.
Le Mer, J., and Roger, P. (2001). Production, oxidation, emission and consumption of methane by soils: a review. Eur. J. Soil Biol. 37 (1), 25–50. doi:10.1016/S1164-5563(01)01067-6
Lewis, S. L., Wheeler, C. E., Mitchard, E. T. A., and Koch, A. (2019). Restoring natural forests is the best way to remove atmospheric carbon. Nature 568 (7750), 25–28. doi:10.1038/d41586-019-01026-8
Lowman, A., McDonald, M. A., Wing, S., and Muhammad, N. (2013). Land application of treated sewage sludge: community health and environmental justice. Environ. Health Perspect. 121 (5), 537–542. doi:10.1289/ehp.1205470
Lteif, A., Whalen, J. K., Bradley, R. L., and Camiré, C. (2007). Mixtures of papermill biosolids and pig slurry improve soil quality and growth of hybrid poplar. Soil Use Manag. 23 (4), 393–403. doi:10.1111/j.1475-2743.2007.00103.x
Lu, Q., He, Z. L., and Stoffella, P. J. (2012). Land application of biosolids in the USA: a review. Appl. Environ. Soil Sci. 2012, 1–11. doi:10.1155/2012/201462
Lynch, J., Cain, M., Pierrehumbert, R., and Allen, M. (2020). Demonstrating GWP*: a means of reporting warming-equivalent emissions that captures the contrasting impacts of short- and long-lived climate pollutants. Environ. Res. Lett. 15, 044023. doi:10.1088/1748-9326/ab6d7e
Maalouf, A., and Mavropoulos, A. (2023). Re-assessing global municipal solid waste generation. Waste Manag. Res. J. a Sustain. Circular Econ. 41 (4), 936–947. doi:10.1177/0734242X221074116
Mabee, W., and Roy, D. N. (2003). Modeling the role of papermill sludge in the organic carbon cycle of paper products. Environ. Rev. 11 (1), 1–16. doi:10.1139/a03-001
Maia, F., De Souza Medeiros, A., Santos, D., Lyra, G. B., Lal, R., Assad, E. D., et al. (2022). Potential of no-till agriculture as a nature-based solution for climate-change mitigation in Brazil. Soil Tillage Res. 220, 105368. doi:10.1016/j.still.2022.105368
Makaruk, A., Miltner, M., and Harasek, M. (2010). Membrane biogas upgrading processes for the production of natural gas substitute. Sep. Purif. Technol. 74 (1), 83–92. doi:10.1016/j.seppur.2010.05.010
Ministère de l’environnement et de la lutte contre les changements climatiques (2020). Stratégie de valorisation de la matière organique. Available at: http://www.environnement.gouv.qc.ca/matieres/organique/strategie-valorisation-matiere-organique.pdf.
Mohajerani, A., Lound, S., Liassos, G., Kurmus, H., Ukwatta, A., and Nazari, M. (2017). Physical, mechanical and chemical properties of biosolids and raw brown coal fly ash, and their combination for road structural fill applications. J. Clean. Prod. 166, 1–11. doi:10.1016/j.jclepro.2017.07.250
Pervaiz, M., and Sain, M. (2015). Recycling of paper mill biosolids: a review on current practices and emerging biorefinery initiatives. Clean. – Soil, Air, Water 43 (6), 919–926. doi:10.1002/clen.201400590
Popoola, L. T., Olawale, T. O., and Salami, L. (2023). A review on the fate and effects of contaminants in biosolids applied on land: hazards and government regulatory policies. Heliyon 9 (10), e19788. doi:10.1016/j.heliyon.2023.e19788
Pöykiö, R., Nurmesniemi, H., and Keiski, R. L. (2007). Environmental risk assessment of heavy metal extractability in a biosludge from the biological wastewater treatment plant of a pulp and paper mill. Environ. Monit. Assess. 128, 153–164. doi:10.1007/s10661-006-9301-y
Primeau, C. (2014). Modes de gestion des biosolides des usines de pâtes et papiers au Québec: Analyse comparative. Université de Sherbrooke.
Rada, E. C., Ragazzi, M., Torretta, V., Castagna, G., Adami, L., and Cioca, L. I. (2018). “Circular economy and waste to energy. Technologies and materials for renewable energy,” in Environment and sustainability: tmrees18 (Beirut: Lebanon), 030050. doi:10.1063/1.5039237
RECYC-QUÉBEC (2018). Bilan 2018 de la gestion des matières résiduelles. Available at: https://www.recyc-quebec.gouv.qc.ca/sites/default/files/documents/bilan-gmr-2018-complet.pdf.
Rodriguez, O., de Castro Andrade, G., Bellote, J., and Tomazello-Filho, M. (2018). Effect of pulp and paper mill sludge on the development of 17-year-old loblolly pine (Punus taeda L.) trees in southern Brazil. For. Ecol. Manag. 422, 179–189. doi:10.1016/j.foreco.2018.04.016
Roman-Perez, C. C., and Hernandez-Ramirez, G. (2022). Nitrous oxide production and nitrogen transformations in a soil amended with biosolids. Can. J. Soil Sci. 102 (2), 505–518. doi:10.1139/cjss-2021-0064
Schroth, M. H., Eugster, W., Gómez, K. E., Gonzalez-Gil, G., Niklaus, P. A., and Oester, P. (2012). Above- and below-ground methane fluxes and methanotrophic activity in a landfill-cover soil. Waste Manag. 32 (5), 879–889. doi:10.1016/j.wasman.2011.11.003
Seddon, N. (2022). Harnessing the potential of nature-based solutions for mitigating and adapting to climate change. Science 376 (6600), 1410–1416. doi:10.1126/science.abn9668
Sharma, B., Sarkar, A., Singh, P., and Singh, R. P. (2017). Agricultural utilization of biosolids: a review on potential effects on soil and plant grown. Waste Manag. 64, 117–132. doi:10.1016/j.wasman.2017.03.002
Siddiqua, A., Hahladakis, J. N., and Al-Attiya, W. A. K. A. (2022). An overview of the environmental pollution and health effects associated with waste landfilling and open dumping. Environ. Sci. Pollut. Res. 29 (39), 58514–58536. doi:10.1007/s11356-022-21578-z
Sun, M., Hanif, A., Wang, T., Gu, Q., and Shang, J. (2023). Ambient temperature NO2 removal by reversible NO2 adsorption on copper-based metal-organic frameworks (MOFs)-derived nanoporous adsorbents. Sep. Purif. Technol. 314, 123563. doi:10.1016/j.seppur.2023.123563
Szabó, L., Soria, A., Forsström, J., Keränen, J. T., and Hytönen, E. (2009). A world model of the pulp and paper industry: demand, energy consumption and emission scenarios to 2030. Environ. Sci. Policy 12 (3), 257–269. doi:10.1016/j.envsci.2009.01.011
Thevathasan, N. V., and Gordon, A. M. (1997). Poplar leaf biomass distribution and nitrogen dynamics in poplar-barley intercropped system in southern Ontario, Canada. Agrofor. Syst. 37 (1), 79–90. doi:10.1023/A:1005853811781
Tomberlin, K., Venditti, R., and Yao, Y. (2020). Life cycle carbon footprint analysis of pulp and paper grades in the United States using production-line-based data and integration. BioRes. 15 (2), 3899–3914. doi:10.15376/biores.15.2.3899-3914
Tremblay, S., and Ouimet, R. (2000). A model for predicting organic carbon stocked in the forest floor of mineral forest soils in Quebec. Note de recherche forestière no. 104. Direction de la recherche forestière, Ministère des ressources naturelles du Québec.
Trlica, A., and Teshima, M. (2011). Assessing soil carbon storage and climate change mitigation in biosolids mine reclamation projects. Sixth Int. Conf. Mine Clos., 551–558. doi:10.36487/acg_rep/1152_123_trlica
Turner, T., Wheeler, R., and Oliver, I. W. (2022). Evaluating land application of pulp and paper mill sludge: a review. J. Environ. Manag. 317, 115439. doi:10.1016/j.jenvman.2022.115439
Van Larebeke, N., Koppen, G., De Craemer, S., Colles, A., Bruckers, L., Den Hond, E., et al. (2023). Per- and polyfluoroalkyl substances (PFAS) and immune system-related diseases: results from the Flemish Environment and Health Study (FLEHS) 2008–2014. Environ. Sci. Eur. 35 (1), 28. doi:10.1186/s12302-023-00731-6
Vaverková, M. D. (2019). Landfill impacts on the environment— review. Geosciences 9 (10), 431. doi:10.3390/geosciences9100431
Wang, C., Zhang, W., Li, X., and Wu, J. (2022). A global meta-analysis of the impacts of tree plantations on biodiversity. Glob. Ecol. Biogeogr. 31 (3), 576–587. doi:10.1111/geb.13440
Wang, Q., Gu, X., Tang, S., Mohammad, A., Singh, D. N., Xie, H., et al. (2022). Gas transport in landfill cover system: a critical appraisal. J. Environ. Manag. 321, 116020. doi:10.1016/j.jenvman.2022.116020
Wang, X., Jia, M., Chen, X., Xu, Y., Lin, X., Kao, C. M., et al. (2014). Greenhouse gas emissions from landfill leachate treatment plants: a comparison of young and aged landfill. Waste Manag. 34 (7), 1156–1164. doi:10.1016/j.wasman.2014.02.004
Zhang, C., Guo, Y., Wang, X., and Chen, S. (2019). Temporal and spatial variation of greenhouse gas emissions from a limited-controlled landfill site. Environ. Int. 127, 387–394. doi:10.1016/j.envint.2019.03.052
Zhang, H., He, P., and Shao, L. (2009). N2O emissions at municipal solid waste landfill sites: effects of CH4 emissions and cover soil. Atmos. Environ. 43 (16), 2623–2631. doi:10.1016/j.atmosenv.2009.02.011
Keywords: biosolids, greenhouse gases, landfilling, methane, nature-based climate solutions, pulp and paper industry
Citation: Laberge S, Courcot B, Lagarde A, Lebel Desrosiers S, Lafore K, Thiffault E, Thiffault N and Bélanger N (2024) Opportunities and challenges to improve carbon and greenhouse gas budgets of the forest industry through better management of pulp and paper by-products. Front. Environ. Sci. 12:1381141. doi: 10.3389/fenvs.2024.1381141
Received: 02 February 2024; Accepted: 29 April 2024;
Published: 14 May 2024.
Edited by:
Chenxi Li, Xi’an University of Architecture and Technology, ChinaReviewed by:
Andreas Magerl, University of Natural Resources and Life Sciences Vienna, AustriaLeonardo Clavijo, Universidad de la República, Uruguay
Copyright © 2024 Laberge, Courcot, Lagarde, Lebel Desrosiers, Lafore, Thiffault, Thiffault and Bélanger. This is an open-access article distributed under the terms of the Creative Commons Attribution License (CC BY). The use, distribution or reproduction in other forums is permitted, provided the original author(s) and the copyright owner(s) are credited and that the original publication in this journal is cited, in accordance with accepted academic practice. No use, distribution or reproduction is permitted which does not comply with these terms.
*Correspondence: Nicolas Bélanger, nicolas.belanger@teluq.ca