Distribution, seasonal characteristics, ecological risks and human health risks of 9 antibiotics in the main water environment of Anhui province, China
- 1College of Resources and Environment, Anhui Agricultural University, Hefei, Anhui, China
- 2Hangzhou Academy of Agricultural Sciences, Hangzhou, Zhejiang, China
This study was performed to determine the status and ecological risk as well as provide a basis for the prevention and control of antibiotic contamination in the drinking water sources of Anhui Province. Ultra Performance Liquid Chromatography (UPLC-MS/MS) was used to measure the detection rate and concentrations of nine antibiotics, classified as sulfonamides (SAs) or tetracyclines (TCs), in water collected from 51 sampling points and from areas with different seasonal characteristics. The risks of the main antibiotics (Sulfamethoxazole (SMZ), Doxycycline (DOC), Sulfadiazine (SDZ), Sulfamerazine (SM2), Sulfadimethoxine (SDM), Doxycycline (DOC), Tetracycline (TC), Oxytetracyline (OTC), and Chlortetracycline (CTC). to the ecosystem and human beings were evaluated using risk quotients (RQs) and target hazard quotients (THQs), respectively. Nine antibiotics were detected in tap water and surface water at concentrations ranging from 1.71 ng L−1–21.92 ng L−1 and 1.54 ng L−1–78.74 ng L−1, respectively. SMZ and DOC were detected in both tap water and surface water. Their highest detection rates in tap water were 59.1% and 63.6%, respectively, and those in surface water were 81.25% and 43.8%, respectively. SDZ, SMZ, SM2, SDM, DOC, TC, OTC, and CTC were detected in the dry and flood seasons, with levels ranging from 2.43 ng L−1–49.43 ng L−1. Among the detected target antibiotics, SMZ, SM2, TC, OTC, and CTC had higher detection rates. The total concentrations of detected antibiotics were higher in fall than in the other seasons. TC and OTC present in different water sources posed a moderate risk. SDZ present in surface water posed a higher ecological risk than that present in tap water and ground water. Meanwhile, the presence of DOC in tap water and the low risk caused by SDM in surface waters should be emphasized.
1 Introduction
Antibiotics are a class of common natural or synthetic compounds used for the prevention and treatment of bacterial infections in humans and of diseases in livestock and aquatic system (Sanganyado and Gwenzi, 2019). In recent decades, antibiotic pollution has seriously threatened water ecosystems and human health, and the environmental pollution problems caused by antibiotics have aroused widespread concern in the world (Browne et al., 2021; Kadivarian et al., 2024). The absorption of antibiotics in the body is limited and about 60%–90% of antibiotics migrate to the environment in their prodrug form (Li et al., 2020). Ultimately, it enters the environment through a variety of pathways, posing a potential risk to ecosystems.
There are more than 200 types of antibiotics in the natural environment, which can be categorized into 10 major groups according to their different chemical structures and functions, among which sulfonamides (SAs), tetracyclines (TCs), quinolones (FQs), macrolides (MACs) and β-lactams are the five major groups of antibiotics consumed in China. Trace amounts of antibiotics are currently detected globally in groundwater, lakes, reservoirs, fish hatcheries and sediments, with contamination levels typically ranging from ng·L−1 to μg·L−1 (Chin et al., 2023). In recent years, more studies have been conducted on the antibiotic contamination status of China’s larger rivers, lakes, estuaries and other regions, such as the Pearl River (Li et al., 2018), Yangtze River (Li et al., 2019), Yellow River (Zhou et al., 2011) and Yellow Sea (Lang et al., 2019), where the presence of a variety of antibiotic contaminants has been reported in water bodies or sediments, with contamination concentrations ranging from the ND∼3,474 ng L−1 level. Antibiotics are present at low concentrations in water bodies, but because of their specific pharmacological functions and bioaccumulation capacity, they are likely to accumulate in the environment, a phenomenon called “pseudo persistence” (Bao et al., 2012). Water bodies are important sinks for antibiotics in the environment, and wastewater treatment plants act as the last line of defense against antibiotics entering the water systems (Zhang et al., 2018; Guo et al., 2022). The limited removal efficiency of antibiotics by traditional wastewater treatment processes has led to the widespread presence of antibiotics in the aquatic environment (Au et al., 2015). In China, antibiotics are more often being detected in surface water bodies (Liu et al., 2020; Wei et al., 2023). Currently, a number of countries in Europe and the United States have been actively working to identify aquatic antibiotic contamination pathways, sources of contamination and ecosystem damage, as well as countermeasures for contamination control, prevention and treatment (Proia et al., 2013; Hanna et al., 2018). As a country that produces and uses antibiotics on a large scale, China is faced with antibiotic contamination in the aquatic environment.
TCs and SAs antibiotics are widely used in human treatment, animal disease control and agricultural feed additives because they are broad-spectrum, stable, economical, easy to use and have good bactericidal effects. They can be transferred to water bodies by various means and can accumulate in water bodies for a long time, resulting in high residual concentrations. Drinking water intake is an important way for antibiotics to enter the human body. Therefore, antibiotic residues in drinking water sources need to be emphasized. In this study, we investigated the amount of five SAs and four TCs contaminated in the main drinking water sources in Anhui Province; not only focusing on the distribution and concentration of antibiotics in aquatic environments, but also evaluating the ecological and human health risks comprehensively, to understand the impacts of antibiotic contamination from a holistic perspective. In addition, the effects of different seasons on antibiotic concentration and distribution were considered, which helps to understand the contribution and impact of seasonal changes on antibiotic pollution in water bodies. The results of this study can extend and enrich the existing databases related to environmental monitoring and contribute to better monitoring and control of antibiotic pollution in the aquatic environment in the future. It provides a scientific basis for the development of strategies for the prevention and control of antibiotic pollution, which is invaluable to policy makers and environmental protection agencies.
2 Materials and methods
2.1 Sample collection
Anhui Province is part of the East China region, spanning the Huaihe River and Yangtze River to the north and south. It is located in the transition area between the warm temperate zone and the subtropical zone, and has a warm and humid climate with four distinct seasons. The average annual precipitation in the province is about 1,200 mm. In terms of the regional distribution, there is generally more precipitation in the south than in the north, and more in the mountains than in the plains. The precipitation in the northern part of Anhui Province is about 750–800 mm, that in the central part of Anhui Province is about 800–1,000 mm, that in the area along the river is about 1,100–1,400 mm, and that in the southern part of Anhui Province is about 1,600 mm. The total water resources of the province account for about 68 billion cubic meters. The main rivers of the province are Huaihe River, measuring 66,900 square kilometers, Yangtze River at 66,000 square kilometers, and Qiantang River at 6,500 square kilometers.
Sample collection was conducted during four different periods of sampling August and December 2011, March and May 2012, respectively. Tap water was collected from pipelines in residential areas, and surface water was collected from drinking water source protection areas. The distribution map of sampling sites is presented in Figure 1; Supplementary Table S1. Sampling Sites 1, 2, and 3 represent Shushan Lake, Dongpu, and Dafangying Reservoir water source protection zones, respectively (Supplementary Figure S1). The water samples were collected below 0.5 m of the water surface. At each site, three water samples were collected at different points using a stainless steel water collector. The samples were mixed, and 1 L was placed in a wide-mouth brown. The samples were transported back to the laboratory as soon as possible and stored at 4°C for 48 h for determination.
2.2 Sample pretreatment
A total of 0.5 L of water sample was filtered using a 0.45-μm membrane. To the filtered water, 0.5 g of disodium ethylenediaminetetraacetic acid was added, and the pH of the water sample was adjusted to 3.0 using 1 mol L−1 dilute hydrochloric acid. The Oasis HLB (500 mg, 6 mL) solid-phase extraction columns were activated with 6 mL of methanol and 12 mL of ultrapure water in turn. The water samples were then upsampled at a flow rate of ∼5–10 mL min−1. After sampling, the column was washed with 5 mL of ultrapure water, dried for 10 min, and then eluted with 6 mL of methanol. The eluate was collected in a 10 mL volumetric flask, blown to near dryness with nitrogen, dissolved in 1.0 mL of methanol-water solution with a volume ratio of 15:85, and then passed through a 0.22-μm filter membrane for analyses using the machine.
2.3 Analytical conditions
The column used was the ACQUITY Ultra Performance Liquid Chromatography (UPLC), BEH C18 column (1.7 μm, 2.1 mm, 100 mm). The mobile phase was 2:98 of methanol: water (V/V) + 0.1% formic acid for phase A and methanol + 0.1% formic acid for phase B. The flow rate was 0.15 mL min−1. The injection volume was 10 μL. The column temperature was 30°C. The gradient elution conditions are presented in Table 1.
2.4 Quality assurance and control
The quantitative analysis of the samples was performed using the internal standard method. The calibration curves of three points were selected for quantification. Working standard solutions of 5, 10, 20, 50, 100, and 500 ng L−1 were prepared, which presented a good linear relationship (r > 0.995). Blank, blank spiking, and matrix spiking were set up to ensure the feasibility of the assay method. Three blank spiked samples and three matrix spiked samples were analyzed using the same method as that of the water samples. The average recoveries of antibiotics spiked with 20, 200, and 1,000 ng L−1 ranged from 78.00% to 92.00%, 82.00%–104.00%, and 81.07%–113.59%, respectively. Their relative standard deviations (RSD) were ∼1.09%–10.18%, ∼0.18%–10.08%, and ∼1.10%–5.83%, respectively. Compared with reported methods, the current method is reproducible, has high accuracy and precision, and can meet the detection requirements.
The quantitative analysis of the samples was performed using the internal standard method. The calibration curves of three points were selected for quantification. Working standard solutions of 5, 10, 20, 50, 100, and 500 ng L−1 were prepared, which presented a good linear relationship (r > 0.995). Blank, blank spiking, and matrix spiking were set up to ensure the feasibility of the assay method. Three blank spiked samples and three matrix spiked samples were analyzed using the same method as that of the water samples. The average recoveries of antibiotics spiked with 20, 200, and 1,000 ng L−1 ranged from 78.00% to 92.00%, 82.00%–104.00%, and 81.07%–113.59%, respectively. Their relative standard deviations (RSD) were ∼1.09%–10.18%, ∼0.18%–10.08%, and ∼1.10%–5.83%, respectively. Compared with reported methods, the current method is reproducible, has high accuracy and precision, and can meet the detection requirements.
2.5 Ecological risk assessment of the antibiotics
In this study, the ecological risk assessment of typical drinking water sources in Anhui Province was carried out using the risk quotient (RQ) method according to the EU technical guidance document on environmental risk assessment (Gueriti et al., 2008). The calculation formula is as follows:
The RQ values for the antibiotics were calculated as follows Eq. 1.
where MEC is the measured environmental concentration (ng·L−1). The maximum value of the measured antibiotic concentration was selected. PNEC is the Predicted No Effect Concentration (ng·L−1). These data were obtained from the literature, and the PNEC values of the most sensitive species were selected. Due to the lack of toxicity data for MAR, FLX, and SMM, the PNEC values could not be obtained, and the ecological risk assessment was not carried out. A low risk level is defined as 0.01 ≤ RQ < 0.1, a medium risk level as 0.1 ≤ RQ < 1, and a high risk level as RQ ≥ 1 (Hernando et al., 2006).
2.6 Health risk assessment
In this study, the health risk of antibiotics in typical water sources in Anhui Province to different age groups was assessed. The primary method used for the assessment was the health risk quotient (RQH) of pollutants ingested by the drinking water route. The quantitative assessment of the impact of antibiotic contamination on health was made using the following formula Eq. 2:
where MEC is the measured concentration of antibiotics (μg·L−1). Based on the principle of maximum risk control, the maximum concentration of measured antibiotics was selected. DWEL is the equivalent value of antibiotics per liter of drinking water (μg·L−1). The formula for calculating DWEL is as follows Eqs 3, 4:
where ADI is the average acceptable daily intake (μg·kg−1·d−1). The data were obtained from the literature. HQ is the maximum risk and was calculated as the average daily intake (μg·kg−1·d−1).
HQ is the highest risk, calculated as 1; AB is the gastrointestinal absorption rate, calculated as 1; FOE is the frequency of exposure (350 days·a−1), calculated as 0.96; BW is the body mass per capita (kg); and DWI is the daily water intake (L·d−1). The BW and DWI data were obtained from the Chinese Population Exposure Parameters Manual. When RQH < 0.01, the risk level was negligible; when 0.01 < RQH < 0.1, the risk level was low; when 0.1 < RQH < 1, the risk level was moderate; and when RQH > 1, the risk level was high (Dai et al., 2021).
2.7 Statistical analysis
Sampling maps were drawn using ArcGIS 10.2. The basic data were analyzed using IBM-SPSS statistics 22. A one-way ANOVA was performed to determine the differences in antibiotic content of the drinking water sources in different areas of Anhui Province. The Turkey’s test was used to test the significance of the differences. An independent samples T-test was used to analyze the seasonal differences in the total amount of antibiotics in the drinking water sources of Anhui Province.
3 Results
3.1 Detection rate and detection value of target antibiotics in drinking water
The detection rates and detection values of target antibiotics in the drinking water sources of Anhui Province are shown in Figure 2. The detection concentrations of the eight target antibiotics showed the following trend: SDZ > SDM > SM2 > OTC > TC > SMZ > CTC > DOC. SMZ and DOC had the highest detection rates of 68.6% and 54.9%, respectively, but the mean values of the detected contents were relatively low. The detected concentrations of SDZ and SDM were 46.37 ng L−1 and 30.44 ng L−1, respectively. They were, however, detected only at five sampling points and had the lowest detection rates (9.80%).
3.2 Detection rates and values of nine antibiotics in different water sources
As shown in Figure 3A, the order of the detection rate of the nine antibiotics in tap water was DOC (63.6%) > SMZ (59.1%) > OTC (40.10%) > SM2 (14.00%) > CTC (5.5%) > TC (4.5%). SDZ, SCP, and SDM were not detected in tap water. The order of detection of the nine antibiotics in ground water was SMZ (64.3%) > DOC (50%) > SM2 (42.90%) > OTC (35.70%) > CTC (28.60%) > TC (14.000%) > SDM (7.20%) > SDZ (7.1%). SCP was not detected. The order of detection of the nine antibiotics in surface water was SMZ (81.25%) > DOC (43.80%) > SM2 (37.50%), CTC (37.50%) > SDZ (25.00%), SDM (25.00%), TC (25.00%), CTC (25.00%), and SCP was not detected.
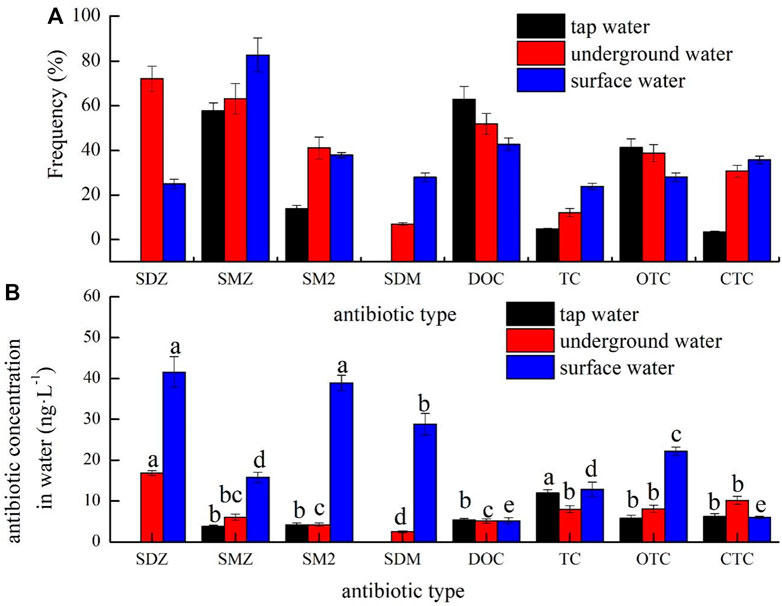
Figure 3. The concentrations (ng·L−1) and frequency (%) of 9 antibiotics in drinking water sources. (A) The detected ratio (%) of 9 antibiotics in drinking water sources. (B) The difference of the average content in drinking water sources.
As shown in Figure 3B, the average contents of SAS in different categories of drinking water sources were in the order of surface water (30.98 ng L−1) > ground water (7.49 ng L−1) > tap water (4.07 ng L−1), and the average contents of TCs in different categories of drinking water sources were in the order of surface water (30.98 ng L−1) > ground water (7.49 ng L−1) > tap water (4.07 ng L−1). The average concentrations of TCs in different categories of drinking water sources was surface water (11.59 ng L−1) > ground water (8.29 ng L−1) > tap water (8.11 ng L−1). There was little difference in the concentrations of TCs detected in ground water and tap water. There was a high level of antibiotic detection in tap water, and the order of detection was SAS (36.40%) > TCS (28.40%). For ground water, the order of detection was TCS (32.00%) > SAS (24.30%), and for surface water, it was SAS (33.75%) > TCS (32.80%).
3.3 Seasonal differences of antibiotic levels in drinking water source protection areas
The concentrations of antibiotics measured in different seasons in the Hefei drinking water source protection zones are presented in Table 2. Except for SCP, which was not detected, all the antibiotics were detected in both the dry (September, October and November) and flood seasons (April, May and June). The average values ranged between 2.43 ng L−1 and 49.43 ng L−1. In fall, SAS was detected at the range of 37.31 ng L−1–78.74 ng L−1. During the other seasons, however, SAS was detected at a lower level or not detected. TCS was not detected in the summer but was detected during the other seasons at levels ranging from 1.68 ng L−1–21.19 ng L−1. Higher levels were detected in winter. Only SAs drugs were detected in fall, but the concentration detected was higher, resulting in a higher total concentration of antibiotics detected in fall. The total concentration of antibiotics detected at Sampling Site 1 was higher than that at Sampling Sites 2 and 3. Two SAs and four TCs were detected in the winter, with a higher concentration of OTC. The total concentration of antibiotics at Sampling Site 3 was higher than that at Sampling Sites 1 and 2. In spring, only SMZ, TC, and CTC were detected, with SMZ and TC being detected at all three sampling sites and CTC being detected only at Sampling Site 2. This resulted in higher total antibiotic concentrations in Sampling Site 2 than in Sampling Sites 1 and 3. In the summer, SMZ and OTC were detected at all three sampling sites, and DOC and CTC were detected only at Sampling Site 3, resulting in higher total antibiotic concentrations in Sampling Site 3 than in Sampling Sites 1 and 2. Total antibiotic levels were higher in Sampling Site 3 than in Sampling Sites 1 and 2.
3.4 Seasonal differences in antibiotic composition
The compositional characteristics of antibiotics detected in the reservoir during the different seasons are shown in Figure 4. Four target antibiotics were detected in fall, winter, and summer, while only three target antibiotics were detected in spring. Among the detected target antibiotics, SMZ, SM2, TC, OTC, and CTC had higher detection rates.
3.5 Risk evaluation of antibiotics in drinking water source protection zones
The RQ values of seven of the nine antibiotics in tap water were in the order TC > OTC > DOC > SDZ > CTC > SM2 > SMZ. SCP and SDM were not detected (Figure 5). The RQ values of seven of the nine antibiotics in ground water were in the order TC > OTC > DOC > SDM > CTC > SMZ > SM2. SDZ and SCP were not detected. The RQ values of seven of the nine antibiotics in surface water were in the order TC > OTC > DOC > SDM > CTC > SMZ > SM2. SCP and SCP were not detected. The RQ values of eight of the nine antibiotics in surface water were in the order: TC > OTC > SDM > DOC > SDZ > SM2 > SMZ > CTC. SCP was not detected. The RQ values of TC and OTC in different water sources are clearly of concern.
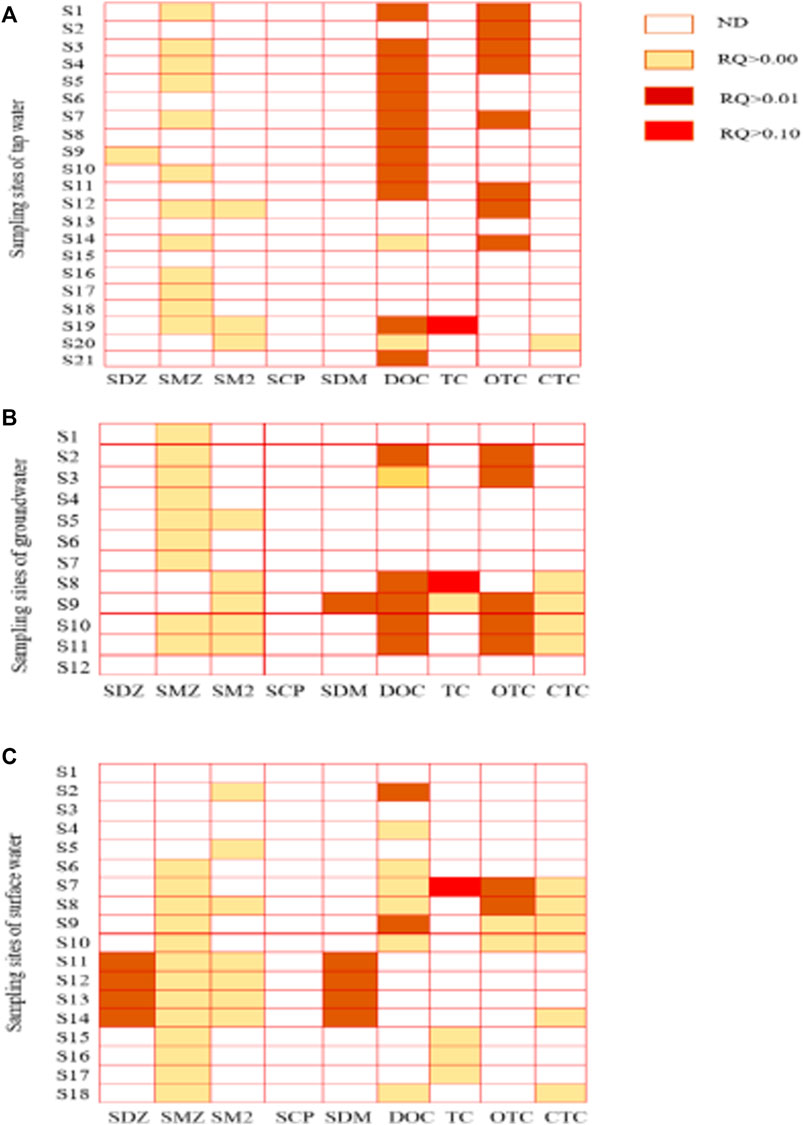
Figure 5. Risk evaluation of antibiotics in drinking water (A) tap water (B) ground water (C) surface water.
RQ values of antibiotics in different water sources were in the range of 1.00 × 10−5 to 2.41 × 10−1. The RQ values of TC were in the range of 0.85 × 10–1 to 2.41 × 10−1, with the maximum value exceeding 0.2, which is of medium risk. This medium risk of TC in tap water and surface water appeared at Sampling Points S9 and S7, respectively. In addition, we found that the RQ values of tap water at S1, S2, S3, S4, S7, S11, S12, and S14, of ground water at S2, S3, S9, S10, and S11, and of OTC in surface water at S7 and S8 were all greater than 0.01, indicating low ecological risk. The ecological risk caused by SDZ in surface water was higher than that of tap water and round water. Surface waters at S11, S12, S13, and S14, however, showed a low risk with RQ values higher than 0.01. The ecological risk caused by DOC in tap water was higher than that in ground water and surface water. In addition, the number of tap water sampling points with low risk was significantly higher than those for ground water and surface water. The RQ values of SMZ, SM2, and CTC in different water sources were less than 0.01, showing no direct ecological risk. In summary, the low risks caused by OTC and OTC in different water sources, DOC in tap water, and SDM in surface water also need to be emphasized.
3.6 Health risk assessment of antibiotics in protected drinking water sources
The health risks caused by antibiotic contamination in different water sources ranged from 1.00 × 10−3 to 7.53 × 10−3 (Figure 6). These values, which are much less than 1, indicate that the residues of these antibiotics are not apparent health hazards to human beings. Overall, the health risk values of the seven antibiotics in tap water were higher than those in surface water and ground water. The health risks of the antibiotics were all higher for adults than for children, which may be related to the higher intake by adults, and were greater for males than for females. The antibiotic with the highest risk quotient in both ground water and surface water was SM2. It had an RQH value greater than 1. The risk posed by SM2 to human health is, therefore, moderate. The RQH values of SDZ, SM2, DOC, and TC in tap water; SDZ in surface water; and DOC, TC, OTC, and CTC in ground water were greater than 0.1, indicating that the risk posed by these antibiotics to human health is low. The smallest risk quotients for tap water, ground water, and surface water were found for SMZ, SDM, and CTC, respectively.
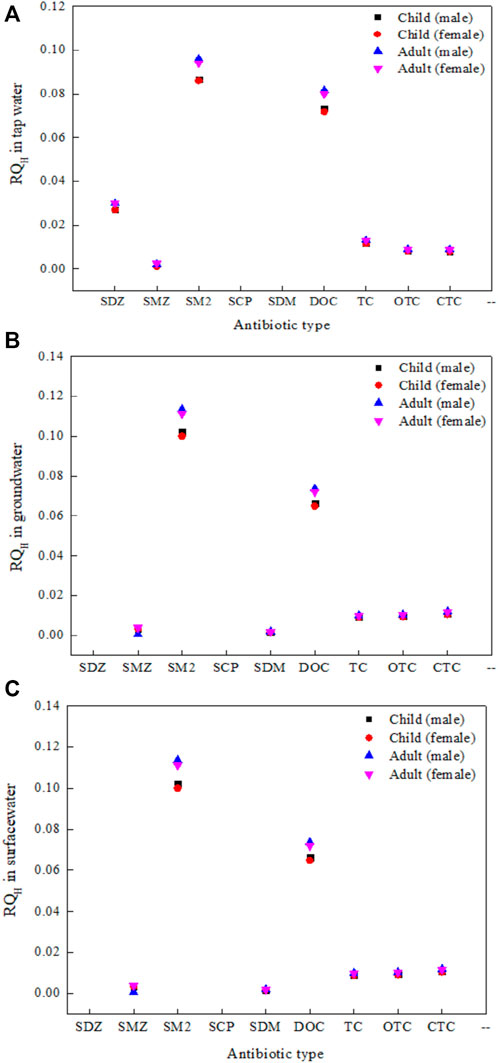
Figure 6. The RQH value of antibiotics in drinking water source protection zones (A) tap water (B) ground water (C) surface water.
4 Discussion
DOC, TC, OTC, and CTC were detected in this study but, overall, their concentrations were lower than SAs. A large amount of SAs are, therefore, being consumed and discharged in the area (Liu et al., 2020). SDZ was found to be the main SAs in the water source of the Yangtze River (Nanjing section). This is similar to our finding; that is, SDZ showed the highest detected concentration. In addition, the large presence of SAs in ground water may be attributed to their weak adsorption to soil, low biodegradability, high mobility (Strauss et al., 2011), and possible further release into wastewater and ground water through runoff or leaching (Wegst-Uhrich et al., 2014; Li et al., 2021). SAs have a highly stable and hydrophilic structure, are not easily degraded and diffuse easily in water (Li et al., 2023). TCs are widely used in livestock and aquaculture, but the concentration in the water column is relatively low, probably due to the relatively short half-life of tetracycline antibiotics and their easy adsorption by the substrate during migration. (Chen et al., 2019). The detected SAs concentrations were, therefore, higher than TCs.
SDZ is mainly used as a veterinary drug, and it is one of the SAs approved for use in aquaculture (Sarmah et al., 2006). The prominent use of SDZ in aquaculture may be related to the high detection rate in the water bodies of this study area. The use rate of SMZ in China is higher than that in other countries, such as Canada (Nasuhoglu et al., 2011). Liu et al. (2018) detected that the concentration and detection frequency of SMZ in lakes and rivers in China were high. In contrast, SMZ concentrations were low in the major water sources of Anhui Province, reflecting differences in antibiotic use in the region.
Chen et al. (2019) found that TCs and OTCs were detected at a frequency of up to 100% in the water bodies of Anqing region, while SMX and SMZ were not detected in any of the samples. QNs and TCs were detected with a higher frequency in the water and accounted for 50.8% and 36.8% of the total antibiotic concentration, respectively. The concentration and types of antibiotics in water varied, therefore, from region to region. Four types of TCs were detected in the ground water of major cities in China. The highest cumulative concentration of OTC, TC, CTC, and DXC tetracyclines was 447.11 ng L−1. The highest concentration of TCs was detected in the ground water of Jianghan Plain (605. 51 ng L−1). TCs are one of the most widely used classes of antibiotics (Hu et al., 2008; Fernando et al., 2016; Ahmed et al., 2017). They are widely used as veterinary drugs in China due to their low cost and side effects. All four TCs were detected in this study, but the detected concentrations of TCs were lower than those of SAs in the Anhui water sources. This may be due to the high adsorption and degradation capacity of TCs (Kuemmerer, 2009; Xu et al., 2014).
In this study, only a small concentration of antibiotics was detected in the ground water. This is consistent with previous reports of the influence of livestock waste on antibiotics in ground water as well as with the findings of the Xuzhou study (Bartelt-Hunt et al., 2011). The comparison shows that antibiotic concentrations in the surface water of Anhui Province are low or moderate compared to other regions. This could be attributed to fewer pollutant sources, higher temperatures, and high summer rainfall in the middle and lower reaches of the region. Summer rainfall in the middle and lower reaches of the Yangtze River Basin was low, and the concentrations of most antibiotics in Anhui Province were similar to the concentrations in Anqing and the Huaihe River Basin. Overall, the concentrations of SAs and TCs in the Hefei drinking water were low.
In this study, the total antibiotic concentrations of SAs and TCs showed the following trend: surface water > ground water > drinking water. The detection frequency of SAs were in the order drinking water > ground water > drinking water, and the antibiotic detection frequency of TCs showed a trend of surface water > ground water > drinking water. Comparing the three sources, the quality of drinking water is better. The total concentration of TCs detected was low. On the one hand, the nature of TCs in the aquatic environment is unstable. They are easily degraded by light, bacteria, and other microorganisms, and sediments can adsorb TCs, resulting in a low concentration of TCs in the aquatic environment (Lundborg and Tamhankar, 2017). On the other hand, drinking water sources are usually highly protected under the advocacy of relevant authorities. In addition, due to the dilution effect of rainwater during periods of abundant water, light can enhance photolysis and microbial metabolism, resulting in low concentrations of TCs in the region (Wang et al., 2021). More than six TCs species were detected in the ground water in Henan Province (Zhang et al., 2024), which is higher than that detected in the present study. In the present study, the detection frequency of TCs was the highest in surface water, and this may be because surface waters are more influenced by anthropogenic activities. The surface water in this study area may also have poor mobility, resulting in TCs being less easily degraded (Zhang, 2020). In the present study, the detection rate of TCs in different water sources of Anhui Province was lower than that of SAs. This may have been due to the high adsorption and degradability of TCs. Overall, the antibiotics in drinking and surface water were predominantly SAs. This finding is similar to that reported by Yan and X et al. Typically, TCs are rarely detected in natural waters, where they have a strong tendency to degrade. The detection rate of SAs in different drinking water sources was, therefore, higher than that of TCs. SAs have also been detected in many parts of the world, such as Korea, Germany, Spain, the United States of America, and Taiwan. The widespread presence of SAs in ground water may be attributed to their weak soil adsorption capacity, low biodegradability, and high mobility (Strauss et al., 2011).
In this study, lower concentrations of TCs and SAs were detected, which is mainly due to the relatively good water quality and protection of drinking water sources in Anhui Province, and the enhanced dilution effect of rainwater during the period of abundance of water, high temperatures, and strong light leading to enhanced photolysis and microbial metabolic activity, However, the types of contaminants found in this testing and detection rates should not be ignored.
5 Conclusion
In this study, nine antibiotics were detected in the drinking water sources of Anhui Province. The detection rate of SAs was higher than that of TCs. Levels of antibiotics in drinking water sources were moderate or low compared to other studies. The highest concentration of antibiotics appeared in flood seasons. Among the detected target antibiotics, SMZ, SM2, TC, OTC, and CTC had higher detection rates. Based on exposure to a single compound, the risk assessment suggested moderate risks from TC and OTC in different water sources. The ecological risks caused by SDZ in surface waters were higher than those in tap water and ground water. At the same time, the low risk caused by DOC in tap water and SDM in surface water should be emphasized. No risk to human health was found in the drinking water sources of Anhui Province. The long-term risks of antibiotics to aquatic life and human health should, however, not be ignored. Future studies should include additional explanatory variables to discuss the seasonality of antibiotic contamination and the effects of this contamination on bacterial growth or antibiotic resistance in freshwater systems. This study provides a scientific basis for the prevention and control of antibiotic pollution and provides additional data for environmental monitoring of drinking water sources.
Data availability statement
The original contributions presented in the study are included in the article/Supplementary Material, further inquiries can be directed to the corresponding author.
Author contributions
JT: Formal Analysis, Methodology, Writing–review and editing. YH: Data curation, Writing–original draft. YZ: Investigation, Supervision, Writing–original draft. XL: Funding acquisition, Resources, Writing–review and editing.
Funding
The author(s) declare that financial support was received for the research, authorship, and/or publication of this article. This study was financially supported by National Key Research and Development Plan project (2023YFD1702100) Chaohu River Basin agricultural non-point source pollution control and intelligent management and control technology integration and application. Innovation training program for college students: Effects of PFCs on Lipid in the Developing Embryos and Fry of Arctic Charr (Salvelinus alpines) S202210364202-AHAU. Studies on the Accumulation, Migration of Perfluorinated compounds in Soybean (Glycine Max L.) S202010364276-AHAU.
Acknowledgments
We thank Yuming Bian and Yuming Yan for his contribution to the linguistic embellishment of this thesis.
Conflict of interest
The authors declare that the research was conducted in the absence of any commercial or financial relationships that could be construed as a potential conflict of interest.
Publisher’s note
All claims expressed in this article are solely those of the authors and do not necessarily represent those of their affiliated organizations, or those of the publisher, the editors and the reviewers. Any product that may be evaluated in this article, or claim that may be made by its manufacturer, is not guaranteed or endorsed by the publisher.
Supplementary material
The Supplementary Material for this article can be found online at: https://www.frontiersin.org/articles/10.3389/fenvs.2024.1408363/full#supplementary-material
References
Ahmed, M. J., Islam, M. A., Asif, M., and Hameed, B. H. (2017). Human hair-derived high surface area porous carbon material for the adsorption isotherm and kinetics of tetracycline antibiotics. Bioresour. Technol. 243, 778–784. doi:10.1016/j.biortech.2017.06.174
Au, S. Y., Bruce, T. F., Bridges, W. C., and Klaine, S. (2015). Responses of Hyalella azteca to acute and chronic microplastic exposures. Environ. Toxicol. Chem. 34 (11), 2564–2572. doi:10.1002/etc.3093
Bao, Y. Y., Zhou, Q. X., Bao, Y. J., Liu, Y. X., Li, W. M., and Xie, X. J. (2012). Adsorption and desorption of three tetracycline antibiotics in petroleum-contaminated soils. China Environ. Sci. 32 (7), 1257–1262. In Chinese.
Bartelt-Hunt, S., Snow, D. D., Damon-Powell, T., and Miesbach, D. (2011). Occurrence of steroid hormones and antibiotics in shallow groundwater impacted by livestock waste control facilities. J. Contam. Hydrol. 123 (3), 94–103. doi:10.1016/j.jconhyd.2010.12.010
Browne, A. J., Chipeta, M. G., Haines-woodhouse, G., Kumaran, E. P. A., Hamadani, B. H. K., Zaraa, S., et al. (2021). Global antibiotic consumption and usage in humans, 2000-18:a spatial modelling study. Lancet Planet. Health 5 (12), e893–e904. doi:10.1016/s2542-5196(21)00280-1
Chen, Q., Ding, H. J., Zhang, W. H., Zhong, J. Y., Wu, Y. X., Li, J. L., et al. (2019). Study on the adsorption of two quinolone antibiotics by the sediment from the lakeside. Environ. Sci. Technol. In Chinese. doi:10.19672/j.cnki.1003-6504.2019.06.016
Chin, J. Y., Ahmad, A. L., and Low, S. C. (2023). Evolution of photocatalytic membrane for antibiotics degradation: perspectives and insights for sustainable environmental remediation. J. Water Process Eng. 51, 103342. doi:10.1016/j.jwpe.2022.103342
Dai, C. M., Li, S., Duan, Y. P., Leong, K. H., Ty, Y. J., Tu, Y. J., et al. (2021). Human health risk assessment of selected pharmaceuticals in the five major river basins, China. Sci. Total Environ. 2021, 149730. doi:10.1016/j.scitotenv.2021.149730
Fernando, D. M., Tun, H. M., Poole, J., Patidar, R., Li, R., Mi, R. D., et al. (2016). Detection of antibiotic resistance genes in source and drinking water samples from a first nations community in Canada. Am. Soc. Microbiol. 82, 4767–4775. doi:10.1128/aem.00798-16
Gueriti, I., Bocquen, e G., James, A., Thybaud, E., and Minier, C. (2008). Environmental risk assessment: a critical approach of the European TGD in an insitu application. Ecotoxicol. Environ. Saf. 71 (1), 291–300. doi:10.1016/j.ecoenv.2008.01.020
Guo, X. Y., Ni, N., Shi, M. L., Zhang, X. H., Yuan, A. B., Wang, N., et al. (2022). RETRACTED: the persistent, bioaccumulative, toxic, and resistance (PBTR) risk assessment framework of antibiotics in the drinking water sources. J. Environ. Manag. 326, 116776. doi:10.1016/J.JENVMAN.2022.116776
Hanna, N., Sun, P., Sun, Q., Li, X. W., Yang, X. W., Ji, X., et al. (2018). Presence of antibiotic residues in various environmental compartments of Shandong province in east-ern China: its potential for resistance development and ecological and human risk. Environ. Int. 114, 131–142. doi:10.1016/j.envint.2018.02.003
Hernando, M. D., Mezuca, M., Fernandez-alba, A. R., and Barceló, D. (2006). Environmental risk assessment of pharmaceutical residues in wastewater effluents, surface waters and sediments. Talanta 69 (2), 334–342. doi:10.1016/j.talanta.2005.09.037
Hu, J. Y., Shi, J. C., Chang, H., Li, D., Yang, M., and Kamagata, Y. C. (2008). Phenotyping and genotyping of antibiotic-resistant Escherichia coli isolated from a natural river basin. Environ. Sci. Technol. 42, 3415–3420. doi:10.1021/es7026746
Kadivarian, S., Abiri, R., Zahedi, H., Babaei, F., and Alvandi, A. (2024). Investigation the frequency of antibiotic resistance genes in drinking water samples by polymerase chain reaction in Kermanshah province. New microbes new Infect. 59 (59), 101234. doi:10.1016/j.nmni.2024.101234
Kuemmerer, K. (2009). Antibiotics in the aquatic environment-A review-Part I. Chemosphere 75, 417–434. doi:10.1016/j.chemosphere.2008.11.086
Lang, H., Chen, L., Hou, G. H., Wang, W., Zou, S., and Luo, X. (2019). Impact of coastal environmental factors on quinolone distribution in intertidal surface sediments of the Bohai Sea and Yellow Sea, China. China. Water Supply 19 (2), 482–491. doi:10.2166/ws.2018.093
Li, B. L., Zhang, H., Wang, J., Sha, X. N., Chen, X. F., and Zhuo, H. H. (2023). Pollution characteristics and risk assessment of antibiotics and resistance genes in different water sources in the Wuhan section of the Yangtze River. Environ. Sci. 44 (4), 2032–2039. doi:10.13227/j.hjkx.202204318
Li, H., Chen, Y., Feng, M. J., Wang, B., Bu, Y. B., and Zhang, S. H. (2020). Pollution characteristics and risk assessment of antibiotics in Nanjing drinking water sources. Acta Sci. Circumstantiae 40 (4), 1269–1277. In Chinese. doi:10.13671/j.hjkxxb.2019.0451
Li, L., Liu, D., Zhang, Q., Song, K., Zhou, X., Tang, Z., et al. (2019). Occurrence and ecological risk assessment of selected antibiotics in the fresh water lakes along the middle and lower reaches of Yangtze River Basin. J. Environ. Manag. 249, 109396. doi:10.1016/j.jenvman.2019.109396
Li, S., Shi, W., Li, H., Xu, N., Zhang, R., Chen, X., et al. (2018). Antibiotics in water and sediments of rivers and coastal area of zhu hai city, Pearl River estuary, south China. Sci. Total Environ. 636, 1009–1019. doi:10.1016/j.scitotenv.2018.04.358
Li, Z. P., Yu, X. P., Yu, F. R., and Huang, X. (2021). Occurrence, sources and fate of pharmaceuticals and personal care products and artificial sweeteners in groundwater. Environ. Sci. Pollut. Res. Int. 28 (17), 20903–20920. doi:10.1007/s11356-021-12721-3
Liu, X., Liu, Y., Lu, S., Guo, X., Lu, H., Qin, P., et al. (2018). Occurrence of typical antibiotics and source analysis based on PCA-MLR model in the East Dongting Lake, China. Ecotoxicol. Environ. Saf. 163, 145–152. doi:10.1016/j.ecoenv.2018.07.067
Liu, Y. H., Feng, M. J., Wang, B., Zhao, X., Guo, R. X., Bu, Y. Q., et al. (2020). Distribution and potential risk assessment of antibiotic pollution in the main drinking water sources of Nanjing, China. Environ. Sci. Pollut. Res. 27, 21429–21441. doi:10.1007/s11356-020-08516-7
Lundborg, C. S., and Tamhankar, A. J. (2017). Antibiotic residues in the environment of south east asia. BMJ 358, j2440. (Clinical Research Ed). doi:10.1136/bmj.j2440
Nasuhoglu, D., Yargeau, V., and Berk, D. (2011). Photo-removal of sulfamethoxazole (SMX) by photolytic and photocatalytic processes in a batch reactor under UV-C radiation (λmax=254nm). J. Hazard Mater 186, 67–75. doi:10.1016/j.jhazmat.2010.10.080
Proia, L., Lupini, G., OsorioV, B. D., Schwartz, T., Amalfitano, S., Fazi, S., et al. (2013). Response of biofilm bacterial communities to antibiotic pollutants in a Mediterranean River. Chemosphere 92 (9), 1126–1135. doi:10.1016/j.chemosphere.2013.01.063
Sanganyado, E., and Gwenzi, W. (2019). Antibiotic resistance in drinking water systems: occurrence, removal, and human health risks. Sci. Total Environ. 669, 785–797. doi:10.1016/j.scitotenv.2019.03.162
Sarmah, A. K., Meyer, M. T., and Boxall, A. B. (2006). A global perspective on the use, sales, exposure pathways, occurrence, fate and effects of veterinary antibiotics (VAs) in the environment. Chemosphere 65, 725–759. doi:10.1016/j.chemosphere.2006.03.026
Strauss, C., Harter, T., and Radke, M. (2011). Effects of pH and manure on transport of sulfonamide antibiotics in soil. J. Environ. Qual. 40 (5), 1652–1660. doi:10.2134/jeq2010.0535
Wang, K., Zhuang, T., Su, Z. X., Chi, M. H., and Wang, H. C. (2021). Antibiotic residues in wastewaters from sewage treatment plants and pharmaceutical industries: occurrence, removal and environmental impacts. Sci. Total Environ. 788, 147811. doi:10.1016/j.scitotenv.2021.147811
Wegst-Uhrich, S. R., Navarro, D. A., Zimmerman, L., and Aga, D. S. (2014). Assessing antibiotic sorption in soil: a literature review and new case studies on sulfonamides and macrolides. Chem. Cent. J. 8 (1), 5–12. doi:10.1186/1752-153x-8-5
Wei, F. Y., Xia, H., Huang, K., and Wei, C. C. (2023). Exogenous mobile genetic elements and their associated integrons drive the enrichment of antibiotic-resistant genes in the river of a valley basin city (Lanzhou, China). Environ. Sci. Pollut. Res. Int. 31, 3195–3206. doi:10.1007/s11356-023-31269-y
Xu, J., Zhang, Y., Zhou, C., Guo, C., Wang, D., du, P., et al. (2014). Distribution, sources and composition of antibiotics in sediment, overlying water and pore water from Taihu Lake, China. Sci. Total Environ. 497-498, 267–273. doi:10.1016/j.scitotenv.2014.07.114
Zhang, G. F. (2020). Removal rules and potential risk assessment of typical quinolone antibiotics by different water treatment processes. Water Purif. Technol. 39 (6), 106111. In Chinese. doi:10.15890/j.cnki.jsjs.2020.06.018
Zhang, P. W., Zhou, H. D., Li, K., Zhao, X. H., Liu, Q. N., Li, D. J., et al. (2018). Occurrence of pharmaceuticals and personal care products, and their associated environmental risks in a large shallow lake in North China. Environ. Geochem. Health 40 (4), 1525–1539. doi:10.1007/s10653-018-0069-0
Zhang, K., Li, K. J., Hu, F. Y., Xin, R., Fan, P. L., Lu, Y. R., et al. (2024). Occurrence characterisitics and influencing factors of antibiotic resistance genes in rural groundwater in Henan Province. Environ Sci Pollu Res Int. 31 (11), 16688–16695. doi:10.1007/s11356-024-32258-5
Zhang, Q. Q., Ying, G. G., Pan, C. G., Liu, Y. S., and Zhao, J. L. (2015). Comprehensive evaluation of antibiotics emission and fate in the river basins of China:source analysis, multimedia modeling, and linkage to bacterial resistance. Environ. Sci. Technol. 49 (11), 6772–6782. doi:10.1021/acs.est.5b00729
Keywords: water, antibiotics, detection rate, ecological risk, human health risk
Citation: Tang J, Han Y, Zha Y and Li X (2024) Distribution, seasonal characteristics, ecological risks and human health risks of 9 antibiotics in the main water environment of Anhui province, China. Front. Environ. Sci. 12:1408363. doi: 10.3389/fenvs.2024.1408363
Received: 28 March 2024; Accepted: 14 May 2024;
Published: 03 June 2024.
Edited by:
Yalçin Tepe, Giresun University, TürkiyeReviewed by:
Fikrat M. Hassan, University of Baghdad, IraqPeng Li, Shanghai Jiao Tong University, China
Copyright © 2024 Tang, Han, Zha and Li. This is an open-access article distributed under the terms of the Creative Commons Attribution License (CC BY). The use, distribution or reproduction in other forums is permitted, provided the original author(s) and the copyright owner(s) are credited and that the original publication in this journal is cited, in accordance with accepted academic practice. No use, distribution or reproduction is permitted which does not comply with these terms.
*Correspondence: Xuede Li, njqh1989@163.com