First to Flush: The Effects of Ambient Noise on Songbird Flight Initiation Distances and Implications for Human Experiences with Nature
- 1Biological Sciences, California Polytechnic State University, San Luis Obispo, CA, United States
- 2Biological Sciences, Boise State University, Boise, ID, United States
Throughout the world, birds represent the primary type of wildlife that people experience on a daily basis. However, a growing body of evidence suggests that alterations to the acoustic environment can negatively affect birds as well as humans in a variety of ways, and altered acoustics from noise pollution has the potential to influence human interactions with wild birds. Birds respond to approaching humans in a manner analogous to approaching predators, but the context of the interaction can also greatly influence the distance at which a bird initiates flight or escape behavior (i.e., flight initiation distance or FID). Here, we hypothesized that reliance on different sensory modalities to balance foraging and threat detection can influence how birds respond to approaching threats in the presence of background noise. We surveyed 12 songbird species in California and Wyoming and categorized each species into one of three foraging guilds: ground foragers, canopy gleaners, and hawking flycatchers and predicted FIDs to decrease, remain the same and increase with noise exposure, respectively. Contrary to expectations, the canopy gleaning and flycatching guilds exhibited mixed responses, with some species exhibiting unchanged FIDs with noise while others exhibited increased FIDs with noise. However, FIDs of all ground foraging species and one canopy gleaner decreased with noise levels. Additionally, we found no evidence of phylogenetic structure among species' mean FID responses and only weak phylogenetic structure for the relationship between FIDs and noise levels. Although our results provide mixed support for foraging strategy as a predictor of bird response to noise, our finding that most of the species we surveyed have shorter FIDs with increases in noise levels suggest that human observers may be able to approach ground foraging species more closely under noisy conditions. From an ecological perspective, however, it remains unclear whether these mixed responses translate into lost foraging opportunity for hypervigilant birds that flee a threat too soon or greater predation risk due to impaired surveillance for those that only respond once approaching threats are near.
Introduction
Anthropogenic noise encroaches on many natural landscapes (Barber et al., 2011; Francis et al., 2011; Lynch et al., 2011; Mennitt et al., 2013). A growing body of research indicates such noise can detrimentally affect wildlife (Barber et al., 2010; Francis and Barber, 2013; Shannon et al., 2016b) and might create a negative feedback process that degrades humans' experiences of nature (Francis et al., in review). In the context of birdwatching, an increasingly popular recreational activity (La Rouch, 2003; Carver, 2013), the ability to approach birds in the wild is a valued human experience that may be threatened by anthropogenic noise. Although there is typically a limit to how close a human can approach a wild animal before the animal initiates an escape, the distance at which flight is initiated varies across taxa and could depend on the animal's acoustic environment.
The acoustic environment serves as a critical medium through which many species, including humans, interact with their surroundings. Humans are motivated by natural sounds, such as bird songs and sounds, to seek out and experience natural places (Haas and Wakefield, 1998; Marin et al., 2011). Recent work has also demonstrated that listening to birdsong has the potential to enhance personal experiences with nature (Newman et al., 2013), improve stress recovery (Ratcliffe et al., 2013), and renew cognitive abilities after mental exertion (Abbott et al., 2016). In addition to these benefits to casual nature-seekers, birdsong is a valued tool for birdwatchers, who can acoustically localize wild birds for viewing or identification. However, in areas of elevated background sound, an observer's ability to detect birds is constrained by masking, the process through which background noise interferes with the perception of an acoustic signal (Pacifici et al., 2008; Ortega and Francis, 2012). Like humans, masking by noise can interfere with birds' abilities to detect and discriminate biologically relevant cues. For example, elevated ambient noise may influence how birds detect and respond to the threat of an approaching predator or human observer, which they perceive in the same manner (Frid and Dill, 2002). Thus, from the human perspective, where the quality of seeing and hearing a bird can depend upon the proximity of an approach, changes to the acoustic environment could indirectly influence the quality of the human experience with birds through changes in bird behavior.
Broadly speaking, anthropogenic noise can affect wildlife in many ways beyond threat detection (Francis and Barber, 2013). Previous research has demonstrated that increased noise can lead to decreased reproductive success (Halfwerk et al., 2011; Kight et al., 2012; Mulholland, 2016), impact community structure and ecological interactions (Francis et al., 2009), and degrade habitat quality (Francis et al., 2009; McClure et al., 2013; Ware et al., 2015). Most relevant to this study are the many ways that noise affects avian behavior (Shannon et al., 2016b), especially aspects of risk assessment and antipredator behavior. Karp and Root (2009) found that free-living hoatzins (Opisthocomus hoazin) increased alertness and flush more quickly when tourists approach while conversing loudly compared to silent approaches. Samia and Blumstein (2015) suggested that escape behavior in birds is largely explained by the flush early and avoid the rush (FEAR) hypothesis, which posits that prey will flee soon after predator detection to avoid costs associated with monitoring the predator. Considering this finding, it might be expected that birds should flush quickly unless threat detection is delayed or otherwise impaired due to interfering circumstances.
Flushing behavior is commonly measured as the distance between an observer and an animal when it flushes (termed “flight initiation distance,” hereafter FID) and is often used as a proxy for a species' tolerance of predators as well as the presence of humans. Previous studies have determined that species identity, starting distance, individual body size, and vegetation cover are all important predictors of FID (Fernández-Juricic et al., 2002; Blumstein, 2003; Blumstein et al., 2003). The influence of noise on FID has been investigated in two non-avian systems. Chan et al. (2010a,b) demonstrated that Caribbean hermit crabs (Coenobita clypeatus) are slower to respond to an intruder when noise is played during the approach. Based on this finding, Chan et al. (2010a) proposed the distracted prey hypothesis, which suggests that animals have finite attention and become distracted from ecologically relevant cues when a stimulus such as background noise occupies some of that attention. Shannon et al. (2016a) found the opposite outcome; black-tailed prairie dogs (Cynomys ludovicianus) flushed more quickly when a human intruder approached under higher sound levels generated from speakers broadcasting roadway noise. The prairie dogs in this study were hypervigilant, committing more time to detecting potential threats through visual surveillance when background sound levels were high (Shannon et al., 2016a). Birds are also known to increase visual alertness when their auditory abilities are impaired by ambient noise. In a study of how noise influences reproductive success, Meillère et al. (2015) found that free-living house sparrows (Passer domesticus) flee their nests more quickly (i.e., increased FID) in disturbed, noisy areas compared to quiet, control areas, possibly due to increased visual vigilance. Quinn et al. (2006) demonstrated that chaffinches (Fringilla coelebs) in a laboratory setting spent more time visually scanning for predators than actively foraging during playbacks of white noise, a stimulus not found in nature, when compared to quiet conditions. Ware et al. (2015) confirmed these findings in a study of white-crowned sparrows (Zonotrichia leucophrys) foraging during playbacks of traffic recordings, a stimulus many free-living birds experience. However, these studies introduced an acute, high intensity noise stressor to measure short-term changes in vigilance and, therefore, provide limited insight to how chronic ambient noise influences the daily lives of free-living birds. Furthermore, to our knowledge, there are no previous studies that have examined how hypervigilance exhibited in captive birds translates to detection of and response to approaching threats in nature. Thus, we sought to test whether study of bird FIDs can provide evidence to clarify the contradicting hypotheses of distraction and hypervigilance. Furthermore, we sought to examine FID responses in light of the different avian feeding ecologies that have the potential to influence detection of approaching threats via auditory and visual surveillance.
Here, we categorize our study species into three foraging guilds based on foraging behavior: ground foragers, canopy gleaners, and hawking flycatchers. We then use existing literature on the sensory ecology linked to each foraging strategy to formulate predictions of how ambient noise might influence FIDs for our foraging guilds.
Most birds rely on vision for both foraging and vigilance and can maintain vigilance while searching for food through peripheral vision and frequent movement of the head and eyes to maximize the visual field (Lima and Bednekoff, 1999; Fernández-Juricic et al., 2008). These behaviors, as well as adapted visual fields, are thought to be primarily determined by feeding ecology (Martin, 2007). Ground foraging birds generally have wide lateral visual fields and engage in frequent head movements to compensate for time spent head-down looking for food (Fernández-Juricic et al., 2008, 2010, 2011). Thus, we hypothesize that ground foraging species rely heavily on acoustic cues for threat detection while foraging and predict that they are more susceptible to the effects of masking in noisy conditions and will exhibit decreasing FIDs as noise increases (Figure 1).
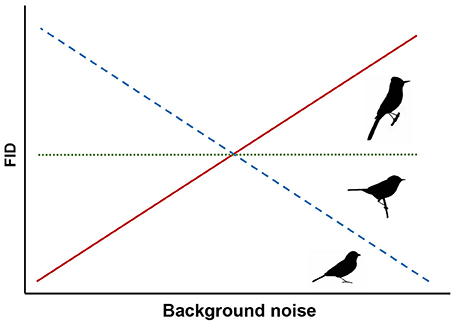
Figure 1. Predicted responses for three foraging guilds of songbirds. We expect ground foragers to have shorter FIDs in noise (dashed line), canopy gleaners to have shorter or unchanged FIDs (dashed or dotted lines), and flycatchers to have unchanged or longer FIDs in noise (dotted or solid lines).
To our knowledge, no previous study has specifically investigated the sensory ecology of species that glean arthropods and fruit in the canopy. However, like the ground foragers, canopy gleaners also rely heavily on vision to both forage and scan for predators. Other potential mechanisms of predator detection, such as con- or hetero-specific alarm calls, might also be influenced by background noise (e.g., Templeton et al., 2016) and could also contribute to slower responses of these species. Yet, because these species often forage high in the canopy rather than on the ground, we predict either a weak negative influence of noise or no change in FID in response to humans approaching at the ground level (Figure 1).
In contrast, flycatching species that sally out from a perch to catch flying insects on the wing visually scan for prey. Gall and Fernández-Juricic (2009) determined that the vision of the flycatching black phoebe (Sayornis nigricans) is primed for tracking active prey in three dimensions, but, to our knowledge, no studies have examined audition of any flycatching species in the context of foraging. With their constant visual vigilance for seeking prey, we hypothesize that these species may also be able to detect approaching threats as an epiphenomenon of scanning for volant prey. Based on this foraging strategy and the FEAR hypothesis (Samia and Blumstein, 2015), we predict flycatching species exhibit unchanged FIDs because they would flush upon first visual detection of an approaching threat while visually scanning for prey regardless of noise level (Figure 1). Alternatively, if flycatching species also compensate for reduced auditory surveillance for threats with increased visual vigilance, FIDs may increase with noise levels because more frequent visual scans for threats would lead to earlier detections (Figure 1). It is important to note that our expectation of varied responses for different foraging guilds is speculative due to limited literature on the visual fields and sensory ecology of species outside the ground foraging guild. However, we ultimately focus on the implications of ambient noise altering avian behavior in the context of human-wildlife interactions. Importantly, changes in FID for common songbird species in response to noise might influence how close human observers can approach wild birds, and thus, alter the quality of an experience with wildlife and nature.
Materials and Methods
We studied avian FIDs in relationship to background noise in urban parks and protected natural spaces throughout San Luis Obispo County, Muir Woods National Monument, California, and Grand Teton National Park, Wyoming. Our sites represented a range of background sound levels to capture natural variation in ambient noise and we did not manipulate the acoustic environment during our observations. Following previously described methods (e.g., Blumstein et al., 2003), a single observer located an individual bird and recorded the species, time, and initial distance between the observer and the target bird (Starting Distance) using an optical range finder (Nikon Aculon, Nikon Vision Co., Japan; TruPulse 360 R, Laser Technology, Inc., Colorado, USA). We targeted birds that were foraging, preening, or otherwise undisturbed by the intruder at the starting distance and not interacting with con- or hetero-specifics. While looking directly at the bird, the observer walked at a standard rate of 0.5 m/s along a straight path toward the target bird and dropped a marker at the distance where the bird flew away from the intruder (Flight Initiation Distance, measured as the distance between this marker and the bird's last perch). Immediately following the bird's escape, the observer measured time-averaged sound levels (Leq; A-weighted Leq, fast response, re. 20 μPa) for at least 1 min at the bird's last perch with a Larson Davis 824 or 831 sound pressure meter or a MicWi436 (MicW Audio, China) microphone paired with the SPLnFFT Sound Meter v6.2 iPhone application (FL's Audio Apps, France), a measurement kit equivalent to a type two sound level meter (Kardous and Shaw, 2014). We also measured wind speed with a Kestrel 4,000 weather meter (Kestrel Meters, USA). During the sound pressure level measurement, the observer scanned the surroundings and counted any pedestrians and their distances to the bird's last perch. We utilized pedestrian activity and whether each site was predominately human-modified (e.g., presence of buildings and other built surfaces, heavily-managed urban parks) to categorize each site as either a predominantly developed or natural area. Because almost all trial locations were within 0.5 km of roadways, sound levels were primarily from anthropogenic sources and did not systematically differ between developed [49.35 ± 4.70 SD dB(A)] and natural areas [50.26 ± 9.55 SD dB(A); Welch's two sample t-test, t = 0.87, df = 155.66, p > 0.38]. The observer then used a surveyor's tape or laser range finder to measure the FID, the distance between the bird's last perch to the dropped marker. If the bird was perched above the ground, we measured the Euclidean distance as the square root of the sum of the squared horizontal distance and squared perch height (Møller et al., 2015). Finally, the observer categorized surrounding vegetation as open, medium, or dense. To avoid sampling the same individual more than once, the observer moved at least 250 m from the first survey before locating a subsequent individual. We also visited sites throughout each location only once to avoid resampling individuals.
Data Analysis
We used a log10 transformation on all distance data to normalize their distributions. We assigned each of the surveyed species to one of three foraging guilds based on foraging behavior: ground foragers, canopy gleaners, and hawking flycatchers (Ehrlich et al., 1988). Because we were most interested in the influence of noise on FIDs, using the entire dataset we first calculated adjusted FIDs as the residuals of a linear regression model where raw FIDs were explained by vegetation category and starting distance, two variables known to strongly influence FID (e.g., Fernández-Juricic et al., 2002; Blumstein, 2003; Blumstein et al., 2005). We then used linear mixed effect models using the lme4 package (Bates et al., 2014) in R with vegetation and starting distance-corrected FID (henceforth adjusted FID) for each foraging guild as a response variable and background sound level, wind speed, Julian date, time of day, developed vs. natural habitat, species and an interaction between sound level and species as fixed effects. Developed vs. natural habitat was used as a covariate to capture exposure to human activity and account for the possibility of habituation to human approach. Models for canopy gleaners did not include developed vs. natural habitat because all individuals were sampled in areas categorized as natural. In all models, we also treated location as a random intercept. We used Akaike information criterion corrected for small sample size (AICc) in model selection. Because of recent criticisms of model-averaging (Cade, 2015), we considered all models with ΔAICc < 2 to be equivalent (Boersma et al., 2016). To gauge the influence of individual predictors, for each model with ΔAICc < 2, we concluded that a predictor variable had a strong effect on adjusted FID when its 95% confidence interval (95% CIs) did not overlap zero.
Finally, foraging guilds may also reflect shared evolutionary histories that could influence variation in FID values among guilds. For example, all species categorized as hawking flycatchers are suboscines in family Tyrannidae. Thus, we tested for phylogenetic structure in mean FID values among species and species-specific model-estimated effect sizes for the influence of background sound levels on adjusted FIDs, including standard errors, using the phylosig function in the R package phytools (Revell, 2012), which incorporates the method from Ives et al. (2007) to account for sampling error. For our phylogenetic hypotheses, we used phylogenies from Jetz et al. (2012) and available from Birdtree.org. However, due to phylogenetic uncertainty among the Jetz et al. set, we used 100 randomly selected trees to calculate mean values for two common metrics for phylogenetic signal: Pagel's λ (Pagel, 1999) and Blomberg's K (Blomberg et al., 2003). Pagel's λ values vary from zero to one. High λ values indicate that closely related species have very similar trait values (i.e., high phylogentic structure). Low λ values indicate that trait values are unrelated to phylogeny. Blomberg's K-values > 1 suggest strong phylogenetic signal and values from zero to 1 suggest no phylogenetic signal to weak phylogenetic signal.
Results
We surveyed a total of 197 individuals of 12 species of songbird across 27 locations from January to July 2016. Due to wind speed exceeding Category 3 on the Beaufort scale, we excluded one observation from the dataset to prevent potential bias introduced to sound measurements (Francis et al., 2011). Of the resulting 196 individuals, 105 were ground foragers, 52 canopy gleaners, and 39 flycatchers (Table 1). These individuals were sampled across 46 developed and 150 natural sites. We conducted trials throughout the day (0,600–1,630); however, we conducted most our observations (179 of 196) between 0,600 and 1,200 h. Wind speed ranged from 0 m/s to 5.5 m/s, with an average of 0.68 ± 0.93 m/s. Background sound levels ranged from 17.0 to 77.4 dB(A) with an average of 50.04 ± 8.68 dB(A). Starting and flight initiation distances averaged 26.76 ± 15.01 and 10.77 ± 7.32 m, respectively. We found no evidence for phylogenetic structure for mean FID (Pagel's λ = 0.01, sd = 0.1; Blomberg's K = 0.41, sd = 0.03), but evidence for a weak to moderate phylogenetic signal for the effect of noise on adjusted FID (Pagel's λ = 0.50, sd = 0.30; Blomberg's K = 0.77, sd = 0.07).
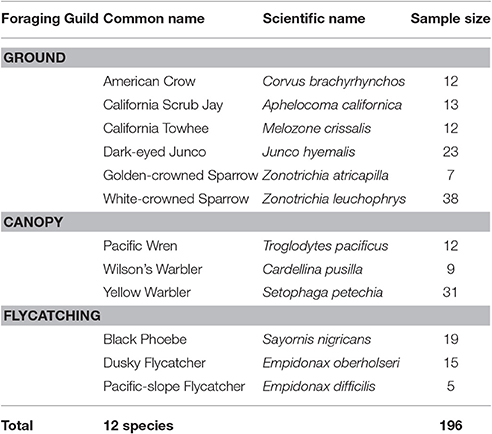
Table 1. We observed 196 individuals from 12 songbird species and grouped the species into three foraging guilds.
Among the top models for ground foraging species, the parameters background noise, diet, and species had strong effects, where 95% confidence intervals did not overlap zero (Table 2). The model that only included the random effect of location (i.e., null) was 2.00 ΔAICc from the top model. We visualized our results using the most parsimonious top model, which demonstrates an overall negative influence of background noise level on FIDs for all ground foraging species (Figure 2B). However, several ground foraging species differed in their overall response distances (i.e., different intercepts per species; Figure 2A, Table 3). Our results indicate that the individuals from the ground foraging guild were generally slower to respond to an observer's approach with elevated background sound and that omnivorous species flush at farther distances than granivorous species.
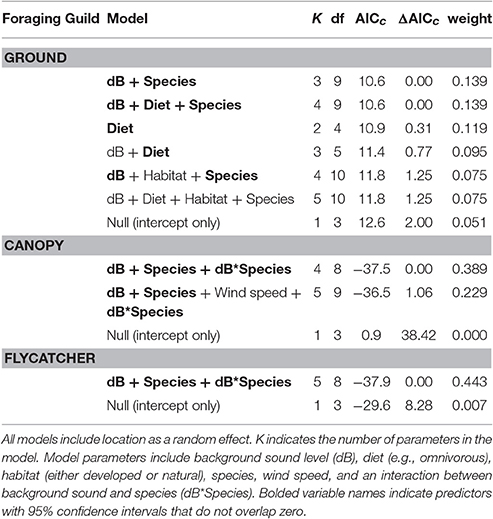
Table 2. This model selection table reports all the models within 2.00 ΔAICc in addition to the null model (intercept only) for each foraging guild.
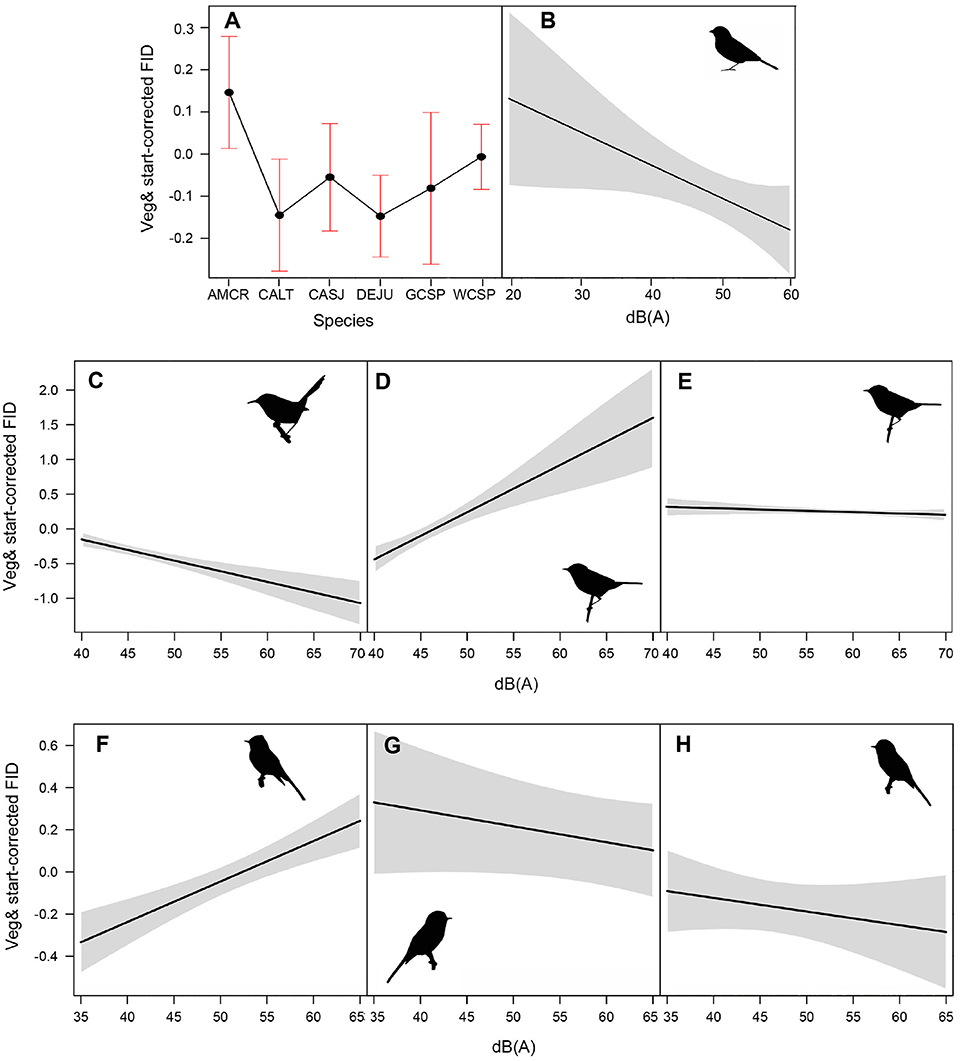
Figure 2. (A) illustrates mean adjusted FID and standard error for each species in the ground foraging guild. Species depicted are American crow (AMCR), California towhee (CALT), California scrub jay (CASJ), dark-eyed junco (DEJU), golden-crowned sparrow (GCSP), and white-crowned sparrow (WCSP). (B–H) illustrate the influence of ambient noise on vegetation and start distance-corrected FID values for all ground foraging species (B), canopy gleaning species Pacific wren (C), Wilson's warbler (D), yellow warbler (E), and flycatching species black phoebe (F), dusky flycatcher (G), and Pacific-slope flycatcher (H). For plotting purposes, we utilized the top-ranking model (lowest AICc and fewest parameters) for each of the foraging guilds (Table 2).
Among the top models for canopy gleaning species, the parameters with strong effects, included background noise, species, and an interaction between the two (Table 2). The null model (location as random intercept only) was 38.42 ΔAICc lower than the top model. Wilson's warbler (Cardellina pusilla) adjusted FIDs were positively influenced by background noise, Pacific wren (Troglodytes pacificus) adjusted FIDs were negatively affected by noise and yellow warblers (Setophaga petechia) appear uninfluenced by noise (Figures 2C–E, Table 4).
In the flycatching guild, the best model contained species, background noise, and an interaction between the two as fixed effects (Table 2). It was 29.6 ΔAICc better than the null model that only included the random effect of location and there were no other models with ΔAICc < 2. Background noise levels had a strong positive influence on black phoebe (S. nigricans) adjusted FIDs (Figure 2F), but adjusted FIDs for both the Pacific-slope flycatcher (Empidonax difficilis) and dusky flycatcher (Empidonax oberholseri) were negatively affected by noise, albeit weakly (Figures 2G,H, Table 5).
Discussion
To our knowledge, this report is the first to specifically address changes in songbird FIDs in response to noise, with implications for how noise influences predation risk and missed foraging opportunity as well as bird behavior relevant to casual nature-seekers and birdwatchers. Through our observations of wild songbird FIDs in varying acoustic conditions, we found evidence that noise influences bird FIDs in a variety of ways. However, we found no evidence that level of habitat development influenced FIDs of ground foraging and flycatching birds, suggesting that habituation to human presence may not strongly influence FID or the relationship between FID and background sound level. We also found no evidence that mean FID values were influenced by relatedness of species in our sample. However, the effect of noise on adjusted FIDs had some phylogenetic signal, likely reflecting that model estimates found identical relationships between noise and adjusted FID among all Emberizid species and because the congener flycatchers demonstrated similar adjusted FID responses with increasing noise. In general, however, our results indicate mixed responses across songbirds that can be species-specific and that might be explained in part by foraging behavior and, possibly, height of perch in the canopy.
All species within the ground foraging guild exhibited shorter FIDs in noise, as we predicted, such that the observer could approach closer to the target bird before it flushed. Shorter FID responses in noisy conditions may be explained by the distracted prey hypothesis, which posits that background noise occupies the target bird's finite attention and thus distracts the individual from other potentially important stimuli (Chan et al., 2010a). Although the distracted prey hypothesis may explain shortened FIDs in noise for some of these bird species, it is impossible to uncouple distraction from the effects of energetic masking. The high background sound in some areas might have masked the sounds of an approaching observer, leading to a slower response from the target bird. Of course, both mechanisms could operate simultaneously. Regardless, the result is that noise reduces an individual's ability to detect approaching threats and likely elevates an individual's risk of predation (Krause and Godin, 1996; Simpson et al., 2015); failure to detect predators at a sufficient distance could be lethal.
Although our results suggest ground foraging birds may be more at risk to predation in noisy environments, the effects of masking and noise on predator abundance and hunting ability must also be considered. Opportunistic avian nest predators avoid noisy areas in a natural gas extraction field (Francis et al., 2009, 2011) and owls, which are specialized acoustic predators, have trouble localizing or foraging in noisy conditions found in gas fields and near roadways (Mason et al., 2016; Senzaki et al., 2016). In laboratory settings, both Quinn et al. (2006) and Ware et al. (2015) demonstrated that ground foraging species spend significantly more time visually scanning for predators when ambient noise is high compared to quiet conditions. If ground foraging birds in our study also increase visual vigilance with noise, our results suggest that they were still unable to detect and respond to approaching threats as quickly as in quiet conditions, despite compensating with visual scans. Notwithstanding the potential ultimate costs of failing to detect predators at an appropriate distance, for human observers seeking to experience wild birds at close range, species that flee at shorter distances in noisy conditions would be easier to approach, view and hear. Finally, diet was also a significant predictor of FID for ground foraging species; species with omnivorous diets exhibited longer FIDs than species with granivorous diets irrespective of noise levels. Although limited evidence can help explain this trend, Francis (2015) demonstrated that the abundance of most avian species decline in noisy areas, species with plant-based diets appear to be less sensitive than those with animal-based diets. It is also possible that other traits unique to the two omnivorous species, which are both corvids, could explain their longer FIDs relative to granivorous species. For example, various measurements of brain size are positively associated with FIDs (Symonds et al., 2016) and corvids are known to have relatively large brains (Emery and Clayton, 2004). Greater cognitive capacity could also potentially mitigate distraction by noise and other stimuli (i.e., distracted prey hypothesis) by permitting individuals to process multiple streams of sensory information and respond to approaching threats appropriately. Future work should explore the relative contributions of cognitive abilities and foraging modalities to explain FIDs or sensitivities to changes in background acoustics in general.
For the canopy gleaning guild, we predicted that FIDs would decrease as a result of distraction or masking due to increased ambient noise. However, we suspected that because these species often utilize high tree perches, they might only exhibit weakly decreased or unchanged FIDs to a human approaching on the ground due to vertical relief from the threat. Although these predictions were not supported for all canopy gleaning species, we observed a trend of decreased FIDs with increasing noise for Pacific wrens (T. pacificus) and a pattern of unchanged FIDs for yellow warblers (S. petechia). Similar to the ground foraging species, Pacific wrens may be more susceptible to the effects of masking or distraction due to frequent sensory modality shifts as they remain acoustically vigilant while visually foraging. Pacific wrens are known to forage in the low canopy and, thus, experience little vertical relief from ground-level threats; however, yellow warblers frequently utilize high perches, which may explain the lack of FID response for the species. Additionally, although our robust sampling of yellow warblers indicates that this species exhibits consistent FID behavior across medium to high sound levels [46.9–77.4 dB(A)], we were unable to conduct any approaches under relatively low ambient sound conditions [<40 dB(A)]. Future work should focus on observing yellow warblers in environments with less ambient noise, as even acoustic cues at these relatively quiet levels can elicit responses in other taxa (Shannon et al., 2016b) and perhaps there is a threshold below which noise might influence FID in yellow warblers. In contrast to our predictions for the canopy gleaning guild, Wilson's warblers (C. pusilla) exhibited a trend of increased FIDs with increasing noise. Wilson's warblers primarily forage by gleaning insects in the canopy, but they are also known to hawk for flying insect prey (Ehrlich et al., 1988) and, thus, might utilize sensory modalities differently when compared to other canopy gleaning species. However, yellow warblers are also occasional flycatchers (Ehrlich et al., 1988) and, yet, do not exhibit the same increased FIDs of Wilson's warblers. Thus, engaging in flycatching behavior cannot solely explain the pattern consistent with hypervigilance in noise among Wilson's warblers. Finally, although two of the canopy gleaning species studied here fit our predictions of decreased FIDs or no response, future research should explicitly include individual perch height as a potential predictor of variation in FIDs both within and across species.
Our observed results for Wilson's warblers follow our prediction for species of the flycatching guild such that these species would exhibit unchanged or increased FIDs in noise due to their foraging strategy of constant visual and aural vigilance that might allow these species to scan for predators and prey simultaneously. However, only one species of the flycatching guild, the black phoebe (S. nigricans), provided support for this prediction with increasing FIDs with increases in noise levels. The other two species of this guild, dusky flycatchers (E. oberholseri) and Pacific-slope flycatchers (E. difficilis), exhibited a weak trend of decreased FIDs with increasing noise. This muted response might be explained by our small sample size or perhaps like the canopy gleaning yellow warblers, by these flycatchers' shared tendency to utilize high perches, thereby relieving the threat of a ground-level approaching human. In the case of the black phoebe, however, our robust sampling in a wide range of ambient noise conditions indicates that this species is generally more likely to flush sooner if background noise is high. Such increased FIDs in noise might be explained by heightened vigilance in noisy conditions, which was demonstrated in laboratory settings with chaffinches (Quinn et al., 2006) and white-crowned sparrows (Ware et al., 2015) as well as in a field experiment with free-ranging prairie dogs (Shannon et al., 2016a). Furthermore, flushing more quickly in noise coincides with FEAR hypothesis, which suggests that birds are likely to flush quickly after detection in order to avoid the need to monitor an approaching threat (Blumstein, 2010). This response might allow for more foraging time if the bird flushes to an area with available food; however, fleeing from a beneficial foraging site may result in lost foraging opportunity and could incur the cost of increased energy expenditure.
In the context of human experience of wildlife, our results indicate that background noise may influence the quality of a birdwatching experience. Due to their hawking behaviors, both Wilson's warblers and black phoebes can be exciting birds to observe. Both species exhibited longer FIDs in noise, indicating that birdwatchers may experience difficulty when seeking these species and perhaps other hawking species in noisy conditions. However, most of the species in our sample trended toward shorter FIDs in noise, which would allow birders to approach closer. This may lead to a surprisingly positive outcome of anthropogenic noise pollution, under which birdwatching experiences are improved through field observations at closer range in noisier conditions. Particularly for new birdwatchers, this close viewing of wild birds may foster a personal and lasting connection with wildlife and lead to increased support for wildlife conservation. Of course, this potential benefit to nature-seekers of visually experiencing birds at a closer range must be viewed in the context of the quickly growing body of literature reporting the negative effects of noise and human disturbance to wild animals (Ellison et al., 2012; Francis and Barber, 2013; Shannon et al., 2016b). Nevertheless, our conclusions offer new perspective on the coexistence of humans and wildlife in an increasingly noisy world.
Ethics Statement
This study was carried out in accordance with the guidelines of the Committee of the Care and Use of Laboratory Animals Resources, National Research Council, and the research protocol was approved by the California Polytechnic State University, Institutional Animal Care and Use Committee (#1519).
Author Contributions
AP, ML, and RW conducted the fieldwork. JB and CF conceived of the project. All authors contributed to analysis and interpretation of the data. AP was primarily responsible with writing the manuscript added by CF and all other authors contributed to writing, editing and, ultimately, approving of the manuscript for publication.
Conflict of Interest Statement
The authors declare that the research was conducted in the absence of any commercial or financial relationships that could be construed as a potential conflict of interest.
Acknowledgments
We thank Gita Kolluru, Crow White and two reviewers for constructive feedback on earlier versions of this paper. This project was funded by National Science Foundation Grant (CNH-1414171) to JB and CF.
References
Abbott, L. C., Taff, D., Newman, P., Benfield, J., and Mowen, A. (2016). The influence of natural sounds on attention restoration. J. Park Recreat. Administr. 34, 5–15. doi: 10.18666/JPRA-2016-V34-I3-6893
Barber, J. R., Burdett, C., Reed, S., Warner, K., Formichella, C., Crooks, K., et al. (2011). Anthropogenic noise exposure in protected natural areas: estimating the scale of ecological consequences. Landsc. Ecol. 26, 1281–1295. doi: 10.1007/s10980-011-9646-7
Barber, J. R., Crooks, K. R., and Fristrup, K. M. (2010). The costs of chronic noise exposure for terrestrial organisms. Trends Ecol. Evol. 25, 180–189. doi: 10.1016/j.tree.2009.08.002
Bates, D., Maechler, M., Bolker, B., and Walker, S. (2014). lme4: Linear Mixed-Effects Models Using Eigen and S4. R package version, 1.
Blomberg, S. P., Garland, T. Jr., and Ives, A. R. (2003). Testing for phylogenetic signal in comparative data: behavioral traits are more labile. Evolution 57, 717–745. doi: 10.1111/j.0014-3820.2003.tb00285.x
Blumstein, D. T. (2003). Flight-initiation distance in birds is dependent on intruder starting distance. J. Wildl. Manag. 67, 852–857. doi: 10.2307/3802692
Blumstein, D. T. (2010). Flush early and avoid the rush: a general rule of antipredator behavior? Behav. Ecol. 21, 440–442. doi: 10.1093/beheco/arq030
Blumstein, D. T., Anthony, L. L., Harcourt, R., and Ross, G. (2003). Testing a key assumption of wildlife buffer zones: is flight initiation distance a species-specific trait? Biol. Conserv. 110, 97–100. doi: 10.1016/S0006-3207(02)00180-5
Blumstein, D. T., Fernández-Juricic, E., Zollner, P. A., and Garity, S. C. (2005). Inter-specific variation in avian responses to human disturbance. J. Appl. Ecol. 42, 943–953. doi: 10.1111/j.1365-2664.2005.01071.x
Boersma, K. S., Nickerson, A., Francis, C. D., and Siepielski, A. M. (2016). Climate extremes are associated with invertebrate taxonomic and functional composition in mountain lakes. Ecol. Evol. 6, 8094–8106. doi: 10.1002/ece3.2517
Cade, B. (2015). Model averaging and muddled multimodal inferences. Ecology 96, 2370–2382. doi: 10.1890/14-1639.1
Carver, E. (2013). Birding in the United States: A Demographic and Economic Analysis: Addendum to the 2011 National Survey of Fishing, Hunting and Wildlife-associated Recreation. Arlington: Division of Federal Aid, US Fish and Wildlife Service.
Chan, A. A. Y.-H., Giraldo-Perez, P., Smith, S., and Blumstein, D. T. (2010a). Anthropogenic noise affects risk assessment and attention: the distracted prey hypothesis. Biol. Lett. 6, 458–461. doi: 10.1098/rsbl.2009.1081
Chan, A. A. Y.-H., Stahlman, W. D., Garlick, D., Fast, C. D., Blumstein, D. T., and Blaisdell, A. P. (2010b). Increased amplitude and duration of acoustic stimuli enhance distraction. Anim. Behav. 80, 1075–1079. doi: 10.1016/j.anbehav.2010.09.025
Ehrlich, P. R., Dobkin, D. S., and Wheye, D. (1988). The Birder's Handbook: A Field Guide to the Natural History of North American Birds Including all Species that Regularly Breed north of Mexico. New York, NY: Simon & Schuster.
Ellison, W. T., Southall, B. L., Clark, C. W., and Frankel, A. S. (2012). A new context-based approach to assess marine mammal behavioral responses to anthropogenic sounds. Conserv. Biol. 26, 21–28. doi: 10.1111/j.1523-1739.2011.01803.x
Emery, N. J., and Clayton, N. S. (2004). The mentality of crows: convergent evolution of intelligence in Corvids and apes. Science 306, 1903–1907. doi: 10.1126/science.1098410
Fernández-Juricic, E., Gall, M. D., Dolan, T., O'Rourke, C., Thomas, S., and Lynch, J. R. (2011). Visual systems and vigilance behaviour of two ground-foraging avian prey species: white-crowned sparrows and California towhees. Anim. Behav. 81, 705–713. doi: 10.1016/j.anbehav.2010.11.014
Fernández-Juricic, E., Gall, M. D., Dolan, T., Tisdale, V., and Martin, G. R. (2008). The visual fields of two ground-foraging birds, House Finches and House Sparrows, allow for simultaneous foraging and anti-predator vigilance. Ibis 150, 779–787. doi: 10.1111/j.1474-919X.2008.00860.x
Fernández-Juricic, E., Jimenez, M. D., and Lucas, E. (2002). Factors affecting intra- and inter-specific variations in the difference between alert distances and flight distances for birds in forested habitats. Can. J. Zool. 80, 1212–1220. doi: 10.1139/z02-104
Fernández-Juricic, E., O'Rourke, C., and Pitlik, T. (2010). Visual coverage and scanning behavior in two corvid species: american crow and Western scrub jay. J. Comp. Physiol. A 196, 879–888. doi: 10.1007/s00359-010-0570-0
Francis, C. D. (2015). Vocal traits and diet explain avian sensitivities to anthropogenic noise. Glob. Change Biol. 21, 1809–1820. doi: 10.1111/gcb.12862
Francis, C. D., and Barber, J. R. (2013). A framework for understanding noise impacts on wildlife: an urgent conservation priority. Front. Ecol. Environ. 11, 305–313. doi: 10.1890/120183
Francis, C. D., Ortega, C. P., and Cruz, A. (2009). Noise pollution changes avian communities and species interactions. Curr. Biol. 19, 1415–1419. doi: 10.1016/j.cub.2009.06.052
Francis, C. D., Paritsis, J., Ortega, C. P., and Cruz, A. (2011). Landscape patterns of avian habitat use and nest success are affected by chronic gas well compressor noise. Landsc. Ecol. 26, 1269–1280. doi: 10.1007/s10980-011-9609-z
Frid, A., and Dill, L. (2002). Human-caused disturbance stimuli as a form of predation risk. Conserv. Ecol. 6:11. doi: 10.5751/ES-00404-060111
Gall, M. D., and Fernández-Juricic, E. (2009). Visual fields, eye movements, and scanning behavior of a sit-and-wait predator, the black phoebe (Sayornis nigricans). J. Comp. Physiol. A 196, 15–22. doi: 10.1007/s00359-009-0488-6
Haas, G. E., and Wakefield, T. J. (1998). National Parks and the American Public: a National Public Opinion Survey on the National Park System: a Summary Report, Conducted by Colorado State University.
Halfwerk, W., Holleman, L. J. M., Lessells, C. M., and Slabbekoorn, H. (2011). Negative impact of traffic noise on avian reproductive success. J. Appl. Ecol. 48, 210–219. doi: 10.1111/j.1365-2664.2010.01914.x
Ives, A. R., Midford, P. E., and Garland, T. Jr. (2007). Within-species variation and measurement error in phylogenetic comparative biology. Syst. Biol. 56, 252–270. doi: 10.1080/10635150701313830
Jetz, W., Thomas, G. H., Joy, J. B., Hartmann, K., and Mooers, A. O. (2012). The global diversity of birds in space and time. Nature 491, 444–448. doi: 10.1038/nature11631
Kardous, C., and Shaw, P. (2014). Evaluation of smartphone sound measurement applications. J. Acoust. Soc. Am. 135, EL186–EL192. doi: 10.1121/1.4865269
Karp, D. S., and Root, T. L. (2009). Sound the stressor: How Hoatzins (Opisthocomus hoazin) react to ecotourist conversation. Biodiver. Conserv. 18, 3733–3742. doi: 10.1007/s10531-009-9675-6
Kight, C. R., Saha, M. S., and Swaddle, J. P. (2012). Anthropogenic noise is associated with reductions in the productivity of breeding Eastern Bluebirds (Sialia sialis). Ecol. Appl. 22, 1989–1996. doi: 10.1890/12-0133.1
Krause, J., and Godin, J.-G. J. (1996). Influence of prey foraging posture on flight behavior and predation risk: predators take advantage of unwary prey. Behav. Ecol. 7, 264–271. doi: 10.1093/beheco/7.3.264
La Rouch, G. P. (2003). Birding in the United States: a Demographic and Economic Analysis: Addendum to the 2001 National Survey of Fishing, Hunting and Wildlife-associated Recreation. Division of Federal Aid, US Fish and Wildlife Service.
Lima, S. L., and Bednekoff, P. A. (1999). Back to the basics of antipredatory vigilance: can nonvigilant animals detect attack? Anim. Behav. 58, 537–543. doi: 10.1006/anbe.1999.1182
Lynch, E., Joyce, D., and Fristrup, K. (2011). An assessment of noise audibility and sound levels in US National Parks. Landsc. Ecol. 26, 1297–1309. doi: 10.1007/s10980-011-9643-x
Marin, L. D., Newman, P., Manning, R., Vaske, J. J., and Stack, D. (2011). Motivation and acceptability norms of human-caused sound in Muir Woods National Monument. Leisure Sci. 33, 147–161. doi: 10.1080/01490400.2011.550224
Martin, G. R. (2007). Visual fields and their functions in birds. J. Ornithol. 148, S547–S562. doi: 10.1007/s10336-007-0213-6
Mason, J. T., McClure, C. J., and Barber, J. R. (2016). Anthropogenic noise impairs owl hunting behavior. Biol. Conserv. 199, 29–32. doi: 10.1016/j.biocon.2016.04.009
McClure, C. J. W., Ware, H. E., Carlisle, J., Kaltenecker, G., Barber, J. R., and Barber, J. R. (2013). An experimental investigation into the effects of traffic noise on distributions of birds: avoiding the phantom road. Proc. Biol. Sci. 280:20132290. doi: 10.1098/rspb.2013.2290
Meillère, A., Brischoux, F., and Angelier, F. (2015). Impact of chronic noise exposure on antipredator behavior: an experiment in breeding house sparrows. Behav. Ecol. 26, 569–577. doi: 10.1093/beheco/aru232
Mennitt, D., Fristrup, K. M., Sherrill, K., and Nelson, L. (2013). “Mapping sound pressure levels on continental scales using a geospatial sound model,” in Proceedings of Inter-Noise (Innsbruck), 1–11.
Møller, A., Tryjanowski, P., Díaz, M., Kwiecinski, Z., Indykiewicz, P., Mitrus, C., et al. (2015). Urban habitats and feeders both contribute to flight initiation distance reduction in birds. Behav. Ecol. 26, 861–865. doi: 10.1093/beheco/arv024
Mulholland, T. I. (2016). Effects of Experimental Noise Exposure on Songbird Nesting Behaviors and Nest Success. MS Thesis, California Polytechnic State University.
Newman, P., Taft, D., Weinzimmer, D., Lawson, S., Trevino, K., Fristrup, K., et al. (2013). “Monitoring and managing anthropogenic noise in national parks: lessons learned from field and laboratory studies,” in Inter-Noise and Noise-Con Congress and Conference Proceedings (Reston: Institute of Noise Control Engineering), 6001–6008
Ortega, C. P., and Francis, C. D. (2012). Effects of gas well compressor noise on ability to detect birds during surveys in northwest New Mexico. Ornithol. Monogr. 49, 78–90. doi: 10.1525/om.2012.74.1.78
Pacifici, K., Simons, T. R., and Pollock, K. H. (2008). Effects of vegetation and background noise on the detection process in auditory avian point-count surveys. Auk 125, 600–607. doi: 10.1525/auk.2008.07078
Pagel, M. (1999). Inferring the historical patterns of biological evolution. Nature 401, 877–884. doi: 10.1038/44766
Quinn, J. L., Whittingham, M. J., Butler, S. J., and Cresswell, W. (2006). Noise, predation risk compensation and vigilance in the chaffinch Fringilla coelebs. J. Avian Biol. 37, 601–608. doi: 10.1111/j.2006.0908-8857.03781.x
Ratcliffe, E., Gatersleben, B., and Sowden, P. T. (2013). Bird sounds and their contributions to perceived attention restoration and stress recovery. J. Environ. Psychol. 36, 221–228. doi: 10.1016/j.jenvp.2013.08.004
Revell, L. J. (2012). phytools: an R package for phylogenetic comparative biology (and other things). Methods Ecol. Evol. 3, 217–223. doi: 10.1111/j.2041-210X.2011.00169.x
Samia, D. S. M., and Blumstein, D. T. (2015). Birds flush early and avoid the rush: An interspecific study. PLoS ONE 10:e0119906. doi: 10.1371/journal.pone.0119906
Senzaki, M., Yamaura, Y., Francis, C. D., and Nakamura, F. (2016). Traffic noise reduces foraging efficiency in wild owls. Sci. Rep. 6:0602. doi: 10.1038/srep30602
Shannon, G., Crooks, K. R., Wittemyer, G., and Fristrup, K. M. (2016a). Road noise causes earlier predator detection and flight response in a free-ranging mammal. Behav. Ecol. 25, 1370–1375. doi: 10.1093/beheco/arw058
Shannon, G., Mckenna, M. F., Angeloni, L. M., Crooks, K. R., Fristrup, K. M., Brown, E., et al. (2016b). A synthesis of two decades of research documenting the effects of noise on wildlife. Biol. Rev. 24, 982–1005. doi: 10.1111/brv.12207
Simpson, S. D., Radford, A. N., Nedelec, S. L., Ferrari, M. C. O., Chivers, D. P., Mccormick, M. I., et al. (2015). Anthropogenic noise increases fish mortality by predation. Nat. Commun. 7:10544. doi: 10.1038/ncomms10544
Symonds, M. R. E., Weston, M. A., van Dongen, W. F. D., Lill, A., Robinson, R. W., and Guay, P.-J. (2016). Time since urbanization but not encephalisation is associated with increased tolerance of human proximity in birds. Front. Ecol. Evol. 4:117. doi: 10.3389/fevo.2016.00117
Templeton, C. N., Zollinger, S. A., and Brumm, H. (2016). Traffic noise drowns out great tit alarm calls. Curr. Biol. 26, R1173–R1174. doi: 10.1016/j.cub.2016.09.058
Keywords: anthropogenic noise, birdwatching, escape behavior, predation risk, vigilance, masking, threat detection
Citation: Petrelli AR, Levenhagen MJ, Wardle R, Barber JR and Francis CD (2017) First to Flush: The Effects of Ambient Noise on Songbird Flight Initiation Distances and Implications for Human Experiences with Nature. Front. Ecol. Evol. 5:67. doi: 10.3389/fevo.2017.00067
Received: 20 January 2017; Accepted: 06 June 2017;
Published: 21 June 2017.
Edited by:
Caroline Isaksson, Lund University, SwedenReviewed by:
Alejandro Ariel Rios-Chelen, Centro Tlaxcala de Biologia de la Conducta, Universidad Autonoma de Tlaxcala, MexicoGraeme Shannon, Bangor University, United Kingdom
Copyright © 2017 Petrelli, Levenhagen, Wardle, Barber and Francis. This is an open-access article distributed under the terms of the Creative Commons Attribution License (CC BY). The use, distribution or reproduction in other forums is permitted, provided the original author(s) or licensor are credited and that the original publication in this journal is cited, in accordance with accepted academic practice. No use, distribution or reproduction is permitted which does not comply with these terms.
*Correspondence: Alissa R. Petrelli, arpetrel@calpoly.edu
Clinton D. Francis, cdfranci@calpoly.edu