Cultivable Bacterial Diversity in the Gut of the Chagas Disease Vector Triatoma dimidiata: Identification of Possible Bacterial Candidates for a Paratransgenesis Approach
- 1Centro Regional de Investigación en Salud Pública, Instituto Nacional de Salud Pública, Tapachula, Mexico
- 2Facultad de Ciencias, Universidad Autónoma de Baja California, Ensenada, Mexico
- 3Laboratorio de Biología Molecular, Escuela de Agronomía, Universidad De la Salle Bajío, Guanajuato, Mexico
- 4Department of Medicine, University of California, San Diego, San Diego, CA, United States
Since bacterial symbionts play a vital role in the metabolism of hematophagous insect vectors the method known as paratrangenesis, which consists of the use of cultivable insect symbionts to interfere with the transmission of vector-transmitted pathogens has been shown to be effective in certain vector control oriented studies. In Chagas disease research a recent study introduced transgenes through a parastransgenic approach and prevented the development of a vector species for this disease. However this approach requires a previous characterization of the bacterial symbionts present in the species vector of interest, since a selection of the cultivable bacterial symbiont used is mandatory. In this study, we describe the gut bacterial diversity of Triatoma dimidiata specimens from Southern Mexico. Bacteria from both wild and laboratory-reared specimens were cultured, and resulting colonies were grown individually, harvested, and subsequently identified by 16S ribosomal loci sequencing. A total of five and three genera and a total of nine and six bacterial species were identified from field captured and laboratory reared Triatoma dimidiata specimens respectively. A majority of Gram positive bacteria were identified, which included the genera Staphylococcus, Bacillus, Brevibacterium, Micrococcus, and Delftia. Given previous studies we propose the use of Staphylococcus saprophyticus, Micrococcus luteus, and Bacillus megaterium as potential candidates for future paratransgenic efforts done with Triatoma dimidiata, which is one of the most important vectors of Chagas disease, in Central and South America. Given the vital association bacterial symbionts play in the metabolism of routes of hematophagous insect vectors Paratransgenesis consists of the use of cultivable insect symbionts to interfere with the transmission of vector-transmitted pathogens.
Introduction
The World Health Organization has estimated Chagas disease affects about 6–7 million people worldwide, while 100 million people are at risk of acquiring the disease in the Americas. The main form of parasite transmission to humans is through the contact with the feces of blood-sucking triatomine bugs (WHO, 2015). Despite the successful use of insecticides through spraying campaigns in many endemic areas, triatomine bugs have not been completely controlled, in part due to a lack of sustained effort by health authorities and in part due to pesticide resistance (Vassena et al., 2000; Dias et al., 2002; Picollo et al., 2005).
The cost associated with the manufacture of environmentally damaging chemicals which can be used as insecticides is usually very high for developing countries. This high cost is one of the main reasons developing countries interrupt vector control programs, including those for Chagas disease. Therefore, new strategies for vector control are needed. Understanding some bionomic aspects of the triatomine insects can inform the design of better control programs to interrupt transmission of the parasite (Ramsey and Schofield, 2003).
Triatoma dimidiata (T. dimidiata) is one of the most important triatomine species involved in transmission of Trypanosoma cruzi, the etiological agent of Chagas disease, in Central and South America (Guhl, 2009). Triatoma dimidiata can be found within human dwellings, increasing the risk for Chagas disease transmission to humans (Dumonteil et al., 2002, 2013; Weeks et al., 2013; Zamora et al., 2015). A study in Colombia described tolerance to pyrethroid insecticides in specimens of T. dimidiata (Reyes et al., 2007), which confirms the importance of developing new vector control tools for this domesticated species.
Insects are inhabited by a wide diversity of microorganisms, most of which are mutualistic symbionts (Akman Gündüz and Douglas, 2009; Russell et al., 2009), since they can positively affect both nutrition and defense of their insect hosts. For example, protection against a virulent nematode (Howardula aoronymphium) by Spiroplasma through the production of a toxin has been observed in Drosophila neotestacea, illustrating the positive effect these interactions can have in the defense system of the hosts (Douglas, 2011; Hamilton et al., 2015). Another example is the bacteria in the digestive tract of insects that appear to be necessary for detoxification of plant material (Bugg et al., 2011). Wolbachia sp. in particular has been found to be responsible for: (1) the regulation of iron concentrations in order to protect its insect hosts from oxidative stress and (2) to provide B-complex vitamins in blood-fed insects (Kremer et al., 2009; Nikoh et al., 2014). A thorough literature review of insects or other arthropods bearing bacteria is shown in the Supplementary Material (Supplementary Table 1). In terms of bacteria associated with triatomine species, the first reports were in the mid-twentieth century. Ryckman and Blankenship (1984) describe 248 references that mentioned parasites, symbionts, and predators of triatomine bugs in a literature compilation covering studies from 1950 through 1984 (Ryckman and Blankenship, 1984).
Triatomine insects feed exclusively on blood throughout their developmental cycle, a diet that is deficient in certain vitamins and nutrients (Dasch and Weiss, 1984). The deficient vitamins are provided by extracellular bacterial symbionts located in the gut. Therefore these bacteria are essential for normal growth and development in Triatomine insects (Eichler and Schaub, 2002). The symbiotic bacteria are transmitted from adult triatomine to their offspring through coprophagy (Dasch and Weiss, 1984).
Studies on insect intestinal bacterial symbionts have provided important information on how to use these microorganisms to control parasites transmitted by insect vectors- a strategy known as paratransgenesis (Hurwitz et al., 2011). It is essential to characterize these symbionts in both wild and laboratory-reared insects, since information concerning the maintenance of symbionts in laboratory conditions is critical to the paratransgenic approach. The paratransgenic method consists of creating recombinant bacteria that express molecules that control the development of the parasite within the vector and are then taken by insects through their diet.
Several paratransgenic approaches have been reported for triatomines. In Rhodnius prolixus, one of the most important vectors in Central and South America, the symbiotic bacterium Rhodococcus rhodnii, has been efficiently transformed and stable expression of transgenes has been achieved (Dotson et al., 2003). Another example is a species of the Corynebacterium genus that was identified in Triatoma infestans as a cultivable symbiont and has been successfully used to express a single chain antibody within the insect (Durvasula et al., 2008). More recently, dsRNA expression in Rhodnius prolixus through transformed Escherichia coli was reported as a model system to control the populations of this vector (Taracena et al., 2015). Two other studies have addressed the paratransgenic approach for this important Chagas disease vector, describing the identity of possible bacterial symbionts (Pamela Pennington, personal communication) and the transformation of some of them for application of this control method in Guatemala (Pennington and Beard, unpublished data).
The main purpose of this study was to identify cultivable bacteria present in the intestine of wild and laboratory T. dimidiata from Southern Mexico, as a first step to a paratransgenic approach with this vector. Our study describes the particular bacterial species associated with this region of Mexico and compares our results to previous studies of the same vector in Central America.
Materials and Methods
Laboratory Insects
Insects from a T. dimidiata colony that had been maintained at Centro Regional de Investigación en Salud Pública, Instituto Nacional de Salud Pública (CRISP/ INSP) (26.5 ± −1°C, 65 ± −5% Relative Humidity, given rabbit blood through feeders) for 5 years were used for this work. Five specimens from every developmental stage were dissected to test for bacteria.
Wild Insects
Twenty adults and one 4th instar nymph of T. dimidiata from five villages within three states in Mexico (Chiapas, Campeche and Oaxaca) were captured between March and August, 2010. In Campeche, collection sites were transition spaces between farming lands and wild areas. In Chiapas and Oaxaca, the collection sites were peridomestic.
Most of the insects' bodies were processed for diagnosis of Trypanosoma cruzi infection; their intestine (total gut) was aseptically dissected and divided to search for bacteria. None of the insects were positive for T. cruzi infection.
Dissection
Insects were anesthetized by incubating at low temperature (−20°C). They were superficial disinfected with 70% ethanol and placed on sterile Petri dishes; sterile forceps and needles were used to dissect organs. Digestive tract was exposed by cutting off the conecctive tissue around the abdomen and separating it the frontal and dorsal plaques. The small intestine or posterior midgut were cut and separated from the stomach and rectum (identification of organs according to Waniek et al., 2012) to be cultured. In laboratory-reared insects, fecal samples were taken by compression of the abdomen 5 days after the last blood meal, and then they were dissected following the same technique already described.
Isolation of Bacteria
Intestine macerates were cultivated on Brain Heart Infusion Agar, selected as an enriched medium to support growth of environmental bacteria. Once incubated at room temperature for 24 h, colonies that were morphologically different among each other were selected and grown in nutrient broth. Bacterial overnight cultures were harvested for DNA extraction.
Bacterial Identification
Bacterial species were identified by amplification of 16S Ribosomal gene using primers 63F: 5′-CAGGCCTAACACATGCAAGTC-3′ and 907R: 5′-CCGTCAATTCMTTTGAGTTT-3 (Marchesi et al., 1998; Muyzer and Smalla, 1998), using as a template the bacterial DNA extracted from bacterial cultures. PCR products were purified and sequenced bidirectionally in a 3730xl DNA Analyzer (Applied Biosystems). Chromas Lite v2.0 was used to edit all chromatograms sequenced and remove primer regions from the sequence. Once sequences were edited they were compared with those stored at Gene Bank through Nucleotide Blast V 2.2.28+. Using the criteria of best e-value, the best hits for each sequence were added to the phylogenetic analyses.
Phylogenetic Analysis
All sequences obtained from the study as well as those best hits described above were used for the phylogenetic study (Table 1). Each amplicon was used as a reference in Gen Bank through Nucleotide Blast V 2.2.28+ to search for its best hit. For every amplicon the best hit or hits (in cases when there was more than one best hit) were added to our symbiont bacteria data set and aligned using MUSCLE (Edgar, 2004) in Seaview version 4 (Gouy et al., 2010). Subsequently jModeltest2 was used to choose the best DNA substitution model for the data set (Darriba et al., 2012). A Bayesian phylogenetic reconstruction was performed in MrBayes (Huelsenbeck and Ronquist, 2001; Ronquist and Huelsenbeck, 2003), with a chain length of 5 million generations, sampling every 1000 generations. The first 25% of trees were discarded as burn-in, and the final topology was edited in Figtree (Rambaut and Drummond, 2008).
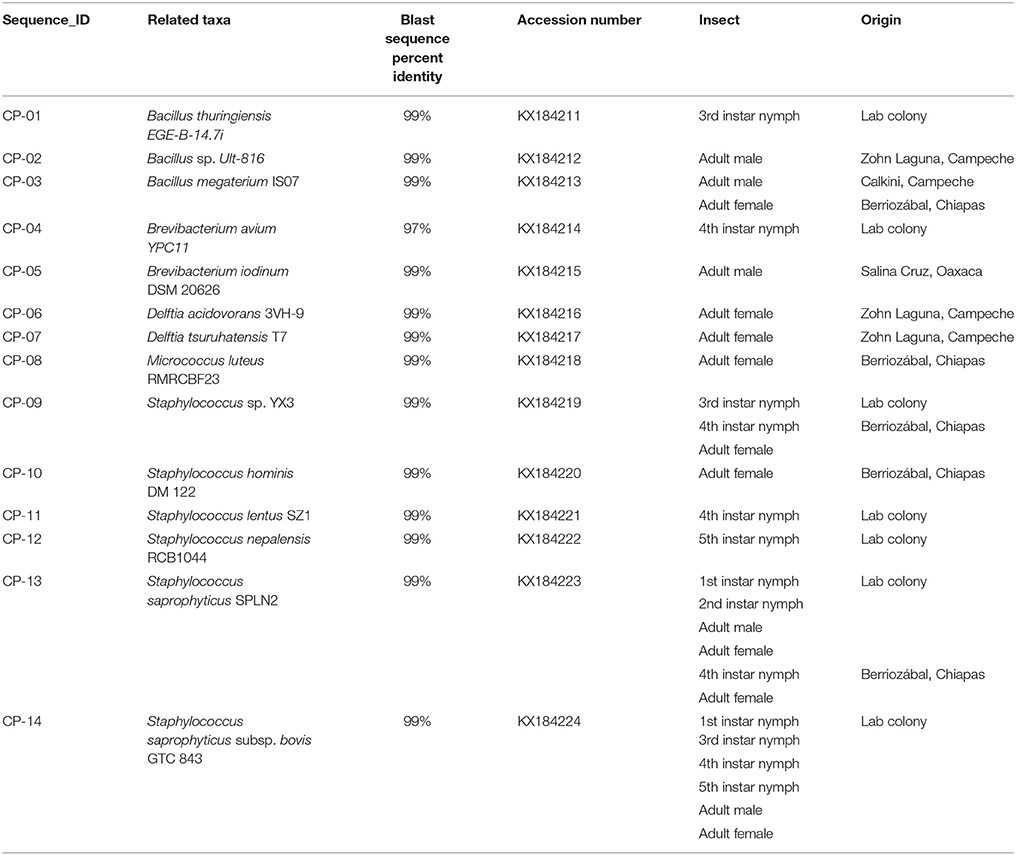
Table 1. Bacterial species associated to 16S rRNA sequences obtained from field-collected and laboratory Triatoma dimidiata specimens.
Results
From the field-collected insects only one type of colony was observed in every culture dish, so only one DNA isolation was made from each insect. For laboratory triatomine, a few morphologically different colonies were observed in every culture dish and some of them were unable to grow in liquid media.
We found 14 different isolates, GenBank accession numbers KX184211-KX184224, from all the T. dimidiata specimens examined (Table 1). The sequences from the 14 different isolates contained a total of 5 different genera (i.e., Brevibacterium, Micrococcus, Bacillus, Staphylococcus & Delftia) (Figure 1). Once all isolates were added to the phylogenetic analysis, we identified three distinct monophyletic bacteria. The phylum with the most number of bacteria was Firmicutes (9/14 isolates), followed by Actinobacteria (3/14 isolates), and Proteobacteria (2/14 isolates) (Figure 1). Of the Firmicutes phylum, the majority of isolates (6/9) had their most significant sequence identity hit to the Staphylococcus genera (i.e., Staphylococcus. sp. YX3, S. saprophyticus, S. saprophyticus subsp. bovis, Staphylococcus nepalensis, Staphylococcus hominis, and Staphylococcus lentus), while the other three Firmicutes isolates had their most significant sequence identity hit to the Bacillus genera (Bacillus thuringiensis, B. sp. Ult-816 and B. megaterium). The Actinobacteria phylum was formed by three isolates that could be grouped into two genera. Two of the isolates had their most significant sequence identity hit to Brevibacterium avium and Brevibacterium iodinum, while the other isolate had its most significant sequence identity hit in Micrococcus luteus. Finally, the two Proteobacteria isolates had their most significant sequence identity hit in Delftia acidovorans and Delftia tsuruhatensis (Figure 1 and Table 1).
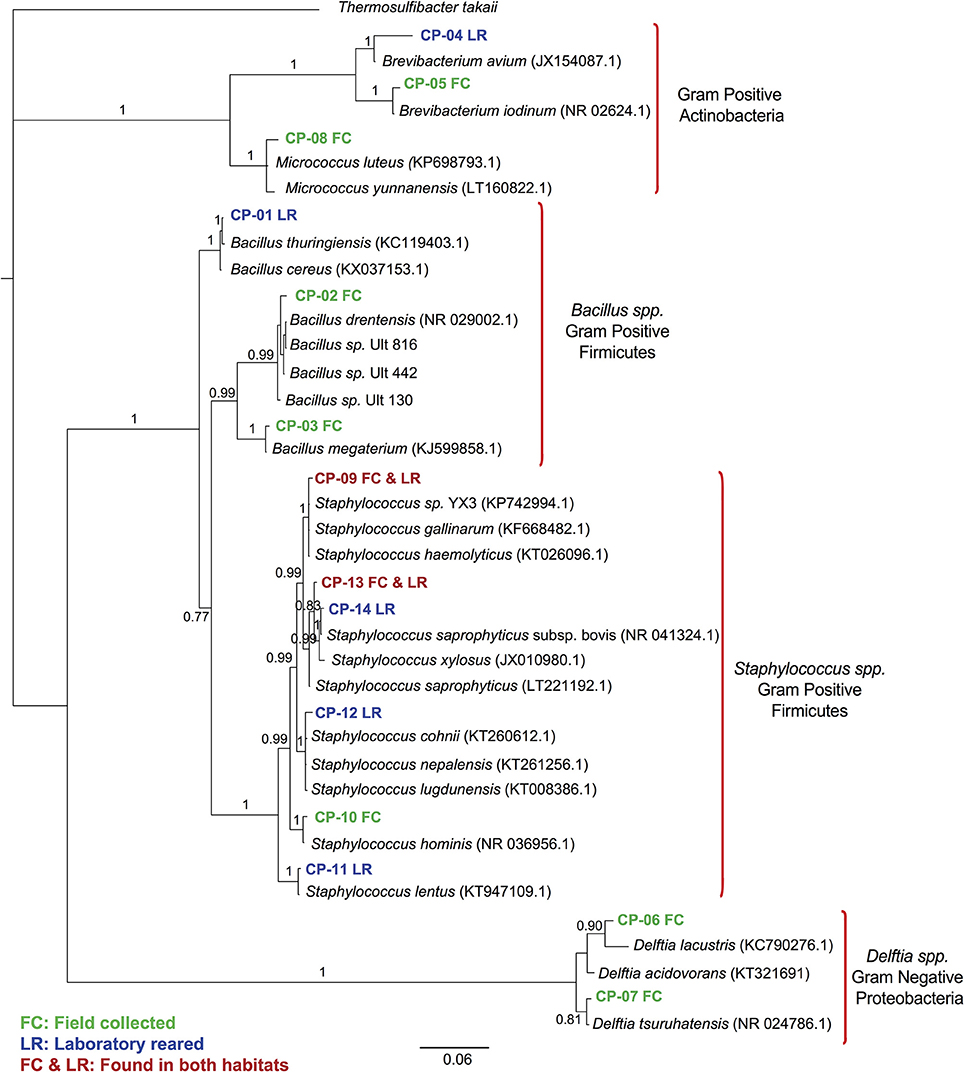
Figure 1. 16S rRNA gene sequence phylogenetic tree of Triatoma dimidiata bacterial symbionts. Bayesian bacterial symbionts phylogeny from all 16S rRNA gene amplicons sequenced from field-collected and laboratory-reared Triatoma dimidiata specimens. Amplicons from our study are labeled CP, whereas species included in the tree are the most closely related bacterial species found on GeneBank. Numbers on branches represent posterior probabilities from the Bayesian analysis.
Field specimens were collected from very similar habitat type (i.e., rural areas), which were generally isolated areas far from roads and people. Most of the specimens were adults, so the comparison between mature and immature insects was limited. Bacillus megaterium was the only bacterial species present in triatomine insects from two distant collection sites (Table 1), whereas Staphylococcus saprophyticus and S. sp. YX3 were the only species isolated from both laboratory-reared and field-collected insects. In addition, Staphylococcus saprophyticus was the only species that was collected in different developmental stages, since it was present in two distinct nymphal stages (1st & 2nd) in both the intestinal tract and the feces as well as in the feces of the lab reared adults, the 4th nymphal stage and the intestine of an adult collected in Chiapas (Table 1). The subspecies identified as S. saprophyticus subsp. bovis was also found in most nymphal stages (except for 2nd stage) and adults in both feces and intestinal tract of laboratory-reared specimens.
Availability of Data and Material
Sequences of isolates of rDNA have been uploaded to GenBank, accession numbers KX184211-KX184224.
Discussion
Previous studies have reported a low diversity of bacteria associated with the microbiota of triatomine insects in comparison to other insect groups (Vallejo et al., 2009; da Mota et al., 2012; Gumiel et al., 2015). Our results agree with this observation since we found only 14 different 16S rRNA sequences among all field collecting sites, laboratory specimens, and life stages of the insects. The 14 isolates belong to 13 different species, all within the phyla Firmicutes, Actinobacteria, or Proteobacteria. We believe there are several reasons behind the low amount of bacterial species found in this haematophagous insect family. One of them being the existence of microbiological consortia, which is a group of bacteria that are interdependent among each other at the metabolic level. Therefore if one of the bacteria present within the consortia cannot grow in the simple microbiological culture media, due to a lack to fulfill the resource requirements of the bacterial species, then none of the bacteria of the consortia will be observed in the culture (Rosas et al., 2004). This has previously been observed with Micrococcus luteus, a species that has been used to “resuscitate” viable-but-not-cultivable bacteria, by the action of a cytokine produced by the former (Mukamolova et al., 1998; Su et al., 2014). Another potential factor influencing the low diversity observed could be the restricted diet of the triatomine and physiological structure of their intestine, as observed in another study that looked for bacteria by culture in Rhodnius prolixus (Nyirady, 1973).
Additionally, it has been observed that these haematophagous insects have low microbiota diversity due to the presence of several antimicrobial peptides. In Rhodnius prolixus (the insect model species for Chagas disease), several studies have described the presence of these antimicrobial peptides which are trypanocidal in nature (Vallejo et al., 2009; Ursic-Bedoya et al., 2011). In T. dimidiata the presence of these antimicrobial peptides has not previously been studied, although the low genetic diversity of bacteria we found among all specimens (field-collected and laboratory-reared) and life stages could potentially point toward the presence of these antitrypanocidal peptides in T. dimidiata.
A greater bacterial diversity was found in field-collected insects of T. dimidiata compared to specimens that had been reared in a laboratory colony (Figure 1 and Table 1). This finding is in line with previous studies of other insects (Rani et al., 2009; Belda et al., 2011; Rinke et al., 2011; Gumiel et al., 2015). The potential explanation behind this observation is that insects reared under laboratory conditions are not under the same selective pressure as wild specimens. As a result, there is less selective pressure to preserve the bacterial symbionts that might not be vital for their insect hosts, and therefore laboratory reared insects will have a poorer bacterial diversity. Additionally, insects reared under laboratory conditions have a more homogenous feeding source than in the wild. In our study the bacteria found in laboratory-reared insects were for the most part different than those found in field-collected insects, therefore both ideas could be contributing to the discrepancy in species. There were only two bacterial species of Staphylococcus (i.e., S. saprophyticus and S. gallinarum) present in both field captured and laboratory insects suggesting these species have kept a close association to their insect hosts despite the adaptation of insects to captivity.
These results are also in accordance with a study by da Mota et al. in 2012 that reported the characterization of the bacterial symbionts found in four triatomine genera, including two species of the Triatoma genus that inhabit South America (Triatoma infestans and T. vitticeps) (da Mota et al., 2012).
Concerning the bacterial species found in T. dimidiata, the 14 distinct isolates described were from five different genera (Brevibacterium, Micrococcus, Bacillus, Staphylococcus, and Delftia), where Staphylococcus was the most abundant genus (6/14 isolates). A comparison among the different life stages was only possible to do among the laboratory-reared specimens, since almost all field-collected specimens were adults. One of the most interesting results was the fact that S. saprophyticus was observed in both field-collected and laboratory-reared insects, as well as throughout the distinct life stages of the laboratory-reared insects, suggesting this species plays a very important role in the microbiota of T. dimidiata. The other five species reported from laboratory-reared insects were only observed in one of the life stages (Bacillus thuringiensis/B. cereus, B. avium, S. gallinarum, S. lentus, and S. nepalensis).
Two other studies have reported identification of bacteria as possible symbionts of T. dimidiata in Guatemala (Handler and James, 2000; Matthews et al., 2011). In Southern Mexico, T. dimidiata apparently only shares Micrococcus luteus as an intestinal symbiont with the Guatemalan specimens; in Mexico Bacillus and Staphylococcus spp. are also found and may correspond to the same species reported in Guatemala (B. cereus, St. xilosus, St. equorum and St. simulans). No members of the Gordona genus were found in our study, contrary to the three species found in Guatemala, among them G. rubropertinctus, which has evidence of being a symbiont (Matthews et al., 2011). Therefore, the same vector may have different bacterial populations due to differing environmental conditions.
In addition to the identification of bacterial taxa associated to T. dimidiata, a thorough literature review also allowed us to identify potential phenotypic associations of the reported bacteria with their arthropod hosts. Our analysis found that out of the 13 species identified in our study, Bacillus megaterium and S. saprophyticus appear to be the most common bacterial species described in other arthropods. A variety of studies globally have described both species in insects and mites (Aksoy and Ozman-Sullivan, 2008; Hillesland et al., 2008; Rani et al., 2009; Valiente Moro et al., 2009, 2013; Joyce et al., 2011; Zouache et al., 2011; Mukhopadhyay et al., 2012; Palavesam et al., 2012; Chandel et al., 2013; He et al., 2013; Tagliavia et al., 2014; Zhang et al., 2014; Maleki-Ravasan et al., 2015). Of these two species, S. saprophyticus was the bacterial species that was most reported, but had no potential phenotypic traits associated with its arthropod hosts. Only one study appeared to propose S. saprophyticus was an opportunistic pathogen of Dermanyssus gallinae, which is a haematophagus mite (Valiente Moro et al., 2009). On the other hand, Bacillus megaterium is a gram positive bacteria from the Firmicutes phylum that has been reported to have the enzymes necessary for the biosynthesis of some B vitamins (Eppinger et al., 2011). Given the lack of B vitamins in a blood diet, it is possible that triatomines obtain these nutrients from Bacillus megaterium (Baines, 1956). Transport and assimilation of iron are other possible functions for this microorganism, since solubilization agents for iron have been shown to be produced by B. megaterium (Santos et al., 2014). Therefore, it is unsurprising that previous studies have proposed B. megaterium for its use in paratransgenic studies of sand flies (which are vectors of Leishmaniasis) (Hillesland et al., 2008; Mukhopadhyay et al., 2012). Bacillus thuringiensis although well known for its insecticide use in GMO's, has few reports of being part of arthropod microbiotas (Chandel et al., 2013; Tagliavia et al., 2014), which makes sense given its insecticidal nature. In fact, an experiment by Nyirady (1973) showed that Rhodnius prolixus, also a vector of Chagas' disease, is killed when exposed to the subspecies thuringiensis. Brevibacterium avium and B. iodinum have only been reported in haematophagous arthropods and are described as a non-pathogenic bacteria with low abundance in their arthropod hosts (Hillesland et al., 2008; Valiente Moro et al., 2009; Palavesam et al., 2012). To our knowledge Delftia tsuruhatensis has not been previously reported in other arthropods, making this study the first report of its presence in an insect. Delftia acidovorans has been previously been reported in a omnivorous ground beetle, as well as in the haemtophagous mite Dermanyssus gallinae in which it is reported as a saprophyte (Valiente Moro et al., 2009; Lundgren and Lehman, 2010). Micrococcus luteus, is a gram positive bacteria from the Actinobacteria phylum that has been previously reported to play a role in regulating the growth of other bacteria (Mukamolova et al., 1998). Although in Rhodnius prolixus it was found to halt insect's development when applied to nymphs, in our study it was found in one of the field-collected insects and may be part of the natural occurring bacteria in T. dimidiata (Gumpert, 1962; Table 1). Staphylococcus homins, Staphylococcus lentus, and Staphylococcus nepalensis have all been reported previously in mites and distinct insect orders, with no apparent phenotypic traits associated for S. hominis (Rani et al., 2009; Chandel et al., 2013; Pandey et al., 2013; Maleki-Ravasan et al., 2015). Staphylococcus lentus and Staphylococcus nepalensis have been reported to be opportunistic pathogens in the haematophagous mite Dermanyssus gallinae (Valiente Moro et al., 2009). Additionally in the common housefly Musca domestica, S. lentus is described to be beneficial to the development of its larvae (Zurek et al., 2000). All these findings reinforce the importance the Staphylococcus genus not only Triatominae, but also in a variety of other arthropods.
Conclusions
Current technological methods used for paratransgenic efforts still rely on the laboratory culture of the bacterial symbionts. This study identified cultivable bacterial symbionts in laboratory-reared specimens and in nature. As well as laying the groundwork for the development of future paratransgenic efforts for T. dimidiata (an important vector of Chagas disease in Mexico and Central America). Further studies, particularly assays of dependence, are needed to confirm the types of host-microbe association (mutualists, pathogens, commensals) and to describe the potential function of these bacteria. Finally, experiments on the influence of Bacillus megaterium, Staphylococcus saprophyticus, and Micrococcus luteus on the development of T. dimidiata are needed in order to evaluate the potential use of these bacterial species for paratransgenic efforts done with Chagas disease control programs.
Author Contributions
TL-O designed the experiments and wrote the article; RM-L designed and performed the experiments; CF-L made the phylogenetic analysis and wrote the article; AC-H contributed to the data analyses and critically reviewed the manuscript; EC critically reviewed the manuscript.
Funding
Funding for this project was provided by internal funds from Centro Regional de Investigación en Salud Pública, Instituto Nacional de Salud Pública and Fondo Institucional de Fomento Regional para el Desarrollo Científico, Tecnológico y de Innovación (CONACyT- FORDECyT Grant No. 193512). Erin Conners was supported in part by a UC MEXUS Dissertation grant.
Conflict of Interest Statement
The authors declare that the research was conducted in the absence of any commercial or financial relationships that could be construed as a potential conflict of interest.
Acknowledgments
Authors would like to thank chemists Jose Domingo Mendez, Liliana Lopez-Tirado, and Karen Estefania Gutierrez for technical support during the experiments.
Supplementary Material
The Supplementary Material for this article can be found online at: https://www.frontiersin.org/articles/10.3389/fevo.2017.00174/full#supplementary-material
References
Akman Gündüz, E., and Douglas, A. (2009). Symbiotic bacteria enable insect to use a nutritionally inadequate diet. Proc. R. Soc. B Biol. Sci. 276, 987–991. doi: 10.1098/rspb.2008.1476
Aksoy, H. M., and Ozman-Sullivan, S. K. (2008). Isolation of Bacillus Megaterium from Aphis Pomi (Homoptera: Aphididae) and assessment of its pathogenicity. J. Plant Pathol. 90, 449–452. Available online at: http://www.jstor.org/stable/41998537
Baines, S. (1956). The role of the symbiotic bacteria in the nutrition of rhodnius prolixus (Hemiptera). J. Exp. Biol. 33, 533–541.
Belda, E., Pedrola, L., Peretó, J., Martínez-Blanch, J. F., Montagud, A., Navarro, E., et al. (2011). Microbial diversity in the midguts of field and lab-reared populations of the European corn borer Ostrinia nubilalis. PLoS ONE 6:e21751. doi: 10.1371/journal.pone.0021751
Bugg, T. D., Ahmad, M., Hardiman, E. M., and Singh, R. (2011). The emerging role for bacteria in lignin degradation and bio-product formation. Curr. Opin. Biotechnol. 22, 394–400. doi: 10.1016/j.copbio.2010.10.009
Chandel, K., Mendki, M. J., Parikh, R. Y., Kulkarni, G., Tikar, S. N., Sukumaran, D., et al. (2013). Midgut microbial community of Culex quinquefasciatus mosquito populations from India. PLoS ONE 8:e80453. doi: 10.1371/journal.pone.0080453
da Mota, F. F., Marinho, L. P., Moreira, C. J., Lima, M. M., Mello, C. B., Garcia, E. S., et al. (2012). Cultivation-independent methods reveal differences among bacterial gut microbiota in triatomine vectors of chagas disease. PLoS Negl. Trop. Dis. 6:e1631. doi: 10.1371/journal.pntd.0001631
Darriba, D., Taboada, G. L., Doallo, R., and Posada, D. (2012). jModelTest 2: more models, new heuristics and parallel computing. Nat. Methods 9:772. doi: 10.1038/nmeth.2109
Dasch, G. A., and Weiss, E. (1984). Endosymbionts of Insects. Vol. 1. Baltimore, MA: Williams and Wilkins.
Dias, J. C., Silveira, A. C., and Schofield, C. J. (2002). The impact of Chagas disease control in Latin America: a review. Mem. Inst. Oswaldo Cruz. 97, 603–612. doi: 10.1590/S0074-02762002000500002
Dotson, E. M., Plikaytis, B., Shinnick, T. M., Durvasula, R. V., and Beard, C. B. (2003). Transformation of Rhodococcus rhodnii, a symbiont of the Chagas disease vector Rhodnius prolixus, with integrative elements of the L1 mycobacteriophage. Infect Genet. Evol. 3, 103–109. doi: 10.1016/S1567-1348(03)00002-9
Douglas, A. E. (2011). Lessons from studying insect symbioses. Cell Host Microbe 10, 359–367. doi: 10.1016/j.chom.2011.09.001
Dumonteil, E., Gourbière, S., Barrera-Pérez, M., Rodriguez-Félix, E., Ruiz-Piña, H., Baños-Lopez, O., et al. (2002). Geographic distribution of Triatoma dimidiata and transmission dynamics of Trypanosoma cruzi in the Yucatan peninsula of Mexico. Am. J. Trop Med. Hyg. 67, 176–183. doi: 10.4269/ajtmh.2002.67.176
Dumonteil, E., Nouvellet, P., Rosecrans, K., Ramirez-Sierra, M., Gamboa-León, R., Cruz-Chan, V., et al. (2013). Eco-bio-social determinants for house infestation by Non-domiciliated Triatoma dimidiata in the Yucatan Peninsula, Mexico. PLoS Negl. Trop. Dis. 7:e2466. doi: 10.1371/journal.pntd.0002466
Durvasula, R. V., Sundaram, R. K., Kirsch, P., Hurwitz, I., Crawford, C. V., Dotson, E., et al. (2008). Genetic transformation of a Corynebacterial symbiont from the Chagas disease vector Triatoma infestans. Exp. Parasitol. 119, 94–98. doi: 10.1016/j.exppara.2007.12.020
Edgar, R. C. (2004). MUSCLE: multiple sequence alignment with high accuracy and high throughput. Nucleic Acids Res. 32, 1792–1797. doi: 10.1093/nar/gkh340
Eichler, S., and Schaub, G. A. (2002). Development of symbionts in triatomine bugs and the effects of infections with trypanosomatids. Exp. Parasitol. 100, 17–27. doi: 10.1006/expr.2001.4653
Eppinger, M., Bunk, B., Johns, M. A., Edirisinghe, J. N., Kutumbaka, K. K., Koenig, S. S., et al. (2011). Genome sequences of the biotechnologically important Bacillus megaterium strains QM B1551 and DSM319. J Bacteriol 193, 4199–4213. doi: 10.1128/JB.00449-11
Gouy, M., Guindon, S., and Gascuel, O. (2010). SeaView Version 4: A Multiplatform Graphical User Interface for Sequence Alignment and Phylogenetic Tree Building. Mol. Biol. Evol. 27, 221–224. doi: 10.1093/molbev/msp259
Guhl, F. (2009). Enfermedad de Chagas: Realidad y perspectivas. Rev. Biomed. 20, 228–234. Available online at: http://www.revbiomed.uady.mx/pdf/rb092037.pdf
Gumiel, M., da Mota, F. F., Rizzo, V. de S., Sarquis, O., de Castro, D. P., Lima, M. M., et al. (2015). Characterization of the microbiota in the guts of Triatoma brasiliensis and Triatoma pseudomaculata infected by Trypanosoma cruzi in natural conditions using culture independent methods. Parasit. Vectors 8, 1–17. doi: 10.1186/s13071-015-0836-z
Gumpert, J. (1962). Untersuchungen uber die Symbiose von Tieren mit Pilzen und Bakterien. Z. Allg. Mikro. 2, 290–302.
Hamilton, P. T., Peng, F., Boulanger, M. J., and Perlman, S. J. (2015). A ribosome-inactivating protein in a Drosophila defensive symbiont. Proc. Natl. Acad. Sci. U.S.A. 113:1518648113. doi: 10.1073/pnas.1518648113
Handler, A., and James, A. (2000). Insect Transgenesis Methods and Applications. eds A. Handler and A. James (Boca Raton, FL: CRC Press).
He, C., Nan, X. N., Zhang, Z. Q., and Li, M. (2013). Composition and diversity analysis of the gut bacterial community of the Oriental armyworm, Mythimna separata, determined by culture-independent and culture-dependent techniques. J. Insect Sci. 13:165. doi: 10.1673/031.013.16501
Hillesland, H., Read, A., Subhadra, B., Hurwitz, I., McKelvey, R., Ghosh, K., et al. (2008). Identification of aerobic gut bacteria from the kala azar vector, Phlebotomus argentipes: a platform for potential paratransgenic manipulation of sand flies. Am. J. Trop. Med. Hyg. 79, 881–886. doi: 10.4269/ajtmh.2008.79.881
Huelsenbeck, J. P., and Ronquist, F. (2001). MRBAYES: Bayesian inference of phylogenetic trees. Bioinformatics 17, 754–755. doi: 10.1093/bioinformatics/17.8.754
Hurwitz, I., Fieck, A., Read, A., Hillesland, H., Klein, N., Kang, A., et al. (2011). Paratransgenic control of vector borne diseases. Int. J. Biol. Sci. 7, 1334–1344. doi: 10.7150/ijbs.7.1334
Joyce, J. D., Nogueira, J. R., Bales, A. A., Pittman, K. E., and Anderson, J. R. (2011). Interactions Between La Crosse Virus and Bacteria Isolated From the Digestive Tract of Aedes albopictus (Diptera: Culicidae). J. Med. Entomol. 48, 389–394. doi: 10.1603/Me09268
Kremer, N., Voronin, D., Charif, D., Mavingui, P., Mollereau, B., and Vavre, F. (2009). Wolbachia interferes with ferritin expression and iron metabolism in insects. Plos Pathog. 5:. e1000630. doi: 10.1371/journal.ppat.1000630
Lundgren, J. G., and Lehman, R. M. (2010). Bacterial gut symbionts contribute to seed digestion in an omnivorous beetle. PLoS ONE 5:e10831. doi: 10.1371/journal.pone.0010831
Maleki-Ravasan, N., Oshaghi, M. A., Afshar, D., Arandian, M. H., Hajikhani, S., Akhavan, A. A., et al. (2015). Aerobic bacterial flora of biotic and abiotic compartments of a hyperendemic Zoonotic Cutaneous Leishmaniasis (ZCL) focus. Parasit Vectors 8:63. doi: 10.1186/s13071-014-0517-3
Marchesi, J. R., Sato, T., Weightman, A. J., Martin, T. A., Fry, J. C., Hiom, S. J., et al. (1998). Design and evaluation of useful bacterium-specific PCR primers that amplify genes coding for bacterial 16S rRNA. Appl. Env. Microbiol. 64, 795–799.
Matthews, S., Rao, V. S., and Durvasula, R. V (2011). Modeling horizontal gene transfer (HGT) in the gut of the Chagas disease vector Rhodnius prolixus. Parasit. Vectors 4:77. doi: 10.1186/1756-3305-4-77
Mukamolova, G. V., Kaprelyants, A. S., Young, D. I., Young, M., and Kell, D. B. (1998). A bacterial cytokine. Proc. Natl. Acad. Sci. U.S.A. 95, 8916–8921.
Mukhopadhyay, J., Braig, H. R., Rowton, E. D., and Ghosh, K. (2012). Naturally occurring culturable aerobic gut flora of adult Phlebotomus papatasi, vector of Leishmania major in the Old World. PLoS ONE 7:e35748. doi: 10.1371/journal.pone.0035748.
Muyzer, G., and Smalla, K. (1998). Application of denaturing gradient gel electrophoresis (DGGE) and temperature gradient gel electrophoresis (TGGE) in microbial ecology. Antonie Van Leeuwenhoek 73, 127–141.
Nikoh, N., Hosokawa, T., Moriyama, M., Oshima, K., Hattori, M., and Fukatsu, T. (2014). Evolutionary origin of insect-Wolbachia nutritional mutualism. Proc. Natl. Acad. Sci. U.S.A. 111, 10257–10262. doi: 10.1073/pnas.1409284111
Nyirady, S. A. (1973). The germfree culture of three species of Triatominae: Triatoma protracta (Uhler), Triatoma rubida (Uhler) and Rhodnius prolixus Stål. J. Med. Entomol. 10, 417–448.
Palavesam, A., Guerrero, F. D., Heekin, A. M., Wang, J., Dowd, S. E., Sun, Y., et al. (2012). Pyrosequencing-Based Analysis of the Microbiome Associated with the Horn Fly, Haematobia irritans. PLoS ONE 7:e44390 doi: 10.1371/journal.pone.0044390
Pandey, N., Singh, A., Rana, V. S., and Rajagopal, R. (2013). Molecular characterization and analysis of bacterial diversity in Aleurocanthus woglumi (Hemiptera: Aleyrodidae). Env. Entomol. 42, 1257–1264. doi: 10.1603/EN13110
Picollo, M. I., Vassena, C., Santo Orihuela, P., Barrios, S., Zaidemberg, M., and Zerba, E. (2005). High resistance to pyrethroid insecticides associated with ineffective field treatments in Triatoma infestans (Hemiptera: Reduviidae) from Northern Argentina. J. Med. Entomol. 42, 637–642. doi: 10.1093/jmedent/42.4.637
Rambaut, A., and Drummond, A. (2008). FigTree: Tree Figure Drawing Tool, Version 1.2.2. Available online at: http://tree.bio.ed.ac.uk/software/figtree
Ramsey, J. M., and Schofield, C. J. (2003). Control of Chagas disease vectors. Salud. Public. Mex. 45, 123–128. doi: 10.1590/S0036-36342003000200010
Rani, A., Sharma, A., Rajagopal, R., Adak, T., and Bhatnagar, R. K. (2009). Bacterial diversity analysis of larvae and adult midgut microflora using culture-dependent and culture-independent methods in lab-reared and field-collected Anopheles stephensi-an Asian malarial vector. BMC Microbiol. 9:96. doi: 10.1186/1471-2180-9-96.
Reyes, M., Angulo, V. M., and Sandoval, C. M. (2007). Efecto tóxico de β-cipermetrina, deltametrina y fenitrotión en cepas de Triatoma dimidiata (Latreille, 1811) y Triatoma maculata (Erichson, 1848) (Hemiptera, Reduviidae). Biomédica 27, 75–82. doi: 10.7705/biomedica.v27i1.250
Rinke, R., Costa, A. S., Fonseca, F. P., Almeida, L. C., Delalibera Júnior, I., and Henrique-Silva, F. (2011). Microbial diversity in the larval gut of field and laboratory populations of the sugarcane weevil Sphenophorus levis (Coleoptera, Curculionidae). Genet Mol. Res. 10, 2679–2691. doi: 10.4238/2011.November.1.1
Ronquist, F., and Huelsenbeck, J. P. (2003). MrBayes 3: Bayesian phylogenetic inference under mixed models. Bioinformatics 19, 1572–1574. doi: 10.1093/bioinformatics/btg180
Rosas, I., Cravioto, A., and Ezcurra, E. (2004). Vibrio Cholerae : Bacteria Ambiental con Diferentes Tipos de Vida. Available online at: http://www2.inecc.gob.mx/publicaciones/descarga.html?cv_pub=593&tipo_file=pdf&filename=440
Russell, J. A., Moreau, C. S., Goldman-Huertas, B., Fujiwara, M., Lohman, D. J., and Pierce, N. E. (2009). Bacterial gut symbionts are tightly linked with the evolution of herbivory in ants. Proc. Natl. Acad. Sci. U.S.A. 106, 21236–21241. doi: 10.1073/pnas.0907926106
Ryckman, R. E., and Blankenship, C. M. (1984). The Triatominae and Triatominae-borne Trypanosomes of North and Central America and the West Indies, a bibliography with index. Bull. Soc. Vector Ecol. 9, 112–430.
Santos, S., Neto, I. F., Machado, M. D., Soares, H. M., and Soares, E. V. (2014). Siderophore production by Bacillus megaterium: effect of growth phase and cultural conditions. Appl. Biochem. Biotechnol. 172, 549–560. doi: 10.1007/s12010-013-0562-y
Su, X., Liu, Y., Hu, J., Ding, L., and Shen, C. (2014). Optimization of protein production by Micrococcus luteus for exploring pollutant-degrading uncultured bacteria. Springerplus 3:117. doi: 10.1186/2193-1801-3-117
Tagliavia, M., Messina, E., Manachini, B., Cappello, S., and Quatrini, P. (2014). The gut microbiota of larvae of Rhynchophorus ferrugineus Oliver (Coleoptera: Curculionidae). BMC Microbiol. 14:136. doi: 10.1186/1471-2180-14-136
Taracena, M. L., Oliveira, P. L., Almendares, O., Umaña, C., Lowenberger, C., Dotson, E. M., et al. (2015). Genetically modifying the insect gut microbiota to control Chagas disease vectors through systemic RNAi. PLoS Negl. Trop. Dis. 9:e0003358. doi: 10.1371/journal.pntd.0003358
Ursic-Bedoya, R., Buchhop, J., Joy, J. B., Durvasula, R., and Lowenberger, C. (2011). Prolixicin: a novel antimicrobial peptide isolated from Rhodnius prolixus with differential activity against bacteria and Trypanosoma cruzi. Insect Mol. Biol. 20, 775–786. doi: 10.1111/j.1365-2583.2011.01107.x
Valiente Moro, C., Thioulouse, J., Chauve, C., Normand, P., and Zenner, L. (2009). Bacterial taxa associated with the hematophagous mite Dermanyssus gallinae detected by 16S rRNA PCR amplification and TTGE fingerprinting. Res. Microbiol. 160, 63–70. doi: 10.1016/j.resmic.2008.10.006
Valiente Moro, C., Tran, F. H., Raharimalala, F. N., Ravelonandro, P., and Mavingui, P. (2013). Diversity of culturable bacteria including Pantoea in wild mosquito Aedes albopictus. BMC Microbiol. 13:70. doi: 10.1186/1471-2180-13-70.
Vallejo, G. A., Guhl, F., and Schaub, G. A. (2009). Triatominae-Trypanosoma cruzi/T. rangeli: Vector-parasite interactions. Acta Trop. 110, 137–147. doi: 10.1016/j.actatropica.2008.10.001
Vassena, C. V., Picollo, M. I., and Zerba, E. N. (2000). Insecticide resistance in Brazilian Triatoma infestans and Venezuelan Rhodnius prolixus. Med. Vet. Entomol. 14, 51–55. doi: 10.1046/j.1365-2915.2000.00203.x
Waniek, P. J., Pacheco Costa, J. E., Jansen, A. M., Costa, J., and Araújo, C. A. C. (2012). Cathepsin L of Triatoma brasiliensis (Reduviidae, Triatominae): sequence characterization, expression pattern and zymography. J. Insect Physiol. 58, 178–187. doi: 10.1016/j.jinsphys.2011.11.008
Weeks, E. N. I., Cordón-Rosales, C., Davies, C., Gezan, S., Yeo, M., and Cameron, M. M. (2013). Risk factors for domestic infestation by the Chagas disease vector, Triatoma dimidiata in Chiquimula, Guatemala. Bull. Entomol. Res. 103, 634–643. doi: 10.1017/S000748531300014X
WHO (2015). Chagas Disease. Fact Sheet #340. Available online at: http://www.who.int/mediacentre/factsheets/fs340/en/
Zamora, D. M. B., Hernández, M. M., Torres, N., Zúniga, C., Sosa, W., De Abrego, V., et al. (2015). Information to act: household characteristics are predictors of domestic infestation with the Chagas vector Triatoma dimidiata in central America. Am. J. Trop. Med. Hyg. 93, 97–107. doi: 10.4269/ajtmh.14-0596
Zhang, Z. Q., He, C., and Li, M. L. (2014). Analysis of intestinal bacterial community diversity of adult Dastarcus helophoroides. J. Insect Sci. 14:114. doi: 10.1093/jis/14.1.114
Zouache, K., Raharimalala, F. N., Raquin, V., Tran-Van, V., Raveloson, L. H., Ravelonandro, P., et al. (2011). Bacterial diversity of field-caught mosquitoes, Aedes albopictus and Aedes aegypti, from different geographic regions of Madagascar. FEMS Microbiol. Ecol. 75, 377–389. doi: 10.1111/j.1574-6941.2010.01012.x
Keywords: paratransgenesis, symbionts, Triatoma dimidiata, triatominae, cultivable bacteria
Citation: Lopez-Ordonez T, Flores-López CA, Montejo-Lopez R, Cruz-Hernandez A and Conners EE (2018) Cultivable Bacterial Diversity in the Gut of the Chagas Disease Vector Triatoma dimidiata: Identification of Possible Bacterial Candidates for a Paratransgenesis Approach. Front. Ecol. Evol. 5:174. doi: 10.3389/fevo.2017.00174
Received: 26 June 2017; Accepted: 22 December 2017;
Published: 13 February 2018.
Edited by:
Rebeca Carballar Lejarazu, University of Pavia, ItalyReviewed by:
Vanessa Michelle Macias, Pennsylvania State University, United StatesDavid Georges Biron, Centre National de la Recherche Scientifique (CNRS), France
Copyright © 2018 Lopez-Ordonez, Flores-López, Montejo-Lopez, Cruz-Hernandez and Conners. This is an open-access article distributed under the terms of the Creative Commons Attribution License (CC BY). The use, distribution or reproduction in other forums is permitted, provided the original author(s) and the copyright owner are credited and that the original publication in this journal is cited, in accordance with accepted academic practice. No use, distribution or reproduction is permitted which does not comply with these terms.
*Correspondence: Carlos A. Flores-López, cflores2@uabc.edu.mx