Urbanization Shapes the Ecology and Evolution of Plant-Arthropod Herbivore Interactions
- 1Department of Biology, University of Toronto Mississauga, Mississauga, ON, Canada
- 2Department of Ecology and Evolutionary Biology, University of Toronto, Toronto, ON, Canada
- 3Centre for Urban Environments, University of Toronto Mississauga, Mississauga, ON, Canada
Urbanization is quickly changing natural and agricultural landscapes, with consequences for the herbivorous arthropods dwelling in or near cities. Here, we review the evidence for the effects of urbanization on the ecology and evolution of plant-herbivore interactions. We first summarize how abiotic factors associated with urbanization affect the ecology and evolution of herbivorous arthropods. Next, we explore how urbanization affects plant-herbivore interactions, by considering how urban environments may disrupt top-down and bottom-up ecological processes that affect herbivory. Abiotic changes in the urban environment, such as the urban heat island effect, have caused shifts in phenology for some herbivorous arthropods. Other abiotic changes in urban areas, including water availability, pollution, and habitat fragmentation, have resulted in changes to physiology, behavior, and population abundance. Native species richness tends to decline in urban areas; however, changes in abundance appear to be species specific. These shifts in ecology suggest that urbanization could affect both adaptive and non-adaptive evolution of herbivorous arthropods and their host plants in urban environments. However, plant-herbivore interactions may be dramatically altered if either arthropods or plants are unable to tolerate urban environments. Thus, while some species can physiologically acclimate or genetically adapt to the abiotic urban environment, many species are expected to decline in abundance. We conclude with suggestions for future research to advance our understanding of how urbanization alters the ecology and evolution of plant-herbivore interactions.
Introduction
The ecological and evolutionary interactions between plants and their herbivores are among the most important interactions in nature. Arthropods have consumed plants for over 415 million years (Labandeira, 2007), and their long history of coevolution has led to the diversification of a broad array of phenotypes and species among both arthropods and plants (Ehrlich and Raven, 1964; Thompson, 1994; Althoff et al., 2014). In contemporary communities, nearly 75% of the total biodiversity and biomass of extant macro-organisms is comprised of plants and their herbivores (Strong et al., 1984; Wilson, 2001). Herbivores are responsible for consuming 5–20% of the total biomass produced annually (Cyr and Pace, 1993; Agrawal, 2011; Turcotte et al., 2014), making them among the most important drivers of ecological and ecosystem processes in natural and managed systems.
Urbanization alters natural and historically agricultural landscapes with the development of cities, suburbs and supporting infrastructure (McDonnell and Pickett, 1990; Faeth et al., 2005). The novel, human built environment shows commonalities in many features throughout the world, such as high human population densities, increased impervious surface (e.g., roads and buildings), elevated pollution (e.g., air quality, light, soil), elevated temperatures (Oke, 1973; Ziter et al., 2019), habitat degradation and fragmentation (Grimm et al., 2008; Seto et al., 2011, 2012). However, cities also vary in some characteristics, such as in city age, human population density, urban infrastructure, city size, local climate, socioeconomics, and government regulations (Grimm et al., 2008). These convergent and divergent changes to urban environments create completely novel ecosystems that are unlike any natural environment. These manifold changes to the biotic and abiotic environments in cities present new challenges to herbivorous arthropods, their predators, and host plants, which can influence both the ecology and evolution of plant-arthropod herbivore interactions in or near cities (Figure 1).
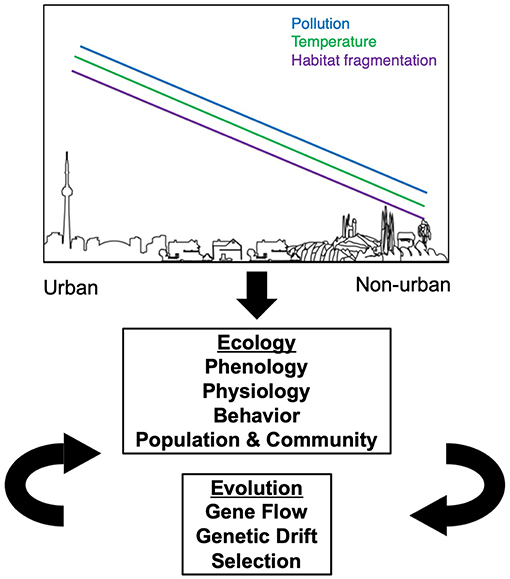
Figure 1. Expected effects of urban environments on the ecology and evolution of herbivorous arthropods. Moving from non-urban to urban landscapes, there is an overall increase in the amount of pollution, temperature, and habitat fragmentation. Each of these three factors influence changes in the ecology of herbivorous arthropods, which in turn can influence their evolution in a feedback loop.
Here, we review evidence for the effects of urbanization on the ecology and evolution of arthropod herbivores and their interactions with plants. We start by summarizing advances in our understanding of how abiotic factors associated with urbanization affect the ecology and evolution of herbivorous arthropods. Next, we explore how urbanization affects plant-herbivore interactions by considering how urban environments may disrupt top-down and bottom-up ecological and evolutionary processes that affect herbivory.
Urban Factors That Can Influence Ecology and Evolution
The urban heat island effect describes the phenomenon whereby cities are warmer than non-urban areas (Oke, 1973; Arnfield, 2003), because of the increased impervious surfaces (e.g., asphalt and concrete) and typically decreased tree cover in cities (Bowler et al., 2010; Ziter et al., 2019). The asphalt and concrete that traps heat can make cities warmer in the day and night throughout the year, which can also extend warm seasons. This urban warming can influence both thermal conditions and breeding seasons of herbivorous arthropods and their predators (Imhoff et al., 2010).
Light and chemical pollution impose stresses on urban-dwelling organisms (Isaksson, 2015). Light pollution alters natural light cycles, which can disrupt circadian rhythms (Gaston et al., 2013). Light pollution can also influence the behavior and, consequently, the survival of arthropods that are attracted to light. Chemical pollution encompasses the release of small particulate matter including harmful airborne gases (e.g., CO, O3, nitrogen oxides) (Isaksson, 2015), which can alter an organism's physiology and behavior, and even increase mutation rates (Yauk and Quinn, 1996; Somers et al., 2002, 2004; Massad and Dyer, 2010). Chemical pollution can also provide nutrients to plants, which influences bottom-up effects on herbivorous arthropods (Martin and Stabler, 2002; Shochat, 2004; Raupp et al., 2010).
The expansion and development of urban areas degrades and fragments the non-urban landscape. This urban expansion decreases the total amount of available natural habitat and increases the isolation of remnant non-urban habitat patches within the urban matrix (Grimm et al., 2008). Organisms that are unable to cross the urban matrix can become isolated and are more likely to experience population declines (Grimm et al., 2008). Such population declines may dramatically alter bottom-up and top-down trophic dynamics within plant-herbivore communities (Hope et al., 2003; McKinney, 2008; Aronson et al., 2014; Figure 2).
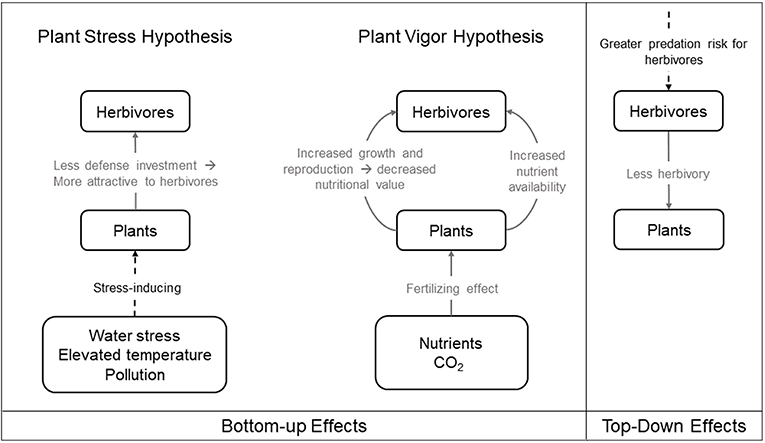
Figure 2. Bottom-up and top-down effects in urban environments. Solid gray line indicates process that benefits recipient, dashed black line indicates process that harms recipient. The left panel illustrates the bottom-up effects on herbivorous arthropods under the two competing hypotheses: the Plant Stress Hypothesis, where plants subject to stress may decrease investment in defenses and thus experience greater herbivore damage, and the Plant Vigor Hypothesis, where plants with more growth are more attractive to herbivores and consequently suffer from greater herbivore attack. The right panel illustrates the top-down effects on herbivorous arthropods.
How Urbanization Affects Herbivorous Arthropods
Urbanization leads to changes in multiple abiotic factors that impact the ecology and evolution of herbivorous arthropods that dwell in or near cities (Table 1). Here, we review how urbanization changes the phenology, physiology, and behavior of populations of herbivorous arthropods, as well as their community assemblage. We then consider how urbanization influences both adaptive and non-adaptive evolution of herbivorous arthropods.
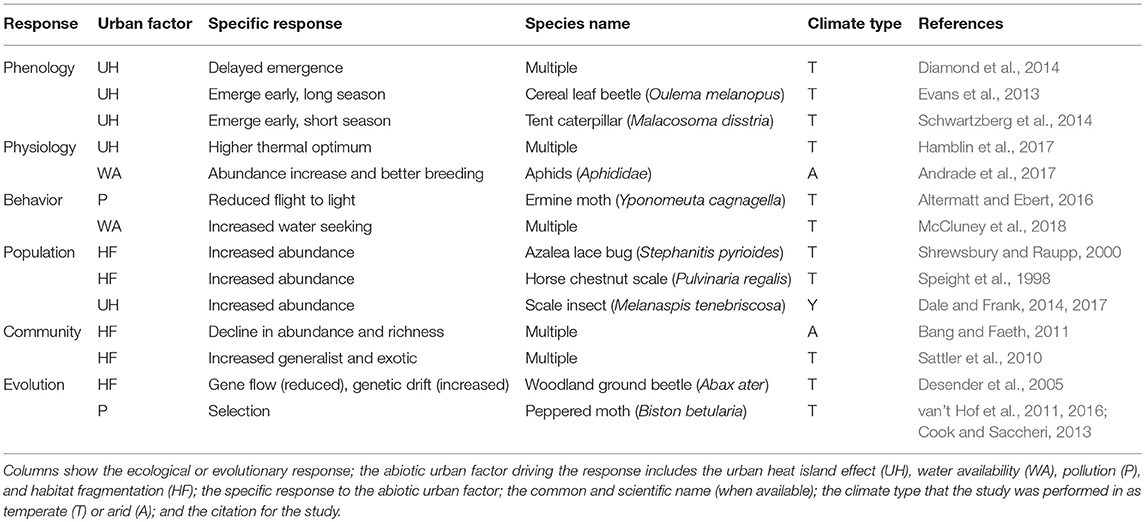
Table 1. Examples of ecological and evolutionary responses to urbanization for herbivorous arthropods.
Physiological Effects
Urbanization can alter water availability through changes in air temperature, humidity, and soil moisture (Hadley, 1994; Sabo et al., 2008; McCluney and Sabo, 2009; Chown et al., 2011; Chown and Duffy, 2015), which can have important physiological consequences for arthropods. Specifically, dehydration is lethal for many arthropods (Hadley, 1994). Water stress likely varies within and between cities, based on the heterogeneous nature of urbanization (Hope et al., 2003; Martin et al., 2004; Schwarz et al., 2015). For example, in the arid city of Phoenix, AZ, USA, aphid abundance within the city is positively correlated with the areas of the city that have increased water availability, a phenomenon caused by irrigation of lawns and parks within the city (Andrade et al., 2017). Because of supplemental water use, arid cities often have reduced water stress on average compared to temperate cities and compared to non-urban areas with similar climate (Buyantuyev and Wu, 2009). For example, during periods of drought, arthropods were less dehydrated in Phoenix, AZ, than arthropods in a temperate city like Raleigh, NC, because the arid city has increased water available compared to non-urban areas as described above. By contrast, temperate cities may also have elevated irrigation in places, but impervious surfaces quickly funnel water to storm drains and sewers instead of into the soil (McCluney et al., 2017). During periods of drought when the soil moisture is depleted, mobile arthropods moved to areas with more water sources. The herbivorous arthropods with reduced mobility significantly increased herbivory on host trees to meet their water demands (McCluney et al., 2018). Therefore, during periods of drought and higher temperatures in temperate cities, where there is less supplemental water available than in arid cities, there can be significant increases in herbivory relative to non-urban areas.
In addition to water availability, urban heat islands can have variable physiological effects on species depending on their thermal optima. Species with higher critical thermal optima tend to be better able to survive in urban areas compared to those with lower critical thermal optima (Youngsteadt et al., 2015; Hamblin et al., 2017). Consequently, species with lower critical thermal optima disproportionately experience population declines in urban areas (Hamblin et al., 2017). Although there seems to be significant variation in thermal tolerance between species, heat tolerance can also be sex-specific. Experimental heatwaves, similar to those found in urban areas, significantly reduced male fertility in the red flour beetle, Tribolium castaneum (Sales et al., 2018). Specifically, male reproductive output was 50% reduced following a heatwave which also carried over to the next generation, and males experienced an even greater reduction in reproduction following a second heatwave. By contrast, females experienced no change in reproductive output under the same heat stress (Sales et al., 2018). Herbivorous arthropods are not only subject to declines from experiencing increased maximum temperatures in urban areas, but also from extended warmer nighttime periods, which can exacerbate the detrimental effects of heat. For example, experimental warming at night reduced survival for the English grain aphid, Sitobion avenae, and reduced adult longevity and fecundity, which together reduced overall population abundance (Zhao et al., 2014). Thus, the diurnal and seasonal effects of elevated urban heat can have detrimental fitness consequences for herbivorous arthropods.
Phenological Shifts
Phenological shifts are expected to occur in herbivorous arthropods when there are phenological limitations associated with lower and upper thermal tolerance (Figure 3). When summer temperatures do not exceed the upper thermal tolerance of organisms, but nevertheless maintain longer warm periods, then we expect an overall longer season in which species are active. When summer temperatures exceed an organism's thermal tolerance, we expect shifts in phenology to earlier (spring) or later (fall) seasons, which may also be shorter if lower thermal tolerance limits do not shift equally. For these reasons, urban heat islands cause some arthropods to shift their phenology, emerging earlier in the spring or later in the fall. For example, in an analysis of 28 species of butterflies in the United Kingdom, those species found in urban areas emerged earlier than the species found in non-urban areas (Dennis et al., 2017). Although urban butterfly species emerged sooner, their total breeding time was generally shorter than that of rural species, indicating that urban butterfly species breed sooner but for a shorter period of time than rural ones (Dennis et al., 2017). Within species, a trend toward earlier emergence in urban areas was observed for 4 of 20 butterfly species studied in Ohio (USA), but another seven species showed significant shifts toward delayed emergence in urban compared to rural populations, and their breeding season was similarly shorter for these species (Diamond et al., 2014). While the breeding season may be shorter for many urban butterflies, other arthropods experience both earlier emergence and an extended breeding season. For example, in New Jersey (USA), urban and agricultural bee communities emerge sooner in the spring than non-urban bees and their breeding season is extended into the fall compared to non-urban bees that end their season in the summer (Harrison et al., 2018). Although the summer season was extended for urban bee communities in general, this extended season is likely species-specific as the bee community assemblages vary significantly between urban and non-urban habitats (Harrison et al., 2018). Therefore, it appears as though a species' thermal tolerance may be one indicator for whether and how urbanization will shift the phenology of an organism (Figure 3).
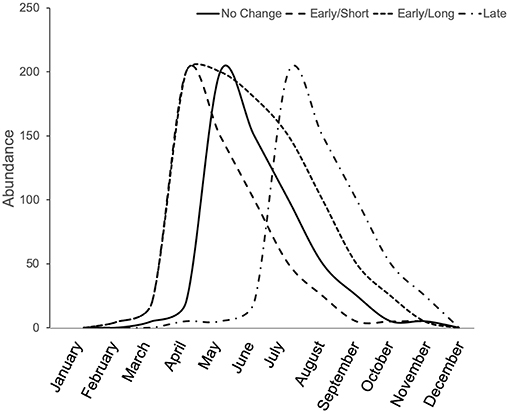
Figure 3. Conceptual illustration of phenological shifts due to the urban heat island effect. Depending on the species' thermal optima and tolerance, the urban heat island effect can result in no change from the natural emergence time (solid line), earlier emergence, potentially with a shorter season (long dash), earlier emergence with an extended season (short dash), or a late emergence, potentially with a shorter season (dash-dot).
Behavioral Shifts
One way that herbivorous arthropods cope with novel urban environments is by changing behavior. Anthropogenic light pollution is inherent to all urban areas and it can disrupt an organism's circadian rhythm (Cinzano et al., 2001; Gaston et al., 2013). Attraction to light can have detrimental consequences for organisms through direct burning from the light source and increased exposure to predators (Warren, 1990; Jones and Francis, 2003). Several arthropod predators (e.g. toads) congregate around lights to take advantage of the flight-to-light behavior (Larsen and Pedersen, 1982). In response to the increased risks associated with flight-to-light in artificially lit urban areas, Altermatt and Ebert (2016) found that some herbivorous arthropods reduce their propensity to fly to light. Urban populations of the herbivorous small ermine moth, Yponomeuta cagnagella, avoid light more than non-urban populations (Altermatt and Ebert, 2016). However, reduced attraction to light may be species-specific and may also be influenced by variation in the lit environment. For example, when street lamps are at high densities, with spectrally diverse light, more moth species are attracted (Plummer et al., 2016). Large moth species are more attracted to light with lamps at high density, whereas small moth species are more attracted to UV- emitting lamps (Plummer et al., 2016). Therefore, the behavioral response to urban factors may be taxon specific. The attraction to light may be linked to body size, where larger arthropods are less timid and thus more attracted to light while smaller ones are more sensitive to predation and thus avoid light in urban areas. It is unclear whether these changes in behavior are the result of phenotypically plastic responses or evolved adaptations, which represents an important avenue for future research.
Population and Community Shifts
The multifarious changes in the environment due to urbanization frequently have large effects on the population ecology of herbivorous arthropods. On average, native species experience reduced abundance and extirpation in urban areas (McIntyre, 2000; Faeth et al., 2005, 2011; Sadler et al., 2006; Sánchez-Bayo and Wyckhuys, 2019). The urban heat island effect can also reduce the abundance of herbivorous arthropods. For example, wild bee abundance declined for every degree Celsius increase caused by urban warming in Raleigh, NC (Hamblin et al., 2018). However, the urban heat island effect can increase population abundance for several herbivorous arthropods, leading to pest outbreaks (Dale and Frank, 2014, 2018; Lahr et al., 2018; Meineke et al., 2018). The scale insect pest, Melanaspis tenebricosa, was most abundant in warmer patches in the city than in cooler patches, causing an infestation on urban street trees which resulted in significant tree damage (Dale and Frank, 2014). Given the differences in abundances among different species, these population responses may be tied to thermal tolerance limits such that the urban heat island causes temperatures beyond a species' tolerance.
Pollution, such as insecticides, will drastically reduce the total abundance of many arthropod species. Pest species, such as termites, are often the target of insecticide use in urban areas as they can cause $40 billion USD annually in damage to human built structures (Rust and Su, 2012). The insecticide use for termites has led to substantial declines in many species of pest termites and new technologies have been developed to reduce the impact of pesticides on non-target species (Su, 2002). Insecticide and herbicide use in agriculture and field margins have caused significant declines in moths and butterflies (Erhardt and Thomas, 1991; Hahn et al., 2015; Forister et al., 2016; Hallmann et al., 2017). In urban gardens, insecticide and herbicide use also causes population declines of local butterfly species (Muratet and Fontaine, 2015). Interestingly, when a targeted pesticide is used and successfully eliminates the target pest, non-target herbivorous pests can experience population outbreaks (Szczepaniec et al., 2011). Regardless of the type of pesticide used, there are typically overall population declines in both pest and non-pest species of herbivorous arthropods.
Environmental heterogeneity in the urban environment leads to reduced arthropod abundance (McIntyre et al., 2001; Rickman and Connor, 2003; Philpott et al., 2014; Vergnes et al., 2014; Smith et al., 2015; Egerer et al., 2017). For example, in Phoenix, AZ, the natural desert and agricultural locations had a significantly higher abundance of herbivorous arthropods than industrial locations (McIntyre et al., 2001). The reduction in abundance is correlated to both habitat type and percent impervious surface with desert having the lowest and industrial having the highest percent impervious surface within the city (McIntyre et al., 2001). The shifts in population abundance are likely species-specific, with pests experiencing “outbreaks” and natives in population decline. As we discuss in the next paragraph, such population shifts are expected to result in changes to the community assemblages within urban areas compared to non-urban habitats.
The total diversity of arthropods in cities can be comparable to the diversity in non-urban areas due to the presence of non-native taxa, which also alter the community composition (Bolger et al., 2000; McIntyre, 2000). Habitat fragmentation in particular has been identified as a driver of shifts in community assembly, whereby communities in habitats that are highly fragmented tend to have more generalist and invasive non-native species than communities with less habitat fragmentation (Bolger et al., 2000; Gibb and Hochuli, 2002; Sattler et al., 2010). Many plants in the urban environment are non-native (Pyšek, 1998; McKinney, 2004; Pickett et al., 2011). This can lead to an abundance of native generalist herbivores, whereas native specialist herbivorous arthropods are likely to experience population declines in urban areas due to a lack of their native host species (Burghardt et al., 2010).
Adaptive and Non-adaptive Evolution
The effects of urbanization on the ecology of arthropods also suggest that urban development could have large impacts on the evolution of arthropod populations. Urbanization can influence gene flow by either creating barriers, such as roads and habitat fragmentation that reduce gene flow (Holderegger and Di Giulio, 2010; Storfer et al., 2010), or habitat corridors and human-mediated dispersal that increase gene flow (Crispo et al., 2011; Arredondo et al., 2018; Miles et al., 2018a,b). Perhaps unsurprisingly, urbanization more frequently reduces gene flow for herbivorous arthropods (Desender et al., 2005; Davis et al., 2010; Schoville et al., 2013; López-Uribe et al., 2015; Vickruck and Richards, 2017) than it elevates it (Keller et al., 2004; Desender et al., 2005; Dronnet et al., 2005). In fact, when calculating the mean pairwise differentiation from the studies that measured the degree of population structure (N = 6; Supplemental Table 1), which is directly affected by the amount of gene flow, there is slightly higher and more variable genetic differentiation (measured by the fixation index FST) between urban populations than between non-urban populations; however, this trend is not significant [t(5) = 1.24, p = 0.26; Figure 4A]. The paucity of studies that measure genetic differentiation between urban and non-urban populations of herbivorous arthropods may be due to the relatively new field of urban evolution and thus patterns of genetic differentiation may become clearer once there are more studies.
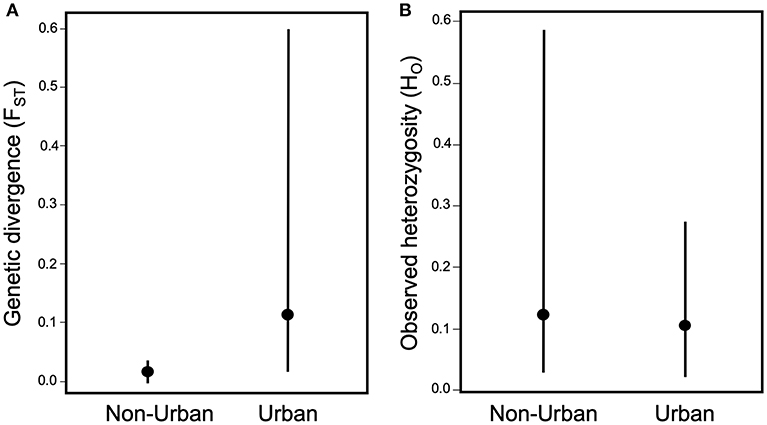
Figure 4. Effect of urbanization on (A) genetic differentiation between populations measured as FST, and (B) genetic diversity within populations measured as observed heterozygosity (HO). Measures of FST are the mean of pairwise values between urban populations and the mean of pairwise values between non-urban populations, extracted from the studies (Supplemental Table 1) of non-adaptive evolution in herbivorous arthropods. Measures of HO are the mean values of urban populations and the mean values of non-urban populations extracted from the studies of non-adaptive evolution (Supplemental Table 1).
Similarly, urbanization typically increases the importance of genetic drift, due to habitat loss that reduces the total population size and fragmentation that reduces local population sizes and restricts gene flow, all of which can reduce the effective population size (Ne) (Johnson and Munshi-South 2017). This increased genetic drift results in reduced genetic diversity within urban populations of herbivorous arthropods (Keller et al., 2004; Desender et al., 2005; Vandergast et al., 2009; Schoville et al., 2013; López-Uribe et al., 2015; Vickruck and Richards, 2017). There is 18% less genetic diversity, measured as observed heterozygosity (HO), in urban compared to non-urban populations across the studies that measured genetic diversity (N = 8); however, this trend is also non-significant [t(7) = 1.19, p = 0.27; Supplemental Table 1; Figure 4B]. If a reduction in genetic diversity is supported more strongly with further studies, then the loss of genetic diversity within urban populations can have multiple negative consequences. Low genetic diversity within populations can increase inbreeding, which can lower fitness due to inbreeding depression and thus reduce local population viability through greater risk of local extinction (Young et al., 1993, 1996; Lowe et al., 2005). Reduced genetic diversity also reduces adaptive evolutionary potential for populations responding to changing environments (Young et al., 1996; Holderegger and Di Giulio, 2010). Therefore, non-adaptive evolution may have a strong influence on the persistence of urban populations for both short-term and long-term.
For populations of herbivorous arthropods that persist or even thrive in urban environments, it is likely that many have undergone rapid evolutionary change (i.e., adaptive evolution) in this new habitat. To date, the peppered moth, Biston betularia, is the only herbivorous arthropod that has been studied in the context of urban adaptation. The industrial pollution that discolored the habitat of the peppered moth, B. betularia, led to an increase in selective predation on the light-colored morph, thus increasing the frequency of the darker-colored morph until pollution mitigation efforts were put in place (Kettlewell, 1955, 1958; Brakefield and Liebert, 2000; Cook et al., 2005; van't Hof et al., 2011, 2016; Cook and Saccheri, 2013). While urbanization can cause direct selective pressures on organisms that live in urban habitats, urban landscapes may also indirectly select for species with specific characteristics. For example, flightless species of beetles are more commonly found in rural environments while species that are capable of flight are found more often in urban environments (Niemelä and Kotze, 2009; Vergnes et al., 2014). These differences in species composition indicate that the urban environment selects for dispersal traits that facilitate movement across highly fragmented urban areas (Vergnes et al., 2014; Aronson et al., 2016). However, it is unclear whether the urban environment acts as an agent of natural selection on the beetle populations. Given all of the factors associated with urbanization that potentially impose natural selection on herbivorous arthropods, there is a need for studies that investigate adaptive evolution. Much of the current literature on adaptive evolution in urban environments is in vertebrate populations, which are often of conservation concern (Rivkin et al., 2018).
How Urbanization Affects Plant-herbivore Interactions
The relationship between urbanization and arthropod herbivory depends both on the degree of urbanization and an herbivore's feeding guild (i.e., leaf-chewing, piercing-sucking, gall-forming, etc.). Previous research has suggested a positive relationship between urbanization and herbivory (Cregg and Dix, 2001). For example, a study of herbarium specimens of four plant species, originating from a region (Northeastern US) that experienced substantial urban development over the past 100+ years, found increasing rates of herbivory through time (Meineke et al., 2018). Conversely, a previous meta-analysis identified no significant correlation between habitat fragmentation and herbivory when considering studies of urban and non-urban sites, even though the abundance and diversity of herbivores decreased (Rossetti et al., 2017). However, other studies suggest that leaf damage in response to fragmentation may be similar among urban and non-urban areas: Christie and Hochuli (2005) found greater herbivory among small remnant edges than among large remnant edges as well as interior sites of both small and large remnants in urban areas, while De Carvalho Guimarães et al. (2014) also found that non-urban edge sites experienced more herbivory than interior sites, especially from Lepidoptera and Orthoptera.
Given the scarcity of studies directly linking urbanization to levels of arthropod herbivory, there is no consensus for which patterns of urban herbivory will occur most often and in various types of cities. Small urban parks may be expected to experience greater herbivore damage than large parks under the predictions posited by Christie and Hochuli (2005) and De Carvalho Guimarães et al. (2014). However, urban parks may experience similar levels of herbivory damage regardless of size under the prediction posed by Rossetti et al. (2017). While relatively few cases have directly linked urbanization with herbivory, several studies shed light on the processes that shape plant-herbivore interactions in cities. These studies, discussed below, illuminate how bottom-up and top-down forces associated with urbanization influence herbivores by benefiting or harming them and their host plants.
Bottom-Up Effects
Urban environmental stressors on plants, including water stress, elevated temperature, and pollution, may affect arthropod herbivores directly, as described in Section “Urban Factors That Can Influence Ecology and Evolution,” or indirectly via bottom-up effects. The Plant Stress Hypothesis (Figure 2) posits that plants subject to stress may decrease investment in defenses and thus endure greater herbivore damage (White, 1969, 1984; see Price, 1991 for review). Two aspects of urbanization, soil compaction and widespread impervious surface coverage, can prevent the absorption of nutrients and water into host plants and render them more susceptible to herbivore colonization (Speight et al., 1998; Huberty and Denno, 2004). Decreased water absorption may also cause plants to have concentrated phloem, with higher amino acid content than watered plants, which can be beneficial to some phloem-sucking insects such as aphids (White, 1984, 2009; Schmitz, 1996), though there is conflicting evidence regarding the effects of chronic water stress on leaf-chewing and phloem-sucking arthropods (Mattson and Haack, 1987; Huberty and Denno, 2004; Meineke and Frank, 2018). Elevated temperatures caused by the urban heat island effect further induces water stress in plants, which makes them grow less and become more susceptible to herbivory in some circumstances (Meineke and Frank, 2018). Water stress also induces stomatal closure, which conserves water but decreases photosynthesis, potentially reducing herbivory from phloem-suckers (Schaffer and Mason, 1990; Hawkes and Sullivan, 2001). As mentioned earlier, elevated urban temperatures that cause decreased arthropod hydration can also lead to increased herbivory (Sabo et al., 2008; McCluney and Sabo, 2009; McCluney et al., 2017). Sap-feeding herbivores may profit from intermittent host water stress (i.e., the Pulsed Stress Hypothesis) by way of increases in nitrogen produced by plants undergoing periodic episodes of stress and recovery (White, 1984; Huberty and Denno, 2004). Roadside herbivores may benefit from leaves with increased nutritional value on trees stressed by de-icing salts (Kropczynska et al., 1988). Fine particulate matter pollutants may further facilitate herbivore attacks, possibly as a result of compromised plant defense (Dohmen, 1985). Thus, the bottom-up effects of urbanization affecting plant defensive chemistry and physiology can benefit herbivores according to the predictions of the Plant Stress Hypothesis (White, 1984), which are associated with detrimental effects on plant growth and fitness.
Urbanization can also increase nutrient deposition and elevate atmospheric carbon dioxide in some circumstances, which can effectively fertilize urban plants and increase their fitness, with concomitant positive effects on herbivore populations. Although the two hypotheses are not mutually exclusive, the Plant Vigor Hypothesis (Figure 2) posits that plants exhibiting vigorous growth may be more susceptible to herbivores and consequently suffer from greater herbivore attack (Price, 1991). Given that plants that thrive in urban areas often prefer high levels of nutrients (Lososová et al., 2006), and they are often limited by nitrogen for growth (LeBauer and Treseder, 2008; Fay et al., 2015), the addition of nutrients from fossil fuel combustion and artificial fertilizers is likely to benefit some herbivores through increased host productivity and quality in specific contexts (Martin and Stabler, 2002; Shochat, 2004; Raupp et al., 2010). For instance, some woody plants exposed to increased nutrient availability benefit urban herbivores including mites, scales, and adelgids (Herms, 2002). Elevated carbon dioxide emission, which is characteristic of cities, can lead to elevated plant growth and reproduction (Bidart-Bouzat et al., 2005). However, these benefits may be counteracted if increased plant growth leads to greater herbivory (Bezemer and Jones, 1998; Stiling and Cornelissen, 2007; Bidart-Bouzat and Imeh-Nathaniel, 2008; Massad and Dyer, 2010). Thus, though multiple aspects of urbanization may enhance plant fitness, these benefits may be short-lived if increased plant vigor ultimately results in intensified herbivore attacks and consequently increases top-down pressure from herbivores.
Top-Down Tri-trophic Effects
Urbanization can also alter top-down tri-trophic cascades (Figure 2). Some insect-eating bird species such as rock pigeons, Columba livia, and the European starling, Sturnus vulgaris, experience high abundance in cities (Aronson et al., 2014), likely because of decreased predation risk (Faeth et al., 2005) and increased food availability (Marzluff et al., 2001), such as through supplementary feeding by humans (Orros and Fellowes, 2012). This increase in bird abundance has been linked to increased predation on urban arthropods (Marzluff et al., 2001; Shochat, 2004), which may also be responsible for decreased foliage loss to some trees (Kozlov et al., 2017). However, more work is needed to understand the role of urbanization in shaping insectivorous bird-prey interactions, as our knowledge is limited to very few studies that suggest that the role of increased bird predation in decreasing herbivore abundance may be weaker than previously thought (but see Faeth et al., 2005; Bang et al., 2012; Turrini et al., 2016). For example, urbanization reduced both insectivorous birds and the gall-forming fly, Eurosta solidaginis, disrupting the typical stabilizing selection of gall size that is found in non-urban areas and thus dampening tri-trophic effects (Start et al., 2018).
Predation from both urban birds and insects may help suppress herbivore outbreaks, though the reduction of herbivores' natural enemies could release herbivores from predator pressure and lead to their proliferation (Raupp et al., 2010). Other tri-trophic cascades reviewed by Aronson et al. (2016), including host-parasite and songbird-nest predator relationships, have been reported to collapse in urban areas (Rodewald et al., 2011; Fischer et al., 2012; Calegaro-Marques and Amato, 2014; Nelson and Forbes, 2014). However, some multi-trophic relationships may persist, as seen with the sustained stability of an ant-aphid mutualism despite existence in a harsh urban environment (Rocha and Fellowes, 2018). More work is needed to clarify the ways in which urbanization affects plant-herbivore-predator interactions, though the majority of studies on tri-trophic interactions suggest a disadvantage to herbivores and thus increase in urban plant fitness.
Evolution of Plant Defenses
Plant defense mechanisms play a large role in structuring the bottom-up and top-down community forces that mediate urban plant-herbivore interactions. Though our understanding of how urbanization influences the evolution of these defense mechanisms is limited, existing research suggests that urbanization can influence the evolution of plant chemical defenses. Presently this has only been studied in white clover, Trifolium repens, which exhibits a Mendelian polymorphism for the production of hydrogen cyanide (HCN), a toxic anti-herbivore chemical defense. At least two studies have shown that plant populations evolve decreased production of HCN within cities, but this evolution does not seem to be related to changes in herbivory. The decreased HCN is instead due to how the presence of the chemical defense affects cold tolerance (Thompson et al., 2016; Johnson et al., 2018). HCN-producing clover experience reduced frost tolerance, whereby lower minimum winter temperatures in cities select against HCN producing plants, in favor of genotypes that lack HCN (Thompson et al., 2016). In addition to chemical defenses, which include secondary metabolites such as cardenolides and tannins (Cipollini and Stiles, 1992), other plant defense mechanisms may be structural (e.g., wax layers, trichomes, thorns; Alba et al., 2011) or phenological, where a plant engages in quick regeneration of vulnerable tissues or avoids herbivory at certain life stages (Saltz and Ward, 2000). Conversely, indirect defenses may involve the recruitment of other organisms (e.g., predators, host-seeking herbivore parasites) to prey on attackers to liberate the plant from herbivory (Sabelis et al., 2001). Studies have also shown that, under relaxed predation threat from herbivores, plants may rapidly evolve a weakened resistance response while increasing their competitive ability (Agrawal et al., 2012; Züst et al., 2012). Future studies investigating the frequency or strength of these defensive strategies in an urban context, such as along an urban-rural gradient, would advance our understanding of the evolution of plant-herbivore interactions in response to urbanization.
Conclusions and Future Directions
Identifying how the ecology and evolution of plant-herbivore interactions are impacted in urban environments will contribute to understanding the role humans play in shaping our environment. As the field is rapidly growing, emerging research cannot be easily simplified into overarching trends: many of the abiotic factors that influence urban herbivorous arthropods appear to be taxon-specific, and the literature suggests varying responses to top-down and bottom-up influences such that some communities stabilize quickly in response to urbanization while others do not. Below, we discuss four research priorities that, if addressed, would markedly improve our understanding of the relationship between urban plants and herbivorous arthropods.
Research in Tropical Cities
The majority of studies focusing on urban plant-herbivore interactions have been conducted in temperate or arid climates. In fact, Raleigh, NC (temperate) and Phoenix, AZ (arid) are overrepresented in much of the studies that identify how urbanization influences herbivorous arthropods and plant-herbivore interactions. One of the reasons that Phoenix, AZ, is overrepresented in this literature is because of the Long-Term Ecological Research (LTER) site, Central Arizona-Phoenix (CAP-LTER), which specifically researches how urbanization influences ecology (Kaiser, 1997). Recently, Magle et al. (2012) identified that the majority of urban wildlife and conservation studies were in North America and Europe, noting that there is a need for future research in tropical ecosystems. Currently, there remains a paucity of tropical urban studies that investigate urban ecology and evolution. This is particularly troublesome as the tropics in Asia, South America, and Africa, which contain some of the world's most prominent and important biodiversity hotspots (Myers et al., 2000; Mcdonald et al., 2008), are areas that are experiencing rapid human population growth and urbanization (Chace and Walsh, 2006).
Many of the conclusions have been taken from studies of temperate regions and one arid region. This limits the overall scope of the many influences that urbanization can have on the ecology and evolution of organisms. For example, water availability varies between types of cities, with temperate cities being less water scarce than arid cities. Perhaps in tropical cities there is less of a pressure for water availability because of natural rainfall. Not only is there a need for studies in the tropics, but also a quantification of the possible stressors that occur between urban and non-urban habitats in each of these ecotypes.
Evolutionary Dynamics
There is a paucity of studies that examine how urbanization affects the evolution of herbivorous arthropods. Perhaps most striking is that only the classic peppered moth has been studied in the context of human impacts on adaptive evolution of an herbivorous arthropod in cities (Kettlewell, 1955, 1958; Brakefield and Liebert, 2000; Cook et al., 2005; van't Hof et al., 2011, 2016; Cook and Saccheri, 2013). In recent reviews of evolution in urban environments, it has been noted that there are only a few studies that have identified evidence for adaptation to urbanization (Donihue and Lambert, 2015; Johnson and Munshi-South, 2017; Rivkin et al., 2018). Urban adaptation has been studied in birds (Yeh, 2004; Partecke and Gwinner, 2007; Mueller et al., 2014), mice (Harris et al., 2013), fish (Whitehead et al., 2010; Wirgin et al., 2011), and even one plant (Cheptou et al., 2008). Studies of urban adaptation continue with a bias toward vertebrates, particularly those of conservation concern (Rivkin et al., 2018). However, given the vastly different life histories, genome sizes, and even mutation rates between vertebrates and invertebrates (e.g., Allio et al., 2017), there remains a large gap in our knowledge of how arthropods may be adapting to the urban environment. Future studies should not only identify potentially adaptive phenotypes, but also investigate the underlying genomic basis of these adaptations. With rapid advances in genome sequencing (Ekblom and Galindo, 2011; Andrews et al., 2016), it is becoming easier and more cost-effective to incorporate more taxa into studies of genome evolution.
Reciprocal Feedbacks Between Ecology and Evolution
It is imperative to account for the interrelationship between ecological and evolutionary processes when studying urban plant-herbivore interactions. Though the fields of urban ecology and urban evolution are still young, it is essential to integrate these perspectives to gain a more complete picture of the eco-evolutionary feedbacks that shape plant-herbivore interactions.
The ecological impacts of urbanization on the evolution of organisms that live in cities is not well-understood, let alone the specific eco-evolutionary relationship between urban-dwelling plants and herbivorous arthropods. Most of our current knowledge of how urbanization affects the evolution of plant defense comes from a single system- white clover, Trifolium repens. It would be valuable to understand how urbanization influences the evolution of plants with varying defense mechanisms in a variety of species, especially those with coevolved specialist herbivores.
Eco-evolutionary feedbacks in urban settings have also been explored in the context of phenotypic selection. Start et al. (2018) describe a tri-trophic interaction among the plant goldenrod, Solidago altissima, a gall-forming parasitic fly, Eurosta solidaginis, and the fly's own predator community, composed of two bird and three parasitoid species. In the absence of urbanization, gall size has been shown to be under stabilizing selection; birds tend to attack larger galls while parasitoids colonize smaller galls (Weis and Abrahamson, 1985). However, the authors conclude that urbanization liberates gall size from this form of selection as gall abundance and avian predation, but not parasitoid presence, increased with distance from the urban center (Start et al., 2018). More research is needed to elucidate the consequences of urbanization on the evolution of multiple populations within communities, and how these ecological changes can then affect community dynamics and patterns.
Heterogeneity of the Urban Landscape
Land use change, habitat fragmentation, and disturbance, which are inherently high in urban areas, are expected to influence population dynamics, the diversity and composition of species, as well as adaptive and non-adaptive dynamics of populations (Rivkin et al., 2018). Although cities tend to exhibit similarities such as increased impervious surface, they can vary in the history of development, disturbance, vegetation cover, and climate (McDonnell et al., 2009; Niemelä, 2011). Additionally, cities are often a mosaic of habitats, with significant heterogeneity of land-use types and constant changes to the landscape within the city (Niemelä, 2011; Pickett et al., 2017). Currently, the consequences of heterogeneity within and between cities has been through the lens of population genetics in vertebrate taxa (reviewed in Rivkin et al., 2018). The effect of such habitat changes and disturbance has not been investigated in the context of plant-herbivore interactions and should be a priority for future research.
Author Contributions
MJ conceived the article. LM and SB wrote the first draft. MJ and HW edited subsequent drafts. All authors wrote the final draft.
Funding
This work was funded by NSERC Discovery grants to MJ and HW, a Canadian Research Chair to MJ, and the NSERC CREATE program ADVENT/ENVIRO.
Conflict of Interest Statement
The authors declare that the research was conducted in the absence of any commercial or financial relationships that could be construed as a potential conflict of interest.
Acknowledgments
We thank LR Rivkin for valuable comments on a draft version of this review. We would also like to thank the reviewers and the editor for their comments and suggestions to improve this review.
Supplementary Material
The Supplementary Material for this article can be found online at: https://www.frontiersin.org/articles/10.3389/fevo.2019.00310/full#supplementary-material
References
Agrawal, A. A. (2011). Current trends in the evolutionary ecology of plant defence. Funct. Ecol. 25, 421–433. doi: 10.1111/j.1365-2435.2010.01796.x
Agrawal, A. A., Hastings, A. P., Johnson, M. T. J., Maron, J. L., and Salminen, J.-P. (2012). Insect herbivores drive real-time ecological and evolutionary change in plant populations. Science 338, 113–116. doi: 10.1126/science.1225977
Alba, J. M., Glas, J. J., Schimmel, B. C. J., and Kant, M. R. (2011). Avoidance and suppression of plant defenses by herbivores and pathogens. J. Plant Interact. 6, 221–227. doi: 10.1080/17429145.2010.551670
Allio, R., Donega, S., Galtier, N., and Nabholz, B. (2017). Large variation in the ratio of mitochondrial to nuclear mutation rate across animals: implications for genetic diversity and the use of mitochondrial DNA as a molecular marker. Mol. Biol. Evol. 34, 2762–2772. doi: 10.1093/molbev/msx197
Altermatt, F., and Ebert, D. (2016). Reduced flight-to-light behaviour of moth populations exposed to long-term urban light pollution. Biol. Lett. 12, 3–6. doi: 10.1098/rsbl.2016.0111
Althoff, D. M., Segraves, K. A., and Johnson, M. T. (2014). Testing for coevolutionary diversification: linking pattern with process. Trends Ecol. Evol. 29, 82–89. doi: 10.1016/j.tree.2013.11.003
Andrade, R., Bateman, H. L., and Kang, Y. (2017). Seasonality and land cover characteristics drive aphid dynamics in an arid city. J. Arid Environ. 144, 12–20. doi: 10.1016/j.jaridenv.2017.04.007
Andrews, K. R., Good, J. M., Miller, M. R., Luikart, G., and Hohenlohe, P. A. (2016). Harnessing the power of RADseq for ecological and evolutionary genomics. Nat. Rev. Genet. 17, 81–92. doi: 10.1038/nrg.2015.28
Arnfield, A. J. (2003). Two decades of urban climate research: a review of turbulence, exchanges of energy and water, and the urban heat island. Int. J. of Climatol. 23, 1–26. doi: 10.1002/joc.859
Aronson, M. F., La Sorte, F. A., Nilon, C. H., Katti, M., Goddard, M. A., Lepczyk, C. A., et al. (2014). A global analysis of the impacts of urbanization on bird and plant diversity reveals key anthropogenic drivers. Proc. Royal Soc. B. 281:20133330. doi: 10.1098/rspb.2013.3330
Aronson, M. F., Nilon, C. H., Lepczyk, C. A., Parker, T. S., Warren, P. S., Cilliers, S. S., et al. (2016). Hierarchical filters determine community assembly of urban species pools. Ecology 97, 2952–2963. doi: 10.1002/ecy.1535
Arredondo, T. M., Marchini, G. L., and Cruzan, M. B. (2018). Evidence for human-mediated range expansion and gene flow in an invasive grass. Proc. R. Soc. B. 285:20181125. doi: 10.1098/rspb.2018.1125
Bang, C., and Faeth, S. H. (2011). Variation in arthropod communities in response to urbanization: seven years of arthropod monitoring in a desert city. Landsc. Urban Plan. 103, 383–399. doi: 10.1016/j.landurbplan.2011.08.013
Bang, C., Faeth, S. H., and Sabo, J. L. (2012). Control of arthropod abundance, richness, and composition in a heterogeneous desert city. Ecol. Monogr. 82, 85–100. doi: 10.1890/11-0828.1
Bezemer, T. M., and Jones, T. H. (1998). Plant-insect herbivore interactions in elevated atmospheric CO2: Quantitative analyses and guild effects. Oikos 82, 212–222. doi: 10.2307/3546961
Bidart-Bouzat, M. G., and Imeh-Nathaniel, A. (2008). Global change effects on plant chemical defenses against insect herbivores. J. Integr. Plant Biol. 50, 1339–1354. doi: 10.1111/j.1744-7909.2008.00751.x
Bidart-Bouzat, M. G., Mithen, R., and Berenbaum, M. R. (2005). Elevated CO2 influences herbivory-induced defense responses of Arabidopsis thaliana. Oecologia 145, 415–424. doi: 10.1007/s00442-005-0158-5
Bolger, D. T., Suarez, A. V., Crooks, K. R., Morrison, S. A., Case, J., Suarez, V., et al. (2000). Arthropods in urban habitat fragments in Southern California: Area, age, and edge effects. Ecol. Appl. 10, 1230–1248. doi: 10.1890/1051-0761(2000)010[1230:AIUHFI]2.0.CO;2
Bowler, D. E., Buyung-Ali, L., Knight, T. M., and Pullin, A. S. (2010). Urban greening to cool towns and cities: a systematic review of the empirical evidence. Landsc. Urban Plan. 97, 147–155. doi: 10.1016/j.landurbplan.2010.05.006
Brakefield, P. M., and Liebert, T. G. (2000). Evolutionary dynamics of declining melanism in the peppered moth in The Netherlands. Proc. R. Soc. B. 267, 1953–1957. doi: 10.1098/rspb.2000.1235
Burghardt, K. T., Tallamy, D. W., Philips, C., and Shropshire, K. J. (2010). Non-native plants reduce abundance, richness, and host specialization in lepidopteran communities. Ecosphere 15, 1–22. doi: 10.1890/ES10-00032.1
Buyantuyev, A., and Wu, J. (2009). Urbanization alters spatiotemporal patterns of ecosystem primary production: a case study of the Phoenix metropolitan region, USA. J. Arid Environ. 73, 512–520. doi: 10.1016/j.jaridenv.2008.12.015
Calegaro-Marques, C., and Amato, S. B. (2014). Urbanization breaks up host-parasite interactions: a case study on parasite community ecology of rufous-bellied thrushes (Turdus rufiventris) along a rural-urban gradient. PLoS ONE 9:e103144. doi: 10.1371/journal.pone.0103144
Chace, J. F., and Walsh, J. J. (2006). Urban effects on native avifauna: a review. Landsc. Urban Plan. 74, 46–69. doi: 10.1016/j.landurbplan.2004.08.007
Cheptou, P.-O., Carrue, O., Rouifed, S., and Cantarel, A. (2008). Rapid evolution of seed dispersal in an urban environment in the weed Crepis sancta. Proc. Natl. Acad. Sci. U.S.A. 105, 3796–3799. doi: 10.1073/pnas.0708446105
Chown, S. L., and Duffy, G. A. (2015). Thermal physiology and urbanization: perspectives on exit, entry and transformation rules. Funct. Ecol. 29, 902–912. doi: 10.1111/1365-2435.12478
Chown, S. L., Sørensen, J. G., and Terblanche, J. S. (2011). Water loss in insects: an environmental change perspective. J. Insect Physiol. 57, 1070–1084. doi: 10.1016/j.jinsphys.2011.05.004
Christie, F. J., and Hochuli, D. F. (2005). Elevated levels of herbivory in urban landscapes: are declines in tree health more than an edge effect? Ecol. Soc. 10, 1–10. doi: 10.5751/ES-00704-100110
Cinzano, P., Falchi, F., and Elvidge, C. D. (2001). The first world atlas of the artificial night sky brightness. Mon. Not. Royal Astron. Soc. 328, 689–707. doi: 10.1046/j.1365-8711.2001.04882.x
Cipollini, M. L., and Stiles, E. W. (1992). Relative risks of microbial rot for fleshy fruits: significance with respect to dispersal and selection for secondary defense. Adv. Ecol. Res. 23, 35–91. doi: 10.1016/S0065-2504(08)60146-4
Cook, L. M., and Saccheri, I. J. (2013). The peppered moth and industrial melanism: evolution of a natural selection case study. J. Hered. 110, 207–212. doi: 10.1038/hdy.2012.92
Cook, L. M., Sutton, S. L., and Crawford, T. J. (2005). Melanic moth frequencies in Yorkshire, an old English industrial hot spot. J. Hered. 96, 522–528. doi: 10.1093/jhered/esi082
Cregg, B. M., and Dix, M. E. (2001). Tree moisture stress and insect damage in urban areas in relation to heat island effects. J. Arboric. 27, 8–17.
Crispo, E., Moore, J., Lee-Yaw, J. A., Gray, S. M., and Haller, B. C. (2011). Broken barriers: human-induced changes to gene flow and introgression in animals. Bioessays 33, 508–518. doi: 10.1002/bies.201000154
Cyr, H., and Pace, M. L. (1993). Magnitude and patterns of herbivory in aquatic and terrestrial ecosystems. Nature 361, 148–150. doi: 10.1038/361148a0
Dale, A. G., and Frank, S. D. (2014). Urban warming trumps natural enemy regulation of herbivorous pests. Ecol. Appl. 24, 1596–1607. doi: 10.1890/13-1961.1
Dale, A. G., and Frank, S. D. (2017). Warming and drought combine to increase pest insect fitness on urban trees. PLoS ONE 12:e0173844. doi: 10.1371/journal.pone.0173844
Dale, A. G., and Frank, S. D. (2018). Urban plants and climate drive unique arthropod interactions with unpredictable consequences. Curr. Opin. Insect Sci. 29, 27–33. doi: 10.1016/j.cois.2018.06.001
Davis, E. S., Murray, T. E., Fitzpatrick, N., Brown, M. J. F., and Paxton, R. J. (2010). Landscape effects on extremely fragmented populations of a rare solitary bee, Colletes floralis. Mol. Ecol. 19, 4922–4935. doi: 10.1111/j.1365-294X.2010.04868.x
De Carvalho Guimarães, C. D., Viana, J. P. R., and Cornelissen, T. (2014). A meta-analysis of the effects of fragmentation on herbivorous insects. Environ. Entomol. 43, 537–545. doi: 10.1603/EN13190
Dennis, E. B., Morgan, B. J. T., Roy, D. B., and Brereton, T. M. (2017). Urban indicators for UK butterflies. Ecol. Indic. 76, 184–193. doi: 10.1016/j.ecolind.2017.01.009
Desender, K., Small, E., Gaublomme, E., and Verdyck, P. (2005). Rural-urban gradients and the population genetic structure of woodland ground beetles. Conserv. Genet. 6, 51–62. doi: 10.1007/s10592-004-7748-3
Diamond, S. E., Cayton, H., Wepprich, T., Jenkins, C. N., Dunn, R. R., Haddad, N. M., et al. (2014). Unexpected phenological responses of butterflies to the interaction of urbanization and geographic temperature. Ecology 95, 2613–2621. doi: 10.1890/13-1848.1
Dohmen, G. P. P. (1985). Secondary effects of air pollution: enhanced aphid growth. Environ. Pollution. Ser. A, Ecol. Biol. 39, 227–234. doi: 10.1016/0143-1471(85)90099-6
Donihue, C. M., and Lambert, M. R. (2015). Adaptive evolution in urban ecosystems. Ambio 44, 194–203. doi: 10.1007/s13280-014-0547-2
Dronnet, S., Chapuisat, M., Vargo, E. L., Lohou, C., and Bagnères, A. G. (2005). Genetic analysis of the breeding system of an invasive subterranean termite, Reticulitermes santonensis, in urban and natural habitats. Mol. Ecol. 14, 1311–1320. doi: 10.1111/j.1365-294X.2005.02508.x
Egerer, M. H., Arel, C., Otoshi, M. D., Quistberg, R. D., Bichier, P., and Philpott, S. M. (2017). Urban arthropods respond variably to changes in landscape context and spatial scale. J. Urban Ecol. 3:jux001. doi: 10.1093/jue/jux001
Ehrlich, P. R., and Raven, P. H. (1964). Butterflies and plants: a study in coevolution. Evolution 18, 586–608. doi: 10.1111/j.1558-5646.1964.tb01674.x
Ekblom, R., and Galindo, J. (2011). Applications of next generation sequencing in molecular ecology of non-model organisms. Heredity 107, 1–15. doi: 10.1038/hdy.2010.152
Erhardt, A., and Thomas, J. A. (1991). “Lepidoptera as indicators of change in the semi-natural grasslands of lowland and upland Europe,” in The Conservation of Insects and their Habitats, eds N. M. Collins and J. A. Thomas (London: Academic Press, 213–237.
Evans, E. W., Carlile, N. R., Innes, M. B., and Pitigala, N. (2013). Warm springs reduce parasitism of the cereal leaf beetle through phenological mismatch. J. Appl. Entomol. 137, 383–391. doi: 10.1111/jen.12028
Faeth, S. H., Bang, C., and Saari, S. (2011). Urban biodiversity: patterns and mechanisms. Ann. N. Y. Acad. Sci. 1223, 69–81. doi: 10.1111/j.1749-6632.2010.05925.x
Faeth, S. H., Marussich, W. A., Shochat, E., and Warren, P. S. (2005). Trophic dynamics in urban communities. Bioscience 55, 399–407. doi: 10.1641/0006-3568(2005)055[0399:TDIUC]2.0.CO;2
Fay, P. A., Prober, S. M., Harpole, W. S., Knops, J. M. H., Bakker, J. D., Borer, E. T., et al. (2015). Grassland productivity limited by multiple nutrients. Nat. Plants 1, 1–5. doi: 10.1038/nplants.2015.80
Fischer, J. D., Cleeton, S. H., Lyons, T. P., and Miller, J. R. (2012). Urbanization and the predation paradox: the role of trophic dynamics in structuring vertebrate communities. BioScience 62, 809–818. doi: 10.1525/bio.2012.62.9.6
Forister, M. L., Cousens, B., Harrison, J. G., Anderson, K., Thorne, J. H., Waetjen, D., et al. (2016). Increasing neonicotinoid use and the declining butterfly fauna of lowland California. Biol. Lett. 12:20160475. doi: 10.1098/rsbl.2016.0475
Gaston, K. J., Bennie, J., Davies, T. W., and Hopkins, J. (2013). The ecological impacts of nighttime light pollution: a mechanistic appraisal. Biol. Rev. 88, 912–927. doi: 10.1111/brv.12036
Gibb, H., and Hochuli, D. F. (2002). Habitat fragmentation in an urban environment: large and small fragments support different arthropod assemblages. Biol. Conserv. 106, 91–100. doi: 10.1016/S0006-3207(01)00232-4
Grimm, N. B., Faeth, S. H., Golubiewski, N. E., Redman, C. L., Wu, J., Bai, X., et al. (2008). Global change and the ecology of cities. Science 319, 756–760. doi: 10.1126/science.1150195
Hahn, M., Schotthöfer, A., Schmitz, J., Franke, L. A., and Brühl, C. A. (2015). The effects of agrochemicals on Lepidoptera, with a focus on moths, and their pollination service in field margin habitats. Agric. Ecosyst. Environ. 207, 153–162. doi: 10.1016/j.agee.2015.04.002
Hallmann, C. A., Sorg, M., Jongejans, E., Siepel, H., Hofland, N., Schwan, H., et al. (2017). More than 75 percent decline over 27 years in total flying insect biomass in protected areas. PLoS ONE 12:e0185809. doi: 10.1371/journal.pone.0185809
Hamblin, A. L., Youngsteadt, E., and Frank, S. D. (2018). Wild bee abundance declines with urban warming, regardless of floral density. Urban Ecosyst. 21, 419–428. doi: 10.1007/s11252-018-0731-4
Hamblin, A. L., Youngsteadt, E., López-Uribe, M. M., and Frank, S. D. (2017). Physiological thermal limits predict differential responses of bees to urban heat-island effects. Biol. Lett. 13:20170125. doi: 10.1098/rsbl.2017.0125
Harris, S. E., Munshi-South, J., Obergfell, C., and O'Neill, R. (2013). Signatures of rapid evolution in urban and rural transcriptomes of white-footed mice (Peromyscus leucopus) in the New York metropolitan area. PLoS ONE 8:e74938. doi: 10.1371/journal.pone.0074938
Harrison, T., Gibbs, J., and Winfree, R. (2018). Forest bees are replaced in agricultural and urban landscapes by native species with different phenologies and life-history traits. Glob. Chang. Biol. 24, 287–296. doi: 10.1111/gcb.13921
Hawkes, C. V., and Sullivan, J. J. (2001). The impact of herbivory on plants in different resource conditions: a meta-analysis. Ecology 82, 2045–2058. doi: 10.1890/0012-9658(2001)082[2045:TIOHOP]2.0.CO;2
Herms, D. (2002). Effects of fertilization on insect resistance of woody ornamental plants: reassessing an entrenched paradigm. Environ. Entomol. 31, 923–933. doi: 10.1603/0046-225X-31.6.923
Holderegger, R., and Di Giulio, M. (2010). The genetic effects of roads: a review of empirical evidence. Basic Appl. Ecol. 11, 522–531. doi: 10.1016/j.baae.2010.06.006
Hope, D., Gries, C., Zhu, W., Fagan, W. F., Redman, C. L., Grimm, N. B., et al. (2003). Socioeconomics drive urban plant diversity. Proc. Natl. Acad. Sci. U.S.A. 100, 8788–8792. doi: 10.1073/pnas.1537557100
Huberty, A. F., and Denno, R. F. (2004). Plant water stress and its consequences for herbivorous insects: a new synthesis. Ecology 85, 1383–1398. doi: 10.1890/03-0352
Imhoff, M. L., Zhang, P., Wolfe, R. E., and Bounoua, L. (2010). Remote sensing of the urban heat island effect across biomes in the continental USA. Remote Sens. Environ. 114, 504–513. doi: 10.1016/j.rse.2009.10.008
Isaksson, C. (2015). Urbanization, oxidative stress and inflammation: a question of evolving, acclimatizing or coping with urban environmental stress. Funct. Ecol. 29, 913–923. doi: 10.1111/1365-2435.12477
Johnson, M. T. J., and Munshi-South, J. (2017). Evolution of life in urban environments. Science 358:eaam8327. doi: 10.1126/science.aam8327
Johnson, M. T. J., Prashad, C. M., Lavoignat, M., and Saini, H. S. (2018). Contrasting the effects of natural selection, genetic drift and gene flow on urban evolution in white clover (Trifolium repens). Proc. Royal Soc. B. 285:20181019. doi: 10.1098/rspb.2018.1019
Jones, J., and Francis, C. M. (2003). The effects of light characteristics on avian mortality at lighthouses. J. Avian Biol. 34, 328–333. doi: 10.1111/j.0908-8857.2003.03183.x
Kaiser, J. (1997). Ecologists to study life in the fast lane. Science 278:375. doi: 10.1126/science.278.5337.375a
Keller, I., Nentwig, W., and Largiadèr, C. R. (2004). Recent habitat fragmentation due to roads can lead to significant genetic differentiation in an abundant flightless ground beetle. Mol. Ecol. 13, 2983–2994. doi: 10.1111/j.1365-294X.2004.02310.x
Kettlewell, H. B. (1955). Recognition of appropriate backgrounds by the pale and black phases of Lepidoptera. Nature 175, 943–944. doi: 10.1038/175943a0
Kettlewell, H. D. (1958). A survey of the frequencies of Biston betularia (L.)(Lep.) and its melanic forms in Great Britain. J. Hered. 12, 51–72. doi: 10.1038/hdy.1958.4
Kozlov, M. V., Lanta, V., Zverev, V., Rainio, K., Kunavin, M. A., and Zvereva, E. L. (2017). Decreased losses of woody plant foliage to insects in large urban areas are explained by bird predation. Glob. Chang. Biol. 23, 4354–4364. doi: 10.1111/gcb.13692
Kropczynska, D., Van De Vrie, M., and Tomczyk, A. (1988). Bionomics of Eotetranychus tiliarium and its phytoseiid predators. Exp. Appl. Acarol. 5, 65–81. doi: 10.1007/BF02053818
Labandeira, C. C. (2007). The origin of herbivory on land: initial patterns of plant tissue consumption by arthropods. Insect Sci. 14, 259–275. doi: 10.1111/j.1744-7917.2007.00152.x
Lahr, E. C., Dunn, R. R., and Frank, S. D. (2018). Getting ahead of the curve: cities as surrogates for global change. Proc. Royal Soc. B. 285:20180643. doi: 10.1098/rspb.2018.0643
Larsen, L. O., and Pedersen, J. N. (1982). The snapping response of the toad Bufo bufo, towards prey dummies at very low light intensities. Amphib-Reptil. 2, 321–327. doi: 10.1163/156853882X00248
LeBauer, D. S., and Treseder, K. K. (2008). Nitrogen limitation of net primary productivity in terrestrial ecosystems is globally distributed. Ecology 89, 371–379. doi: 10.1890/06-2057.1
López-Uribe, M. M., Morreale, S. J., Santiago, C. K., and Danforth, B. N. (2015). Nest suitability, fine-scale population structure and male-mediated dispersal of a solitary ground nesting bee in an urban landscape. PLoS ONE 10:e0125719. doi: 10.1371/journal.pone.0125719
Lososová, Z., Chytrý, M., Kühn, I., Hájek, O., Horáková, V., Pyšek, P., et al. (2006). Patterns of plant traits in annual vegetation of man-made habitats in central Europe. Perspect. Plant Ecol. Evol. Syst. 8, 69–81. doi: 10.1016/j.ppees.2006.07.001
Lowe, A. J., Boshier, D., Ward, M., Bacles, C. F. E., and Navarro, C. (2005). Genetic resource impacts of habitat loss and degradation; Reconciling empirical evidence and predicted theory for neotropical trees. J. Hered. 95, 255–273. doi: 10.1038/sj.hdy.6800725
Magle, S. B., Hunt, V. M., Vernon, M., and Crooks, K. R. (2012). Urban wildlife research: past, present, and future. Biol. Conserv. 155, 23–32. doi: 10.1016/j.biocon.2012.06.018
Martin, C. A., and Stabler, L. B. (2002). Plant gas exchange and water status in urban desert landscapes. J. Arid. Environ. 51, 235–254. doi: 10.1006/jare.2001.0946
Martin, C. A., Warren, P. S., and Kinzig, A. P. (2004). Neighborhood socioeconomic status is a useful predictor of perennial landscape vegetation in residential neighborhoods and embedded small parks of Phoenix, AZ. Landscape Urban Plan. 69, 355–368. doi: 10.1016/j.landurbplan.2003.10.034
Marzluff, J. M., Bowman, R., and Donnelly, R. (2001). Avian Ecology and Conservation in an Urbanizing World. Boston, MA: Kluwer Academic. doi: 10.1007/978-1-4615-1531-9
Massad, T. J., and Dyer, L. A. (2010). A meta-analysis of the effects of global environmental change on plant-herbivore interactions. Arthropod Plant Interact. 4, 181–188. doi: 10.1007/s11829-010-9102-7
Mattson, W. J., and Haack, R. A. (1987). The role of drought in outbreaks of plant-eating insects. Bioscience 37, 110–118. doi: 10.2307/1310365
McCluney, K. E., Burdine, J. D., and Frank, S. D. (2017). Variation in arthropod hydration across US cities with distinct climate. J. Urban Ecol. 3:jux003. doi: 10.1093/jue/jux003
McCluney, K. E., George, T., and Frank, S. D. (2018). Water availability influences arthropod water demand, hydration and community composition on urban trees. J. Urban Ecol. 4:juy003. doi: 10.1093/jue/juy003
McCluney, K. E., and Sabo, J. L. (2009). Water availability directly determines per capita consumption at two trophic levels. Ecology 90, 1463–1469. doi: 10.1890/08-1626.1
Mcdonald, R. I., Kareiva, P., and Forman, R. T. T. (2008). The implications of current and future urbanization for global protected areas and biodiversity conservation. Biol. Conserv. 141, 1695–1703. doi: 10.1016/j.biocon.2008.04.025
McDonnell, M. J., Hahs, A. K., and Breuste, J. H. (2009). Ecology of Cities and Towns: A Comparative Approach. Cambridge, UK: Cambridge University Press. doi: 10.1017/CBO9780511609763
McDonnell, M. J., and Pickett, S. T. A. (1990). Ecosystem structure and function along urban-rural gradients: An unexploited opportunity for ecology. Ecology 71, 1232–1237. doi: 10.2307/1938259
McIntyre, N. E. (2000). Ecology of urban arthropods: a review and a call to action. Ann. Entomol. Soc. Am. 93, 825–835. doi: 10.1603/0013-8746(2000)093[0825:EOUAAR]2.0.CO;2
McIntyre, N. E., Rango, J., Fagan, W. F., and Faeth, S. H. (2001). Ground arthropod community structure in a heterogeneous urban environment. Landsc. Urban Plan. 52, 257–274. doi: 10.1016/S0169-2046(00)00122-5
McKinney, M. L. (2004). Measuring floristic homogenization by non-native plants in North America. Glob. Ecol. Biogeogr. 13, 47–53. doi: 10.1111/j.1466-882X.2004.00059.x
McKinney, M. L. (2008). Effects of urbanization on species richness: a review of plants and animals. Urban Ecosyst. 11, 161–176. doi: 10.1007/s11252-007-0045-4
Meineke, E. K., Classen, A. T., Sanders, N. J., and Davies, J. T. (2018). Herbarium specimens reveal increasing herbivory over the past century. J. Ecol. 107, 105–117. doi: 10.1111/1365-2745.13057
Meineke, E. K., and Frank, S. D. (2018). Water availability drives urban tree growth responses to herbivory and warming. J. Appl. Ecol. 55, 1701–1713. doi: 10.1111/1365-2664.13130
Miles, L. S., Dyer, R. J., and Verrelli, B. C. (2018a). Urban hubs of connectivity: contrasting patterns of gene flow within and among cities in the western black widow spider. Proc. Royal Soc. B. 285:20181224. doi: 10.1098/rspb.2018.1224
Miles, L. S., Johnson, J. C., Dyer, R. J., and Verrelli, B. C. (2018b). Urbanization as a facilitator of gene flow in a human health pest. Mol. Ecol. 27, 3219–3230. doi: 10.1111/mec.14783
Mueller, J. C., Edelaar, P., Carrete, M., Serrano, D., Potti, J., Blas, J., et al. (2014). Behaviour-related DRD 4 polymorphisms in invasive bird populations. Mol. Ecol. 23, 2876–2885. doi: 10.1111/mec.12763
Muratet, A., and Fontaine, B. (2015). Contrasting impacts of pesticides on butterflies and bumblebees in private gardens in France. Biol. Conserv. 182, 148–154. doi: 10.1016/j.biocon.2014.11.045
Myers, N., Mittermeier, R. A., Mittermeier, C. G., da Fonseca, G. A. B., and Kent, J. (2000). Biodiversity hotspots for conservation priorities. Nature 403, 853–858. doi: 10.1038/35002501
Nelson, A. E., and Forbes, A. A. (2014). Urban land use decouples plant-herbivore-parasitoid interactions at multiple spatial scales. PLoS ONE 9:e102127. doi: 10.1371/journal.pone.0102127
Niemelä, J. (2011). Urban Ecology: Patterns, Processes, and Applications. Oxford, UK: Oxford University Press. doi: 10.1093/acprof:oso/9780199563562.001.0001
Niemelä, J., and Kotze, D. J. (2009). Carabid beetle assemblages along urban to rural gradients: a review. Landscape Urban Plan. 92, 65–71. doi: 10.1016/j.landurbplan.2009.05.016
Oke, T. R. (1973). City size and the urban heat island. Atmos. Environ. Pergamon Pres. 7, 769–779. doi: 10.1016/0004-6981(73)90140-6
Orros, M., and Fellowes, M. (2012). Supplementary feeding of wild birds indirectly affects the local abundance of arthropod prey. Basic Appl. Ecol. 13, 286–293. doi: 10.1016/j.baae.2012.03.001
Partecke, J., and Gwinner, E. (2007). Increased sedentariness in European blackbirds following urbanization: a consequence of local adaptation? Ecology 88, 882–890. doi: 10.1890/06-1105
Philpott, S. M., Cotton, J., Bichier, P., Friedrich, R. L., Moorhead, L. C., Uno, S., et al. (2014). Local and landscape drivers of arthropod abundance, richness, and trophic composition in urban habitats. Urban Ecosyst. 17, 513–532. doi: 10.1007/s11252-013-0333-0
Pickett, S. T., Cadenasso, M. L., Grove, J. M., Boone, C. G., Groffman, P. M., Irwin, E., et al. (2011). Urban ecological systems: Scientific foundations and a decade of progress. J. Environ. Manage. 92, 331–362. doi: 10.1016/j.jenvman.2010.08.022
Pickett, S. T. A., Cadenasso, M. L., Rosi-Marshall, E. J., Belt, K. T., Groffman, P. M., Grove, J. M., et al. (2017). Dynamic heterogeneity: A framework to promote ecological integration and hypothesis generation in urban systems. Urban Ecosyst. 20, 1–14. doi: 10.1007/s11252-016-0574-9
Plummer, K. E., Hale, J. D., O'Callaghan, M. J., Sadler, J. P., and Siriwardena, G. M. (2016). Investigating the impact of street lighting changes on garden moth communities. J. Urban Ecol. 2:juw004. doi: 10.1093/jue/juw004
Price, P. W. (1991). The plant vigor hypothesis and herbivore Attack. Oikos 62, 244–251. doi: 10.2307/3545270
Pyšek, P. (1998). Alien and native species in Central European urban floras: a quantitative comparison. J. Biogeogr. 25, 155–163. doi: 10.1046/j.1365-2699.1998.251177.x
Raupp, M. J., Shrewsbury, P. M., and Herms, D. A. (2010). Ecology of Herbivorous Arthropods in Urban Landscapes. Annu. Rev. Entomol. 55, 19–38. doi: 10.1146/annurev-ento-112408-085351
Rickman, J. K., and Connor, E. F. (2003). The effect of urbanization on the quality of remnant habitats for leaf-mining Lepidoptera on Quercus agrifolia. Ecography 26, 777–787. doi: 10.1111/j.0906-7590.2003.03345.x
Rivkin, L. R., Santangelo, J. S., Alberti, M., Aronson, M. F. J., de Keyzer, C. W., Diamond, S. E., et al. (2018). A roadmap for urban evolutionary ecology. Evol. Appl. 18, 1–15. doi: 10.1111/eva.12734
Rocha, E. A., and Fellowes, M. D. E. (2018). Does urbanization explain differences in interactions between an insect herbivore and its natural enemies and mutualists? Urban Ecosyst. 21, 405–417. doi: 10.1007/s11252-017-0727-5
Rodewald, A. D., Kearns, L. J., and Shustack, D. P. (2011). Anthropogenic resource subsidies decouple predator-prey relationships. Ecol. Appl. 21, 936–943. doi: 10.1890/10-0863.1
Rossetti, M. R., Tscharntke, T., Aguilar, R., and Batáry, P. (2017). Responses of insect herbivores and herbivory to habitat fragmentation: a hierarchical meta-analysis. Ecol. Lett. 20, 264–272. doi: 10.1111/ele.12723
Rust, M. K., and Su, N. Y. (2012). Managing social insects of urban importance. Annu. Rev. Ent. 57, 355–375. doi: 10.1146/annurev-ento-120710-100634
Sabelis, M. W., Janssen, A., and Kant, M. R. (2001). The enemy of my enemy is my ally. Science 291, 2104–2105. doi: 10.1126/science.1059939
Sabo, J. L., McCluney, K. E., Marusenko, Y., Keller, A., and Soykan, C. U. (2008). Greenfall links groundwater to aboveground food webs in desert river floodplains. Ecol. Monogr. 78, 615–631. doi: 10.1890/07-1382.1
Sadler, J. P., Small, E. C., Fiszpan, H., Telfer, M. G., and Niemel, äJ. (2006). Investigating environmental variation and landscape characteristics of an urban-rural gradient using woodland carabid assemblages. J. Biogeogr. 33, 1126–1138. doi: 10.1111/j.1365-2699.2006.01476.x
Sales, K., Vasudeva, R., Dickinson, M., Godwin, J., Lumley, A., Michalczyk, Ł., et al. (2018). Experimental heatwaves compromise sperm function and cause transgenerational damage in a model insect. Nat. Commun. 9:4771. doi: 10.1038/s41467-018-07273-z
Saltz, D., and Ward, D. (2000). Responding to a three-pronged attack: desert lilies subject to herbivory by dorcas gazelles. Plant Ecol. 148, 127–138. doi: 10.1023/A:1009895512145
Sánchez-Bayo, F., and Wyckhuys, K. A. (2019). Worldwide decline of the entomofauna: a review of its drivers. Biol. Conserv. 232, 8–27. doi: 10.1016/j.biocon.2019.01.020
Sattler, T., Duelli, P., Obrist, M. K., Arlettaz, R., and Moretti, M. (2010). Response of arthropod species richness and functional groups to urban habitat structure and management. Landsc. Ecol. 25, 941–954. doi: 10.1007/s10980-010-9473-2
Schaffer, B., and Mason, L. J. (1990). Effects of scale insect herbivory and shading on net gas exchange and growth of a subtropical tree species (Guaiacum sanctum L.). Oecologia 84, 468–473. doi: 10.1007/BF00328162
Schmitz, G. (1996). Urban ruderal sites as secondary habitats for phytophagous insects. Verh. Ges. Okol. 26, 581–585.
Schoville, S. D., Widmer, I., Deschamps-Cottin, M., and Manel, S. (2013). Morphological clines and weak drift along an urbanization gradient in the butterfly, Pieris rapae. PLoS ONE 8:e83095. doi: 10.1371/journal.pone.0083095
Schwartzberg, E. G., Jamieson, M. A., Raffa, K. F., Reich, P. B., Montgomery, R. A., and Lindroth, R. L. (2014). Simulated climate warming alters phenological synchrony between an outbreak insect herbivore and host trees. Oecologia 175, 1041–1049. doi: 10.1007/s00442-014-2960-4
Schwarz, K., Fragkias, M., Boone, C. G., Zhou, W., McHale, M., Grove, J. M., et al. (2015). Trees grow on money: Urban tree canopy cover and environmental justice. PLoS ONE 10:e0122051. doi: 10.1371/journal.pone.0122051
Seto, K. C., Fragkias, M., Gueneralp, B., and Reilly, M. K. (2011). A meta-analysis of global urban land expansion. PLoS ONE 6:e23777. doi: 10.1371/journal.pone.0023777
Seto, K. C., Güneralp, B., and Hutyra, L. R. (2012). Global forecasts of urban expansion to 2030 and direct impacts on biodiversity and carbon pools. Proc. Natl. Acad. Sci U.S.A. 109, 16083–16088. doi: 10.1073/pnas.1211658109
Shochat, E. (2004). Credit or debit? Resource input changes population dynamics of city-slicker birds. Oikos 106, 622–626. doi: 10.1111/j.0030-1299.2004.13159.x
Shrewsbury, P. M., and Raupp, M. J. (2000). Evaluation of components of vegetational texture for predicting azalea lace bug, Stephanitis pyrioides (Heteroptera: Tingidae), abundance in managed landscapes. Environ. Entomol. 29, 919–926. doi: 10.1603/0046-225X-29.5.919
Smith, L. S., Broyles, M. E. J., Larzleer, H. K., and Fellowes, M. D. E. (2015). Adding ecological value to the urban lawnscape. Insect abundance and diversity in grass-free lawns. Biodivers. Conserv. 24, 47–62. doi: 10.1007/s10531-014-0788-1
Somers, C. M., McCarry, B. E., Malek, F., and Quinn, J. S. (2004). Reduction of particulate air pollution lowers the risk of heritable mutations in mice. Science 304, 1008–1010. doi: 10.1126/science.1095815
Somers, C. M., Yauk, C. L., White, P. A., Parfett, C. L., and Quinn, J. S. (2002). Air pollution induces heritable DNA mutations. Proc. Natl. Acad. Sci. U.S.A. 99, 15904–15907. doi: 10.1073/pnas.252499499
Speight, M. R., Hails, R. S., Gilbert, M., and Foggo, A. (1998). Horse Chestnut Scale (Pulvinaria regalis) (Homoptera: Coccidae) and Urban host tree environment. Ecology 79, 1503–1513. doi: 10.1890/0012-9658(1998)079[1503:HCSPRH]2.0.CO;2
Start, D., Bonner, C., Weis, A. E., and Gilbert, B. (2018). Consumer-resource interactions along urbanization gradients drive natural selection. Evolution 72, 1863–1873. doi: 10.1111/evo.13544
Stiling, P., and Cornelissen, T. (2007). How does elevated carbon dioxide (CO2) affect plant-herbivore interactions? A field experiment and meta-analysis of CO2-mediated changes on plant chemistry and herbivore performance. Glob. Change Biol. 13, 1823–1842. doi: 10.1111/j.1365-2486.2007.01392.x
Storfer, A., Murphy, M. A., Spear, S. F., Holderegger, R., and Waits, L. P. (2010). Landscape genetics: where are we now? Mol. Ecol. 19, 3496–3514. doi: 10.1111/j.1365-294X.2010.04691.x
Strong, D. R., Lawton, J. H., and Southwood, R. (1984). Insects on Plants. Cambridge, MA: Harvard University Press.
Szczepaniec, A., Creary, S. F., Laskowski, K. L., Nyrop, J. P., and Raupp, M. J. (2011). Neonicotinoid insecticide imidacloprid causes outbreaks of spider mites on elm trees in urban landscapes. PLoS ONE 6:e20018. doi: 10.1371/journal.pone.0020018
Thompson, J. N. (1994). The Coevolutionary Process. Chicago, IL: University of Chicago Press. doi: 10.7208/chicago/9780226797670.001.0001
Thompson, K. A., Renaudin, M., and Johnson, M. T. J. (2016). Urbanization drives parallel adaptive clines in plant populations. Proc. R. Soc. B. 283:20162180. doi: 10.1098/rspb.2016.2180
Turcotte, M. M., Davies, T. J., Thomsen, C. J., and Johnson, M. T. (2014). Macroecological and macroevolutionary patterns of leaf herbivory across vascular plants. Proc. Royal Soc. B. 281:20140555. doi: 10.1098/rspb.2014.0555
Turrini, T., Sanders, D., and Knop, E. (2016). Effects of urbanization on direct and indirect interactions. Ecol. Appl. 26, 664–675. doi: 10.1890/14-1787
Vandergast, A. G., Lewallen, E. A., Deas, J., Bohonak, A. J., Weissman, D. B., and Fisher, R. N. (2009). Loss of genetic connectivity and diversity in urban microreserves in a southern California endemic Jerusalem cricket (Orthoptera: Stenopelmatidae: Stenopelmatus n. sp. “santa monica”). J. Insect Conserv. 13, 329–345. doi: 10.1007/s10841-008-9176-z
van't Hof, A. E., Campagne, P., Rigden, D. J., Yung, C. J., Lingley, J., Quail, M. A., et al. (2016). The industrial melanism mutation in British peppered moths is a transposable element. Nature 534, 102–105. doi: 10.1038/nature17951
van't Hof, A. E., Edmonds, N., Dalíkov, áM., Marec, F., and Saccheri, I. J. (2011). Industrial melanism in British peppered moths has a singular and recent mutational origin. Science 332, 958–960. doi: 10.1126/science.1203043
Vergnes, A., Pellissier, V., Lemperiere, G., Rollard, C., and Clergeau, P. (2014). Urban densification causes the decline of ground-dwelling arthropods. Biodivers. Conserv. 23, 1859–1877. doi: 10.1007/s10531-014-0689-3
Vickruck, J. L., and Richards, M. H. (2017). Nesting habits influence population genetic structure of a bee living in anthropogenic disturbance. Mol. Ecol. 26, 2674–2686. doi: 10.1111/mec.14064
Warren, A. D. (1990). Predation of five species of Noctuidae at ultraviolet light by the Western Yellowjacket (Hymenoptera: Vespidae). J. Lepidopterists' Soc. 44:32.
Weis, A. E., and Abrahamson, W. G. (1985). Potential selective pressures by parasitoids on a plant- herbivore interaction. Ecology 66, 1261–1269. doi: 10.2307/1939179
White, T. C. R. (1969). An index to measure weather-induced stress of trees associated with outbreaks of psyllids in Australia. Ecology 50, 905–909. doi: 10.2307/1933707
White, T. C. R. (1984). The abundance of invertebrate herbivores in relation to the availability of nitrogen in stressed food plants. Oecologia 6, 90–105. doi: 10.1007/BF00379790
White, T. C. R. (2009). Plant vigour versus plant stress: a false dichotomy. Oikos 118, 807–808. doi: 10.1111/j.1600-0706.2009.17495.x
Whitehead, A., Triant, D. A., Champlin, D., and Nacci, D. (2010). Comparative transcriptomics implicates mechanisms of evolved pollution tolerance in a killifish population. Mol. Ecol. 19, 5186–5203. doi: 10.1111/j.1365-294X.2010.04829.x
Wirgin, I., Roy, N. K., Loftus, M., Chambers, R. C., Franks, D. G., and Hahn, M. E. (2011). Mechanistic basis of resistance to PCBs in Atlantic tomcod from the Hudson River. Science 331, 1322–1325. doi: 10.1126/science.1197296
Yauk, C. L., and Quinn, J. S. (1996). Multilocus DNA fingerprinting reveals high rate of heritable genetic mutation in herring gulls nesting in an industrialized urban site. Proc. Natl. Acad. Sci. U.S.A. 93, 12137–12141. doi: 10.1073/pnas.93.22.12137
Yeh, P. J. (2004). Rapid evolution of a sexually selected trait following population establishment in a novel habitat. Evolution 58, 166–174. doi: 10.1111/j.0014-3820.2004.tb01583.x
Young, A., Boyle, T., and Brown, T. (1996). The population genetic consequences of habitat fragmentation for plants. Trends Ecol. Evol. 11, 413–418. doi: 10.1016/0169-5347(96)10045-8
Young, A. G., Merriam, H. G., and Warwick, S. I. (1993). The effects of forest fragmentation on genetic variation in Acer saccharum Marsh. (sugar maple) populations. J. Hered. 71, 277–289. doi: 10.1038/hdy.1993.136
Youngsteadt, E., Dale, A. G., Terando, A. J., Dunn, R. R., and Frank, S. D. (2015). Do cities simulate climate change? A comparison of herbivore response to urban and global warming. Glob. Change Biol. 21, 97–105. doi: 10.1111/gcb.12692
Zhao, F., Zhang, W., Hoffmann, A. A., and Ma, C. S. (2014). Night warming on hot days produces novel impacts on development, survival and reproduction in a small arthropod. J. An. Ecol. 83, 769–778. doi: 10.1111/1365-2656.12196
Ziter, C. D., Pedersen, E. J., Kucharik, C. J., and Turner, M. G. (2019). Scale-dependent interactions between tree canopy cover and impervious surfaces reduce daytime urban heat during summer. Proc. Natl. Acad. Sci. U.S.A. 116:201817561. doi: 10.1073/pnas.1817561116
Keywords: urban, plant-arthropod herbivore interaction, ecological processes, eco-evolutionary feedbacks, herbivorous arthropod
Citation: Miles LS, Breitbart ST, Wagner HH and Johnson MTJ (2019) Urbanization Shapes the Ecology and Evolution of Plant-Arthropod Herbivore Interactions. Front. Ecol. Evol. 7:310. doi: 10.3389/fevo.2019.00310
Received: 30 April 2019; Accepted: 31 July 2019;
Published: 16 August 2019.
Edited by:
Gina Marie Wimp, Georgetown University, United StatesReviewed by:
Christofer Bang, Arizona State University, United StatesHolly Martinson, McDaniel College, United States
Copyright © 2019 Miles, Breitbart, Wagner and Johnson. This is an open-access article distributed under the terms of the Creative Commons Attribution License (CC BY). The use, distribution or reproduction in other forums is permitted, provided the original author(s) and the copyright owner(s) are credited and that the original publication in this journal is cited, in accordance with accepted academic practice. No use, distribution or reproduction is permitted which does not comply with these terms.
*Correspondence: Lindsay S. Miles, lindsay.miles@utoronto.ca; Sophie T. Breitbart, sophie.breitbart@gmail.com