Sexual Dimorphism and Body Reconstruction of a Hornless Rhinocerotid, Plesiaceratherium gracile, From the Early Miocene of the Shanwang Basin, Shandong, China
- 1Human Anatomy and Histology Department, Henan University of Chinese Medicine, Zhengzhou, China
- 2Key Laboratory of Vertebrate Evolution and Human Origins, Institute of Vertebrate Paleontology and Paleoanthropology, Chinese Academy of Sciences, Beijing, China
- 3State Key Laboratory of Palaeobiology and Stratigraphy, Nanjing Institute of Geology and Palaeontology, Chinese Academy of Sciences, Nanjing, China
- 4CAS Center for Excellence in Life and Paleoenvironment, Beijing, China
- 5University of Chinese Academy of Sciences, Beijing, China
- 6Tianyu Museum of Natural History, Pingyi, Shandong, China
- 7Paleontology Department, Museum of Linqu County, Linqu, China
We investigated the sexual dimorphism and reconstructed the body of Plesiaceratherium gracile based on the numerous new specimens from the Early Miocene Shanwang Basin, China. The sexual dimorphism of P. gracile is reflected by the size of both the lower incisor i2 [length male/female (M/F) ratio = 2.69, width M/F = 1.63] and the upper incisor I1 (length M/F = 1.63). Other mensural variations reveal their ranges greatly overlapping on both genders, with a low M/F ratio; hence, they cannot be interpreted as sexual dimorphism. The large-sized body and the strong sexual difference of incisors suggest that P. gracile is polygynous and has a solitary lifestyle. When we reconstructed the body of P. gracile, we found that the head-body length is 2,796–3,117 mm, the shoulder height is 1,476–1,627 mm, and the body weight is about 1,198 kg. All skeletons have the ventral edge of the thorax at the level of the olecranon of the ulna. The spinous processes of the posterior thoracic vertebrae and the lumbar vertebrae are always vertical, the metatarso-femur ratio and the tibio-femur ratio are high, and the two joint angles between the scapula, the humerus, and the radius are 102°–143° and 115°–160°, respectively, indicating that P. gracile has a mediportal limb and a horse-gallop running type. Furthermore, the limb-segment comparisons of P. gracile and other rhinoceroses demonstrate that the proximal long bones, including the scapula, the humerus, and the femur, are important for the body height, regardless of whether their body is large or small-sized; the distal parts of the limb, including the metacarpus and the metatarsus, are sensitive to body mass changes.
Introduction
Intraspecific variation records nearly every aspects of individual difference within a species, the most interesting of which is sexual dimorphism. Pocock (1945) was the first to note that the secondary sexual character of the extant Asiatic rhinoceroses lies in the horns. Groves (1982) recognized more sexual differences in the body size and the skull of the Asiatic rhinoceroses, including several skull measures. Owen-Smith (1988) found that the gender of the African white rhinoceros could be judged based on the difference of the body and horn sizes. Dinerstein (1991) focused on the detailed sexual dimorphism of the greater one-horned rhinoceros, recording the difference of the mandible and the skull. With regard to the fossil rhinocerotids, many studies have revealed that sexual dimorphism lies in the presence of the horn or the size of the lower incisors (Osborn, 1898a, b; Peterson, 1920; Cerdeño and Sánchez, 2000; Mead, 2000; Deng, 2001, 2005; Mihlbachler, 2005, 2007; Chen et al., 2010). However, the sexual dimorphism of the skeleton in fossil rhinoceroses remains controversial. Loose (1975) stated that there is no significant sexual difference in the skull of African species and Dicerorhinus sumatrensis. Borsuk-Bialynicka (1973) mentioned that bimodality in the occiput width, maximum skull length, orbit-nuchal crest length, and orbit-nares length suggested the presence of sexual dimorphism in the skull of Coelodonta antiquitatis. Voorhies (1990) suggested that sexual dimorphism is evident in the ulna and radius dimensions of Barstovian Teleoceras medicornutum and Hemphillian T. fossiger, respectively. Mihlbachler (2007) recorded the skeleton of Menoceras arikarense and suggested that the greater degree of sexual bimodality of the nasal horn may relate to the small-sized body, but found no sexual difference in body size. Mead (2000) provided a detailed analysis on the skeleton of Teleoceras major and pointed out the cross section outline of the concerning long bones has the sexual difference. More recently, the gender of extinct rhinoceroses has been estimated by means of paleoproteomic analyses (Cappellini et al., 2019).
Mass estimation is different based on the equations from different measures and taxonomic levels (Mead, 2000; Qiu and Wang, 2007). Damuth (1990) investigated the linear relation between body weight and the measures of the head-body length and teeth. Janis (1990) focused on the measures of the skull and teeth. Scott (1990) further provided information on the length and width of limb bones. Before Damuth and MacFadden (1990) collected such papers about mass estimation, Legendre (1986) once tried to establish the linear relation between body weight and the crown area of M1, while Anderson et al. (1985) also noted the relation between the circumference of the long bones and the body weight. The equation based on these parameters has been supported by MacFadden and Hulbert (1990) and Mead (2000) for estimating the body weight of rhinoceroses. Cerdeño and Nieto (1995) once used the astragalus to estimate the body size; fortunately, we now have other, more reliable, measures. All these abovementioned equations were established based on data from higher taxa level, such as mammals, all ungulates, or perissodactyls and hyracoids. The earlier work of Freeman and King (1969) tried to establish several equations for the mass estimation of the extant black rhinoceros based on the measures of the head-body length and the limb bones. In this study, we use such different equations to estimate the body weight of Plesiaceratherium gracile.
Size increase is a common feature in nearly all mammals, including the rhinocerotids, but the subfamily Aceratheriinae shows a clear tendency of size decrease (Heissig, 1989; Prothero and Schoch, 1989; Cerdeño and Nieto, 1995; Cerdeño, 1998; Prothero, 2005). P. gracile, from the Early Miocene, is a less specialized representative of Aceratheriinae, characterized by the moderately enlarged upper incisor I1, longer nasal bone, deeper nasal notch, slightly complicated cheek teeth, and longer and slender metapodials (Young, 1937; Yan, 1983; Yan and Heissig, 1986). Based on the ratio of height to length of the second molar (Fortelius et al., 2006), the cheek teeth of P. gracile are mesodont. The Shanwang Basin is famous for the well-preserved skeletons of P. gracile and other large mammals, which were produced from the Early Miocene Shanwang Formation (Young, 1937; Deng et al., 2003). In the Shanwang Formation, there are three fossil beds: the intermediate diatomaceous bed, the underlying sandstone bed, and the overlying sandstone bed. All well-preserved skeletons of P. gracile were recovered from the diatomaceous bed, while specimens from the underlying sandstone bed are very rare and always badly preserved (Yan et al., 1983; Deng et al., 2003). Numerous skeletons of P. gracile give us a chance to analyze sexual dimorphism, reconstruct the body, and provide new insights on the size evolution of the limb bones.
Materials and Methods
All specimens of P. gracile used in this study come from the diatomaceous shale of the Early Miocene Shanwang Basin, with well-preserved bones but with only one side exposed. Therefore, some measures listed in previous studies estimating sexual dimorphism could not be obtained, such as the circumference of the limb bones and the width of the symphysis (Mead, 2000; Chen et al., 2010). Fifty-two specimens are newly reported, except the skull STM 44-68, previously reported by Lu et al. (2016) (Supplementary Table 1). Because there were several very badly crushed skeletons, our study is based on the most accessible and informative specimens. To avoid age-related morphological changes, all specimens used in the analysis were of adult individuals, judged by the established criteria for estimating their age (Goddard, 1970; Hitchins, 1978; Hillman-Smith et al., 1986; Deng, 2001). The measurements are according to the criteria established by Guérin (1980) and Qiu and Wang (2007).
For each measure, we calculated the Student’s t-test and conducted a descriptive analysis, including mean, maximum, minimum, standard deviation, coefficient of variation, kurtosis, skewness, and Shapiro–Wilk test. Following many previous studies, we provided the male/female (M/F) ratio for estimating the sexual difference, which is called dimorphic ratio (dr) for the means of each measure. The new specimens included several skeletons with normal posture, so we could measure the shoulder height, the hind-limb height, and the body length. Along with the measures of the skull, teeth, and limb bones, we reconstructed the skeleton of P. gracile. Meanwhile, recognition of sexual dimorphism offered a chance to discuss the social behavior of P. gracile.
Body weight was estimated using previously established equations, based on length of the skull, head-body, M1, and m1 (Freeman and King, 1969; Damuth, 1990; Janis, 1990; Scott, 1990; Silva and Downing, 1995). As for the extant rhinoceroses, comparative data are from Guérin (1980), and head-body lengths are from different papers (Groves, 1972; Groves and Kurt, 1972; Laurie et al., 1983; Hillman-Smith and Groves, 1994).
For the limb bone, we postulate the locomotion type and compare limb elements between P. gracile and several rhinoceroses. The former was estimated based on the discussion of Gregory (1912) and Slijper (1946), and also the suggestion of Christiansen (2002). The results are determined through line and spine charts.
To avoid long tables, we have provided the abbreviations of the measures here, which are the same as in the text tables, Supplementary Tables, and figures.
Institutional abbreviations are IVPP OV, Vertebrate specimens housed in the Institute of Vertebrate Paleontology and Paleoanthropology (IVPP), Chinese Academy of Sciences, Beijing, China; S, prefix of the fossils from the Early Miocene Shanwang Basin housed in the Museum of Linqu County, Linqu, Shandong, China; SNGPM, Shanwang National GeoPark Museum, Linqu, Shandong, China; STM, Shandong Tianyu Museum, Pinyi, Shandong, China.
Abbreviations of parameters listed in the table of the descriptive analysis are min: minimum; n, number of mensural data; SD, standard deviation; cv, coefficient of variation; skew, skewness; skew.2se, significant test of skewness; kurt, kurtosis; kurt.2se, significant test of kurtosis; sw: Shapiro-Wilk test; p, significance of Student’s t-test; d, dimorphic ratio, male mean/female mean.
Abbreviations for skull are DCAO, distance between occipital crest and lacrimal tubercle or anterior rim of orbit; DCPO, distance between occipital crest and postorbital process; DCN, distance between occipital crest and nasal tip; DOM3, distance between occipital condyle and M3; DON, distance between occipital condyle and nasal tip; DONN, distance between nasal notch and orbit; DONT, distance between orbit and nasal tip; DOP, distance between occipital condyle and premaxillary tip; HMC, height of mandible condyle; HZ, height of zygomatic arch between upper edge and lower edge posterior to the orbit; LM, maximum length of mandible; LN, distance between nasal tips and bottom of nasal notch; LS, length of symphysis; LZ, length of zygomatic arch from anterior edge below orbit to posterior edge above pseudoauditory meatus; WV, maximum width of vertical ramus of mandible.
Abbreviations for teeth are DP, deciduous upper premolars; I, upper incisors; i, lower incisors; L, length; M, upper molars; m, lower molars; P, upper premolars; p, lower premolars; W, ventral width.
Abbreviations for postcranial bones are BL, body length; C.V., cervical vertebra; DWMc3, distal width of Mc III; FFA, fore-limb first joint angle; FSA, fore-limb second joint angle; FLP1, maximum length of first phalanx of fore-limb; FLP2, maximum length of second phalanx of fore-limb; FLP3, maximum length of third phalanx of fore-limb; FPWP1, maximum proximal width of first phalanx of fore-limb; FPWP2, maximum proximal width of second phalanx of fore-limb; FPWP3, maximum proximal width of third phalanx of fore-limb; HFA, hind-limb first joint angle; HLP1, maximum length of first phalanx of hind-limb; HLP2, maximum length of second phalanx of hind-limb; HLP3, maximum length of third phalanx of hind-limb; HPWP1, maximum proximal width of first phalanx of hind-limb; HPWP2, maximum proximal width of second phalanx of hind-limb; HPWP3, maximum proximal width of third phalanx of hind-limb; HSA, hind-limb second joint angle; LF, maximum length of femur; LH, maximum length of humerus; LMc3, maximum length of Mc III; LMt3, maximum length of Mt III; LR, maximum length of radius; LS, maximum length of scapula; LT, maximum length of tibia; L.V., lumbar vertebrae; Mc, metacarpus; Mt, metatarsus; NWMc3, narrowest width of Mc III; PWF, maximum proximal width of femur; PWH, maximum proximal width of humerus; PWMc3, maximum proximal width of Mc III; PWMt3, maximum proximal width of Mt III; PWR, maximum proximal width of radius; PWS, maximum proximal width of scapula; SH, shoulder height; TPW, maximum proximal width of tibia; T.V., thoracic vertebrae.
Abbreviations of body mass estimation are HBL, head and body length; SUML, antero-posterior length of upper molar (M1); SLML, antero-posterior length of lower molar (m1).
Results
Sexual Dimorphism
Plesiaceratherium is a hornless rhinoceros, so the gender cannot be determined based on the horn difference, which is relatively evident on some horned rhinocerotids. Given that the size of incisors has long been recognized as reflecting sexual dimorphism, we first investigated this feature and found that the length of i2 is the most dimorphic character (dr = 2.69), followed by its width (dr = 1.63) (Figures 1–3 and Table 1). All accessible specimens for measuring i2 were adult individuals, with moderately worn cheek teeth. The length of i2 has a wider range in the male. Concerning upper incisors, we noted the length measures of 10 I1 of the adult specimens, including two older females and one older male with highly worn M1. The measures of I1 between the two genders are widely separated, and the mean ratio is similar to that of the width of i2 (dr = 1.63) (Figure 3, Supplementary Figure 1, and Table 1).
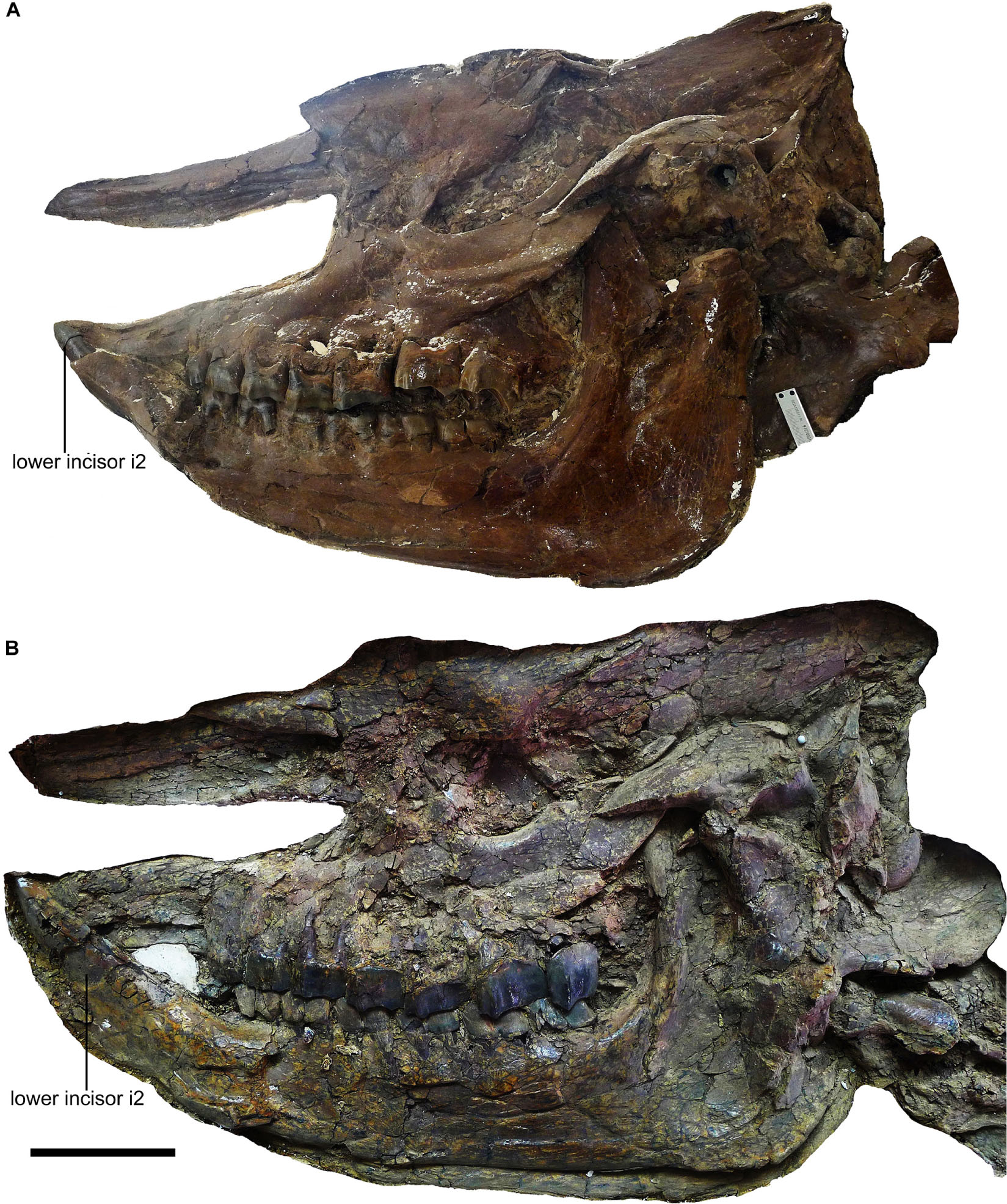
Figure 1. Skulls of Plesiaceratherium gracile from the Early Miocene Shanwang Basin. (A) STM 44-62, the female skull; (B) STM 44-127, the male skull. Scale bar = 100 mm.
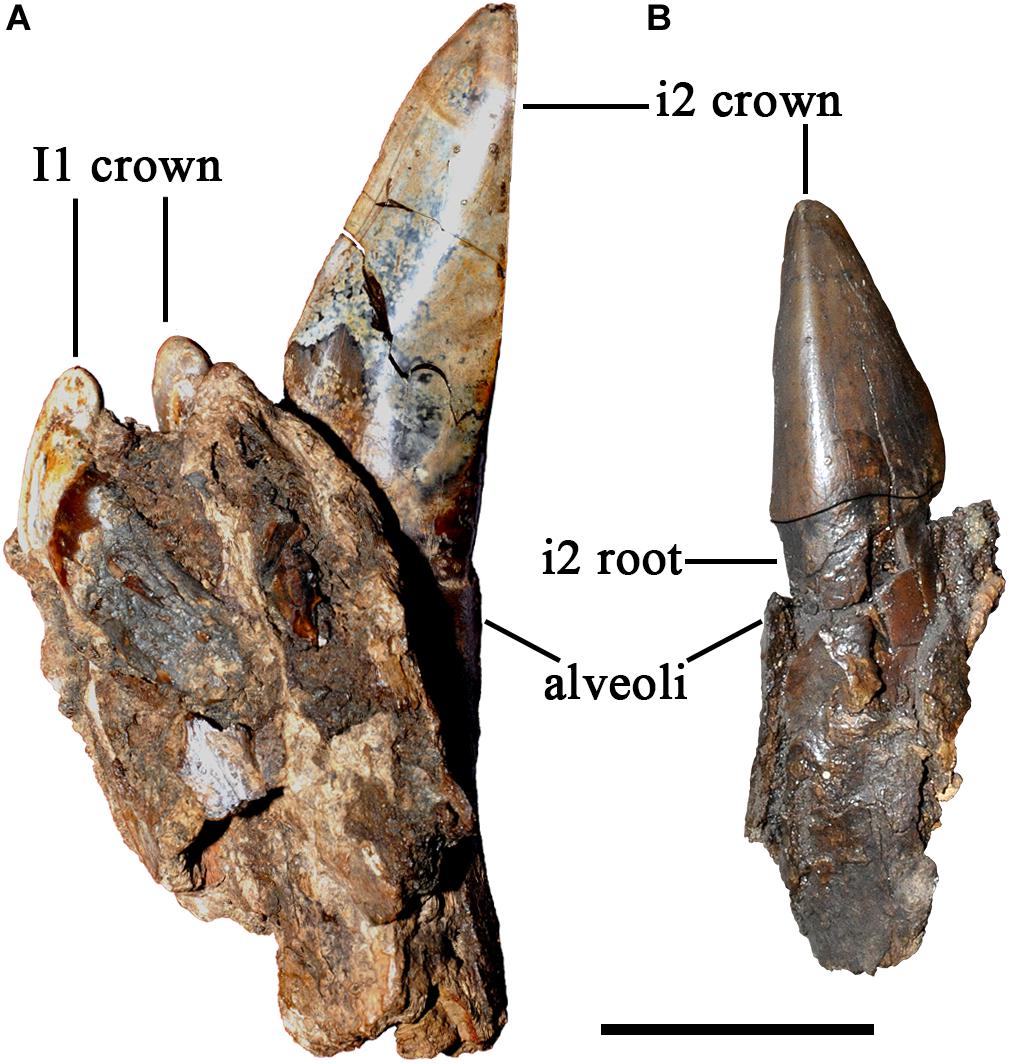
Figure 2. Incisors of Plesiaceratherium gracile from the Early Miocene Shanwang Basin. (A) S700015, male, ventral view of i2; (B) S700014, female, occlusal view of incisor i2. Scale bar = 20 mm.
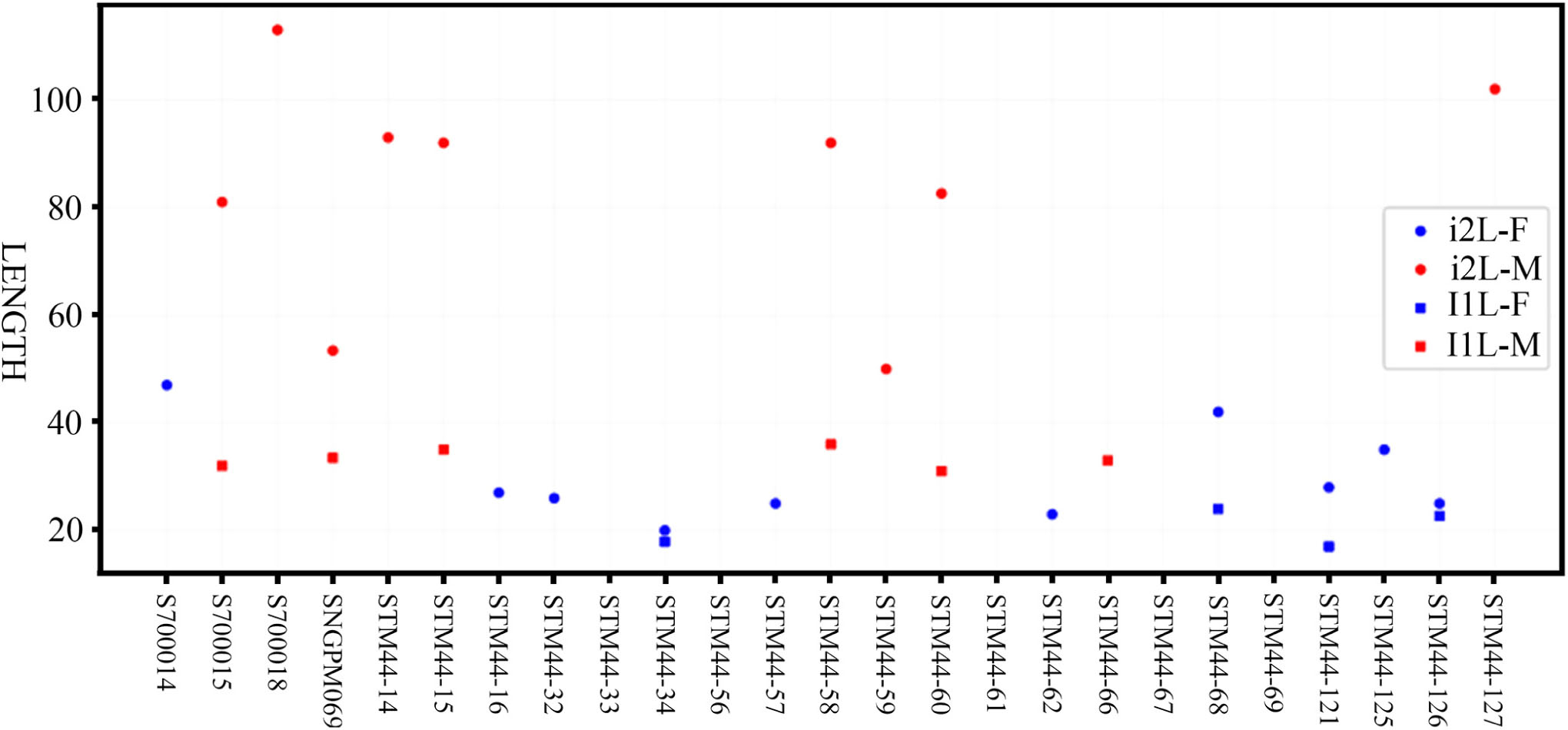
Figure 3. Length distribution of the upper (I1) and lower (i2) incisors of Plesiaceratherium gracile from the Early Miocene Shanwang Basin. Original data in Supplementary Data 3.
Meanwhile, based on the dimorphism of the incisors, we compared several non-mensural characters between two genders, such as the posterior position of the nasal notch and the presence of the mesostyle of P2. The posterior position of the symphysis is below dp1 or moved backward to p2, bearing an evident intraspecific variation but not being sexually significant. The presence of the first lower cheek teeth dp1 is variable and has no sexual difference or other non-mensural characters (Supplementary Table 1).
Among the cheek tooth measures, the lengths of P2 and M1 seem to have a significant Student’s t-test value, p < 0.05 (Table 2). However, the M/F ratio of these two measures is 1.09, much lower than the value of the incisors, and the lengths between two genders are greatly overlapping.
On the skull, the mean of the female length is longer than that of the male in several measures, including DCN (dr = 0.97), LN (DR = 0.93), DONN (dr = 0.89), DONT (dr = 0.95), and LZ (dr = 0.95) (Table 3). The ranges of these measures between two genders are overlapping, and their t-test values are not significant. On the other hand, the height of the zygomatic arch varies more widely in the male specimens and has a relatively higher sex ratio (dr = 1.06) (Table 3). Most of these measures are in a normal distribution and have a low value of skewness and kurtosis. Meanwhile, the t-test value is not significant. The measures of the symphysis on both genders are nearly totally overlapping (Table 4).
Like measures of the skull, the limb bones of the female specimens always have a larger mean than do the male. However, the scapula in the male is longer than that of the female (dr = 1.04), with the latter having a higher kurtosis. The humerus and the radius have the same M/F ratio (dr = 0.98) and a lower cv. The M/F ratio of the Mc III is more evident (dr = 0.96), and the male has a relatively wider range, 172–205 mm. Furthermore, the female has a longer femur and tibia, but the ratio is lower. The length of the Mt III in the male is slightly longer than in the female (dr = 1.01), and the proximal width has a higher M/F ratio, up to 1.2. It is noteworthy that all mean measures of proximal, narrowest, and distal widths of the long bones in the male specimens are larger than those of the female (Table 5).
Body Size
The direct measure of seven articulated skeletons of P. gracile revealed that the body length from the atlas to the ischial tuberosity is 2,148–2,469 mm (Supplementary Table 5). The mean skull length is 649 mm (Table 3) and mean of the head-body length of P. gracile is 2,991 mm, with a range of 2,796–3,117 mm. We measured different parts of the spinal column of the different individuals and composed a complete vertebral series. Based on the nearly undeformed pelvis of S 700018, the inclination of the ala-acetabulum axis of the coxa relative to the horizontal plane is about 56.4°. Combining such different parts of the body, including the 7 C.V., 18 T.V., 5 L.V., and the pelvis, the estimated body length is about 2,162 mm and the head-body length (considering the mean head length) is about 2,811 mm, within the range of the direct measure mentioned above (Supplementary Table 6). The spinous process of the T.V. is inclined posteriorly, but sometimes it is vertical in the last two T.V. The L.V. also have a vertical spinous process. In the medium-sized individual STM 44-62, the linear length of the rib is about 830 mm. Regardless of the size, all skeletons have the ventral edge of the thorax flushing with the olecranon of the ulna.
Based on five less distorted skeletons, the shoulder height from the tip of the T.V. to the distal extremity of the fore-limb is about 1,491.8 mm, with a range of 1,438–1,603 mm, and the height of the hind-limb is about 1,526 mm, with a range 1,476–1,627 mm (Supplementary Table 5). Given that all skeletons have curled bodies as a result of drowning in the lake, the shoulder height probably has been underestimated, and it is also difficult to precisely predict the joint angle between the adjacent long bones. Among numerous skeletons, only STM 44–60 has a nearly straight fore-limb; its shoulder height is 1,603 mm, and the angle between the scapula and the humerus is 143° and between the humerus and the radius is 160°. In the slightly curled body S 700018, the first angle of the fore-limb is 128° and the second is 131°. In the most curled skeleton STM 44–61, the first angle of the fore-limb is 102°, the second is 115°; the angle of the hind-limb between the coxa and the femur is 101.2° and between the femur and the tibia is 91.8°. We could not find a skeleton with an intact hind-limb, but S 700018 is just slightly curled and could be used for body reconstruction: the first angle is 133.1° and the second is 137.8°. All accessible skeletons show a slightly longer hind-limb although they are deformed in a different degree. In the best-preserved S 700018, the hind-limb from the tip of the sacrum to the distal extremity is 1,532 mm, slightly longer than the fore-limb. The reconstruction of the joint angle of the fore-limb is based on STM 44–60, while that of the hind-limb is based on the S 700018. Given that the curled body and limb would inevitably reduce the body height, we used the larger value for the skeleton reconstruction (Figure 4).
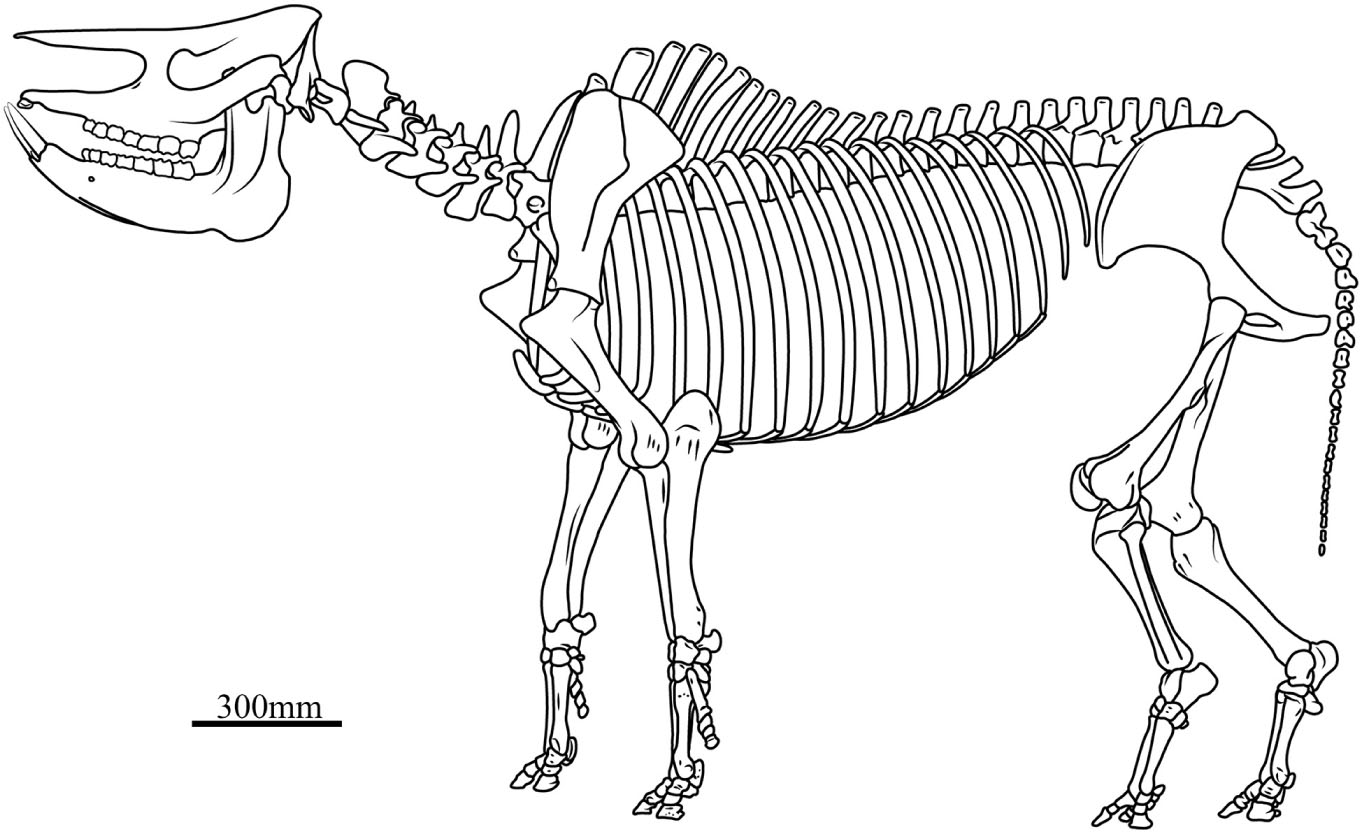
Figure 4. Skeletal reconstruction of Plesiaceratherium gracile. In this skeleton, the head-body length is 2991 mm, the shoulder height is 1600 mm, and the skull length, the vertebrae length, and the limb bones length are the mean of each measure listed in Supplementary Tables 2, 5, 6, respectively.
Body weight based on the mean head-body length (HBL) was calculated as 832.1 kg following the equations for mammals and as 996.6 kg following the equations for all ungulates (Table 6). Using the equations for the black rhinoceroses, the estimation based on the mean HBL is 1,198.1 kg, and that from the largest HBL is 1,362.8 kg. Using Janis’s (1990) equation for perissodactyls and hyracoids, the estimation based on the total skull length (TSL) is 1,130.3 kg. According to the weight record of the extant rhinoceroses, using the equations for all ungulates established by Damuth (1990), the estimated weight based on the molar size is high and even exceeded the heaviest weight records; but, for P. gracile, using Janis’s (1990) equations, the more reasonable molar-based estimations provided a range of 1,092.9–2,244.5 kg.
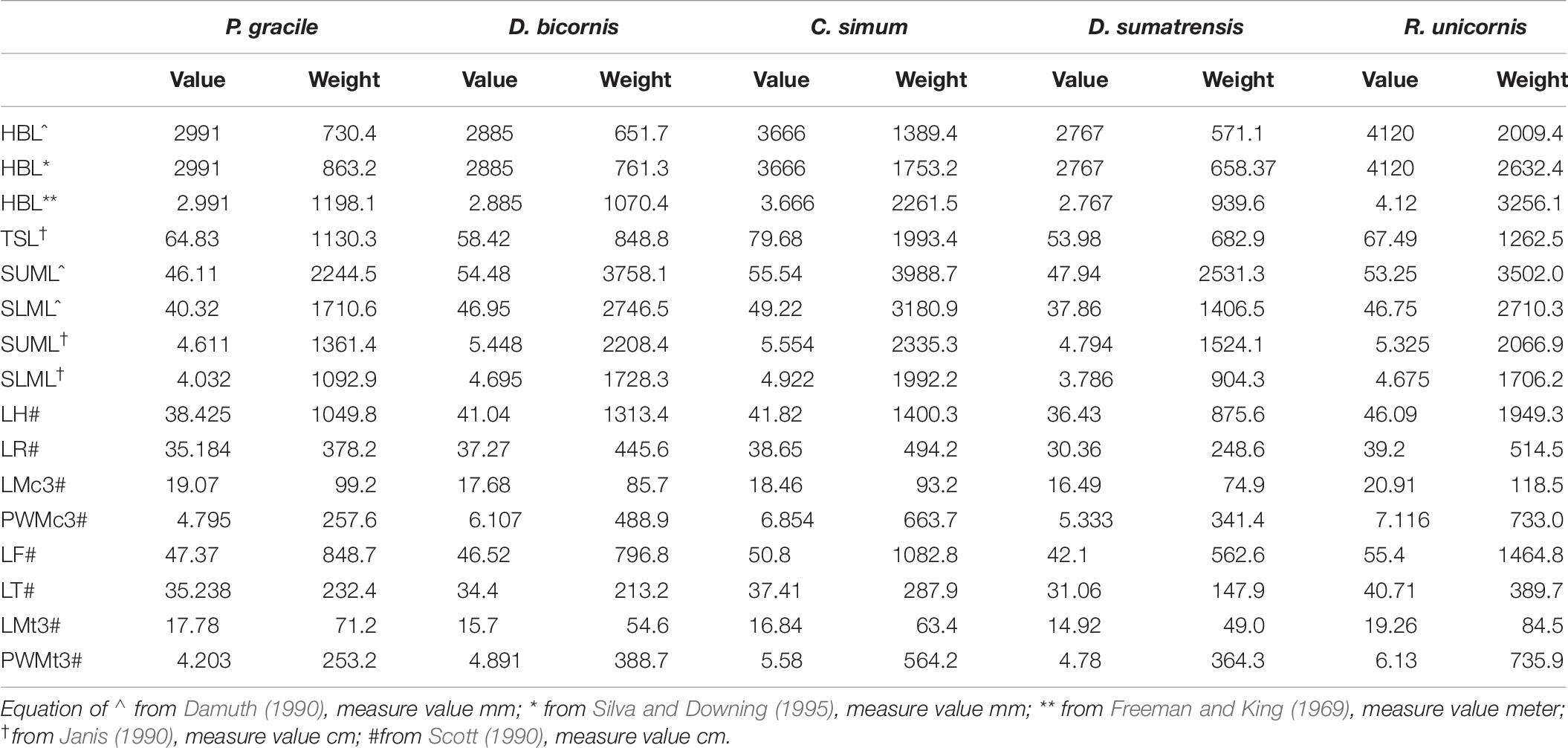
Table 6. Mass estimation of Plesiaceratherium gracile and comparisons with extant rhinos (weight/kg).
Discussion
Sexual Dimorphism and Behavior
Sexual Dimorphism
The size difference of the lower incisor i2 is the strongest sexual dimorphic character in rhinocerotids. The enlarged tusk i2 has occurred since the Eocene rhinocerotids Teletaceras and Trigonias (Prothero and Schoch, 1989) and was specialized to a great level in the Oligocene rhinocerotid Mesaceratherium and Aprotodon (Beliajeva, 1954; Borissiak, 1954; Heissig, 1969; Qiu and Xie, 1997; Deng, 2013). The sexual difference of the incisor i2 has been demonstrated in many Neogene rhinocerotids, Menoceras, Aphelops, Alicornops, Chilotherium, and Teleoceras (Osborn, 1898a, b; Cerdeño and Sánchez, 2000; Mead, 2000; Mihlbachler, 2005, 2007; Chen et al., 2010). The measures of P. gracile demonstrated that not only the tusk i2 but also the upper incisor I1 is sexually different and almost does not involve size overlapping between both genders (Table 1). This has not yet been noted in other rhinoceroses.
In our analysis, measures of the skull and limbs of P. gracile show a clear possibility that the females have a longer skull and body than do the males, which have a more robust skull and body. However, the M/F ratio of such measures is much lower than that of the incisors; more importantly, measures between the two genders are greatly overlapping. Mead (2000) listed many significant sexual dimorphisms in limbs of Teleoceras major, especially the cross-section of the radius (dr = 1.34) and the femur (dr = 1.19), with widely separated ranges. Chen et al. (2010) also suggested that the male specimens are larger than the female in mean values of the width of the mastoid process on the occipital area, and both genders have a nearly totally separated range. Additionally, Chen et al. (2010) revealed that the width of the mandibular symphysis that related with the size of i2 is sexually different, with moderately overlapping ranges. However, studies on the extant rhinoceroses show a wide overlap between genders on relevant skull measures, such as the occipital-nasal length or the nasal width in C. simum, R. unicornis, and D. sumatrensis (Heller, 1913; Guérin, 1980; Groves, 1982). Owen-Smith (1988) determined the gender of the African white rhinoceros mainly based on the size difference of the horn, and then postulated the sexual difference of the body size.
It remains controversial whether a size difference between two genders extensively exists in rhinoceroses. The most evident sexual dimorphism in rhinoceroses is reflected in the horns, incisors, or features directly related to them, such as the nasal and symphysis widths. The sexual dimorphism should be easily identified independently and also be as unambiguous as possible. In P. gracile, our study reveals that sexual dimorphism is easily recognized from the size of the incisors (i2 and I1) but not from the size of the skull and limb bones.
Social Behavior
Trivers (1972) suggested that the stronger the dimorphism in a species, the more likely it is to be polygynous. The extant R. unicornis, D. sumatrensis, and C. simum have a great degree of sexual dimorphism in the horn or incisor size and are polygynous (Pocock, 1946; Owen-Smith, 1988; Dinerstein, 1991; Hillman-Smith and Groves, 1994). Chilotherium wimani and Teleoceras are two advanced rhinoceroses with very large i2, whose dimorphic ratio is 1.73 and 2.02, respectively (Mead, 2000; Chen et al., 2010). In P. gracile, the lengths of the lower incisor i2 do not overlap between the two genders. Such a difference is not weaker than that of Ch. wimani and Teleoceras, indicative of the presence of polygyny.
From the behavioral and ecological perspective, Cerdeño (1998) established three anatomic types of rhinoceroses: small and cursorial, medium-large size with intermediate gracility, and large and strongly brachypodial. The gregarious lifestyle of the small and cursorial species has an advantage in protecting them from predators and ensuring the continuation of the species (Cerdeño and Nieto, 1995). Size increase enabled them to defend themselves against predators and occasionally constitute small groups. The extant rhinos have a large-sized body, and they are all solitary or form “small groups” of mother-offspring units (Groves, 1972; Groves and Kurt, 1972; Laurie et al., 1983; Hillman-Smith and Groves, 1994). P. gracile belongs to the second type, having the advantage of living a solitary life.
However, the large number of P. gracile specimens from the Early Miocene Shanwang Basin could be interpreted as a consequence of their gregarious social behavior. In this basin, the badly crushed fragments of P. gracile from the sandstone bed are rare, but the well-preserved skeletons from the diatomaceous bed are numerous (Yan et al., 1983; Deng et al., 2003). In fact, the extensive amount of rhinoceros material could not be due to an abnormal natural catastrophic event; the alternative possibility is that they drowned in the lake progressively through time. On the other hand, the specimens from the sandstone beds indeed were deposited through a normal burial process, but this is rare in the Shanwang Formation of the Shanwang Basin, indicative of a small number of P. gracile living around the deposition area. After all, mass mortality resulting from an abnormal natural catastrophe would generate more specimens from the sandstone beds than from the diatomaceous, as is the case of the large number of Chilotherium specimens from the Linxia Basin in western China, where an extensive drought occurred during the Late Miocene and Chilotherium remains formed many lentoid fossil concentrations (Liang and Deng, 2005). It is clear that the number of skeletons of P. gracile from the Shanwang Basin has nothing to do with a gregarious way of life.
Postcranial Bone Analysis
Locomotion Adaptation
Slijper (1946) suggested that the spinous process of the T.V. and L.V. inclines to a different direction in mammals that have a different running type, including the leap gallop and the horse gallop. In equids, in order to perform the “horse gallop,” the longissimus dorsi is very developed, and the spinous processes of the posterior 4–5 T.V. and the L.V. incline forward to forming the most efficient angle with the longissimus dorsi (Sisson, 1953). In the skeleton reconstruction of the primitive rhinoceroses Trigonias and Juxia, the posterior 4–5 T.V. and the L.V. are vertical, still having the ability of “horse gallop” (Scott, 1941; Qiu and Wang, 2007). In the extant R. unicornis (IVPP OV 1383) and C. simum, the spinous processes of the posterior T.V. and most of the L.V. are vertical or inclined posteriorly, and the running efficiency of the longissimus dorsi is declined, indicative of probably weakened running ability (Slijper, 1946; Groves, 1972). In the skeleton of P. gracile, the spinous processes of all T.V. are inclined anteriorly, or only the last two T.V. and the L.V. have a vertical spinous process (Figure 4). Thus, we conclude that P. gracile would have a horse gallop locomotion style and a running ability that is close to that of Trigonias and Juxia.
Gregory (1912) divided mammals into four types based on locomotion: cursorial, subcursorial, mediportal, and graviportal, using the limb segments ratio to estimate the locomotion type, namely the metatarso-femoral ratio and tibio-femoral ratio. He considered the four extant rhinoceroses as mediportal and Teleoceras as graviportal. According to this hypothesis, P. gracile would be mediportal, with values of 0.37 and 0.49 for the respective ratios, close to those of four extant rhinoceroses, but higher than those of Teleoceras proterum, which has a massive body and graviportal limb (Supplementary Table 7).
The bent of the limb joint in horses or other cursorial mammals is correlated with a bounding gallop or trotting gait (Gregory, 1912). Graviportal ungulates have evolved a vertical shaft to transmit the body weight and enlarge the stride (Gregory, 1912). In the graviportal rhinoceros Teleoceras from the late Miocene, the first and second angles of the fore-limb was found to be 139° and 160°, respectively, which supports this hypothesis (Osborn, 1898a; Gregory, 1912). In the mediportal large-sized rhinoceros C. simum, the first and the second angles of the fore-limb was 123° and 135°, respectively (Groves, 1972). P. gracile is close to the extant C. simum in terms of the joint angle of the long bones, being a mediportal type.
Limb Length
The Early Miocene P. gracile has a simple occlusal pattern of upper cheek teeth, moderately enlarged incisors, and long and narrow metapodials; it is less specialized than the Late Miocene species of Chilotherium and Teleoceras (Young, 1937; Yan, 1983; Yan and Heissig, 1986). To investigate the condition of the limb segments in P. gracile, we compared the measures and the proportions of the limb bones with the primitive rhinoceros Trigonias osborni from the Paleogene, the massive Teleoceras major and T. proterum from the Late Miocene, and the four extant rhinoceroses. The result reveals an obvious tendency of size increase from Tr. osborni to R. unicornis in the length of the limb, and each limb segment contributes to this elongation. The proximal long bones of the fore-limb show a similar increasing tendency during the progress of the body size increase among these rhinoceroses (Figure 5). However, the proportion of each limb segment relative to the limb length is different, and P. gracile and D. sumatrensis show an increased proportion of the metacarpus when compared with other rhinoceroses (Figure 6). The limb segments of the hind-limb have a pattern of elongation distinct from the fore-limb. The elongation of the metatarsals of P. gracile is proportionally similar to that of the femur with increasing size. However, relative to Tr. osborni, all other rhinoceroses have a proportional increase of the femur but a decrease of the metatarsals (Figures 5, 6).
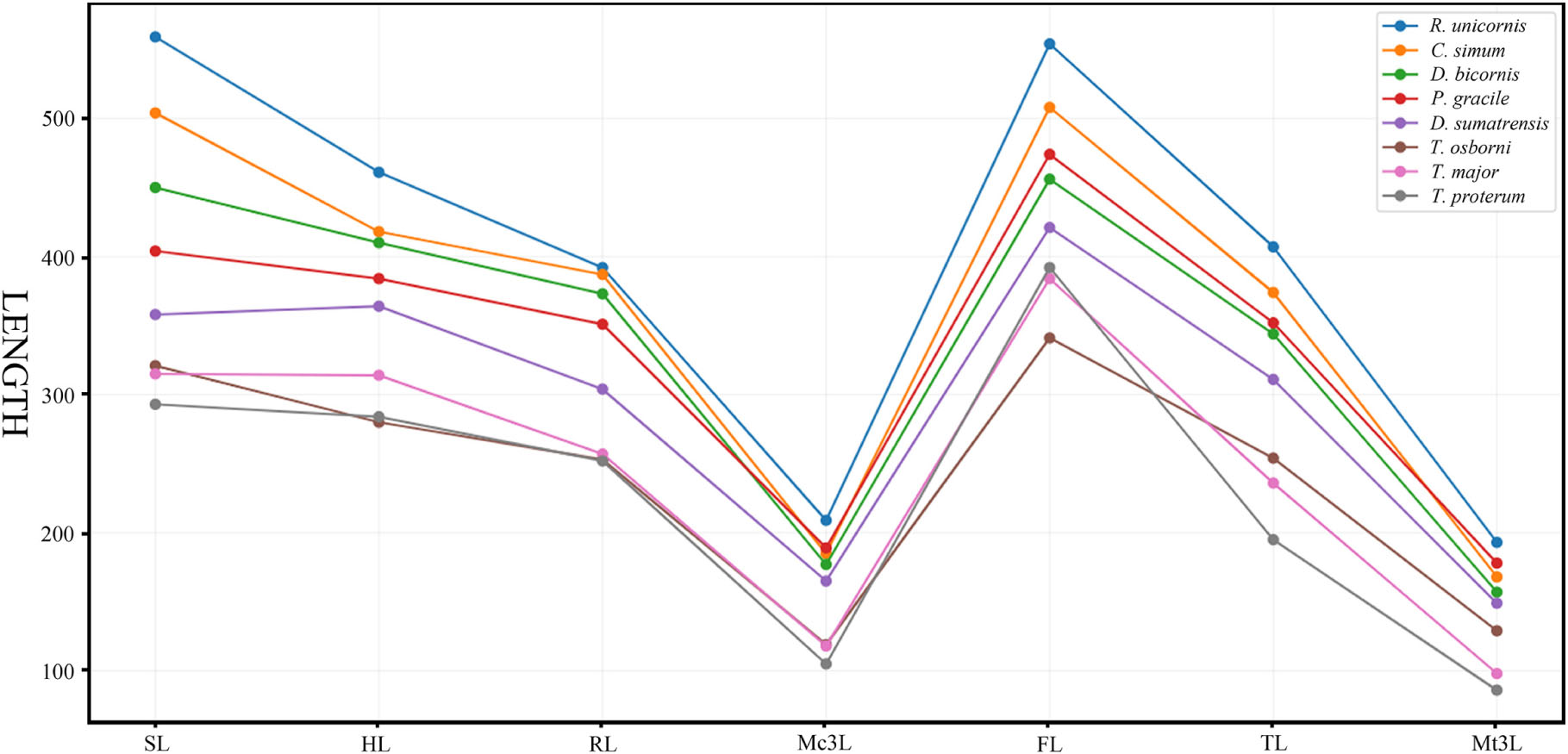
Figure 5. Comparative length of the limb bones in Plesiaceratherium gracile and other rhinos. Original data in Supplementary Table 7. See abbreviations in text. Data for three extant rhinos from Guérin (1980); data for Trigonias osborni and Teleoceras proterum from Prothero (2005).
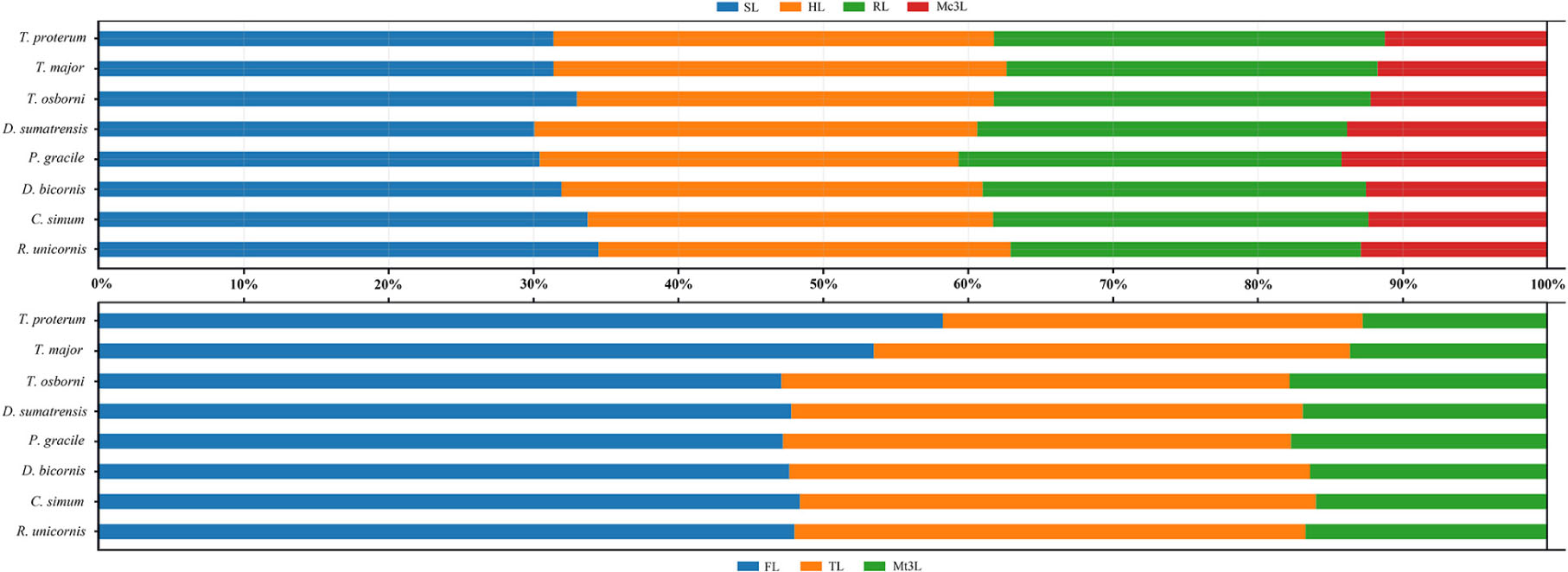
Figure 6. Comparative limb bone proportion in Plesiaceratherium gracile and other rhinos. Original data in the Supplementary Table 7. See abbreviations in text.
The analyses of T. major and T. proterum confirm that the shortening of both the proximal and distal parts of the limb contributes to the decreasing of the body size, particularly the metatarsus. With further shrinking of the body size in T. proterum relative to T. major, the tibia has a more evident decrease of the length than does the radius, consistent with the analysis of relevant limb-segments in terms of the sexual difference of the limb bones in Teleoceras by Mead (2000). However, the femur of T. proterum is not shortened and has a proportional increase relative to other limb bones. The significance of the distal limb parts in supporting body weight is further documented by the large and heaviest extant rhinoceros C. simum, in which both the metacarpus and the metatarsus are proportionally smaller than other large- or medium-sized rhinoceroses listed in Figures 5, 6.
Conclusion
The mensural intraspecific variations in P. gracile are wide ranging. We recognize the size of i2 and I1 as significant sexual dimorphism of P. gracile. With respect to other features, the female skull and limb have a larger mean value than those of the male, but the female has a relatively narrower proximal width of the long bones. Meanwhile, the mean length of P2 and M1 of the male is larger than that of the female. However, all these measures show a high degree of overlap; therefore, they do not individually contribute to determining the gender.
Concerning body size, many well-preserved skeletons of P. gracile reveal that its head-body length varies between 2,796 and 3,117 mm, and the shoulder height between 1,476 and 1,627 mm. The mass estimation suggests a body weight of about 1,198 kg, with the highest value being 2,244 kg. The bend of the limb and spinal column indicates a mediportal limb, and the running type would be horse gallop. Based on the length of the limb bones among rhinoceroses with different sizes and degrees of evolution, we conclude that the proximal long bones, including the scapula, the humerus, and the femur, are important for a longer limb, and the distal part, namely the metapodials, is important for bearing the body mass. P. gracile has a relatively large size, which, together with the strong dimorphic incisors, allows the inference of polygynous behavior and a solitary lifestyle for this rhinocerotid species.
Data Availability Statement
All datasets presented in this study are included in the article/Supplementary Material.
Author Contributions
All authors listed have made a substantial, direct and intellectual contribution to the work, and approved it for publication.
Funding
This research was supported by the Key Laboratory of Vertebrate Evolution and Human Origins of Chinese Academy of Sciences (Institute of Vertebrate Paleontology and Paleoanthropology, CAS) (LVEHO19003), State Key Laboratory of Palaeobiology and Stratigraphy (Nanjing Institute of Geology and Palaeontology, CAS) (No. 203113), and Strategic Priority Cultivating Research Program, Chinese Academy of Sciences (XDB26000000, XDA20070203, QYZDY-SSW-DQC022, and GJHZ1885).
Conflict of Interest
The authors declare that the research was conducted in the absence of any commercial or financial relationships that could be construed as a potential conflict of interest.
Acknowledgments
We greatly appreciate the valuable help we have received from Dr. Wang X.L. of Linyi University and Dr. Wang S.Q. of the Institute of Vertebrate Paleontology and Paleoanthropology (IVPP), Chinese Academy of Sciences (CAS). The photograph used in this paper has been processed by Mr. Y. Xu and Mr. Y. Chen of IVPP, CAS. We are grateful to the editor Dr. Luca Pandolfi, reviewer Dr. Esperanza Cerdeño, and reviewer for their valuable comments on the manuscript. Special gratitude must be extended to the staff of the Shandong Tianyu Museum of Natural History and that of the Shanwang National Geopark of China, for assistance during field work.
Supplementary Material
The Supplementary Material for this article can be found online at: https://www.frontiersin.org/articles/10.3389/fevo.2020.544076/full#supplementary-material
References
Anderson, J. F., Hall-Martin, A., and Russell, D. A. (1985). Long-bone circumference and weight in mammals, birds and dinosaurs. J. Zool. 207, 53–61. doi: 10.1111/j.1469-7998.1985.tb04915.x
Beliajeva, E. I. (1954). New material of Tertiary Rhinocerotids (in Russian). Trans. Paleontol. Inst. Acad. Sci. USSR 47, 24–54.
Borissiak, P. M. (1954). The oldest Aceratherium from Kazahstan. Trans. Paleontol. Inst. Acad. Sci. USSR 47, 5–23.
Borsuk-Bialynicka, M. (1973). Studies on the Pleistocene rhinoceros Coelodonta antiquitatis (Blumenbach). Palaeontol. Polo. 29:148.
Cappellini, E., Welker, F., Pandolfi, L., Lyon, D., Chan, Y. L., Rook, L., et al. (2019). Early pleistocene enamel proteome from Dmanisi resolves Stephanorhinus phylogeny. Nature 574, 103–107. doi: 10.1038/s41586-019-1555-y
Cerdeño, E. (1998). Diversity and evolutionary trends of the family Rhinocerotidae (Perissodactyla). Palaeogeogr. Palaeoclimatol. Palaeoecol. 141, 13–34. doi: 10.1016/s0031-0182(98)00003-0
Cerdeño, E., and Nieto, M. (1995). Changes on Western European Rhinocerotidae related to climatic variations. Palaeogeogr. Palaeoclimatol. Palaeoecol. 114, 325–338. doi: 10.1016/0031-0182(94)00085-m
Cerdeño, E., and Sánchez, B. (2000). Intraspecific variation and evolutionary trends of Alicornops simorrense (Rhinocerotidae) in Spain. Zool. Scripta. 29, 275–305.
Chen, S. K., Deng, T., Hou, S. K., Shi, Q. Q., and Pang, L. B. (2010). Sexual dimorphism in perissodactyl rhinocerotid Chilotherium wimani from the late Miocene of the Linxia Basin (Gansu. China). Acta Palaeontol. Polo. 55, 587–597. doi: 10.4202/app.2009.0001
Christiansen, P. (2002). Locomotion in terrestrial mammals: the influence of body mass, limb length and bone proportions on speed. Zool. J. Linn. Soc. 136, 685–714. doi: 10.1046/j.1096-3642.2002.00041.x
Damuth, J. (1990). “Problems in estimating body masses of archaic ungulates using dental measurements,” in Body Size in Mammalian Paleobiology: Estimation and Biological Implications, eds J. Damuth and B. J. MacFadden (Cambridge: Cambridge University Press), 229–253.
Damuth, J., and MacFadden, B. J. (1990). Body Size in Mammalian Paleobiology: Estimation and Biological Implications. New York, NY: Cambridge University Press.
Deng, T. (2001). “Cranial ontogenesis of Chilotherium wimani (Perissodactyla, Rhinocerotidae),” in Proceeding of the 8th Annual Meeting of the Chinese Society of Vertebrate Paleontology, eds T. Deng and Y. Wang (Beijing: China Ocean Press), 101–112.
Deng, T. (2005). New discovery of Iranotherium morgani (Perissodactyla, Rhinocerotidae) from the late Miocene of the Linxia Basin in Gansu, China, and its sexual dimorphism. J. Vert. Paleontol. 25, 442–450. doi: 10.1671/0272-4634(2005)025[0442:ndoimp]2.0.co;2
Deng, T. (2013). Incisor fossils of Aprotodon (Perissodactyla, Rhinocerotidae) from the early miocene shangzhuang formation of the Linxia Basin in Gansu, China. Vert. PalAsiat. 51, 131–140.
Deng, T., Wang, W. M., and Yue, L. P. (2003). Recent advances of the establishment of the Shanwang Stage in the Chinese Neogene. Vert. PalAsiat. 41, 314–323.
Dinerstein, E. (1991). Sexual dimorphism in the greater one-horned rhinoceros (Rhinoceros unicornis). J. Mammal. 72, 450–457. doi: 10.2307/1382127
Fortelius, M., Eronen, J., Liu, L., Pushkina, D., Tesakov, A., Vislobokova, I., et al. (2006). Late Miocene and Pliocene large land mammals and climatic changes in Eurasia. Palaeogeogr. Palaeoclimatol. Palaeoecol. 238, 219–227. doi: 10.1016/j.palaeo.2006.03.042
Freeman, G. H., and King, J. M. (1969). Relations amongst various linear measurements and weight for black rhinoceroses in Kenya. East Afr. Wildl. J. 7, 67–72. doi: 10.1111/j.1365-2028.1969.tb01194.x
Goddard, J. (1970). Age criteria and vital statistics of a black rhinoceros population. East Afr. J. Wild. J. 8, 105–121. doi: 10.1111/j.1365-2028.1970.tb00834.x
Gregory, W. K. (1912). Notes on the principles of quadrupedal locomotion and on the mechanism of the limbs in hoofed animals. Ann. N. Y. Acad. Sci. 22, 267–294. doi: 10.1111/j.1749-6632.1912.tb55164.x
Groves, C. P., and Kurt, F. (1972). Dicerorhinus sumatrensis. Mamm. Spec. 21, 1–6. doi: 10.2307/3503818
Groves, P. (1982). The skulls of Asian rhinoceroses: wild and captive. Zoo Biol. 1, 251–261. doi: 10.1002/zoo.1430010309
Guérin, C. (1980). Les rhinocéros (Mammalia, Perissodactyla) du miocène terminal au Pléistocène supérieur en Europe occidentale: comparaison avec les espèces actuelles. Doc. Lab. Géol. Lyon 79:1182.
Heissig, K. (1969). Die Rhinocerotidae (Mammalia) aus der oberligozänen spaltenfüllung von gaimersheim bei Ingolstadt in Bayern und ihre phylogenetische Stellung. Abh. Bayer. Akad. Wiss. Math Naturwiss 138:133.
Heissig, K. (1989). “Rhinocerotidae,” in The Evolution of Perissodactyls, eds D. R. Prothero and R. M. Schoch (New York: Oxford University Press), 399–417.
Hillman-Smith, A. K. K., Owen-Smith, N., Anderson, J. L., Hall-Martin, A. J., and Selaladi, J. P. (1986). Age estimation of the White rhinoceros (Ceratotherium simum). J. Zool. Lond. 210, 355–379. doi: 10.1111/j.1469-7998.1986.tb03639.x
Hillman-Smith, K., and Groves, C. P. (1994). Diceros bicornis. Mamm. Spec. 455, 1–8. doi: 10.2307/3504292
Hitchins, P. M. (1978). Age determination of the black rhinoceros (Diceros bicornis Linn.) in Zululand. South Afr. J. Wild. Res. 8, 71–80. doi: 10.1638/1042-7260(2000)031[0071:alliaj]2.0.co;2
Janis, C. M. (1990). “Correlation of cranial and dental variables with body size in ungulates and macropodoids,” in Body Size in Mammalian Paleobiology: Estimation and Biological Implications, eds J. Damuth and B. J. MacFadden (New York: Cambridge University Press), 255–299.
Legendre, S. (1986). Analysis of mammalian communities from the Late Eocene and Oligocene of southern France. Palaeovert 16, 121–191.
Liang, Z., and Deng, T. (2005). Age structure and habitat of the rhinoceros Chilotherium during the Late Miocene in the Linxia Basin, Gansu, China. Vert. PalAsia 43, 219–230.
Loose, H. (1975). Pleistocene Rhinocerotidae of W. Europe with reference to the recent two-horned species of Africa and S.E. Asia. Scripta Geol. 33, 1–59.
Lu, X. K., Zheng, X. T., Sullivan, C., and Tan, J. (2016). A skull of Plesiaceratherium gracile (Rhinocerotidae, Perissodactyla) from a new lower Miocene locality in Shandong Province, China, and the phylogenetic position of Plesiaceratherium. J. Vert. Paleontol. 36:e1095201. doi: 10.1080/02724634.2016.1095201
MacFadden, B. J., and Hulbert, R. C. (1990). “Body size estimates and size distribution of ungulate mammals from the Late Miocene Love Bone Bed of Florida”, in Body Size in Mammalian Paleobiology: Estimation and Biological Implications, eds J. Damuth and B. J. MacFadden (New York: Cambridge University Press), 337–363.
Mead, A. J. (2000). Sexual dimorphism and paleoecology in Teleoceras, a North American rhinoceros. Paleobiology 26, 689–706. doi: 10.1666/0094-8373(2000)026<0689:sdapit>2.0.co;2
Mihlbachler, M. C. (2005). Linking sexual dimorphism and sociality in rhinoceroses: insights from Teleoceras proterum and Aphelops malacorhinus from the late Miocene of Florida. Bull. Flo. Mus. Nat. Hist. 45, 495–520.
Mihlbachler, M. C. (2007). Sexual dimorphism and mortality bias in a small Miocene North American rhino, Menoceras arikarense: Insights into the coevolution of sexual dimorphism and sociality in rhinos. J. Mammal. Evol. 14, 217–238. doi: 10.1007/s10914-007-9048-4
Osborn, H. F. (1898a). A complete skeleton of Teleoceras fossiger. Notes upon the growth and sexual characters of this species. Mem. Am. Mus. Nat. Hist. 10, 51–59.
Owen-Smith, R. N. (1988). Megaherbivores: the Influence of Very Large Body Size on Ecology. New York, NY: Cambridge University Press.
Pocock, R. I. (1945). A sexual difference in the skulls of Asiatic rhinoceroses. Proc. Zool. Soc. Lond. 115, 319–322. doi: 10.1111/j.1096-3642.1946.tb00095.x
Pocock, R. I. (1946). Some structural variations in the second upper molar of the lesser one-horned rhinoceros (Rhinoceros sondaicus). Proc. Zool. Soc. Lond. 115, 310–311.
Prothero, D. R., and Schoch, R. M. (1989). “Rhinocerotidae,” in The Evolution of Perissodactyls, eds D. R. Prothero and R. M. Schoch (New York, NY: Oxford University Press), 530–537.
Qiu, Z. X., and Xie, J. Y. (1997). A new species of Aprotodon (Perissodactyla, Rhinocerotidae) from Lanzhou Basin, Gansu, China. Vert. PalAsiat. 35, 250–267.
Scott, K. M. (1990). “Postcranial dimensions of ungulates as predictors of body mass,” in Body Size in Mammalian Paleobiology: Estimation and Biological Implications, eds J. Damuth and B. J. MacFadden (Cambridge: Cambridge University Press), 301–335.
Scott, W. B. (1941). The mammalian fauna of the White River Oligocene, Part V, Perissodactyla. Tran. Am. Phil. Soc. 28, 747–980.
Silva, M., and Downing, J. A. (1995). The allometric scaling of density and body mass: a nonlinear relationship for terrestrial mammals. Am. Nat. 145, 704–727. doi: 10.1086/285764
Sisson, S. B. (1953). The Anatomy of the Domestic Animals, 4th Edn, Philadelphia: Saunders W. B. Company.
Slijper, E. J. (1946). Comparative biologic-anatomical investigations on the vertebral column and spinal musculature of mammals. Verh. Kon. Neder. Acad. Wetensch. Tweede Sect. 42:128.
Trivers, R. L. (1972). “Parental investment and sexual selection,” in Sexual Selection and the Descent of Man, ed. B. Campbell (Chicago: Aldine Press), 1871–1971.
Voorhies, M. R. (1990). Vertebrate Paleontology of the Proposed Norden Reservoir Area, Brown, Cherry, and Keya Paha Counties, Nebraska. Lincoln: Division of Archeological Research, University Nebraska.
Yan, D. F. (1983). Über die klassifikation und morphologie des schädel von Plesiaceratherium. Vert. PalAsiat. 21, 134–143.
Yan, D. F., and Heissig, K. (1986). Revision and autopodial morphology of the Chinese-European rhinocerotid genus Plesiaceratherium Young, 1937. Zitteliana 14, 81–110.
Yan, D. F., Qiu, Z. D., and Meng, Z. Y. (1983). Miocene stratigraphy and mammals of Shangwang. Shandong. Vert. PalAsiat. 21, 210–222.
Keywords: Plesiaceratherium, Early Miocene, Shanwang Basin, Rhinocerotidae, Aceratheriinae, body size, sexual diamorphism
Citation: Lu X, Deng T, Zheng X and Li F (2020) Sexual Dimorphism and Body Reconstruction of a Hornless Rhinocerotid, Plesiaceratherium gracile, From the Early Miocene of the Shanwang Basin, Shandong, China. Front. Ecol. Evol. 8:544076. doi: 10.3389/fevo.2020.544076
Received: 17 April 2020; Accepted: 26 August 2020;
Published: 23 September 2020.
Edited by:
Luca Pandolfi, University of Florence, ItalyCopyright © 2020 Lu, Deng, Zheng and Li. This is an open-access article distributed under the terms of the Creative Commons Attribution License (CC BY). The use, distribution or reproduction in other forums is permitted, provided the original author(s) and the copyright owner(s) are credited and that the original publication in this journal is cited, in accordance with accepted academic practice. No use, distribution or reproduction is permitted which does not comply with these terms.
*Correspondence: Tao Deng, dengtao@ivpp.ac.cn