How Can Termites Achieve Their Unparalleled Postembryonic Developmental Plasticity? A Test for the Role of Intermolt-Specific High Juvenile Hormone Titers
- 1Behavioural Biology, University of Osnabrück, Osnabrück, Germany
- 2Evolutionary Biology and Ecology, University of Freiburg, Freiburg, Germany
Termites are “social cockroaches” and amongst the most phenotypically plastic insects. The different castes (i.e., two types of reproductives, workers, and soldiers) within termite societies are all encoded by a single genome and present the result of differential postembryonic development. Besides the default progressive development into winged sexuals of solitary hemimetabolous insects, termites have two postembryonic, non-terminal molts (stationary and regressive; i.e., molts associated, respectively, with no change or reduction of size/morphological differentiation) which allow them to retain workers, and two terminal developmental types to become soldiers and replacement reproductives. Despite this unique plasticity, especially the mechanisms underlying the non-terminal development are poorly understood. In 1982, Nijhout and Wheeler proposed a model how this diversity might have evolved. They proposed that varying juvenile hormone (JH) titers at the start, mid-phase, and end of each intermolt period account for the developmental diversity. We tested this rarely addressed model in the lower termite Cryptotermes secundus using phase-specific pharmacological manipulations of JH titers. Our results partially support the Nijhout and Wheeler model. These data are supplemented with gene expression studies of JH-related genes that characterize different postembryonic developmental trajectories. Our study provides new insights into the evolution of the unique postembryonic developmental plasticity of termites that constitutes the foundation of their social life.
Introduction
Termites (Infraorder: Isoptera) are unparalleled when it comes to developmental plasticity in insects which is the basis of their caste system (Noirot, 1990; Roisin, 2000; Korb and Hartfelder, 2008; Roisin and Korb, 2011; Korb and Thorne, 2017). Like in all eusocial insects, their colonies are characterized by overlapping generations and a reproducing caste, the queens (and in termites, also kings), and non-reproducing workers that generally perform tasks like brood care and foraging (Figure 1). Due to their different ancestry, termites as “social cockroaches” (Inward et al., 2007) have a hemimetabolous development, while eusocial Hymenoptera (ants, bees, and wasps) belong to the holometabolous insects. In line, the workers of eusocial Hymenoptera are imaginal stages, while those of termites are immature instars, which can be arrested in their development in some lineages (Noirot, 1990; Roisin, 2000; Korb and Hartfelder, 2008; Roisin and Korb, 2011).
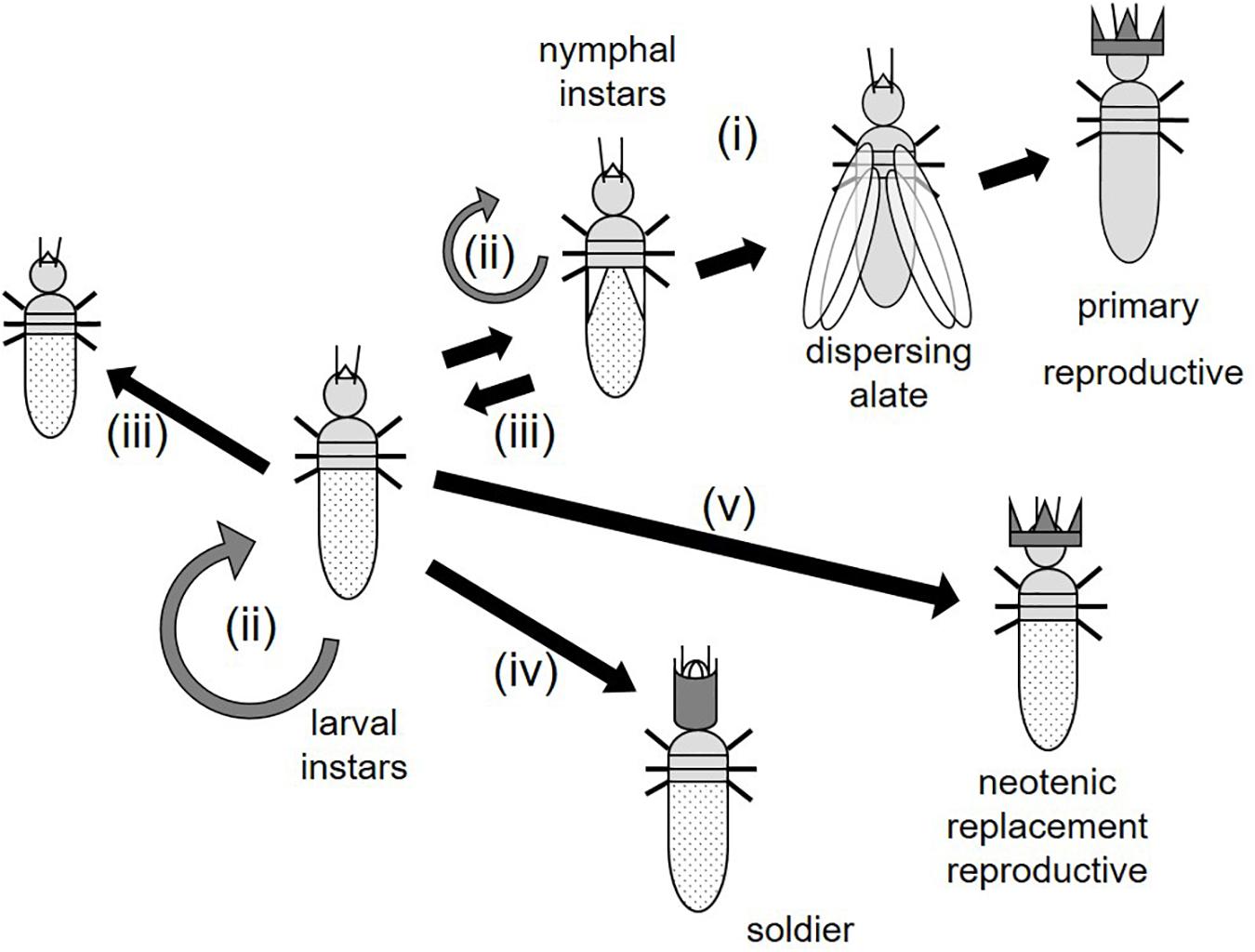
Figure 1. Developmental plasticity of wood-dwelling termites. Immatures (larval and nymphal instars) can (i) develop progressively via several nymphal instars into winged sexuals (alates) that disperse and found a nest as primary reproductives, (ii) remain in the same instar by doing stationary molts (gray circles), (iii) develop regressively into an “earlier” instar, (iv) develop into a soldier, or (v) a neotenic replacement reproductive. For further information, see text. Adapted from Korb et al. (2012).
The highest developmental plasticity is observed in the two lower termite families Archotermopsidae (dampwood termites) and Kalotermitidae (drywood termites) as all eggs hatching within a colony appear totipotent to perform all molting types (Roisin, 2000; Roisin and Korb, 2011). These wood-dwelling termites (one-piece nesting, sensu Abe, 1987) nest in a single piece of wood that serves as food and shelter and which workers never leave to forage outside. Here the functional workers (ontogenetically also defined as “pseudergates sensu lato”; for simplicity, we will refer to them as “workers” in the following) are totipotent immatures that can develop into three terminal castes (Noirot and Pasteels, 1987; Noirot, 1990; Roisin, 2000; Korb and Hartfelder, 2008; Roisin and Korb, 2011; Figure 1). First, they can develop via one to several nymphal instars (instars with wingbuds) into winged sexuals that disperse and found a new colony. Second, they can become sterile soldiers via a presoldier instar. Third, they can develop with a single (sometimes two) molt/s into neotenic (replacement) reproductives that reproduce within the natal nest, especially when the current reproductives die or are unhealthy. The soldiers and neotenic reproductives are synapomorphies which distinguish termites from their subsocial sister taxon, the Cryptocercus woodroaches (Noirot and Pasteels, 1987; Noirot, 1990; Roisin, 2000; Korb and Hartfelder, 2008). This “progressive” development that finally results in terminal castes is complemented by two additional molting types that “allow” immatures staying as workers in the nest (Figure 1): (i) stationary molts during which individuals molt without any change of size or morphology, and (ii) regressive molts which are associated with reduction of size and/or developmental traits (especially wing buds). While stationary molts also occur in “solitary” cockroaches (Bell et al., 2007), regressive molts are unique to termites. This makes termites examples of unparalleled postembryonic developmental diversity. Developmental details (e.g., in the number of nymphal instars) can differ between species (Roisin and Korb, 2011). Development is regulated by hormones. In insects there are two major morphogenic hormones known to control development: juvenile hormone (JH) and ecdysone (e.g., Nijhout, 1994; Gilbert, 2012).
How can interactions between just two morphogenic hormones produce such diversity in molting types? Like in most insects, JHIII is the only JH moiety produced by termite corpora allata and detected in hemolymph (Lanzrein et al., 1985; Park and Raina, 2004; Brent et al., 2005; Yagi et al., 2005; Cornette et al., 2008). Based on seminal termite research in the 1950’s and 60’s (summarized in Lüscher, 1974b; Lenz, 1976), Nijhout and Wheeler (1982) developed a model (NW-model hereafter) to explain the developmental diversity in termites. They proposed three JH sensitive phases during an intermolt period (i.e., the time between two consecutive molts) which determine the subsequent molting type of a “lower termite” worker (Figure 2). These three phases occur at the start (phase 1), during mid-phase (phase 2), and toward the end (phase 3) of an intermolt period and they control, respectively, sexual reproductive traits, non-sexual adult traits (e.g., eyes and wings), and soldier traits (for more details see Figure 2). According to NW-model, a worker that develops progressively into a nymphal instar, and finally into a winged imago, is proposed to have low JH titers throughout all three sensitive phases (Figure 2). Continuously high JH titers characterize presoldier-soldier differentiation (Figure 2). Development of neotenic replacement reproductives requires low JH titers at the start of an intermolt period that increase later. As neotenic molts have shortened intermolt periods (Springhetti, 1972; Lüscher, 1974b; Hoffmann and Korb, 2011), they molt before the third sensitive phase (Figure 2). Finally, the reverse pattern with first high, then low JH titers is suggested to characterize individuals that remain immature workers. This last proposition does not distinguish between stationary and regressive molts. In addition, Lüscher (1956) and Springhetti (1969) suggested that regressive molts are associated with (constantly) high JH titers and that high JH titers during nymphal stages prevent development of termite workers into sexuals.
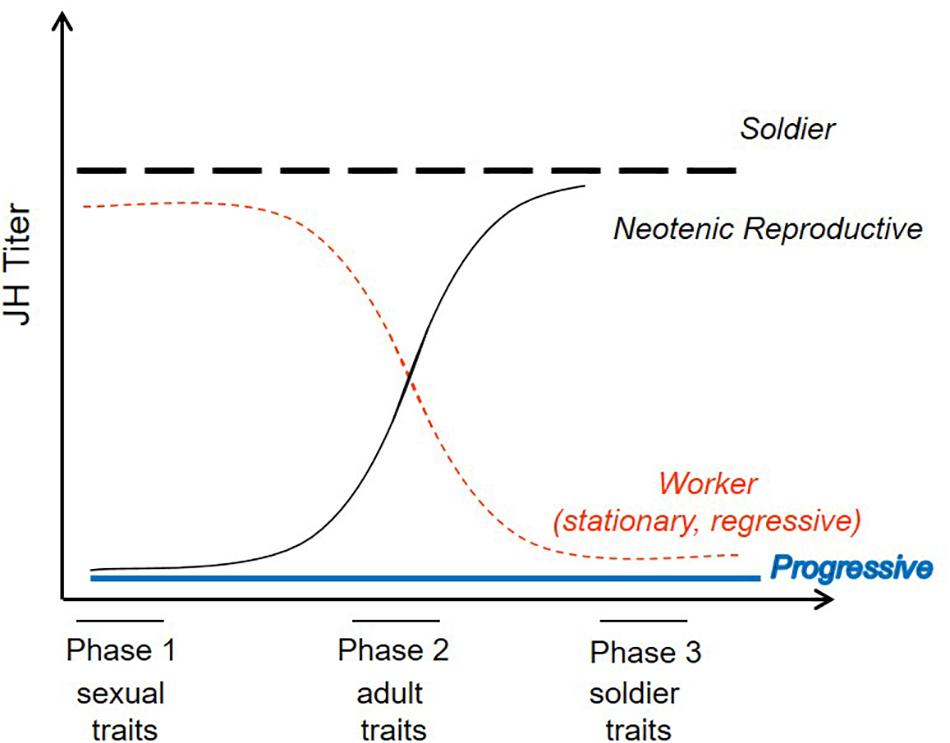
Figure 2. Model proposed by Nijhout and Wheeler (1982) to explain the endocrine regulation of molting types in termites. JH titers during three sensitive periods (phases) between two consecutive molts determine the subsequent molting type of a termite worker. Continuously high or low titers result, respectively, in presoldier/soldier differentiation or progressive development toward winged imagoes. Titers that vary during the intermolt period account for neotenic and continued worker development. For further information, see text. Adapted from Nijhout and Wheeler (1982).
Except for the terminal molts into soldiers (see recent reviews: Miura and Scharf, 2011; Watanabe et al., 2014; Korb, 2015) and partly neotenic reproductives (Elliott and Stay, 2008; reviewed in: Korb, 2015), the NW-model remains largely untested. Very few studies addressed differences between progressive, stationary and regressive postembryonic development; these studies provide some evidence that progressive development is associated low JH titers (Lüscher, 1974b; Cornette et al., 2008; Korb et al., 2009, Korb et al., 2012). We systematically manipulated JH titers during all three phases of an intermolt period in nymphal instars of the wood-dwelling, drywood termite Cryptotermes secundus Hill (Kalotermitidae) to determine the influence of JH on development. These data are supplemented with gene expression studies of JH-related genes that are supposed to be associated with postembryonic developmental trajectories.
C. secundus is well suited to do these experiments. The developmental trajectories of the totipotent workers, comprising older larval, and nymphal instars, are well known (Korb and Katrantzis, 2004; Korb et al., 2009, Korb et al., 2012). C. secundus has three worker larval and five nymphal instars and except for the 4th, penultimate nymphal instar the intermolt period lasts about 2 months (Korb and Katrantzis, 2004; Korb et al., 2009, Korb et al., 2012). All instars can develop progressively and stationary (including nymphs); regressive development is possible for all instars, except the first and second larval instar. All instars that can develop regressively, can also develop into soldiers and neotenic replacement reproductives (Korb and Katrantzis, 2004; Hoffmann and Korb, 2011). Progressive development from 1st to 5th nymphal instar is associated with decreasing hemolymph JH titers and regressive molts have been hypothesized to be linked with high JH titers (Korb et al., 2009). However, during an intermolt period JH titers vary considerably (Korb et al., 2012) and it is unclear whether and how different phases account for different molting types.
We tested the importance of JH in determining development by phase-specific manipulations using the JH analogon methoprene. Methoprene is a stable JH analogon that has been used successful in several experiments to induce soldier development in termites, generally in soldierless groups (e.g., Tarver et al., 2012; Maekawa et al., 2014). We did our experiments with complete colonies, including soldiers and reproductives. This is important in order to separate a social effect due to the presence/absence of castes from the endocrine effect of varying JH titers (Watanabe et al., 2014; Korb and Bellés, 2017). This is not possible with dish-assays that consist of workers only.
Materials and Methods
Collection and Maintenance of Termites
Complete Cryptotermes secundus colonies were collected from Ceriops tagal trees in the mangrove area near Darwin (NT, Australia; 12°30′S 131°00′E) as described elsewhere (Korb and Lenz, 2004). Colonies were set up in standardized Pinus radiata wood blocks adjusted to colony size, providing abundant resource conditions (1 termite: about 10 cm3 wood; for details see Korb and Lenz, 2004). Colonies were transferred to Germany and kept in climate chambers (12 h day/night cycle, 28°C, 70% relative humidity). Under these conditions, colony development as well as molting types and developmental trajectories of individuals in relocated lab colonies are indistinguishable from relocated colonies kept in the field (Korb and Katrantzis, 2004).
Preparation of Experimental Colonies
In order to follow individual development, wood blocks were split in Germany and their colonies were transferred into a drilled chamber of a new abundant-food wood block, which allowed monitoring of individuals (for details see: Korb and Schmidinger, 2004). To prevent the termites from disappearing into the wood, newly dug tunnels were blocked with soft paper. Wood chips and humid filter paper was provided as additional food and water supply.
All our experiments were done with complete colonies including soldiers and workers. “Artificial” groups consisting of workers only are often used in similar studies. However, such worker groups are less appropriate because they lack important social interactions leading to artificial results. Thus, it is for instance known that the absence of castes influence development of individuals (e.g., Lüscher, 1974b; Lenz, 1976; Korb and Katrantzis, 2004; Maekawa et al., 2012; Watanabe et al., 2014). Our set-up also prevented neotenic and soldier differentiation which is triggered in C. secundus by the absence of the corresponding caste (Korb et al., 2003; Hoffmann and Korb, 2011). All colonies used in this experiment were a maximum of 2 years in the laboratory when the experiments were performed. To avoid seasonal differences all experiments were done between April and August when nymphal development takes place (Korb and Katrantzis, 2004).
For individual identification, workers were arbitrarily selected and marked with an individual color code of enamel paint (Revell, Germany) on the abdomen and/or thorax. Individuals were checked at least four times per week throughout the duration of the experiment to ensure they retained their markings.
Before a molt, individuals become whitish and translucent. These individuals were separated for the length of the molt. Their developmental stage was classified into instars according to wing bud shape and size before and after the molt (for more details see: Korb and Katrantzis, 2004) to identify molting types.
Endocrine Experiment
In order to do phase-specific manipulations we had to follow single individuals over extended periods (generally about 3–5 months). Freshly marked individuals were followed until their next molt which defined the start of the subsequent intermolt period. We performed three sets of experiments that addressed, respectively, phase 1, 2, and 3 of the intermolt period. Accordingly, we treated nymphal instars within the next 2 days after the molt (phase 1), 4 weeks after the molt (phase 2), or before the subsequent molt (indicated by their whitish and translucent appearance, which occurs 3–6 days before the next molt) (phase 3). After treatment all individuals were monitored until their next molt to determine subsequent, experimentally affected molting types.
The manipulations of JH titers consisted in topical application of the JH analogon, methoprene (99%, Sigma-Aldrich, Germany) (2 μl of 1 μg methoprene/10 μl acetone solution) on nymphs. Untreated nymphs served as controls (for a test of potential solvent effects, see next section “Supplementary Experiments and Rational for Set-Ups”). In total, we marked and followed over 1,000 individuals of which we could record the development of 870 individuals (control: 792, 15 colonies; methoprene treated individuals: phase 1: 51 individuals from five colonies; phase 2: 12 individuals from four colonies; phase 3: 15 individuals from to two colonies) from a total of 26 different colonies (see Supplementary Data Set 1). Individuals of the different treatments always originated from different colonies. Despite intensive efforts of monitoring all individuals at least four times a week, we were unable to follow over 50% of the treated individuals until the end of our experiments because they lost their individual identifying marks during the months-long duration of this experiment. As C. secundus has small colonies of generally less than 200 individuals (Korb and Lenz, 2004; Korb and Schneider, 2007) (here between 53 and 215 individuals), we were able to record the development of a considerable fraction of individuals within each colony.
Supplementary Experiments and Rational for Set-Ups
We did several supplementary experiments to test the suitability of methods. First, we compared the development between acetone (solvent) and untreated individuals. It showed that acetone does not seem to affect a nymphs subsequent molting type compared to untreated individuals (chi2 contingency analysis: Nacetone = 44, Nuntreated = 792, χ22,836 = 3.12, P = 0.210). Second, we tested the suitability of methoprene to emulate effects of increased JH titers. We chose a concentration of 2 μl of 1 μg methoprene/10 μl acetone solution per nymph based on former studies (Tarver et al., 2012; Maekawa et al., 2014), taking into account body size differences between species. This separate experiment showed that the mortality rate of methoprene-treated nymphs using these concentrations did not differ significantly from those of control individuals (survival untreated: 72%, survival methoprene: 88%; chi2 contingency analysis: Nmethoprene = 17, Nuntreated = 25, χ21,42 = 1.58, P = 0.208). This experiment covered a period until, and including, the next molt of an individual; most mortality occurred during molting when the animals are separated from the colony. Finally, we tested whether such a methoprene treatment results in an upregulation of JH signaling as increased JH titers do. Note, methoprene is an JH analogon and not JH, hence its titers cannot be determined using methods such as radio-immunoassays that measure JH directly. Therefore, we measured gene expression of genes involved in JH signaling by performing quantitative realtime PCR (qRT-PCR) experiment (see next section “qRT-PCR”). We quantified the expression of Kr-h1 (Krüppel-homolog 1, CsecKr-h1), which is an early response gene upregulated under high JH titers in insects (e.g., Bellés et al., 2005; Bellés and Santos, 2014; Jindra et al., 2015). Additionally, we tested genes supposed to be involved in JH biosynthesis: two JH epoxidases (P450-15A1; CsecJH epoxidase1 and CsecJH epoxidase2) and the supposed two JH receptor genes, CsecMet (Methoprene-tolerant) and CsecTai (Taiman) (for more details on genes and methods, see below) (Harrison et al., 2018; Jongepier et al., 2018). If methoprene treatment functions efficiently, these four JH biosynthesis genes should not be affected by methoprene treatment. As expected, none of the genes associated with JH biosynthesis was affected by our methoprene treatment (Kruskal Wallis tests: always P > 0.305) but CsecKr-h1 was significantly upregulated in methoprene-treated individuals compared to untreated and acetone treated individuals (Kruskal Wallis test: χ22,30 = 7.51, P = 0.023, Dunn-Bonferroni Post hoc tests: Nuntreated = 19, Nacetone = 5, Nmethoprene = 6; untreated vs. methoprene: z = −2.52, P = 0.035; acetone vs. methoprene: z = −2.36, P = 0.055; untreated vs. acetone: z = 0.50, P = 1.00). These results show that our methoprene treatment specifically up-regulated JH signaling and that acetone did not have a similar JH effect.
qRT-PCR
To obtain insights into JH titers and genetic mechanisms underlying postembryonic development, we performed qRT-PCR experiments of selected genes expressed in the head and thorax that are known to be associated with JH signaling in other insects, including cockroaches (e.g., Bellés et al., 2005; Bellés and Santos, 2014; Jindra et al., 2015):
(i) CsecKr-h1, the C. secundus 1:1 ortholog of the early response gene of JH signaling in the fruit fly D. melanogaster Kr-h1;
(ii) two JH epoxidases (P450-15A genes), CsecJH epoxidase1 and CsecJH epoxidase2; these two genes have been identified as an ortholog and a paralog of the D. melanogaster JH epoxidase that synthesize JH (Harrison et al., 2018; Jongepier et al., 2018). As we do not know which of both genes has the same function in C. secundus, we tested both genes.
(iii) CsecMet, a 1:1 ortholog of the gene Methoprene-tolerant (met) of the cockroach Blattella germanica whose protein functions as receptor of JH (Jindra et al., 2015);
(iv) CsecTai, a 1:1 ortholog of the B. germanica gene Taiman (Tai) which encodes a protein that dimerizises with Met upon JH binding (Jindra et al., 2015). The resulting JH-Met-Tai complex then induces transcription of the early response gene Kr-h1 in B. germanica and other insects (Jindra et al., 2015).
qRT-PCR was performed as described elsewhere (Elsner et al., 2018). In short, total RNA of head and thorax was extracted according to the peqGOLD TriFast protocol as described in Elsner et al. (2018). RNA concentration and purity were measured with a Nanodrop 2000c (peqLab) and the RNA was diluted to 25 ng/μl with nuclease-free H2O. Reverse transcription and qRT-PCR were performed with a LightCycler 96 (Roche) using the QuantiTect SYBR Green RT-PCR Kit (Qiagen). We used a reaction volume of 25 μl. After reverse transcription for 1,800 s at 50°C and an initial activation for 900 s at 95°C, 50 amplification cycles were run. These consisted of 15 s of denaturation at 95°C, 30 s of annealing at 62°C, and 70 s of extension at 72°C. This was followed by a melting step with 10 s at 95°C, 60 s at 65°C, and 1 s at 97°C, followed by a cooling step for 30 s at 37°C. We checked the amplification and melting curves to ensure target specificity. We used 18S rRNA to normalize expression, as it was previously established to be stable expressed in termites (Weil et al., 2007). Three technical replicates per gene and sample were done. For primer sequences see Supplementary Table S1.
Using this approach (i) we tested whether methropene results in increased JH signaling (see above), (ii) we inferred the JH titers before and after a molt, and (iii) we associated molting types (progressive, stationary, regressive) with JH related gene expression and JH titers after a molt. Note, it is impossible to determine gene expression before a molt and link it with the potential subsequent molting type as one cannot predict how an individual would have molted if one would not have killed it for gene expression analysis.
For these experiments, we used another set of 17 C. secundus colonies. The experimental procedure was identical to that described in “Endocrine manipulations.” All gene expression data are provided as Supplementary Data Set 2.
Statistical Analyses
Endocrine Experiment
To test whether an increase in JH signaling influenced developmental trajectories of nymphs, we compared the frequency of molting types (i.e., the number how often a molting type occurred) after methoprene treatment with those of control individuals. Colony identity affected molting type frequencies. Hence, we performed generalized mixed models with multinomial error distributions and “treatment” as fixed factor and “colony ID” as random factor to control for colony effects. All models differed significantly compared to a null model (see section “Results”). With post hoc tests we compared changes in frequencies relative to the proportion of regressive molts. To do this, we applied the Satterthwaite approach which is especially useful if the data are unbalanced like in our case where we had more control than treated individuals. All analyses were done separately for each phase to identify which phase is crucial for affecting developmental trajectories.
Gene Expression Studies
For none of the five studied genes, gene expression was affected by colony identity (Kruskal-Wallis tests: always P > 0.100). Hence, we analyzed gene expression data with Mann Whitney-U-tests (before vs. after molt) or Kruskal Wallis-tests (all other analyses) and applied FDR correction for multiple testing.
All statistical analyses were performed using IBM SPSS 26 and all tests were two-tailed.
Results
Effect of Methoprene on Molting Type Frequencies
During the study period, untreated, control nymphs mainly performed stationary molts (360 molts, 45.5%), followed by progressive (280 molts, 35.4%) and regressive molts (152 molts, 19.2%) (Figure 3).
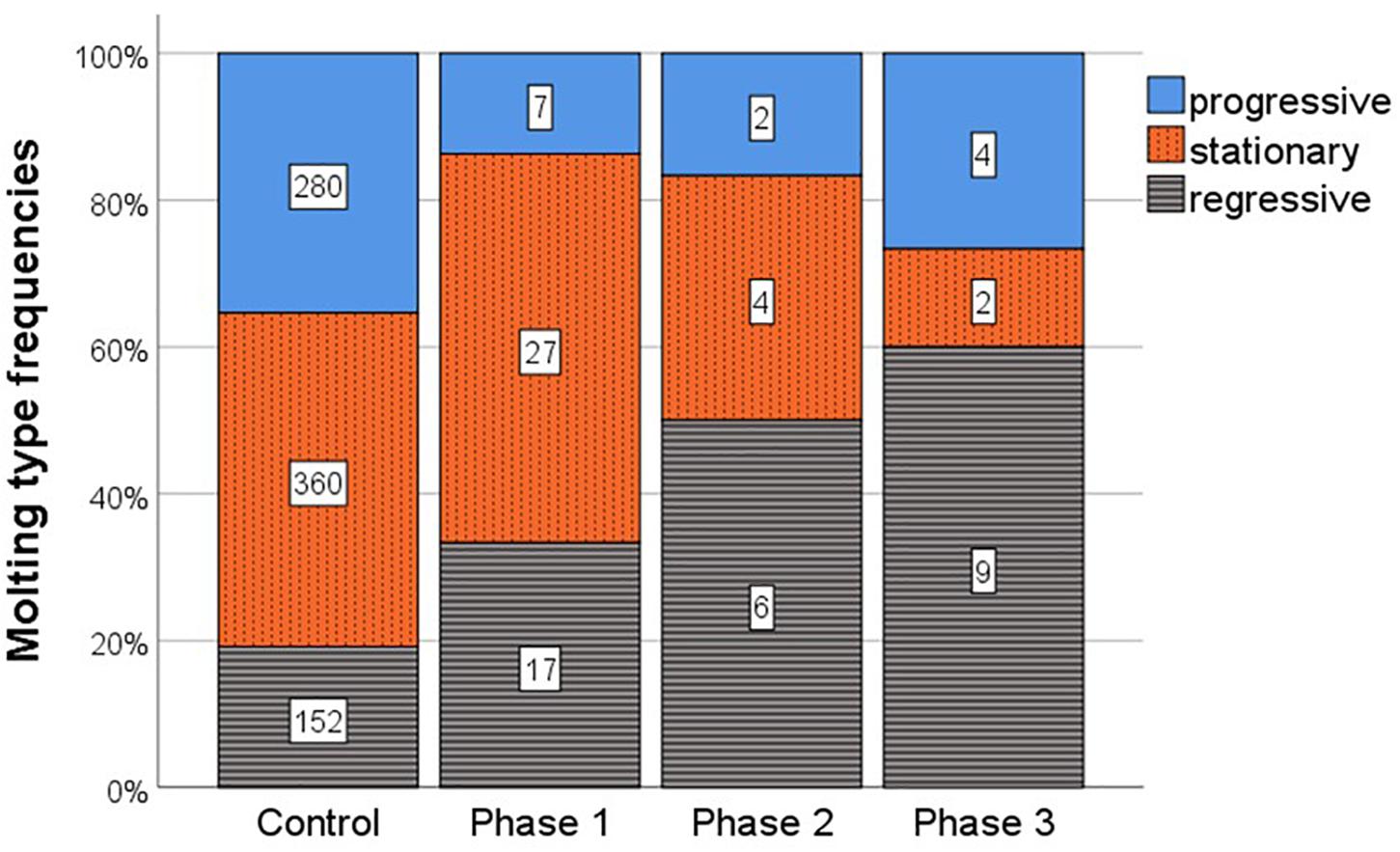
Figure 3. Molting type frequencies (i.e., number of each type of molt) after induction of JH signaling with methoprene during three phases of the intermolt period and in untreated control individuals. Shown are the frequencies of progressive (blue, filled), stationary (orange, dotted vertical stripes), and regressive (gray/black, horizontal stripes) molting types. Numbers inside bar show absolute numbers of each type of molt, the y-axis represents this as relative proportions.
Increased JH signaling induced by methoprene treatment resulted during all three phases in altered molting types. Methoprene treatment directly after a molt (phase 1) significantly changed molting type frequencies [F(2, 839) = 13.56, P < 0.001] with more regressive and less progressive molts compared to control individuals (Figure 3). Post hoc testing revealed that compared to the frequency of regressive molts, the number of progressive molts significantly decreased, while those of stationary molts was unaffected (progressive vs. regressive: t = −5.13, P < 0.001; stationary vs. regressive: t = −1.23, P = 0.218).
Also methoprene treatment during mid-phase of the molting period (phase 2) resulted in significantly altered molting type frequencies [F(2, 800) = 7.75, P < 0.001], again with more regressive and less progressive molts, while the proportion of stationary molts slightly declined (Figure 3). In line, compared to the frequency of regressive molts, the number of progressive molts was significantly reduced and there was a trend that stationary molts also declined (progressive vs. regressive: t = −2.08, P < 0.001; stationary vs. regressive: t = −1.94, P = 0.052).
Methoprene treatment directly before a molt (phase 3) also changed molting type frequencies significantly [F(2, 802) = 134.67, P < 0.001], with an increase in regressive molts and fewer progressive as well as stationary molts (Figure 3). Compared to the frequency of regressive molts, the frequency of progressive molts as well as stationary molts significantly declined (progressive vs. regressive: t = −4.80, P < 0.001; progressive vs. stationary: t = −3.00, P = 0.003) (Figure 3).
We did not observe neotenic- or pre-/soldier development, as expected when using complete colonies in which the presence of the corresponding castes inhibits terminal differentiation.
Expression of JH-Related Genes
Comparing the expression of JH-related gene before (phase 3) and after a molt (phase 1) revealed no significant differences (Mann-Whitney U-test: always P > 0.270; for more details see Supplementary Table S2 and Supplementary Figure S1), except for CsecKr-h1 which was higher expressed before than after a molt (Nbefore molt = 22, Nafter molt = 19, U = 78.00, P = 0.001) (Figure 4). This indicates that JH production did not differ but that JH signaling was higher before than after a molt.
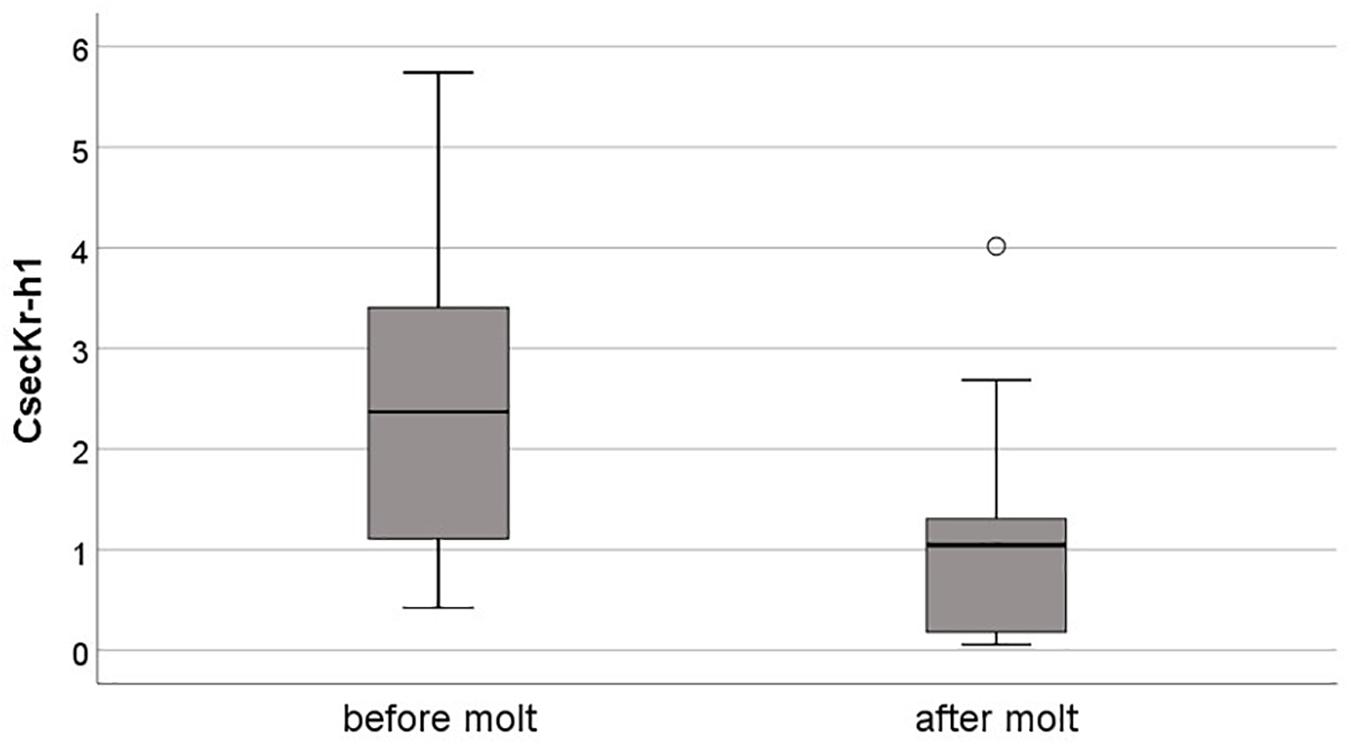
Figure 4. Relative expression of the CsecKr-h1 before and after a molt in untreated individuals. The expression of this early response gene of JH signaling was significantly higher before than after a molt. Shown are boxplots with median, quartiles, minimal and maximal values, and outliers (open circle), the later are cases that have values at least three times the height of the boxes.
After a molt, gene expression did not differ between molting types for the genes CsecJH epoxidase2 and CsecTai (Kruskal Wallis tests: always P > 0.800; for more details see Supplementary Table S3 and Supplementary Figure S2). Yet, CsecJH epoxidase1 and CsecMet expression differed significantly between molting types, and CsecKr-h1 by trend (CsecJH epoxidase1: χ22,19 = 8.16, P = 0.017; CsecMet: χ22,19 = 7.24, P = 0.027; CsecKr-h1: χ22,19 = 5.88, P = 0.053) (Figure 5). All three genes had their highest expression after stationary molts (Figure 5), indicating that JH production as well as signaling are up-regulated after a stationary molt.
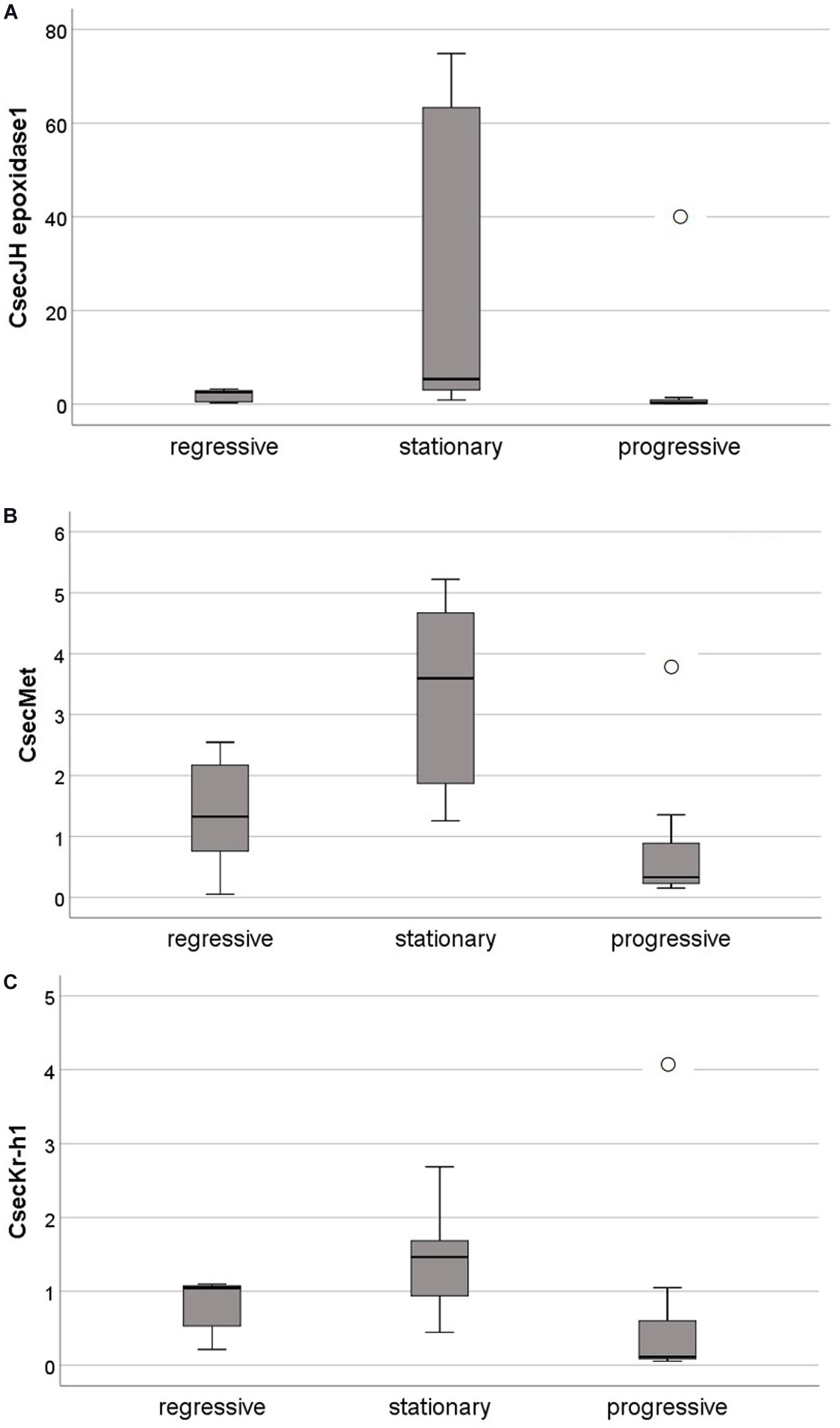
Figure 5. Relative expression of genes related to JH biosynthesis and JH signaling after a molt, in relation to molting types. All shown genes were significantly (by trend for CsecKr-h1) higher expressed after stationary than progressive and regressive molts. (A) CsecJH epoxidase1, a genes supposed to catalyze the last step of JH biosynthesis. (B) CsecMet, the 1:1 ortholog of met from the cockroach Blattella germanica whose protein functions as receptor of JH. (C) CsecKr-h1, the 1:1 ortholog of Kr-h1 in D. melanogaster and B. germanica which functions as early response gene of JH signaling. Shown are boxplots with median, quartiles, minimal and maximal values, and outliers (open circle), the later are cases that have values at least three times the height of the boxes.
Discussion
Our results suggest that hemolymph JH titers during all phases are crucial in determining the developmental trajectory of C. secundus nymphs. Increasing JH signaling with methoprene always influenced molting type frequencies compared to untreated control individuals (Figure 3). As a caveat, it should be noted that we did not test the methoprene treated individuals against solvent treated individual in our phase-specific experiment. Yet, we found no solvent (acetone) effect on molting types in our supplementary experiments.
During all phases an increase in JH signaling resulted in an elevated proportion of regressive molts. During all phases this was at the cost of progressive molts which became less common (Figure 3). Starting in phase 2, also the number of stationary molts decreased, while they slightly—but not significantly—increased during phase 1. Our results imply that (i) high JH titers, regardless of the intermolt period, favor regressive development, (ii) progressive development is associated with low—or at least not high—JH titers during all phases of the intermolt period, and (iii) stationary molts seem to be less influenced by varying JH titers during the first phase (i.e., directly after the molt) but they also require rather low titers thereafter, especially before the next molt.
We adjusted our methoprene concentrations to what was used before in termite studies (see Methods). Yet, we cannot completely rule out that we observed a pharmacological rather than a physiological effect due to emulating artificially high JH titers. If this was the case, postembryonic developmental plasticity could not be explained by varying JH titers during the intermolt period as the NW model predicted. Given the evidence that exists that varying JH titers are associated with different developmental trajectories in termites in general (reviewed in Korb, 2015; for soldiers: Miura and Scharf, 2011) and the fact that our results are in line with a former study that found an association of high JH titers with regressive molts in C. secundus (Korb et al., 2009), we think that it is unlikely that we produced highly artificial results in our study.
Updating the NW Model to Explain Termite’s Development Plasticity
Our data confirmed the NW model and former suggestions in that progressive molts require rather low JH titers throughout the molting period (Springhetti, 1969; Nijhout and Wheeler, 1982; Korb et al., 2009; Korb and Bellés, 2017; Figures 2, 6). In line, average JH titers decline in C. secundus (Korb et al., 2009) as well as Hodotermes sjostedti (Cornette et al., 2008) with progressive development along the nymphal-alate line.
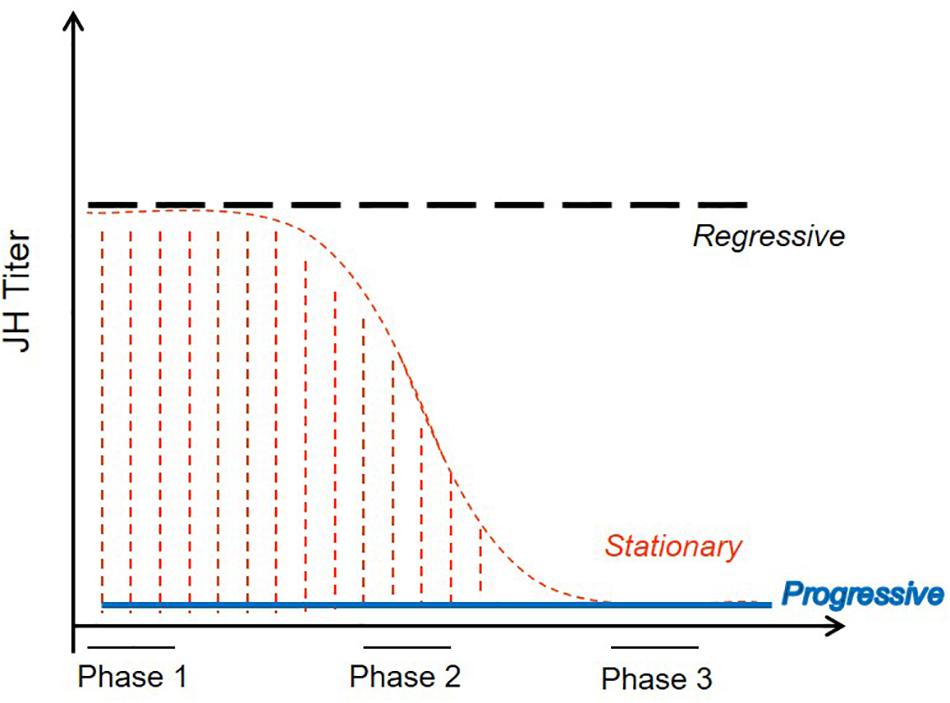
Figure 6. According to current results, revised model to explain the unparalleled diversity of postembryonic, non-terminal developmental trajectories of wood-dwelling termites. High JH titers throughout the intermolt period increase the likelihood of regressive development (black, broken line). Progressive molts (blue, continuous line) require low JH titers throughout the intermolt period. Stationary molts (orange, dotted line), by contrast, can have medium-high JH titers during phase 1 but require increasing low JH titers thereafter. The presence of reproductives and soldiers prevent terminal molts into the corresponding castes. For further information, see text.
The NW model did not explicitly mention regressive molts. Yet, it suggested that individuals that remain immature workers—which comprises regressive and stationary molts—are characterized by first high, then low JH titers (Figure 2). This corresponds with our results for the stationary molts which seem to require low JH signaling during phase 3 (Figure 3). However, the NW model’s suggestion that low JH titers toward the end of the intermolt period characterize individuals that remain immatures, is not in line with our findings that regressive molts were induced by high JH signaling during phase 3 (Figure 3). Yet, our results correspond with previous hypotheses (Lüscher, 1956, 1974b; Springhetti, 1969) and first evidence (Korb et al., 2009) that regressive molts are the results of high JH titers throughout the intermolt period.
According to the NW model as well as several experimental studies using JH analoga and in vitro measurements (e.g., Elliott and Stay, 2008; for recent reviews see: Miura and Scharf, 2011; Watanabe et al., 2014; Korb, 2015), high JH titers lead to soldier development. This seems to contrast with our results that they elicit regressive molts. However, presoldier/soldier development generally require an absence of the soldiers which prevent the differentiation of further soldiers (e.g., Castle, 1934; Lüscher, 1974a; Haverty and Howard, 1981; Lefeuve and Bordereau, 1984; Henderson, 1998; Korb et al., 2003; Park and Raina, 2005; Tarver et al., 2010; Miura and Scharf, 2011; Watanabe et al., 2011, 2014; for few exception see Lenz, 1976; Hrdy et al., 2006).
Our experiments were done with complete colonies, including soldiers, and soldiers inhibit soldier differentiation in C. secundus (Korb et al., 2003; Roux and Korb, 2004). Thus, high JH signaling in the presence of soldiers seems to trigger regressive molts while it may lead to soldier differentiation in the absence of soldiers. This corresponds to a recently proposed model for the evolution of termite developmental plasticity based on “shared endocrinology” between postembryonic, non-terminal and terminal molts derived from a cockroach ancestor (Korb and Bellés, 2017). According to this model, JH titers in combination with social interactions could explain termite plasticity. High JH titers in the presence of soldiers result in regressive molts, while presoldier/soldier development is induced when soldiers are lacking. Correspondingly, constantly low JH titers trigger either progressive postembryonic development or a terminal neotenic molt, depending on the presence and absence of reproductives, respectively. In our experiment, the presence of reproductives prevented neotenic differentiation in C. secundus as it does in other Kalotermitidae (e.g., Grassé and Noirot, 1946; Lüscher, 1952; Lüscher, 1974b; Lenz, 1976; Hoffmann and Korb, 2011; for a recent review see: Korb, 2015). This model of shared endocrinology is in line with several recent studies which also stress the importance of social interactions in explaining phenotypic plasticity in termites (e.g., Tarver et al., 2010; Watanabe et al., 2014; Oguchi et al., 2020).
What Next?
Our study is the first that explicitly tested the NW model by phase-specific endocrine manipulations which led to a revision of the NW model and it improved our understanding of unparalleled diversity of postembryonic development in termites (Figure 6). Yet, it has some caveats that should be addressed in further studies. The sample sizes were relatively small for the later phase manipulations compared to the control treatment. However, we found statistical support for changes caused by methoprene treatment, which suggests that the effects were large. Additional studies, including also other species, should test the robustness of our results. Such investigations should most suitably use acetone (solvent) treated individuals as control. In our supplementary experiments, we found no evidence that acetone had an effect on molting types. Neither the direct comparison of molting types with untreated individuals revealed significant differences nor our gene expression experiment suggested an effect of acetone on JH signalling in workers. More studies could substantiate our results.
Our studies gives directions for future research by identifying a number of specific questions: How does JH-related gene expression vary during the different phases? We determined it for phase 1 and found that it varies and that it seems affected by the preceding molting type. As a consequence, does a preceding molting type influence the direction of the subsequent molt and hence does a sequential pattern of molting types emerge? We have some preliminary data (unpublished) that this seems not to be the case. Are there any predictors of an individual’s upcoming molting type? This appears as one of the most urgent quests to unravel the molecular mechanisms underlying the different developmental trajectories. Our study opened a new door by performing intermolt/phase-specific manipulations of JH signaling, which have not been done before. By doing so, it revealed new insights into the puzzle how termites can achieve their extraordinary diversity of postembryonic developmental plasticity, with a “standard” endocrine toolkit.
Data Availability Statement
All data are uploaded as Supplementary Material, which we have updated and which are attached.
Author Contributions
JK conceptualized and designed the experiments, analyzed the data, and wrote the manuscript. CG and MF performed endocrine experiments and analyzed the data. AG performed qPCR experiments and analyzed the data. All authors read the manuscript and approved publication of the manuscript.
Funding
This work was supported by the Deutsche Forschungsgemein-schaft (DFG) (KO1895/6 and KO1895/20-1).
Conflict of Interest
The authors declare that the research was conducted in the absence of any commercial or financial relationships that could be construed as a potential conflict of interest.
Acknowledgments
We thank Parks and Wildlife Commission, Northern Territory, and Environment Australia for permission to collect (permits 26851 and 30073) and export (permits WT2007-4154 and WT2008-4184) the termites; Charles Darwin University (Australia), and especially S. Garnett and the Horticulture and Aquaculture team, for providing logistic support during termite collection; Katharina Hoffmann for help in collecting termites and performing experiments, the editor FC for providing the opportunity to submit this work and two reviewers for helpful comments on the manuscript. The study was conducted according to the Nagoya protocol.
Supplementary Material
The Supplementary Material for this article can be found online at: https://www.frontiersin.org/articles/10.3389/fevo.2021.619594/full#supplementary-material
References
Abe, T. (1987). “Evolution of life types in termites,” in Evolution and Coadaptation in Biotic Communities, eds S. Kawano, J. H. Connell, and T. Hidaka (Tokyo: University of Tokyo Press), 125–148.
Bell, W. J., Roth, L. M., and Nalepa, C. A. (2007). Cockroaches: Ecology, Behavior, and Natural History. Baltimore, MD: The Johns Hopkins University Press.
Bellés, X., Martín, D., and Piulachs, M.-D. (2005). The mevalonate pathway and the synthesis of juvenile hormone in insects. Annu. Rev. Entomol. 50, 181–199. doi: 10.1146/annurev.ento.50.071803.130356
Bellés, X., and Santos, C. G. (2014). The MEKRE93 (Methoprene tolerant-Krüppel homolog 1-E93) pathway in the regulation of insect metamorphosis, and the homology of the pupal stage. Insect Biochem. Mol. Biol. 52, 60–68. doi: 10.1016/j.ibmb.2014.06.009
Brent, C. S., Schal, C., and Vargo, E. L. (2005). Endocrine changes in maturing primary queens of Zootermopsis angusticollis. J. Insect Physiol. 51, 1200–1209. doi: 10.1016/j.jinsphys.2005.06.009
Castle, G. B. (1934). “The damp-wood termite of the Western United States, genus Zootermopsis (formerly, Termopsis),” in Termites and Termite Control, ed. G. A. Kofoid (Berkeley, CA: University of California Press), 273–310.
Cornette, R., Gotoh, H., Koshikawa, S., and Miura, T. (2008). Juvenile hormone titers and caste differentiation in the damp-wood termite Hodotermopsis sjostedti (Isoptera, Termopsidae). J. Insect Physiol. 54, 922–930. doi: 10.1016/j.jinsphys.2008.04.017
Elliott, K. L., and Stay, B. (2008). Changes in juvenile hormone synthesis in the termite Reticulitermes flavipes during development of soldiers and neotenic reproductives from groups of isolated workers. J. Insect Physiol. 54, 492–500. doi: 10.1016/j.jinsphys.2007.11.008
Elsner, D., Meusemann, K., and Korb, J. (2018). Longevity and transposon defense, the case of termite reproductives. Proc. Natl. Acad. Sci. U.S.A. 115, 5504–5509. doi: 10.1073/pnas.1804046115
Grassé, P. P., and Noirot, C. (1946). La production des sexués néoteniques chez le termite á cou jaune (Calotermes flavicollis): inhibition germinale et inhibition somatique. Compt. Rend. Acad. Sci. 223, 869–871.
Harrison, M. C., Jongepier, E., Robertson, H. M., Arning, N., Bitard-Feildel, T., Chao, H., et al. (2018). Hemimetabolous genomes reveal molecular basis of termite eusociality. Nat. Ecol. Evol. 2, 557–566. doi: 10.1038/s41559-017-0459-1
Haverty, M. I., and Howard, R. W. (1981). Production of soldiers and maintenance of soldier proportions by laboratory experimental groups of Reticulitermes flavipes (Kollar) and Reticulitermes virginicus (Banks) (Isoptera: Rhinotermitidae). Insectes Soc. 28, 32–39. doi: 10.1007/bf02223620
Henderson, G. (1998). “Primer pheromones and possible soldier caste influence on the evolution of sociality in lower termites,” in Phermone Communication in Social Insects, eds R. K. Vander Meer, M. D. Breed, K. E. Espelie, and M. L. Winston (Boulder, CO: Westview Press), 314–330. doi: 10.1201/9780429301575-13
Hoffmann, K., and Korb, J. (2011). Is there conflict over direct reproduction in lower termite colonies? Anim. Behav. 81, 265–274. doi: 10.1016/j.anbehav.2010.10.017
Hrdy, I., Kuldová, J., Hanus, R., and Wimmer, Z. (2006). Juvenile hormone III, hydroprene and a juvenogen as soldier caste differentiation regulators in three Reticulitermes species: potential of juvenile hormone analogues in termite control. Pest Manag. Sci. 62, 848–854. doi: 10.1002/ps.1244
Inward, D., Beccaloni, G., and Eggleton, P. (2007). Death of an order: a comprehensive molecular phylogenetic study confirms that termites are eusocial cockroaches. Biol. Lett. 3, 331–335. doi: 10.1098/rsbl.2007.0102
Jindra, M., Belles, X., and Shinoda, T. (2015). Molecular basis of juvenile hormone signaling. Curr. Opin. Insect Sci. 11, 39–46. doi: 10.1016/j.cois.2015.08.004
Jongepier, E., Kemena, C., Lopez-Ezquerra, A., Bellés, X., Bornberg-Bauer, E., and Korb, J. (2018). Remodeling of the juvenile hormone pathway through caste-biased gene expression and positive selection along a gradient of termite eusociality. J. Exp. Zool. B Mol. Dev. Evol. 330, 296–304. doi: 10.1002/jez.b.22805
Korb, J. (2015). “Juvenile hormone, a central regulator of termite caste polyphenism,” in Advances in Insect Physiology 48: Physiology, Behavior, and Genomics of Social Insects, eds C. Kent and A. Zayed (Oxford: Elsevier), 131–161.
Korb, J., and Bellés, X. (2017). Juvenile hormone and hemimetabolan eusociality: a comparison of cockroaches with termites. Curr. Opin. Insect Sci. 22, 109–116. doi: 10.1016/j.cois.2017.06.002
Korb, J., and Hartfelder, K. (2008). Life history and development – a framework for understanding the ample developmental plasticity in lower termites. Biol. Rev. Camb. Philos. Soc. 83, 295–313.
Korb, J., Hoffmann, K., and Hartfelder, K. (2009). Endocrine signatures underlying plasticity in postembryonic development of a lower termite, Cryptotermes secundus (Kalotermitidae). Evol. Dev. 11, 269–277. doi: 10.1111/j.1525-142x.2009.00329.x
Korb, J., Hoffmann, K., and Hartfelder, K. (2012). Molting dynamics and juvenile hormone titer profiles in the nymphal stages of a lower termite, Cryptotermes secundus (Kalotermitidae) – signatures of developmental plasticity. J. Insect Physiol. 58, 376–383. doi: 10.1016/j.jinsphys.2011.12.016
Korb, J., and Katrantzis, S. (2004). Influence of environmental conditions on the expression of the sexual dispersal phenotype in a lower termite: implications for the evolution of workers in termites. Evol. Dev. 6, 342–352. doi: 10.1111/j.1525-142x.2004.04042.x
Korb, J., and Lenz, M. (2004). Reproductive decision-making in the termite, Cryptotermes secundus (Kalotermitidae), under variable food conditions. Behav. Ecol. 15, 390–395. doi: 10.1093/beheco/arh033
Korb, J., Roux, E., and Lenz, M. (2003). Proximate factors influencing soldier development in the basal termite Cryptotermes secundus (Hill). Insectes Soc. 50, 299–303. doi: 10.1007/s00040-003-0674-4
Korb, J., and Schmidinger, S. (2004). Help or disperse? Cooperation in termites influenced by food conditions. Behav. Ecol. Sociobiol. 56, 89–95. doi: 10.1007/s00265-004-0757-x
Korb, J., and Schneider, K. (2007). Does kin structure explain the occurrence of workers in a lower termite? Evol. Ecol. 21, 817–828. doi: 10.1007/s10682-006-9153-5
Korb, J., and Thorne, B. (2017). “Sociality in termites,” in Comparative Social Evolution, eds D. R. Rubenstein and P. Abbot (Cambridge: Cambridge University Press), 124–153.
Lanzrein, B., Gentinetta, V., and Fehr, R. (1985). “Titres of juvenile hormone and ecdysteroids in reproductives and eggs of Macrotermes michaelseni: relation to caste determination?,” in Caste Differentiation in Social Insects, eds J. A. L. Watson, B. M. Okot-Kotber, and C. Noirot (Oxford: Pergamon Press), 307–328. doi: 10.1016/b978-0-08-030783-1.50027-6
Lefeuve, P., and Bordereau, C. (1984). Soldier formation regulated by a primer pheromone from the soldier frontal gland in a higher termite, Nasutitermes lujae. Proc. Natl. Acad. Sci. U.S.A. 81, 7665–7668. doi: 10.1073/pnas.81.23.7665
Lenz, M. (1976). “The dependence of hormone effects in termite caste determination on external factors,” in Phase and Caste Determination in Insects - Endocrine Aspects, ed. M. Lüscher (Oxford: Pergamon Press), 73–89. doi: 10.1016/b978-0-08-021256-2.50012-1
Lüscher, M. (1952). Die Produktion und Elimination von Ersatzgeschlechtstieren bei der Termite Kalotermes flavicollis (Fabr.). Zeitschrift vergl. Physiol. 34, 123–141.
Lüscher, M. (1956). Die Entstehung von Ersatzgeschlechtstieren bei der Termite Kalotermes flavicollis (Fabr.). Insectes Soc. 3, 119–128. doi: 10.1007/bf02230672
Lüscher, M. (1974a). Die Kompetenz zur Soldatenbildung bei Larven (Pseudergaten) der Termite Zootermopsis angusticollis. Rev. Suisse Zool. 81, 711–714.
Lüscher, M. (1974b). “Kasten und Kastendifferenzierung bei niederen Termiten,” in Sozialpolymorphismus bei Insekten, ed. G. H. Schmidt (Stuttgart: Wissenschaftliche Verlagsgesellschaft), 694–739.
Maekawa, K., Hayashi, Y., Lee, T., and Lo, N. (2014). Presoldier differentiation of Australian termite species induced by juvenile hormone analogues. Austral Entomol. 53, 138–143. doi: 10.1111/aen.12060
Maekawa, K., Nakamura, S., and Watanabe, D. (2012). Termite soldier differentiation in incipient colonies is related to parental proctodeal trophallactic behavior. Zoolog. Sci. 29, 213–217. doi: 10.2108/zsj.29.213
Miura, T., and Scharf, M. E. (2011). “Molecular basis underlying caste differentiation in termites,” in Biology of Termites: A Modern Synthesis, eds D. E. Bignell, Y. Roisin, and N. Lo (London: Springer Press), 211–254. doi: 10.1007/978-90-481-3977-4_9
Nijhout, H. F., and Wheeler, D. E. (1982). Juvenile hormone and the physiological basis of insect polyphenisms. Q. Rev. Biol. 57, 109–133. doi: 10.1086/412671
Noirot, C. (1990). “Sexual castes and reproductive strategies in termites,” in An Evolutionary Approach to Castes and Reproduction, ed. W. Engels (Berlin: Springer Verlag), 5–35. doi: 10.1007/978-3-642-74490-7_3
Noirot, C., and Pasteels, J. M. (1987). Ontogenic development and evolution of the worker caste in termites. Experientia 43, 851–860. doi: 10.1007/bf01951642
Oguchi, K., Sugime, Y., Shimoji, H., Hayashi, Y., and Miura, T. (2020). Male neotenic reproductives accelerate additional differentiation of female reproductives by lowering JH titer in termites. Sci. Rep. 10:9435.
Park, Y. I., and Raina, A. K. (2004). Juvenile hormone III titers and regulation of soldier caste in Coptotermes formosanus (Isoptera: Rhinotermitidae). J. Insect Physiol. 50, 561–566. doi: 10.1016/j.jinsphys.2004.04.002
Park, Y. I., and Raina, A. K. (2005). Regulation of juvenile hormone titers by soldiers in the Formosan subterranean termite Coptotermes formosanus. J. Insect Physiol. 51, 385–391. doi: 10.1016/j.jinsphys.2005.02.001
Roisin, Y. (2000). “Diversity and evolution of caste patterns,” in Termites: Evolution, Sociality, Symbioses, Ecology, eds T. Abe, D. E. Bignell, and M. Higashi (Dordrecht: Kluwer Academic Publishers), 95–119. doi: 10.1007/978-94-017-3223-9_5
Roisin, Y., and Korb, J. (2011). “Social organisation and the status of workers in termites,” in Biology of Termites: A Modern Synthesis, eds D. E. Bignell, Y. Roisin, and N. Lo (London: Springer), 133–164. doi: 10.1007/978-90-481-3977-4_6
Roux, E. A., and Korb, J. (2004). Evolution of eusociality and the soldier caste in termites: a validation of the intrinsic benefit hypothesis. J. Evol. Biol. 17, 869–875. doi: 10.1111/j.1420-9101.2004.00727.x
Springhetti, A. (1969). Il controllo sociale della differenziazione degli alati in Kalotermes flavicollis Fabr. (Isoptera). Ann. Univ. Ferrara Sez III Biol. Anim. 3, 73–96.
Springhetti, A. (1972). The competence of Kalotermes flavicollis F. (Isoptera) pseudergates to differentiate into soldiers. Monit. Zool. Ital. 6, 97–111.
Tarver, M. R., Florane, C., Zhang, D., Grimm, C., and Lax, A. R. (2012). Methoprene and temperature effects on caste differentiation and protein composition in the Formosan subterranean termite Coptotermes fomosanus. J. Insect Sci. 12:e18.
Tarver, M. R., Zhou, X. G., and Scharf, M. E. (2010). Socio-environmental and endocrine influences on developmental and caste-regulatory gene expression in the eusocial termite Reticulitermes flavipes. BMC Mol. Biol. 11:e28. doi: 10.1186/1471-2199-11-28
Watanabe, D., Gotoh, H., Miura, T., and Maekawa, K. (2011). Soldier presence suppressed presoldier differentiation through a rapid decrease of JH in the termite Reticulitermes speratus. J. Insect Physiol. 57, 791–795. doi: 10.1016/j.jinsphys.2011.03.005
Watanabe, D., Gotoh, H., Miura, T., and Maekawa, K. (2014). Social interactions affecting caste development through physiological actions in termites. Front. Physiol. 5:e127. doi: 10.3389/fphys.2014.00127
Weil, T., Rehli, M., and Korb, J. (2007). Molecular basis for the reproductive division of labour in a lower termite. BMC Genomics 8:e198. doi: 10.1186/1471-2164-8-198
Yagi, K. J., Kwok, R., Chan, K. K., Setter, R. R., Myles, T. G., Tobe, S. S., et al. (2005). Phe-Gly-Leu-amide allatostatin in the termite Reticulitermes flavipes: content in brain and corpus allatum and effect on juvenile hormone synthesis. J. Insect Physiol. 51, 357–365. doi: 10.1016/j.jinsphys.2004.12.006
Keywords: developmental plasticity, social insect, juvenile hormone, molt, methoprene, JH epoxidase, krüppel-homolog 1
Citation: Korb J, Greiner C, Foget M and Geiler A (2021) How Can Termites Achieve Their Unparalleled Postembryonic Developmental Plasticity? A Test for the Role of Intermolt-Specific High Juvenile Hormone Titers. Front. Ecol. Evol. 9:619594. doi: 10.3389/fevo.2021.619594
Received: 20 October 2020; Accepted: 09 February 2021;
Published: 03 March 2021.
Edited by:
Fernando Casares, Andalusian Center for Development Biology (CABD), SpainReviewed by:
Bertrand Fouks, University of Münster, GermanyVincent Debat, Muséum National d’Histoire Naturelle, France
Copyright © 2021 Korb, Greiner, Foget and Geiler. This is an open-access article distributed under the terms of the Creative Commons Attribution License (CC BY). The use, distribution or reproduction in other forums is permitted, provided the original author(s) and the copyright owner(s) are credited and that the original publication in this journal is cited, in accordance with accepted academic practice. No use, distribution or reproduction is permitted which does not comply with these terms.
*Correspondence: Judith Korb, judith.korb@biologie.uni-freiburg.de