Fire in Semi-Arid Shrublands and Woodlands: Spatial and Temporal Patterns in an Australian Landscape
- 1Centre for Ecosystem Management, School of Science, Edith Cowan University, Perth, WA, Australia
- 2School of Life and Environmental Sciences, University of Sydney, Sydney, NSW, Australia
Semi-arid landscapes are of interest to fire ecologists because they are generally located in the climatic transition zone between arid lands (where fires tend to be rare due to lack of fuel, but are enhanced following large rainfall episodes) and more mesic regions (where fire activity tends to be enhanced following severe rainfall deficits). Here we report on the characteristics of the contemporary fire regimes operating in a semi-arid region of inland south-western Australia with rainfall averaging around 300 mm per annum. To characterize fire regimes, we analyzed a geodatabase of fire scars (1960–2018) to derive fire preferences for each major vegetation type and fire episode and used known fire intervals to model fire hazard over time and calculate typical fire frequencies. We also used super epoch analysis and correlations to explore relationships between annual fire extent and rainfall received before the fire. We found fires strongly favored sandplain shrublands, and these tended to experience hot crown fires once every 100 years (median fire interval), with fire hazard increasing linearly over time. In contrast, fires were rare in eucalypt woodland and other vegetation types, with a median interval of 870 years and broadly consistent fire hazard over time. Annual fire extent was most strongly linked with high rainfall in the year prior to fire, and this was particularly so for eucalypt woodlands. Large-scale fires in shrublands tended to favor areas burnt in previous large fires, whereas in woodlands they favored edges. In conclusion, we found divergent fire regimes across the major vegetation types of the region. Sandplain shrublands were similar to Mediterranean shrublands in that they experienced intense stand-replacing wildfires which recovered vigorously although slowly, meaning burnt shrublands did not experience fires again for at least 25 and 100 years on average. In contrast, eucalypt woodlands were fire sensitive (trees readily killed by fire) and experienced fires mostly around the edges, spreading into core areas only after large rainfall events elevated fuel levels. Overall, both vegetation types subscribed to typical arid-zone fire regimes where elevated rainfall, and not drought, promoted fires, although the role of fuel accumulation over time was more important in the shrublands.
Introduction
Fire influences the composition and function of many ecosystems across the globe (He et al., 2019). It is generally accepted that the biota of these ecosystems have become adapted to natural fire regimes over millennia, and any major deviations from this regime will likely influence their composition, structure and/or functioning (Bowman et al., 2009; Avitabile et al., 2013). Effectively managing fire within terrestrial ecosystems thus relies on knowledge of both past and present fire regimes. Understanding fire regimes can also help fire managers predict future fire behavior, manage fuels and fire risks, and plan and implement appropriate fire intervals and fire age distributions for biodiversity conservation (Driscoll et al., 2010).
Both historic and contemporary fire regimes are poorly known for many ecosystems, especially so for large, remote, and sparsely populated regions, which includes large expanses of the world’s drylands. Fire is a relatively rare event in arid lands because rainfall and productivity are too low to support the dense vegetation and continuous fuel needed to sustain regular fires (Pausas and Bradstock, 2007; Pausas and Ribeiro, 2013). There are exceptions to this though, such as landscapes dominated by xerophytic perennial grasses (e.g., Triodia grasslands in arid Australia), areas lower in the landscape which develop denser vegetation, periods of unusually high rainfall which result in exceptional grass and forb growth, or landscapes where invasive species, particularly alien grasses, enhance fuel loads (Allan and Southgate, 2002; Keeley et al., 2012; Balch et al., 2013; van Etten and Burrows, 2018).
Semi-arid zones occur in the transition between mesic regions where fire regimes are typically driven by occasional droughts that render vegetation more flammable -and arid lands where fire regimes are typically driven by rainfall-enhanced fuels resulting from rare high rainfall events (Pausas and Paula, 2012; McLauchlan et al., 2020). These drivers tend to unfold once sufficient vegetation recovery after fire has occurred, although fuel accumulation can continue for decades after recovery in some ecosystems, and so time since fire (TSF) can continue to shape fire hazard, whilst in other ecosystems fire weather plays a more important role, post-recovery (Moritz et al., 2004). Semi-arid zones, given sufficient TSF, can support shrub-dominated vegetation dense enough to enable crown fires, which are typically intense and “stand-replacing” (Keeley et al., 2012; Dalgleish et al., 2015). However, they can also support more open vegetation which rarely experiences fire (Gosper et al., 2013a,b). In the western United States, McKenzie and Littell (2017) identified hybrid ecoregions in intermediate rainfall zones where both recent drought and abundant rainfall in previous years can encourage fires, but it is unclear if this due to temporal wet-dry sequences or spatial configurations of ecosystems with divergent fire regimes and drivers.
Across the transition from an arid to Mediterranean-type climate in southern Australia, fire frequency and extent increases in a southerly direction in line with increasing rainfall, productivity, and vegetation cover (Pausas and Bradstock, 2007). Gibson et al. (2015) also found increasing fire frequency with increasing rainfall across this gradient, but also greater influence of TSF driving fire intervals, although pre-fire rainfall was the most important driver across the whole gradient. They also found that fuel accumulation (i.e., TSF) was a more influential driver on more fertile patches of soil. This demonstrates the importance of productivity variation in explaining differences in fire regimes at both regional and landscape scales. Indeed, landscapes are not always uniform in terms of the spatial arrangement of fire regimes (as well as extent of individual fires), potentially varying from regularly burnt and highly fire-prone patches or vegetation types to areas that rarely or never experience fire, which reflects variation in topography, geomorphology and vegetation (Turner and Romme, 1994; Kane et al., 2015; McLauchlan et al., 2020).
Understanding the controls of fires at the landscape scale and why some areas are preferentially burnt over others, is important for both researchers and land managers (Archibald et al., 2009). Moreover, fine-scale patchiness in burn area and intensity may occur, even in the most fire-prone vegetation, contributing to heterogeneity of vegetation at this and broader spatial scales (Turner et al., 1994; Schoennagel et al., 2009). Such patchiness may arise from local-scale variation in vegetation and fuel, the residual effect(s) of previous fires, or may reflect stochastic factors related to fire behavior. The idea of ecological memory is important too, with burnt patches in the landscape potentially becoming preferred pathways for subsequent fires, which may then lead to further differentiation of landscape patterns over time (Peterson, 2002).
The main method used to map and analyze fire history from regional to landscape scales, involves manipulation, comparison and analysis of remotely sensed imagery to detect recently burnt areas. This imagery is typically obtained from satellites, which routinely became available from about 1972, and have improved in spectral range and resolution since then (Turner et al., 2008; Avitabile et al., 2013). To obtain fire scar information before this time requires interpretation of aerial photography, a methodology which suffers from intermittent coverage, variable quality and resolution, and narrow spectral range, although it is generally suitable for arid/semi-arid regions as fire scars are often detectable for many years before the vegetation fully regenerates (Burrows and Christensen, 1990). Although modeling reflectance data from satellite imagery to estimate long-term fire history is a promising approach for some ecosystems (Callister et al., 2016), fire mapping at regional scales is mostly limited to the last few decades. Once obtained, accurately mapped fire scars can be analyzed using GIS and statistical models to determine the key characteristics of fire regimes such as typical fire intervals (e.g., McCarthy et al., 2001), fire preferences (e.g., Moreira et al., 2009), and relationships with climate anomalies and other potential drivers (e.g., O’Donnell et al., 2011a).
In this paper, we analyzed mapped fire scars to quantify and describe the contemporary fire regimes of a poorly known area of inland, semi-arid Western Australia. The study area is covered by a complex mosaic of several distinct vegetation types, some of which are dense fire-prone shrublands, but others appear to be fire sensitive. Local land managers have been concerned over the size and frequency of recent fires (Braun, 2006; Parsons and Gosper, 2011). We use this case study to address broader ecological questions regarding landscape-scale fire preferences and regimes: (1) are spatial patterns of fire random or deterministic?; (2) how important are occasional large fires in shaping fire history and fire regimes?; (3) do landscapes with contrasting vegetation types have different fire regimes and, if so, how can they coexist?; and (4) are major fires in this semi-arid transition zone mostly driven by above- or below-average rainfall episodes, or some combination of these?
Materials and Methods
Study Area
The study area covers ∼3,300 km2 of the transitional zone between the Southwest Botanical Province of Western Australia (which has a Mediterranean-type climate) and the arid Eremean Botanical Province (Figure 1). It is located approximately 400 km north-east of Perth and the nearest coastline lies about 200 km to the west. Almost all fires are confined to the study area because it is bounded by large salt lakes to the east and west, the heavily cleared wheatbelt to the south, and more arid and sparse vegetation to the north (Figure 1). This study area is therefore ideal for studying fire patterns. It is also an area of high conservation value due to its high diversity of plant taxa (Hopper and Gioia, 2004) and high degree of intactness (i.e., uncleared and little modified by human activity).
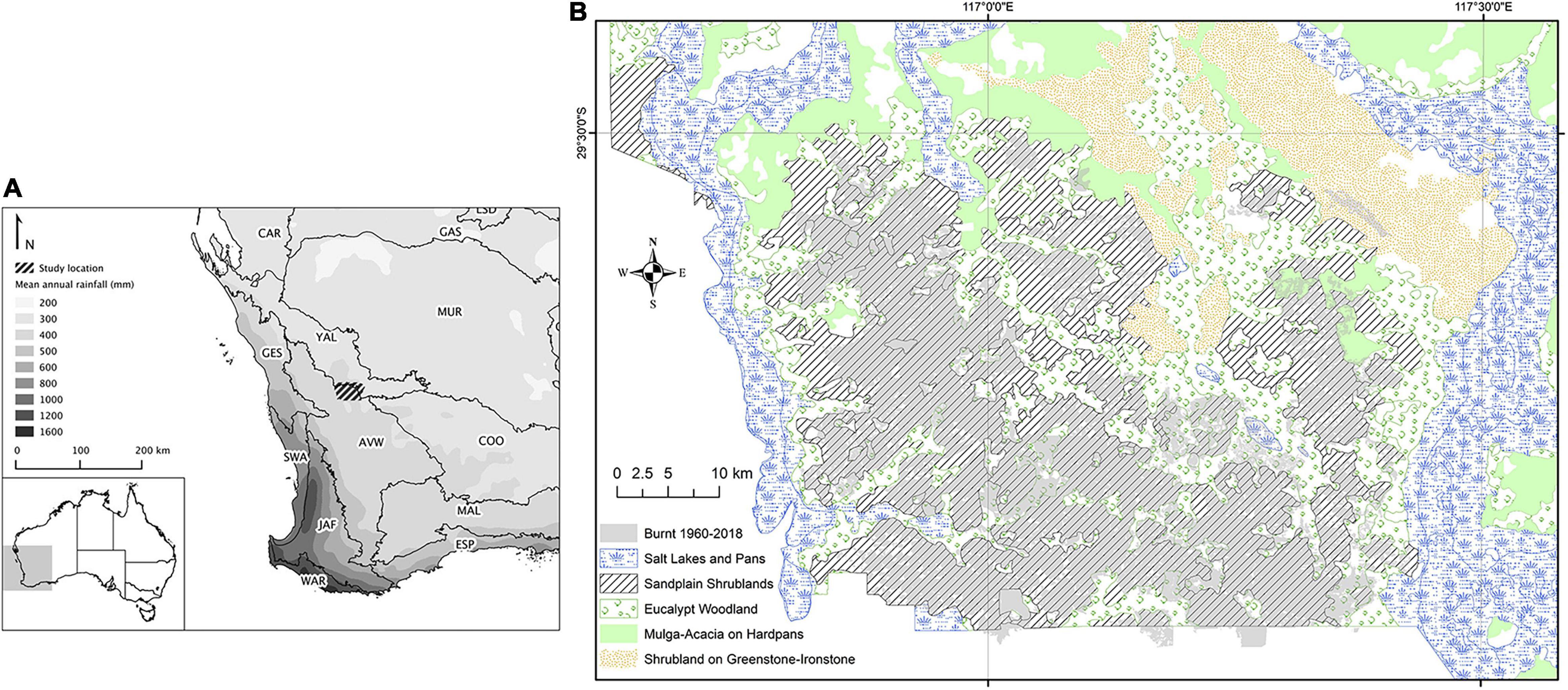
Figure 1. Maps of study area showing: (A) location within Australia and region relative to average annual rainfall and Australian bioregions, and (B) areas burnt 1960–2018 (shaded in gray) in relation to major vegetation types as per legend.
The climate of the study area is semi-arid with average annual rainfall of c.300 mm, of which just over one third falls in cooler months (June to August inclusive), and an aridity index (ratio of average precipitation to potential evaporation) of approximately 0.15. A climatic gradient exists across the study area, running in a more-or-less north-easterly direction. Based on interpolated climate surfaces using rainfall data from 1976 to 2005 (ANUCLIM v6.1; Xu and Hutchinson, 2011), average annual rainfall declines gradually from 300 mm in south-west corner to 280 mm in the north-east corner, with the proportion of summer rain (January–March inclusive) increasing from 20 to 25%. Land tenure consists of vacant Crown land and destocked former pastoral leases, previously grazed by sheep (although lightly so in sandplain shrublands), but now mostly managed for conservation.
Vegetation of the study area comprises a mosaic of recurring vegetation types with 15 land systems described for the study area (Payne et al., 1998). These land systems have been grouped into five major land cover types as per recommendations of Payne et al. (1998), each having distinctive vegetation characteristics. These are hereafter called “vegetation types” in this paper and used as the basis for many of the analyses. These vegetation types are: (1) mulga woodland comprising Acacia aneura and other Acacia trees and tall shrubs on extensive hardpan plains and red loams, mostly found in northern parts of study area; (2) sandplain shrublands comprising dense shrub vegetation on gently undulating yellow sandplains derived from weathering of underlying granite and dominated by shrubs of Acacia, Myrtaceae (particularly Melaleuca), Proteaceae (e.g., Hakea, Grevillea), Allocasuarina, Hibbertia and Rutaceae (e.g., Philotheca, Phebalium); (3) more open tall shrublands of mixed species, but mostly Acacia and Allocasuarina, on ironstone and greenstone hills and rises; (4) eucalypt woodlands on alluvial plains and drainage lines dominated by york gum, Eucalyptus loxophleba, but also containing stands of salmon gum E. salmonophloia and gimlet E. salubris; and (5) salt lakes margins of fringing saltmarsh dominated by samphires, Tecticornia spp.
Of these vegetation types, we focus mostly on the sandplain shrublands, which correspond to the “Joseph” and “Bannar” land systems of Payne et al. (1998) and Beard et al.’s (2013) “tall shrubland thickets” (Figure 1), because it is by far the most widespread and most fire-prone, and as it has been well studied in terms of the effects of fire on various aspects of the biota (Doherty et al., 2015, 2017; Davis et al., 2016). Fires in this system tend to be intense crown fires that remove most of the available foliage and fuel, leaving only scorched larger stems (Braun, 2006; Dalgleish et al., 2015).
Fire-Scar Mapping
Existing digitized and geo-referenced fire mapping of the study area was obtained from several sources which used Landsat imagery and available aerial photographs to detect fire scars for the period 1969–2004 (Shu et al., 2004; Braun, 2006; Parsons and Gosper, 2011). These authors used a temporal sequence of satellite imagery (Landsat TM) consisting of annual summer images, supplemented with aerial photography taken in 1980 and 1969, with burnt areas identified by a dramatic change in reflectance between temporally successive images, with local knowledge used to distinguish fire events from other types of abrupt vegetation changes. For 2005–2018, a period of very few fires in the study area, we used a combination of MODIS burn area product (Boschetti et al., 2019) and Landsat TM imagery to manually digitize fire scars, and were also guided by reports from land managers on fire incidences and their burn boundaries. For 1960–1969, we used visual interpretation of available aerial photographs which covered at least part of study area (years 1959, 1960, 1962, 1968, and 1969) to map fires and estimate the year of fire based on degree of vegetation recovery (using later aerial photography with known fire dates as a guide to estimate vegetation recovery rates for the major vegetation types). Vegetation recovery after fire is typically very slow in the study area with fire scars evident for at least 10 years in most circumstances (O’Donnell et al., 2011b). To obtain the month when individual fires occurred, we used a combination of the Firewatch website1 which displays monthly MODIS burned area maps, Landsat imagery via LandsatLook Viewer2, and historical anecdotes; however month of fire for years prior to 1982 were uncertain due to lack of resolution and sufficient time sequences, and fires prior to 1972 (before satellite imagery became available) only within the nearest 0.5–2 years. We accept that the dates assigned to fire scars detected in the period 1950–1965 are particularly uncertain and that we may have missed some smaller fires in this period given the poor resolution of available aerial photography. However, we are confident that fires from 1960 to 2018 have been accurately mapped in space and are temporally accurate from 1968 and this facilitated the analysis of spatial extent and fire history, respectively. Digitized fire-scar mapping was thoroughly checked and field validated in this and previous studies (e.g., Parsons and Gosper, 2011; Dalgleish et al., 2015; Knuckey et al., 2016; Doherty et al., 2017).
Spatial Analyses
To assess landscape-scale fire preferences, we performed spatial intersections between mapped fire scars and the five broad vegetation types within a GIS (ArcGIS v10; ESRI, 2011). The spatial area of each intersection was calculated using the GIS and then compared to total area of each vegetation type available within the study area. All layers were reprojected to the same map projection and datum before spatial analyses.
To investigate whether individual fires preferentially burned certain vegetation types over others, we calculated selection ratios for each discrete fire event in the study area, except for a few very small fires and those occurring before 1970 as individual fire events could not be always distinguished from each other. This approach is based on habitat selection principles relating consumed to available resources (Manly et al., 1993), but has been applied to fire studies by comparing the proportion of different vegetation types in a burned area (consumed) to that of the burn and surrounding area (available) (Moreira et al., 2009; O’Donnell et al., 2011b). For a given vegetation type i, the selection ratio (w) is calculated as wi = oi/πi (Manly et al., 1993), where oi is the proportion of the burned area covered by vegetation type i, and πi is the proportion of available land in the burn area and surrounding buffer occupied by vegetation type i. If a given vegetation type is burnt in exact proportion to its availability, then w = 1. If the vegetation type is burnt more than expected by chance (i.e., preferentially), then w > 1. If the vegetation is burnt less than expected by chance (i.e., avoided), then w < 1. Different authors have used different sizes and shapes of buffers (which makes direct comparisons between studies difficult); however, for the purpose of this study we followed the advice of Oliveira et al. (2014) and made buffers approximately twice as large and the same shape as the burnt area of each fire. We did this by creating buffers of a width proportional to the size of the fire scar (varying from 0.3 km for small fires to 3.5 km for our largest fires) around the perimeter of each fire and then dissolving all separate buffers to create a single buffer in ArcGIS. Mean selection ratios of vegetation types were calculated and compared using one-way ANOVA. Selection ratios were also calculated for individual fires and episodes of several large wildfires (e.g., 2000–2002) to determine if they selectively favored previously burnt or unburnt areas. To determine if fires tended to favor areas closer to edge of vegetation type rather than toward their interior, the distance to nearest vegetation edge was calculated for 1,000 random points across the study area (using the proximity tool within GIS) and then means of such distances were compared between burnt and unburnt points using t-tests for each major vegetation type.
Relationships Between Fire Occurrence and Rainfall
We adapted a widely used statistical method for exploring relationships between time-series variables called superimposed epoch analysis (SEA; Chree, 1913) to determine associations between occurrence of fire(s) in a particular year and rainfall in corresponding and preceding years (Sherriff and Veblen, 2008; O’Donnell et al., 2011a). We used the financial year (FY) rather than calendar year in this analysis as this captured the main fire season (November to May) when most fires occurred and largest areas were burned. We firstly obtained monthly rainfall data for nine evenly spaced points across the study area from SILO3 which uses spatial interpolation of available weather station data to estimate monthly rainfall for each 0.05 degree grid cell. We then summed the monthly data for each FY and averaged the nine points to obtain the study area rainfall for each FY. We then calculated the average rainfall for FYs which recorded a fire, which we refer to as “fire years” (t0; n = 21) and compared this to the overall mean FY rainfall for the region across the whole study period using standard (z-) scores and then z-tests to determine significance of anomalies in mean rainfall between fire years and all years. We then repeated the calculation of standard scores and test statistics for anomalies between the mean study area rainfall of successive years prior to fire years (t–1, t–2 and t–3) and the mean rainfall across all years, as well as for cumulative mean rainfall for current and previous years (up to 3 years prior to fire, with and without year of fire). We then repeated SEA to calculate (and test for) anomalies in mean rainfall for years of major fires (n = 5), and years of no fire (n = 33). Major fire years were defined as those with total annual burn area in excess of 50 km2 which, although arbitrarily selected, corresponded to a clear break in the annual burn area distribution, with all “minor” fire years having less than 30 km2 burnt. We used only rainfall and fire data for the period where fires could be confidently placed into a financial year (i.e., 1968–1969 to 2017–2018). In addition to SEA, we calculated Pearson’s correlation coefficients between annual burn area and annual rainfall totals for year of fire, as well as rainfall for years prior to fire (single years and cumulative totals).
Fire Intervals
We used survival analysis to estimate and compare the fire intervals of the major vegetation types of the study area using the methodology developed by Johnson and Gutsell (1994) and McCarthy et al. (2001). Using 1,000 random point locations across the study area (with minimum allowed distance between points of 1 km to reduce potential for spatial autocorrelation), we interrogated the spatial data on fire history in the GIS; if the point had experienced two fires, we then calculated the bounded (uncensored) fire interval (in years and months); if only one fire had occurred we calculated two unbounded (censored) intervals (time since fire from current, and time to fire from 1950); if no fire occurred, we assumed an interval at least 65 years (censored). We decided to use both censored and uncensored intervals in the analyses to avoid underestimation of fire intervals given they are relatively long in our study area with large parts of the study area not burnt during the study period; this follows the approach used in other similar studies in southern semi-arid Australia (O’Donnell et al., 2011b; Gibson et al., 2015). This resulted in 923 intervals which included 489 for sandplain shrublands (10.9% of which were uncensored), and 292 for eucalypt woodland (2.2% uncensored). The remaining random points were in non-vegetation areas, e.g., salt lakes. Sufficient uncensored data was only available for these two major vegetation types to undertake survival analysis. We used random points rather than calculating intervals between individual fires due to the relatively low number of discrete fires and the difficulty in distinguishing between discrete fires, especially for some pre-1970 mapped scars and years when several large fires occurred (as other have done, e.g., Gibson et al., 2015; Rogeau et al., 2016).
From these intervals, we used the survreg function in R package “survival” (Diex, 2013) to fit Weibull curves to the data and provide maximum likelihood estimates of Weibull parameters for the two major vegetation types. We then converted these to Weibull equation parameters b and c, and calculated the median Weibull fire interval using MEI = b(ln2)1/c as per Moritz et al. (2004).
Results
Landscape Fire Patterns
Of the five major types of vegetation found in the study area, fire has predominately occurred in the shrublands on sandplains (Table 1 and Figure 1), with around two thirds of this vegetation type experiencing at least one fire from the early 1960s to the end of the study period in 2018. Some 20% of these sandplain shrublands experienced more than one fire in this period (Table 1), although very few areas were burnt three or more times (<1% of this vegetation type). Although sandplains dominate the study area, especially the southern portion, accounting for about 42% of the total area, fires preferentially occur here as they account for about 84% of the total burned area (Table 1).
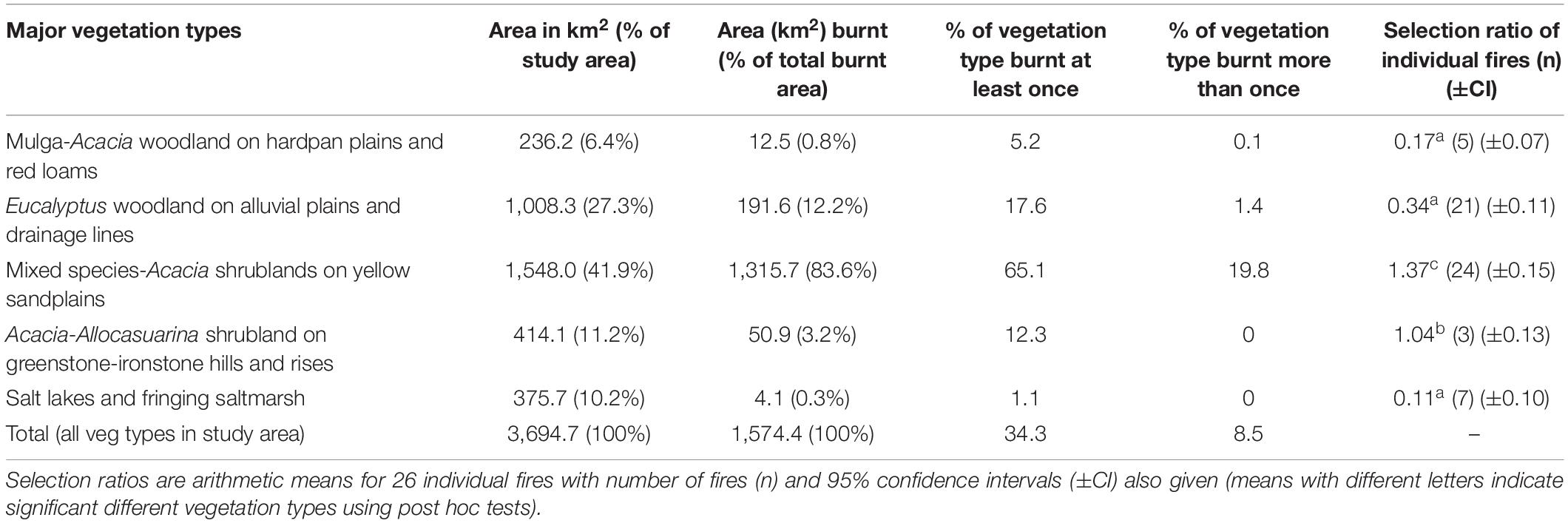
Table 1. The total area and proportion of each major vegetation type of study area burnt in the period 1960–2018, and selection ratio for individual fires.
Fires were less common in other vegetation types (Table 1) and were rare in saltmarsh and mulga vegetation, mainly occurring where such vegetation bordered sandplain shrublands (Figure 1). Fires burning in sandplain shrublands also occasionally crossed into and burnt the edges of neighboring eucalypt woodland on alluvial drainage lines and plains. However, in the very south of the study area, some fires burn right through these eucalypt woodlands patches (Figure 1). Although some 20% of the eucalypt woodlands has experienced fire in the last 50 years, almost none of these burnt areas have experienced repeated fire (Table 1).
Individual fires also showed a clear preference for sandplain shrublands burning significantly more than expected based on available area, whereas fires clearly avoided saltmarsh, eucalypt woodlands and mulga vegetation, burning well below what was available (Table 1). Individual fires in shrublands on greenstone/ironstone uplands tended to burn areas equivalent to their availability (Table 1).
Fire Re-occurrence and Patterns Within Vegetation Types
For most of the vegetation types studied, the bulk of their area remained unburnt over the ∼58 years of fire records, with very few areas experiencing more than one fire (Table 1). The clear exception was the sandplain shrublands where roughly 45% by area had experienced one fire and ∼20% two fires (Table 1). The major wildfires (by extent) in these shrublands could be grouped into two discrete 2–3 year periods (1966–1969, and 2000–2002; Figure 2). Around 66% of sandplain areas burnt in these large fires of late 1960s were burnt again in early 2000s (Table 2) and indeed fires in 2000–2002 were much more likely to occur in areas burnt in the 1966–1969 fires than areas not burnt by these fires relative to the area available (Table 2).
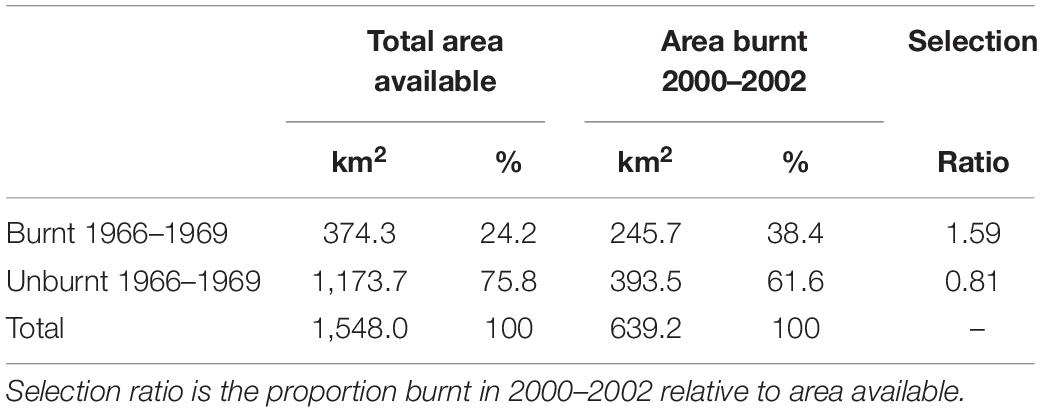
Table 2. Comparison of two periods experiencing very large wildfires in the study area (1966–1969 and 2000–2002) showing areas (and percentage) of total sandplain shrubland burnt and unburnt in 1966–1969 and the area (and percentage) of these burnt in later fires of 2000–2002.
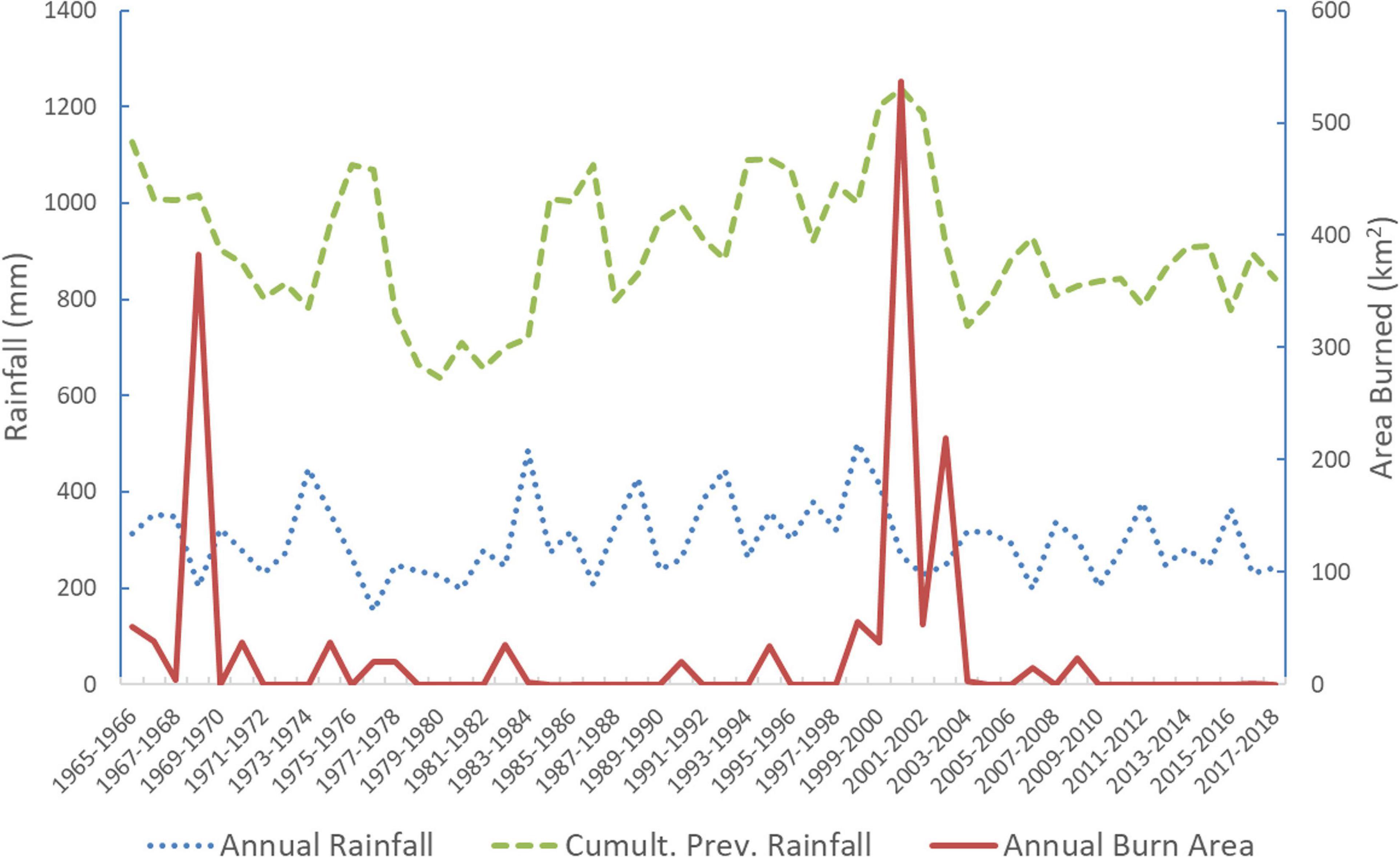
Figure 2. Relationships between annual rainfall, previous 3-year rainfall and burn area (all based on financial year). Both regional average rainfall for each financial year and cumulative 3-year rainfall (total rainfall from 1 to 3 years prior to financial year) are shown.
Of the major vegetation types, it was only in sandplain shrublands where fires were more likely to occur distant from its edge (i.e., boundary with other vegetation types) than close to its edge. Eucalypt woodland was more likely to burn in areas close to its edge, whereas for all other types no preference in terms of position of fires relative to their edges was found (Table 3).
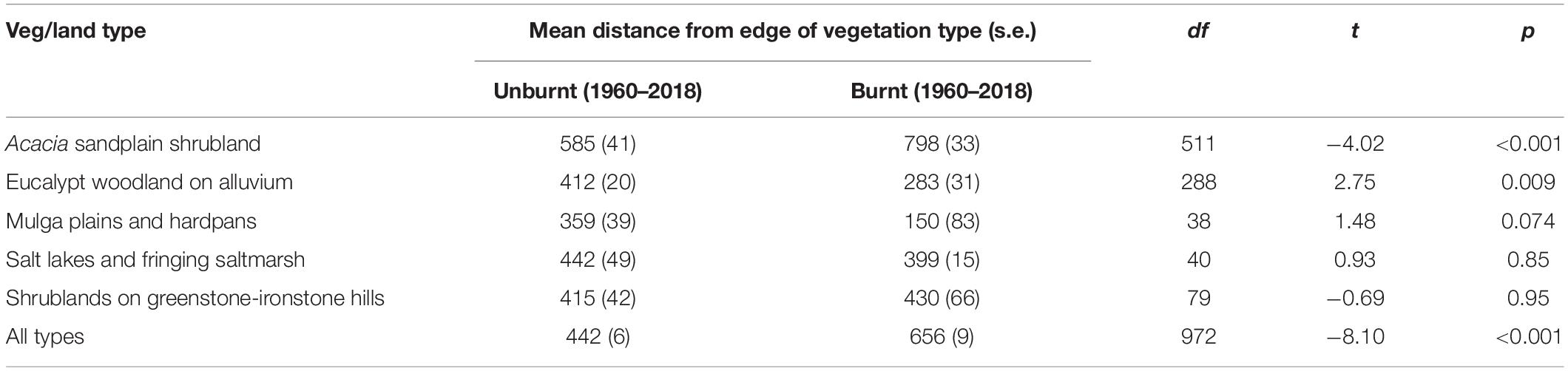
Table 3. Mean distance (m) of 1,000 randomly selected points to the nearest edge of its vegetation type for burnt and unburnt points.
Temporal Fire Patterns and Fire Intervals
Except for the aforementioned two periods 1967–1969 and 2000–2002 when several large wildfires occurred (e.g., four fires each exceeding 100 km2 occurred in December 2000, January 2001, February 2002 and December 2002), most years experienced either no fires or only relatively small fires in terms of burn area (Figure 2). Annual burn area was positively correlated with regional rainfall experienced in the second year prior to the fire year (r = 0.35), as well as 2–3 years of cumulative rainfall in years preceding the fire (i.e., not including year of fire; Table 4), whereas the (3-year) cumulative burn area was positively correlated with rainfall in the second and third years prior to fire (r = 0.36 and 0.39, respectively) and cumulative rainfall totals spanning from 2 to 4 years prior (Table 4). The strongest correlation found was between cumulative 3-year burn area and cumulative 3 years of rainfall in years prior to fire year (r = 0.52). Eucalypt woodland burn area was more strongly correlated with regional rainfall in the 2 years prior to fire year (r = 0.44) than was burn area in sandplain shrublands (r = 0.36; Table 4).
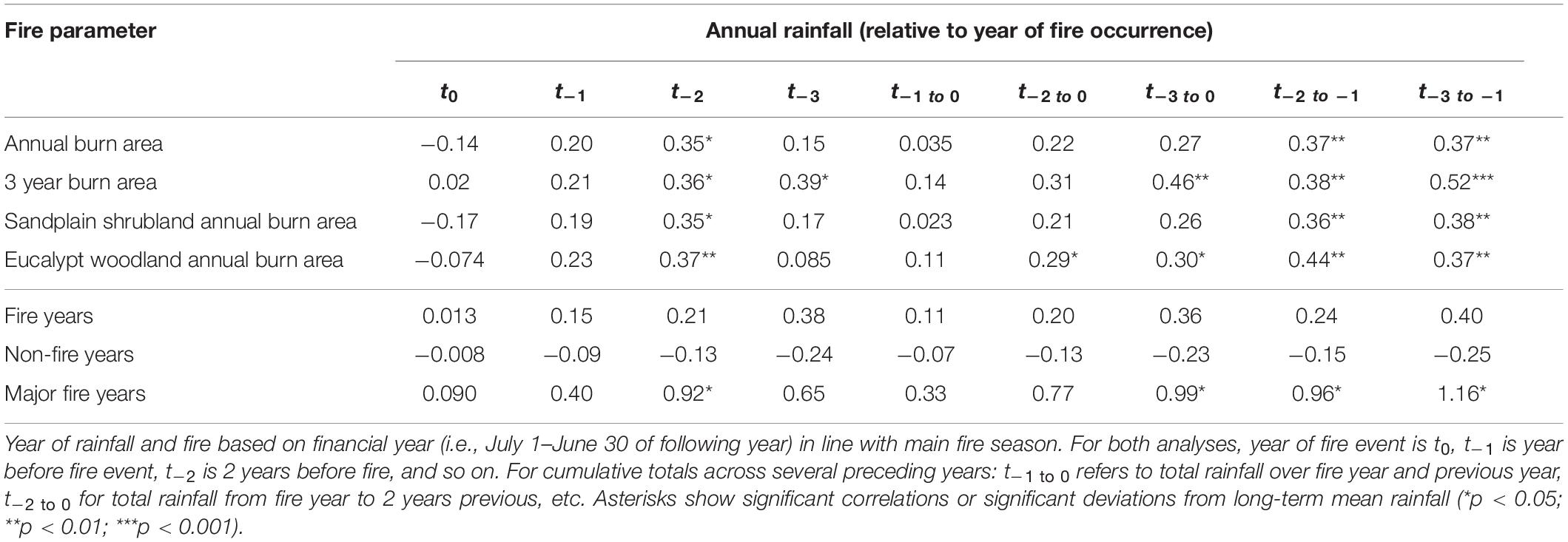
Table 4. Fire–rainfall relationships shown for single year and multi-year (cumulative) totals using (1) correlations between annual burn areas and annual rainfall (upper half of table); and (2) anomalies between mean annual rainfall associated with fire event year(s) and long-term regional averages (1968–2018) expressed as standard scores (lower half).
The time-series analyses (SEA) showed that years with major fire events were more likely to occur when the second year prior to fire year had significantly higher than average rainfall, as well when there were sequences of years with above average rainfall prior to the year of fire (Table 4). However, there were no significant deviations between average rainfall and rainfall in years with fire (of all sizes), nor in years with no fire (Table 4).
Fire interval estimation using survival analysis was only possible for the two most common vegetation types. In terms of the fitted Weibull model, parameter b was ∼124 for sandplain shrublands, which can be interpreted as the estimated fire interval (in years) that will be exceeded ∼37% of the time. This translates into an estimated median fire interval of 103 years for this vegetation type (Table 5). The estimated parameter c of 1.95 indicates an almost linear increase in fire hazard over time. In contrast, the estimated median fire interval for eucalypt woodlands was much longer (870 years although with relatively large standard error), and the parameter c estimate of 1.25 suggests closer to constant fire hazard over time (which is indicated by a value of 1; Moritz et al., 2004).
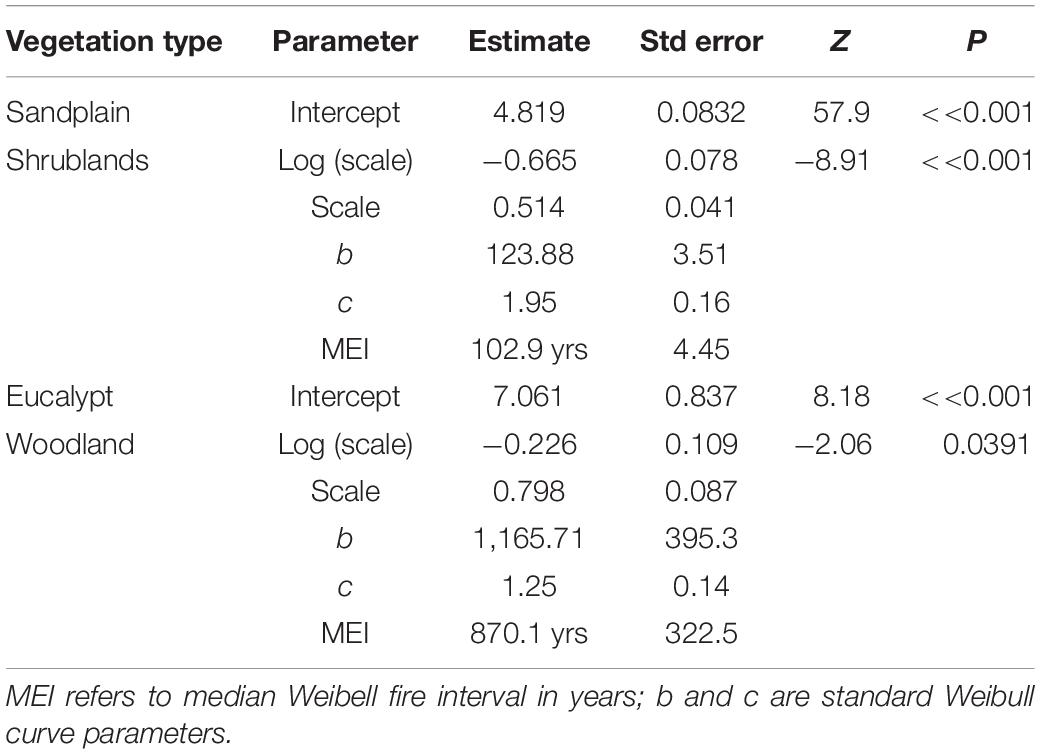
Table 5. Parameters of Weibull model fitted to fire interval data for two major vegetation types of study area.
Discussion
Landscape Fire Preferences
Our spatial analyses of fires across the landscape show wildfires clearly favor shrublands on yellow sandplains over other vegetation, with both individual fires and overall burn area preferencing this widespread vegetation type. Fires in these shrublands are typically large intense crown fires because, given sufficient time since fire, the vegetation develops dense and reasonably continuous canopies over large areas (Braun, 2006; Dalgleish et al., 2015; Kelso et al., 2015). Within these shrublands, fires tended to be non-random, preferentially burning away from edges and in areas burnt by previous large fires, suggesting some ecological memory in terms of fire pathways (Peterson, 2002; Johnstone et al., 2016). Preference for reburning of previously burnt vegetation has been reported in a range of vegetation types, and generally reflects either positive feedbacks where fires increase the flammability of vegetation, or that fire pathways are shaped by inherent landscape patterns of vegetation, topography and/or ignition sources (Pausas et al., 2017; McLauchlan et al., 2020). In a nearby region, O’Donnell et al. (2011b) found connectivity of more flammable vegetation across the landscape was critical in determining fire spread and, therefore, fire intervals, which seems to be important in our study area as well given many areas of the long-unburnt sandplain shrubland occurred in isolated patches surrounded by less flammable vegetation types, such as salt lakes/pans and woodlands (Figure 1). Preferential burning manifests itself in the uneven spatial distribution and configuration of long-unburnt patches of sandplain shrublands across our study landscape, which will be the subject of a future paper given such patches have been demonstrated to be vital habitat for certain species of birds (Davis et al., 2016), reptiles and small mammals (Doherty et al., 2015), and plants (Knuckey et al., 2016).
Other shrubland types occur in the study area but these are generally less dense and occupy relatively small and isolated areas (e.g., ironstone hills, greenstone ranges), and so experience relatively few fires (which are mostly started by lightning or by people who use existing roads and tracks network which tend to be away from uplands; Braun, 2006). Saltmarsh shrublands also experienced few fires, which is expected given the generally low flammability of the sparse and mostly succulent vegetation (van Etten and Burrows, 2018).
The second-most burnt vegetation type was eucalypt woodland (with 17% of its area burnt at least once) but, in contrast to sandplain shrublands, fires here occurred mostly near the edges, entering from adjoining vegetation types. This vegetation is sensitive to fire, with the dominant eucalypt trees tending to be killed outright when burnt, although seedling regeneration has been reported to be strong following fire, as is typical of obligate seeders (Hollenbach, 2008; Gosper et al., 2013a, 2016). Fire is rare in the other type of woodland of the study area, the mulga woodlands in the northern part of study area, which agrees with what is known for this vegetation type across arid Australia (Murphy et al., 2013; van Etten and Burrows, 2018).
Fire Intervals
Average (median) fire return intervals were estimated to be around 100 years in sandplain shrublands with fire hazard and likelihood increasing linearly with time since last fire, although well-above rainfall periods were also found to contribute to the likelihood of large fires in this vegetation. This average interval aligns with known fuel dynamics for these shrublands with total fuel loads accumulating slowly and gradually after fire for at least 50–80 years with little evidence of plateau or peaks in fuel loads over this timeframe (Dalgleish et al., 2015). Fuel accumulation is so slow and patchy across these shrublands that it is unlikely that extensive wildfires can occur again for at least 20 years, and up to 30 years, post fire, which aligns with the general paucity of areas burnt twice across our ∼50 year study period (representing only 20% of shrubland area) and almost no areas burnt three times. Most of twice-burnt areas were located in the largest continuous swathes of sandplain shrublands in the southern part of the study area, with most of these being areas burnt by major wildfires in mid-late 1960s and again by large wildfires between 2000 and 2002 (representing a 32–36 year interval). These decadal-scale fire intervals are similar to that reported for sandplain shrublands in the Lake Johnston area, some 400 km to the south-east, although within the same broad rainfall zone (O’Donnell et al., 2011b). Studies of other semi-arid shrublands have also reported linearly increasing fire hazard with time (McCaw, 1997; O’Donnell et al., 2011b; Fernandes et al., 2012), although shrublands in other generally wetter and more coastal Mediterranean-type climates, both in Australia and elsewhere, generally seem to have a weaker relationship between vegetation age and fire hazard, with fire weather seemingly more important (Moritz et al., 2004; Van Wilgen et al., 2010; Fontaine et al., 2012).
The eucalypt woodlands, in contrast, have typically very long fire intervals (estimated at 870 years on average, although highly variable) with only minor increases in fire hazard over time and a slightly stronger link between above-average rainfall and fire occurrence. This concords with other studies of valley-floor eucalypt woodlands in the broader “transitional-rainfall” region of Western Australia in terms of their very long fire intervals, low fuel levels and accumulation rates, and the importance of large rainfall events in promoting fires (O’Donnell et al., 2011b; Gosper et al., 2013a,b), characteristics which Gosper et al. (2016) argue separate them from other eucalypt woodland ecosystems of Australia, such as savanna and temperate grassy woodlands.
Fire Drivers
Large deluges of rain, or sustained periods of above-average rainfall, are likely to temporally increase fuel levels and connectivity in the eucalypt woodlands through promotion of annual plants, particularly grasses (O’Donnell et al., 2011a), which is a common driver of fire occurrence in many arid ecosystems (Balch et al., 2013; Gibson et al., 2015; van Etten and Burrows, 2018). Importantly, fire was most closely associated with rain 2 years before fire, which suggests that a period of drying and curing of annual plants is required before fuels become flammable. This explains fire traveling through large patches of eucalypt woodland around 1–3 years following the period May 1998–April 2000 during which over twice the usual rainfall occurred in the study area, which corresponds to one of the wettest periods in inland southern Western Australia for 200 years (O’Donnell et al., 2018). In most other circumstances, fire only traveled into these woodlands (from shrublands) for a short distance at their edge, which could reflect transitional fuel configurations and/or fire weather conditions at edges (Gartner et al., 2012; Driscoll et al., 2021). More research is needed to quantify fuel levels of eucalypt woodlands, both at edges and in the interior, and especially following wet periods, to confirm that rainfall is a major driver of fires via temporary fuel enhancement. Further, many eucalypt woodlands in the study area were grazed by sheep until about 2003, which may have played a role in suppressing fuel levels up to this time.
Fire occurrence in sandplain shrublands was also positively associated with rainfall before fire events, although correlations were more modest. These shrublands lack grasses, and their high density/cover tends to discourage ground annuals, so wet episodes may stimulate shrub growth and continuity, thereby promoting fire. This finding, however, is at odds with that reported for many other dense shrubland ecosystems where drought has been reported to promote crown fires through lowering fuel moisture and increasing the dead fuel component (Keeley et al., 2012; Turco et al., 2017). Ladbrook et al. (2018) found positive associations between pre-fire rainfall and fire extent in more open Acacia shrublands in arid Western Australia (some 500 km north-west of our study area) with most burning occurring in the years after an extremely wet period in that region. Indeed, across Australia’s arid zone, fire activity is strongly linked to antecedent rainfall (Turner et al., 2008), as we report here. It may be that major wet-dry cycles are critical in promoting fires, as has been reported in some arid ecosystems (Balch et al., 2013), and more analysis is recommended to explore relationships between fire and climatic fluctuations. Although we found a negative correlation between fire extent and rainfall in the fire year, it was not statistically significant. Further, the role of fire weather (temperature, humidity and wind speed during and in days preceding the fire) in driving fire spread needs further exploration given its importance in other shrubland ecosystems (Keeley et al., 2012; Clarke et al., 2014; Keeley and Syphard, 2019); unfortunately such pre-fire weather data was absent for the vast majority of our mapped fire scars.
Conclusion and Management Implications
Studies of fire regimes and their drivers typically seek generalizations based on climate or region (e.g., Rodrigues et al., 2019). Much of our study landscape is dominated by a complex mosaic of two distinct vegetation types which were found to have highly contrasting fire regimes—one a dense shrubland prone to intense crown fires at relatively modest intervals, although with strongly developed adaptations to regenerate after fire (via both seedlings and resprouting; Knuckey et al., 2016); the other a low-fuel woodland ecosystem dominated by a fire-sensitive obligate seeder, where fires are very rare and limited mostly to edges. Such divergent fire regimes and preferential burning patterns have been reported across many other arid and semi-arid landscapes in Australia (Nicholas et al., 2011; van Etten and Burrows, 2018) and elsewhere (Heyerdahl et al., 2001; McLauchlan et al., 2020).
Fire drivers operating in each of our two major vegetation types also appear to be different in some respects, albeit both show a clear positive response to pre-fire rainfall. In the shrubland, fire age is important in shaping fire hazard, albeit over decadal scales. However, the role of rainfall in driving fire occurrence and hazard is more clear-cut in woodlands as fuels tend to remain low and patchy, and do not accumulate except following ample rainfall. These woodlands, therefore, subscribe to the fuel-limited model of fire regimes characteristic of arid lands (Pausas and Ribeiro, 2013). Drivers of shrubland fires in our study area, on the other hand, are only fuel limited during recovery following wildfire (which is relatively slow), but not when mature. However, there is no strong evidence that fire in mature shrubland is drought-driven as is the case with shrubland ecosystems from more productive (mesic) climates (Pausas and Ribeiro, 2013).
Woodland and shrublands ecosystems are often adjoining each other in our study area so the critical ecological question is how do the such disparate fire regimes develop and persist in proximity? Fundamentally, the vegetation patterning across the landscape is an expression of the mosaic of different soil and landform types (specifically sandplain and alluvial drainage systems for these two main vegetation types, respectively). The ecotone between these two vegetation types is of particular interest as this is where vegetation properties will intergrade and where fire is most likely to cross-over from shrubland and enter woodland ecosystems (Gartner et al., 2012). Ecotones between fire-prone and fire sensitive vegetation can be dynamic, changing with fire history, and can be challenging to manage (Nicholas et al., 2011; Just et al., 2016). However, they also provide opportunities to protect fire sensitive vegetation through active fuel management or clearing of fire breaks located near edges (Parks et al., 2015). Management should aim to limit or constrain large summer wildfires in sandplain shrublands, especially as they can burn into fire sensitive woodland and other vegetation types, and also reduce the extent of long unburnt shrubland habitat that is so vital for certain species of flora and fauna in our study area.
Data Availability Statement
The raw data supporting the conclusions of this article will be made available by the authors, without undue reservation.
Author Contributions
EE, TD, and RD wrote and edited the manuscript. EE analyzed the data. All authors contributed to the article and approved the submitted version.
Funding
TD was supported by the Discovery Early Career Research Award from the Australian Research Council (DE200100157).
Conflict of Interest
The authors declare that the research was conducted in the absence of any commercial or financial relationships that could be construed as a potential conflict of interest.
Acknowledgments
We are grateful to Bush Heritage Australia for providing data and advice which greatly assisted us in the analyses, and to for both Bush Heritage Australia and Australian Wildlife Conservancy for access to their property and facilities.
Footnotes
- ^ http://firewatch-pro.landgate.wa.gov.au/
- ^ https://landsatlook.usgs.gov/
- ^ https://www.longpaddock.qld.gov.au/silo
References
Allan, G. E., and Southgate, R. I. (2002). “Fire regimes in the spinifex landscapes of Australia,” in Flammable Australia: The Fire Regimes and Biodiversity of a Continent, eds R. A. Bradstock, J. E. Williams, and A. M. Gill (Cambridge: Cambridge University Press), 145–176.
Archibald, S., Roy, D. P., van Wilgen, B. W., and Scholes, R. J. (2009). What limits fire? An examination of drivers of burnt area in Southern Africa. Global Change Biol. 15, 613–630. doi: 10.1111/j.1365-2486.2008.01754.x
Avitabile, S. C., Callister, K. E., Kelly, L. T., Haslem, A., Fraser, L., Nimmo, D. G., et al. (2013). Systematic fire mapping is critical for fire ecology, planning and management: case study in the semi-arid Murray Mallee, south-eastern Australia. Landscape Urban Plan. 117, 81–91. doi: 10.1016/j.landurbplan.2013.04.017
Balch, J. K., Bradley, B. A., D’Antonio, C. M., and Gómez-Dans, J. (2013). Introduced annual grass increases regional fire activity across the arid western USA (1980-2009). Global Change Biol. 19, 173–183. doi: 10.1111/gcb.12046
Beard, J. S., Beeston, G. R., Harvey, J. M., Hopkins, A. J. M., and Shepherd, D. P. (2013). The Vegetation of Western Australia at the 1: 3,000,000 Scale. Explanatory Memoir, 2nd Edn, Vol. 9. Perth, WA: Conservation Science Western Australia, 1–152.
Boschetti, L., Roy, D. P., Giglio, L., Huang, H., Zubkova, M., and Humber, M. L. (2019). Global validation of the collection 6 MODIS burned area product. Remote Sens. Environ. 235:111490. doi: 10.1016/j.rse.2019.111490
Bowman, D. M., Balch, J. K., Artaxo, P., Bond, W. J., Carlson, J. M., Cochrane, M. A., et al. (2009). Fire in the Earth system. Science 324, 481–484. doi: 10.1126/science.1163886
Burrows, N. D., and Christensen, P. E. S. (1990). “A survey of Aboriginal fire patterns in the Western Desert of Australia,” in Fire and the Environment: Ecological and Cultural Perspectives, Proceedings of an International Symposium, Knoxville, Tennessee, eds S. C. Nodvin and A. W. Thomas (Asheville, NC: USDA Forest Service, Southeastern Forest Experiment Station), 297–305.
Callister, K. E., Griffioen, P. A., Avitabile, S. C., Haslem, A., Kelly, L. T., Kenny, S. A., et al. (2016). Historical maps from modern images: using remote sensing to model and map century-long vegetation change in a fire-prone region. PLoS One 11:e0150808. doi: 10.1371/journal.pone.0150808
Chree, C. (1913). Some phenomena of sunspots and of terrestrial magnetism at Kew Observatory. Philos. T. Roy. Soc. A 212, 75–116.
Clarke, P. J., Knox, K. J., Bradstock, R. A., Munoz-Robles, C., and Kumar, L. (2014). Vegetation, terrain and fire history shape the impact of extreme weather on fire severity and ecosystem response. J. Veg. Sci. 25, 1033–1044. doi: 10.1111/jvs.12166
Dalgleish, S. A., van Etten, E. J. B., Stock, W. D., and Knuckey, C. (2015). Fuel dynamics and vegetation recovery after fire in a semiarid Australian shrubland. Int. J. Wildland Fire 24, 613–623. doi: 10.1071/WF14128
Davis, R. A., Doherty, T. S., van Etten, E. J., Radford, J. Q., Holmes, F., Knuckey, C., et al. (2016). Conserving long unburnt vegetation is important for bird species, guilds and diversity. Biodivers. Conserv. 25, 2709–2722. doi: 10.1007/s10531-016-1196-5
Diex, D. M. (2013). Survival Analysis in R. Available online at: https://www.openintro.org/download.php?file=survival_analysis_in_R&referrer=/stat/surv.php (accessed March 15, 2020).
Doherty, T. S., Davis, R. A., van Etten, E. J. B., Collier, N., and Krawiec, J. (2015). Response of a shrubland mammal and reptile community to a history of landscape-scale wildfire. Int. J. Wildland Fire 24, 534–543. doi: 10.1071/WF14115
Doherty, T. S., van Etten, E. J. B., Davis, R. A., Knuckey, C., Radford, J. Q., and Dalgleish, S. A. (2017). Ecosystem responses to fire: identifying cross-taxa contrasts and complementarities to inform management strategies. Ecosystems 20, 872–884. doi: 10.1007/s10021-016-0082-z
Driscoll, D. A., Armenteras, D., Bennett, A., Brotons, L., Clarke, M., Doherty, T. S., et al. (2021). How fire interacts with habitat loss and fragmentation. Biol. Rev. 96, 976–988. doi: 10.1111/brv.12687
Driscoll, D. A., Lindenmayer, D. B., Bennett, A. F., Bode, M., Bradstock, R. A., Cary, G. J., et al. (2010). Fire management for biodiversity conservation: key research questions and our capacity to answer them. Biol. Conserv. 143, 1928–1939. doi: 10.1016/j.biocon.2010.05.026
Fernandes, P. M., Loureiro, C., Magalhães, M., Ferreira, P., and Fernandes, M. (2012). Fuel age, weather and burn probability in Portugal. Int. J. Wildland Fire 21, 380–384. doi: 10.1071/WF10063
Fontaine, J. A., Westcott, V. C., Enright, N. J., Lade, J. C., and Miller, B. P. (2012). Fire behaviour in south-western Australian shrublands: evaluating the influence of fuel age and fire weather. Int. J. Wildland Fire 21, 385–395. doi: 10.1071/WF11065
Gartner, M. H., Veblen, T. T., Sherriff, R. L., and Schoennagel, T. L. (2012). Proximity to grasslands influences fire frequency and sensitivity to climate variability in ponderosa pine forests of the Colorado Front Range. Int. J. Wildland Fire 21, 562–571. doi: 10.1071/WF10103
Gibson, R. K., Bradstock, R. A., Penman, T., Keith, D. A., and Driscoll, D. A. (2015). Climatic, vegetation and edaphic influences on the probability of fire across mediterranean woodlands of south−eastern Australia. J. Biogeogr. 42, 1750–1760. doi: 10.1111/jbi.12547
Gosper, C. R., Prober, S. M., and Yates, C. J. (2013a). Multi-century changes in vegetation structure and fuel availability in fire-sensitive eucalypt woodlands. Forest Ecol. Manag. 310, 102–109. doi: 10.1016/j.foreco.2013.08.005
Gosper, C. R., Prober, S. M., and Yates, C. J. (2016). Continental−scale syntheses of Australian pyromes – misclassification of south−western eucalypt woodlands misinforms management. J. Biogeogr. 43, 858–861. doi: 10.1111/jbi.12693
Gosper, C. R., Prober, S. M., Yates, C. J., and Wiehl, G. (2013b). Estimating the time since fire of long-unburnt Eucalyptus salubris (Myrtaceae) stands in the Great Western Woodlands. Aust. J. Bot. 61, 11–21. doi: 10.1071/BT12212
He, T., Lamont, B. B., and Pausas, J. G. (2019). Fire as a key driver of Earth’s biodiversity. Biol. Rev. 94, 1983–2010. doi: 10.1111/brv.12544
Heyerdahl, E. K., Brubaker, L. B., and Agee, J. K. (2001). Spatial controls of historical fire regimes: a multiscale example from the interior west, USA. Ecology 82, 660–678.
Hollenbach, M. B. (2008). Fire Ecology and Management of Eucalyptus loxophleba Woodlands in the Northern Wheatbelt of Western Australia. diploma thesis. Hanover: Institute of Geobotany & Institute of Environmental Planning, Leibniz University of Hannover.
Hopper, S. D., and Gioia, P. (2004). The southwest Australian floristic region: evolution and conservation of a global biodiversity hotspot. Annu. Rev. Ecol. Syst. 35, 623–650. doi: 10.1146/annurev.ecolsys.35.112202.130201
Johnson, E. A., and Gutsell, S. L. (1994). “Fire frequency models, methods and interpretations,” in Advances in Ecological Research, Vol. 25, eds M. Begon and A. H. Fitter (London: Academic Press), 239–287.
Johnstone, J. F., Allen, C. D., Franklin, J. F., Frelich, L. E., Harvey, B. J., Higuera, P. E., et al. (2016). Changing disturbance regimes, ecological memory, and forest resilience. Front. Ecol. Environ. 14:369–378. doi: 10.1002/fee.1311
Just, M. G., Hohmann, M. G., and Hoffmann, W. A. (2016). Where fire stops: vegetation structure and microclimate influence fire spread along an ecotonal gradient. Plant Ecol. 217, 631–644. doi: 10.1007/s11258-015-0545-x
Kane, V. R., Lutz, J. A., Cansler, C. A., Povak, N. A., Churchill, D. J., Smith, D. F., et al. (2015). Water balance and topography predict fire and forest structure patterns. Forest Ecol. Manag. 338, 1–13. doi: 10.1016/j.foreco.2014.10.038
Keeley, J. E., Bond, W. J., Bradstock, R. A., Pausas, J. G., and Rundel, P. W. (2012). Fire in Mediterranean Ecosystems: Ecology, Evolution and Management. Cambridge: Cambridge University Press.
Keeley, J. E., and Syphard, A. D. (2019). Twenty-first century California, USA, wildfires: fuel-dominated vs. wind-dominated fires. Fire Ecol. 15, 24. doi: 10.1186/s42408-019-0041-0
Kelso, J. K., Mellor, D., Murphy, M. E., and Milne, G. J. (2015). Techniques for evaluating wildfire simulators via the simulation of historical fires using the Australis simulator. Int. J. Wildland Fire 24, 784–797. doi: 10.1071/WF14047
Knuckey, C. G., van Etten, E. J., and Doherty, T. S. (2016). Effects of long−term fire exclusion and frequent fire on plant community composition: a case study from semi−arid shrublands. Aust. Ecol. 41, 964–975. doi: 10.1111/aec.12388
Ladbrook, M., van Etten, E. J. B., and Stock, W. D. (2018). Contemporary fire regimes of the arid Carnarvon Basin region of Western Australia. Fire 1:51. doi: 10.3390/fire1030051
Manly, B., McDonald, L. L., and Thomas, D. L. (1993). Resource Selection by Animals: Statistical Design and Analysis for Field Studies. London: Chapman and Hall.
McCarthy, M. A., Gill, A. M., and Bradstock, R. (2001). Theoretical fire-interval distributions. Int. J. Wildland Fire 10, 73–77. doi: 10.1071/WF01013
McCaw, W. L. (1997). Predicting Fire Spread in Western Australian Mallee-Heath Shrubland. Ph.D thesis. Canberra: University College, University of New South Wales.
McKenzie, D., and Littell, J. S. (2017). Climate change and the eco−hydrology of fire: will area burned increase in a warming western USA? Ecol. Appl. 27, 26–36. doi: 10.1002/eap.1420
McLauchlan, K. K., Higuera, P. E., Miesel, J., Rogers, B. M., Schweitzer, J., Shuman, J. K., et al. (2020). Fire as a fundamental ecological process: research advances and frontiers. J. Ecol. 108, 2047–2069. doi: 10.1111/1365-2745.13403
Moreira, F., Vaz, P., Catry, F., and Silva, J. S. (2009). Regional variations in wildfire susceptibility of land-cover types in Portugal: implications for landscape management to minimize fire hazard. Int. J. Wildland Fire 18, 563–574. doi: 10.1071/WF07098
Moritz, M. A., Keeley, J. E., Johnson, E. A., and Schaffner, A. A. (2004). Testing a basic assumption of shrubland fire management: how important is fuel age? Front. Ecol. Environ. 2:67–72.
Murphy, B. P., Bradstock, R. A., Boer, M. M., Carter, J., Cary, G. J., Cochrane, M. A., et al. (2013). Fire regimes of Australia: a pyrogeographic model system. J. Biogeogr. 40, 1048–1058. doi: 10.1111/jbi.12065
Nicholas, A. M. M., Franklin, D. C., and Bowman, D. M. J. S. (2011). Floristic uniformity across abrupt boundaries between Triodia hummock grassland and Acacia shrubland on an Australian desert sandplain. J. Arid Environ. 75, 1090–1096. doi: 10.1016/j.jaridenv.2011.06.016
O’Donnell, A. J., Boer, M. M., McCaw, W. L., and Grierson, P. F. (2011b). Vegetation and landscape connectivity control wildfire intervals in unmanaged semi−arid shrublands and woodlands in Australia. J. Biogeogr. 38, 112–124. doi: 10.1111/j.1365-2699.2010.02381.x
O’Donnell, A. J., Boer, M. M., McCaw, W. L., and Grierson, P. F. (2011a). Climatic anomalies drive wildfire occurrence and extent in semi−arid shrublands and woodlands of southwest Australia. Ecosphere 2, 1–15. doi: 10.1890/ES11-00189.1
O’Donnell, A. J., Cook, E. R., Palmer, J. G., Turney, C. S., and Grierson, P. F. (2018). Potential for tree rings to reveal spatial patterns of past drought variability across western Australia. Environ. Res. Lett. 13:024020. doi: 10.1088/1748-9326/aaa204
Oliveira, S., Moreira, F., Boca, R., San-Miguel-Ayanz, J., and Pereira, J. M. (2014). Assessment of fire selectivity in relation to land cover and topography: a comparison between Southern European countries. Int. J. Wildland Fire 23, 620–630. doi: 10.1071/WF12053
Parks, S. A., Holsinger, L. M., Miller, C., and Nelson, C. R. (2015). Wildland fire as a self−regulating mechanism: the role of previous burns and weather in limiting fire progression. Ecol. Appl. 25, 1478–1492. doi: 10.1890/14-1430.1
Parsons, B. C., and Gosper, C. R. (2011). Contemporary fire regimes in a fragmented and an unfragmented landscape: implications for vegetation structure and persistence of the fire-sensitive malleefowl. Int. J. Wildland Fire 20, 184–194. doi: 10.1071/WF09099
Pausas, J. G., and Bradstock, R. A. (2007). Fire persistence traits of plants along a productivity and disturbance gradient in mediterranean shrublands of south-east Australia. Global Ecol. Biogeogr. 16, 330–340. doi: 10.1111/j.1466-8238.2006.00283.x
Pausas, J. G., Keeley, J. E., and Schwilk, D. W. (2017). Flammability as an ecological and evolutionary driver. J. Ecol. 105, 289–297. doi: 10.1111/1365-2745.12691
Pausas, J. G., and Paula, S. (2012). Fuel shapes the fire-climate relationship: evidence from Mediterranean ecosystems. Global Ecol. Biogeogr. 21, 1074–1082. doi: 10.1111/j.1466-8238.2012.00769.x
Pausas, J. G., and Ribeiro, E. (2013). The global fire–productivity relationship. Global Ecol. Biogeogr. 22, 728–736. doi: 10.1111/geb.12043
Payne, A. L., Van Vreeswyk, A. M. E., Leighton, K. A., Pringle, H. J., and Hennig, P. (1998). An Inventory and Condition Survey of the Sandstone-Yalgoo-Paynes Find area, Western Australia. Technical Bulletin No. 90. Western Australia: Department of Agriculture and Food.
Peterson, G. D. (2002). Contagious disturbance, ecological memory, and the emergence of landscape pattern. Ecosystems 5, 329–338. doi: 10.1007/s10021-001-0077-1
Rodrigues, M., Costafreda-Aumedes, S., Comas, C., and Vega-García, C. (2019). Spatial stratification of wildfire drivers towards enhanced definition of large-fire regime zoning and fire seasons. Sci. Total Environ. 689, 634–644. doi: 10.1016/j.scitotenv.2019.06.467
Rogeau, M. P., Flannigan, M. D., Hawkes, B. C., Parisien, M. A., and Arthur, R. (2016). Spatial and temporal variations of fire regimes in the Canadian Rocky Mountains and Foothills of southern Alberta. Int. J. Wildland Fire 25, 1117–1130. doi: 10.1071/WF15120
Schoennagel, T., Smithwick, E. A., and Turner, M. G. (2009). Landscape heterogeneity following large fires: insights from Yellowstone National Park, USA. Int. J. Wildland Fire 17, 742–753. doi: 10.1071/WF07146
Sherriff, R. L., and Veblen, T. T. (2008). Variability in fire–climate relationships in ponderosa pine forests in the Colorado Front Range. Int. J. Wildland Fire 17, 50–59. doi: 10.1071/WF07029
Shu, S., Metcalfe, G., Müller, C., and Cann, P. (2004). “Application of remote sensing in fire management in Western Australia,” in Proceedings of the Australasian Fire Authorities Annual Conference, 7-9 October 2004, Perth, WA.
Turco, M., von Hardenberg, J., AghaKouchak, A., Llasat, M. C., Provenzale, A., and Trigo, R. M. (2017). On the key role of droughts in the dynamics of summer fires in Mediterranean Europe. Sci. Rep. 7:81. doi: 10.1038/s41598-017-00116-9
Turner, D., Ostendorf, B., and Lewis, M. (2008). An introduction to patterns of fire in arid and semi-arid Australia, 1998-2004. Rangeland J. 30, 95–107. doi: 10.1071/RJ07039
Turner, M. G., Hargrove, W. W., Gardner, R. H., and Romme, W. H. (1994). Effects of fire on landscape heterogeneity in Yellowstone National Park. Wyoming. J. Veg. Sci. 5, 731–742. doi: 10.2307/3235886
Turner, M. G., and Romme, W. H. (1994). Landscape dynamics in crown fire ecosystems. Landscape Ecol. 9, 59–77. doi: 10.1007/BF00135079
van Etten, E. J. B., and Burrows, N. D. (2018). “Fire regimes and ecology of arid Australia,” in On the Ecology of Australia’s Arid Zone, ed. H. Lambers (Dordrecht: Springer), 243–282. doi: 10.1007/978-3-319-93943-8_10
Van Wilgen, B. W., Forsyth, G. G., de Klerk, H., Das, S., Khuluse, S., and Schmitz, P. (2010). Fire management in Mediterranean-climate shrublands: a case study from the Cape fynbos, South Africa. J. Appl. Ecol. 47, 631–638. doi: 10.1111/j.1365-2664.2010.01800.x
Keywords: fire regime, spatial patterns, semi-arid, fire drivers, fire ecology
Citation: van Etten EJB, Davis RA and Doherty TS (2021) Fire in Semi-Arid Shrublands and Woodlands: Spatial and Temporal Patterns in an Australian Landscape. Front. Ecol. Evol. 9:653870. doi: 10.3389/fevo.2021.653870
Received: 15 January 2021; Accepted: 20 May 2021;
Published: 15 June 2021.
Edited by:
Mauro Fois, University of Cagliari, ItalyReviewed by:
Rebecca Gibson, Environment, Energy, and Science, NSW Department of Planning, Industry and Environment, AustraliaMahmoud Bayat, Research Institute of Forests and Rangelands, Iran
Copyright © 2021 van Etten, Davis and Doherty. This is an open-access article distributed under the terms of the Creative Commons Attribution License (CC BY). The use, distribution or reproduction in other forums is permitted, provided the original author(s) and the copyright owner(s) are credited and that the original publication in this journal is cited, in accordance with accepted academic practice. No use, distribution or reproduction is permitted which does not comply with these terms.
*Correspondence: Eddie J. B. van Etten, e.van_etten@ecu.edu.au