Socioecology of the Australian Tree Skink (Egernia striolata)
- 1Department of Biological Sciences, Macquarie University, Sydney, NSW, Australia
- 2Division of Ecology and Evolution, Research School of Biology, The Australian National University, Canberra, ACT, Australia
- 3School of Natural Sciences, University of Tasmania, Hobart, TAS, Australia
- 4School of Psychology and Neuroscience, University of St. Andrews, St. Andrews, United Kingdom
There is great diversity in social behavior across the animal kingdom. Understanding the factors responsible for this diversity can help inform theory about how sociality evolves and is maintained. The Australian Tree Skink (Egernia striolata) exhibits inter- and intra-population variability in sociality and is therefore a good system for informing models of social evolution. Here, we conducted a multi-year study of a Tree Skink population to describe intra-population variation in the social organization and mating system of this species. Skinks aggregated in small groups of 2–5 individuals, and these aggregations were typically associated with shared shelter sites (crevices and hollows within rocks and trees). Aggregations were typically made up of one or more adult females and, often, one male and/or juvenile(s). Social network and spatial overlap analyses showed that social associations were strongly biased toward kin. Tree skinks also exhibited high site fidelity regardless of age or sex. There were high levels of genetic monogamy observed with most females (87%) and males (68%) only breeding with a single partner. Our results indicate that Tree Skinks reside in small family groups and are monogamous, which corresponds with existing research across populations. Similar to previous work, our study area consisted of discrete habitat patches (i.e., rock outcrops, trees, or both), which likely limits offspring dispersal and promotes social tolerance between parents and their offspring. Our study clearly demonstrates that there is intra-population variability in Tree Skink social behavior, but it also provides evidence that there is a high degree of inter-population consistency in sociality across their geographic range. We also highlight promising possible avenues for future research, specifically discussing the importance of studying the nature and extent of Tree Skink parental care and quantifying the fitness outcomes of kin-based sociality in this species, which are topics that will further our understanding of the mechanisms underlying variation in vertebrate social behavior.
Introduction
In a broad sense, animal sociality is the extent that conspecifics interact with one another and the complexity of these social interactions (i.e., the number of conspecifics in a social group, the nature of interactions, as well as their mating and care systems) (Kappeler, 2019). The extent of sociality itself varies considerably within and between individuals (i.e., social plasticity or competence as per Taborsky and Oliveira, 2012), among populations, and across taxa (Ward and Webster, 2016). There are many potential drivers of this social variation. For example, variation in resources (e.g., shelters, food, mates) may cause animals to group together for access (Graves and Duvall, 1995; Hatchwell and Komdeur, 2000). If, in turn, individuals residing in groups experience higher fitness, then selection favors sociality (Ward and Webster, 2016). Limited resources may also delay offspring dispersal from their natal social group, and association with their parents may offer protection, thus selecting for the formation of kin-based sociality and parental care (Halliwell et al., 2017). Ultimately, if we are to understand how the spectrum of animal sociality evolved, we need to connect the processes that underpin variation in social behavior at the level of the individual with patterns of social diversification we see at the population and species level. Further, understanding the factors that mediate this variation in sociality, as well as how this sets the stage for the evolutionary origins of sociality, are fundamental goals in animal behavior and evolutionary biology.
The majority of our insights into these processes are based on studies that have focused on a narrow range of species (e.g., group-living mammals like the African Striped Mouse, Rhabdomys pumilio, Mallarino et al., 2019; birds like the Acorn Woodpecker, Melanerpes formicivorus, Stacey and Ligon, 1987; and eusocial insects, Jeanson and Weidenmüller, 2014). As a result, research on social evolution has largely progressed within particular taxonomic silos (Elgar, 2015). In contrast, a range of other taxonomic groups have largely been ignored. This is particularly the case for reptiles (Bonnet et al., 2002; Rosenthal et al., 2017). The occurrence of conspecific aggregations has been investigated in less than 1% of all squamate reptiles (Gardner et al., 2016) with studies describing the composition, stability and genetic structure of social groups even rarer (i.e., testing for the presence of family-living; Whiting and While, 2017). One of the reasons for this is that reptile sociality tends to be less obvious than that observed in other social organisms. However, recent advances in spatial ecology and molecular techniques are providing new opportunities to study kin-based sociality in reptiles. While the Australian Egernia-group of skinks have been the “poster child” of family living in lizards (Chapple, 2003; While et al., 2015), it has also been identified in other lizards too (Xantusia vigilis: Davis et al., 2011; Liolaemus leopardinus: Brito, 2017). The sociality observed in these lizards is generally consistent with definitions of family-living; revolving around a stable association between parents(s) and their offspring after hatching or birth (Kramer and Meunier, 2019). In reptiles, kin associations can extend to other forms of aggregation too, like between juveniles (e.g., crèches in hatchling Iguana iguana, Burghardt et al., 1977) and between adults (e.g., the lizard Intellagama lesueurii, Piza-Roca et al., 2019; and the snake Crotalus horridus, Clark et al., 2012), but due to the lack of basic information regarding reptile sociality, we have a poor understanding of how social behavior and sociality varies both within and among individuals and populations, respectively.
The Australian Tree Skink (Egernia striolata) is reported to be family-living across its range in south-eastern Australia (Bustard, 1970; Swanson, 1976; Ehmann, 1992; Bonnett, 1999; Derez, 2004; Michael et al., 2010; Duckett et al., 2012). However, Tree Skink sociality has been suggested to vary considerably both within and between populations, and, thus, sociality of this species has been described as facultative (Chapple, 2003; Riley, 2017; Whiting and While, 2017). For example, group composition of Tree Skinks varies across populations in New South Wales in association with key microhabitat features (e.g., number of crevices, crevice dimensions, and height of home sites) (Michael et al., 2010). This has led researchers to hypothesize that forested habitats that have an abundance of shelter sites (i.e., crevices and hollows) reduces aggregation tendency in this species, and saxicolous habitats that have limited shelter sites increases Tree Skink grouping behavior. However, other research has suggested that individuals associate independently of crevice site availability in both forest and rock habitats (Bonnett, 1999; Duckett et al., 2012). Yet, it is unclear where this inter-population variation in Tree Skink sociality arises from. Most of the previous studies on this species were anecdotal in nature and/or lacked detailed data relating to the complexity of this species’ sociality within populations. Further, differences in study methodologies may also be the source of some of this variation (e.g., sampling intensity and method, differences in detectability between observers, and error rates of molecular data). An alternative explanation is that the inter-population variation in sociality that has been observed simply reflects a high degree of within and among individual variation in social behavior. This last hypothesis is what our study aims to investigate. Thus, to gain a comprehensive understanding of the complexity of Tree Skink sociality, we conducted a 2-year socioecological study of a Tree Skink population within a mixed habitat (i.e., rock outcrop and forest) that aimed to:
1. Describe Tree Skink habitat use and fidelity,
2. Quantify the demographics, size, and stability of aggregations (i.e., social organization as per Kappeler, 2019),
3. Test for sex-biased dispersal,
4. Describe the Tree Skink mating system through parentage assignment, and
5. Study the nature of Tree Skink social associations and test whether they are influenced by kinship (i.e., social structure as per Kappeler, 2019).
To achieve these aims we conducted a mark-recapture study and collected data on individual social behavior and space use. We genotyped individuals at thousands of single nucleotide polymorphisms (SNPs) to characterize this species’ mating system and dispersal patterns. In addition, we used this genotypic data, in combination with spatial overlap and social network analyses, to describe this species social behavior, organization, and structure, as well as identify any kinship patterns guiding their sociality. Our integrative approach allowed increased accuracy and power in kin and parentage assignment (Foroughirad et al., 2019; Piza-Roca et al., 2019), as well as in quantification of the strength of social associations (Whitehead, 2008). We used these methods in combination with longitudinal field data to better understand the factors mediating variation in Tree Skink sociality at the individual level. Here we compare our findings with previous work to hypothesize about whether individual variation in social behavior may, when viewed at the population-level, result in intra-specific variation in social organization.
Materials and Methods
All our methods were approved by the Macquarie University Animal Ethics Committee (ARA # 2013/039) and approved by the New South Wales National Parks and Wildlife Service, Office of Environment and Heritage (License # SL101264).
Study Species
The Tree Skink, Egernia striolata, is a medium-sized skink (180–220 mm in total adult body length) that is sexually monomorphic in coloration and morphology, except for slight sex-specific differences in head shape and size (i.e., males have larger heads; Duckett et al., 2012; Cogger, 2014). Females are viviparous and give birth to a litter of 1–6 offspring from January to March each year (Chapple, 2003). The Tree Skink lifespan is unknown, but is estimated to be between 10 and 20 years based on data from similar species (i.e., White’s Skink, Liopholis whitii: G. While, pers. comm.). Tree skinks live in cracks, hollow limbs, beneath the bark of standing trees or fallen timber, and crevices in rock outcrops (Cogger, 2014). Individuals rely on these crevices as a “home-base” to forage, bask beside, and to take retreat. Tree Skinks are widely distributed, and can be found in dry sclerophyll forest, woodlands, and rock outcrops throughout central- and south-eastern Australia (inclusive of Queensland, New South Wales, and South Australia; Figure 1). For more details on the natural history of Tree Skinks refer to Supplementary Materials.
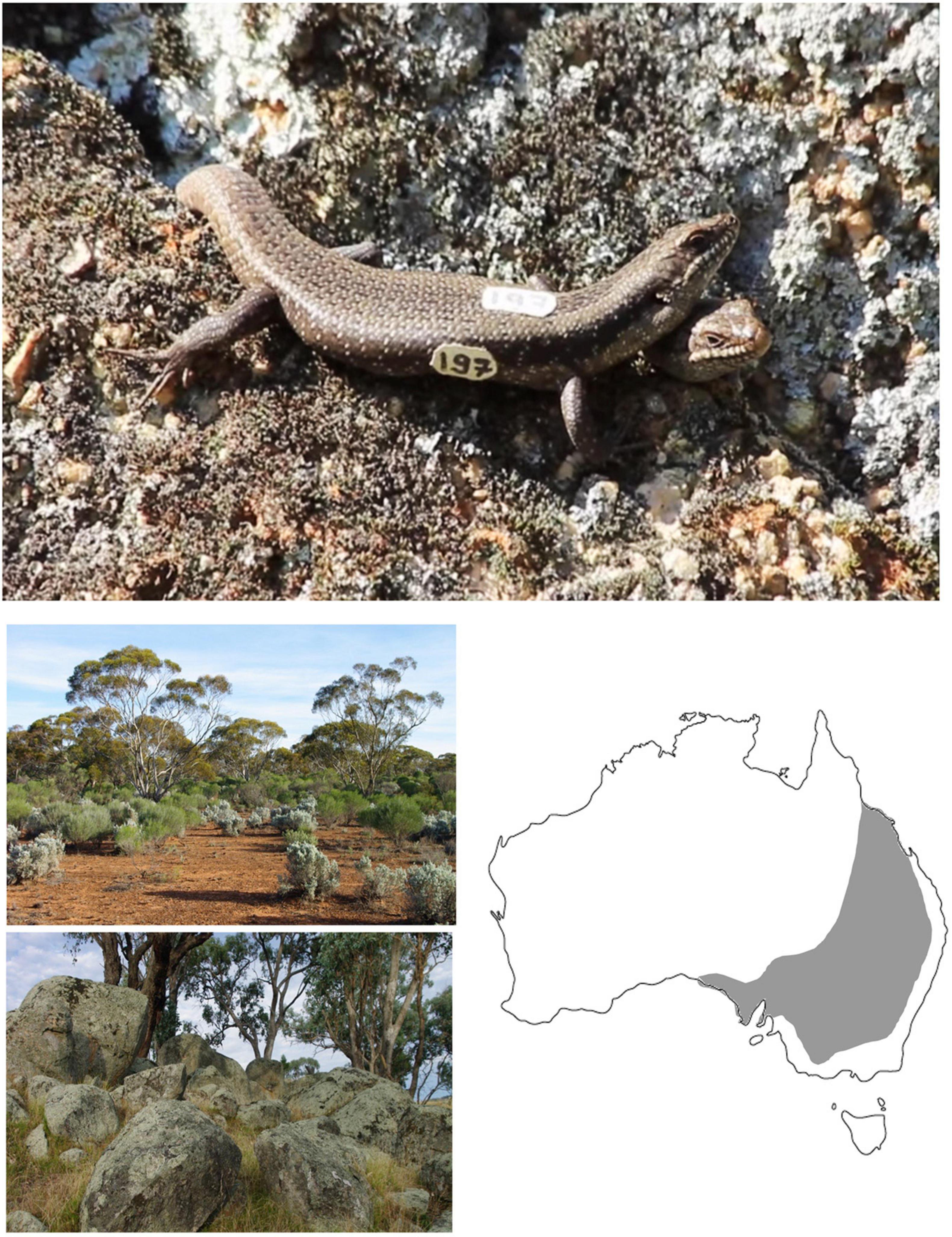
Figure 1. Tree Skinks (Egernia striolata) aggregate within crevices and hollows in rocks and trees (top; photo credit James O’Hanlon). They inhabit both forest and rocky habitats (bottom left; two examples of Tree Skink habitat photographed by Julia Riley) within their range in eastern Australia (bottom right; range map adapted from Cogger, 2014).
Mark-Recapture Surveys and Behavioral Observations
We conducted field surveys across the skink’s active season (August to May) from December 2013 to March 2016. Our field site was 2.5 ha (250,000 m2) and consisted of isolated rock outcrops and trees within an agricultural field in Albury, NSW, Australia (–35.98°S, 146.97°E; Supplementary Figure 1). At this site, we numerically marked 40 trees and 126 rocks that were habitat for Tree Skinks and mapped their locations using a GPS to an error of ± 3 m (Garmin eTrex Legend HCx).
Field surveys were conducted in December 2013, May 2014, September/October 2014, November 2014, January 2015, March 2015, October 2015, December 2015, and March 2016. These field surveys spanned two Tree Skink active seasons (2014/15 and 2015/16), which is the period during the year these skinks are not dormant (i.e., the spring, summer, and fall). Methodology was identical for all field surveys. First, all individuals in the population were captured using lassos, Elliott traps, and hand captures. Once captured, individuals were weighed (using a digital scale to 0.01 g) and measured (snout-vent and total length to 1 mm using a clear standard ruler). Sex was determined by eversion of the hemipenes. Skinks were identified as adults or juveniles using snout-vent length (SVL) as a guide. The cut-off used in our study to delineate adults was SVL > 80 mm, which is similar to cut-offs used in other studies of this species to delineate sexual maturity (Bonnett, 1999; Derez, 2004). At each individual’s first capture, it was permanently marked via subdermal insertion of a microchip (PIT-tag), or, if too small for insertion of a microchip (SVL < 60 mm), it was uniquely toe-clipped (Borges-Landaez and Shine, 2003). Tissue for genetic analysis was collected by trimming<5 mm of an individual’s tail tip with scissors, which was then stored in 100% ethanol. This approach has a minimal impact because Tree Skinks regenerate their tails and tips naturally break off due to wear. Lastly, we temporarily marked all individuals with uniquely numbered cloth tape (Tesa®, Hamburg, Germany; Olsson and Shine, 2000) for visual identification from a distance. There is no evidence that visually marking lizards impacts their predation rate (Calderon-Chalco and Putman, 2019) or affects their natural behavior.
Within each active season (2014/15 and 2015/16), the mark-recapture surveys as described above were conducted four times for 7–10 days each, and then were followed by visual observations of the skinks to collect spatial and behavioral data for 7–10 days. During the observation period, we walked around the survey site, visually locating skinks from a distance with binoculars. We then recorded the spatial location of the individual (rock or tree number) and whether individuals were aggregating with a conspecific or not. We also noted the nature of these aggregations—if individuals were situated on the same rock/tree, within two body lengths (∼40 cm) of one another, sharing a crevice, in visual contact (i.e., within each other’s line of sight), and/or in physical contact. Tree Skinks live in near-permanent association with crevices in rock outcrops as well as cracks, hollow limbs, or spaces beneath the bark of standing trees or fallen timber (Cogger, 2014). These crevices and hollows are the anchor to their social aggregations (Bustard, 1970; While et al., 2015). This means that skinks which share the same rock or tree, in most cases, form a social group (Bonnett, 1999; Duckett et al., 2012). We recorded this spatial and behavioral data a maximum of twice per day with 4 h separating observation periods (i.e., morning and afternoon observations). Across this study we captured and observed 203 individuals, which is 85% of the estimated population size (240 ± 42.2 SE; refer to Supplementary Materials for details). The population consisted of 17% juveniles, and the sex ratio was female-skewed (Supplementary Table 1). In the 2014/15 active season we recorded 1,308 observations of 139 individuals. In the 2015/16 active season, we recorded 2,285 observations of 127 individuals.
Analyzing Tree Skink Sociality
We analyzed the spatial and behavioral observational data collected over 2 study years (n = 3,593 observations of skinks) and brought multiple lines of evidence together to describe the nature of Tree Skink aggregations and social associations. Specifically, we (1) summarized aggregation size, composition, and stability across active seasons, (2) calculated the spatial overlap between skinks in our population as a proxy for social associations (as per Bull and Baghurst, 1998; Osterwalder et al., 2004; While et al., 2009a; Qi et al., 2012; Strickland et al., 2014), and (3) quantified social associations between individuals using social network analyses which allowed for a finer scale understanding of the nature of social interactions between individuals (as per Leu et al., 2016; Piza-Roca et al., 2019). Below we detail each of these components.
Size, Composition, and Stability of Social Groups
We considered skinks to be aggregating if individuals were on the same rock or tree during one of the twice-daily observation periods (similar to the definition used in Bonnett, 1999; Duckett et al., 2012). This definition was also used in our social network analyses (see section “Social Network Analysis”). Tree skinks use multiple lines of communication (i.e., visual, tactile, olfactory) to guide their social interactions (Fox et al., 2003; Baeckens and Whiting, 2021). Olfactory cues from scat-piling may influence individual habitat use and avoidance/preference for rocks/trees inhabited by a particular set of conspecifics on the landscape (Bull et al., 1999, 2000). Our definition (i.e., shared rock/tree use) of aggregations was therefore inclusive of all possible means of communication guiding Tree Skink social behavior. Based on our knowledge of the natural-history of this species as well as our field observations, it was clear that if skinks were on the same rock or tree they were aware of each other. Further, for reptiles like E. striolata, with cryptic social behavior and where social interactions are concentrated around a shared resource, definitions like ours for aggregations have been highly reliable at documenting social systems (Doody et al., 2013; Farine, 2015; Whiting and While, 2017).
We found that in 1007/3593 (28%) of behavioral observations individual Tree Skinks were aggregating with at least one conspecific. In total, we observed 438 aggregations (≥2 individuals on the same rock or tree) across our study’s two active seasons. Of these 438 aggregations, we found that 190 were unique (i.e., each group consisted of a distinct set of individuals). We then calculated size and categorized the demographic composition for each of these unique aggregations. We also assessed whether there was stability in these unique aggregations across our study by categorizing whether they were observed (or not) in both active seasons. This summary was used to give an indication of this species’ social organization and its variability at our study site.
Habitat Use and Spatial Overlap
To analyze Tree Skink habitat use and site fidelity, we used data from skinks with >10 spatial observations (n = 132). To summarize habitat use at our study site, we counted the number of times these skinks were observed on rocks vs. trees. To categorize this species’ site fidelity we also counted the number of times skinks were observed at each tree/rock location (see Supplementary Materials for more details).
As mentioned above, spatial overlap between skinks also gives an indication of their social associations. Therefore, to calculate spatial overlap between skinks at our study site, we first calculated the home range for each skink. We followed Smith (1995) and Qi et al. (2012) to determine the minimum number of spatial observations needed for home range estimation by regressing individual home range area (see details on estimation below) by the number of times sighted. We started by including all individuals that had at least 6 observations and found there was not a statistically significant relationship between home range size and the number of observations [R2 = 0.01, F(1,148) = 1.65, p = 0.20]. We also determined that Tree Skink home and core range overlap was highly correlated between study years (see Supplementary Materials). Thus, in our analysis of spatial overlap, we included skinks that had 6 or more spatial observations recorded for them over our 2 field seasons. The dataset included 4,830 spatial observations of 146 tree skinks. The number of locations varied between individuals from a minimum of 5 to a maximum of 106 (mean = 32.2, standard deviation = 22.42, median = 28). This and all analyses carried out in this study, unless stated otherwise, were completed in R version 3.4.4 (R Core Team, 2018). We estimated home ranges using bivariate normal kernel analysis with a reference bandwidth smoothing parameter (using the R package “adehabitatHR” and the function “kernelUD”; Calenge, 2011). We calculated home range area using 95% isopleths, and core area using 50% isopleths using the function “getverticeshr” (While et al., 2009b; Calenge, 2011). Although 95% isopleths reflect the area in which Tree Skinks carry out all their activities (i.e., foraging, dispersal, thermoregulation, etc.), it is hypothesized that the core area estimated using 50% isopleths reflects where most social behavior occurs for saxicolous reptiles (While et al., 2009b). Spatial overlap was calculated as the area (m2) each skink overlapped another’s home- and core-range.
Social Network Analysis
To quantify social associations between skinks within our population we used social network analysis. We restricted our analyses to data collected during the observation periods (a total of 3,593 observations of 167 skinks across 2 study years), excluding data generated during the mark-recapture surveys because physically capturing skinks likely altered their natural social behavior. Social network construction and analysis was performed using the “asnipe” R package (Farine, 2013). We combined observational data across all study periods because social associations were highly correlated across active seasons (see Supplementary Materials). For our social network analyses, we defined social associations as we did in see section “Size, Composition, and Stability of Social Groups”: individuals were associating if they were on the same rock or tree during one of the twice-daily observation periods. Association strength for each pair of skinks (dyad) was calculated using the half-weight association index (HWI). The HWI ranges between 0 (never observed on the same rock or tree) and 1 (always observed on the same rock or tree). HWI is typically used when an entire population cannot be observed during each sampling period (Cairns and Schwager, 1987), as is the case in our study. We constructed a social network with edge weights that were association strength values. This resulted in a weighted, undirected social network in which individuals were connected with HWI > 0 (Figure 2). This social network (i.e., a matrix containing all pairwise HWI reflecting Tree Skink association strength) was then used in downstream analyses. This overall social network contained social associations that occur both randomly and non-randomly (Whitehead, 2008). To determine if associations were significantly different from random, we identified a dyad as having a preferred association when their HWI was twice the mean, including all zeros (Gero et al., 2005). In our study, this means that dyads with an HWI > 0.004 were identified as preferred. This value was chosen because it is approximately twice the expected value if associations were completely random. The network of preferred social associations was also used in downstream analysis, to investigate if non-random associations between skinks were directed to related individuals.
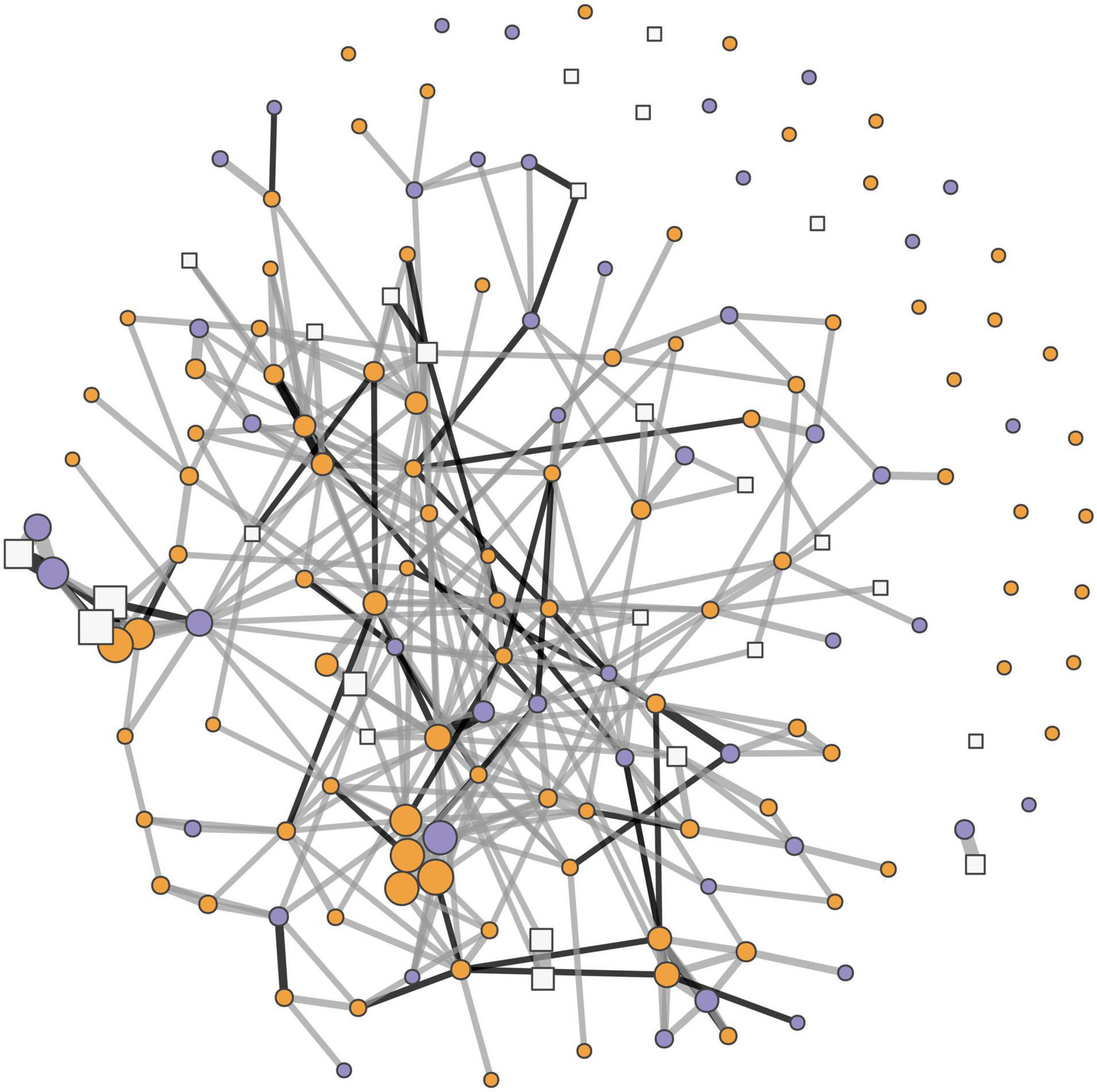
Figure 2. A social network depicting all the (overall) social associations between Tree Skinks (Egernia striolata) in our Albury, NSW population over two active seasons (2014–2016). Adults are represented with circles (orange are female, and purple are male), and juveniles are represented with white squares. The size of nodes reflects an individual’s weighted degree (i.e., the strength of their social associations or HWI). The thickness of connecting lines (edges) correspond to the HWI values (i.e., the strength of associations between skinks). Genetic relatedness estimates of more than 0.125, representative of at least first cousins, are depicted with black lines (edges), and all other lines are gray.
From the overall social network, we summarized three network metrics that quantify an individual’s social behavior: binary degree, weighted degree, and the coefficient of variation (CV) of edge weights. Binary degree is a tally of skinks the focal individuals were observed associating with. Weighted degree (also called “strength”; Whitehead, 2008) is the sum of edge weights (i.e., the dyadic HWIs) of the focal skink (Whitehead, 2008). Both binary and weighted degree reflects the sociability of an individual. The CV of edge weights (similar to “social differentiation” or “clustering coefficient”; Whitehead, 2008) of a focal skink quantifies variability of an individual’s associations. High CV values suggests that the focal skink’s associations are variable; in contrast, low CV values means that focal skink’s associations with conspecifics are relatively homogeneous (Riley et al., 2018b). We used three separate linear models to test if these network metrics differed between adult female, adult male, and juvenile skinks (details in Supplementary Materials).
Molecular Analyses to Estimate Genetic Relatedness
We used molecular analyses to quantify the genetic relatedness between individuals within our population. DNA was extracted from tissue samples using GenCatch™ Blood and Tissue Genomic Mini Prep Kits (Epoch Life Science, Inc., Sugarland, Texas), followed by genotyping of SNPs by Diversity Arrays Technology Pty Ltd. (Canberra, Australia). The resulting SNP dataset was filtered to ensure it consisted of loci that were physically unlinked and conformed to the expectations of HWE (more details in Supplementary Information and Riley et al., 2021). Our final filtered dataset consisted of 2,105 SNPs. We used the program COANCESTRY (Wang, 2011) to estimate relatedness between individual Tree Skinks. Through data simulation (see Supplementary Materials) we determined the dyadic likelihood relatedness estimator (Milligan, 2003) was the most appropriate for our data, and we used it to calculate pairwise relatedness values (R). This resulted in a matrix of pairwise relatedness values for our Tree Skink population, which was used in three independent downstream analyses. First, we combined this genetic relatedness data with our sociality data to test whether kinship plays a role in Tree Skink sociality (see section “Relationships Between Social Associations, Spatial Overlap, and Genetic Relatedness”). Second, we carried out a spatial autocorrelation analyses to explore broad-scale genetic structure at the population-level (see section “Dispersal: Spatial Autocorrelation”). Third, we combined this genetic relatedness data with parentage analyses to describe the mating system of our population (see section “Mating System”).
Relationships Between Social Associations, Spatial Overlap, and Genetic Relatedness
To investigate relationships between social associations, spatial overlap, and relatedness, we used the Multiple Regression Quadratic Assignment Procedure (MRQAP) following Dekker et al. (2007) with 10,000 permutations (using the function “mrqap.dsp” from the “asnipe” R package; Farine, 2013; Farine and Whitehead, 2015) and the double permutation approach for our hypothesis-testing as described in Farine and Carter (2020). This double permutation approach has been shown to reduce both type I- (false positives) and type-II error rates (false negatives) that are often a concern in social network analyses (Farine and Carter, 2021).
Our first analyses assessed whether Tree Skink social associations were correlated with relatedness (i.e., directed toward kin; see Piza-Roca et al., 2019 for a similar analysis framework). To achieve this, we ran two separate MRQAPs correlating either the overall or preferred association strength matrices against the relatedness matrix. Second, we assessed if spatial overlap occurred between relatives by running two separate MRQAPs correlating either the home- or core-range overlap matrices against the relatedness matrix. We analyzed the relationship between Tree Skink relatedness vs. association strength and spatial overlap separately because the latter two matrices were significantly related to one another (see Supplementary Materials). This avoided any confounding effects of including both these matrices in the same MRQAP. The relationship between association strength and spatial overlap is, indeed, logical, especially based on our definition of Tree Skink social groups. Third, with a separate MQRAP, we examined if skink demographics and size affected Tree Skink social associations. In this analysis, the overall association strength matrix was the dependent matrix, and three predictor matrices were included in our model: the relatedness matrix, demographic similarity (i.e., whether dyads were between two adult females, adult males, or juveniles), and size (SVL) similarly (details in Supplementary Materials). These predictor matrices were not strongly correlated (r < |0.05| in all cases), as assessed via pairwise comparisons with a Mantel test (function “mantel” in the R package “vegan” using 1,000 permutations; Oksanen et al., 2019).
Dispersal: Spatial Autocorrelation
We used a multi-locus spatial autocorrelation analysis in GENALEX 6.5 (Peakall and Smouse, 2006), as described in Peakall et al. (1995, 2003) and Smouse and Peakall (1999) to investigate Tree Skink dispersal and philopatry. The output of this analysis is a measure of genotypic similarity between dyads (r; also termed the autocorrelation coefficient) at predefined distance classes. For this analysis, we calculated matrices of genotypic distance based on our SNP dataset and Euclidean spatial distance based on the location each skink was most often observed during our 2-year study. These matrices were calculated separately for adult females, adult males, and juveniles to allow testing for differences in spatial genetic structure among demographic groups (Chapple and Keogh, 2005; Banks and Peakall, 2012; Piza-Roca et al., 2019). Juvenile SVL was also included in the analyses to reflect differences in age—if a juvenile was captured multiple times we used average SVL across repeated measures. Only skinks with all data (spatial, genetic, and morphometric) were included in the analyses (nF = 112, nM = 52, nJ = 34). Tests for significance of r were performed through 9,999 spatial permutations and we calculated 95% confidence intervals for estimates of r using 10,000 bootstraps (Peakall et al., 2003). We examined the spatial autocorrelation across the whole study population by analyzing pairwise genotypic data grouped into four distance categories (i.e., pairwise comparisons at 0.0–3.0 m, 3.1–10.0 m, 10.1–50.0 m, and 50.1–127.0 m). Selection of the distance classes strongly influences the ability to detect differences in genetic structure (Peakall et al., 2003; Banks and Peakall, 2012). By comparing a range of distance categories, we determined that these categories provided an adequate sample size while providing fine-scale information about spatial genetic structure (see Supplementary Materials).
Mating System
To determine the mating system of our Tree Skink population, we assessed the relatedness of offspring within litters of known mothers. In both field seasons, we collected gravid females (during January 2015 in the 2014/15 field season, and December 2015 in 2015/16 field season) and then transported them to, and maintained them at, Macquarie University, New South Wales, Australia until parturition (housing and husbandry details in Supplementary Materials). We visually checked enclosures twice daily to see if females had given birth. Within 12 h after birth, we recorded offspring morphometrics, marked each individual uniquely with a toe-clip (Borges-Landaez and Shine, 2003), and took a tissue sample for genetic analyses. We released offspring and their mothers at their site of capture a month before the final survey period of each season in March. Temporary removal of gravid females was required because the genetics of entire litters need to be sampled to quantify rates of multiple paternity and, in the wild, capturing full litters of offspring is unachievable due to high mortality and low capture rates of neonates. Other studies of Egernia-group skink sociality have previously used this approach (O’Connor and Shine, 2004; While et al., 2009b) with no effect on natural social behavior, and, similarly, in our study we did not observe any impact on female social behavior post-release.
We aimed to quantify the mating system for females in our main study population in Albury (Supplementary Figure 1) by identifying paternity of each litter. Unfortunately, we ended up with a low sample size of litters from this population (n = 17); even though a total of 53 females were caught and brought back to the laboratory to give birth across both study years, which represented 45% of known females within the population. In 2014/15, we collected 42 females, and only 1 of these females gave birth to 2 offspring across 2 days (5 and 6 February 2015). It is unknown if females gave birth before being caught in 2014/15, or if skinks were not gravid at our study due to other factors (e.g., biennial reproduction, consequences of drought). In 2015/16, we captured 28 females (17 that were also sampled the year before) and 16 gave birth to 35 offspring. Parturition occurred between 10 January and 11 March 2016. In total, only 2 and 57% of females sampled each year gave birth, which suggests Tree Skinks might not reproduce annually (see similar findings in L. whitii: While et al., 2009b; and in other Egernia-group skinks: Chapple, 2003). During the same time, we also captured 39 gravid female Tree Skinks from sites nearby our main study population for an experimental study investigating the effect of social environment on behavioral development (Riley et al., 2016, 2017, 2018a,b). No genetic samples were taken from males in these areas, so paternity could not be identified; sibship, however, can be genetically quantified and the likely mating system thereby established. A total of 39 litters of 88 offspring from wild-caught females were born from 10 February to 12 March 2014, and 17 January to 22 February 2015.
To maximize our sample size, we used genetic data from all litters (n = 55) for parentage analysis. The parentage analysis included genotypes from known mothers, as well as all males within our main study population. We used COLONY (Jones and Wang, 2010) and CERVUS (Marshall et al., 1998) to estimate paternity, and then verified paternity using our relatedness estimates (see more details in Supplementary Materials and a similar approach in Riley et al., 2021). In all cases, the results from all three methods agreed. These data give an indication of the mating system of Tree Skinks, which is important information about the sociality of this species (Whiting and While, 2017).
Results
Habitat Use
Lizards made use of both rock and tree habitats at our study site (see Supplementary Materials), and all sexes and life stages showed strong site fidelity. Specifically, while both females and males were observed at a variety of rock and tree locations (an average of 6 ± 3 standard deviation, and 6 ± 4, respectively), they were observed at just one of those locations in most of their observations (55 and 61%, respectively). Juveniles showed even higher site fidelity, as they were observed at their primary location in 75% of their observations.
Social System
Social Organization
In 28% (1,007/3,593) of behavioral observations, individual skinks were observed within an aggregation. Of the 438 aggregations we observed during our study, 190 were unique (i.e., consisted of a distinct set of individuals). The size of these unique Tree Skink aggregations varied between 2 and 5 individuals (Supplementary Table 3). The most observed composition was one male and one female (33% or n = 62/190) followed by multiple females (with no males; 22% or n = 42/190 female pairs, 6% or n = 11/190 groups of three females, and n = 1/190 group of four females) (Supplementary Table 4). Juveniles were more likely to aggregate with only females than with males or adults of mixed sexes (Supplementary Table 4). Of these unique aggregations, we observed 73 more than once during our study and 30% of these (n = 22/73) remained consistent across active seasons. In addition, Tree Skink social associations and spatial overlap were highly correlated across years (see Supplementary Materials), which suggests these aggregations reflect consistent social groupings.
Kinship, Social Associations, and Spatial Overlap
We found evidence that social associations were stronger between Tree Skinks that are more genetically related to one another (i.e., kin). Genetic relatedness was a significant predictor of overall [MQRAP estimate corrected with double-permutations (r) = 0.061, p < 0.001; Figure 3] and preferred association strength [MQRAP estimate corrected with double-permutations (r) = 0.061, p < 0.001]. These results were driven largely by associations between full siblings and between parents and offspring (Figure 3). Further supporting this pattern, we also observed that aggregations with adults and juveniles generally had higher within-group relatedness than adult-only groups (Supplementary Table 4). The average association strength (HWI) in our overall social network was 0.002 ± 0.0001 (SE), but, when only HWI > 0 are considered, the average is 0.094 ± 0.005, and the range of HWI > 0 is 0.013–0.762. Overall association strength was not significantly influenced by skink age or sex, or size similarity (Supplementary Table 5). This is evidence that the degree to which Tree Skinks associate with one another is not simply restricted between particular age classes, sexes (as is also shown in Supplementary Table 4), or individuals of a similar size.
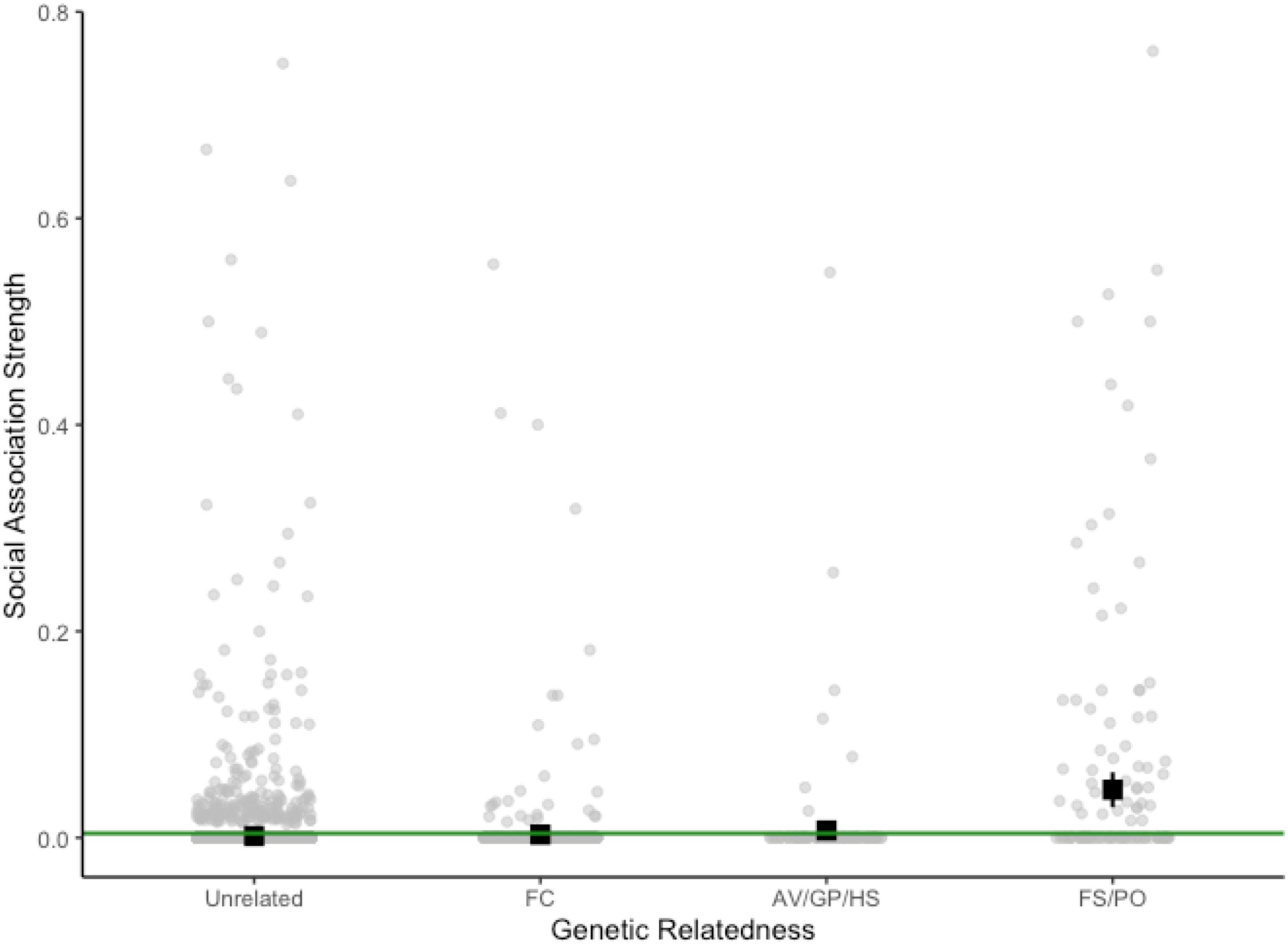
Figure 3. Strength of social associations (HWI) that we observed between Tree Skink dyads in our Albury, NSW population over two active seasons (2014–2016) as plotted against the genetic relatedness between those individuals. The green line represents the threshold (HWI > 0.004) over which individual social relationships are considered “preferred.” The genetic relatedness categories were delineated based on our approximate error rates depicted in Supplementary Figure 3: unrelated (R = 0–0.625, n = 9,425 dyads), first cousin (FC, R = 0.625–0.188, n = 949 dyads), avuncular/grandparent-grandchild/half sibling (AV/GP/HS; R = 0.188–0.313, n = 172 dyads) and full sibling/parent-offspring (FS/PO, R = 0.313–0.664, n = 184 dyads). For each category of genetic relatedness, the black square and lines show the mean HWI ± 95% confidence intervals. In this plot each individual is replicated multiple times, such that counts represent all possible pairwise combinations of individuals (n = 10,730 dyads).
We also found evidence that Tree Skinks that overlapped in their space-use were also more likely to be relatives because genetic relatedness was significantly, positively related to home- [MQRAP estimate (r) = 497.956, p < 0.001] and core-range overlap [MQRAP estimate (r) = 60.177, p < 0.001]. Thirty-one percent of skink dyads (3,255/10,585) overlapped in their home ranges, of which the home-range overlap averaged 378 ± 10 m2 and ranged between 1 and 6,078 m2. Core-range overlap occurred in 8% of skink dyads (848/10,585), which averaged 41 ± 4 m2 and ranged between 1 and 1,309 m2.
Social Structure
From our analysis of social network metrics (see section “Social Network Analysis”), we found that the nature of Tree Skink social associations differed depending on their sex and age (Table 1). Juveniles associated with more individuals (aka. binary degree) than females and males (βcorr = 3.393, βrand 95% range: 0.031–1.874, prand < 0.001). Male and female skinks did not differ in the number of conspecifics they associated with. Juveniles also had stronger social associations (aka. weighted degree) than females and males (βcorr = 0.044, βrand 95% range: 0.001–0.081, prand < 0.001). Males had weaker social associations than females. Juvenile social associations were also more homogeneous (i.e., less variable) than females and males (βcorr = –143.003, βrand 95% range: –86.073 to 66.709, prand < 0.001). Males had more variable social associations (aka. a greater CV of edge weights) than females.
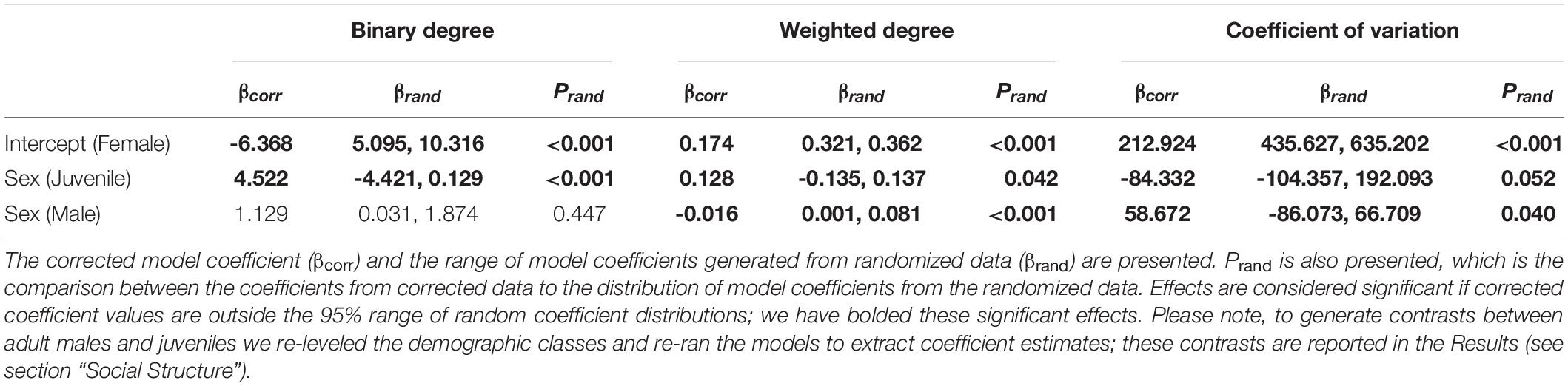
Table 1. Summary of our linear models testing for the effects of demographic classes (adult female, adult male, or juvenile) on social network metrics (see Supplementary Materials section 1.3.2 for details).
Dispersal
We found that adult Tree Skinks of each sex are largely philopatric with no genetic evidence for sex-biased dispersal. The juveniles appeared to be pre-dispersal (i.e., a higher r than adults from 0.0–3.0 m). For adults of each sex and juveniles, there was a significant decline in genetic relatedness with spatial distance. These patterns were relatively consistent among adult females, adult males, and juveniles, as well as distance classes (see Supplementary Materials). In general, there was a gradual decline in r-values after about 50 m and, after this, values become negative with increasing geographical distance (Figure 4).
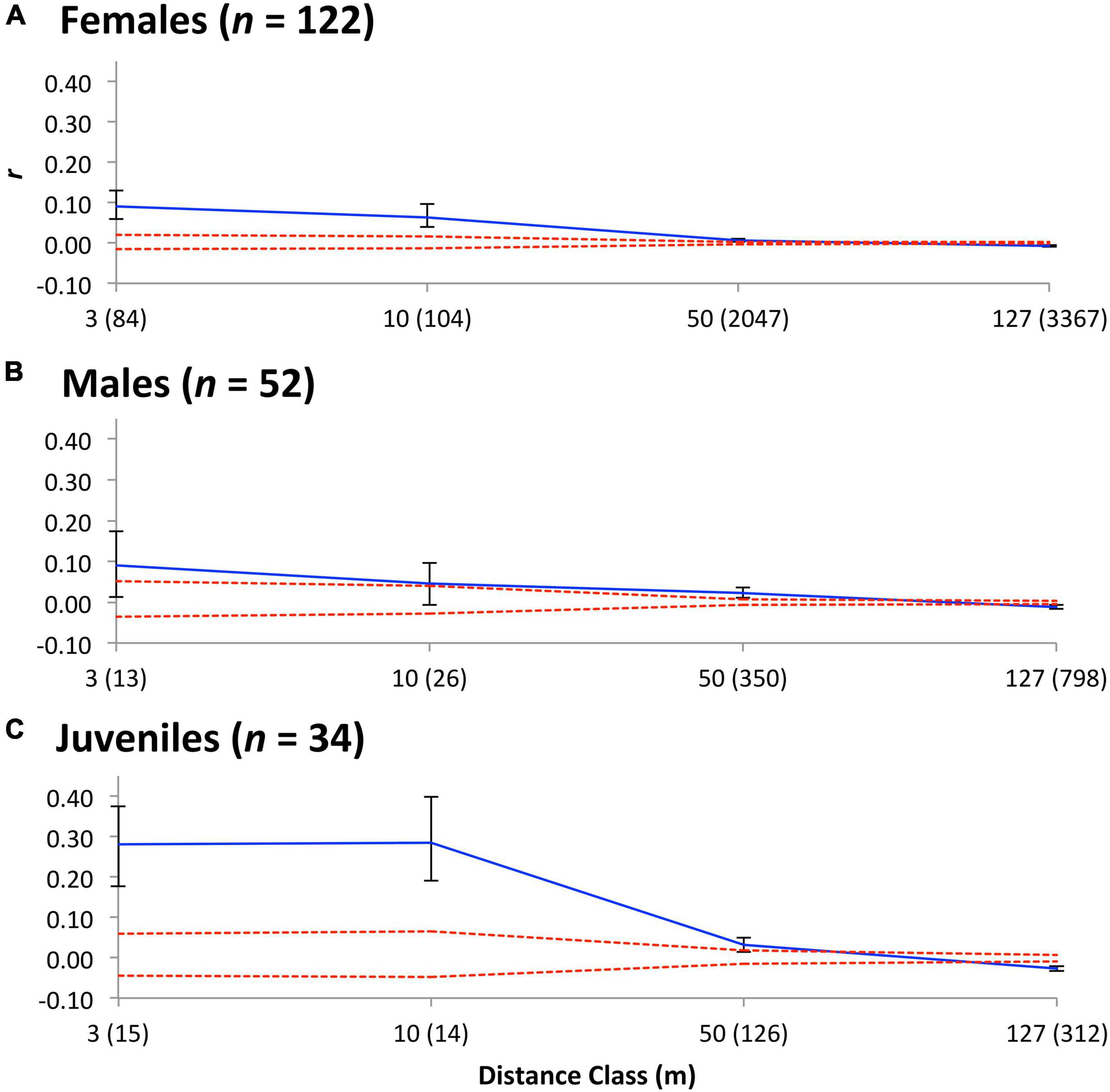
Figure 4. Correlograms displaying the genetic correlation (r) for Tree Skink (A) adult females, (B) adult males, and (C) juveniles over a function of distance (m). Sample sizes at each distance are shown in brackets following each class label. Dotted lines represent 95% confidence intervals reflecting the null hypothesis of a random distribution of genotypes, which were determined with 10,000 bootstraps.
Litter Size, Birthing Asynchrony, and Mating System
The average litter size was 2.27 individuals (standard deviation = 0.80, median = 2), and ranged from 1 to 4 offspring (n = 56). Females that gave birth to more than one offspring (n = 46) gave birth across 1.67 days (standard deviation = 0.60, median = 2, min = 1, max = 3). Thus, Tree Skinks in this population do experience birthing asynchrony (i.e., a spread in birth separated by a minimum of 12 h; While et al., 2007) like other Egernia-group skinks (Chapple, 2003; While et al., 2007). In fact, 28/46 (61%) of litters with more than one offspring were born asynchronously.
Parentage analyses showed that the mating system of Tree Skinks is predominantly monogamous. Of the 46 litters with more than one offspring, only 13% (n = 6) had mixed parentage. In total, 45 males sired the 55 litters born in this study. Parentage within a litter was only ever mixed between 2 males. There was little evidence for strong male reproductive skew: most males (68% or 31/45) only mated with one female, and the remaining males contributed to the litter of either 2 or 3 females.
For known mating pairs from our main study site (where mother identity was known and paternity was identified during parentage analyses, n = 15), the majority were unrelated (87% or n = 13/15). Of the related mating pairs, one represented a first-cousin relationship, and the other represented a half-sibling/avuncular/grandparent-grandchild relationship. On average, the genetic relatedness between mating pairs was 0.034 (standard deviation = 0.067, range = 0.000–0.234). The average association strength between these known mating pairs from our overall social network was 0.175 (standard deviation = 0.168, median = 0.111, ranged = 0.000–0.489). Thus, 73% (n = 11/15) of mating pair associations are preferred (i.e., association strength, HWI, values > 0.004), and this is suggestive of social, in addition to genetic, monogamy.
Discussion
Our study of a single population of Tree Skinks revealed a social system based on small, relatively stable, kin-based groups. Associations most commonly consisted of one adult male and one adult female, but adult females also aggregated with one another (up to a maximum of 4 skinks, and sometimes with one male present). These female-only groups were often made up of relatives (R = 0.125, or a “first cousin” relationship, and above) with 68% of these relatives either full-sibling or parent-offspring relationships. Juveniles were usually found associating with adult females. We only observed one juvenile-only group, which was made up of two full siblings, during the 2 study years (Supplementary Table 4). Although we observed a diversity of social associations and intra-population variability in social behavior throughout our study, the spatial overlap and social associations of Tree Skinks were strongly biased toward kin. The strongest social associations occurred within full-sibling and parent-offspring relationships, which suggests that many consistent social associations may be of nuclear families that share crevice sites and spend their time together. To further support this idea, their mating system reflected both social and genetic monogamy, as most females and males mated with the one partner with whom they had a preferred association. That said, the term “nuclear family” is typically used describe a social group consisting of parent(s) caring for the offspring of a single reproductive event (Kramer and Meunier, 2019). Thus, more research is needed to establish the care system of this species and determine whether offspring from one (or more than one) reproductive event are present in Tree Skink social groups. We discuss this and other future research topics our study has highlighted below.
Comparison of Sociality Across Studies and Species
Comparing our findings to previous work on Tree Skink sociality may approximate the degree of inter-population variability in Tree Skink social organization. In our population, Tree Skinks were gregarious—28% of behavioral observations of skinks were of individuals aggregating. This is likely a conservative estimate of their aggregative tendency, because this percentage includes only direct observations of associations between individuals and does not consider individuals who co-occurred together but who were not directly observed with one another (such as inside crevices). Such cryptic social associations are relatively common particularly for social species that do not display particularly overt social behavior. Previous studies have reported the percentage of observations of Tree Skinks in groups as 13% (Bustard, 1970), 47% (Michael et al., 2010), 63% (Duckett et al., 2012), and 78% (Bonnett, 1999). Maximum group size was 5 at our study site, which is similar to the range of maximal group sizes observed in other studies: 3 (Bustard, 1970), 4 (Michael et al., 2010; Duckett et al., 2012), and 6 (Bonnett, 1999). The most common group composition observed in our study was one female and one male, presumably breeding pairs. Breeding pairs, or groups of two when Tree Skinks were not sexed, were the most-observed group composition in other studies too (Bonnett, 1999; Michael et al., 2010). Variation in social structure amongst studies is not just related to adult group composition but also the frequency of adult-offspring associations. Indeed, in all studies, including ours, adults and juveniles were observed sharing crevices and basking together; however, the frequency of those associations varies among studies (Bustard, 1970; Bonnett, 1999; Derez, 2004; Michael et al., 2010; Duckett et al., 2012). Our study found evidence that suggests aggregations are stable in this species. Notably, spatial overlap and social associations were highly correlated between study seasons. Also, 30% of aggregations consisting of the exact same composition of individuals were observed over both study seasons. In addition to similarities in Tree Skink social organization, our study and the two others that included molecular analyses have all found that Tree Skink groups consist of kin (Bonnett, 1999; Duckett et al., 2012). Our study also found that both spatial overlap and social associations were positively correlated with relatedness—indicating that both space use and social interactions were biased toward kin in our population. Overall, our study was largely congruent with previous work on this species. This demonstrates there is considerable consistency in the basic components of sociality across Tree Skink populations, despite substantial variation in social behavior at the individual level (e.g., social plasticity).
Tree Skinks, across all sex and age classes, exhibited high site-fidelity and dispersal appeared limited. Philopatry is a trait common in gregarious and family-living animals (Waser and Jones, 1983; Ekman, 2006), so it is not surprising that family-living Tree Skinks also exhibit it. For example, in the Yakka Skink (E. rugosa), on average, 50% of individuals remained at their original site of capture for at least 5 years (Peck et al., 2017). The lack of evidence we found for sex-biased dispersal in Tree Skinks was unexpected, as either female- or male-biased dispersal is often viewed as a mechanism to avoid inbreeding in social species (Pusey, 1992; Gardner et al., 2013; Whiting and While, 2017). In our study, the majority of known mating pairs were unrelated, and, thus, it appears that Tree Skinks can avoid inbreeding, and perhaps do so through kin recognition (Bull et al., 2001).
Most females and males in our Tree Skink population produced offspring with one partner. Rock-dwelling Tree Skinks in South Australia also exhibited low levels of genetic polygamy (1 of 6 litters had multiple paternity: Bonnett, 1999). Despite the low sample size, this is comparable to our observed rate of 13% multiple paternity. This level of polygamy is on the low-end of the variation documented in Egernia-group species (Chapple, 2003; Whiting and While, 2017). For example, the socially monogamous Sleepy Lizard (Tiliqua rugosa) exhibits a rate of multiple paternity of 19% (Bull, 2000). Several other Egernia spp. or close relatives also show modest rates of multiple paternity within litters: Cunningham’s Skinks (E. cunninghami 3%: Stow and Sunnucks, 2004) White’s Skinks (Liopholis whitii 11.6–17%: Chapple and Keogh, 2005; While et al., 2009b), Black Rock Skinks (E. saxatilis 20%: Chapple, 2003), and Gidgee Skinks (E. stokesii 25%: Gardner et al., 2002). The evidence of multiple mating within a socially monogamous mating system observed in most group- and family-living Egernia-group species including Tree Skinks, is consistent with studies of socially monogamous bird species (Birkhead and Møller, 1995; Brouwer and Griffith, 2019). Outside of the Egernia-group of skinks, multiple paternity within clutches or litters is the norm in lizards (an average of 53% and ranges between 11 and 87%: summarized from Table 15.1 in Wapstra and Olsson, 2014). Based on our data, we suggest that Tree Skinks are both socially and genetically monogamous. For females whose mates were identified, the majority exhibited preferred social associations. However, most of our paternity data were collected within 1 year, and only multiple years of sampling could tell us if there is consistency in mating partners and patterns in this species, similar to what has been seen in Sleepy Lizards (Bull et al., 1998; Bull, 2000) and White’s Skinks (While et al., 2009b). Future research is needed to tease out consistency in mating systems and mate choice in Tree Skinks.
Our evidence that social associations between parents and offspring are largely preferred, and our observation that adult and juveniles are often aggregating in our populations (25% or 47/190 of unique aggregations observed), raises interesting questions regarding the nature of these groupings. We know from other Egernia-group species that such parent-offspring associations have the potential to provide significant benefits to offspring in terms of reduced risk of infanticide (O’Connor and Shine, 2004), reduced predation risk (Watson et al., 2020), increased access to resources (Munch et al., 2018) and, in the long-term, potential inheritance of territories (Gardner et al., 2001). Targeted study of the nature of Tree Skink parent offspring aggregations, perhaps though the use of focal individual observations or trail cameras which have been enlightening in regard to the extent of parental care present in Cunningham’s skinks (Watson et al., 2020), may afford novel insights into direct parental care and communication (see Supplementary Materials for anecdotal observations) within Egernia-group skinks. The potential for communication, and potentially, cultural transmission, is significant in this species, because both juveniles (Riley et al., 2018a) and adults (Whiting et al., 2018) learn socially from one another.
Variation in Social Behavior at the Individual and Population Level
Our study highlights intra-population variability in social organization and structure occurring within our Tree Skink population. We observed a substantial variety of group compositions across 2 years. We also found individual social behavior differed among Tree Skink age classes and sexes. Our detailed observations revealed that juvenile skinks associated with more individuals and these associations were stronger and less variable than the associations of adult males and females. Female skinks, although they had a similar number of social connections to males, their connections were stronger and less variable (i.e., were observed more often within their groups than males). These sex- and age-class-specific differences in Tree Skink social behavior may have the capacity to underpin inter-population variability in sociality depending on demographic differences between populations. Future work is needed to understand the role this variation plays both in individual fitness and the evolution of sociality. First, how does variation in individual social behavior link to fitness? Previous work has shown, within an experimental context, that Tree Skinks can be plastic in their social associations, potentially maximizing individual benefits within a dynamically changing environment (Riley et al., 2018b). In the field, Bonnett (1999) found that individual vigilance rates (head movements per minute) were lower for grouped sub-adults than lone sub-adults, but this trend was not observed in adults. These studies both suggest potential benefits of aggregation for Tree Skinks, but clear links to individual fitness are yet to be determined. Second, future research should aim to identify a link between inter-individual variation in social traits and the degree of inter-population variability in sociality that has often been discussed in Tree Skinks, and ultimately, between Egernia-group species too.
There are challenges associated with comparing social systems across studies with differing methodologies (Whitehead, 2008; Castles et al., 2014). For example, concerns regarding experimental designs needed to detect kin-based sociality (i.e., minimum sample sizes; Foroughirad et al., 2019, the type of molecular analyses; Santure et al., 2010; Hauser et al., 2011) are critical to guide future research in this field. However, the similarity between studies on Tree Skinks sociality spanning ∼20 years suggests that some categorizations of sociality may be robust to variation in methodology. The value of insights generated by long-term field studies on vertebrate sociality (e.g., acorn woodpeckers, Koenig et al., 2019; meerkats, Russell et al., 2007; sperm whales, Gero et al., 2015 and as reviewed in Clutton-Brock and Sheldon, 2010; Hughes et al., 2017) is clear. Yet, for the purposes of an initial description of a species’ social system, simpler sampling methods, such as that used in Duckett et al. (2012), may suffice. With limited resources for research, and the huge knowledge gaps we face in the field of reptile sociality, it is important to have accessible methodologies to start studying the sociality present in promising, understudied reptile groups (e.g., Cordylidae; Mouton, 2011, and Iguanidae; Burghardt et al., 1977). Further, simpler sampling methods may be more easily replicated across populations of one species or multiple species, which could offer a logistically feasible comparative approach to study the drivers of variation in sociality.
Conclusion
The combination of our findings indicates that Tree Skinks live in small, kin-based social groups centered around their use of rock and tree crevices and are monogamous. Tree Skinks are unique in that multiple investigations of their social, and to a lesser extent, their mating system, have been undertaken, with varying methodologies and time scales. This is rare in a field where less than 1% of squamates’ aggregation tendency has been studied (Gardner et al., 2016). Interestingly, the social and mating systems of Tree Skinks appear to be largely consistent across their range despite a high degree of intra-population variability in social behavior, social organization, and structure occurring within populations. This raises the question of whether this species’ social system is facultative (as suggested in Chapple, 2003; Riley, 2017; Whiting and While, 2017), and if it is, what circumstances are necessary for Tree Skinks to transition to solitary life rather than family-living. Future research examining variation in sociality using a comparative approach across populations or species, or an experimental process that teases apart potential sources of variation (as per Visagie et al., 2005; Schutz et al., 2007) may allow us to gain a greater understanding of the evolution of reptile family life. The detailed understanding of the complexity of Tree Skink sociality our study provides a foundation from which more targeted research can be undertaken.
Data Availability Statement
The data for this study are available from Open Source Framework (OSF) and can be found at the following link: https://osf.io/bn9te/ (doi: 10.17605/OSF.IO/BN9TE).
Ethics Statement
The animal study was reviewed and approved by the Macquarie University Animal Ethics Committee (ARA # 2013/039).
Author Contributions
Initials of authors are presented in alphabetical order by first initial and do not represent relative contributions. DWAN, GMW, JLR, MJW, and RWB all conceptualized this study. JLR, MJW, and RWB obtained research funding for this study. DWAN and MJW obtained ethics approval and permits. JLR led fieldwork, and DWAN, GMW, and MJW all contributed to field data collection. AJS, PB, and SD provided guidance and training on molecular analyses that JLR completed. JLR conducted data summaries, as well as social network and statistical analyses and led the writing of this manuscript. All authors contributed to editing and finalizing the final manuscript for publication.
Funding
Financial support for this research was provided by The Australian Research Council (ARC DP130102998 grant to MJW and RWB), the Australian Government (Endeavour Fellowship to JLR), the Australian Museum, the Australasian Society for the Study of Animal Behaviour, the Natural Science and Engineering Council of Canada (scholarship to JLR), and Macquarie University (research funding and scholarship to JLR).
Conflict of Interest
The authors declare that the research was conducted in the absence of any commercial or financial relationships that could be construed as a potential conflict of interest.
Publisher’s Note
All claims expressed in this article are solely those of the authors and do not necessarily represent those of their affiliated organizations, or those of the publisher, the editors and the reviewers. Any product that may be evaluated in this article, or claim that may be made by its manufacturer, is not guaranteed or endorsed by the publisher.
Acknowledgments
We thank S. Attersley, J. Baxter-Gilbert, M. Berry, R. Brent, I. Damas-Moreira, S. Deventer, R. Everett, M. Favre, M. Francis, G. Heard, O. Monck-Whipp, J. Ocock, J. O’Hanlon, D. Paris, F. Passas, S. Perkins, R. Robinson, R. Shepard, B. Smagala, and A. Tak for their assistance in the field. Also, thanks to F. Kar, P. Vine, C. Wilson, and the exceptional volunteer team at Macquarie University’s Lizard Lab for their care of lizards. K. Bilgmann, P. Momigliano, J. Thompson, L. Waldon, and S. Yadev deserve thanks for their help with genetic analyses. We thank to J. Paterson for his help with spatial analyses, C. Rouleau for his inspiration on plotting social association and kinship data, and H. Whitehead and D. Farine for their advice on social network analyses. We also thank M. Gardner and J. Clayton for supplying hard-to-obtain literature, as well as two reviewers and M. Elgar for their feedback that greatly improved this manuscript.
Supplementary Material
The Supplementary Material for this article can be found online at: https://www.frontiersin.org/articles/10.3389/fevo.2021.722455/full#supplementary-material
References
Baeckens, S., and Whiting, M. J. (2021). Investment in chemical signalling glands facilitates the evolution of sociality in lizards. Proc. R. Soc. Lond. B 288:202002438. doi: 10.1098/rspb.2020.2438
Banks, S. C., and Peakall, R. (2012). Genetic spatial autocorrelation can readily detect sex-biased dispersal. Mol. Ecol. 21, 2092–2105. doi: 10.1111/j.1365-294X.2012.05485.x
Birkhead, T. R., and Møller, A. P. (1995). Extra-pair copulation and extra-pair paternity in birds. Anim. Behav. 49, 843–848.
Bonnett, M. (1999). The Ecology, Behaviour and Genetic Relationships of a Population of Egernia striolata. Unpublished Honours thesis. Bedford Park SA: Flinders University.
Borges-Landaez, P. A., and Shine, R. (2003). Influence of toe-clipping on running speed in Eulamprus quoyii, an Australian scincid lizard. J. Herpetol. 37, 592–595. doi: 10.1670/26-02n
Brito, E. S. (2017). Group Living, Parental Care, Age Structure, and Genetic Relatedness in Liolaemus leopardinus, A High-Elevation Lizard from the Andes of Chile. Unpublished Doctoral dissertation. Stillwater, OK: Oklahoma State University.
Brouwer, L., and Griffith, S. C. (2019). Extra-pair paternity in birds. Mol. Ecol. 28, 4864–4882. doi: 10.1111/mec.15259
Bull, C. M., and Baghurst, B. C. (1998). Home range overlap of mothers and their offspring in the sleepy lizard, Tiliqua rugosa. Behav. Ecol. Sociobiol. 42, 357–362.
Bull, C. M., Cooper, S. J., and Baghurst, B. C. (1998). Social monogamy and extra-pair fertilization in an Australian lizard, Tiliqua rugosa. Behav. Ecol. Sociobiol. 44, 63–72. doi: 10.1007/s002650050515
Bull, C. M., Griffin, C. L., and Johnston, G. R. (1999). Olfactory discrimination in scat-piling lizards. Behav. Ecol. 10, 136–140. doi: 10.1093/beheco/10.2.136
Bull, C. M., Griffin, C. L., Lanham, E. J., and Johnston, G. R. (2000). Recognition of pheromones from group members in a gregarious lizard, Egernia stokesii. J. Herpetol. 34, 91–99.
Bull, M. C., Griffin, C. L., Bonnett, M., Gardner, M. G., and Cooper, S. J. (2001). Discrimination between related and unrelated individuals in the Australian lizard, Egernia striolata. Behav. Ecol. Sociobiol. 50, 173–179. doi: 10.1007/s002650100348
Burghardt, G. M., Greene, H. W., and Rand, A. S. (1977). Social behavior in hatchling green iguanas: life at a reptile rookery. Science 195, 689–691. doi: 10.1126/science.195.4279.689
Bustard, H. R. (1970). A population study of the scincid lizard Egernia striolata in northern New South Wales. KNAW 73:186.
Cairns, S. J., and Schwager, S. J. (1987). A comparison of association indices. Anim. Behav. 35, 1454–1469. doi: 10.1016/s0003-3472(87)80018-0
Calderon-Chalco, K. A., and Putman, B. (2019). The effect of paint marking on predation risk in Western Fence Lizards: a test using clay models. Herpetol. Conserv. Biol. 14, 80–90.
Calenge, C. (2011). Home Range Estimation in R: The adehabitatHR Package. Available online at: https://cran.r-project.org > adehabitatHR > vignettes (accessed March, 2019).
Castles, M., Heinsohn, R., Marshall, H. H., Lee, A. E. G., Cowlishaw, G., and Carter, A. J. (2014). Social networks created with different techniques are not comparable. Anim. Behav. 96, 59–67. doi: 10.1016/j.anbehav.2014.07.023
Chapple, D. G. (2003). Ecology, life-history, and behavior in the Australian scincid genus Egernia, with comments on the evolution of complex sociality in lizards. Herpetol. Monogr. 17, 145–180. doi: 10.1655/0733-1347(2003)017[0145:elabit]2.0.co;2
Chapple, D. G., and Keogh, J. S. (2005). Complex mating system and dispersal patterns in a social lizard, Egernia whitii. Mol. Ecol. 14, 1215–1227. doi: 10.1111/j.1365-294X.2005.02486.x
Clark, R. W., Brown, W. S., Stechert, R., and Greene, H. W. (2012). Cryptic sociality in rattlesnakes (Crotalus horridus) detected by kinship analysis. Biol. Lett. 8, 523–525. doi: 10.1098/rsbl.2011.1217
Clutton-Brock, T., and Sheldon, B. C. (2010). Individuals and populations: the role of long-term, individual-based studies of animals in ecology and evolutionary biology. Trends Ecol. Evol. 25, 562–573. doi: 10.1016/j.tree.2010.08.002
Davis, A. R., Corl, A., Surget-Groba, Y., and Sinervo, B. (2011). Convergent evolution of kin-based sociality in a lizard. Proc. R. Soc. Lond. B Biol. Sci. 278, 1507–1514. doi: 10.1098/rspb.2010.1703
Dekker, D., Krackhardt, D., and Snijders, T. A. (2007). Sensitivity of MRQAP tests to collinearity and autocorrelation conditions. Psychometrika 72, 563–581. doi: 10.1007/s11336-007-9016-1
Derez, C. (2004). Sexual Dimorphism, Spatial Ecology and Behaviour in a Tree-Dwelling Population of Egernia striolata. Unpublished Honours thesis. Bedford Park SA: Flinders University.
Doody, J. S., Burghardt, G. M., and Dinets, V. (2013). Breaking the social-non-social dichotomy: a role for reptiles in vertebrate social behavior research? Ethology 119, 95–103. doi: 10.1111/eth.12047
Duckett, P. E., Morgan, M. H., and Stow, A. J. (2012). Tree-dwelling populations of the skink Egernia striolata aggregate in groups of close kin. Copeia 2012, 130–134. doi: 10.1643/ce-10-183
Elgar, M. A. (2015). Integrating insights across diverse taxa: challenges for understanding social evolution. Front. Ecol. Evol. 3:124. doi: 10.3389/fevo.2015.00124
Farine, D. R. (2013). Animal social network inference and permutations for ecologists in R using asnipe. Methods Ecol. Evol. 4, 1187–1194.
Farine, D. R. (2015). Proximity as a proxy for interactions: issues of scale in social network analysis. Anim. Behav. 104, E1–E5.
Farine, D. R., and Carter, G. G. (2021). Permutation tests for hypothesis testing with animal social data: problems and potential solutions. Methods Ecol. Evol. 0, 1–13. doi: 10.1111/2041-210X.13741
Farine, D. R., and Whitehead, H. (2015). Constructing, conducting and interpreting animal social network analysis. J. Anim. Ecol. 84, 1144–1163. doi: 10.1111/1365-2656.12418
Foroughirad, V., Levengood, A. L., Mann, J., and Frère, C. H. (2019). Quality and quantity of genetic relatedness data affect the analysis of social structure. Mol. Ecol. Resour. 19, 1181–1194. doi: 10.1111/1755-0998.13028
Fox, S. F., McCoy, J. K., and Baird, T. A. (2003). Lizard Social Behavior. Baltimore, MD: Johns Hopkins University Press.
Gardner, M. G., Bull, C. M., and Cooper, S. J. B. (2002). High levels of genetic monogamy in the group-living Australian lizard Egernia stokesii. Mol. Ecol. 11, 1787–1794. doi: 10.1046/j.1365-294x.2002.01552.x
Gardner, M. G., Bull, C. M., Cooper, S. J. B., and Duffield, G. A. (2001). Genetic evidence for a family structure in stable social aggregations of the Australian lizard Egernia stokesii. Mol. Ecol. 10, 175–183. doi: 10.1046/j.1365-294x.2001.01171.x
Gardner, M. G., Godfrey, S. S., Fenner, A. L., Donnellan, S. C., and Bull, C. M. (2013). Fine-scale spatial structuring as an inbreeding avoidance mechanism in the social skink Egernia stokesii. Aust. J. Zool. 60, 272–277. doi: 10.1071/zo12089
Gardner, M. G., Pearson, S. K., Johnston, G. R., and Schwarz, M. P. (2016). Group living in squamate reptiles: a review of evidence for stable aggregations. Biol. Rev. 91, 925–936. doi: 10.1111/brv.12201
Gero, S., Bejder, L., Whitehead, H., Mann, J., and Connor, R. C. (2005). Behaviourally specific preferred associations in bottlenose dolphins, Tursiops spp. Can. J. Zool. 83, 1566–1573. doi: 10.1139/z05-155
Gero, S., Gordon, J., and Whitehead, H. (2015). Individualized social preferences and long-term social fidelity between social units of sperm whales. Anim. Behav. 102, 15–23. doi: 10.1016/j.anbehav.2015.01.008
Graves, B. M., and Duvall, D. (1995). Aggregation of squamate reptiles associated with gestation, oviposition, and parturition. Herpetol. Monogr. 9, 102–119. doi: 10.2307/1466999
Halliwell, B., Uller, T., Chapple, D. G., Gardner, M. G., Wapstra, E., and While, G. M. (2017). Habitat saturation promotes delayed dispersal in a social reptile. Behav. Ecol. 11, 515–522. doi: 10.1093/beheco/arw181
Hatchwell, B., and Komdeur, J. (2000). Ecological constraints, life history traits and the evolution of cooperative breeding. Anim. Behav. 59, 1079–1086. doi: 10.1006/anbe.2000.1394
Hauser, L., Baird, M., Hilborn, R. A. Y., Seeb, L. W., and Seeb, J. E. (2011). An empirical comparison of SNPs and microsatellites for parentage and kinship assignment in a wild sockeye salmon (Oncorhynchus nerka) population. Mol. Ecol. Resour. 11, 150–161. doi: 10.1111/j.1755-0998.2010.02961.x
Hughes, B. B., Beas-Luna, R. B., Barner, A. K., Brewitt, K., Brumbaugh, D. R., Cerny-Chipman, E. B., et al. (2017). Long-term studies contribute disproportionately to ecology and policy. Bioscience. 67, 271–281. doi: 10.1093/biosci/biw185
Jeanson, R., and Weidenmüller, A. (2014). Interindividual variability in social insects–proximate causes and ultimate consequences. Biol. Rev. 89, 671–687. doi: 10.1111/brv.12074
Jones, O. R., and Wang, J. (2010). COLONY: a program for parentage and sibship inference from multilocus genotype data. Mol. Ecol. Resour. 10, 551–555. doi: 10.1111/j.1755-0998.2009.02787.x
Koenig, W. D., Walters, E. L., and Barve, S. (2019). Does helping-at-the-nest help? The case of the acorn woodpecker. Front. Ecol. Evol. 7:272. doi: 10.3389/fevo.2019.00272
Kramer, J., and Meunier, J. (2019). The other facets of family life and their role in the evolution of animal sociality. Biol. Rev. 94, 199–215. doi: 10.1111/brv.12443
Leu, S., Farine, D. R., Wey, T. W., and Bull, C. M. (2016). Environment modulates population social structure: experimental evidence from replicated social networks of wild lizards. Anim. Behav. 111, 23–31. doi: 10.1016/j.anbehav.2015.10.001
Mallarino, R., Pillay, N., Hoekstra, H. E., and Schradin, C. (2019). African striped mice. Curr. Biol. 28, R293–R305.
Marshall, T., Slate, J., Kruuk, L., and Pemberton, J. (1998). Statistical confidence for likelihood-based paternity inference in natural populations. Mol. Ecol. 7, 639–655. doi: 10.1046/j.1365-294x.1998.00374.x
Michael, D. R., Cunningham, R. B., and Lindenmayer, D. B. (2010). The social elite: habitat heterogeneity, complexity and quality in granite inselbergs influence patterns of aggregation in Egernia striolata (Lygosominae: Scincidae). Austral Ecol. 35, 862–870.
Mouton, P. L. F. N. (2011). Aggregation behaviour of lizards in the arid western regions of South Africa. Afr. J. Herpetol. 60, 155–170. doi: 10.1080/21564574.2011.562926
Munch, K. L., Noble, D. W., Budd, L., Row, A., Wapstra, E., and While, G. M. (2018). Maternal presence facilitates plasticity in offspring behavior: insights into the evolution of parental care. Behav. Ecol. 29, 1298–1306.
O’Connor, D. E., and Shine, R. (2004). Parental care protects against infanticide in the lizard Egernia saxatilis (Scincidae). Anim. Behav. 68, 1361–1369.
Oksanen, J., Blanchet, F. G., Friendly, M., Kindt, R., Legendre, P., McGlinn, D., et al. (2019). vegan: Community Ecology Package. R Package Version 2.5-6. Available online at: https://CRAN.R-project.org/package=vegan (accessed November 28, 2020).
Olsson, M., and Shine, R. (2000). Ownership influences the outcome of male-male contents in the scincid lizard, Niveoscincus microlepidotus. Behav. Ecol. 11, 587–590.
Osterwalder, K., Klingenböck, A., and Shine, R. (2004). Field studies on a social lizard: home range and social organization in an Australian skink, Egernia major. Austral Ecol. 29, 241–249. doi: 10.1111/j.1442-9993.2004.01339.x
Peakall, R., and Smouse, P. E. (2006). GENALEX 6: genetic analysis in Excel. Population genetic software for teaching and research. Mol. Ecol. Resour. 6, 288–295. doi: 10.1093/bioinformatics/bts460
Peakall, R., Ruibal, M., and Lindemayer, D. B. (2003). Spatial autocorrelation analysis offers new insights into gene flow in the Australian bush rat, Rattus fuscipes. Evolution 57, 1182–1195. doi: 10.1111/j.0014-3820.2003.tb00327.x
Peakall, R., Smouse, P. E., and Huff, D. R. (1995). Evolutionary implications of allozyme and RAPD variation in diploid populations of diocecious Buffalograss Buchloe dactyloides. Mol. Ecol. 4, 135–147. doi: 10.1111/j.1365-294x.1995.tb00203.x
Peck, S., Gardner, M. G., Seddon, J. M., and Baxter, G. (2017). Life history characteristics of the yakka skink, Egernia rugosa, indicate long-term social structure. Aust. J. Zool. 64, 335–343. doi: 10.1071/zo16061
Piza-Roca, C., Strickland, K., Kent, N., and Frère, C. H. (2019). Presence of kin-biased social associations in a lizard with no parental care: the eastern water dragon (Intellagama lesueurii). Behav. Ecol. 30, 1406–1415. doi: 10.1093/beheco/arz093
Pusey, A. E. (1992). “The primate perspective on dispersal,” in Animal Dispersal, eds N. C. Stenseth and W. Z. Lidicker (Dordrecht: Springer).
Qi, Y., Noble, D. W., Fu, J., and Whiting, M. J. (2012). Spatial and social organization in a burrow-dwelling lizard (Phrynocephalus vlangalii) from China. PLoS One 7:e41130. doi: 10.1371/journal.pone.0041130
R Core Team. (2018). R: A Language and Environment for Statistical Computing. Vienna: R Foundation for Statistical Computing.
Riley, J. L. (2017). Social Environment Impacts Behavioural Development of a Family-living Lizard. Ph.D. Dissertation, Macquarie University, New South Wales, Australia.
Riley, J. L., Guidou, C., Fryns, C., Mourier, J., Leu, S. T., Noble, D. W. A., et al. (2018b). Isolation rearing does not constrain social plasticity in a family-living lizard. Behav. Ecol. 29, 563–573. doi: 10.1093/beheco/ary007
Riley, J. L., Küchler, A., Damasio, T., Noble, D. W. A., Byrne, R. W., and Whiting, M. J. (2018a). Learning ability is unaffected by isolation rearing in a family-living lizard. Behav. Ecol. Sociobiol. 72:20.
Riley, J. L., Noble, D. W. A., Byrne, R. W., and Whiting, M. J. (2016). Does social environment influence learning ability in a family-living lizard? Anim. Cogn. 20, 449–458. doi: 10.1007/s10071-016-1068-0
Riley, J. L., Noble, D. W. A., Byrne, R. W., and Whiting, M. J. (2017). Early social environment influences in the behaviour of a family-living lizard. R. Soc. Open Sci. 4:161082. doi: 10.1098/rsos.161082
Riley, J. L., Stow, A., Bolton, P. E., Dennison, S., Byrne, R., and Whiting, M. J. (2021). Sperm storage in a family-living lizard, the Tree Skink (Egernia striolata). J. Hered. 112, 526–534. doi: 10.1093/jhered/esab048
Rosenthal, M. F., Gertler, M., Hamilton, A. D., Prasad, S., and Andrade, M. C. (2017). Taxonomic bias in animal behaviour publications. Anim. Behav. 127, 83–89. doi: 10.1016/j.anbehav.2017.02.017
Russell, A. F., Young, A. J., Spong, G., Jordan, N. R., and Clutton-Brock, T. H. (2007). Helpers increase the reproductive potential of offspring in cooperative meerkats. Proc. R. Soc. Lond. B Biol. Sci. 274, 513–520. doi: 10.1098/rspb.2006.3698
Santure, A. W., Stapley, J., Ball, A. D., Birkhead, T. R., Burke, T., and Slate, J. O. N. (2010). On the use of large marker panels to estimate inbreeding and relatedness: empirical and simulation studies of a pedigreed zebra finch population typed at 771 SNPs. Mol. Ecol. 19, 1439–1451. doi: 10.1111/j.1365-294X.2010.04554.x
Schutz, L., Stuart-Fox, D., and Whiting, M. J. (2007). Does the lizard Platysaurus broadleyi aggregate because of social factors? J. Herpetol. 41, 354–359. doi: 10.1670/0022-1511(2007)41[354:dtlpba]2.0.co;2
Smith, G. (1995). Home range size, overlap, and individual growth in the lizard, Sceloporus virgatus. Acta Oecol. 16, 413–421. doi: 10.2307/1441362
Smouse, P. E., and Peakall, R. (1999). Spatial autocorrelation analysis of individual multiallele and multilocus genetic structure. Heredity 82, 561–573. doi: 10.1038/sj.hdy.6885180
Stacey, P. B., and Ligon, J. D. (1987). Territory quality and dispersal options in the acorn woodpecker, and a challenge to the habitat-saturation model of cooperative breeding. Am. Nat. 130, 654–676. doi: 10.1086/284737
Stow, A. J., and Sunnucks, P. (2004). High mate and site fidelity in Cunningham’s skinks (Egernia cunninghami) in natural and fragmented habitat. Mol. Ecol. 13, 419–430. doi: 10.1046/j.1365-294x.2003.02061.x
Strickland, K., Gardiner, R., Schultz, A. J., and Frère, C. H. (2014). The social life of eastern water dragons: sex differences, spatial overlap and genetic relatedness. Anim. Behav. 97, 53–61.
Taborsky, B., and Oliveira, R. F. (2012). Social competence: an evolutionary approach. Trends Ecol. Evol. 12, 679–688. doi: 10.1016/j.tree.2012.09.003
Visagie, L., Mouton, P., Le, F. N., and Bauwens, D. (2005). Experimental analysis of grouping behaviour in cordylid lizards. Herpetol. J. 15, 91–96.
Wang, J. (2011). Coancestry: a program for simulating, estimating and analyzing relatedness and inbreeding coefficients. Mol. Ecol. Resour. 11, 141–145. doi: 10.1111/j.1755-0998.2010.02885.x
Wapstra, E., and Olsson, M. (2014). “The evolution of polyandry and patterns of multiple paternity in lizards,” in Reproductive Biology and the Phylogeny of Lizards and Tuatara, eds J. Rheubert, D. Siegel, S. Trauth, and B. Jamieson (Boca Raton, FL: CRC Press).
Ward, A. J. W., and Webster, M. (2016). Sociality: The Behaviour of Group-Living Animals. Cham: Springer International Publishing.
Waser, P. M., and Jones, W. T. (1983). Natal philopatry among solitary mammals. Q. Rev. Biol. 58, 355–390. doi: 10.1086/413385
Watson, G. S., Green, D. W., and Watson, J. A. (2020). Observations supporting parental care by a viviparous reptile: aggressive behaviour against predators demonstrated by the Cunningham’s skink. Aust. J. Zool. 67, 180–183. doi: 10.1071/zo20024
While, G. M., Chapple, D. G., Gardner, M. G., Uller, T., and Whiting, M. J. (2015). Egernia lizards. Curr. Biol. 25, R593–R595.
While, G. M., Jones, S. M., and Wapstra, E. (2007). Birthing asynchrony is not a consequence of asynchronous offspring development in a non-avian vertebrate, the Australian skink Egernia whitii. Funct. Ecol. 21, 513–519. doi: 10.1111/j.1365-2435.2007.01272.x
While, G. M., Uller, T., and Wapstra, E. (2009a). Family conflict and the evolution of sociality in reptiles. Behav. Ecol. 20, 245–250. doi: 10.1093/beheco/arp015
While, G. M., Uller, T., and Wapstra, E. (2009b). Within-population variation in social strategies characterize the social and mating system of an Australian lizard, Egernia whitii. Austral Ecol. 34, 938–949. doi: 10.1111/j.1442-9993.2009.02002.x
Whiting, M. J., and While, G. M. (2017). “Sociality in lizards,” in Comparative Social Evolution, eds D. R. Rubenstein and P. Abbot (Cambridge, MA: Cambridge University Press).
Keywords: lizard, mating system, monogamy, nuclear family, sociobiology, social organization, social structure, squamate reptile
Citation: Riley JL, Noble DWA, Stow AJ, Bolton PE, While GM, Dennison S, Byrne RW and Whiting MJ (2021) Socioecology of the Australian Tree Skink (Egernia striolata). Front. Ecol. Evol. 9:722455. doi: 10.3389/fevo.2021.722455
Received: 08 June 2021; Accepted: 22 November 2021;
Published: 10 December 2021.
Edited by:
Mark A. Elgar, The University of Melbourne, AustraliaReviewed by:
Erin Siracusa, University of Exeter, United KingdomJulian Evans, University of Zurich, Switzerland
Copyright © 2021 Riley, Noble, Stow, Bolton, While, Dennison, Byrne and Whiting. This is an open-access article distributed under the terms of the Creative Commons Attribution License (CC BY). The use, distribution or reproduction in other forums is permitted, provided the original author(s) and the copyright owner(s) are credited and that the original publication in this journal is cited, in accordance with accepted academic practice. No use, distribution or reproduction is permitted which does not comply with these terms.
*Correspondence: Julia L. Riley, jriley@mta.ca
†Present address: Julia L. Riley, Department of Biology, Mount Allison University, Sackville, NB, Canada; Peri E. Bolton, Department of Biology, East Carolina University, Greenville, NC, United States