Environmentally Modulated Repeat Evolution of Polymorphic Arctic Charr Life History Traits
- Freshwater Ecology Group, Department of Arctic Marine Biology, UiT The Arctic University of Norway, Tromsø, Norway
Sympatric Arctic charr, Salvelinus alpinus (L. 1758), morphs have flexible but repeated life history strategies tested across five Norwegian lakes. In several Scandinavian polymorphic Arctic charr populations differentiated by their diet and habitat use, a large littoral omnivorous (LO) morph commonly cooccurs with a smaller profundal spawning (PB/PZ) morph. A third, large piscivorous (PP) morph is also known to occur within a portion of Arctic charr populations in the profundal habitat along with the PB/PZ individuals. Life history traits, such as age at maturity, growth, and diet are known to differ among coexisting morphs. Notably, the PP morph was the longest morph with the oldest age at maturity while the PB/PZ morph showed the shortest lengths overall and youngest age with LO morph being intermediate in both traits. Growth parameters differed across all the morphs. When examining growth within morph groups, the LO morph was found to have different growth across all lakes, while similar reproductive investments and different energy acquisition patterns were seen within the PB/PZ and PP morphs. These results suggest repeat evolution in several life history strategies of reproductively isolated Arctic charr sympatric morphs, notably for the first time in the PP morph, while also highlighting the importance of the local environment in modulating life history traits.
Introduction
An organism’s life history strategy combines all traits that relate to its overall lifetime fitness (Stearns, 1976). The traits influencing lifetime fitness include, but are not limited to, age and size at maturity, growth rate, and fecundity (Stearns, 1976). Size and growth influences the timing of maturity in fishes (Roff, 1984; Perkins and Jager, 2011) and fecundity increases with fish size (Sandlund et al., 1992; Smalås et al., 2017) leading to tradeoffs in the timing of maturity and fecundity (Stearns, 1976).
Evolution is a constantly occurring process that results in individual opportunities for a species to adapt its life history strategy in relation to the currently experienced environmental conditions (Barton, 2007). In fishes, growth is indeterminate and therefore life history can be highly variable and related to the environment in which each fish grows (Wootton, 1990; Barton, 2007) and the exploited resources (Skúlason and Smith, 1995). In fishes, phenotypic plasticity and variation in traits, including life history related traits, are commonly exhibited (Gross, 1985; Ferguson et al., 1990; Wootton, 1990; Skúlason and Smith, 1995; Klemetsen, 2013; Muir et al., 2015; Chavarie et al., 2016; Meuthen et al., 2018; Rennison et al., 2019). Life history traits evolve, therefore local adaptations towards evolutionary stable strategies are seen among populations.
In general, salmonids demonstrate extreme plasticity in life history traits and extensive local adaptation (Klemetsen, 2010; Birnie-Gauvin et al., 2021). Within species variation in life history is exhibited in multiple members of the salmonid family, notably whitefish (Coregonus laveratus L. 1758), brown trout (Salmo trutta L. 1758), the Pacific salmons (Oncorhynchus spp.), and the charrs (Salvelinus spp.) (Hutchings and Morris, 1985; Crespi and Teo, 2002; Klemetsen, 2013; Muir et al., 2015; Birnie-Gauvin et al., 2021). Since their invasion of the Arctic in the late-Pleistocene, Arctic charr have demonstrated parallel evolution of a variety of traits across their entire distribution. Arctic charr demonstrate incredible diversity within the species (Klemetsen, 2010) and have even been referred to as being the most variable vertebrate on earth (Klemetsen, 2013). Within a single waterbody, up to four different Arctic charr morphs are known to co-occur (Skúlason et al., 1989a,1996; Snorrason et al., 1994; Østbye et al., 2020). The life history strategies adopted by the various sympatric morphs are all unique with variable age at maturity, growth, timing of spawning, diet, and habitat utilization. Across the species’ Holarctic distribution instances of polymorphic Arctic charr populations are common. The specific life history traits of each morph allows individuals to maximize fitness in their respective niche.
The variation in life history traits among co-occuring morphs have been described within multiple waterbodies. In Northern Scandinavia, the co-occuring morphs are commonly categorized by diet and habitat use with an increasing number of morphs often related to lake depth (Østbye et al., 2020). In the most simple case of polymorphism in Arctic charr, where two morphs are present within a system, there is a larger omnivorous feeding morph (LO) found in the littoral and pelagic zones and a second smaller benthic dwelling morph (PB/PZ) often in the deeper profundal zone within the lake (Hindar and Jonsson, 1982; Svedäng, 1990; Klemetsen et al., 1997; Moccetti et al., 2019). In general the LO morph is described as having greater lengths at age and delayed age at maturation relative to their small profundal (PB/PZ) conspecifics (Klemetsen et al., 1997; Smalås et al., 2013; Knudsen et al., 2015; Moccetti et al., 2019). Available estimates of the maximum attainable length [i.e., von Bertalanffy growth parameter for asymptotic length (L)] from the studied populations exceed 330 mm in the LO morph and are under 200 mm for the PB/PZ morph (Smalås et al., 2013; Knudsen et al., 2019). In instances where three morphs are present both the LO and PB/PZ morphs cooccur in addition to a piscivorous (PP) morph (Smalås et al., 2013; Moccetti et al., 2019). The PP morph occupies the same colder and profundal habitat as the PB/PZ morphs and is characterized as having an old age at maturity, long and slender body shape, and darker colouration (Smalås et al., 2013) typical of predatory fish. The presence of a fourth morph, often similar to the previously described PB/PZ morph, is less common and seems to be adapated to the local environmental conditions (Hindar and Jonsson, 1982, 1993; Jonsson et al., 1988; Skúlason et al., 1989b; Snorrason et al., 1994; Østbye et al., 2020).
This work aims to examine the repeatability in life history parameters of five polymorphic Arctic charr populations. Three of the studied lakes have bi-morphic Arctic charr with the presence of the LO and PB/PZ morphs while two lakes also include the third PP morph. Previous work has confirmed the parallel evolution in terms of genetic, trophic, and morphological phenotypes across some of the morph pairs included in this study (Knudsen et al., 2016; Saltykova et al., 2017; Moccetti et al., 2019). Based on the knowledge of the differing niches used by the various morphs we expect the morphs to differ in several life-history traits such as length distribution, age and size at maturity, and growth. We predict that the LO morph will show longer and older fish, greater growth, longer lengths- and greater ages at maturity relative to the profundal morphs (PB/PZ) in each lake. When the PP morph is present in the system it will show size distributions consisting of the oldest and largest individuals, with the slowest growth, the oldest ages- and longest lengths at maturity compared to the two other morphs present in the system. We predict the variation in the parameters describing life history for each morph type (LO, PB/PZ, and PP) to be greater among the morphs than the variation seen across all lakes.
Materials and Methods
Lake Description and Fish Collection
Arctic charr used in this study were sampled from five Norwegian lakes. Lake Limingen (64°42′13″N, 13°32′40″E) is located in central Norway in Trøndelag county while the remaining four lakes are located in Troms county in Northern Norway (Figure 1). These lakes have been described in other work (Klemetsen et al., 1997; Smalås et al., 2013; Knudsen et al., 2019; Moccetti et al., 2019) and the following is an overview of previous descriptions. Lakes in Troms county are typically ice covered from November/December to May/June and are described as being dimictic and oligotrophic (Klemetsen et al., 1997; Smalås et al., 2013; Moccetti et al., 2019). Lake Fjellfrøsvatn (69°05′08″N, 19°20′04″E) is part of the Målselv river system and has a surface area of 6.5 km2 and a depth of 88m (Klemetsen et al., 1997). Brown trout (Salmo trutta) is the only fish species other than the bimorphic Arctic charr (LO and PB) found in this lake (Klemetsen et al., 1997). The other lake containing two Arctic charr morps (LO and PZ) is Skøvatn (69°01′50″N, 17°52′25″E). This lake is connected to the marine environment and supports populations of partially anadromous Arctic charr and brown trout, and Atlantic salmon (S. salar L. 1758) (Moccetti et al., 2019). Skøvatn is 119 m deep at its deepest point and has an area of 6.2 km2 (Moccetti et al., 2019). Skogsfjordvatn (69°55′48″N, 19°9′36″E) is the largest of the northern lakes in the study with an area of 13.6 km2 and a maximum depth of approximately 100 m. It contains, in addition to the trimorphic Arctic charr (LO, PB, and PP) with few anadromous individuals, brown trout, Atlantic salmon, three-spined stickleback (Gasterosteus aculeatus L. 1758) and some European eel (Anguilla anguilla L. 1758) (Smalås et al., 2013). Tårnvatn (69°18′43″N, 18°21′29″E) has a population of trimorphic Arctic charr (LO, PB, and PP) and brown trout and is 53 m deep and 3.21 km2 (Moccetti et al., 2019). Lake Limingen has a surface area of 95.7 km2 and a maximum depth of 192 m. It is described as being dimictic and oligotrophic and contains in addition to the trimorphic Arctic charr (LO, PB, and PP), brown trout, minnow (Phoxinus phoxinus L. 1758; introduced in 1980s), and three-spined stickleback (Knudsen et al., 2019).
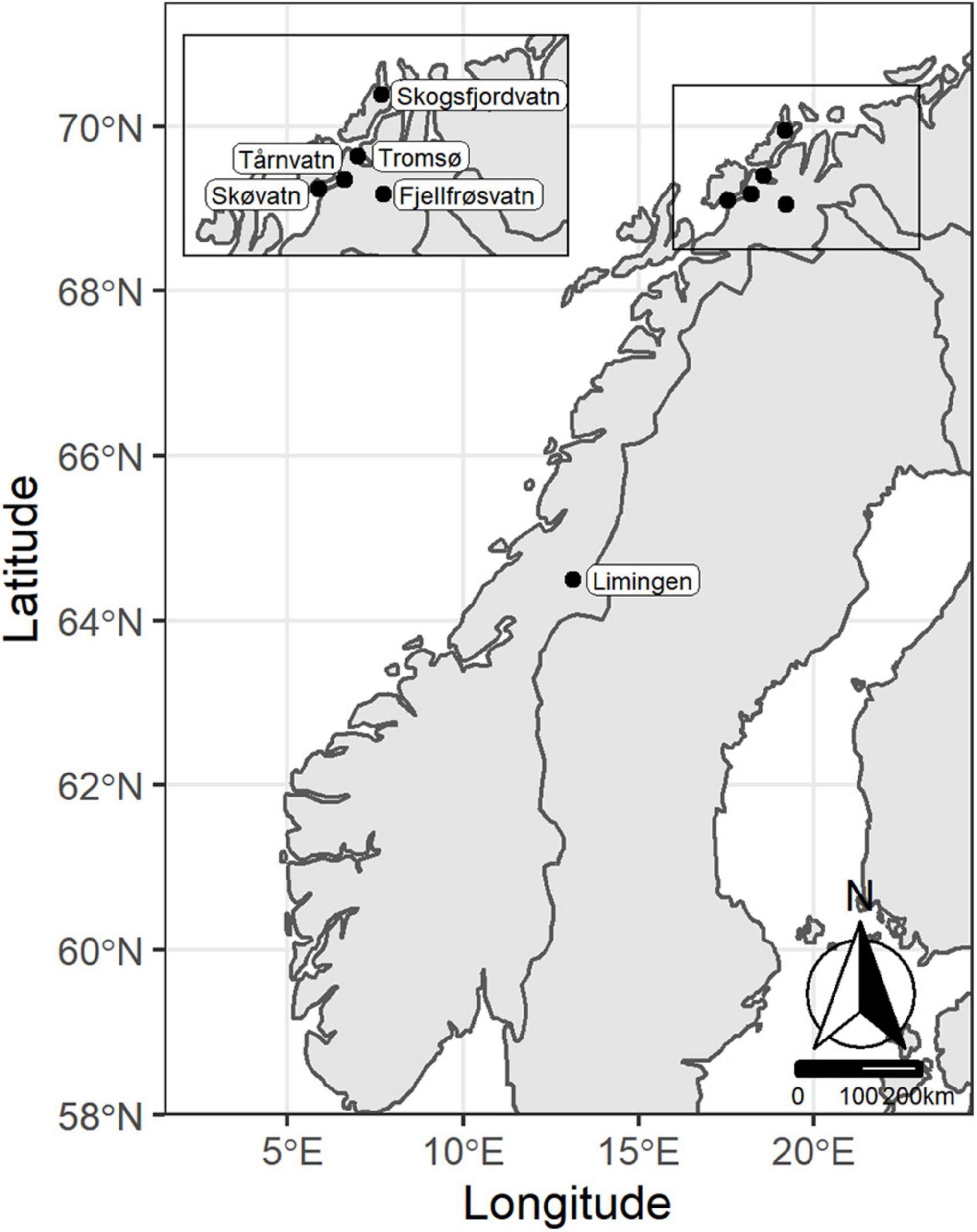
Figure 1. Map of five Norwegian lakes with polymorphic Arctic charr populations used in the present study. Map prepared using the packages ggplot2 (Wickham, 2016), sf (Pebesma, 2018), ggsn (Santos Baquero, 2019), and ggspatial (Dunnington, 2021) in R (R Development Core Team, 2018) using the RStudio (RStudio Team, 2020) interface with map queried from the package rnaturalearth (South, 2017) using the ne_countries function.
Fish were sampled using survey gillnets of 1.5 × 40 m dimensions which were made up of eight 5 m panels with mesh sizes of 10, 12.5, 15, 18, 22, 26, 35, and 45 mm and Nordic multi-mesh gill-nets of 12 2.5 m panels with mesh sizes from 5 to 55 mm. Additionally, a series of standard nets of 1.5 × 30 m dimensions with mesh sizes of 8, 10, or 12.5 mm were used in profundal and littoral habitats. Gillnets were placed at varying depths in the littoral (<15 m) and the profundal (>20 m) habitats. Additional survey nets placed in the pelagic habitat measured 6 × 40 m with mesh sizes of 8, 10, or 12.5 mm [further details in Klemetsen et al. (1997), Smalås et al. (2013), Smalås et al. (2017), Knudsen et al. (2019), and Moccetti et al. (2019)]. A pelagic pair trawl was used additionally to nets in lake Limingen which caught 63 LO Arctic charr [see details in Sandlund et al. (2017)].
Fish processing consisted of obtaining length (mm, fork length) and weight (g) measurements. Gonads were assessed for sex and maturity status. The key used for maturity status varied among datasets compiled for this study where some used a seven-stage scale and others a 3 stage-scale. Maturity status was re-coded using a 2 stage-scale for comparability as 1 for immature individual, where there is no indication of reproductive maturity in the year of sampling, or 2 for mature individuals. Mature individuals include those where gonads are either developed enough for spawning in the coming reproductive season and individuals with evidence of having already spawned in the sampling year or previous years. Sagittal otoliths were extracted and preserved in ethanol for later surface otolith readings in glycerol for age estimates of each individual fish. Each fish was assigned to their respective morph based on visual inspection of head and body morphology, maturation status, and colouration (Smalås et al., 2013; Skoglund et al., 2015). Genetic analyses on a subsample of the data demonstrated reproductive isolation between morphs and that visual classification of morph type is effective (Præbel et al., 2016; Simonsen et al., 2017; Moccetti et al., 2019).
Data Analyses
In all modeling, data exploration to assess the suitability of data to the statistical tests and to ensure no assumptions were violated was performed using a protocol heavily relying on data visualization using graphical plotting methods (Zuur et al., 2010). All analyses were conducted in R-4.1.0 (R Development Core Team, 2018) using the R studio (RStudio Team, 2020) interface and various packages. All figures were plotted with the use of the ggplot2 package (Wickham, 2016).
Length at Age
Data exploration using coplots prior to modeling fish length at age provided evidence for a morph-age and lake-age interaction. An ANCOVA was an unwise statistical choice as it would assume an equal effect of age for all morphs and in all lakes. Boxplots showed that the PB/PZ morph had a lower variance relative to the two other morphs. A generalized least squares [gls function nlme package version 3.1-152 (Pinheiro et al., 2019)] approach was selected for model building as it permitted the incorporation of the heterogeneity of variance within the final model. Data exploration using coplots suggested that variance should increase with age and could also potentially be variable among morphs or lakes. Age was included in the model as a continuous variable while lake and morph were included as categorical variables. Akaike’s information criterion (anova function, stats package (R Development Core Team, 2018)) was used to evaluate potential models and the one with the lowest AIC value was retained as final model.
Growth Modeling
Although the von Bertalanffy growth model is often used in growth modeling of fishes, including Arctic charr, one of the main critiques of the model is that it has very little biological significance (Quince et al., 2008; Boukal et al., 2014). The biphasic model is built upon theory relating to metabolism and energetics; therefore the estimated terms relate to energy taken up from the environment and the organisms’ conversion of that energy into growth and/or reproduction. The biphasic model used here assumes that immature individuals invest the entirety of their energy towards growth, however, the proportional investment in growth is reduced once an individual reaches sexual maturity. We assume here that the investment in reproduction is constant in the adult stage. The equation for the biphasic growth model is as follows:
where, H = c(1−β)b−(1−β) and R = (1q−1(1−β)r)−1 (Boukal et al., 2014). In the model: β= 0.7 (Jobling, 1983) and is the scaling exponent for the relationship between food consumption and growth in weight; α is a scaling exponent describing the relationship between length and weight calculated for each population; L is the length (in mm); q = 1, this term is the correction for the difference in energy requirement for production of gonadal tissue vs somatic tissues which was assumed to be equal in the present study; t represents the age of the fish with tmat being the age at maturity for the population; c is estimated and informs us about the environment through representing the energy available to the organism for growth; r is estimated and informs us on the energy consumed which is diverted to reproduction.
Age at maturity was calculated using a cumulative distribution function (i.e., ogive) where the point of 50% maturity was used. All individuals with an age lower than the age at maturity were assessed with a model using r = 0. Length at age zero (i.e., model intercept) is unknown for our populations and was estimated to be 45mm for LO and PP morphs and 42mm for PB/PZ morphs (Frost, 1965). Model parameters c and r were estimated for each morph within each lake using a non-linear least-squares estimation with the nls function from base R. Models were evaluated using an analysis of residual sum of squares (AoRSS; also called extra sum-of-squares; Chen et al., 1992; Haddon, 2011; Ogle, 2016) to determine if the modeled curves differed among morphs within lake and within morph among lakes. If curves were determined to differ, further analyses of pairwise comparisons using likelihood ratio tests (Kimura, 1980; Haddon, 2011; Ogle, 2016) were performed to determine which of the parameters, r or c, differed in the comparison in question. This test compares whether the difference in the terms is significant between pooled and unpooled samples. Significance was corrected by dividing 0.05 by the number of comparisons, 20 in the case of LO and PB/PZ morphs (α0.0025), and 6 in the case of the PP morph (α0.0083).
A50 and L50
Age (A50) and Length (L50) where 50% of the population have reached maturity were calculated using a logistic regression (glm function, stats package base R (R Development Core Team, 2018)). Sexes were pooled for the analysis as sample size of groups (morph within lake) were small (e.g., only 7 mature individuals of the PP morph in Limingen). Data exploration with coplots suggested interactions between lake, age, and morph. Model selection was performed with the drop1 (stats package base R (R Development Core Team, 2018) command, starting from the most complex model, to evaluate if removing terms resulted in a statistically similar fit (Zuur et al., 2009). Once the model was fit, the estimate of age or length where 50% of the population reached maturity was calculated by dividing the intercept by the slope for each morph within each lake (Collett, 1991).
Results
Of the five lakes included in this study two lakes contained two Arctic charr morphs and three lakes were composed of three morphs. In the LO (N = 2741) morph age ranged from 1 to 16 years, length from 64 to 494 mm, and weight from 2.2 to 1220 g (Table 1). The PB/PZ morph (N = 353) varied in age from 1 to 13 years, length from 72 to 272 mm, and weight from 3.4 to 231.1 g (Table 1). Age, weight, and length in the PP morph (N = 233) ranged from 2 to 17 years, 99 to 517 mm, and 8.2 and 1668 g, respectively (Table 1).
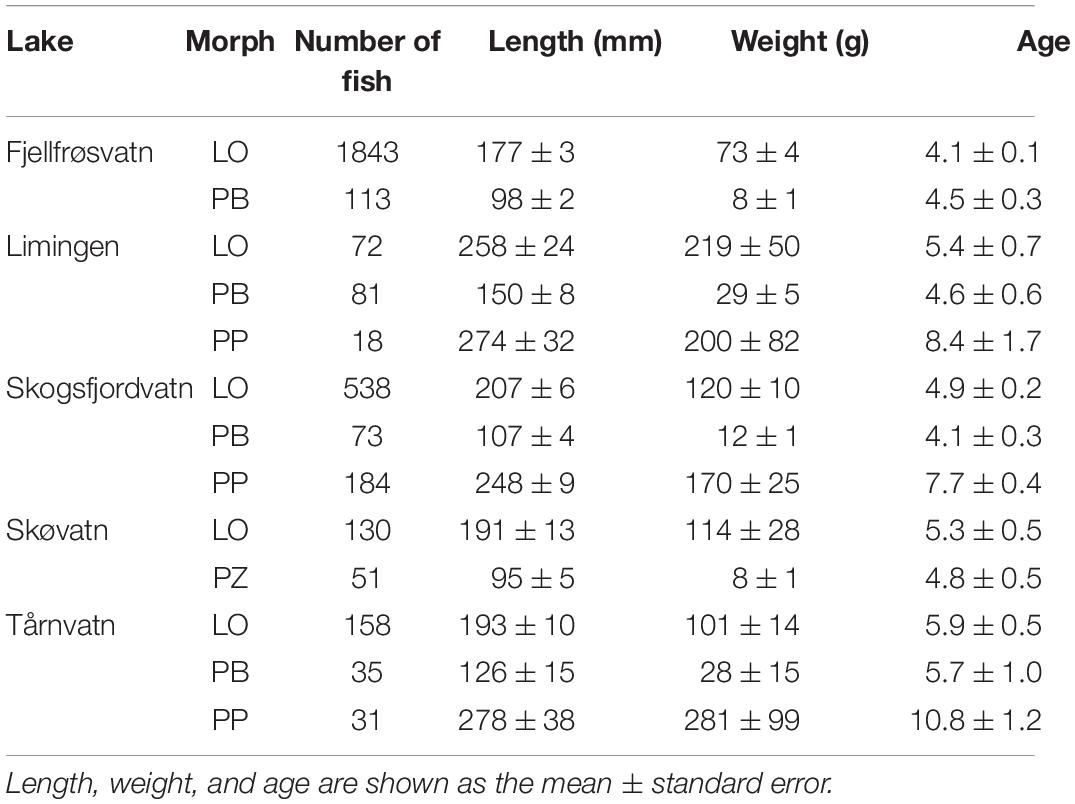
Table 1. Metadata and sample size of Arctic charr samples from five Norwegian lakes used in the research.
Length at Age
The analysis for fish length at age included individuals (N = 3327) across the five lakes and of the three possible morphs that had complete information on age and length. The AIC indicated that the model with a variance structure that allowed for different variances for each morph, with the PB/PZ morph having the lowest variance and the PP morph the highest variance (σ LO = 1.00, PB/PZ = 0.56, and PP = 1.11), while also allowing for an increase in variance with increasing ages (δ = 0.14) best described the data (Supplementary Table 1). The final model did not use sex as a predictor (χ2(1) = 2.50,p = 0.1135) therefore males and females had similar length at age. The final model was as follows:
The estimated coefficients and standard errors of the fixed components of the model are shown in Supplementary Table 2. The residual standard error of the model is 14.09545.
Fish length increased with age (estimate 27.50, p < 0.001) for all groups, and the PB/PZ (estimate 7.20, p = 0.005) and PP (estimate 28.58, p < 0.001) morphs had larger intercepts relative to the LO morph. The effect of age on length, however, differed across morphs (Figure 2 and Supplementary Table 2). The positive effect of age on length was lower in morphs PB/PZ (estimate −20.31, p < 0.001) and PP (−8.78 p < 0.001) relative to LO which relates to growth patterns presented further in the results section. Within all morph groups, charr from Tårnvatn (estimate −1.87, p < 0.001) had the shortest lengths at age while Limingen had the highest lengths at age (estimate 7.55, p < 0.001; Figure 2).
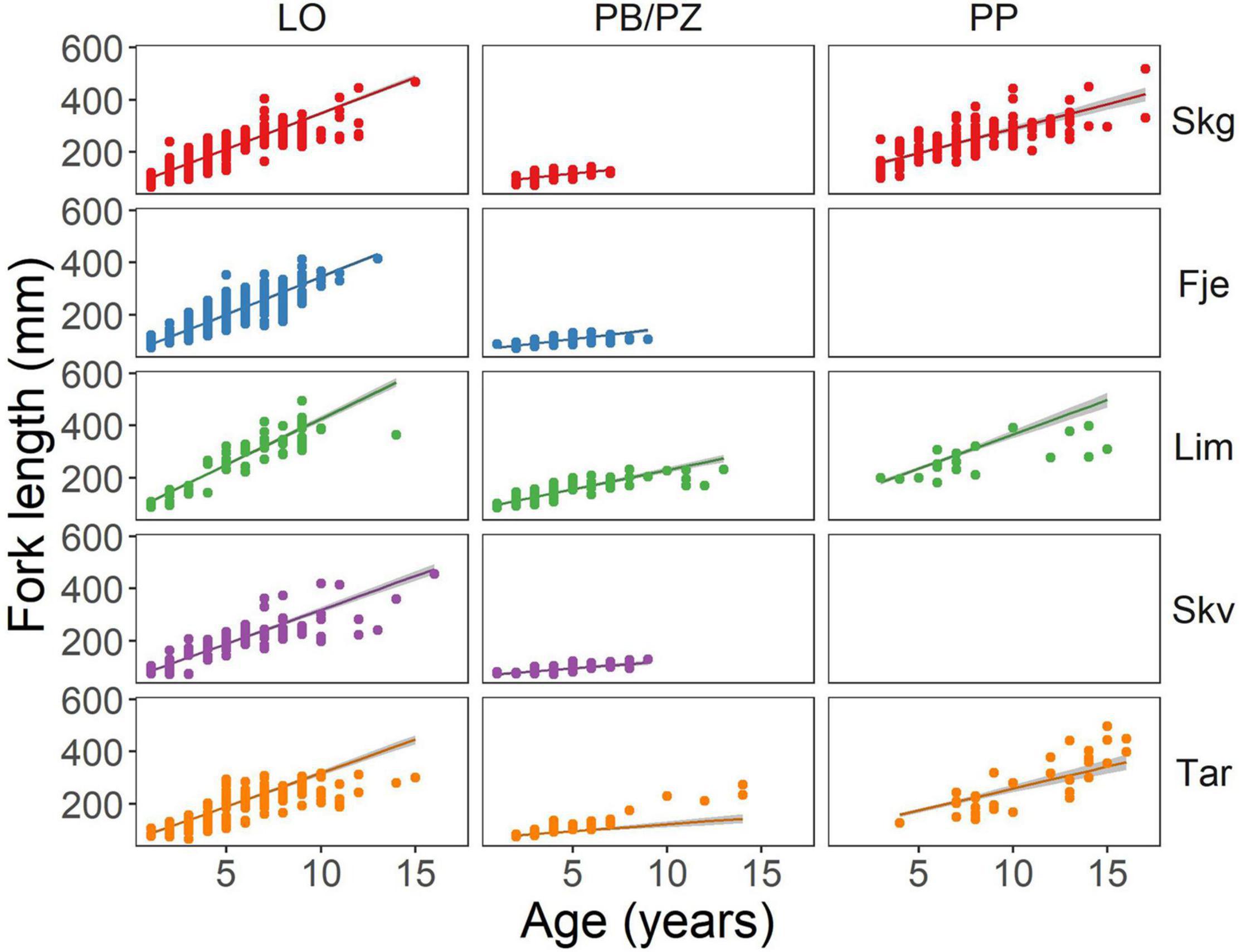
Figure 2. Scatter plot of Arctic charr fork length (mm) at age for each morph type within each lake included in the study. The model is represented by a line with shading representing the 95% confidence interval. Skg, Skogsfjordvatn; Fje, Fjellfrøsvatn; Lim, Limingen; Skv, Skøvatn; and Tar, Tårnvatn.
Growth Modeling
The biphasic growth model, unlike the Von Bertalanffy growth model, incorporates biologically significant parameters. Estimated model parameters r, relating to reproductive investment, and c, related to energy acquisition, for each population along with the reference equation parameters used for the likelihood ratio tests are found in Table 2. The initial tests of curve coincidence, with AoRSS, informed us that there are differences in parameter estimates within each comparable morph (Figure 3), and within each lake (Table 3). Further pairwise comparisons using a likelihood ratio approach informed us which parameters, r and/or c, differed and between which lake/morph. The estimates for c in the LO morph ranged from 1.28 to 2.04 and for the parameter r the range was from 0.13 to 0.41. In the LO morph, c was unique between each lake, whereas r did not differ between LO individuals from the following lake pairs: Skogsfjordvatn and Tårnvatn, Skogsfjordvatn and Limingen, Skogsfjordvatn and Skøvatn, and Skøvatn and Limingen (Tables 2, 4). For the PB/PZ morph in Skogsfjordvatn, Skøvatn, and Fjellfrøsvatn no differences were found in the parameter c which ranged from 0.57 to 2.30 across all lakes (Tables 2, 4). In the PB/PZ morph the parameter r, relating to reproductive investment, ranged from 0.01 to 0.98 and was similar across most pairs while only differing between Limingen and Skogsfjordvatn, Limingen and Skøvatn, and Limingen and Tårnvatn (Tables 2, 4). Finally, the only difference in parameters in the PP morph was between Tårnvatn and Limingen for the parameter c which ranged from 0.92 to 1.43 (Tables 2, 4). The parameter r in the PP morph ranged from −0.05 to 0.31 and did not differ among the three lakes (Tables 2, 4).
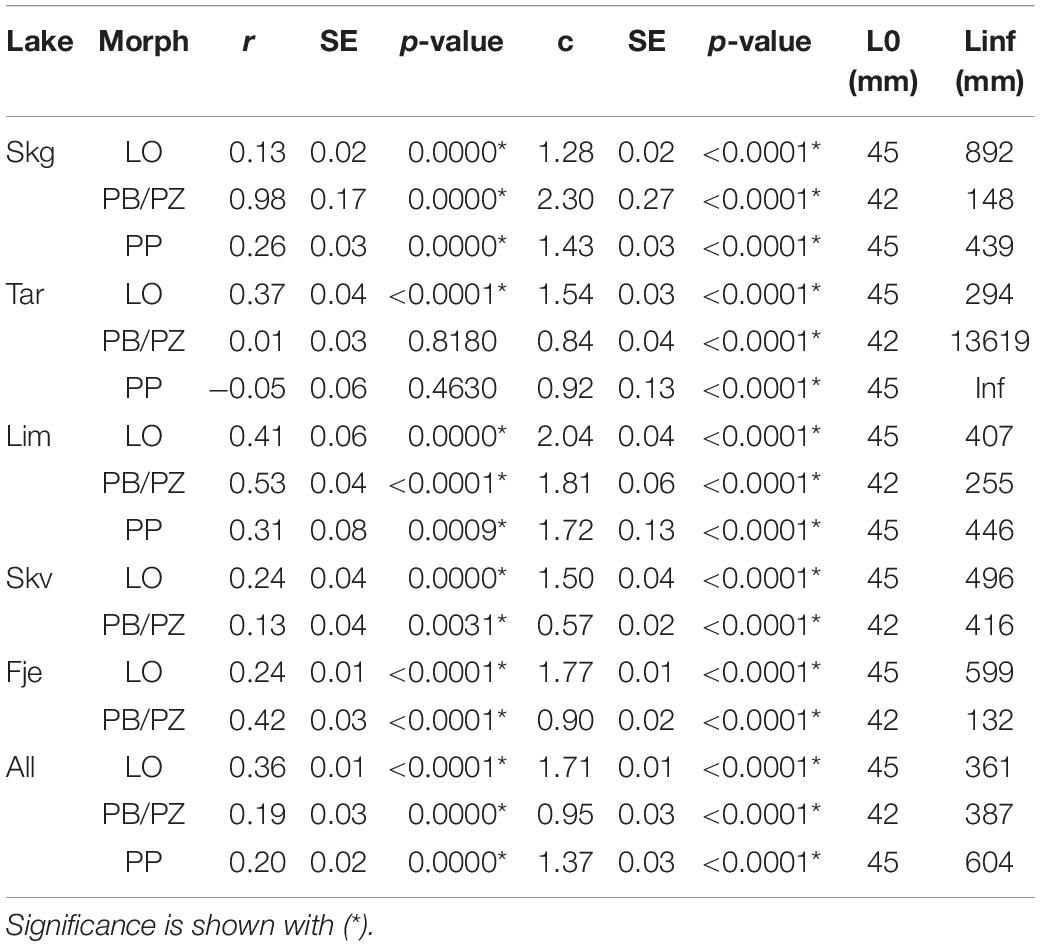
Table 2. Estimated coefficients and their standard error for parameters r and c of the biphasic growth model along with the L0 value used the estimating of each curve for Arctic charr morphs (LO, PB/PZ, and PP) from five Norwegian lakes.
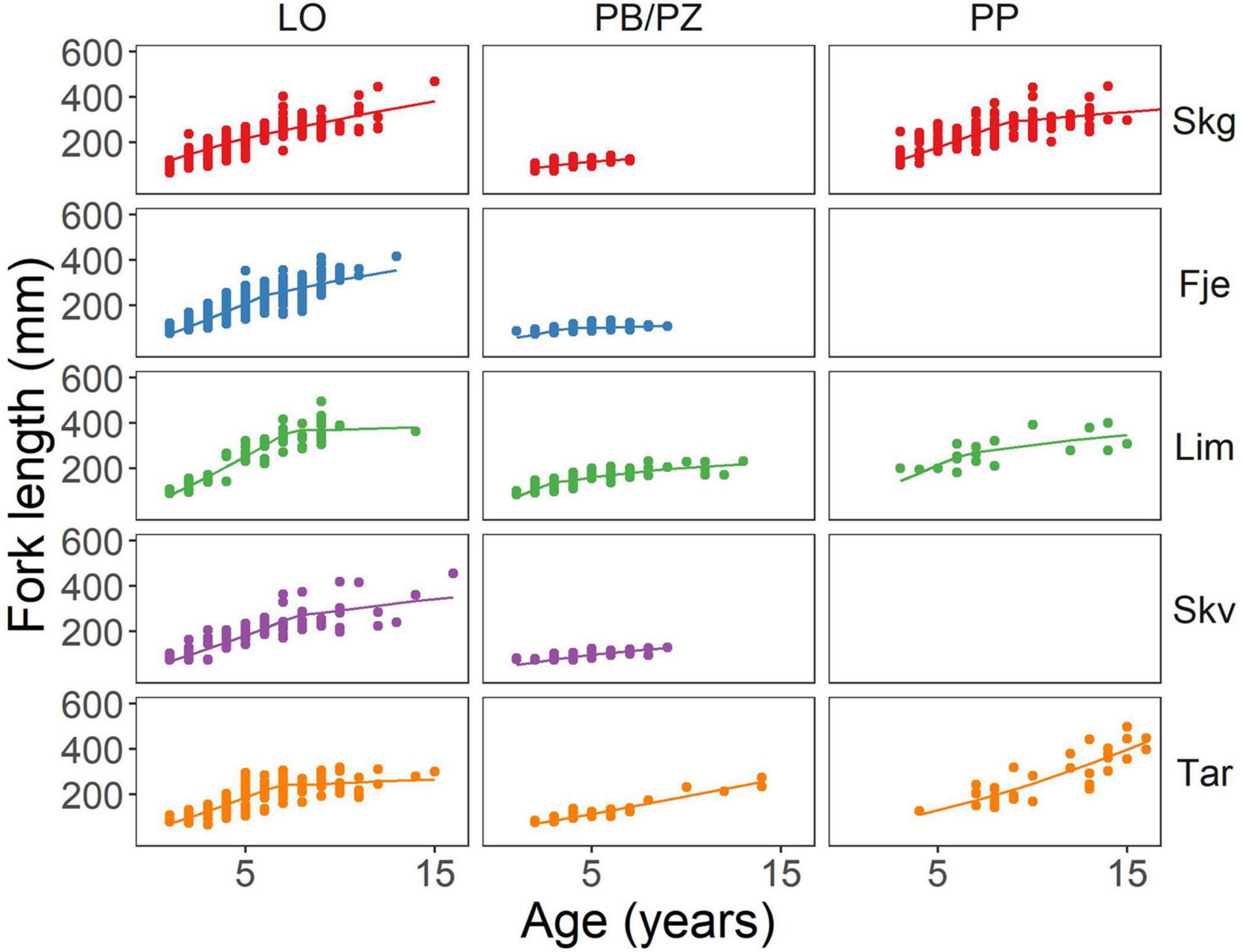
Figure 3. Biphasic growth model-based length at age estimates of three Arctic charr morphs in the studied lakes. The point of inflection in the model indicates the age where energy is diverted from somatic growth to reproductive investment. Skg, Skogsfjordvatn; Fje, Fjellfrøsvatn; Lim, Limingen; Skv, Skøvatn; and Tar, Tårnvatn.
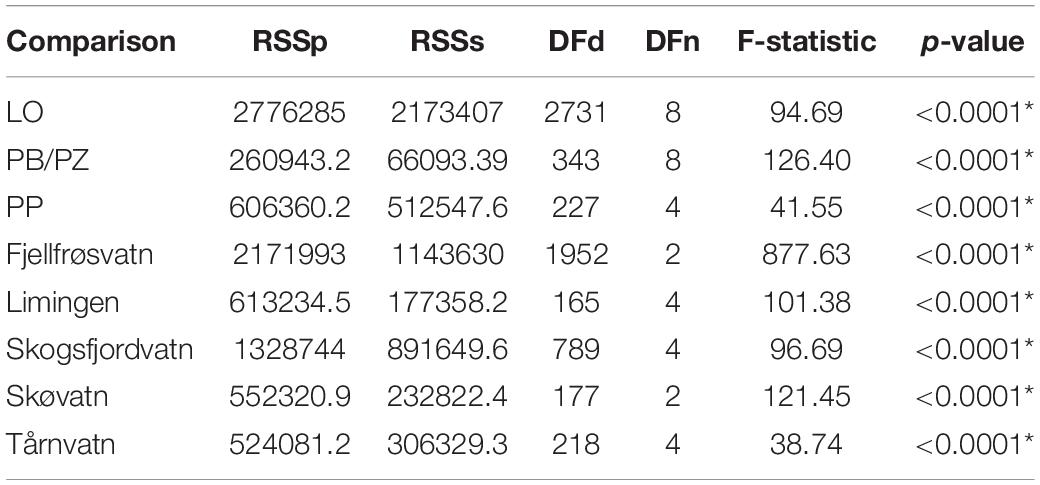
Table 3. Analysis of residual sum of squares comparing the overall incidence of curves modeled by the bi-phasic growth model within morph type across all lakes (LO, PB/PZ, and PP) and among morphs within lake.
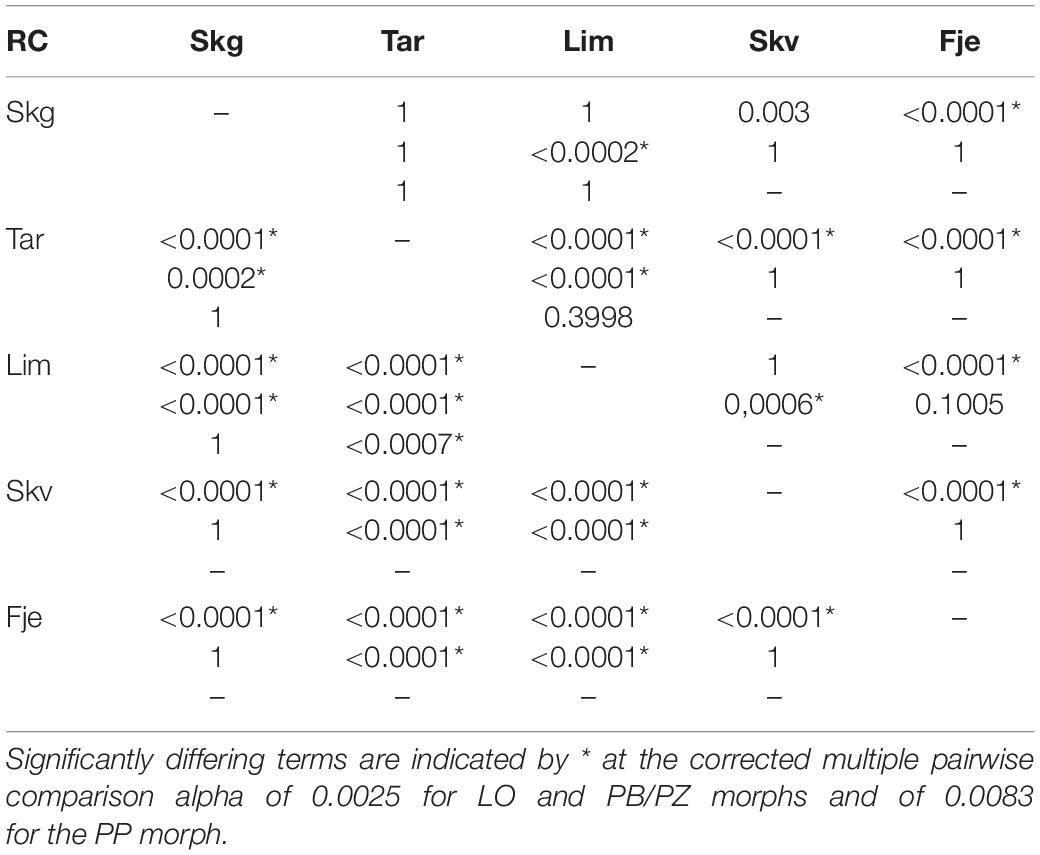
Table 4. Likelihood Ratio Test p-values from pairwise comparisons testing for differences when parameters r or c were kept constant in the LO morph (top), PB/PZ (mid), and PP (bottom).
A50 and L50
Age where 50% of the population has matured (hereafter age at maturity) was estimated using all individuals with complete information on age or length (N = 3323), and maturity. Age and length at 50% maturity were influenced by the combination of factors morph type (LO, PB/PZ, and PP) and lake. The equation for the model used was as follows where A50 and Age are replaced by L50 and Length, respectively, when determining the length at maturity:
The estimates of the model coefficients for A50 are found in Supplementary Table 3. The probability of being mature increased with age (estimate 1.49, p < 0.001). The general trend in the age at maturity across lake was that the PB/PZ morph had the lowest age at maturity, the LO an intermediate age at maturity and the PP morph the highest age at maturity (Figure 4, Supplementary Table 3, and Supplementary Figure 1). However, in some lakes, pairs of coexisting morphs may have very similar ages at maturity, notably in Skøvatn where the LO and PB/PZ morphs had an estimated A50 of 5.8- and 6.0 years, respectively, while in Tårnvatn which had an estimated age at maturity of 6.9 years for the LO and 7.5 years for the PP morph (Figure 4 and Supplementary Table 4). Age influenced the probability of maturing differently in the various morphs. The positive effect of age on probability of reaching maturity was reduced in the PP morph relative to the LO morph (estimate −0.88, p < 0.00, Supplementary Table 3) where the PP morph (overall mean 7.3 years) reached maturity at older ages relative to LO morph (overall mean 6.3 years). Individuals in lakes Tårnvatn and Skøvatn reached sexual maturity at higher ages relative to individuals in Skogsfjordvatn (estimates −0.83, −1.11, respectively, p < 0.01, Supplementary Table 3). Of note, the age morph interaction in Tårnvatn was modified (estimate 0.71, p < 0.02, Supplementary Table 3) where individuals of the PP morph (7.5 years) reached maturity at a lower age relative to individuals of the PP morph in Skogsfjordvatn (8.3 years). The PB/PZ morphs in lake Skøvatn and Tårnvatn had a further reduction in the probability of attaining sexual maturity (estimate −5.03, −14.29, p < 0.02, 0,03, respectively, Supplementary Table 3), therefore matured at an older age (6.0- and 5.9 years, Supplementary Table 4) relative to the PB/PZ morph of lake Skogsfjordvatn (2.6 years, Supplementary Table 4).
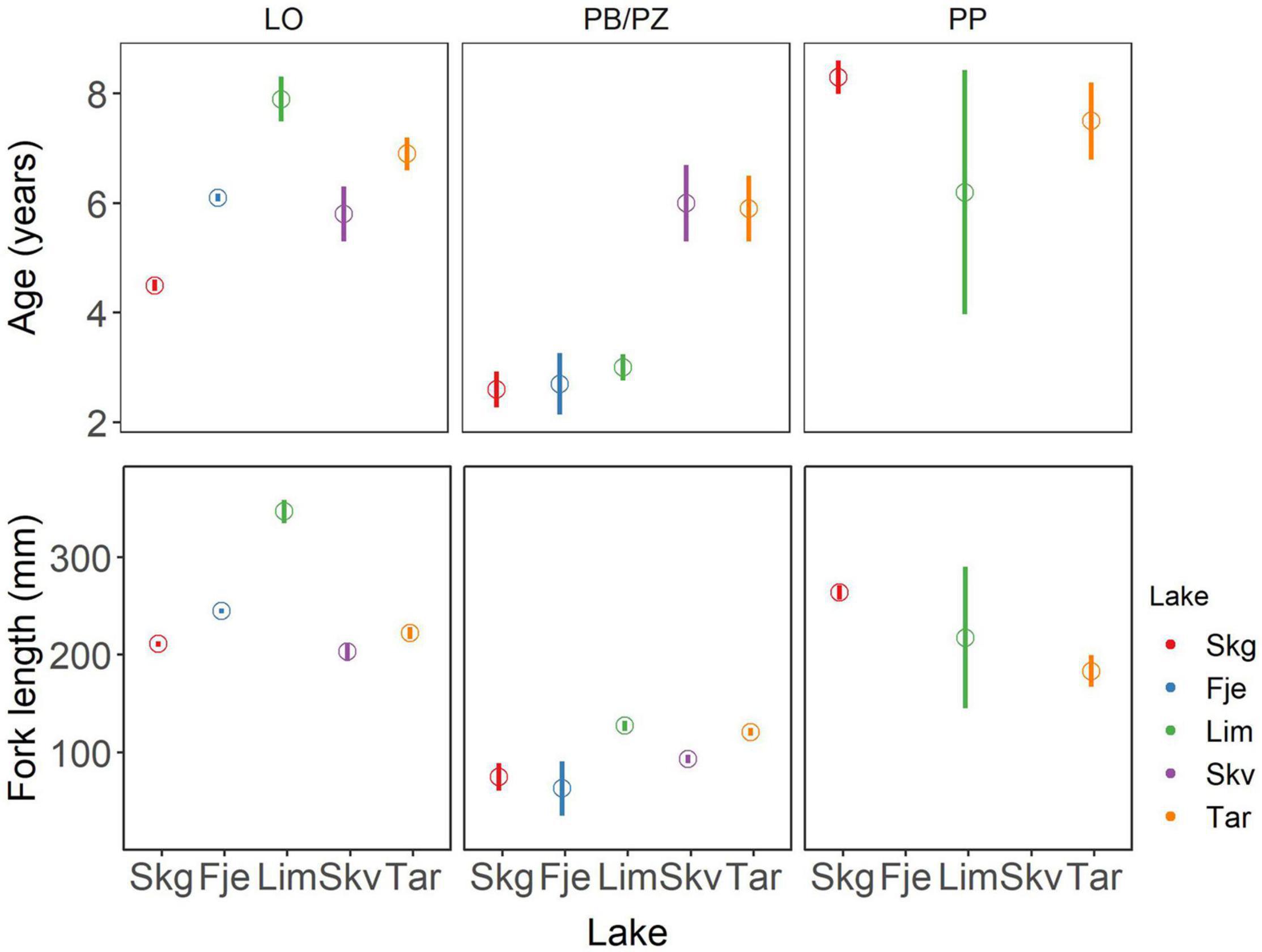
Figure 4. Age and length where 50% of the population is mature in three Arctic charr morphs from the 5 studied lakes. Open circles represent the estimated obtained from logistic regression with standard error. Skg, Skogsfjordvatn; Fje, Fjellfrøsvatn; Lim, Limingen; Skv, Skøvatn; and Tar, Tårnvatn.
The coefficient estimates for length where 50 percent of the population have reached sexual maturity (hereafter length at maturity) are found in Supplementary Table 6. Length had a positive effect on the probability of maturing where, as a fish grows longer it increased its probability of reaching sexual maturity (estimate = 0.06, p < 0.001, Supplementary Table 6). The probability of maturing was increased in the PB/PZ (estimate = 9.35, p < 0.001, Supplementary Table 6) and PP (estimate = 6.31, p < 0.01, Supplementary Table 6) morphs, therefore these morphs reached sexual maturity at shorter lengths relative to the LO morph (Figure 4, Supplementary Figure 2, and Supplementary Table 7). The positive effect of length on the probability of maturing was lower in the PP morph, which resulted in a greater length at maturity in the PP morph (estimate −0.04, p < 0.001, Supplementary Table 6) relative to LO.
Generally, the probability of reaching sexual maturity in all individuals from the lakes Fjellfrøsvatn, Skøvatn, and Tårnvatn was increased (i.e., maturity at shorter lengths) relative to individuals from lake Skogsfjordvatn (Supplementary Table 6). However, a negative interaction between lake and fish length was present in all lakes resulting in greater length at maturity relative to Skogsfjordvatn (Supplementary Table 6). In general, individuals from Limingen were longer at sexual maturity compared to those from Skogsfjordvatn (estimate −0.02, p < 0.04, Supplementary Table 6). Individuals of the PB/PZ morph in Tårnvatn (L50 = 104 mm) Skøvatn (L50 = 102 mm) were longer at maturity than those of Skogsfjordvatn (L50 = 75 mm) through interactions between morph and lake (estimate = −15.78, −13.52, repectively, p < 0.01, Supplementary Table 6), and further in Skøvatn through an interacion among morph, lake, and length (estimate 0.08, p = 0.02, Supplementary Table 6).
Discussion
This study provides evidence from Norwegian lakes supporting the repeated evolution in life history traits of the Arctic charr morph complexes. Distinct populations within each waterbody had differentiation in the analyzed life history traits and corresponding morphs among lakes demonstrated similar patterns in life history parameters. The analysis of length at age showed that the distinct morphs have different patterns and that these patterns show consistencies in the comparable morphs across lakes. Further analyses of growth patterns demonstrated that all populations adopt unique growth trajectories while also highlighting the importance of ontogenetic niche shifts and the investment in reproduction in the differentiation of the morphs. Finally patterns in age and size (length, mm) at maturity supported the results from the reproductive investment in the biphasic growth modeling with the PB/PZ morph having the youngest age at maturity and the PP morph delaying maturation to older ages.
Fish length at age followed the expected pattern with the PB/PZ morph being shortest, the LO morph being longer, and the PP morph, when present, exceeding the length of the LO morph. This study corroborates that the PB/PZ morph is often referred to as a “dwarf” (Hindar and Jonsson, 1982; Svedäng, 1990; Klemetsen et al., 1997). The PB/PZ morph is also described as being paedomorphic (Skoglund et al., 2015; Simonsen et al., 2017), which is retaining their juvenile traits into adulthood. Our results are consistent with these often used (although, rightfully so, less in more recent literature) anthropomorphic terms. When the third PP morph is present, individuals of this morph are on average the longest and oldest fish of all the morphs. This is consistent with multiple studies reporting length in polymorphic Arctic charr populations (Snorrason et al., 1994; Klemetsen et al., 1997; Smalås et al., 2013; Sandlund et al., 2017). However, we determined that at a given age, the LO morph is longer than the PP morph of the same age. Although with a relatively slower growth, the PP morph is able to outgrow their LO counterpart through a higher estimated asymptotic length and greater longevity (Smalås et al., 2013).
Examinations of somatic growth in this study demonstrated that there is high variation in growth within the various studied Arctic charr populations. The sympatric morphs had significantly different growth trajectories which is in accordance with other studies (Smalås et al., 2013; Knudsen et al., 2015, 2019). We determined that all sympatric morphs have different growth trajectories but did not further examine how the model parameters differed among the three morphs as the aim here was to examine repeatability of evolution within comparable morphs. Within similar morph groupings, we determined that the LO morph across all lakes has a very large variation in the estimated parameters for both energy acquisition and reproductive investment. The parameter c, relating to the energy acquired, ranged from 1.28 to 2.04 and was found to be different in all pairwise comparisons across lakes. When comparing the morphs while disregarding the lakes, we see that the LO strategy is a high investment in both growth and reproduction. The growth strategy adopted by the LO morph seems to be quite broad, however. This suggests heterogeneity among the environments along with local adaptation. The limnological descriptions of the studied lakes are relatively comparable, however, it is likely that the LO morphs within the different lakes focus their realized niche differently related to environmental heterogeneity as Arctic charr is known to have specialist individuals who restrict themselves to narrow realized niches (Amundsen, 1995). The lakes studied here contain differing number of species, including brown trout which is a competitor and predator of Arctic charr, therefore imposing varying pressures on Arctic charr niche (Prati et al., 2021). Of interest would be to assess the growth of the morphs in relation to environmental variables, such as lake size (Eloranta et al., 2015), latitude (Chavarie et al., 2010), temperature (Knudsen et al., 2015), and/or competition (Prati et al., 2021), to assess the contribution of the environment to the realized niche of Arctic charr morphs. Among the pairwise comparisons examining the LO morph’s investment in reproduction few similarities (4 of 10) were found, highlighting the breadth in the LO morphs life history strategy.
Growth within the PB/PZ morph had more similarities than the parameters of the growth modeling of the LO morph. Three of 10 pairwise comparisons did not differ in the parameter c while only two differed for the parameter r in the PB/PZ morph. A similar pattern was seen in the PP morph where the populations in the different lakes did not differ in reproductive investment with only one pairwise comparison differing for the energy acquisition term. Similarities in the parameter accounting for energy acquired from the environment were expected as both the PB/PZ and PP morphs occupy a narrower realized diet and habitat niche relative to the LO morph. The PB/PZ morph is known to have a more specialized diet than their generalist LO counterparts (Sandlund et al., 1992; Knudsen et al., 2019; Moccetti et al., 2019) while the PP morph mostly consumes fish prey at least as adults (Amundsen, 1994; Skoglund et al., 2015; Knudsen et al., 2016, 2019). The only difference found when comparing growth parameters in the PP morph was in the term for energy taken from the environment (c) where PP individuals from Tårnvatn have a lower abundance of fish in the stomach content than the PP morph in Limingen (Knudsen et al., 2019; Moccetti et al., 2019). Tårnvatn is the only lake supporting trimorphic Arctic charr in the absence of small fish species, such as minnow and stickleback. Piscivorous fish in Tårnvatn may only prey upon smaller salmonids and must therefore supplement their diet with other food sources and potentially expanding their niche to overlap with the other morphs (Moccetti et al., 2019). Indeed, the reproductive isolation between the two profundal morphs in Tårnvatn is not complete (Moccetti et al., 2019). The narrow niches occupied by each morph leave little to no opportunities for individual specialists within the PB/PZ and PP population’s niche (Amundsen, 1995) resulting in very similar energy acquisition potential among lakes. These results suggest that Arctic charr can specialize within various niches (Prati et al., 2021) even within what we consider comparable morphs. However, the constraints put on by the narrowed niche seem to influence the reproductive tradeoffs in a similar manner within the morph types.
Once an individual matures, it is assumed that the investment in somatic growth is reduced, and energy is directed towards the production of gonads. Our results corroborate this assumption as growth slowed down significantly following the onset of maturation in all populations with the exception of the PP morph in Tårnvatn. We suppose that this is related to the change in diet to a piscivorous life style as ontogenetic niche shifts influence growth rate, with a piscivorous diet being a very efficient food source (Barton, 2007), which would explain the negative value for r in the biphasic growth model. In the case of Tårnvatn, where only salmonids are present, a shift to a piscivorous diet would occur at a larger size than in the other lakes in this study which contain smaller fish species in addition to the salmonids. Ontogenetic niche shifts occur when the cost of the current preferred prey outweighs the benefits of this prey source (Forseth et al., 1994). Prey size is related to the predator’s size therefore we would predict that small individuals, notably juveniles, would share more similarities in behavior (i.e., predator avoidance), and trophic position and feeding preference (Amundsen et al., 2008; Skoglund et al., 2015; Knudsen et al., 2019; Moccetti et al., 2019). These similarities would be reduced as individuals of each morph age, become larger, and shift to their “adult niche” (Moccetti et al., 2019). Individuals of the PP morph are known to have very low growth during their early years (Smalås et al., 2013) likely related to the poorer performance of their piscivorous jaw morphology (Saltykova et al., 2017) on benthos, especially in competition with the PB/PZ morph, and their use of the colder profundal habitat. Their eventual size related shift to a piscivorous diet (Amundsen, 1994; Moccetti et al., 2019) along with their relatively long life span shown here likely compensate for their slow start. For the first time, repeatability in life history traits of the PP morph across multiple lakes is substantiated here.
The expected trends in age and length at maturity were observed in the study with the PB/PZ morph having the youngest age and shortest length at maturity. There were clear indications in tradeoffs in timing of reproduction and fecundity based on the timing of the onset of maturity in the studied populations with the PB/PZ morph maturing earliest. By adopting a fast life history and reproducing early at a relatively smaller size, the PB/PZ morph can avoid predation before their first reproductive event whilst the other morphs reproducing at larger sizes increase the reproductive output of a single reproductive event (Smalås et al., 2017). The smaller PB/PZ morph is known to have a lower fecundity (Sandlund et al., 1992; Smalås et al., 2017). An early reproduction may allow for multiple reproductive seasons in the iteroparous Arctic charr as the number of reproductive events is a greater contributor to fitness than a single large reproductive event (Stearns, 1992). In addition, a lower fecundity in the PB/PZ morph might be offset by a relatively large investment in gonadal vs somatic growth (Sandlund et al., 1992) which we see evidence of here with the relatively high r for the PB/PZ morph in the biphasic growth modeling.
Maturation in the PP morph, however, seems to have a more complex relationship with age and length and is potentially related to the relatively limited sample size in this study. The PP morph matures at older ages while still being shorter than their LO counterparts (except for length in Skogsfjordvatn). The PP morph has a relatively long and slender shape body (Smalås et al., 2013) with a large head (Skoglund et al., 2015) and a lower condition factor relative to the two other sympatric morphs (unpublished G. Grenier). Condition is known to be a good predictor maturity where a minimum threshold is necessary for successful maturation (Dutil, 1986). During their juvenile years deep in the cold profundal habitat growth in the PP morph is relatively slow (Smalås et al., 2013, this study). To consume fish prey, which is relatively large in relation to other potential prey sources in freshwater environments, the gape-limited predator’s size must permit the consumption of prey of such size. The combination of slow growth (in length and girth) along with a large head suggests that the PP morph concentrates its energy into developing a phenotype permissible of piscivory before initiating reproduction. Thus, the PP morph requires more time to attain a gape permissible of piscivory and obtain the required nutritional status for maturation, hence their reproduction at shorter lengths but older ages than the LO individuals.
Through Arctic charr’s iteroparous nature, multiple strategies are viable options when it comes to maximize fitness through variations of age and size at maturity along with annual investment in reproductive effort and fecundity. When considering age at maturity, there is much variation within each comparable morph type among lake analyses once again suggest local adaptation. With the large variation in life history seen among comparable morphs, here length seems to be a superior predictor of maturity relative to age.
Future work could examine the point in life where an individual’s life history trajectory is set to determine the extent to which genetics and environment influence individual life history. This would help elucidate the process of evolution and speciation within the Arctic charr complex along with the evolutionary history of the various morphs. In addition, studies of life history and evolution in Arctic and subarctic fish communities are warranted as salmonids are predicted to be negatively impacted within these communities in relation to climate change (Hayden et al., 2017). Further studies examining the contribution of the environment to the evolution of Arctic charr life history are necessary to protect and preserve the world’s most variable vertebrate (Klemetsen, 2013).
Conclusion
Our results support repeatability in the evolution of life history traits between morphs in Arctic charr, while also highlighting the importance of local adaptation in the modulation of life history traits of these morphs. This is further supported by similar patterns of parallelism in other traits, such as skull morphology, diet, and parasite load, in Arctic charr inhabiting similar environments within Northern Scandinavia (Eloranta et al., 2013; Siwertsson et al., 2013, 2016; Skoglund et al., 2015). Notably, this is the first study to substantiate repeatability in life history traits of the PP morph. Within the five studied lakes, the PP morph adopts a slow life history strategy while the PB/PZ morph adopts a fast strategy and the LO morph finds itself in an intermediate strategy. The LO morph demonstrates a wide variability in possible life history traits through its generalist nature while the PB/PZ and PP morphs occupy a more specialized niche thus more restricted variation in life history parameters. The PP morph improves its growth trajectory through an ontogenetic niche shift and has an old age at maturity. The PB/PZ morph avoids the need to grow big by maturing early and at smaller sizes. The strategies seen within each morph seem to be evolutionarily stable strategies that have evolved repeatably.
Data Availability Statement
Requests to access these datasets should be directed to GG, gabrielle.grenier@uit.no.
Ethics Statement
Ethical review and approval was not required for the animal study because this study was performed using archived samples therefore no approval was directly required.
Author Contributions
GG conceived and designed the study with contributions from RKn and RKj, performed the statistical analyses with contributions from AS, and wrote the first draft of the manuscript. All authors contributed to manuscript revisions, read, and approved the submitted version.
Conflict of Interest
The authors declare that the research was conducted in the absence of any commercial or financial relationships that could be construed as a potential conflict of interest.
Publisher’s Note
All claims expressed in this article are solely those of the authors and do not necessarily represent those of their affiliated organizations, or those of the publisher, the editors and the reviewers. Any product that may be evaluated in this article, or claim that may be made by its manufacturer, is not guaranteed or endorsed by the publisher.
Acknowledgments
We would like to acknowledge members of Freshwater Ecology Group, past and present, for contributing to the collection of samples. Special thanks to Laina Dalsbø, Cecilie Bye, Karin S. Johansen, and Paolo Moccetti for all your work in both the field and the lab. Thank you to Louise Chavarie and two referees for comments that helped improve the manuscript.
Supplementary Material
The Supplementary Material for this article can be found online at: https://www.frontiersin.org/articles/10.3389/fevo.2021.771309/full#supplementary-material
References
Amundsen, P.-A. (1994). Piscivory and cannibalism in Arctic charr. J. Fish Biol. 45, 181–189. doi: 10.1111/j.1095-8649.1994.tb01092.x
Amundsen, P.-A. (1995). Feeding strategy of Arctic charr (Salvelinus alpinus): general opportunist, but individual specialist. Nord. J. Freshw. Res. 71, 150–156.
Amundsen, P.-A., Knudsen, R., and Klemetsen, A. (2008). Seasonal and ontogenetic variations in resource use by two sympatric Arctic charr morphs. Environ. Biol. Fishes 83, 45–55. doi: 10.1007/s10641-007-9262-1
Birnie-Gauvin, K., Bordeleau, X., Cooke, S. J., Davidsen, J. G., Eldøy, S. H., Eliason, E. J., et al. (2021). Life-history strategies in salmonids: the role of physiology and its consequences. Biol. Rev. 96, 2304–2320. doi: 10.1111/brv.12753
Boukal, D. S., Dieckmann, U., Enberg, K., Heino, M., and Jørgensen, C. (2014). Life-history implications of the allometric scaling of growth. J. Theor. Biol. 359, 199–207. doi: 10.1016/j.jtbi.2014.05.022
Chavarie, L., Dempson, J. B., Schwarz, C. J., Reist, J. D., Power, G., and Power, M. (2010). Latitudinal variation in growth among Arctic charr in eastern North America: evidence for countergradient variation? Hydrobiologia 650, 161–177. doi: 10.1007/s10750-009-0043-z
Chavarie, L., Howland, K., Venturelli, P., Kissinger, B. C., Tallman, R., and Tonn, W. (2016). Life-history variation among four shallow-water morphotypes of lake trout from Great Bear Lake, Canada. J. Great Lakes Res. 42, 193–203. doi: 10.1016/j.jglr.2015.07.006
Chen, Y., Jackson, D. A., and Harvey, H. H. (1992). A comparison of von Bertalanffy and polynomial functions in modelling fish growth data. Can. J. Fish. Aquat. Sci. 49, 1228–1235. doi: 10.1139/f92-138
Collett, D. (1991). Modelling Binary Data, 1st Edn. Boston, MA: Springer. doi: 10.1007/978-1-4899-4475-7
Crespi, B. J., and Teo, R. (2002). Comparative phylogenetic analysis of the evolution of semelparity and life history in salmonid fishes. Evolution (N. Y.) 56, 1008–1020. doi: 10.1111/j.0014-3820.2002.tb01412.x
Dunnington, D. (2021). ggspatial: Spatial Data Framework for ggplot2. R Package Version 1.1.5. Available online at: https://CRAN.R-project.org/package=ggspatial
Dutil, J.-D. (1986). Energetic constraints and spawning interval in the anadromous Arctic charr (Salvelinus alpinus). Copeia 1986, 945–955. doi: 10.2307/1445291
Eloranta, A. P., Kahilainen, K. K., Amundsen, P. A., Knudsen, R., Harrod, C., and Jones, R. I. (2015). Lake size and fish diversity determine resource use and trophic position of a top predator in high-latitude lakes. Ecol. Evol. 5, 1664–1675. doi: 10.1002/ece3.1464
Eloranta, A. P., Knudsen, R., and Amundsen, P.-A. (2013). Niche segregation of coexisting Arctic charr (Salvelinus alpinus) and brown trout (Salmo trutta) constrains food web coupling in subarctic lakes. Freshw. Biol. 58, 207–221. doi: 10.1111/fwb.12052
Ferguson, M. M., Noakes, D. L. G., Skúlason, S., and Snorrason, S. S. (1990). Life-history styles and somatic allocation in iteroporous arctic charr and semelparous pink salmon. Environ. Biol. Fishes 28, 267–272. doi: 10.1007/BF00751040
Forseth, T., Ugedal, O., and Jonsson, B. (1994). The energy budget, niche shift, reproduction and growth in a population of Arctic charr, Salvelinus alpinus. J. Anim. Ecol. 63:116. doi: 10.2307/5588
Frost, W. E. (1965). Breeding habits of Windermere charr, Salvelinus willughbii (Günther), and their bearing on speciation of these fish. Proc. R. Soc. Lond. Ser. B Biol. Sci. 163, 232–284.
Gross, M. R. (1985). Disruptive selection for alternative life histories in salmon. Nature 313, 47–48. doi: 10.1038/313047a0
Haddon, M. (2011). Modelling and Quantitative Methods in Fisheries, 2nd Edn. New York, NY: Chapman and Hall/CRC. doi: 10.1201/9781439894170
Hayden, B., Myllykangas, J. P., Rolls, R. J., and Kahilainen, K. K. (2017). Climate and productivity shape fish and invertebrate community structure in subarctic lakes. Freshw. Biol. 62, 990–1003. doi: 10.1111/fwb.12919
Hindar, K., and Jonsson, B. (1982). Habitat and food segregation of dwarf and normal Arctic Charr (Salvelinus alpinus) from Vangsvatnet Lake, western Norway. Can. J. Fish. Aquat. Sci. 39, 1030–1045. doi: 10.1139/f82-138
Hindar, K., and Jonsson, B. (1993). Ecological polymorphism in Arctic charr. Biol. J. Linn. Soc. 48, 63–74. doi: 10.1111/j.1095-8312.1993.tb00877.x
Hutchings, J. A., and Morris, D. W. (1985). The influence of phylogeny, size and behaviour on patterns of covaritation in salmonid life histories. Oikos 45, 118–124.
Jobling, M. (1983). Growth studies with fish-overcoming the problems of size variation. J. Fish Biol. 22, 153–157. doi: 10.1111/j.1095-8649.1983.tb04735.x
Jonsson, B., Skúlason, S., Snorrason, S. S., Sandlund, O. T., Malmquist, H. J., Jónasson, P. M., et al. (1988). Life history variation of polymorphic Arctic charr (Salvelinus alpinus) in Thingvallavatn, Iceland. Can. J. Fish. Aquat. Sci. 45, 1537–1547. doi: 10.1139/f88-182
Kimura, D. K. (1980). Likelihood methods for the von Bertalanffy growth curve. Fish. Bull. 77, 765–776.
Klemetsen, A. (2010). The charr problem revisited?: exceptional phenotypic plasticity promotes ecological speciation in postglacial lakes. Freshw. Rev. 3, 49–74. doi: 10.1608/FRJ-3.1.3
Klemetsen, A. (2013). The most variable vertebrate on earth. J. Ichthyol. 53, 781–791. doi: 10.1134/S0032945213100044
Klemetsen, A., Amundsen, P.-A., Knudsen, R., and Hermansen, B. (1997). A profundal, winter-spawning morph of Arctic charr Salvelinus alpinus (L.) in lake Fjellfrøsvatn, Northern Norway. Nord. J. Freshw. Res. 73, 13–23.
Knudsen, R., Amundsen, P.-A., Eloranta, A. P., Hayden, B., Siwertsson, A., and Klemetsen, A. (2016). Parallel evolution of profundal Arctic charr morphs in two contrasting fish communities. Hydrobiologia 783, 239–248. doi: 10.1007/s10750-016-2647-4
Knudsen, R., Eloranta, A. P., Paterson, R. A., and Sandlund, O. T. (2019). Introduction of Mysis relicta (Mysida) reduces niche segregation between deep-water Arctic charr morphs. Hydrobiologia 840, 245–260. doi: 10.1007/s10750-019-3953-4
Knudsen, R., Johnsen, H. K., Sæther, B.-S., and Siikavuopio, S. I. (2015). Divergent growth patterns between juveniles of two sympatric Arctic charr morphs with contrasting depth gradient niche preferences. Aquat. Ecol. 49, 33–42. doi: 10.1007/s10452-015-9502-y
Meuthen, D., Baldauf, S. A., Bakker, T. C. M., and Thünken, T. (2018). Neglected patterns of variation in phenotypic plasticity: age- and sex-specific antipredator plasticity in a cichlid fish. Am. Nat. 191, 475–490. doi: 10.1086/696264
Moccetti, P., Siwertsson, A., Kjær, R., Amundsen, P. A., Præbel, K., Tamayo, A. M. P., et al. (2019). Contrasting patterns in trophic niche evolution of polymorphic Arctic charr populations in two subarctic Norwegian lakes. Hydrobiologia 840, 281–299. doi: 10.1007/s10750-019-3969-9
Muir, A. M., Hansen, M. J., Bronte, C. R., and Krueger, C. C. (2015). If Arctic charr Salvelinus alpinus is “the most diverse vertebrate”, what is the lake charr Salvelinus namaycush? Fish Fish. 17, 1194–1207. doi: 10.1111/faf.12114
Østbye, K., Hagen Hassve, M., Peris Tamayo, A. M., Hagenlund, M., Vogler, T., and Præbel, K. (2020). “And if you gaze long into an abyss, the abyss gazes also into thee”: four morphs of Arctic charr adapting to a depth gradient in Lake Tinnsjøen. Evol. Appl. 13, 1240–1261. doi: 10.1111/eva.12983
Pebesma, E. (2018). Simple features for R: standardized support for spatial vector data. R J. 10, 439–446. doi: 10.32614/RJ-2018-009
Perkins, T. A., and Jager, H. I. (2011). Falling behind: delayed growth explains life-history variation in snake river fall chinook salmon. Trans. Am. Fish. Soc. 140, 959–972. doi: 10.1080/00028487.2011.599257
Pinheiro, J., Bates, D., Debroy, S., Sarkar, D., and R Core Team (2019). nlme: Linear and Nonlinear Mixed Effects Models. R package version 3.1-153. Available online at: https://CRAN.R-project.org/package=nlme
Præbel, K., Couton, M., Knudsen, R., and Amundsen, P.-A. (2016). Genetic consequences of allopatric and sympatric divergence in Arctic charr (Salvelinus alpinus (L.)) from Fjellfrøsvatn as inferred by microsatellite markers. Hydrobiologia 783, 257–267. doi: 10.1007/s10750-016-2648-3
Prati, S., Henriksen, E. H., Knudsen, R., Klemetsen, A., and Amundsen, P. (2021). The effect of inter- and intraspecific competition on individual and population niche widths: a four-decade study on two interacting salmonids. Oikos 130, 1679–1691. doi: 10.1111/oik.08375
Quince, C., Abrams, P. A., Shuter, B. J., and Lester, N. P. (2008). Biphasic growth in fish I: theoretical foundations. J. Theor. Biol. 254, 197–206. doi: 10.1016/j.jtbi.2008.05.029
R Development Core Team (2018). A Language and Environment for Statistical Computing. Vienna: R Foundation for Statistical Computing.
Rennison, D. J., Rudman, S. M., and Schluter, D. (2019). Genetics of adaptation: experimental test of a biotic mechanism driving divergence in traits and genes. Evol. Lett. 3, 513–520. doi: 10.1002/evl3.135
Roff, D. A. (1984). The evolution of life history parameters in teleosts. Can. J. Fish. Aquat. Sci. 41, 989–1000. doi: 10.1139/f84-114
Saltykova, E., Siwertsson, A., and Knudsen, R. (2017). Parallel phenotypic evolution of skull-bone structures and head measurements of Arctic charr morphs in two subarctic lakes. Environ. Biol. Fishes 100, 137–148. doi: 10.1007/s10641-016-0564-z
Sandlund, O. T., Brabrand, Å, Davidsen, J. G., Øystein, K., Heggberget, T. G., Knudsen, R., et al. (2017). Fiskebiologiske Undersøkelser i Limingen, Nord-Trøndelag, 2016. Trondheim: Norsk institutt for naturforskning.
Sandlund, O. T., Gunnarsson, K., Jónasson, P. M., Jonsson, B., Lindem, T., Magnússon, K. P., et al. (1992). The Arctic charr Salvelinus alpinus in Thingvallavatn. Oikos 64, 305–351.
Santos Baquero, O. (2019). ggsn: North Symbols and Scale Bars for Maps Created with “ggplot2” or “ggmap.” R package version 0.5.0. Available online at: https://CRAN.R-project.org/package=ggsn
Simonsen, M. K., Siwertsson, A., Adams, C. E., Amundsen, P. A., Præbel, K., and Knudsen, R. (2017). Allometric trajectories of body and head morphology in three sympatric Arctic charr (Salvelinus alpinus (L.)) morphs. Ecol. Evol. 7, 7277–7289. doi: 10.1002/ece3.3224
Siwertsson, A., Knudsen, R., Præbel, K., Adams, C. E., Newton, J., and Amundsen, P.-A. (2013). Discrete foraging niches promote ecological, phenotypic, and genetic divergence in sympatric whitefish (Coregonus lavaretus). Evol. Ecol. 27, 547–564. doi: 10.1007/s10682-012-9607-x
Siwertsson, A., Refsnes, B., Frainer, A., Amundsen, P.-A., and Knudsen, R. (2016). Divergence and parallelism of parasite infections in Arctic charr morphs from deep and shallow lake habitats. Hydrobiologia 783, 131–143. doi: 10.1007/s10750-015-2563-z
Skoglund, S., Siwertsson, A., Amundsen, P.-A., and Knudsen, R. (2015). Morphological divergence between three Arctic charr morphs–the significance of the deep-water environment. Ecol. Evol. 5, 3114–3129. doi: 10.1002/ece3.1573
Skúlason, S., Noakes, D. L. G., and Snorrason, S. S. (1989a). Ontogeny of trophic morphology in four sympatric morphs of arctic charr Salvelinus alpinus in Thingvallavatn. Iceland. Biol. J. Linn. Soc. 38, 281–301. doi: 10.1111/j.1095-8312.1989.tb01579.x
Skúlason, S., Snorrason, S. S., Noakes, D. L. G., Ferguson, M. M., and Malmquist, H. J. (1989b). Segregation in spawning and early life history among polymorphic Arctic charr, Salvelinus alpinus, in Thingvallavatn, Iceland. J. Fish Biol. 35, 225–232. doi: 10.1111/j.1095-8649.1989.tb03065.x
Skúlason, S., and Smith, T. B. (1995). Resource polymorphisms in vertebrates. Trends Ecol. Evol. 10, 366–370. doi: 10.1016/S0169-5347(00)89135-1
Skúlason, S., Snorrason, S. S., Noakes, D. L. G., and Ferguson, M. M. (1996). Genetic basis of life history variations among sympatric morphs of Arctic char Salvelinus alpinus. Can. J. Fish. Aquat. Sci. 53, 1807–1813. doi: 10.1139/cjfas-53-8-1807
Smalås, A., Amundsen, P.-A., and Knudsen, R. (2013). Contrasting life history strategies of sympatric Arctic charr morphs, Salvelinus alpinus. J. Ichthyol. 53, 856–866. doi: 10.1134/S0032945213100111
Smalås, A., Amundsen, P. A., and Knudsen, R. (2017). The trade-off between fecundity and egg size in a polymorphic population of Arctic charr (Salvelinus alpinus (L.)) in Skogsfjordvatn, subarctic Norway. Ecol. Evol. 7, 2018–2024. doi: 10.1002/ece3.2669
Snorrason, S. S., Skúlason, S., Jonsson, B., Malmquist, H. J., Jonasson, P. M., Sandlund, O. T., et al. (1994). Trophic specialization in Arctic charr Salvelinus alpinus (Pisces; Salmonidae): morphological divergence and ontogenetic niche shifts. Biol. J. Linn. Soc. 52, 1–18. doi: 10.1111/j.1095-8312.1994.tb00975.x
South, A. (2017). rnaturalearth: World Map Data from Natural Earth. R package version 0.1.0. Available online at: https://CRAN.R-project.org/package=rnaturalearthdata
Svedäng, H. (1990). Genetic basis of life-history variation of dwarf and normal Arctic charr, Salvelinus alpinus (L.), in Stora Rösjön, central Sweden. J. Fish Biol. 36, 917–932. doi: 10.1111/j.1095-8649.1990.tb05638.x
Wickham, H. (2016). ggplot2: Elegant Graphics for Data Analysis, 2nd Edn. New York, NY: Springer-Verlag. doi: 10.1007/978-3-319-24277-4
Wootton, R. J. (1990). Ecology of Teleost Fishes. New York, NY: Chapman & Hall. doi: 10.1007/978-94-009-0829-1
Zuur, A. F., Ieno, E. N., and Elphick, C. S. (2010). A protocol for data exploration to avoid common statistical problems. Methods Ecol. Evol. 1, 3–14. doi: 10.1111/j.2041-210x.2009.00001.x
Keywords: growth, maturity, sympatric populations, ecological evolution, biphasic growth model, Salvelinus alpinus L, reproductive investment, size-at-age
Citation: Grenier G, Smalås A, Kjær R and Knudsen R (2021) Environmentally Modulated Repeat Evolution of Polymorphic Arctic Charr Life History Traits. Front. Ecol. Evol. 9:771309. doi: 10.3389/fevo.2021.771309
Received: 06 September 2021; Accepted: 25 November 2021;
Published: 13 December 2021.
Edited by:
Claudia Fichtel, Deutsches Primatenzentrum, GermanyReviewed by:
Peter Dijkstra, Central Michigan University, United StatesRebecca Nagel, Bielefeld University, Germany
Copyright © 2021 Grenier, Smalås, Kjær and Knudsen. This is an open-access article distributed under the terms of the Creative Commons Attribution License (CC BY). The use, distribution or reproduction in other forums is permitted, provided the original author(s) and the copyright owner(s) are credited and that the original publication in this journal is cited, in accordance with accepted academic practice. No use, distribution or reproduction is permitted which does not comply with these terms.
*Correspondence: Gabrielle Grenier, gabrielle.grenier@uit.no
†ORCID: Gabrielle Grenier, orcid.org/0000-0002-7418-0812; Aslak Smalås, orcid.org/0000-0002-6316-2811; Rune Knudsen, orcid.org/0000-0001-8579-4687