Comparison of recycled vapor contribution to precipitation in urban vs. rural area—A case study in western China
- 1Ecohydrology Observation and Research Station of the Southern Qinling Mountains, Shangluo University, Shangluo, Shaanxi, China
- 2Key Laboratory of Mountain Surface Processes and Ecological Regulation, Institute of Mountain Hazards and Environment, Chinese Academy of Sciences, Chengdu, China
- 3School of Geography and Tourism, Chongqing Normal University, Chongqing, China
- 4State Key laboratory of Hydraulics and Mountain River Engineering, Sichuan University, Chengdu, China
While urbanization’s strong effect on local precipitation has been widely documented, knowledge of how urbanization affects isotopic composition in precipitation is still lacking. In the present study, deuterium-excess (d-excess) served to quantify the contribution of recycled vapor to precipitation in Chengdu city (China) and a nearby rural area. Precipitation from the urban and rural areas showed no significant difference in δ18O values (p > 0.05). The rural area had significantly higher d-excess (24.29 ± 7.39‰) than the urban (12.71 ± 4.88‰) through the seasons due to higher evapotranspiration flux in the rural area. In summer, however, urban precipitation amount was higher than that of the rural area. Based on d-excess model, the average ratio of recycled vapor was 8.2% in Chengdu, which was lower than in the rural area (36.1%). This highlights the effect of urbanization in decreasing the proportion of vapor from local evapotranspiration contributing to precipitation but blocked much advected moisture. This also implied that precipitation taken in cities were used to represent upwind advected vapor or used as referenced isotopic records for paleoclimate reconstruction based on tree rings or stalagmites sampled in rural area may be erroneous as the effect of urbanization on precipitation vapor. Further studies are needed to explore the effect of urbanization on vapor source of precipitation under different climatic zones.
Introduction
With the development of global urbanization, the variation of urban hydrological processes has become increasingly prominent, and the mechanism of urban water cycle has undergone profound changes, resulting in increasing water risks and water environmental problems, such as frequently happened storm, shortage of water resources and deterioration of water quality (Mrekva et al., 2012; Wang et al., 2014; Sang and Yang, 2017). These disasters have caused huge economic losses and threaten the safety of infrastructure and human lives (Yang et al., 2021). Among multiple effects of urbanization on the natural environment, the greatest ones are changes to surface air temperature and precipitation (Ren et al., 2008; Hamdi et al., 2009; Song et al., 2014). However, exploring how urbanization induces changes in rainfall patterns is difficult, due to the heterogeneity of spatiotemporal distribution of rainfall, a lack of direct observations of precipitation vapor source and the unrelenting progress of urbanization (Liu and Niyogi, 2019).
Water vapor contributing to precipitation consists of advected vapor from the ocean and recycled vapor from the continent (Gimeno et al., 2020). The accelerated climate change has imposed great impacts on the contribution of vapor sources to precipitation. Under the trend of global warming, recycled vapor has played an increasingly important role in the formation of precipitation due to the increase in surface evapotranspiration capacity (Singh et al., 2017; Ampuero et al., 2020; Cui et al., 2022). However, quantifying the effect of urbanization on the precipitation composition is still a challenge in hydrological studies especially through field observation.
Hydrogen and oxygen stable isotopes, which are widely used tools for hydrological tracing, are closely associated with the atmospheric recycling pattern and water source of precipitation. For example, satellite-derived estimation identified that continental evapotranspiration are slightly isotope-depleted compared to oceanic evaporation, leading to decreased D/H isotope in summer precipitation at a global scale (Good et al., 2015). However, Cluett et al. (2021) found that increases in local evaporation will promote the enrichment of D/H isotope in precipitation. Isotope-enabled climate models also demonstrated that transpiration yielded more isotope-enriched atmospheric vapor than ocean evaporation, suggesting continental evapotranspiration would increase heavy isotopes contents in summer precipitation at many sites (Risi et al., 2013). These inconsistent findings reflect that under the influences of global warming and human activities such as urbanization, the variation patterns of isotopes in precipitation are complex and uncertain (Masson-Delmotte et al., 2021). The precipitation isotopes record the information of climate change and human activities’ effects. Consequently, a better understanding of these effects on precipitation isotopes could improve our knowledge on the information contained in precipitation isotope proxies such as tree ring or stalagmite. In addition, sampling of precipitation for isotopic analysis has mostly been carried out in cities, particularly megacities (IAEA, 2014). These isotopic records are often used as references for paleoclimatic reconstruction by isotopic values measured in tree rings or stalagmites, which are primarily located in depopulated zones (e.g., mountains or rural areas). A systematic comparison of water vapor and isotopic composition in precipitation between urban and rural areas will be helpful in answering questions regarding the effects of urbanization on precipitation patterns, and the possible uncertainties of precipitation isotope-based paleoclimate reconstruction brought by sampling locations.
Several studies have analyzed the amount of recycled vapor in precipitation using simple bulk methods or conceptualizations of the hydrological cycle (Van Der Ent et al., 2014; Baker and Spracklen, 2022). However, these methods provided only a rough estimate of vapor dynamics over a large region, and are subject to several assumptions (Van Der Ent et al., 2014). Hydrogen and oxygen isotopes in water (i.e., δ2H and δ18O, respectively) and the derivative proxy of d-excess [d = δ2H–8 δ18O (‰)], have been shown to be useful indicators for the variability of moisture recycling (Dansgaard, 1964). The d-excess depends strictly on environmental factors in the vapor source area, remains stable during the transport process, and is influenced by the sub-cloud secondary evaporation and recycled moisture (Pang et al., 2011; Wang S. J. et al., 2016). Therefore, this proxy can be used to indicate the meteorological conditions in the oceanic vapor source and reflect the degree of isotopic non-equilibrium fractionation during landing process (Froehlich et al., 2004). However, quite few studies were conducted to compare the isotopic composition and d-excess between adjacent urban and rural areas, limiting the exploring of water vapor sources and local atmospheric cycling processes. Assuming that local precipitation consists of land surface evaporated (recycled) vapor and advected vapor, Peng et al. (2005) proposed a d-excess-based model for estimating the proportion of recycled vapor in precipitation, whose accuracy in calculating the vapor source was further validated by Griffis et al. (2016).
In Sichuan Basin of southwest China, the precipitation is mainly sourced from the South China Sea, Western Pacific and Indian Ocean, driven by the monsoon alternation (Xia et al., 2020). Previous study has shown that this region presents complex temporal isotopic characteristics, associated with both water vapor source and environmental factors (Xia et al., 2020). In this study, this model was employed to determine the vapor composition of precipitation sampled in the city of Chengdu and a nearby rural area, based on the hypothesis that urban and rural areas would have the same recycled moisture ratio in their monthly precipitation. The findings will help improve the understanding of the impact of urban development on the atmospheric circulation and provide insights for further optimization of isotope-based paleoclimate reconstruction.
Materials and methods
Locations of the study sites
Chengdu (102°54′–104°53′E, 30°05–31°26′N), the provincial capital of Sichuan Province, is the most important city in western China in terms of economic activity and tourism. The population density increases greatly and economy grows fastly since the 1980s, associated with rapid urbanization. The Chengdu sampling site was situated at Sichuan University (30.63°N, 104.08°E). The corresponding rural area was monitored in the village of Jieliu, at an experimental station of the Chinese Academy of Sciences (31.26°N, 105.45°E), some 148 km away from the Chengdu site (Figure 1). Chengdu site is located in the hinterland of Chengdu plain where the terrain is flat. Jieliu site is located in Yanting County, an area whose topography is medium to deep hills, where multi-stage terraces form due to the horizontal sand mudstone interlayers. Both urban and rural areas belong to subtropical monsoon climate zone, with distinct dry and rainy seasons. During the rainy season (May to October), they are mainly controlled by the southeast monsoon from the northwest Pacific Ocean and the southwest monsoon from the Indian Ocean, with concentrated precipitation and high temperature; in the dry season (November to May of the following year), they are controlled by the northwest monsoon from Siberia, with little precipitation and low temperature. In Chengdu City, the annual mean precipitation is about 1000 mm and the mean temperature is 16°C. In Yanting County, the annual mean precipitation is 826 mm and the mean temperature is 17.3°C. In both sites, precipitation distributes unevenly through the year. About 70 % of the total amount happens in summer months. The total population of Chengdu City reaches 21 million and that of Yanting County is only 0.38 million. The main types of land uses in the urban and rural sites are urban-and-industrial land and forest land, respectively.
Quantification of recycled moisture proportion
Monthly d-excess was calculated by weighting the quantity of precipitation for each precipitation event:
where,
d is the monthly d-excess,
di is the d-excess of ith precipitation event during the month,
i is the number of the precipitation event considered,
n is the total number of precipitation events considered, and Pi is the amount of precipitation received during the month’s ith event.
The d-excess model used for the recycled moisture proportion could be expressed as follows (Zhang et al., 2021):
where,
dc, de, dt, and dad represent the d-excess values of precipitation at the cloud base, in evaporation vapor, in transpiration vapor, and in advected vapor, respectively,
fad,ft and fe are the proportional contributions of advected vapor, transpiration vapor and evaporation vapor to overall precipitation, respectively,
fre is the recycling ratio of transpired and evaporated vapor within the total precipitation,
m in the model was assumed to be equivalent to the ratio of monthly transpiration flux (Et) to monthly evaporation flux (Ee), which were derived from the GLEAM model output of the v3.5b dataset (Martens et al., 2017).
Monthly Et, Ee and m values in Chengdu and Jieliu are shown in Supplementary material.
Based on Eqs 1–5, the fraction of recycled ratio in local precipitation can be obtained as (Zhang et al., 2021):
The d-excess values of the advected vapor (dad) at each study area was assessed from precipitable water simulated by Isotope Reanalysis data version 2 (IsoGSM2) (Yoshimura et al., 2008). We assumed there to be no difference between the advected moisture for Chengdu and Jieliu because of the relatively small distance between them. The de can be calculated using the Craig-Gordon model (Craig and Gordon, 1965) (see Supplementary material). Assuming that the initial isotopic composition of the precipitation at the cloud base is in isotopic equilibrium with the surrounding water vapor, dc can be calculated by the model proposed by Froehlich et al. (2008) (see Supplementary material). The dt depends on the value of weighted multi-year precipitation, assuming there is no isotopic fractionation during plant uptake and transpiration at the specific study sites (Dawson and Ehleringer, 1991).
An unpaired statistical t-test was used to compare the difference of variables between the urban and rural areas by R software (R Core Development Team, 2020).
Results and discussion
Isotopic compositions of precipitation at urban and rural sites
Figure 2 compares local meteoric water lines (LMWLs) for Chengdu and Jieliu, along with the global meteoric water line (GMWL). The δ2H/δ18O slopes of the two sites were similar and both were slightly greater than that of the GMWL. The intercept value of the LMWL of Jieliu was greater than that of the GMWL, suggesting that Jieliu was strongly affected by recycled moisture over the entire year. The intercept value of the LMWL of Chengdu was close to that of GMWL, suggesting that the proportion of recycled moisture in the precipitation was small (Trenberth et al., 2003).
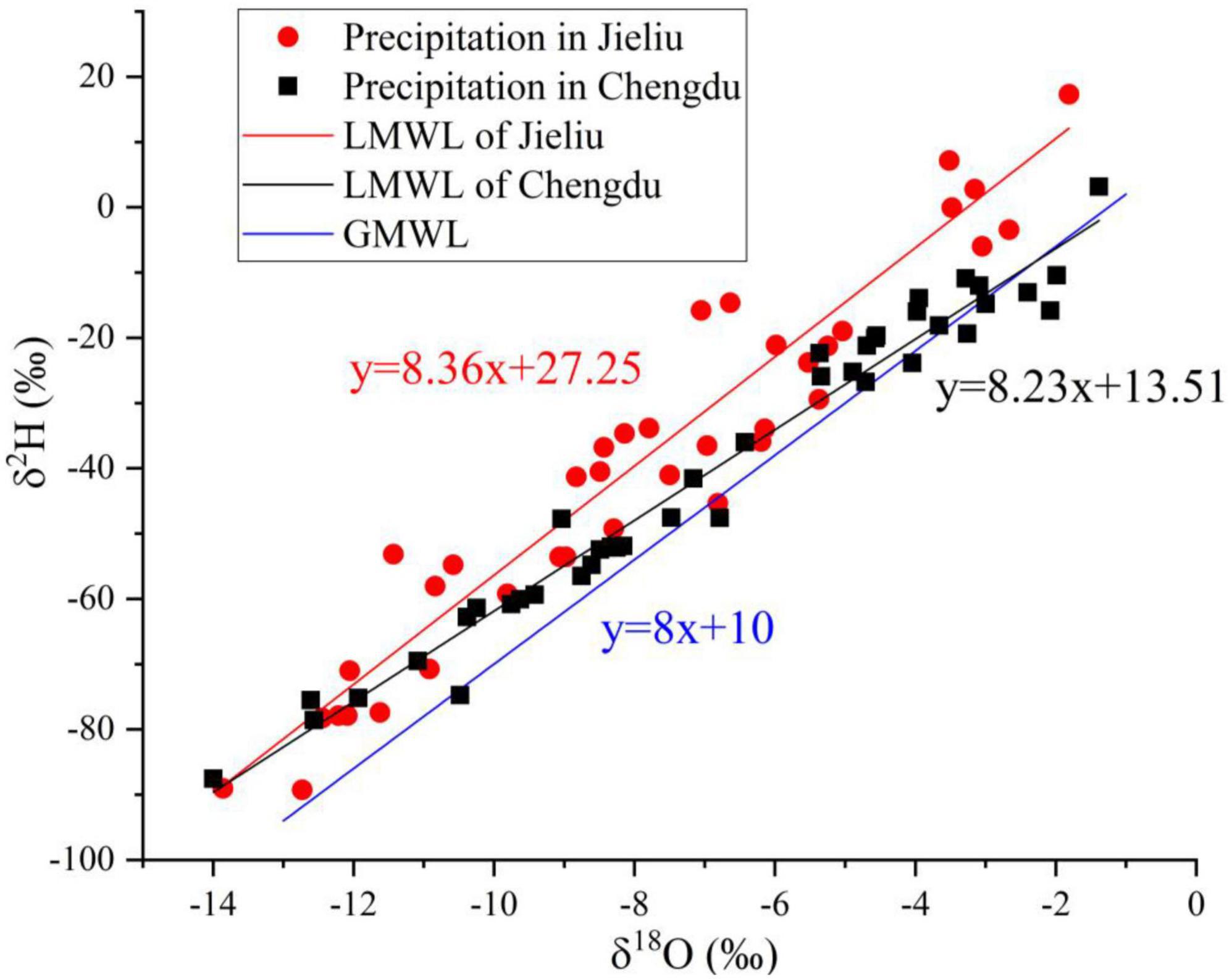
Figure 2. Comparison of the local meteoric water lines (LMWLs) of Chengdu and Jieliu, along with the global meteoric water line (GMWL).
The d-excess, δ18O and precipitation amount were compared between the urban and rural sites from January 2017 to December 2020 (Figure 3). The d-excess in Chengdu varied substantially (1.23—22.28‰, with an average value of 12.71 ± 4.88 ‰. This mean value was close to the global average of 10‰. The d-excess in Jieliu precipitation ranged from 9.13 to 40.62‰, with an average value of 24.29 ± 7.39‰. The d-excess at the rural site showed significantly greater values than those found in the urban area (p ≤ 0.05).
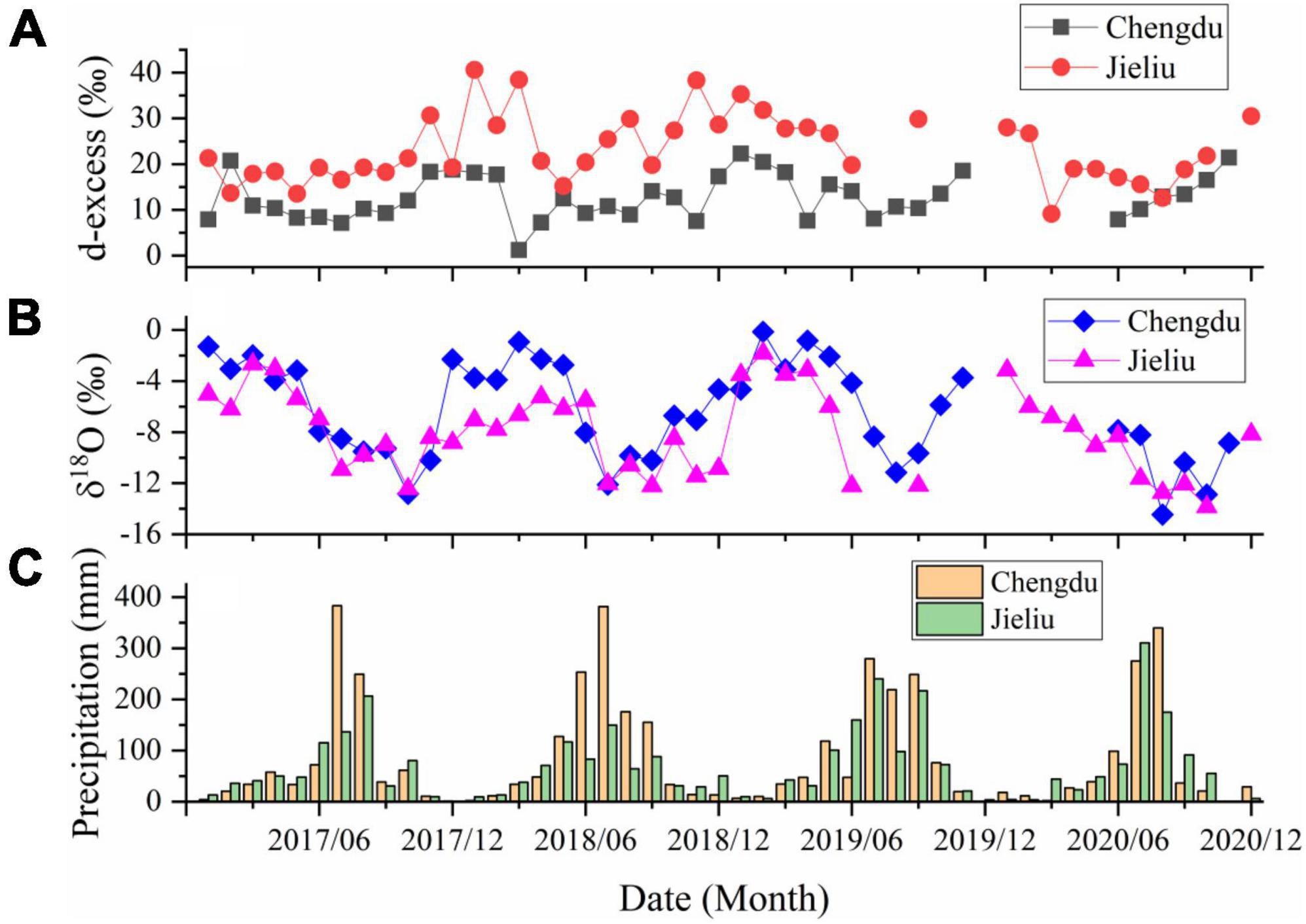
Figure 3. Temporal variations of (A) d-excess, (B) δ18O, (C) precipitation amount. In Jieliu there was no isotopic precipitation sampling in July, August, October to December 2019 and in November 2020. Isotopic data for precipitation were not available from December 2019 to May 2020, and in December 2020 in Chengdu.
The δ18O values at Chengdu ranged from −14.45 to −0.15‰ with an average value of −6.40 ± 3.86‰ during the period of observation. At Jieliu, precipitation δ18O values ranged from −13.85 to −1.81‰ with an average value of −7.96 ± 3.30‰. Precipitation δ18O at the rural site showed a smaller range and more depleted values compared to the city, although they were not significantly different (p = 0.051).
In the summer months, precipitation in the city was usually greater than in rural areas (Figure 3C). The mean value of monthly precipitation amounts throughout the 4-year observation period in Chengdu was 94.88 ± 137.59 mm, which was greater than the 65.14 ± 65.81 mm in Jieliu although the difference was not significant (p > 0.05). Simultaneously, the variation of the amount of precipitation in the city was also higher than that in the village. While precipitation amounts over the study period were not significantly higher in Chengdu city than in Jieliu village across all seasons, monthly precipitation amounts in spring, autumn and winter were greater (though not significantly so) in the rural area than in the urban area. In other areas across the world, higher precipitation amount happens in urban area than in the rural area has also been widely reported (Huff and Changnon, 1972; Rao, 1980; Çiçek and Turkoglu, 2005). Such pattern exists because buildings in urban area block the continuous landward penetration of a regional climatic rainband. This change in rainfall variation is associated with a slowing of the penetration of wind with rain by urban friction which actually lead to the enhanced convergence over the city (Li et al., 2021).
The d-excess at the urban site clearly showed much lower values compared to those at the rural site. During the water evaporation process, the ratio between 18O and 2H in the remaining water become higher compared with that under equilibrium condition due to the dynamic isotope fractionation, leading to decreased d-excess (<10‰) (Froehlich et al., 2008). Conversely, following the mass balance, d-excess of evaporated vapor would increase, and the precipitation formed by this vapor would also be characterized by a higher d-excess value (larger than 10‰). A relatively high d-excess commonly indicates the involvement of a large proportion of recycled vapor in local precipitation (Froehlich et al., 2008; Pang et al., 2011).
Comparing the recycling ratio in urban and rural areas
In this study, the d-excess of advected moisture in Chengdu and Jieliu was obtained by modeled results from IsoGSM2. However, this dataset failed to calculate the recycled moisture in Chengdu as most d-excess in precipitation (12.71 ± 4.88‰) was lower than the advected moisture derived from the IsoGSM2 model (16.76 ± 1.80‰). As the evaporated water presented higher d-excess than the remaining water, the d-excess of precipitation did not plot inside the triangle area formed by the d-excess of three end-members including advected, evaporated and transpired vapor.
In this study, we tried to use the global average d-excess value of 10‰ instead of the d-excess of modeled advected moisture. A comparison of monthly fluctuations of the recycling ratio (fre) including the fraction of evaporation (fev) is shown in Figure 4. The fre varied greatly with time throughout the entire year in both Chengdu and Jieliu. In Chengdu, the fre ranged from 0 to 51.8% with an average annual value of 8.2%, while fev showed a narrower range, from 0 to 16.9% with a mean of 4.05%. In Jieliu, fre ranged from 0 to 97.4% with an average annual value of 36.1%, while fev ranged from 0 to 28.5% with a mean value of 12.3%. The contribution of recycled vapor to precipitation in Chengdu was lower than that in Jieliu in terms of less transpirational and evaporative sources. In previous studies, it was widely reported that the evapotranspiration strength is closely related to urban development and would be reduced in urban areas, by water balance, remote sensing and meteorological methods (Dow and DeWalle, 2000; Wang C. et al., 2016; Jiang and Weng, 2017). Our results verified this kind of urban climatic change by employing an isotope-based model to conduct rural-urban vapor source comparison in southwest China. The low proportion of recycled vapor in urban precipitation can be explained by the reduced contribution from evapotranspiration sources caused by the decreased vegetation coverage and construction of urban facilities. On one hand, the decreased vegetation coverage will reduce the transpiration of plants. On the other hand, the rapid drainage process and impervious surface (e.g., cement pavements and buildings) will have an impact on the spatial and temporal changes of surface evaporation in urban areas. The construction of urban drainage systems and the increased area of impervious or weakly pervious surface have improved the efficiency of rainwater drainage, and evaporation can only occurs in a short period after precipitation. It is reported in other study areas that light and moderate rainfall is lower in cities than in areas with high vegetation cover (Yuan et al., 2017), which agrees with the results of vapor sources comparison in our study. In both Chengdu and Jieliu, the ratios of recycled vapor were characterized by relatively low values in summer and autumn and high values in winter and spring. This was consistent with the reported values in other regions using this d-excess-based method (Wang S. et al., 2016; Zhu et al., 2019). Wang S. et al. (2016) found that fre of large oases in Urumqi was much higher than that of small oases (15.2 vs. <5%). Using the same method, Zhu et al. (2019) concluded that in the monsoon marginal zone, the fre of an oasis (28%) was significantly higher than that of mountain areas (17%) and desert areas (15%). This phenomenon was attributed to the high vegetation cover in the oases, which added significant amounts of evaporated (e.g., interception by the canopy) and transpired vapor to local precipitation.
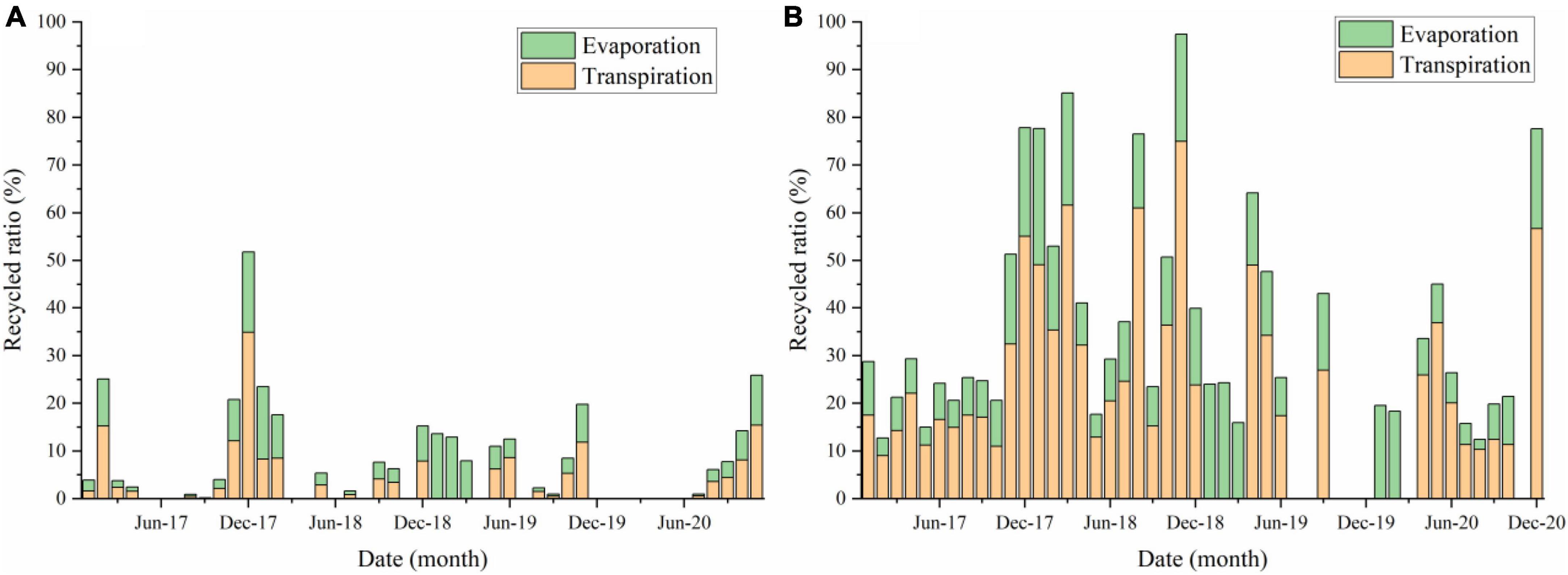
Figure 4. Monthly fluctuations of the recycled vapor ratio (fre), including contributions of transpiration and evaporation in (A) Chengdu and (B) Jieliu. Negative values in Chengdu were set to 0 assuming no recycled vapor in the precipitation.
Our study reveals the difference of recycled vapor contribution in local precipitation between urban and rural areas, which derived from significant difference in isotopic composition and calculated indicators such as d-excess. Currently, neighboring stations from global network of isotopes in precipitation (GNIP) have often been used as references and/or in calculations in many studies involved in δ18O or δ2H at local or regional scales (Hobson et al., 2019; Zhang et al., 2021). However, as precipitation samples for most GNIP stations are taken in cities, these precipitation-isotope-related variables, especially d-excess, should be used with caution in isotopic studies (e.g., paleoclimate reconstruction by tree rings or stalagmites) conducted in rural or depopulated areas, as indicated in the present study.
There’s limitation in the estimation of recycled vapor ratios in the present study. The assumption that d-excess value of advected vapor is 10‰ might bring some uncertainty to the calculation of recycled vapor ratio in precipitation. Also, this value cannot reflect the change of advected moisture. For example, precipitation d-excess over several months was lower than 10‰, which implied that advected vapor with a d-excess lower than 10‰ participated in the precipitation formation. Most previous studies used the calculated d-excess of precipitation from upwind sites to represent advected vapor (Wang S. J. et al., 2016; Zhang et al., 2021). However, the continuous and long-term records of precipitation isotopes at upwind sites were not available for most cases. For this reason, datasets with records in different periods, such as the widely used GNIP observations during the 1970s and 80s, were usually selected as substitution. This also introduced error for the different study periods. In addition, the precipitation vapor is also from local evaporation and transpiration which may vary following the progress of urbanization. This implied that upwind precipitation isotopes may not represent the actual values of d-excess of advected vapor. Therefore, more accurate measurement or calculation of the d-excess in advected moisture is needed for better usage of the d-excess model and understanding the effects of climate change and human activities on precipitation composition in the future.
Conclusion
Based on the 4-year observation on precipitation isotopes and meteorological variables in Chengdu and Jieliu in southwest China, this study compared the isotopic characteristics between urban and rural areas, and analyzed differences in the contribution of recycled vapor in local precipitation accordingly. Compared with the urban area, δ18O in precipitation showed lower values and smaller variation range in the rural area, while the d-excess exhibited significant higher values, as well as the intercept of LMWL. Summer precipitation amount showed higher value in urban area than rural one due to the influence of urban land covers on vapor transportation. Fraction of recycled vapor in local precipitation is quantified by d-excess-based model. During dry seasons, it showed higher recycled vapor contribution in precipitation in rural site than that in urban. This indicating that precipitation forms primarily from evapotranspiration in a rural area rather than advected vapor in an urban area. This highlights the effect of urbanization on the source composition of rainfall moisture and precipitation intensity, further resulting in alterations of atmospheric and hydrological cycles in urban areas. Consequently, urbanization is an important driving factor controlling the amount and composition of precipitation. In the further study focusing on the source and transport of water vapor, vapor contributions identified by isotope-based method should be verified in combination with other technologies such as remote sensing inversion or more field monitoring studies. Relationship between the expansion area of urban and precipitation composition should be quantitatively explored by comparison between the precipitation in more urban and rural regions.
Data availability statement
The raw data supporting the conclusions of this article will be made available by the authors, without undue reservation.
Author contributions
PZ: formal analysis, software, methodology, writing-original draft, and writing–review and editing. CX: investigation, formal analysis, and writing–review and editing. GL: supervision and writing–review and editing. JT and MZ: investigation and data curation. All authors contributed to the article and approved the submitted version.
Funding
This work was financially supported by National Natural Science Foundation of China (41471188), Innovation team of water source protection of middle route of South-to-North Water Diversion (No. SK2017-44), the Science and Technology Research Program of Chongqing Municipal Education Commission (No. KJQN202300541), and the Start-up Funding from Chongqing Normal University (No. 23XLB004).
Acknowledgments
We would like to thank the editor and reviewers for their help in improving the manuscript.
Conflict of interest
The authors declare that the research was conducted in the absence of any commercial or financial relationships that could be construed as a potential conflict of interest.
The reviewer WM declared a shared affiliation with the authors JT and MZ.
Publisher’s note
All claims expressed in this article are solely those of the authors and do not necessarily represent those of their affiliated organizations, or those of the publisher, the editors and the reviewers. Any product that may be evaluated in this article, or claim that may be made by its manufacturer, is not guaranteed or endorsed by the publisher.
Supplementary material
The Supplementary Material for this article can be found online at: https://www.frontiersin.org/articles/10.3389/fevo.2022.1012071/full#supplementary-material
References
Ampuero, A., Stríkis, N. M., Apaéstegui, J., Vuille, M., Novello, V. F., Espinoza, J. C., et al. (2020). The forest effects on the isotopic composition of rainfall in the Northwestern amazon basin. J. Geophys. Res. Atmos. 125:e2019JD031445. doi: 10.1029/2019JD031445
Baker, J. C. A., and Spracklen, D. V. (2022). Divergent representation of precipitation recycling in the amazon and the Congo in CMIP6 models. Geophys. Res. Lett. 49:e2021GL095136. doi: 10.1029/2021GL095136
Çiçek, I., and Turkoglu, N. (2005). Urban effects on precipitation in Ankara. Atmosfera 18, 173–187. doi: 10.1016/j.watres.2021.117866
Cluett, A. A., Thomas, E. K., Evans, S. M., and Keys, P. W. (2021). Seasonal variations in moisture origin explain spatial contrast in precipitation isotope seasonality on coastal western Greenland. J. Geophys. Res. Atmos. 126:e2020JD033543. doi: 10.1029/2020JD033543
Craig, H., and Gordon, L. (1965). Deuterium and oxygen 18 variations in the ocean and the marine atmosphere. Available online at: http://yncenter.sites.yale.edu/sites/default/files/shen_jing_jan_2013.pdf (accessed April, 2020).
Cui, J., Lian, X., Huntingford, C., Gimeno, L., Wang, T., Ding, J., et al. (2022). Global water availability boosted by vegetation-driven changes in atmospheric moisture transport. Nat. Geosci. 15, 982–988. doi: 10.1038/s41561-022-01061-7
Dawson, T. E., and Ehleringer, J. R. (1991). Streamside trees that do not use stream water. Nature 350, 335–337. doi: 10.1038/350335a0
Dow, C. L., and DeWalle, D. R. (2000). Trends in evaporation and Bowen Ratio on urbanizing watersheds in eastern United States. Water Resour. Res. 36, 1835–1843. doi: 10.1029/2000WR900062
Froehlich, K., Gonfiantini, R., and Aggarwal, P. (2004). Isotope hydrology at IAEA: History and activities. Wallingford: IAHS-AISH Publication, 125–132.
Froehlich, K., Kralik, M., Papesch, W., Rank, D., Scheifinger, H., and Stichler, W. (2008). Deuterium excess in precipitation of Alpine regions–moisture recycling. Isotopes Environ. Health Stud. 44, 61–70. doi: 10.1080/10256010801887208
Gimeno, L., Nieto, R., and Sorí, R. (2020). The growing importance of oceanic moisture sources for continental precipitation. NPJ Clim. Atmos. Sci. 3:27. doi: 10.1038/s41612-020-00133-y
Good, S. P., Noone, D., Kurita, N., Benetti, M., and Bowen, G. J. (2015). D/H isotope ratios in the global hydrologic cycle. Geophys. Res. Lett. 42, 5042–5050. doi: 10.1002/2015GL064117
Griffis, T. J., Wood, J. D., Baker, J. M., Lee, X., Xiao, K., Chen, Z., et al. (2016). Investigating the source, transport, and isotope composition of water vapor in the planetary boundary layer. Atmos. Chem. Phys. 16, 5139–5157. doi: 10.5194/acp-16-5139-2016
Hamdi, R., Deckmyn, A., Termonia, P., Demarée, G. R., Baguis, P., Vanhuysse, S., et al. (2009). Effects of historical urbanization in the Brussels Capital Region on surface air temperature time series: A model study. J. Appl. Meteorol. Clim. 48, 2181–2196.
Hobson, K. A., Kardynal, K. J., and Koehler, G. (2019). Expanding the isotopic toolbox to track monarch butterfly (Danaus plexippus) origins and migration: On the utility of stable oxygen isotope (δ18O) measurements. Front. Ecol. Evol. 7:224. doi: 10.3389/fevo.2019.00224
Huff, F. A., and Changnon, S. A. (1972). Climatological assessment of urban effects on precipitation at St. Louis. J. Appl. Meteorol. 11, 823–842.
Jiang, Y., and Weng, Q. (2017). Estimation of hourly and daily evapotranspiration and soil moisture using downscaled LST over various urban surfaces. Gisci. Remote Sens. 54, 95–117. doi: 10.1080/15481603.2016.1258971
Li, Z., Chan, J. C. L., Zhao, K., and Chen, X. (2021). Impacts of urban expansion on the diurnal variations of summer monsoon precipitation over the South China coast. J. Geophys. Res. Atmos. 126:e2021JD035318. doi: 10.1029/2021JD035318
Liu, J., and Niyogi, D. (2019). Meta-analysis of urbanization impact on rainfall modification. Sci. Rep. 9:7301. doi: 10.1038/s41598-019-42494-2
Martens, B., Miralles, D. G., Lievens, H., Van Der Schalie, R., De Jeu, R. A. M., Fernández-Prieto, D., et al. (2017). GLEAM v3: Satellite-based land evaporation and root-zone soil moisture. Geosci. Model Dev. 10, 1903–1925. doi: 10.5194/gmd-10-1903-2017
Masson-Delmotte, V., Zhai, P., Pirani, A., Connors, S. L., Péan, C., Berger, S., et al. (2021). Climate change 2021: The physical science basis. Contribution of working group I to the sixth assessment report of the intergovernmental panel on climate change. Cambridge: Cambridge University Press.
Mrekva, L., Engi, Z., and Tóth, G. (2012). Urban flood risk and hydrology. Z. Geomorphol. 56, 21–35. doi: 10.1127/0372-8854/2012/S-00083
Pang, Z., Kong, Y., Froehlich, K., Huang, T., Yuan, L., Li, Z., et al. (2011). Processes affecting isotopes in precipitation of an arid region. Tellus Ser. B Chem. Phys. Meteorol. 63, 352–359. doi: 10.1111/j.1600-0889.2011.00532.x
Peng, H., Mayer, B., Norman, A. L., and Krouse, H. R. (2005). Modelling of hydrogen and oxygen isotope compositions for local precipitation. Tellus Ser. B Chem. Phys. Meteorol. 57, 273–282. doi: 10.1111/j.1600-0889.2005.00145.x
R Core Development Team (2020). A language and environment for statistical computing. Vienna: R Core Team.
Rao, A. R. (1980). Stochastic analysis of annual rainfall affected by urbanization. J. Appl. Meteorol. 19, 41–52. doi: 10.1016/j.watres.2019.115372
Ren, G., Zhou, Y., Chu, Z., Zhou, J., Zhang, A., Guo, J., et al. (2008). Urbanization effects on observed surface air temperature trends in North China. J. Clim. 21, 1333–1348.
Risi, C., Noone, D., Frankenberg, C., and Worden, J. (2013). Role of continental recycling in intraseasonal variations of continental moisture as deduced from model simulations and water vapor isotopic measurements. Water Resour. Res. 49, 4136–4156. doi: 10.1002/wrcr.20312
Sang, Y. F., and Yang, M. (2017). Urban waterlogs control in China: More effective strategies and actions are needed. Nat. Hazards 85, 1291–1294. doi: 10.1007/s11069-016-2614-4
Singh, H. K. A., Bitz, C. M., Donohoe, A., and Rasch, P. J. (2017). A source-receptor perspective on the polar hydrologic cycle: Sources, seasonality, and arctic-antarctic parity in the hydrologic cycle response to CO2 doubling. J. Clim. 30, 9999–10017. doi: 10.1175/JCLI-D-16-0917.1
Song, X., Zhang, J., AghaKouchak, A., Roy, S. S., Xuan, Y., Wang, G., et al. (2014). Rapid urbanization and changes in spatiotemporal characteristics of precipitation in Beijing metropolitan area. J. Geophys. Res. Atmos. 119, 11250–11271.
Trenberth, K. E., Dai, A., Rasmussen, R. M., and Parsons, D. B. (2003). The changing character of precipitation. Bull. Am. Meteorol. Soc. 84, 1205–1217. doi: 10.1175/BAMS-84-9-1205
Van Der Ent, R. J., Wang-Erlandsson, L., Keys, P. W., and Savenije, H. H. G. (2014). Contrasting roles of interception and transpiration in the hydrological cycle–part 2: Moisture recycling. Earth Syst. Dyn. 5, 471–489. doi: 10.5194/esd-5-471-2014
Wang, J., Wang, H., Gao, X., Liu, J., Chen, S., and Shao, W. (2014). Review on urban hydrology. Chin. Sci. Bull. 59, 3581–3590. doi: 10.1360/n972014-00327
Wang, S. J., Zhang, M. J., Che, Y. J., Zhu, X. F., and Liu, X. M. (2016). Influence of below-cloud evaporation on deuterium excess in precipitation of arid central Asia and its meteorological controls. J. Hydrometeorol. 17, 1973–1984. doi: 10.1175/Jhm-D-15-0203.1
Wang, C., Yang, J., Myint, S. W., Wang, Z. H., and Tong, B. (2016). Empirical modeling and spatio-temporal patterns of urban evapotranspiration for the phoenix metropolitan area, Arizona. Gisci. Remote Sens. 53, 778–792. doi: 10.1080/15481603.2016.1243399
Wang, S., Zhang, M., Che, Y., Chen, F., and Qiang, F. (2016). Contribution of recycled moisture to precipitation in oases of arid central Asia: A stable isotope approach. Water Resour. Res. 52, 3246–3257. doi: 10.1002/2015WR018135
Xia, C., Liu, G., Chen, K., Hu, Y., Zhou, J., Liu, Y., et al. (2020). Stable isotope characteristics for precipitation events and their responses to moisture and environmental changes during the summer monsoon period in southwestern China. Pol. J. Environ. Stud. 29, 2429–2445. doi: 10.15244/pjoes/110445
Yang, L., Ni, G., Tian, F., and Niyogi, D. (2021). Urbanization exacerbated rainfall over European suburbs under a warming climate. Geophys. Res. Lett. 48:e2021GL095987. doi: 10.1029/2021GL095987
Yoshimura, K., Kanamitsu, M., Noone, D., and Oki, T. (2008). Historical isotope simulation using reanalysis atmospheric data. J. Geophys. Res. 113:108. doi: 10.1029/2008JD010074
Yuan, Y., Zhai, P., Li, J., and Chen, Y. (2017). Changes in classifed precipitation in the urban, suburban and mountain areas of Beijing. Adv. Clim. Change Res. 8, 279–285. doi: 10.1016/j.accre.2017.10.002
Zhang, F., Huang, T., Man, W., Hu, H., Long, Y., Li, Z., et al. (2021). Contribution of recycled moisture to precipitation: A modified D-Excess-based model. Geophys. Res. Lett. 48:e2021GL095909.
Keywords: hydrogen and oxygen stable isotopes, recycled vapor ratio, evapotranspiration, urbanization, precipitation
Citation: Zhao P, Xia C, Liu G, Tang J and Zhou M (2023) Comparison of recycled vapor contribution to precipitation in urban vs. rural area—A case study in western China. Front. Ecol. Evol. 10:1012071. doi: 10.3389/fevo.2022.1012071
Received: 05 August 2022; Accepted: 22 November 2022;
Published: 11 December 2023.
Edited by:
Farrukh Chishtie, The University of British Columbia, CanadaReviewed by:
Svetlana Botsyun, University of Tübingen, GermanyWeiqiang Ma, Institute of Tibetan Plateau Research (CAS), China
Nilendu Singh, Free University of Bozen-Bolzano, Italy
Copyright © 2023 Zhao, Xia, Liu, Tang and Zhou. This is an open-access article distributed under the terms of the Creative Commons Attribution License (CC BY). The use, distribution or reproduction in other forums is permitted, provided the original author(s) and the copyright owner(s) are credited and that the original publication in this journal is cited, in accordance with accepted academic practice. No use, distribution or reproduction is permitted which does not comply with these terms.
*Correspondence: Guodong Liu, liugd988@163.com
†These authors have contributed equally to this work