Initial herbivory and exposure to herbivory-induced volatiles enhance arthropod species richness by diversifying community assemblages
- 1Faculty of Agriculture, Kindai University, Nakamachi, Nara, Japan
- 2Center for Ecological Research, Kyoto University, Otsu, Shiga, Japan
- 3Center for Biodiversity Science, Ryukoku University, Otsu, Shiga, Japan
- 4Faculty of Advanced Science and Technology, Ryukoku University, Otsu, Shiga, Japan
Plant ecological traits affect the species identity of plant-colonizing arthropods, which in turn induces species-specific trait changes in plants, forming feedback between plants and arthropods. Such feedback can amplify initial differences in species composition, leading to large variations (i.e., high β diversity). We hypothesized that the differences in plant initial conditions have sustained effects on arthropod community composition and species richness. To test this hypothesis, we monitored arthropod community assembly on a willow tree species, Salix eriocarpa, which was experimentally manipulated into three initial treatments: undamaged (in chamber 1); damaged by the specialist leaf beetle, Plagiodera versicolora (chamber 2); and “exposed” plants that were undamaged but were exposed to volatiles from damaged plants (in chamber 2). The arrival and population dynamics of the leaf beetle were affected by the plant’s initial condition (chamber 1 vs. 2), which could result from the microscale environmental heterogeneity between chambers (chamber effect) and/or from the herbivory-related impacts (direct herbivory and exposure to induced volatiles in chamber 2). The community composition on damaged and exposed plants became significantly different on day 32. In addition, the divergence in composition between plant individuals was significantly smaller in undamaged plants (chamber 1) than in damaged and exposed plants (chamber 2) on day 60. The compositional variations (β diversity) between chambers, between treatments, and between days, comprised a large proportion (two third) of the total species richness (γ diversity) in the whole community of arthropods. These results suggest that plant initial condition is a key driver of community assembly and the maintenance of species diversity.
Introduction
Arthropod communities on plants are one of the model systems for community assembly studies (Lewinsohn et al., 2005; Barber and Marquis, 2011; Pechal et al., 2014). Growing evidence demonstrates that diverse plant responses induced by herbivory are major drivers of population and community dynamics of local arthropod communities (Ohgushi, 2005), which determine the local diversity of arthropods (Nakamura et al., 2006). For example, induced direct resistance and compensative regrowth influence the subsequent arrival, survival, and reproduction of other herbivores (Ohgushi, 2005) and their natural enemies (Nakamura et al., 2005). It follows that population dynamics (Underwood, 1999) and species composition and richness (Utsumi and Ohgushi, 2009) would be affected by these plant responses. Herbivory-induced plant volatiles (HIPVs) also influence arthropods. HIPVs affect the behavior of herbivores and carnivores (Yoneya and Miki, 2015 and references therein) and can serve as indirect defense (Heil, 2004; Arimura et al., 2009; Turlings and Erb, 2018). HIPVs affect herbivore communities and their natural insect predators (Shiojiri et al., 2009; Fatouros et al., 2012; Xiao et al., 2012). One of the intriguing functions of HIPVs is to mediate communication between plants. Neighboring plants receive HIPVs and theninduce direct and indirect defenses (Arimura et al., 2000; Choh and Takabayashi, 2006; Karban et al., 2014; Yoneya et al., 2014b) by releasing carnivore attractants (Piesik et al., 2012) and affecting herbivore and carnivore dispersal (Morrell and Kessler, 2017). Communication between plants has potentially profound effects on community dynamics. Compared to the effects of plant responses to direct herbivory (Utsumi and Ohgushi, 2009; Xiao et al., 2012), the effects of communication between plants on herbivore population dynamics, and arthropod community composition and species richness, remain relatively underexplored. Through spatial propagation of defense responses by communication between plants, plant volatiles have impacts on arthropod communities at a larger spatial scale than herbivore-induced direct defenses (Morrell and Kessler, 2017).
Which arthropod species colonizes a plant first depends on the plant’s ecological traits. In turn, the arthropod colonization induces species-specific plant changes, forming feedback dynamics between plant traits and arthropod composition. Following the “priority effect” concept in community assembly theory (Chase, 2003; Fukami et al., 2005), such feedback between plants and arthropods (Utsumi et al., 2010) is expected to amplify initial differences in the species composition of arthropod communities on plants (i.e., high β diversity; Anderson et al., 2011) leading to large variations. Therefore, we hypothesize that the initial condition of plants can have sustained effects on arthropod community composition and species richness. In addition, even starting from identical plant initial conditions, such feedback may induce divergence in species composition between individual plants when early-colonizing species differ between plants.
To test these hypotheses, we focused on arthropod communities on a willow tree species, Salix eriocarpa. For this species, we have accumulated knowledge of (1) arthropod communities (Nakamura et al., 2005; Utsumi and Ohgushi, 2009; Yoneya and Takabayashi, 2013), (2) the chemical characteristics of plant volatiles (Yoneya et al., 2009a, 2010), and (3) their impacts on the behavior of herbivorous and predatory arthropods (Yoneya et al., 2009a, 2009b, 2010; Yoneya and Takabayashi, 2013). In addition, the plant volatiles induced by the leaf beetle Plagiodera versicolora negatively affect the tissue quality of the neighboring conspecifics, which is suggested by the reduced survival rate and elongated developmental duration of the P. versicolora’s larva feeding on these conspecifics (Yoneya et al., 2014b). We monitored arthropod community assembly on plants manipulated into three initial conditions: undamaged; damaged by the specialist leaf beetle, Plagiodera versicolora; and “exposed” (plants that were undamaged but were exposed to volatiles from damaged conspecific plants).
Materials and methods
Plants
We cut a total of 80, 2- or 3-year-old and 18 cm-long shoots from five S. eriocarpa trees in the floodplain of the Yasu River (35°N, 136°E) in Siga Prefecture, central Japan in early May 2008. We potted the shoots individually in humus (15 cm diameter × 13 cm high pots) and maintained them in a climate-controlled chamber (25°C ± 3, L18:D6) until new leaves emerged (ca. 30 d). We then transferred the potted shoots to a greenhouse (25°C ± 3, L18,D6) and supplied the fertilizer (Hyponex, HYPONeX Japan, Osaka, Japan) every 2 weeks for 2 months.
We maintained the colony of P. versicolora on willow leaves in a climate-controlled chamber [LD 18:6, 25 ± 2°C, relative humidity (RH) 50–70%]. We reared the adults, which emerged at the same timing, with fresh willow leaves for 2–3 days until sexual maturation, and then used them for plant preconditioning. We inoculated one female and one male P. versicolora on each of 16 undamaged plants and covered each of them with a mesh bag (30 × 70 cm), which represented damaged (infested) plants (D). Then, we put these damaged plants together with 16 undamaged (uninfested) plants that were covered with a mesh bag (30 × 70 cm) in a greenhouse (25 3°C, 50–60 RH, L18:D6) at the same day when adult leaf beetles started feeding on plants and kept them together for 10 days to expose undamaged plants to volatiles from the damaged plants (D). We allowed these adult leaf beetles to feed on leaves and lay eggs for 3 days, then removed the adults from the damaged plants at day 4. Before removing the adults, the female laid one or two clutches of eggs on the leaves. Larvae hatched from the eggs from day 4 to 6. There were at least 10 larvae feeding on a plant. We allowed feeding by these larvae for 5 to 7 days just before transferring the plants to our experimental garden. Hereafter, these undamaged plants maintained together with damaged plants (D) in a greenhouse were called exposed plants (E). As a control, we prepared 16 undamaged plants (U) that had been exposed to volatiles from 16 uninfested plants under the same conditions in another greenhouse (25 3°C, 50–60 RH, L18, D6) with the same standard as that maintained damaged (D) and exposed plants (E). These two greenhouses were located at the Center for Ecological Research (CER) of Kyoto University (34° 58′ 17″ N, 135° 57′ 32″ E, Otsu, Japan, 10.6 km south of the Yasu River). We used 16 exposed plants (E), 16 undamaged plants (U), and 16 damaged plants (D) for the field experiments. Leaf beetles on damaged plants were removed before the field experiment to exclude the direct effects of the presence of the leaf beetles on subsequent colonization by arthropods.
Population and community monitoring
We transferred 48 plants (16 plants of undamaged, damaged, and exposed) to a path in one of the experimental gardens of the CER at 06:00 on 10 June 2008, where willow trees of S. eriocarpa, S. integra, S. miyabiana, S. triandra, had been grown for more than 10 years. We placed the plants at 1 m intervals in two lines (at 2 m between lines). Each line had four plots containing six plants, which had been subjected to three different treatments (undamaged, exposed, and damaged; two plants for each treatment, a total of 16 plants for each treatment). The location of the plants in the plots was randomized. We counted the number of arthropods on plants every hour from 07:00 until 12:00 (six observations in total), every 2 h until 18:00 (three observations in total), and at 06:00 the next day to investigate the first immigration (phase 1). We continued the census at 09:00 and 16:00 every day until day 8 (phase 2). Thereafter, we censused on day 10 at 9:00 and 16:00 and day 32, and 60 at 9:00, 16:00, and 9:00 the next day (phase 3). There are accumulated knowledge of arthropod community on Salix eriocarpa (e.g., Nakamura et al., 2006; Utsumi et al., 2009; Utsumi and Ohgushi, 2009; Yoneya et al., 2014a) in Japan. We identified every arthropod species except spiders and flies (see Supplementary Table S1), which we found by eyes, using loupes and digital camera (μ1020, Olympus Co., Tokyo) without collecting them. Spiders were categorized in four groups by size (less than 5 mm excluding legs, or more) and behavior (having spiderweb or not) (Supplementary Table S1). Among flies, only parasitoids (2 species) of P. versicolora were identified and the other flies were grouped into a group as “the other flies” (see Supplementary Table S1).
Statistical analysis
Due to logistical constraints, we could not prepare multiple greenhouses for each of the different plant initial conditions as replications. It was also ineffective to exchange plant individuals between greenhouses in the middle of the preconditioning period (10 days) for avoiding chamber effects since the remaining plant volatiles in greenhouses were expected to substantially affect plant physiology. Since interannual variations in community dynamics are high in general under seasonal environments (Dakos et al., 2009), it was not realistic to design multiyear replications. Therefore, there were potentially so-called chamber effects (or pseudoreplication issues: Oksanen, 2001; Porter et al., 2015) between plant individuals preconditioned in two greenhouses; hereafter we call the first greenhouse with U plants and the second one with D and E plants chamber C1 and chamber C2, respectively.
By acknowledging the chamber effects, all of our statistical tests followed two steps; we firstly compared plants between two chambers and secondly compared damaged and exposed plants from chamber C2. The differences detectable in the first step would include both chamber effects (i.e., effects of microscale heterogeneity of environments such as temperature and humidity) and herbivory-related impacts while those in the second step would represent the difference between HIPV-exposure effects and direct damage effects but do not include chamber effects. Even if it was impossible to distinguish chamber effects from treatment effects, what we intended to detect were the sustained impacts of plant initial conditions (including chamber effects) on the subsequent community assembly.
We used the gls function in the nlme package, including temporal autocorrelation structure of order one [i.e., AR(1)] for a repeated measures ANOVA to investigate the differences in population dynamics of P. versicolora between chambers for each phase. For phases 2 and 3, we used the averages of within-day replicates. We also conducted post-hoc t-test between chambers and between damaged and exposed plants for specific time points. For the following analyses of species composition and richness, we excluded P. versicolora data since large P. versicolora populations could mask the roles of other species in the community structure and diversity. Indeed, including the P. versicolora data made results unclear (see Supplementary Figure S1). We conducted all of the following statistical analyses using the vegan package (Oksanen et al., 2018) in R4.1.3 (R Core Team, 2018). All of the raw data sets, R notebook code, and R notebook html are available as Supplementary material.
To investigate sustained shifts in community composition, we first calculated the representative community composition from the repeated observations for each plant individual within a day (on days 10, 32, and 60; Supplementary Appendix S1). In the second step, for the representative community composition from days 10, 32, and 60, we used PERMDISP (vegan::betadisper) and PERMANOVA (vegan::adonis) analyses to compare the community divergence between individual plants within each condition (i.e., distance to the centroid of each plant initial condition) and the differences in community composition (centroid position) between chambers (C1 and C2) and two treatments (damaged, and exposed), respectively (Anderson, 2006; see Supplementary Appendix S1; Supplementary Figure S1 for additional analysis).
When quantifying species diversity, we only considered species richness, i.e., the number of species. Because species identification for some arthropods such as flies and spiders was difficult, a few taxonomic units in our analyses potentially include multiples species (Supplementary Table S1). However, for simplicity, we use the term “species” richness in this study as the number of the identified taxonomic units. We focused on the average species richness on an individual plant and the total richness across all of the individuals within the same plant treatment or chamber. We also standardized the total species richness by the coverage-based rarefaction (Chao and Jost, 2012) from presence/absence (incidence) data on each plant individual (Colwell et al., 2004), as well as its asymptotic estimate (Chao index: Chao et al., 2006), using the iNEXT package. For both the coverage-based and asymptotic estimates, we assumed a significant difference when the 95% confidence intervals of the estimates did not overlap. We further hierarchically partitioned the total richness (i.e., γ diversity) into α and β diversity to compare the richness difference between chambers, treatments, and days (Crist et al., 2003; Supplementary Table S2 and Supplementary Figure S5).
Results
Leaf beetle population
We observed seven species of leaf beetles on plants. Plagiodera versicolora was the dominant species among all observed arthropods and the other leaf beetles were rarely observed (Supplementary Table S). For example, Smaragdina semiaurantiaca was the most frequently observed among the other leaf beetles, however, only one time during phase 1 and four times thereafter. We only used the data of P. versicolora, for the following analysis. The arrival of adult beetles in phase 1 (≤ 24 h) differed between chambers (repeated measures ANOVA, p = 0.0285). The population size of adult leaf beetles per individual tree was greater in the chamber C1 than those in the chamber C2 at 12 h and 24 h, respectively (p = 0.015, 0.027) while there were no differences between damaged and exposed plants (p = 0.33, 0.33) (Figure 1A). However, there were no differences between chambers in phases 2 (2–7 d, ANOVA, p = 0.725) and 3 (10–60 d, ANOVA, p = 0.135) (Figures 1B,C). On day 10, the average population size on damaged plants was lower than that on exposed plants (t-test: p = 0.041).
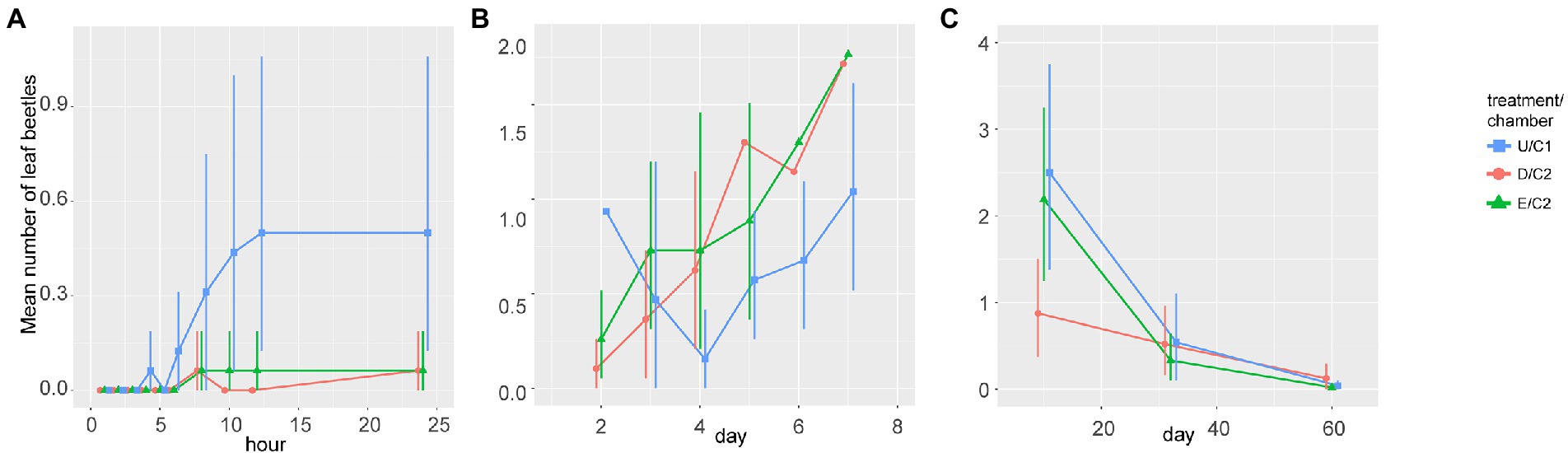
Figure 1. Population dynamics of leaf beetles, Plagiodera versicolora. Comparison between the number of adults per plant among undamaged (U), exposed (E), and damaged (D) conditions in phase 1 (A), phase 2 (B), and phase 3 (C). Bars represent 95% confidence intervals.
Community composition
In phase 3 (day 10, 32, 60), the total number of observed species was 48, which includes herbivores, predators, and parasitoids (Supplementary Table S1). Based on the rank abundance curves (Supplementary Figure S2), we identified the top 10 abundant species, which consisted of 7 herbivores, 2 predators, and 1 parasitoid. Some of these groups demonstrated a temporal succession. For example, the spider groups <5 mm with spiderweb and without spiderweb tended to be abundant on day 32 (Supplementary Figures S3A,F), and the leaf roller, Phyllocolpa sp., appeared only on day 60. However, the other groups did not show such clear patterns (Supplementary Figure S3). The following analyses were based on the dissimilarity of the overall community composition between individual plants, chambers, and treatments. The community divergence (i.e., distance to centroid) between plant individuals in the chamber C1 (U plants) depended on dates (PERMDISP: df = 2, F = 7.1717, p = 0.0021). In particular, the divergence was smaller on day 60 than on day 10 and on day 32 (p = 0.0055 and p = 0.0168). Such temporal differences were not observed in damaged plants (PERMDISP: df = 2, F = 0.3821, p = 0.6793) and exposed plants (PERMDISP: df = 2, F = 1.1044, p = 0.3376; Figure 2A). Then, on day 60, the community divergence was smaller in chamber C1 (undamaged plants) than chamber C2 (damaged + exposed plants) (PERMDISP: df = 1, F = 7.9155, p = 0.0092). The community composition (centroid position) was not significantly different between the chambers on any day but there was a difference between damaged and exposed on day 32 (PERMANOVA: df = 1, F = 2.4132, p = 0.0043, Figure 2B). On day 60, although there were significantly greater inter-chamber variations than intra-chamber variations (undamaged vs. damaged + exposed, PERMANOVA: df = 1, F = 1.8611, p = 0.0365), it was not possible to conclude that the centroid position was different between them due to the heterogeneous dispersion (PERMDISP as shown above). On day 60, there was no difference in the centroid position between damaged and exposed (PERMANOVA: df = 1, F = 1.4895, p = 0.1079). There were also significant differences in the centroid between sampling days within damaged plants and within exposed plants (PERMANOVA, p = 0.0001).
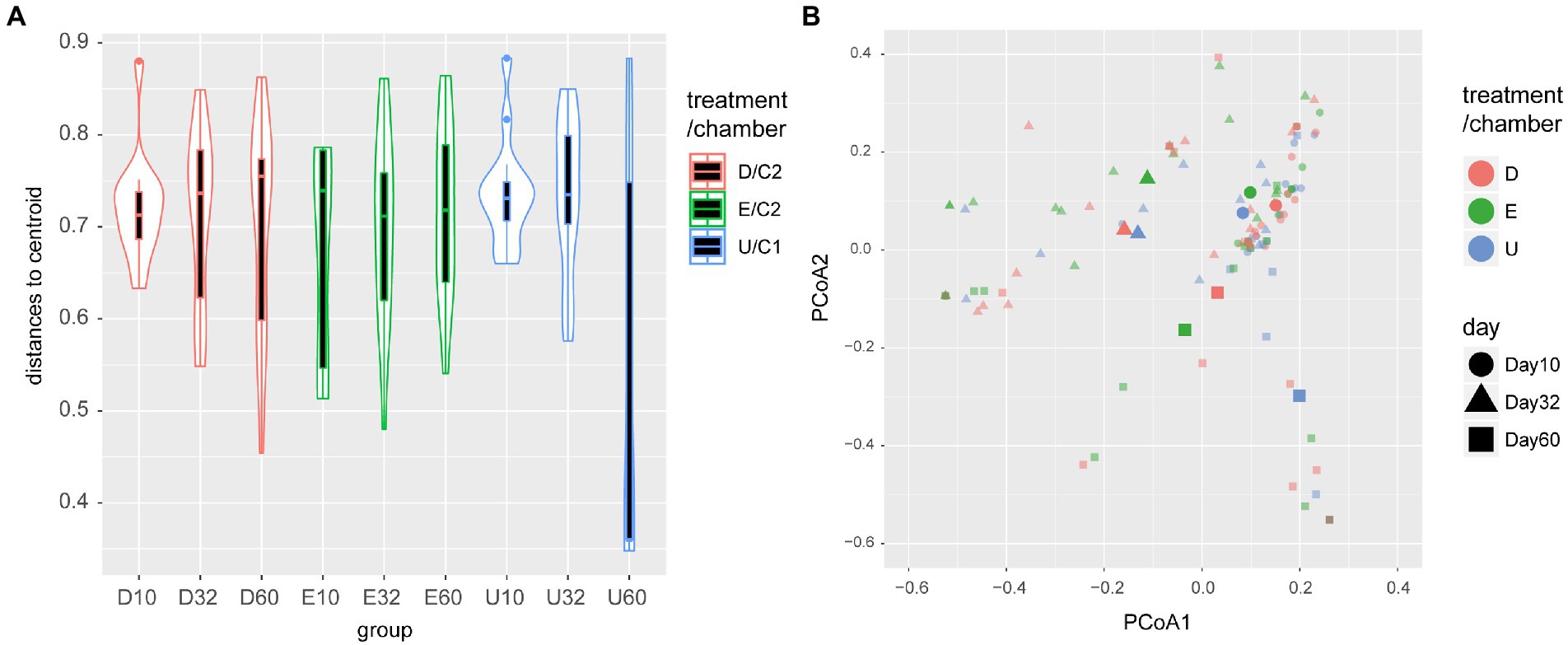
Figure 2. Community composition depending on plant initial conditions and time. (A) Divergence of community composition between plant individuals within each plant initial condition. Only for undamaged plants (chamber C1), there was a significant difference between days. In addition, on day 60, the divergence of composition in chamber C1 was smaller than in Chamber C2 (PERMDISP, p = 0.0092) (B) PCoA ordination and plant initial conditions (undamaged, damaged, or exposed) and sampling day (10, 32, or 60). The points with large marks (circle, triangle, and rectangle) are the spatial medians, which are representative of the community composition of each plant initial condition at each sampling day. Small marks represent each plant individual.
Species richness
There were no differences in the observed average species richness per individual plant between plant initial conditions on day 10, but plants in chamber C2 (exposed and damaged plants) showed statistically greater richness than those in chamber C1 (undamaged plants) on days 32 and 60 but the differences were minor (Supplementary Appendix S1; Supplementary Figure S4, using function vegan::renyi). For the standardized species richness for each treatment, damaged plants harbored greater richness than exposed plants (Figure 3A: on day 32 and as the whole period). In addition, because of the compositional differences between the three initial conditions (Figure 2B), the total species richness for all three plant initial conditions was greater than species richness for any individual condition (UED > U, E, and D in Figure 3A; Supplementary Table S3). Similarly, due to the temporal changes in composition (represented by different shapes in Figure 2B), for all of the combinations (U, E, D, and UED), the species richness standardized for the whole period was significantly greater than the estimates for each sampling day, except for the exposed plants on day 10 (Figure 3A). Finally, asymptotic species richness for all conditions combined (UED) was greater than that of chamber C1 (U, undamaged), and that of C2 (E + D, exposed + damaged) was greater than that of C1 (U, undamaged) (Figure 3B). Diversity decomposition indicated that β diversities between chambers/treatments/time (=32 in total) comprised a greater proportion of γ diversity (=48) than β diversity between individual plants (=13.84) and α diversity per plant (=2.16) (Figure 3C; Supplementary Appendix S1).
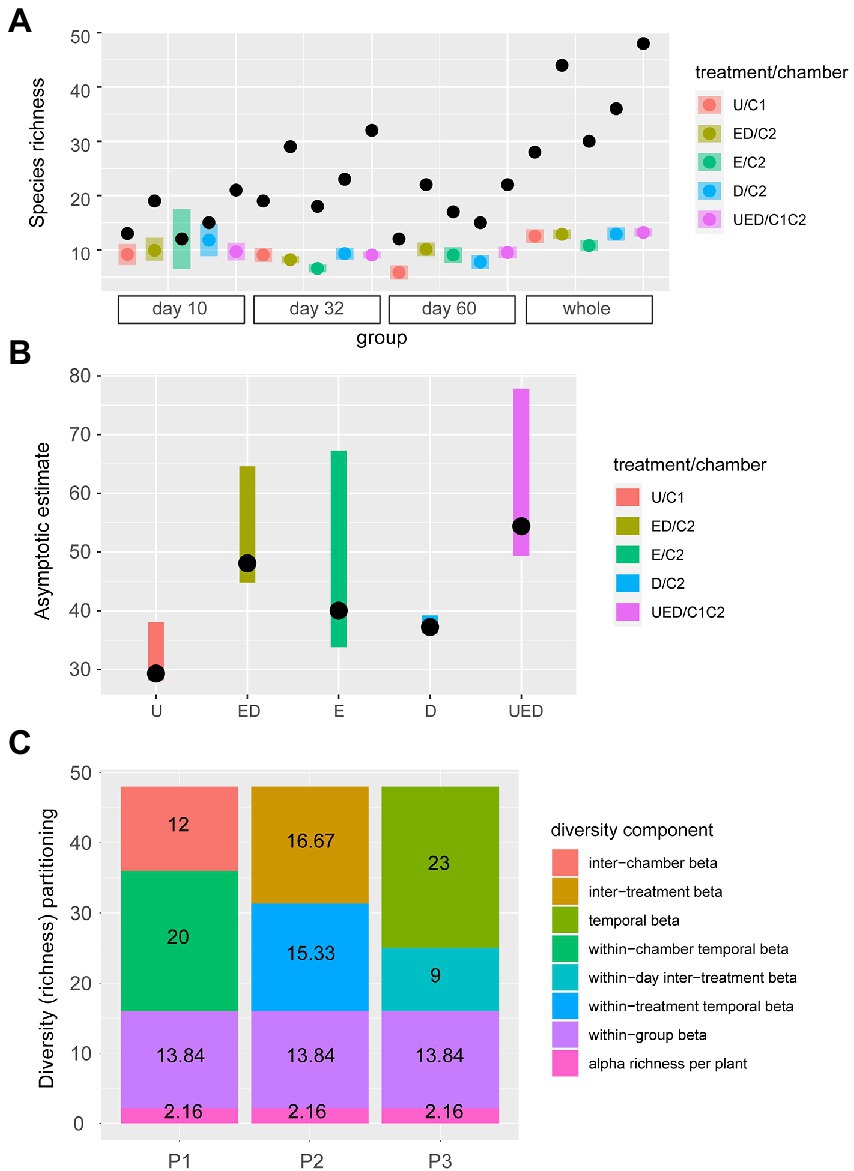
Figure 3. Variation in species richness. (A) Species richness standardized by coverage (68%) depending on the plant initial conditions (treatments: undamaged (U), damaged (D), or exposed (E) and chamber C1 and C2 (ED), sampling day (10, 32, or 60), or combined (UED). The black points, colored points, and bars represent the observed richness, the average of the estimated richness, and 95% confidence intervals of the estimate, respectively. The results were grouped into each sampling day (day 10, day 32, and day 60), and the whole period (whole). (B) Asymptotic estimate of species richness. The points and bars represent the point estimate of asymptotic richness and its 95% confidence intervals. (C) Partitioning of γ species diversity. For all three partitioning methods (P1, P2, and P3), the α diversity (average richness per individual per sampling day) and within-group β diversity (variations within 16 replicates per treatment per sampling day) were common. The calculations are presented in Supplementary Appendix S1; Supplementary Figure S5; and Supplementary Table S2 in the Supplementary material.
Discussion
Sustained effects of plant preconditioning on community assemblage
This study clearly illustrates the sustained effects of short-term plant preconditioning for 10 days on species community assemblages for 60 days. Since no plant trait was measured and logistical limitations made it challenging to fully separate treatment effects from chamber effects it is difficult to identify the mechanisms with which species immigration and population dynamics were influenced by plant initial conditions (undamaged, exposed, or damaged). However, it would be possible to narrow down the likely mechanisms. The differences observed in community composition and species richness between damaged and exposed plants imply the substantial effect of direct herbivory on plant qualities and traits and then community assembly without any chamber effects. Therefore, we would argue that the differences between chambers (undamaged plants vs. exposed and damaged plants) did not result merely from the unmeasurable environmental heterogeneity, that is, the chamber effects. Rather, it would be likely that those include the effects of herbivory-related preconditioning, the direct damages and exposure to the damaged plants, as well.
Herbivory-related preconditioning hindered the early arrival within 24 h of P. versicolora compared to undamaged plants probably because these plants were poorer quality resources (Yoneya et al., 2014b) and thus were avoided by the leaf beetles. In fact, the previous bioassay experiment demonstrates that the exposure of S. eriocarpa to airborne factors from damaged conspecifics negatively affected the performance of P. versicolora, e.g., the survival rate of its larvae, developmental duration, and pupal weight (Yoneya et al., 2014b). At the same time, it could result simply from the chamber effect; the microscale environment (e.g., temperature and moisture) in chamber C2 (exposed and damaged plants) acted as mild stresses, leading to poorer plant quality of exposed and damaged plants, independently of the herbivory-related preconditioning. Nevertheless, the evidence that damaged plants harbored smaller population size than exposed plants on day 10 (Figure 1C) implies a negative effect of direct herbivory on plant resource quality. This would be because of the induced resistance as it is common in Salicaceae trees (Clausen et al., 1989; Raupp and Sadof, 1989; Simon and Hilker, 2003). However, the differences in the averaged population sizes between the three conditions over a longer time scale >30 days were unexpectedly small and also not statistically significant. This implies that the effects of plant initial conditions including chamber effects were weakened with the sequential arrival of other species. In contrast, our hypotheses as to the sustained effects of initial plant conditions on species composition and their contribution to enhancing species richness were supported. The differentiation in species composition between exposed and damaged plants became substantial on day 32.
One may argue that the induced responses to herbivory and exposure to VOCs and their impacts on arthropod communities of woody plants last longer than those of herbaceous plants. For example, herbivore-induced regrowth and nutritious changes last several months in willow systems (Nakamura et al., 2006) while the induced resistance in a soybean system only lasts 2 weeks (Srinivas et al., 2001). However, even on a herbaceous plant, the presence of a keystone herbivore, such as aphid on tall goldenrod, and plant induced responses to it can have monthly-scale impacts on arthropod communities (Ando et al., 2017). The longevity of the induced responses, the individual plant size, the complexity of modular structures, and their effects on the degree of within-individual heterogeneity of plant traits could all contribute to potential differences in arthropod communities between herbaceous and woody plants. Large willow trees have relatively high complexity of structure and within-individual heterogeneity of nutritious quality affects arthropod communities and species diversity (Utsumi et al., 2009). Since we conducted the experiments using the potted small willow trees, the observed differences between individual trees within each preconditioning and between preconditioning can be realized as variations between modules within a single large tree in natural environments where within-tree signaling via VOCs is common for the systemic responses (Frost et al., 2007).
Chamber effects
The likely environmental heterogeneity between greenhouses (chamber C1 and C2) included temperature (25 3°C) and humidity (50–60 RH), which could act as mild stress (high temperature and drought stress) and affect the emission of VOCs (Niinemets, 2010). However, the induced increases in VOC emission quickly recover to the normal level after the stress release. For example, the levels of emission of isoprene and monoterpene were recovered or lower than those before heating in 2 h (Loreto et al., 1998, 2006). Isoprene emission levels were recovered 12 days after mild drought stress (Pegoraro et al., 2004) while such recovery needed longer than months for severer drought stress (Bertin and Staudt, 1996). Since it is difficult to imagine the presence of severe stresses in the climate-controlled greenhouses, it was unlikely that direct effects of 10 day’s mild stress (if any) during the preconditioning on plant physiology were sustained for 60 days after stress release. Nevertheless, it is still worth noting that microscale environmental heterogeneity, even without herbivory-related effects at all, had sustained influences on community assembly, not because of sustained direct effects of environmental stress on plant physiology but probably because of the sequential feedback between plants and arthropods (Utsumi et al., 2010).
Compositional divergence and impact on species richness
Initial plant conditions also had sustained effects on species richness (Figure 3). Inter-individual variation in species composition was higher on plants from chamber C2 (exposed and damaged plants) than from chamber C1 (undamaged plants) until day 60 (Figure 2B). This resulted in greater observed and standardized species richness on day 60 (Figure 3A) and greater asymptotic richness (Figure 3B) on plants from chamber C2 than from chamber C1. In summary, the community-wide high gamma species richness (=48: Supplementary Table S3) was maintained by beta richness with plant initial conditions (chambers or treatments) and time (=32) as well as alpha richness (=2.16) and beta richness between 16 replicates within each treatment (=13.84) (Figure 3C; Supplementary Table S2). Our findings using small-sized plant individuals can be extrapolated to arthropod community assemblages on much larger willow trees in a natural environment. We hypothesize that the spatial heterogeneity of the earliest-arriving arthropods generates the heterogeneous distribution of plant traits within a plant population via direct herbivory and exposure to HIPVs, leading to arthropod community divergence (= high β richness) and thus contributes to higher gamma species richness for the whole willow populations. The effects of plant–plant signaling (by exposure to HIPVs) on emigration of arthropods, which was demonstrated in a goldenrod system (Morrell and Kessler, 2017), as well as immigration from the species pool, would be responsible for such divergence.
Priority effect caused by plant induced responses
The differentiation on day 32 in community composition between exposed and damaged plants strongly implies the presence of a priority effect. The willow plant preconditioning experimentally prepared in this study can be regarded as a simulation of the environments arising from the initial arrival of herbivores. Damaged plants occur when the leaf beetle is the earliest arriving species, while exposed plants represent those plants that receive leaf-beetle-induced VOCs before actual herbivory. The undamaged plant corresponds to a plant that is not conditioned by arthropods at all. The order and timing of species arrival patterns have been shown to influence the community structure, species richness, and ecosystem function as a priority effect in community assembly theory (Chase, 2003; Fukami, 2015). One of the mechanisms of the priority effect is that the earliest-arriving species modifies the environment (or niche) in a species-specific way, which in turn affects the subsequent arrival of other species, leading to the divergence of community composition or alternative stable states (Fukami, 2015). Willow ecophysiological traits (e.g., levels of defense products, the emission of VOCs, and compensative regrowth) act as biotic environments for arthropods on plants, and they are easily modified by herbivory (Utsumi and Ohgushi, 2009; Yoneya and Takabayashi, 2013), although these plant traits were not measured in this study. Therefore, plant-arthropod feedback (Utsumi et al., 2010) might act as the mechanism to cause the priority effect. As one of the implications for such feedback, at the individual plant level on day 32, pairwise dissimilarity in community composition was greater with increasing difference in the leaf beetle abundance between plants (Mantel test, p = 0.0226 for the whole data (C1 + C2) and p = 0.0166 for the data from chamber C2 only, Supplementary Figure S6). This result may imply that leaf beetles, and the whole arthropod community, responded in a similar way to inter-individual variations in plant traits or that leaf beetle is a keystone species that determines community composition through strong impacts on plant traits (e.g., Utsumi, 2015). For a better mechanistic understanding of the roles of priority effects in arthropod community assembly, diverse responses of plant traits (e.g., composition of secondary metabolites and allocation between growth and defense) against herbivory (Utsumi and Ohgushi, 2009) and VOCs (Yoneya et al., 2010) and their temporal dynamics should be investigated (Haukioja, 2005; Frost et al., 2008).
Data availability statement
The original contributions presented in the study are included in the article/Supplementary material, further inquiries can be directed to the corresponding author.
Author contributions
KY and JT: designed the experiments. KY: conducted the experiments. KY and TM: analyzed the data. All of three wrote the manuscript. All authors contributed to the article and approved the submitted version.
Funding
This study was supported by scientific research grants (A: 22H00425) to JT and KY, and (C: 20K06818) to KY from the Ministry of Education, Culture, Sports, Science and Technology of Japan (MEXT).
Conflict of interest
The authors declare that the research was conducted in the absence of any commercial or financial relationships that could be construed as a potential conflict of interest.
Publisher’s note
All claims expressed in this article are solely those of the authors and do not necessarily represent those of their affiliated organizations, or those of the publisher, the editors and the reviewers. Any product that may be evaluated in this article, or claim that may be made by its manufacturer, is not guaranteed or endorsed by the publisher.
Supplementary material
The Supplementary material for this article can be found online at: https://www.frontiersin.org/articles/10.3389/fevo.2022.1031664/full#supplementary-material
References
Anderson, M. J. (2006). Distance-based tests for homogeneity of multivariate dispersions. Biometrics 62, 245–253. doi: 10.1111/j.1541-0420.2005.00440.x
Anderson, M. J., Crist, T. O., Chase, J. M., Vellend, M., Inouye, B. D., Freestone, A. L., et al. (2011). Navigating the multiple meanings of β diversity: a roadmap for the practicing ecologist. Ecol. Lett. 14, 19–28. doi: 10.1111/j.1461-0248.2010.01552.x
Ando, Y., Utsumi, S., and Ohgushi, T. (2017). Aphid as a network creator for the plant-associated arthropod community and its consequence for plant reproductive success. Funct. Ecol. 31, 632–641. doi: 10.1111/1365-2435.12780
Arimura, G., Matsui, K., and Takabayashi, J. (2009). Chemical and molecular ecology of herbivore-induced plant volatiles: proximate factors and their ultimate functions. Plant Cell Physiol. 50, 911–923. doi: 10.1093/pcp/pcp030
Arimura, G., Ozawa, R., Shimoda, T., Nishioka, T., and Takabayashi, J. (2000). Herbivory-induced volatiles elicit defence genes in lima bean leaves. Nature 406, 512–515. doi: 10.1038/35020072
Barber, N. A., and Marquis, R. J. (2011). Leaf quality, predator, and stochastic processes in the assembly of a diverse herbivore community. Ecology 92, 699–708. doi: 10.1890/10-0125.1
Bertin, N., and Staudt, M. (1996). Effect of water stress on monoterpene emissions from young potted holm oak (Quercus ilex L.) trees. Oecologia 107, 456–462. doi: 10.1007/BF00333935
Chao, A., Chazdon, R. L., Colwell, R. K., and Shen, T. T. (2006). Abundance-based similarity indices and their estimation when there are unseen species in samples. Biometrics 62, 361–371. doi: 10.1111/j.1541-0420.2005.00489.x
Chao, A., and Jost, L. (2012). Coverage-based rarefaction and extrapolation: standardizing samples by completeness rather than size. Ecology 93, 553–2547. doi: 10.1890/11-1952.1
Chase, J. M. (2003). Community assembly: when should history matter? Oecologia 136, 489–498. doi: 10.1007/s00442-003-1311-7
Choh, Y., and Takabayashi, J. (2006). Intact lima bean plants exposed to herbivore-induced plant volatiles attract predatory mites and spider mites at different levels according to plant parts. Appl. Entomol. Zool. 41, 537–543. doi: 10.1303/aez.2006.537
Clausen, P., Bryant, P., and Werner, A. (1989). Chemical model for short-term induction in quaking aspen (Populus tremuloides) foliage against herbivores. J. Chem. Ecol. 15, 2335–2346. doi: 10.1007/BF01012085
Colwell, R. K., Mao, C. X., and Chang, J. (2004). Interpolating, extrapolating, and comparing incidence-based species accumulation curves. Ecology 85, 2717–2727. doi: 10.1890/03-0557
Crist, T. O., Veech, J. A., Gering, J. C., and Summerville, K. S. (2003). Partitioning species diversity across landscapes and regions: a hierarchical analysis of α, β, and γ diversity. Am. Nat. 162:743. doi: 10.1086/378901
Dakos, V., Beninca, E., van Nes, E. H., Philippart, C. J. M., Scheffer, M., and Huisman, J. (2009). Interannual variability in species composition explained as seasonally entrained chaos. Proc. R. Soc. B 276, 2871–2880. doi: 10.1098/rspb.2009.0584
Fatouros, N. E., Lucas-Barbosa, D., Weldegergis, B. T., Pashalidou, F. G., van Loon, J. J. A., Dicke, M., et al. (2012). Plant volatiles induced by herbivore egg deposition affect insects of different trophic levels. PLoS One 7:e43607. doi: 10.1371/journal.pone.0043607
Frost, C. J., Appel, H. M., Carlson, J. E., De Moraes, C. M., Mescher, M. C., and Schultz, J. C. (2007). Within-plant signalling via volatiles overcomes vascular constraints on systemic signalling and primes responses against herbivores. Ecol. Lett. 10, 490–498. doi: 10.1111/j.1461-0248.2007.01043.x
Frost, C. J., Mescher, M. C., Carlson, J. E., and De Moraes, C. M. (2008). Plant defense priming against herbivores: getting ready for a different battle. Plant Physiol. 146, 818–824. doi: 10.1104/pp.107.113027
Fukami, T. (2015). Historical contingency in community assembly: integrating niches, species pools, and priority effects. Annu. Rev. Ecol. Evol. Syst. 46, 1–23. doi: 10.1146/annurev-ecolsys-110411-160340
Fukami, T., Bezemer, T. M., Mortimer, S. R., and van der Putten, W. H. (2005). Species divergence and trait convergence in experimental plant community assembly. Ecol. Lett. 8, 1283–1290. doi: 10.1111/j.1461-0248.2005.00829.x
Haukioja, E. (2005). Plant defenses and population fluctuations of forest defoliators: mechanism-based scenarios. Ann. Zool. Fenn. 42, 313–325.
Heil, M. (2004). Induction of two indirect defences benefits Lima bean (Phaseolus lunatus, Fabaceae) in nature. J. Ecol. 92, 527–536. doi: 10.1111/j.0022-0477.2004.00890.x
Karban, R., Yang, J. H., and Edwards, J. H. (2014). Volatile communication between plants that affects herbivory: a meta-analysis. Ecol. Lett. 17, 44–52. doi: 10.1111/ele.12205
Lewinsohn, T. M., Novotny, V., and Basset, Y. (2005). Insects on plants: diversity of herbivore assemblages revisited. Annu. Rev. Ecol. Evol. Syst. 36, 597–620. doi: 10.1146/annurev.ecolsys.36.091704.175520
Loreto, F., Barta, C., Brilli, F., and Nogues, I. (2006). On the induction of volatile organic compound emissions by plants as consequence of wounding or fluctuations of light and temperature. Plant. Cell. Environ. 29, 1820–1828. doi: 10.1111/j.1365-3040.2006.01561.x
Loreto, F., Förster, A., Dürr, M., Csiky, O., and Seufert, G. (1998). On the monoterpene emission under heat stress and on the increased thermotolerance of leaves of Quercus ilex L. fumigated with selected monoterpenes. Plant. Cell. Env. 21, 101–107. doi: 10.1046/j.1365-3040.1998.00268.x
Morrell, K., and Kessler, A. (2017). Plant communication in a widespread goldenrod: keeping herbivores on the move. Funct. Ecol. 31, 1049–1061. doi: 10.1111/1365-2435.12793
Nakamura, M., Kagata, H., and Ohgushi, T. (2006). Trunk cutting initiates bottom-up cascades in a tri-trophic system: sprouting increases biodiversity of herbivorous and predaceous arthropods on willows. Oikos 113, 259–268. doi: 10.1111/j.2006.0030-1299.14251.x
Nakamura, M., Utsumi, S., Miki, T., and Ohgushi, T. (2005). Flood initiates bottom-up cascades in a tri-trophic system: host plant regrowth increases densities of a leaf beetle and its predators. J. Anim. Ecol. 74, 683–691. doi: 10.1111/j.1365-2656.2005.00960.x
Niinemets, Ü. (2010). Mild versus severe stress and BVOCs: thresholds, priming and consequences. Trends Plant Sci. 15, 145–153. doi: 10.1016/j.tplants.2009.11.008
Ohgushi, T. (2005). Indirect interaction webs: herbivore-induced effects through trait change in plants. Annu. Rev. Ecol. Evol. Syst. 36, 81–105. doi: 10.1146/annurev.ecolsys.36.091704.175523
Oksanen, L. (2001). Logic of experiment in ecology: is pseudoreplication a pseudoissue? Oikos 94, 27–38. doi: 10.1034/j.1600-0706.2001.11311.x
Oksanen, J., Blanchet, F. G., Kindt, R., Legendre, P., Minchin, P., O’Hara, R. B., et al. (2018). Vegan: community ecology package. R package version 2, 5–2. https://CRAN.R-project.org/package=vegan
Pechal, J. L., Benbow, M. E., Crippen, T. L., Tarone, A. M., and Tomberlin, J. K. (2014). Delayed insect access alters carrion decomposition and nectophagous insect community assembly. Ecosphere 5:art45. doi: 10.1890/ES14-00022.1
Pegoraro, E., Rey, A., Greenberg, J., Harley, P., Grace, J., Malhi, Y., et al. (2004). Effect of drought on isoprene emission rates from leaves of Quercus virginiana Mill. Atmos. Environ. 38, 6149–6156. doi: 10.1016/j.atmosenv.2004.07.028
Piesik, D., Panka, D., Jeske, M., Wenda-Piesik, A., Delaney, K. J., and Weaver, D. K. (2012). Volatile induction of infected and neighbouring uninfected plants potentially influence attraction/repellence of a cereal herbivore. J. Appl. Entomol. 137, 296–309. doi: 10.1111/j.1439-0418.2012.01742.x
Porter, A. S., Evans-Fitz, G. C., McElwain, J. C., Yiotis, C., and Elliott-Kingston, C. (2015). How well do you know your growth chambers? Testing for chamber effect using plant traits. Plant Methods 11:44. doi: 10.1186/s13007-015-0088-0
R Core Team. (2018). R: A Language and Environment for Statistical computing. Vienna, Austria: R Foundation for Statistical Computing.
Raupp, M. J., and Sadof, C. S. (1989). Behavioral responses of a leaf beetle to injury-related changes in its salicaceous host. Oecologia 80, 154–157. doi: 10.1007/BF00380144
Shiojiri, K., Karban, R., and Ishizaki, S. (2009). Volatile communication among sagebrush branches affects herbivory: timing of active cues. Arthropod Plant Interact. 3, 99–104. doi: 10.1007/s11829-009-9060-0
Simon, M., and Hilker, M. (2003). Herbivores and pathogens on willow: do they affect each other? Agric. Forest. Ent. 5, 275–284. doi: 10.1046/j.1461-9563.2003.00189.x
Srinivas, P., Danielson, S. D., Smith, C. M., and Foster, J. E. (2001). Cross-resistance and resistance longevity as induced by bean leaf beetle, Cerotoma trifurcata and soybean Looper, Pseudoplusia includens herbivory on soybean. J. Insect Sci. 1:5. doi: 10.1673/031.001.0501
Turlings, T. C. J., and Erb, M. (2018). Tritrophic interactions mediated by herbivore-induced plant volatiles: mechanisms, ecological relevance, and application potential. Annu. Rev. Entomol. 63, 433–452. doi: 10.1146/annurev-ento-020117-043507
Underwood, N. (1999). The influence of plant and herbivore characteristics on the interaction between induced resistance and herbivore population dynamics. Am. Nat. 153, 282–294. doi: 10.1086/303174
Utsumi, S. (2015). Feeding evolution of a herbivore influences an arthropod community through plants: implications for plant-mediated eco-evolutionary feedback loop. J. Ecol. 103, 829–839. doi: 10.1111/1365-2745.12419
Utsumi, S., Ando, Y., and Miki, T. (2010). Linkages among trait-mediated indirect effects: a new framework for the indirect interaction web. Popul. Ecol. 52, 485–497. doi: 10.1007/s10144-010-0237-2
Utsumi, S., Nakamura, M., and Ohgushi, T. (2009). Community consequences of herbivore-induced bottom-up trophic cascades: the importance of resource heterogeneity: induced resource heterogeneity for community. J. Anim. Ecol. 78, 953–963. doi: 10.1111/j.1365-2656.2009.01570.x
Utsumi, S., and Ohgushi, T. (2009). Community-wide impacts of herbivore-induced plant regrowth on arthropods in a multi-willow species system. Oikos 118, 1805–1815. doi: 10.1111/j.1600-0706.2009.17580.x
Xiao, Y., Wang, Q., Erb, M., Turlings, T. C. J., Ge, L., et al. (2012). Specific herbivore-induced volatiles defend plants and determine insect community composition in the field. Ecol. Lett. 15, 1130–1139. doi: 10.1111/j.1461-0248.2012.01835.x
Yoneya, K., Inui, Y., Ishihara, M., and Takabayashi, J. (2014a). Herbivore-constructed leaf shelters on Salix eriocarpa shoots affect arthropod communities. J. Plant Interact. 9, 364–369. doi: 10.1080/17429145.2013.837973
Yoneya, K., Kugimiya, S., and Takabayashi, J. (2009a). Can herbivore-induced plant volatiles inform predatory insects about the most suitable stage of its prey? Physiol. Entomol. 34, 379–386. doi: 10.1111/j.1365-3032.2009.00701.x
Yoneya, K., Kugimiya, S., and Takabayashi, J. (2009b). Do adult leaf beetles (Plagiodera versicolora) discriminate between odors from uninfested and leaf-beetle-infested willow shoots? J. Plant Interact. 4, 125–129. doi: 10.1080/17429140802710658
Yoneya, K., Kugimiya, S., and Takabayashi, J. (2014b). Leaf beetle larvae, Plagiodera versicolora (Coleoptera: Chrysomelidae), show decreased performance on uninfested host plants exposed to airborne factors from plants infested by conspecific larvae. Appl. Entomol. Zool. 49, 249–253. doi: 10.1007/s13355-013-0243-x
Yoneya, K., and Miki, T. (2015). Coevolution of foraging behavior in herbivores and their natural enemies predicts multifunctionality of herbivore-induced plant volatiles. Funct. Ecol. 29, 451–461. doi: 10.1111/1365-2435.12398
Yoneya, K., Ozawa, R., and Takabayashi, J. (2010). Specialist leaf beetle larvae use volatiles from willow leaves infested by conspecifics for reaggregation in a tree. J. Chem. Ecol. 36, 671–679. doi: 10.1007/s10886-010-9808-0
Keywords: herbivory, VOCs, community assembly, plant-arthropod feedback, Salix eriocarpa, Plagiodera versicolora, β diversity
Citation: Yoneya K, Miki T and Takabayashi J (2023) Initial herbivory and exposure to herbivory-induced volatiles enhance arthropod species richness by diversifying community assemblages. Front. Ecol. Evol. 10:1031664. doi: 10.3389/fevo.2022.1031664
Edited by:
Maria L. Pappas, Democritus University of Thrace, GreeceReviewed by:
Luca Pietro Casacci, University of Turin, ItalyNina Xiaoning Zhang, Swedish University of Agricultural Sciences, Sweden
Copyright © 2023 Yoneya, Miki and Takabayashi. This is an open-access article distributed under the terms of the Creative Commons Attribution License (CC BY). The use, distribution or reproduction in other forums is permitted, provided the original author(s) and the copyright owner(s) are credited and that the original publication in this journal is cited, in accordance with accepted academic practice. No use, distribution or reproduction is permitted which does not comply with these terms.
*Correspondence: Kinuyo Yoneya, ✉ yoneya@nara.kindai.ac.jp