Think Fast!: Vervet Monkeys Assess the Risk of Being Displaced by a Dominant Competitor When Making Foraging Decisions
- 1Department of Anthropology, University of Toronto Scarborough, Toronto, ON, Canada
- 2Department of Anthropology, University of Toronto, Toronto, ON, Canada
- 3Department of Biology, Concordia University, Montreal, QC, Canada
Foraging animals need to quickly assess the costs and benefits of different foraging decisions, including resource quantity, quality, preference, ease of access, dispersion, distance, and predation risk. Social animals also need to take social context into account and adapt foraging strategies that maximize net resource intake and minimize contest competition with conspecifics. We used an experimental approach to investigate how social context impacts wild vervet monkey (Chlorocebus pygerythrus) foraging decisions in a multi-destination pentagon array. We baited four platforms with less-preferred corn and one platform with a larger, preferred resource (half banana) that required handling time. We ran over 1,000 trials and found that when monkeys foraged alone, they usually took the path that minimized travel distance but prioritized the preferred-food platform when in competition. However, the foraging strategy chosen by low-ranking individuals depended on the handling skill of the decision maker (i.e., time it would take them to retrieve the banana), the relative rank of their audience members (i.e., who has priority-of-access to resources), and the distance audience members were from the experiment site (i.e., their travel time). When the risk of being displaced by a dominant competitor was low (because they were far away and/or because the decision-maker was skilled in retrieving the banana), low-ranking individuals chose a route that minimized travel costs. Conversely, when the risk of losing food to a dominant competitor was high, decision-makers rushed for the preferred-food platform at the onset of the trial. When the risk of displacement was moderate because a dominant audience member was at least 50 m away, low-ranking individuals partly prioritized the preferred-food platform but took the time to stop for one platform of corn on the way. This strategy increased the total amount of food obtained during the trial. These findings suggest that lower-ranking individuals, who experienced high contest competition at the foraging experiment, calculated the risk of being displaced by a dominant competitor when making foraging decisions. This experiment demonstrates that vervets go through a complex decision-making process that simultaneously considers the profitability of different foraging decisions and their social context.
Introduction
Since optimal foraging theory was first proposed more than 50 years ago (MacArthur and Pianka, 1966), behavioral ecologists have dedicated immense effort in understanding how animals optimize resource intake. Foraging individuals may consider resource quantity, quality, preference, ease of access, dispersion, distance, visibility, predation risk, and the level of competition (Croy and Hughes, 1991; Menzel, 1997; Giraldeau and Caraco, 2000; Stephens et al., 2007; Menzel et al., 2008; Fortin and Fortin, 2009; Marshall et al., 2012; Sayers and Menzel, 2012; Teichroeb and Aguado, 2016; Kumpan et al., 2019). The profitability of a given food item is typically framed as energy gained divided by pursuit plus handling time (Pyke, 1984; Stephens and Krebs, 1986). Thus, profitability increases considerably as pursuit and handling time approach zero, leading to strong selection pressure to decrease the costs of these two factors, if possible (e.g., Anholt et al., 1987; Stillman et al., 2000; Catania and Remple, 2005; Cooper and Anderson, 2006; Paredes et al., 2015; Wilson et al., 2015). Pursuit often equates to the distance needed to travel to get to a food site (Janson, 2000, 2007). Handling time is typically manual processing of food items to remove the edible portion or processing the food in the mouth before swallowing (Isbell et al., 1998; Cadieu et al., 2008; Gunst et al., 2010; Sayers and Menzel, 2012); both of which reduce the intake of new food items. The energy spent in pursuit selects for cognitive abilities to remember the location of food patches and their characteristics, determining a route that will minimize travel costs and remembering how visual distance to rewards relates to travel time (Shettleworth, 2010; Janmaat et al., 2014). While handling time may be a key selective pressure for social learning as food extraction and manipulation skills are often transmitted socially (Whiten and Mesoudi, 2008; Reader and Biro, 2010; Thornton and Clutton-Brock, 2011).
In gregarious animals, social context can also impact decision making because group members are potentially competitors for important resources like food or mates (Bugnyar and Heinrich, 2005; Dally et al., 2006; Rosati and Hare, 2012; Teichroeb and Aguado, 2016). In primates, within-group contest competition for food items can be intense, imposing costs on animals such as being displaced at feeding sites and potentially leading to the risk of injury or even death (Wrangham, 1981; Janson, 1985; Vogel, 2005; Kumpan et al., 2019). Contest (or interference) competition arises when limiting resources are clumped and defensible and direct conflict occurs over which individuals control them (Nicholson, 1954; Janson and van Schaik, 1988), which often leads to the formation of dominance hierarchies (Shively, 1985). While dominance hierarchies decrease the need to engage in contest competition, contest competition still occurs. Consequently, a comprehensive understanding of foraging behavior in gregarious animals can only be built if we investigate how decision-makers modify their behavior in the face of competition.
Research in a number of species shows that foragers modify their behavior when in competition. For example, studies conducted on various herbivores have found that foraging individuals increase their intake rates as the number of potential competitors present increases (Molvar and Bowyer, 1994; Fritz and de Garine-Wichatitsky, 1996; Shrader et al., 2006). Similarly, vervet monkeys (Chlorocebus pygerythrus) choose paths that minimize travel distance when foraging alone, but prioritize high-reward sites when competitors are present (Teichroeb and Aguado, 2016). Furthermore, other studies suggest that foraging decisions are not only based on whether an individual is currently competing with a conspecific, but that decision makers also assess the likelihood of losing food to a competitor. For example, when deciding whether to try and access a food patch, subordinate pigs (Sus scrofa) use the location and movement trajectory of a dominant competitor to determine whether they will be able to arrive at the patch ahead of the competitor (Held et al., 2002). Ravens (Corvus corax) consider the visual perspective of conspecifics to differentiate between knowledgeable and ignorant competitors (Bugnyar and Heinrich, 2005), and both California scrub-jays (Aphelocoma californica) and Eurasian jays (Garrulus glandarius) selectively cached food that audience members were least motivated to steal (Ostojić et al., 2017).
The goal of this study was to examine how social context impacted route choice in a social primate, the vervet monkey. We have been using carefully designed foraging experiments with high trial sample sizes to understand how this species solves multi-destination routes (Teichroeb, 2015; Teichroeb and Smeltzer, 2018) and makes multifactor foraging decisions (Teichroeb and Aguado, 2016; Kumpan et al., 2019). Because vervets will leave their foraging group to visit the experiment site alone, but will also participate when surrounded by group members, this experimental paradigm is also ideal for examining how social context impacts foraging decisions. Since our previous work has shown that foraging vervet monkeys modify their route choice when a competitor is present (Teichroeb and Aguado, 2016), this study focused on investigating how the risk of contest competition impacted foraging decisions.
To facilitate comparisons with our previous work (Teichroeb and Aguado, 2016; Kumpan et al., 2019), we used the same pentagon-shaped foraging array with platforms (i.e., food patches) five meters apart (Lihoreau et al., 2011). In this array, the most efficient path is to start with the nearest platform and move around the outside of the array as this route minimizes travel distance (Figure 1; ESM 1). We baited four platforms in the pentagon with less-preferred corn kernels and one platform was randomly chosen in each trial to contain a larger, preferred reward with high handling time (i.e., a half-banana in an unopenable box with a small hole cut in the top). This box required the monkeys to manipulate it (e.g., tip, roll, shake) to retrieve the banana, and so mimicked the handling costs that this species often faces when feeding on natural food items (Isbell and Young, 1993). Importantly, food resources with high handling times elicit frequent contest competition (Sirot, 2000; Korstjens et al., 2002; Marshall et al., 2012; Wikberg et al., 2013), and have a high risk of kleptoparasitism (Steele and Hockey, 1995; Broom and Ruxton, 2003), because the time spent handling gives competitors time to try and obtain the food item. Therefore, by imposing high handling time on the preferred food, we increased the risk that an audience member would be able to travel to the experiment site and take priority-of-access to the preferred-food/high-handling time platform (hereafter “preferred-food platform”) or steal the food item from the handling individual. By imposing high handling time on the preferred-food reward, we increased the risk that lower-ranked and/or unskilled individuals would lose this preferred-food reward to an audience member. To investigate whether the risk of contest competition impacts the decision-making process, we considered both the forager’s speed in retrieving the banana from the box (i.e., their handling skill, where as individuals got more experienced and skilled, handling time decreased) and the composition and location of their audience. Not all audience members are equally likely/able to displace a group member at a food resource. Subordinate audience members cannot take priority-of-access to contested resources, and audience members who are too far away will have long travel times to reach the experiment site.
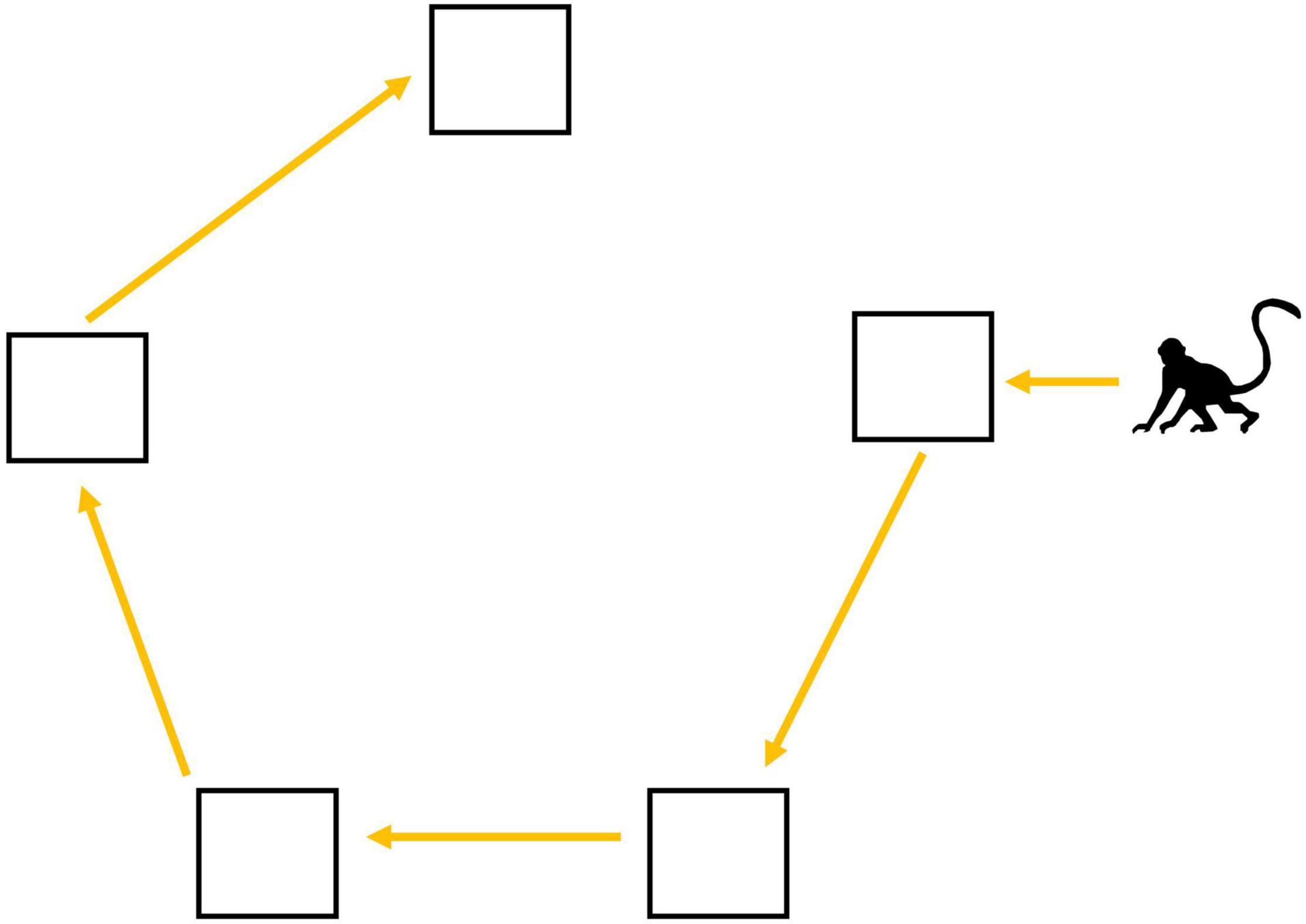
Figure 1. Travel path that minimizes travel distance if visiting all food patches in a pentagon-shaped foraging array.
We always endeavored to place the preferred-food reward so that it was not on the decision-makers nearest platform. This was accomplished by placing the preferred-food reward on a platform that was not the nearest to any individual present at the experiment site, or when this was not possible because there were many monkeys present, to avoid placing it near high-ranking individuals or individuals that had recently been participating in the experiment. By placing the preferred-food reward more than one platform away from likely participants, we ensured that decision-makers had to choose between starting with their nearest platform or eating their preferred food first. In our analyses, we examine the factors that impacted the first two decisions the focal monkey made in each trial (Figure 2); which platform to visit first (i.e., Decision 1) and which platform to visit second (i.e., Decision 2). This approach allowed us to investigate the extent to which monkeys prioritized their preferred-food platform, vs. chose the nearest one or two platforms of corn. With this approach we were able to identify three distinct strategies used by the vervets (Figure 2). The first strategy was to rush for the banana at the onset of the trial (Figures 2A,G; ESM 2, 3), “prioritizing the preferred-food platform.” The second was to stop for “one platform of corn en route to their preferred food” (Figures 2D,I; ESM 4). This intermediate strategy still prioritized the banana but ensured the focal monkey was able to obtain at least one platform of corn before competitor(s) arrived (and ate corn while they themselves handled the box). The third strategy was to take the route that would “minimize travel distance” if visiting all five platforms (Figure 1; ESM 1), by starting at the nearest platform and then continuing to next corn platform encountered when moving around the outside of the array (Figure 2J; ESM 5). Individuals selecting their two nearest platforms of corn could be doing so because (1) they were unwilling to try and obtain the preferred food-reward, or (2) they planned to visit the preferred-food platform when it was encountered along this shortest-distance path (Figure 1). In either case, starting with the two nearest platforms of corn would minimize the distance travelled compared to selecting corn platforms further away.
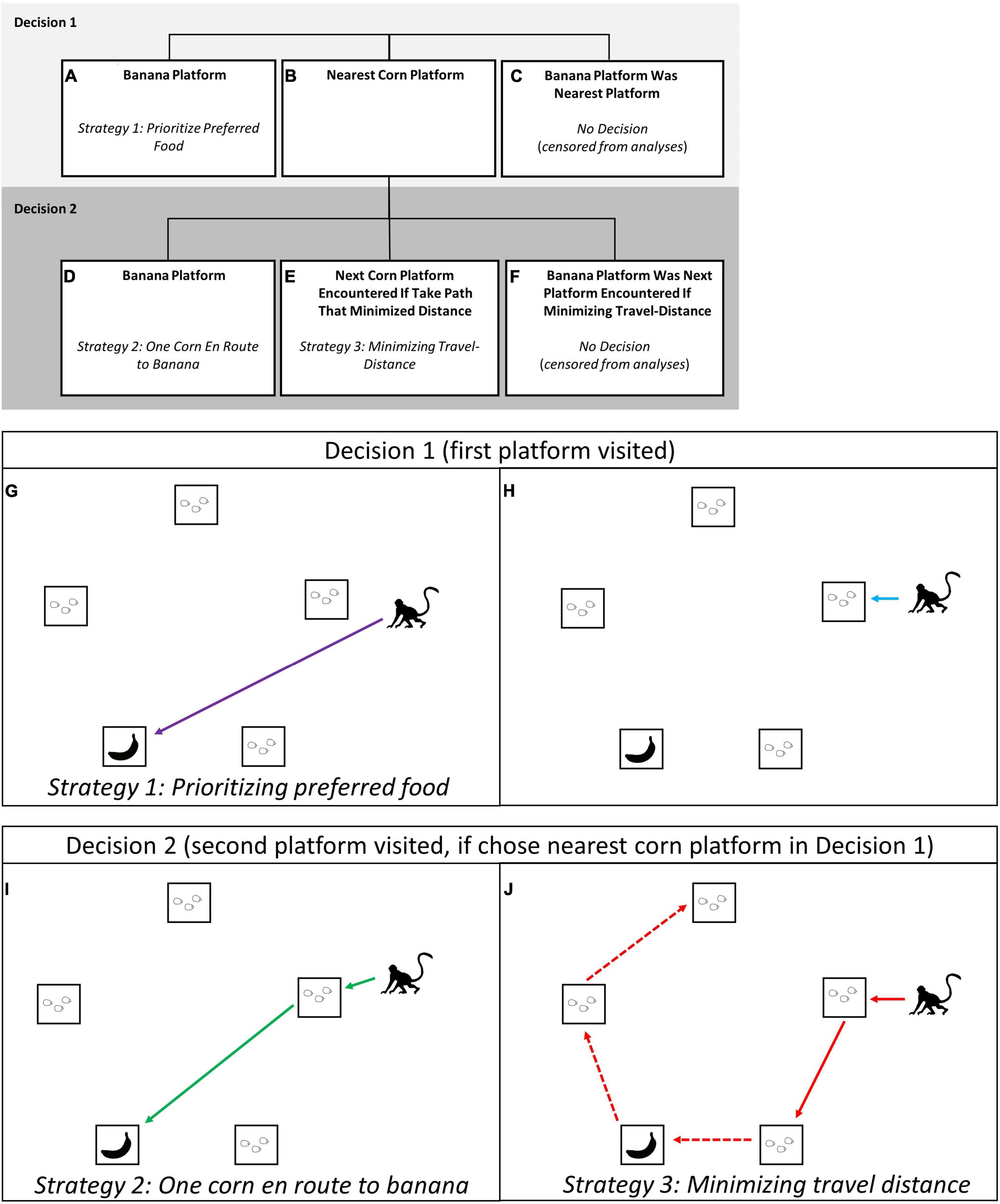
Figure 2. Within this multi-destination foraging experiment, vervet monkeys at Nabugabo, Uganda needed to make two decisions: which platform to visit first [Decision 1: (A,B,G,H), and which to visit second, Decision 2: (D,E,I,J)]. Decision 1 was a choice between rushing for the platform with the banana (A,G), a strategy which prioritized the preferred-food platform, or (B,H) to start at the nearest corn platform. We attempted to bait platforms such that the preferred food (banana) was not the nearest platform; trials in which the banana was on the nearest platform were censored from the analysis because these trials did not require the monkey to choose between minimizing travel distance and prioritizing preferred food (C). Individuals who chose to visit their nearest corn platform first could then decide if they wanted to (D,I) proceed immediately afterward to the preferred-food platform, or (E,J) travel around the pentagon array in a trajectory that would minimize travel distance, getting the preferred-food when they came to it. Trials in which the platforms were baited such that the preferred-food platform was the second platform encountered when taking the path that minimized travel distance (F) were censored from the analyses because monkeys in these trials did not have to choose between the platform with their preferred food and minimizing travel distance. Note: in box (J) we use the dashed arrow to show the route that a monkey foraging efficiently was expected to take, however, they may not have obtained the rewards on all five platforms if in competition.
Given our previous findings (Teichroeb and Aguado, 2016), we expected that solitarily foraging vervets, who are able to obtain the food rewards on all five platforms, should forage efficiently by minimizing travel distance as they move through the array (Figures 1, 2E,J). Alternative routes would involve cutting across the array and then back-tracking, increasing travel costs. Conversely, monkeys in competition were predicted to assess the risk of contest competition, and prioritize the preferred-food platform when the risk of being displaced by a dominant competitor was high. Although females can be dominant to males in this species (Young et al., 2017; Hemelrijk et al., 2020; Li et al., 2021), all adult males were dominant to all other group members during the time of this study. Therefore, we expected that adult males would be less vulnerable to contest competition than the other age-sex classes that participated in the experiment, and so would show a greater propensity to minimize travel distance rather than prioritizing the preferred-food platform. Hence, we expected them to visit their two nearest corn platforms first, and obtain the preferred food-reward as it was encountered. Conversely, we expected that adult females, subadult males, and subadult females would be more likely to bypass less preferred corn platforms and rush to retrieve the banana from the box. Furthermore, we expected these lower-ranked individuals to be more likely to prioritize the preferred-food platform when their audience contained group members that out-ranked them, particularly when dominant individuals were relatively close to the experiment (i.e., had short travel times and could approach the array and displace subordinates). Lastly, we expected that handling skill would modify route choice, with proficient monkeys showing a lower propensity to prioritize the preferred-food platform. Speed in retrieving the banana from the box should give decision makers extra time to consume corn before visiting the preferred-food platform, maximizing the total food rewards obtained.
Materials and Methods
Study Site and Animals
This research was carried out at Lake Nabugabo, Masaka District, central Uganda (0°22′-12°S, 31°54′E). Lake Nabugabo is a small lake (8.2 × 5 km2) located on the western edge of Lake Victoria at an elevation of 1,136 m. Our research station is on the western side of Lake Nabugabo, in an area of mixed primary and secondary forest fragments, wetland, farmer’s fields, and tourist camps (Chapman et al., 2016). The study subjects were a habituated group of vervet monkeys (Chlorocebus pygerythrus) referred to as K group, which had been followed continuously since 2016 and had previously participated in a foraging experiment (Kumpan et al., 2019). All individuals were identifiable by their natural markings. At the time of the study, the group contained 38–44 individuals (4–7 adult males, 10 adult females, 3 subadult males, 5 subadult females, 16–19 juveniles and infants).
Experimental Design
This experiment was conducted from January to April 2019. We arranged five wooden platforms (wooden tables, 0.75 m high, with a square flat top 0.75 m × 0.75 m) in a pentagon array with a distance of 5 m between each platform (Figure 1; as in: Teichroeb and Aguado, 2016; Kumpan et al., 2019). The array was placed in a relatively open area among frequently visited feeding patches for K group, so that we could also record accurate data on the distance of approaching competitors and which individuals were in the audience along with their approximate distance. Similarly, by conducting the experiment in the open, any monkeys present within approximately 100 m of the experiment site could also see the array, the location of the different food rewards, and potential competitors.
We first ran two-choice experimental preference trials to verify that the vervets preferred the banana over the dried corn. All five individuals tested chose banana over corn in the quantities the platforms were baited with. After preferences were determined, we baited the platforms on each trial as follows: four platforms were baited with three kernels of the less-preferred dried corn (soaked in boiling water to soften it) and one platform was baited with the preferred banana in an unopenable, plastic, rectangular box with a small hole cut in the top on one side (Figure 3A). Because the sides of the box were transparent, the half banana was visible inside of the box. Handling times varied from 1 s to 69 s, with the average observed handling time being 6.7 s. The platform where the box was located was randomized each trial, but we avoided placing it on the platform nearest to any of the monkeys that were present at the experiment site. Thus, the monkeys usually had to choose between their nearest platform containing corn and the preferred-food platform at a greater distance. The first monkey to feed at a platform was deemed the “focal” and any subsequent individuals “competitors.” The behavior of the monkeys in the array was video recorded on each trial with narration by TJA-R and later these data were coded into Microsoft Excel by TJA-R, EV, and KA. During trials, we recorded which platform contained the preferred-food/high-handling time target (i.e., the banana in the box), the number of animals participating and their identities, the composition of the audience and the distance of these individuals (e.g., < 25 m, 26–50 m, 51–75 m, 76–100 m, > 100 m/out-of-sight), the order of platform visitation, which individual received the rewards on each platform, the duration of handling time (i.e., time spent manipulating the box to get the banana out, Figure 3B), and any social interactions that occurred. The proximity of audience members to the experiment site was recorded in distance categories because it was not feasible to collect more precise distance estimates for all group members within 100 m.
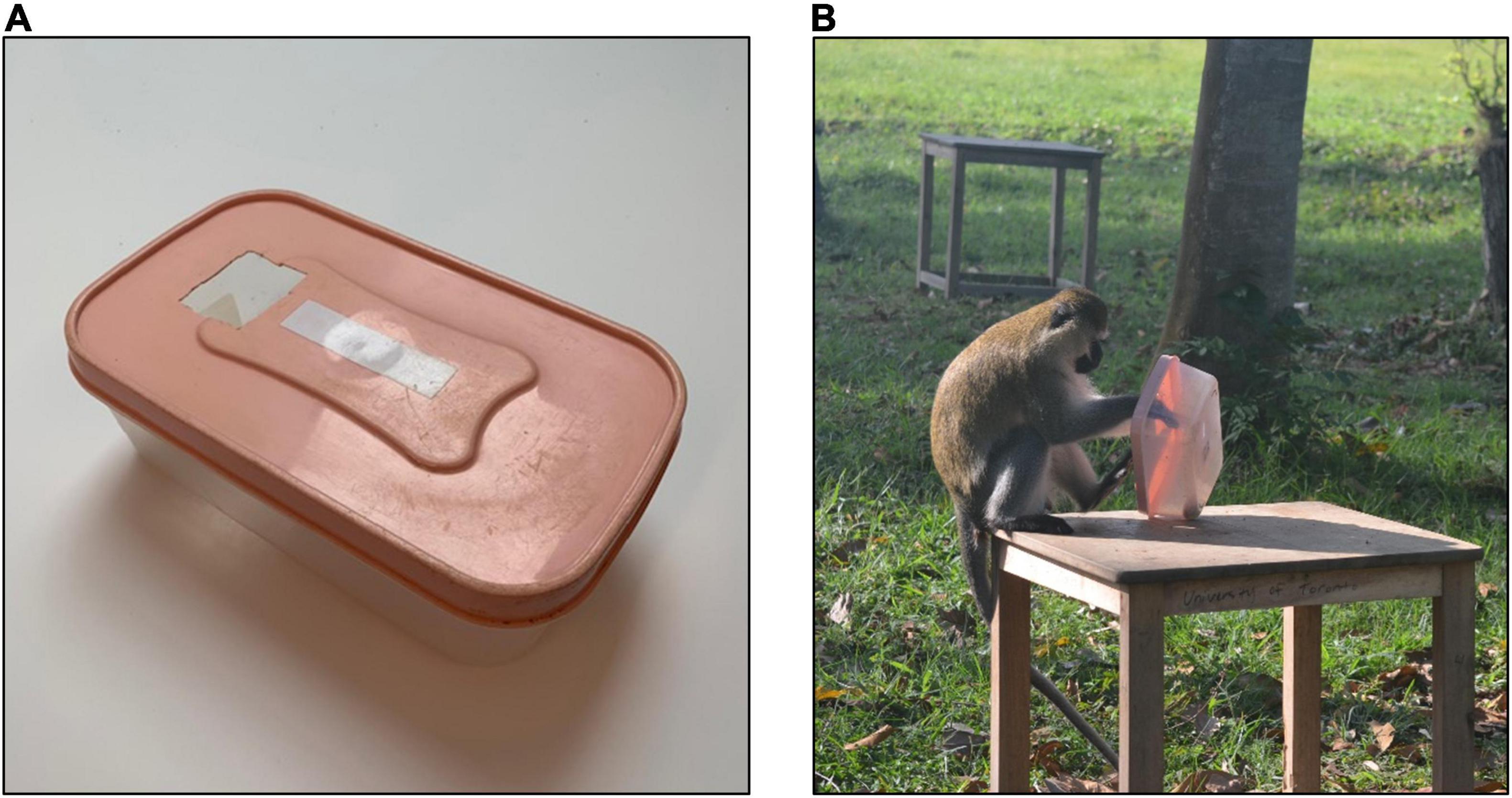
Figure 3. The preferred-food/high-handling time platform contained (A) a plastic box with a hole cut in the top, which could not be opened, with a half banana inside. (B) Individuals had to manipulate the box to get the banana out and this was recorded as their handling time [Photo credits: (A) JT, (B) TJA-R].
The social interactions that occurred around the foraging experiment, as well as ongoing behavioral data collection, allowed us to determine the dominance hierarchy for K group. There was a lot of flux in dominance relationships at the time of the experiment due to new male immigration and dominance challenges among some of the females. We therefore chose to use Elo-ratings (Elo, 1961, 1978; Albers and de Vries, 2001; Neumann et al., 2011) to quantify both the focal’s rank, and whether they had higher-ranking audience members. We used the known ordinal ranks at the onset of the experiment, determined using the long-term behavioral data collected at the study site, as the “startvalue” when estimating Elo-ratings. Decided dyadic contests that were observed throughout the study period were used to calculate the Elo-rating for each group member on each day. These Elo-ratings were used as the “focal’s rank” in our models, and we also used the daily Elo-ratings to determine if the focal was higher vs. lower-ranking than each group member present in their audience. We then coded the distance to the nearest higher-ranking audience member. Elo-ratings were calculated using the “EloRating” package (version 0.46.11, Neumann and Kulik, 2020) in R (version 3.6, R Core Team, 2019).
Data Analyses
We used generalized linear mixed models (GLMMs) to examine the frequency that the age-sex classes (adult males vs. adult females and subadults) selected different food rewards in Decision 1 and Decision 2. We used four GLMMs to assess the factors that impacted vervet decision-making in this foraging experiment: adult male choices in Decision 1, adult female/subadult choices in Decision 2, adult male choices in Decision 2, and adult female/subadult choices in Decision 2. Because all adult males were dominant to all other group members during the study period, and because adult males rarely approached the experimental site at the same time as other adult males, adult males were rarely focal individuals when there was a dominant competitor present. Thus, adult males experienced very little contest competition when participating in the foraging experiment and were unlikely to lose food to a dominant competitor. Consequently, we expected adult males to go through a different decision-making process, and so we modeled adult males separately from adult females, subadult males, and subadult females. Juveniles were not able to gain access to the foraging experiment and so were not included in this study.
In each GLMM we included individual ID as a random effect to control for repeated observations of individuals (pseudo replication) (Zuur et al., 2009). We did not include random slopes as the data did not support the more complex model structure (Bates et al., 2015a; Matuschek et al., 2017; Harrison et al., 2018). We censored any individual with less than 10 trials from our analyses. We also censored any trials in which the focal accessed a food reward before all the platforms were baited, because in such cases, the platform they choose to visit first may have been impacted by the presence/location/movement of the observer. The response variable in the Decision 1 models was whether the focal monkey chose the preferred-food platform (i.e., a strategy of strongly prioritizing the banana), vs. their nearest corn platform (Figure 2). In the Decision 2 models, the response variable was whether the focal visited the preferred-food platform second (i.e., a one corn en route to the banana strategy, prioritizing the banana to a lesser extent) vs. the next corn platform (i.e., a route that minimized travel distance if visiting all five platforms) (Figure 2). Because both of these response variables are dichotomous, we set a binomial error structure and logit link function. We censored any Decision 1 trials in which the preferred-food reward was placed on the focal’s nearest platform, as well as Decision 2 trials in which the preferred-food reward was on the next platform encountered if moving around the outside of the pentagon array (Figures 2C,F). We did so because in these trials, the focal did not have to choose between a route that would minimize travel distance (if visiting all five platforms) (Figure 1) and prioritizing the preferred-food reward (Figures 2G,I). We also censored trials in which the foraging strategy of the focal did not fit into the decision tree outlined in Figure 1. In total, we censored 18 trials (1.7% of the 1,046 Decision 1 trials) in which the focal did not choose either their nearest corn platform or the preferred-food platform when making Decision 1 (i.e., they visited a corn platform that was not their nearest), and 20 trials (3.9% of the 515 Decision 2 trials) in which the focal did not choose the next corn platform encountered or the preferred-food platform when making Decision 2 (i.e., they only visited one corn platform before being displaced by a dominant competitor or they fed at two corn platforms that were not the first and second platforms encountered).
Predictor variables included (1) the focal’s dominance rank, (2) their handling skill, (3) whether they were foraging in competition, and (4) the risk of losing food rewards to a dominant competitor. We quantified dominance rank using the daily Elo-rating of the focal individual. Because individuals tended to decrease their handling time as they gained more experience handling the box (Arseneau-Robar, unpublished data), we used the focal’s average handling time across their five most recent opportunities removing the banana from the box as an index of their current handling skill. We determined whether the focal had a competitor present at the onset of the trial if another group member (dominant or subordinate) was in close proximity to the platforms (<25 m) at the onset of the trial, and attempted to access the platforms by approaching a platform closely, jumping up onto it and/or feeding (i.e., whether the focal was foraging in competition). Lastly, we indexed the risk of losing food to a dominant competitor using the distance to the nearest dominant audience member. While all four of the predictor variables were included in adult female/subadult models, the variable “distance to the nearest dominant audience member” was not included in the adult-male models (i.e., the adult male models only included rank, recent handling time, and whether the focal was foraging in competition as predictors). This was done because, unlike the other age-sex classes, adult males rarely had a dominant audience member (Figure 4) because no group members out-ranked the alpha male and so one of the seven adult males included in this study could never have a dominant audience member. Additionally, subordinate males rarely had a dominant audience member because the low levels of male-male tolerance in vervet monkeys meant that low-ranking adult males typically waited for higher-ranking adult males to lose interest in the platforms and leave the area before they approached the experiment site. The lack of trials in which adult males had dominant audience members (Figure 4) created unbalanced models that failed to converge, and so we removed this predictor variable to improve model performance.
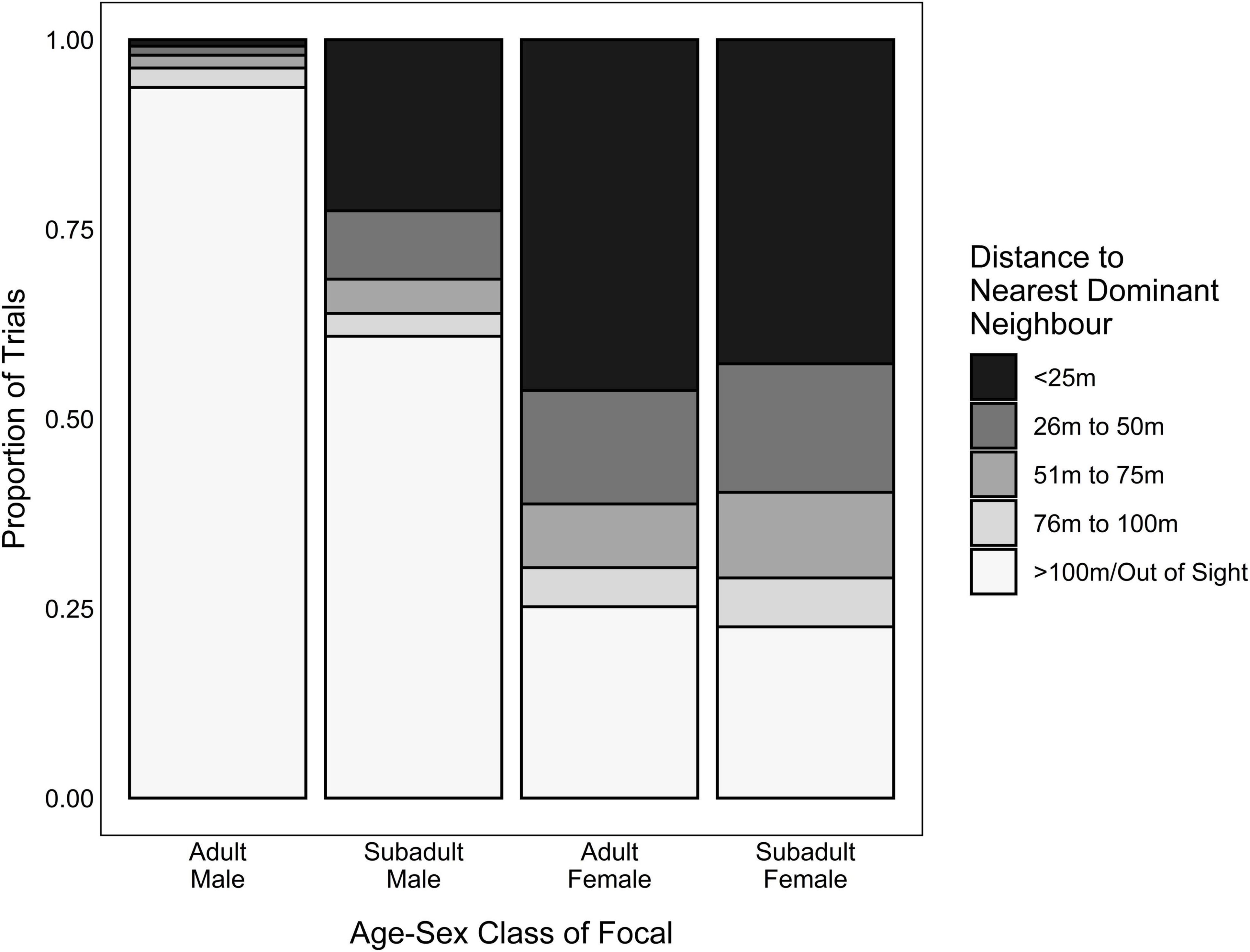
Figure 4. Proportion of trials where the distance to the nearest dominant neighbor was within 25 m, 26–50 m, 51–75 m, 76–100 m or > 100 m/out of sight, for each age-sex class that participated in the foraging experiment.
All analyses were conducted in R (version 3.6, R Core Team, 2019). We used the “car” package (version 3.0-7, Fox and Weisberg, 2019) to calculate variance inflation factors (VIF). These were all low (<3) and pairwise correlation coefficients were all well below 0.8, indicating there was no multicollinearity among predictors (Zuur et al., 2009; Field et al., 2012). We assessed model stability by using the “influence.ME” package (version 0.9-9, Nieuwenhuis et al., 2012) to calculate Cook’s distances and DFBeta values. Both Cook’s distance values and DFBeta values were concerningly high (Belsley et al., 2004; Field et al., 2012) for one male (i.e., level of the random effect) in the adult male Decision 2 model, and removing this influential case impacted the fixed effects that were significant in this model. Therefore, we have presented the model output from both the full and reduced datasets. We used the “lme4” package (version 1.1-21, Bates et al., 2015b) to build GLMMs, and the “DHARMa” package (version 0.3.3.0, Hartig, 2021) to check for over- or under-dispersion. For the distance to nearest dominant audience member variable, we set “>100 m/out-of-sight” as the reference category, and compared the other factor levels to it. We assessed the significance of predictor variables using 95% profile confidence intervals (lme4, version 1.1-21, Bates et al., 2015b), and the overall fit of each GLMM using a likelihood ratio test to compare the full model to the null model, which included the intercept and random effects. Lastly, we used the “MuMIn” package (version 1.43.17, Bartoń, 2020) to estimate the total variance explained [delta R2 GLMM(c)] by each model (Nakagawa and Schielzeth, 2013).
Results
In this experiment, three main foraging strategies were exhibited by the vervet monkeys: rushing for the platform with the banana at the onset of the trial (i.e., prioritizing their preferred food), the intermediate strategy of stopping for one platform of corn en route to the preferred-food platform, and taking the route that minimized travel distance if visiting all five platforms (Figure 2). These three strategies accounted for the choices that decision-makers made in the majority of trials (98% of trials in Decision 1 and 96% of trials in Decision 2). Of the 1,028 trials in which we were able to assess Decision 1, the monkeys prioritized the preferred-food platform in 22% of cases. When the focal monkey visited their nearest corn platform first, and the preferred-food platform was not the next platform encountered if minimizing travel distance (Decision 2), the focal typically displayed one of the two following patterns of behavior. They could still prioritize the preferred-food platform but take the time to obtain one platform of corn on the way (i.e., a one corn platform en route to the banana), or they could visit the next corn platform encountered if taking the path that minimized travel costs. The monkeys used the one corn en route strategy in 23% of N = 495 trials and chose the next corn platform encountered in 77% of trials. When individuals visited the next corn platform encountered second (i.e., the solid arrows in Figure 2J), they continued on to the preferred-food platform in 91% of cases (i.e., the dotted arrows in Figure 2J), indicating that the “minimizing travel distance” strategy predominantly reflected a plan to visit the preferred-food platform when it was encountered (ESM 1,5), not that the focal was unwilling to try and obtain the preferred food-reward. Overall, focal individuals obtained the banana in 93% of trials, showing that their foraging strategies were typically effective in ensuring they gained access to their preferred food-reward. Focals were most likely to lose the preferred-food rewards to a competitor when exhibiting the minimizing travel distance strategy (banana lost in 7.7% of trials), and were least likely to lose the preferred-food reward when they rushed for the food patch containing the banana at the onset of the trial (2.2% of trials Strategy 1 was exhibited).
Whether or not the focal monkey was foraging in competition [i.e., competitor(s) were actively approaching the array or were within it] impacted the propensity to prioritize the platform with the preferred food. Of the N = 818 trials in which the preferred-food reward was 2 platforms away from the nearest platform (i.e., the trials included in the Decision 2 analyses), decision-makers prioritized the preferred-food reward by rushing for it at the onset of the trial in 56% of trials in which they were in competition from the onset (N = 422), but only 22% of the trials in which they were not in direct competition (N = 396). Conversely, decision-makers exhibited the minimizing travel distance strategy in only 28% of the trials in which they were competition, but 66% of the trials in which they started the trial foraging alone. The one corn en route to the preferred reward strategy was utilized in 16% of trials when in competition, and 12% of trials where no competitor was present at the onset of the trial.
Adult males prioritized the preferred-food by rushing for the banana at the onset of the trial in only 12% of trials. Conversely, when the focal was an adult female, subadult male or subadult female, they visited the preferred-food platform first in 35% of trials (Figure 5A). This difference, however, was not statistically significant (GLMM: N = 1,028, z = −0.868, P = 0.385). The only factor that influenced the propensity for adult males to prioritize the preferred-food platform was whether or not they made Decision 1 in the presence of a competitor (Table 1); however, this trend was not statistically significant. Furthermore, the poor performance of the adult-male/Decision 1 model compared to the null model (Likelihood ratio test: N = 572, X2 = 4.69, P = 0.196) suggests that the presence of a competitor did not have a consistent impact on their initial platform choice.
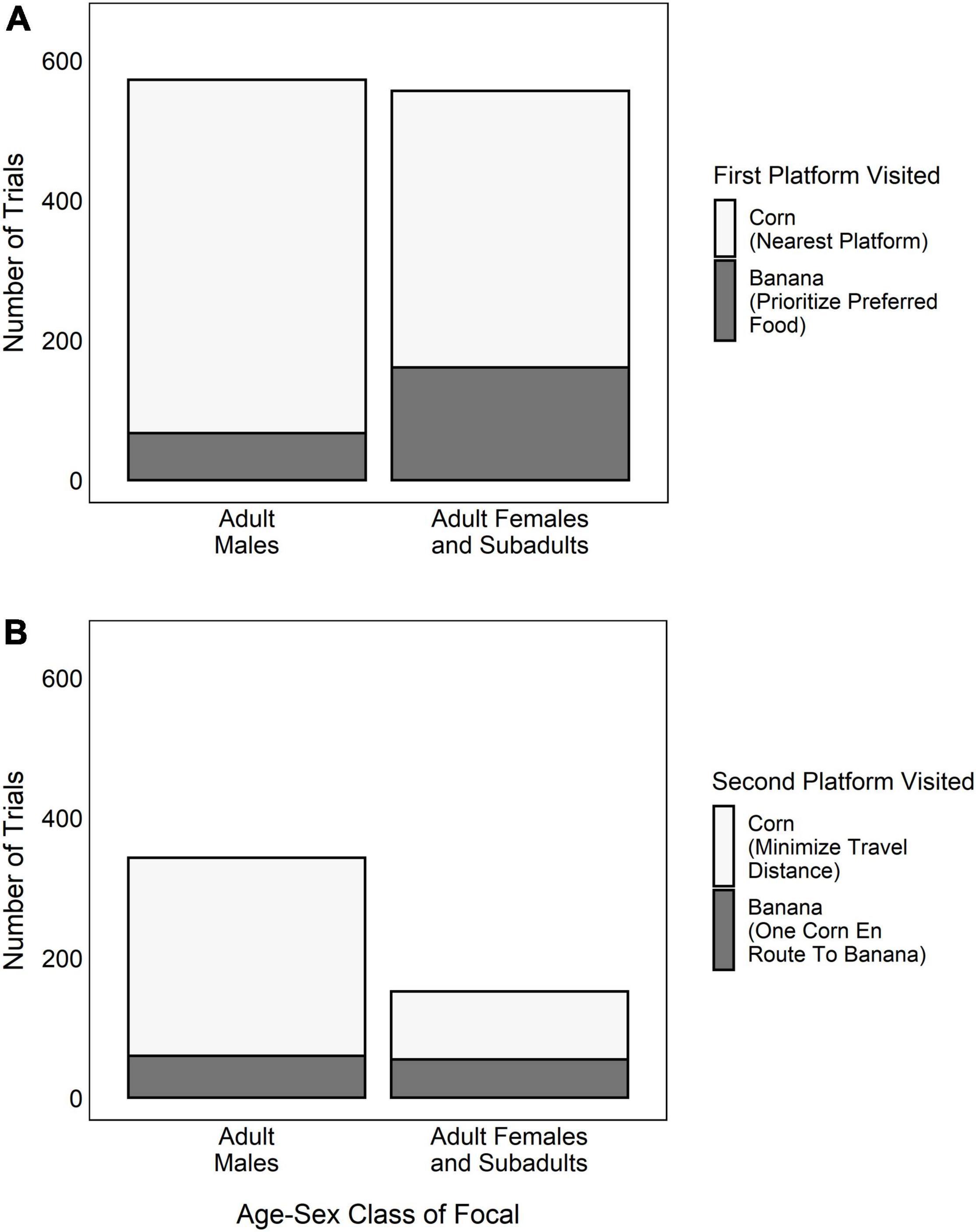
Figure 5. The number of trials in which adult males and adult females/subadults each chose different food rewards when making (A) Decision 1 (i.e., which platform to visit first), and (B) Decision 2 (i.e., which platform to visit second, if they had chosen their nearest corn platform in Decision 1).
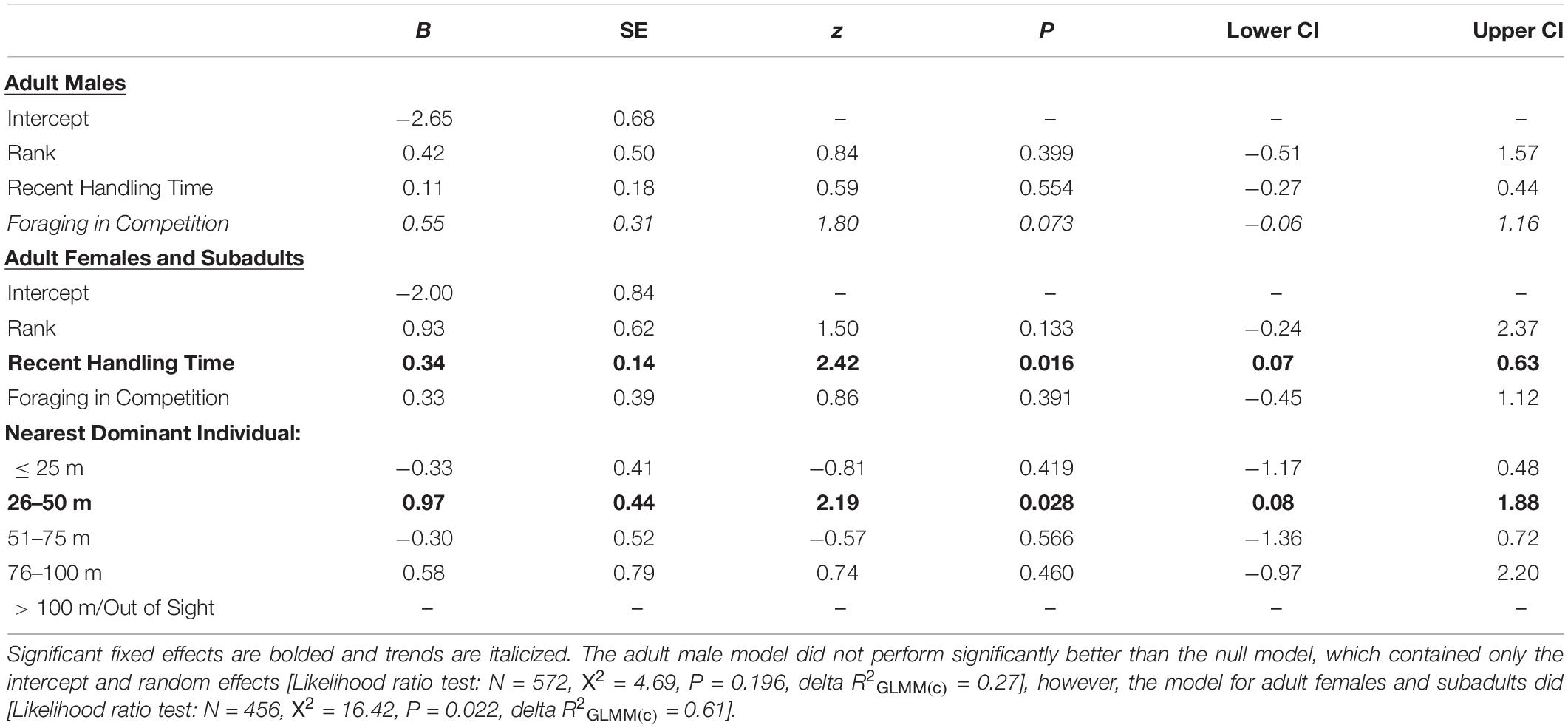
Table 1. Likelihood the first platform the focal individual visited (Decision 1) was the one containing the preferred-food/high-handling time food reward (i.e., the banana in a box that necessitated handling).
The age-sex classes that were low-ranking (i.e., adult females and subadults) were significantly more likely to prioritize the preferred-food platform if they were still inefficient at retrieving the banana from the box (i.e., their five most recent handling times were relatively long) (Table 1; ESM 2). We also found having a dominant group member 26–50 m away (Table 1; ESM 3), relative to the reference category (>100 m/out-of-sight), to have a significant impact on the initial platform choice of females/subadults, indicating that females/subadults were more likely to prioritize the preferred-food platform when there was a risk of being displaced by a dominant competitor. Adult females and subadults also showed a strong propensity to weigh the risk of contest competition when making Decision 2. We found that adult females/subadults were significantly more likely to visit the preferred-food platform second (i.e., do one corn platform en route to the banana instead of minimizing travel distance) when they had a dominant audience member 51–75 m away, than when there were no higher-ranking group members within 100 m/in sight (Table 2; ESM 4). That females/subadults choose the preferred-food platform first when there was a dominant competitor 26–50 m away, and were more likely to select it second when there was a dominant competitor 51–75 m away, suggests that the monkeys consider not only the relative rank of their audience members, but the travel time it would take potential competitors to arrive at the experiment site. Handling skill did not impact second platform choice in females/subadults, but they were more likely to prioritize the preferred-food reward if a competitor was present (Table 2).
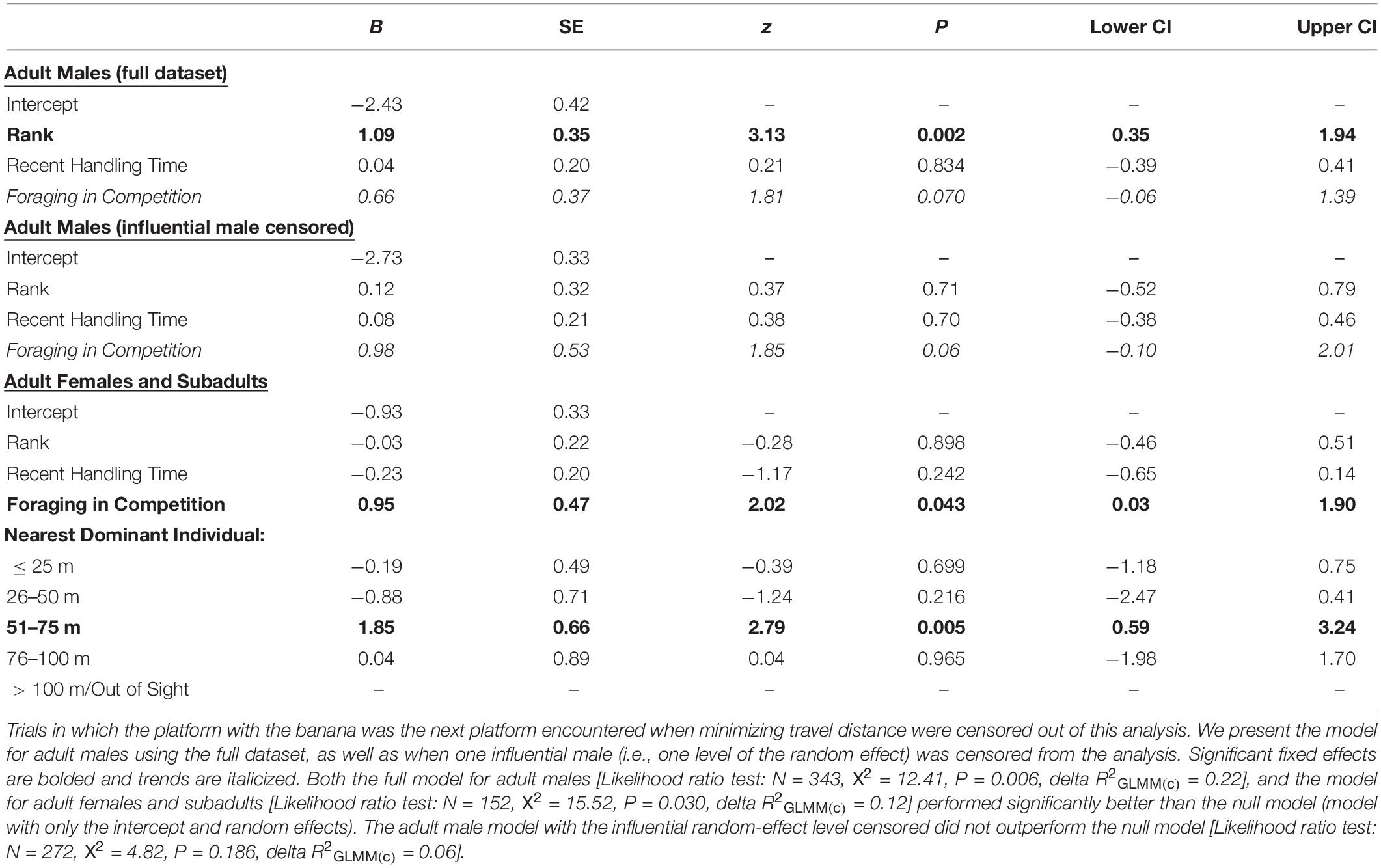
Table 2. In cases where the focal individual had chosen their nearest corn platform in Decision 1, the likelihood that the second platform visited (Decision 2) was the preferred-food/high-handling time one (i.e., a “one corn en route to the banana” strategy) instead of the next corn platform encountered if minimizing travel distance (i.e., a “minimizing travel distance” strategy).
Adult males were more likely to select the preferred-food platform second (i.e., do one corn en route to the banana) in trials where they were in competition (Table 2). However, overall adult males were significantly less likely to employ a one corn en route to the banana strategy than were adult females/subadults (GLMM: N = 495, z = −2.43, P = 0.015; Figure 5B). Of the trials in which they selected their nearest corn platform for Decision 1, adult males chose to minimize travel distance in 83% of trials and do one corn en route to the banana in 18% of trials (Figure 5B). Adult females and subadults used the one corn en route to the banana strategy in 36% of cases (Figure 5B). Lastly, we found that rank influenced Decision 2 for adult males, with higher-ranking males being more likely to do one corn en route to the banana than lower-ranking males (Table 2). However, this result was driven by the male who was the alpha male in the group for the majority of the study period. When he was censored from the analysis, we found no effect of rank on the propensity for adult males to use a one corn en route strategy. Adult males also showed a weak tendency to choose the platform with the preferred-food reward when making Decision 2 if they were foraging in competition (i.e., trend effect; Table 2).
Discussion
The results from this study demonstrate that vervet monkeys adapt their foraging decisions, depending on their experience and the current social context, to maximize food intake and decrease costs. Consistent with previous studies (Teichroeb and Aguado, 2016), solitarily foraging vervets were more likely to forage efficiently, going through the array along the path that minimized travel distance, while monkeys foraging in competition [i.e., with competitor(s) in close proximity (<25 m) to the platforms and attempting to access food] were more likely to prioritize the preferred-food platform. Beyond this, the large sample size in this study allowed us to show that vervet foraging decisions were much more complex than this simple dichotomy. Adult males, who had little risk of being displaced by a dominant competitor, tended to take the path that minimized travel distance unless they were foraging in direct competition. Given that they rarely had dominant audience members, it is likely that adult males were sensitive to the risk that a subordinate group member would steal the banana before they were able to displace them, or they wanted to avoid the conflict that might erupt from doing so. Adult females and subadults, whose lower rank meant they experienced high levels of contest competition at the experiment, appeared to assess the likelihood they would lose the preferred-food reward to a dominant competitor if they stopped to feed at low-value corn platforms first. If they were still slow at retrieving the banana from the food box (i.e., high handling-time, low skill), or if a higher-ranking group member was relatively close by (26–50 m away) so as to have a short travel time, adult females and subadults were more likely to rush for the preferred-food platform at the onset of the trial. However, if dominant audience member(s) had a longer travel time because they were 51–75 m away, adult females and subadults were more likely to take the time to eat one platform of corn on their way to their preferred food reward.
Handling times were highly variable (range: 1–69 s) and experience with the box improved most vervet’s skill in retrieving the banana from the box (i.e., decreased handling time) (Arseneau-Robar, unpublished data). For low-ranking individuals, handling skill impacted route choice decisions. Since food profitability improves considerably as handling time decreases (Pyke, 1984; Stephens and Krebs, 1986) and aggression levels decrease (Sirot, 2000; Johnson et al., 2001), experienced and proficient vervets could put in less effort, receive less aggression, and improve their energy gain. This allowed low-ranked individuals to forage more efficiently by saving on distance costs, and get all of the resources in the array when dominants were far enough away that their travel time to the array was great. Surprisingly, having a dominant audience member within 25 m did not affect the routing decisions of low-ranking monkeys as much as having dominants further away. This pattern was likely observed because when two individuals were within 25 m of the platforms at the onset of the trial, the lower-ranking individual typically waited for the dominant to take priority-of-access to the platforms of their choice. As a result, subordinate individuals tended to be the competitor rather than the focal, meaning we had a small sample size of trials in which the focal had a dominant audience member within 25 m. The propensity of subordinates to wait and see how many platforms they would be tolerated at highlights the need for future work to examine the contexts in which dominants tolerate subordinates at the array. Such investigations will improve our understanding of how both dominant and subordinate individuals make flexible decisions when foraging in competition.
We predicted that proficiency and skill in handling time would lead to more efficient foraging routes, regardless of dominance rank. Dominant animals, however, did not seem to make route choice decisions based on their skill level. Relative to subordinates, dominants were more likely to minimize travel distance, probably because they were not under pressure from audience members. Despite this, we did find that the alpha male was more likely to employ the intermediate strategy of getting one corn en route to the preferred-food platform, as opposed to minimizing travel distance. This pattern may have arose because he could monopolize the experiment until satiated. When nearing satiation, decision-makers sometimes decreased their consumption of the less-preferred corn, only eating the platform that was directly on their travel path to the more-preferred banana. If they continued to run trials, they could eventually only visit the platform with the banana, leaving all the corn platforms for a subordinate group member. This is similar to dominant male chacma baboons, who have been previously shown to prefer high-handling time patches because of their ability to monopolize them and kleptoparasitize others if they are already present (Marshall et al., 2012). Alternatively, the alpha male may have displayed high levels of tolerance toward competitors, allowing them to consume a larger proportion of the food rewards provided (ESM 6).
Animal foraging behavior is often altered in social contexts where an audience is present (Giraldeau and Caraco, 2000) and the risk of kleptoparastism increases (e.g., Hockey and Steele, 1990; Heinrich and Pepper, 1998; Jones et al., 2018). The presence of a dominance hierarchy usually means that the consequences of contest competition for food are experienced much more intensely by low-ranking animals that may need to adopt alternate strategies to gain food (e.g., Adams et al., 1998; Hollis et al., 2004a,b). In previous research, the effects of dominance rank and an audience in altering foraging behavior have been most clearly demonstrated in food caching species (Clarke and Kramer, 1994; Lahti and Rytkönen, 1996; Lahti et al., 1998; Dally et al., 2005; Samson and Manser, 2016). For example, in Cape ground squirrels (Xerus inauris), only low-ranked individuals, who were likely to lose food to dominants, avoided caching food when audience members were attentive to them (Samson and Manser, 2016). In primates, species dominance style has been linked to the strategies used by subordinates to retrieve food around dominants, with more despotic macaque species showing sneakier tactics by subordinates than more tolerant macaques (Gomez-Melara et al., 2021).
In the case of our vervet subjects, we observed the development of complex strategizing and decision-making by low-ranking individuals. Because male vervet monkeys are approximately 1.5 times larger than females and have bigger canines, adult males tend to be dominant to other age-sex classes [for exceptions see Young et al. (2017), Hemelrijk et al. (2020), and Li et al. (2021)]. Since the experimental array was set up in an area with good visibility for at least 100 m in each direction, low-ranking individuals were able to accurately assess competitor pressure, which allowed them to plan ahead (i.e., Mugan and MacIver, 2020) and make future-oriented predictions about competitor movements. Indeed, low-ranking monkeys in our study made quick routing decisions that were predicated on their own handling skill, the composition of the audience, and how far away certain individuals were. To do this, they needed to identify others and their relative rank and then assess whether they had enough time and were skilled enough to get corn and extricate the banana before dominants, given their distance and potential speed of travel, were able to arrive at the array. This shows complex, multifactor decisions that consider a great deal of contextual information. Interesting questions for future studies would be whether low-ranking monkeys further modulate their decisions according to features of their audience members other than their relative rank. For instance, do decision-makers consider how fast different group members can run or whether that audience member previously approached the experiment to be a competitor? Do they monitor audience members to see if they are attending to the experimental site, or not, as a means of assessing the likelihood the audience member will approach?
Simple heuristic rules can be used to decide on an action based on whether or not a dominant is in the audience (e.g., Cercocebus torquatus atys, Range and Noë, 2005); however, choosing an action based on the relative distance of dominants and their likely speed may require flexible decision-making abilities (Janmaat et al., 2014), and greater cognitive effort. Relational physical, spatial, and temporal reasoning are needed to track a particular individual and infer where and how fast they will move. These types of cognitive skills are likely ancient though, given their importance in prey tracking, as well as the long history of gregariousness in many animal lineages. Yoo et al. (2020) used joystick-trained rhesus macaques (Macaca mulatta) to do a pursuit task where “prey” were programed to follow intelligent escape algorithms. They found that the monkeys used the position, velocity, and acceleration (i.e., the three Newtonian variables) of prey to make accurate predictions about their future locations. In both this task and an evasion task (Yoo et al., 2021), neurons in the dorsal anterior cingulate cortex (dACC), which receives information from reward and navigational regions of the brain and transmits information to motor brain regions, were identified as active. Thus, the dACC may play an important role in tracking the elemental physical variables of position, velocity, and acceleration for the self, the prey, and the predator. It is possible that the same neural processes are involved when tracking the movements of competing group members to make predictions about their future positions relative to one’s own.
For decades, researchers have debated whether social or ecological selective pressures have driven the evolution of advanced cognition (Jolly, 1966; Parker and Gibson, 1977; Milton, 1981; Gibson, 1986; Byrne and Whiten, 1988; Byrne, 1988; Tomasello and Call, 1997; Dunbar, 1998; Sol et al., 2005; de Waal, 2007; Dunbar and Shultz, 2007; Burkart et al., 2009; Sol, 2009). However, it is increasingly argued that these social and ecological hypotheses are not mutually exclusive (Cunningham and Janson, 2007; Janson and Byrne, 2007), and that each of these selective pressures may have had domain-specific effects on the cognitive systems a species evolves (Rosati, 2017). Our study highlights the important role that social context plays in shaping foraging decisions, and the complexity that this dimension adds to the decision-making process. To successfully outcompete their group members, decision-makers need to attend to, process and respond to a multitude of ecological and social stimuli simultaneously, and flexibly adapt their behavior to optimize resource acquisition while also mitigating the loss of resources to competitors. Our findings suggest that in gregarious species, where foraging often takes place in dynamic social contexts, decision-makers likely utilize numerous social and ecological cognitive processes simultaneously. How social and ecological cognitive processes integrate together to determine the capacity for flexible decision-making behavior in gregarious species, is an exciting avenue of future research in comparative cognition.
Data Availability Statement
The raw data supporting the conclusions of this article will be made available by the authors, without undue reservation.
Ethics Statement
The methods were approved by the Uganda Wildlife Authority, the Uganda National Council for Science and Technology, and the University of Toronto Animal Care Committee.
Author Contributions
TJA-R and JT designed the study, analyzed the data, and wrote the manuscript. JT and PS funded the study. TJA-R collected the data. KA, EV, and TJA-R coded the data from video. All authors helped to edit the manuscript.
Funding
This research was funded by a University of Toronto Arts and Sciences Postdoctoral Fellowship, Concordia University, and a Natural Sciences and Engineering Research Council of Canada Discovery Grant (RGPIN_2016-06321).
Conflict of Interest
The authors declare that the research was conducted in the absence of any commercial or financial relationships that could be construed as a potential conflict of interest.
Publisher’s Note
All claims expressed in this article are solely those of the authors and do not necessarily represent those of their affiliated organizations, or those of the publisher, the editors and the reviewers. Any product that may be evaluated in this article, or claim that may be made by its manufacturer, is not guaranteed or endorsed by the publisher.
Acknowledgments
We are grateful to Eve Smeltzer, Lindah Nabukenya, Dennis Twinomugisha, Matovu Ponsyano, Livingstone Katwere, Hillary Tashobya, Nic Robar, Herman Kasozi, and the management team at Nabugabo for their assistance in the field. We would like to thank Karline Janmaat, Francine Dolins, Cécile Garcia, Dora Biro, and Sandrine Prat for the invitation to this special issue of Frontiers in Ecology and Evolution.
Supplementary Material
The Supplementary Material for this article can be found online at: https://www.frontiersin.org/articles/10.3389/fevo.2022.775288/full#supplementary-material
References
Adams, C. E., Huntingford, F. A., Turnbull, J. F., and Beattie, C. (1998). Alternative competitive strategies and the cost of food acquisition in juvenile Atlantic salmon (Salmo salar). Aquaculture 167, 17–26. doi: 10.1016/S0044-8486(98)00302-0
Albers, P. C. H., and de Vries, H. (2001). Elo-rating as a tool in the sequential estimation of dominance strengths. Anim. Behav. 61, 489–495. doi: 10.1006/anbe.2000.1571
Anholt, B. R., Ludwig, D., and Rasmussen, J. B. (1987). Optimal pursuit times: How long should predators pursue their prey? Theor. Popul. Biol. 31, 453–464. doi: 10.1016/0040-5809(87)90015-3
Bartoń, K. (2020). Mu-MIn: Multi-model Inference. R package version 1.43.17. Available online at: https://CRAN.R-project.org/package=MuMIn
Bates, D., Kliegel, R., Sasishth, S., and Baayen, H. (2015a). Parsimonious Mixed Models. Available online at: https://arxiv.org/abs/1506.04967 (accessed March 20, 2022).
Bates, D., Maechler, M., Bolker, B., and Walker, S. (2015b). Fitting linear mixed-effects models using lme4. J. Stat. Software 67, 1–48. doi: 10.18637/jss.v067.i01
Belsley, D. A., Kuh, E., and Welsch, R. E. (2004). Regression Diagnostics: Identifying Influential Data and Sources of Collinearity. Hoboken NJ: John Wiley and Sons.
Broom, M., and Ruxton, G. D. (2003). Evolutionary stable kleptoparasitism: consequences of different prey types. Behav. Ecol. 14, 23–33. doi: 10.1093/beheco/14.1.23
Bugnyar, T., and Heinrich, B. (2005). Ravens, Corvus corax, differentiate between knowledgeable and ignorant competitors. Proc. Biol. Sci. 272, 1641–1646. doi: 10.1098/rspb.2005.3144
Burkart, J. M., Hrdy, S. B., and van Schaik, C. P. (2009). Cooperative breeding and human cognitive evolution. Evol. Anthropol. 18, 175–186. doi: 10.1002/evan.20222
Byrne, R. W. (1988). “The technical intelligence hypothesis: an additional evolutionary stimulus to intelligence?,” in Machiavellian Intelligence: Social Expertise and Evolution of Intellect in Monkeys, Apes, and Humans, eds R. W. Byrne and A. Whiten (Oxford: Oxford University Press), 289–311. doi: 10.1017/cbo9780511525636.012
Byrne, R. W., and Whiten, A. (eds) (1988). Machiavellian Intelligence: Social Expertise and Evolution of Intellect in Monkeys, Apes, and Humans. Oxford: Oxford University Press.
Cadieu, N., Winterton, P., and Cadieu, J. C. (2008). Social transmission of food handling in the context of triadic interactions between adults and young canaries (Serinus canaria). Behav. Ecol. Sociobiol. 62, 795–804. doi: 10.1007/s00265-007-0505-0
Catania, K. C., and Remple, F. E. (2005). Asymptotic prey profitability drives star-nosed moles to the foraging speed limit. Nature 433, 519–522. doi: 10.1038/nature3250
Chapman, C. A., Twinomugisha, D., Teichroeb, J. A., Valenta, K., Sengupta, R., Sarkar, D., et al. (2016). “How do primates survive among humans? Mechanisms employed by vervet monkeys at Lake Nabugabo, Uganda,” in Ethnoprimatology, ed. M. Waller (Berlin: Springer), 77–94. doi: 10.1007/978-3-319-30469-4_5
Clarke, M. F., and Kramer, D. L. (1994). Scatter-hoarding by a larder-hoarding rodent: intraspecific variation in the hoarding behaviour of the eastern chipmunk, Tamias striatus. Anim. Behav. 48, 299–308. doi: 10.1006/anbe.1994.1243
Cooper, W. E., and Anderson, R. A. (2006). Adjusting prey handling times and methods affects profitability in the broad-headed skink (Eumeces laticeps). Herpetologica 62, 356–365. doi: 10.1655/0018-0831200662
R Core Team (2019). R: A Language and Environment for Statistical Computing. Available online at: http://www.R-project.org/
Croy, M. I., and Hughes, R. N. (1991). Effects of food supply, hunger, danger and competition on choice of foraging location by the fifteen-spined stickleback, Spinachia spinachia L. Anim. Behav. 42, 131–139. doi: 10.1016/S0003-3472(05)80613-X
Cunningham, E., and Janson, C. (2007). A socioecological perspective on primate cognition, past and present. Anim. Cogn. 10, 273–281. doi: 10.1007/s10071-007-0078-3
Dally, J. M., Emery, N. J., and Clayton, N. S. (2005). The social suppression of caching in western scrub-jays (Aphelocoma californica). Behaviour 142, 961–977. doi: 10.1163/1568539055010084
Dally, J. M., Emery, N. J., and Clayton, N. S. (2006). Foodcaching western scrub-jays keep track of who was watching when. Science 312, 1662–1665. doi: 10.1126/science1126539
Dunbar, R. I. M. (1998). The social brain hypothesis. Evol. Anthropol. 6, 178–190. doi: 10.1002/(SICI)1520-650519986:5<178:AID-EVAN<3.0.CO;2-8
Dunbar, R. I. M., and Shultz, S. (2007). Evolution in the social brain. Science 317, 1344–1347. doi: 10.1126/science.1145463
Field, A., Miles, J., and Field, Z. (2012). Discovering Statistics Using R. Thousand Oaks, CA: Sage Publications.
Fortin, D., and Fortin, M. E. (2009). Group-size-dependent association between food profitability, predation risk and distribution of free-ranging bison. Anim. Behav. 78, 887–892. doi: 10.1016/j.anbehav.2009.06.026
Fox, J., and Weisberg, S. (2019). An R Companion to Applied Regression. Thousand Oaks, CA: Sage Publications.
Fritz, H., and de Garine-Wichatitsky, M. (1996). Foraging in a social antelope: effects of group size on foraging choices and resource perception in impala. J. Anim. Ecol. 65, 736–742. doi: 10.2307/5672
Gibson, K. R. (1986). “Cognition, brain size and the extraction of embedded food resources,” in Primate Ontogeny, Cognition, and Social Behaviour, eds J. G. Else and P. C. Lee (Cambridge: Cambridge University Press), 93–104.
Giraldeau, L.-A., and Caraco, T. (2000). Social Foraging Theory. Princeton, NJ: Princeton University Press.
Gomez-Melara, J. L., Acosta-Naranjo, R., Castellano-Navarro, A., Francés, V. B., Caicoya, A. L., MacIntosh, A. J., et al. (2021). Dominance style predicts differences in food retrieval strategies. Sci. Rep. 11:2726. doi: 10.1038/s41598-021-82198-0
Gunst, N., Boinski, S., and Fragaszy, D. M. (2010). Development of skilled detection and extraction of embedded prey by wild brown capuchin monkeys (Cebus apella apella). J. Comp. Psychol. 124, 194–204. doi: 10.1037/a0017723
Harrison, X. A., Donaldson, L., Correa-Cano, M. E., Evans, J., Fisher, D. N., Goodwin, C. E. D., et al. (2018). A brief introduction to mixed effects modelling and multi-model inference in ecology. PeerJ 6:e4794. doi: 10.7717/peerj.4794
Hartig, F. (2021). DHARMa: Residual Diagnostics for Hierarchical (Multi-Level/Mixed) Regression Models. R package version 0.3.3.0. Available online at: https://CRAN.R-project.org/package=DHARMa
Heinrich, B., and Pepper, J. W. (1998). Influence of competitors on caching behaviour in the common raven, Corvus corax. Anim. Behav. 56, 1083–1090. doi: 10.1006/anbe.1998.0906
Held, S., Mendl, M., Devereux, C., and Byrne, R. W. (2002). Foraging pigs alter their behaviour in response to exploitation. Anim. Behav. 64, 157–166. doi: 10.1006/anbe.2002.3044
Hemelrijk, C. K., Wubs, M., Gort, G., Botting, J., and van de Waal, E. (2020). Dynamics of intersexual dominance and adult sex-ratio in wild vervet monkeys. Front. Psychol. 11:839. doi: 10.3389/fpsyg.2020.00839
Hockey, P. A., and Steele, W. K. (1990). “Intraspecific kleptoparasitism and foraging efficiency as constraints on food selection by kelp gulls Larus dominicanus,” in Behavioural Mechanisms of Food Selection, ed. R. N. Hughes (Berlin: Springer), 679–706. doi: 10.1007/978-3-642-75118-9_33
Hollis, K. L., Langworthy-Lam, K. S., Blouin, L. A., and Romano, M. C. (2004a). Novel strategies of subordinate fish competing for food: learning when to fold. Anim. Behav. 68, 1155–1164. doi: 10.1016/j.anbehav.2003.11.022
Hollis, K. L., Blouin, L. A., Romano, M. C., Langworthy-Lam, K. S., and Siegenthaler, J. (2004b). Maintaining a competitive edge: dominance hierarchies, food competition and strategies to secure food in green anoles (Anolis carolinensis) and firemouth cichlids (Thorichthys meeki). Int. J. Comp. Psychol. 17, 222–240.
Isbell, L. A., Pruetz, J. D., and Young, T. P. (1998). Movements of vervets (Cercopithecus aethiops) and patas monkeys (Erythrocebus patas) as estimators of food resource size, density, and distribution. Behav. Ecol. Sociobiol. 42, 123–133. doi: 10.1007/s002650050420
Isbell, L. A., and Young, T. P. (1993). Social and ecological influences on activity budgets of vervet monkeys, and their implications for group living. Behav. Ecol. Sociobiol. 32, 377–385. doi: 10.1007/BF00168821
Janmaat, K. R., Polansky, L., Ban, S. D., and Boesch, C. (2014). Wild chimpanzees plan their breakfast time, type, and location. Proc. Nat. Acad. Sci. U.S.A. 111, 16343–16348. doi: 10.1073/pnas.1407524111
Janson, C. (1985). Aggressive competition and individual food consumption in wild brown capuchin monkeys (Cebus apella). Behav. Ecol. Sociobiol. 18, 125–138. doi: 10.1007/BF00299041
Janson, C. H. (2000). “Spatial movement strategies: theory, evidence, and challenges,” in On the Move: How and Why Animals Travel in Groups, eds S. Bionski and P. A. Garber (Chicago IL: University of Chicago Press), 165–203.
Janson, C. H. (2007). Experimental evidence for route integration and strategic planning in wild capuchin monkeys. Anim. Cogn. 10, 341–356. doi: 10.1007/s10071-007-0079-2
Janson, C. H., and Byrne, R. (2007). What wild primates know about resources: opening up the black box. Anim. Cogn. 10, 357–367. doi: 10.1007/s10071-007-0080-9
Janson, C. H., and van Schaik, C. P. (1988). Recognizing the many faces of primate food competition: methods. Behaviour 105, 165–186. doi: 10.1163/156853988X00502
Johnson, C., Grant, J. W., and Giraldeau, L. A. (2001). The effect of handling time on interference among house sparrows foraging at different seed densities. Behaviour 138, 597–614. doi: 10.1163/156853901216924494
Jolly, A. (1966). Lemur social behavior and primate intelligence. Science 153, 501–506. doi: 10.1126/science.153.3735.501
Jones, N. A., Webster, M., Templeton, C. N., Schuster, S., and Rendell, L. (2018). Presence of an audience and consistent interindividual differences affect archerfish shooting behaviour. Anim. Behav. 141, 95–103. doi: 10.1016/j.anbehav.2018.04.024
Korstjens, A. H., Sterck, E. H. M., and Noë, R. (2002). How adaptive or phylogenetically inert is primate social behaviour? A test with two sympatric colobines. Behaviour 139, 203–225. doi: 10.1163/156853902760102654
Kumpan, L. T., Rothman, J. M., Chapman, C. A., and Teichroeb, J. A. (2019). Playing it safe? Solitary vervet monkeys (Chlorocebus pygerythrus) choose high-quality foods more than those in competition. Am. J. Primatol. 81:e23002. doi: 10.1002/ajp.23002
Lahti, K., Koivula, K., Rytkönen, S., Mustonen, T., Welling, P., Pravosudov, V. V., et al. (1998). Social influences on food caching in willow tits: a field experiment. Behav. Ecol. 9, 122–129. doi: 10.1093/beheco/9.2.122
Lahti, K., and Rytkönen, S. (1996). Presence of conspecifics, time of day and age affect willow tit food hoarding. Anim. Behav. 52, 631–636. doi: 10.1006/anbe.1996.0204
Li, M. F., Arseneau-Robar, T. J. M., Smeltzer, E. A., and Teichroeb, J. A. (2021). Be early or be tolerated: vervet monkey, Chlorocebus pygerythrus, foraging strategies in a dispersed resource. Anim. Behav. 176, 1–15. doi: 10.1016/j.anbehav.2021.03.010
Lihoreau, M., Chittka, L., and Raine, N. E. (2011). Trade-off between travel distance and prioritization of high-reward sites in traplining bumblebees. Func. Ecol. 25, 1284–1292. doi: 10.1111/j.1365-2435.2011.01881.x
MacArthur, R. H., and Pianka, E. (1966). On optimal use of a patchy environment. Am. Nat. 100, 603–609. doi: 10.1086/282454
Marshall, H. H., Carter, A. J., Coulson, T., Rowcliffe, J. M., and Cowlishaw, G. (2012). Exploring foraging decisions in a social primate using discrete-choice models. Am. Nat. 180, 481–495. doi: 10.1086/667587
Matuschek, H., Kliegl, R., Vasishth, S., Baayen, H., and Bates, D. (2017). Balancing Type I error and power in linear mixed models. J. Mem. Lang. 94, 305–315. doi: 10.1016/j.jml.2017.01.001
Menzel, C. R. (1997). “Primates’ knowledge of their natural habitat: as indicated by foraging,” in Machiavellian Intelligence II: Extensions and Evaluations, eds A. Whiten and R. W. Byrne (Cambridge: Cambridge University Press), 207–239. doi: 10.1017/cbo9780511525636.009
Menzel, C. R., Menzel, E. W. Jr., Kelley, J. W., Chan, B., Evans, T. A., and Stone, B. W. (2008). The rank ordering problem in primate foraging. Am. J. Primatol. 70(Suppl. 1):64. doi: 10.1093/acprof:oso/9780195326598.003.0004
Milton, K. (1981). Distribution patterns of tropical plant foods as an evolutionary stimulus to primate mental development. Am. Anthropol. 83, 534–548. doi: 10.1525/aa.1981.83.3.02a00020
Molvar, E. M., and Bowyer, R. T. (1994). Costs and benefits of group living in a recently social ungulate: the Alaskan moose. J. Mamm. 75, 621–630. doi: 10.2307/1382509
Mugan, U., and MacIver, M. A. (2020). Spatial planning with long visual range benefits escape from visual predators in complex naturalistic environments. Nat. Commun. 11:3057. doi: 10.1038/s41467-020-16102-1
Nakagawa, S., and Schielzeth, H. (2013). A general and simple method for obtaining R2 from generalized linear mixed-effects models. Methods Ecol. Evol. 4, 133–142. doi: 10.1111/j.2041-210x.2012.00261.x
Neumann, C., Duboscq, J., Dubuc, C., Ginting, A., Irwan, A. M., Agil, M., et al. (2011). Assessing dominance hierarchies: validation and advantages of progressive evaluation with Elo-rating. Anim. Behav. 82, 911–921. doi: 10.1016/j.anbehav.2011.07.016
Neumann, C., and Kulik, L. (2020). EloRating-package: Animal Dominance Hierarchies by Elo Rating. R package version 0.46.11. Available online at: https://CRAN.R-project.org/package=EloRating
Nicholson, A. J. (1954). An outline of the dynamics of animal populations. Aust. J. Zool. 2, 9–65. doi: 10.1071/ZO9540009
Nieuwenhuis, R., Grotenhuis, M., and Pelzer, B. (2012). Influence.ME: tools for detecting influential data in mixed effects models. R J. 4, 38–47. doi: 10.32614/RJ-2012-011
Ostojić, L., Legg, E. W., Brecht, K. F., Lange, F., Deininger, C., Mendl, M., et al. (2017). Current desires of conspecific observers affect cache-protection strategies in California scrub-jays and Eurasian jays. Curr. Biol. 27, R51–R53. doi: 10.1016/j.cub.2016.11.020
Paredes, R., Orben, R. A., Roby, D. D., Irons, D. B., Young, R., Renner, H., et al. (2015). Foraging ecology during nesting influences body size in a pursuit-diving seabird. Mar. Ecol. Prog. Ser. 533, 261–276. doi: 10.3354/meps11388
Parker, S. T., and Gibson, K. R. (1977). Object manipulation, tool use and sensorimotor intelligence as feeding adaptations in Cebus monkeys and great apes. J. Hum. Evol. 6, 623–641. doi: 10.1016/S0047-2484(77)80135-8
Pyke, G. H. (1984). Optimal foraging theory: a critical review. Ann. Rev. Ecol. Syst. 15, 523–575. doi: 10.1146/annurev.es.15.110184.002515
Range, F., and Noë, R. (2005). Can simple rules account for the pattern of triadic interactions in juvenile and adult female sooty mangabeys? Anim. Behav. 69, 445–452. doi: 10.1016/j.anbehav.2004.02.025
Reader, S. M., and Biro, D. (2010). Experimental identification of social learning in wild animals. Learn. Behav. 38, 265–283. doi: 10.3758/LB.38.3.265
Rosati, A. G. (2017). Foraging cognition: reviving the ecological intelligence hypothesis. Trends Cogn. Sci. 21, 691–702. doi: 10.1016/j.tics.2017.05.011
Rosati, A. G., and Hare, B. (2012). Decision-making across social contexts: competition increases preference for risk in chimpanzees. Anim. Behav. 84, 869–879. doi: 10.1016/j.anbehav.2012.07.010
Samson, J., and Manser, M. B. (2016). Caching in the presence of competitors: Are Cape ground squirrels (Xerus inauris) sensitive to audience attentiveness? Anim. Cogn. 19, 31–38. doi: 10.1007/s10071-015-0910.0
Sayers, K., and Menzel, C. R. (2012). Memory and foraging theory: chimpanzee utilization of optimality heuristics in the rank-order recovery of hidden foods. Anim. Behav. 84, 795–803. doi: 10.1016/j.anbehav.2012.06.034
Shively, C. (1985). ““The evolution of dominance hierarchies in nonhuman primate society”,” in Power, Dominance, and Nonverbal Behavior, eds S. L. Ellysen and J. F. Dovidio (Berlin: Springer), 67–87. doi: 10.1007/978-1-4612-5106-4_4
Shrader, A. M., Kerley, G. I. H., Kotler, B. P., and Brown, J. S. (2006). Social information, social feeding, and competition in group-living goats (Capra hircus). Behav. Ecol. 18, 103–107. doi: 10.1093/beheco/arl057
Sirot, E. (2000). An evolutionarily stable strategy for aggressiveness in feeding groups. Behav. Ecol. 11, 351–356. doi: 10.1093/bheco/11.4.351
Sol, D. (2009). Revisiting the cognitive buffer hypothesis for the evolution of large brains. Biol. Lett. 5, 130–133. doi: 10.1098/rsbl.2008.0621
Sol, D., Duncan, R. P., Blackburn, T. M., Cassey, P., and Lefebvre, L. (2005). Big brains, enhanced cognition, and response of birds to novel environments. Proc. Natl. Acad. Sci. U.S.A. 102, 5460–5465. doi: 10.1083/pnas.0408145102
Steele, W. K., and Hockey, P. A. (1995). Factors influencing rate and success of intraspecific kleptoparasitism among kelp gulls (Larus dominicanus). Auk 112, 847–859. doi: 10.2307/4089017
Stephens, D. W., Brown, J. S., and Ydenberg, R. C. (2007). Foraging: Behavior and Ecology. Chicago, IL: University of Chicago Press.
Stephens, D. W., and Krebs, J. R. (1986). Foraging Theory. Princeton, NJ: Princeton University Press.
Stillman, R. A., Caldow, R. W. G., Goss-Custard, J. D., and Alexander, M. J. (2000). Individual variation in intake rate: the relative importance of foraging efficiency and dominance. J. Anim. Ecol. 69, 484–493. doi: 10.1046/j.1365-2656.2000.00410.x
Teichroeb, J. A. (2015). Vervet monkeys use paths consistent with context-specific spatial movement heuristics. Ecol. Evol. 5, 4706–4716. doi: 10.1002/ece3.1755
Teichroeb, J. A., and Aguado, W. D. (2016). Foraging vervet monkeys optimize travel distance when alone but prioritize high-reward food sites when in competition. Anim. Behav. 115, 1–10. doi: 10.1016/j.anbehav.2016.02.020
Teichroeb, J. A., and Smeltzer, E. A. (2018). Vervet monkey (Chlorocebus pygerythrus) behavior in a multi-destination route: evidence for planning ahead when heuristics fail. PLoS One 13:e0198076. doi: 10.1371/journal.pone.0198076
Thornton, A., and Clutton-Brock, T. (2011). Social learning and the development of individual and group behaviour in mammal societies. Philos. Trans. R. Soc. Lond. B Biol. Sci. 366, 978–987. doi: 10.1098/rstb.2010.0312
Vogel, E. R. (2005). Rank differences in energy intake rates in white-faced capuchin monkeys, Cebus capucinus: the effects of contest competition. Behav. Ecol. Sociobiol. 58, 333–344. doi: 10.1007/s00265-005-0960-4
Whiten, A., and Mesoudi, A. (2008). Establishing an experimental science of culture: animal social diffusion experiments. Philos. Trans. R. Soc. Lond. B Biol. Sci. 363, 3477–3488. doi: 10.1098/rstb.2008.0134
Wikberg, E. C., Teichroeb, J. A., Bãdescu, I., and Sicotte, P. (2013). Individualistic female dominance hierarchies with varying strength in a highly folivorous population of black-and-white colobus. Behaviour 150, 295–320. doi: 10.1163/1568539X-00003050
Wilson, R. P., Griffiths, I. W., Mills, M. G., Carbone, C., Wilson, J. W., and Scantlebury, D. M. (2015). Mass enhances speed but diminishes turn capacity in terrestrial pursuit predators. eLife 4:e06487. doi: 10.7554/eLife.06487
Wrangham, R. W. (1981). Drinking competition in vervet monkeys. Anim. Behav. 29, 904–910. doi: 10.1016//S0003-3472(81)80027-9
Yoo, S. B. M., Tu, J. C., and Hayden, B. Y. (2021). Multicentric tracking of multiple agents by anterior cingulate cortex during pursuit and evasion. Nat. Commun. 12:1985. doi: 10.1038/s41467-021-22195-z
Yoo, S. B. M., Tu, J. C., Piantadosi, S. T., and Hayden, B. Y. (2020). The neural basis of predictive pursuit. Nat. Neurosci. 23, 252–259. doi: 10.1038/s41593-019-0561-6
Young, C., McFarland, R., Barrett, L., and Henzi, S. P. (2017). Formidable females and the power trajectories of socially integrated male vervet monkeys. Anim. Behav. 125, 61–67. doi: 10.1016/j.anbehav.2017.01.006
Keywords: distance optimization, optimal foraging theory, multi-destination array, decision making, social context, foraging experiment, handling time, dominance rank
Citation: Arseneau-Robar TJM, Anderson KA, Vasey EN, Sicotte P and Teichroeb JA (2022) Think Fast!: Vervet Monkeys Assess the Risk of Being Displaced by a Dominant Competitor When Making Foraging Decisions. Front. Ecol. Evol. 10:775288. doi: 10.3389/fevo.2022.775288
Received: 13 September 2021; Accepted: 28 March 2022;
Published: 22 April 2022.
Edited by:
Cécile Garcia, UMR 7206 Eco Anthropologie et Ethnobiologie (EAE), FranceReviewed by:
Daizaburo Shizuka, University of Nebraska-Lincoln, United StatesIain James Gordon, Australian National University, Australia
Karline Janmaat, University of Amsterdam, Netherlands
Copyright © 2022 Arseneau-Robar, Anderson, Vasey, Sicotte and Teichroeb. This is an open-access article distributed under the terms of the Creative Commons Attribution License (CC BY). The use, distribution or reproduction in other forums is permitted, provided the original author(s) and the copyright owner(s) are credited and that the original publication in this journal is cited, in accordance with accepted academic practice. No use, distribution or reproduction is permitted which does not comply with these terms.
*Correspondence: Julie A. Teichroeb, julie.teichroeb@utoronto.ca