Forest Diversity Reduces the Prevalence of Pathogens Transmitted by the Tick Ixodes ricinus
- 1INRAE, University of Bordeaux, UMR Biogeco, Cestas, France
- 2Paris-Saclay University, AgroParisTech, INRAE, JRU MIA-Paris, Paris, France
- 3INRAE, UMR 1136 “Interactions Arbres Micro-Organismes,” Centre INRAE Grand Est-Nancy, Champenoux, France
- 4ANSES, INRAE, Ecole Nationale Vétérinaire d’Alfort, UMR BIPAR, Laboratoire de Santé Animale, Maisons-Alfort, France
- 5Geobotany, Faculty of Biology, University of Freiburg, Freiburg, Germany
Tick-borne diseases represent the majority of vector-borne human diseases in Europe, with Ixodes ricinus, mostly present in forests, as the main vector. Studies show that vertebrate hosts diversification would decrease the prevalence of these pathogens. However, it is not well known whether habitat diversity can have similar impact on ticks and their infection rates. We measured the presence and abundance of different stages of I. ricinus, and the prevalence of associated pathogens in a large-scale forest experiment in which we manipulated tree diversity and moisture level. We showed that larval abundance was influenced by tree species identity, with larvae being more present in pine plots than in oak plots, while nymph abundance increased with canopy tree density. The proportion of Borrelia burgdorferi s.l.-infected nymphs decreased with increasing tree diversity. Our findings suggest that tree overstorey composition, structure and diversity, can affect tick abundance and pathogen prevalence. They support the idea that forest habitats may have “diluting” or “amplifying” effects on tick-borne diseases with direct relevance for human health.
Introduction
A growing body of observational and experimental evidence confirms that more diverse forests provide better support to the provision of ecosystem services and improve nature’s contributions to people (Brockerhoff et al., 2017; Messier et al., 2021). Provisioning services such as biomass production (Zhang et al., 2012; Jactel et al., 2018), regulating services such as climate change mitigation through carbon sequestration (Harmon et al., 2013; Lange et al., 2015), pest and fungal disease control (Jactel et al., 2017, 2021), habitat provision for biodiversity (Ampoorter et al., 2020; Stemmelen et al., 2021) are on average often higher in mixed forests than in pure forests. However, it is important to note that forests and other ecosystems can also provide “disservices,” i.e., risks for humans (Dunn, 2010), such as those related to human health. Ecosystem disservices have been much less in the focus of science, although they have recently gained attention with the emergence of epidemics affecting human populations that are increasingly in contact with the wilderness due to deforestation (Jones et al., 2013; Smith et al., 2014; Everard et al., 2020). Even less known is the role of forest diversity in reducing the risk of zoonotic diseases, i.e., whether biodiversity in forests can mitigate ecosystem disservices. The dilution effect hypothesis (Schmidt and Ostfeld, 2001; Halliday et al., 2020) suggests that host species diversity would play a protective role in the spread of pathogens because some host species would not allow the multiplication of viruses or bacteria (low reservoir competence), constituting transmission dead ends, or because the complexity of food webs would allow top down regulation of disease hosts. This theory seems to apply well to the risk of tick-borne diseases (Diuk-Wasser et al., 2021). For example, LoGiudice et al. (2003) predicted a reduction in the prevalence of Lyme disease caused by bacteria of the genus Borrelia, with increasing host diversity. Hofmeester et al. (2017) reported that increasing the abundance of predatory mammals reduced the density of disease-carrying tick nymphs in prey rodents. American studies on Ixodes scapularis-borne disease showed that the diversity of vertebrate hosts could decrease Borrelia prevalence in ticks through the dilution effect of competent hosts by incompetent hosts (Ostfeld and Keesing, 2012; Linske et al., 2018). However, these arguments remain controversial, because the density of competent hosts may not always decrease sufficiently with increasing host diversity to reduce the density of infected nymphs, or because ticks can show a strong preference for competent hosts (Randolph and Dobson, 2012). For example, Ruyts et al. (2018) found no dilution effects in European forests for Ixodes ricinus, the most common tick species in western Europe (de la Fuente, 2008). In addition to this host-dilution hypothesis, Ehrmann et al. (2018) suggested that habitats can also be classified into “dilution” and “amplification” habitats that reduce or increase the prevalence of tick-borne disease vectors, respectively, at the local patch or even regional landscape scale. Such “dilution habitats” were characterized by a structurally and functionally diverse tree layer, diverse and abundant understorey vegetation, and increased levels of deadwood in European forests. However, we still lack investigations that are able to disentangle the role of tree diversity from that of other environmental factors in regulating tick abundance and the prevalence of tick-borne diseases. Experiments that manipulate tree diversity independently from other factors offer one promising way into this direction.
The main vector of tick-borne diseases in Europe (de la Fuente, 2008), I. ricinus (castor bean tick) is mainly present in forests and woodlands (Estrada-Peña, 2001). It is an obligate hematophagous that requires three blood meals to complete its life cycle and pass from larva to nymph, from nymph to immature adult and from immature adult to egg-laying female, respectively. These different stages depend on different habitat characteristics and microclimates (Ehrmann et al., 2017). I. ricinus is responsible for the transmission of several pathogenic bacteria, viruses and protozoa (Rizzoli et al., 2014; Prinz et al., 2017) including the bacterial complex Borrelia burgdorferi sensu lato, the tick-borne agent of Lyme borreliosis. But other pathologies are also emerging, related to Anaplasma, Borrelia, Neoehrlichia, Rickettsia and Babesia species (Kilpatrick and Randolph, 2012; Keesing and Ostfeld, 2021). Because there is no vaccine available against these growing diseases (Steere et al., 2004) with sometimes very serious health consequences, it is very important to search for methods to prevent the risk of transmission.
Ticks can be infected in three ways: (i) by feeding a blood meal on infected hosts (ii) by co-feeding, which is a direct transmission from a tick to another one feeding on the same host at the same time, and (iii) by transovarial (or vertical) transmission. This latter pathway is only possible for a few pathogens (Sprong et al., 2009; Burri et al., 2014) such as some Rickettsia or Babesia species for unfed I. ricinus larvae, and is the main way for larvae to become infected before a first blood meal. Knowing that the probability for one tick to be infected increases with the number of blood meals taken and that nymphs are more abundant in the environment than adults, many studies consider the density of infected nymphs as an indicator of the level of contamination risk in the environment (Mather et al., 1996; Stafford et al., 1998; Diuk-Wasser et al., 2012; Pepin et al., 2012). As a result, the presence and abundance of tick larvae remain less frequently monitored. Although larvae have a lower potential for transmission, their survival in the environment influences directly the abundance of future nymphs, infected or not, and indirectly the epidemiological risk associated (Ehrmann et al., 2017).
The risk of tick bites and disease transmission in forests is directly influenced by the density of infected ticks in the environment (Fischhoff et al., 2019), which in turn depends on interactions between biotic (e.g., host community) and abiotic (e.g., microclimate) conditions. Moreover, understorey vegetation plays a central role in the population dynamics of ticks. First of all, the structure of the understorey vegetation directly conditions the ability to reach the hosts for the questing ticks that are hanging to plant parts (McCoy and Boulanger, 2015). Then the understorey may provide more or less favorable feeding, breeding or resting sites for the vertebrate hosts that ensure the survival, infection and transport of ticks (Allan et al., 2010). Finally, the structure and composition of the understorey condition the microclimate that affects the survival and questing activity of ticks (McCoy and Boulanger, 2015; Ehrmann et al., 2017) and more particularly the humidity (Boyard et al., 2007; Estrada-Peña, 2008). Forest diversity may therefore indirectly influence tick abundance through its effect on understorey vegetation. Several studies have indeed established a causal link between tree diversity and understorey diversity or composition (Gazol and Ibáñez, 2009; Ampoorter et al., 2014; Corcket et al., 2020). Tree species diversity and composition have also been shown to mitigate understorey microclimate (De Frenne et al., 2019). Mixed forests are characterized by a high degree of canopy closure, made possible by a greater complementarity in crown shape, a process known as canopy packing (Jucker et al., 2015; Williams et al., 2017). A denser canopy then produces a shading effect that can increase the humidity in the understory, which favors the survival of ticks in case of drought. Other studies have demonstrated that forest species diversity can alter the abundance and foraging behavior of herbivorous vertebrates (i.e., potential tick hosts), often with contrasting effects depending on the size of these animals, reducing the browsing activity of small animals (e.g., mice and voles) and increasing that of large ones (e.g., moose and deer) (Milligan and Koricheva, 2013; Jactel et al., 2017). However, very few studies have attempted to measure the effects of forest species diversity on tick abundance and tick-borne pathogen prevalence (but see Ruyts et al., 2016; Ehrmann et al., 2017, 2018) and, to our knowledge, none has isolated the effect of tree species richness from other environmental drivers in an experimental setting.
In this study, we used a long-term experiment where both tree diversity and water supply are manipulated to test their interactive effects on I. ricinus larvae and nymphs abundance and on associated pathogens. We sampled ticks in forest plots ranging from one (monoculture) to three tree species mixtures, in both irrigated and non-irrigated conditions. More particularly, we tested the following hypotheses:
(1) Tick abundance and proportion of infected tick nymphs are lower in mixed than in pure forest plots, due to stand characteristics associated with “habitat-dilution” effects.
(2) Effects of tree species diversity on ticks are principally mediated by changes in understorey density and composition, being the main habitat for larvae and nymphs.
(3) The negative effects of drought (in non-irrigated plots) on ticks are mitigated by forest diversity, due to higher crown space filling and shading of the understorey.
Although we were unable to sample vertebrate hosts, our goal was to quantify the direct and indirect (microclimate, understorey) drivers of the effects of overstorey tree diversity on the risk of disease transmission by ticks (Figure 1).
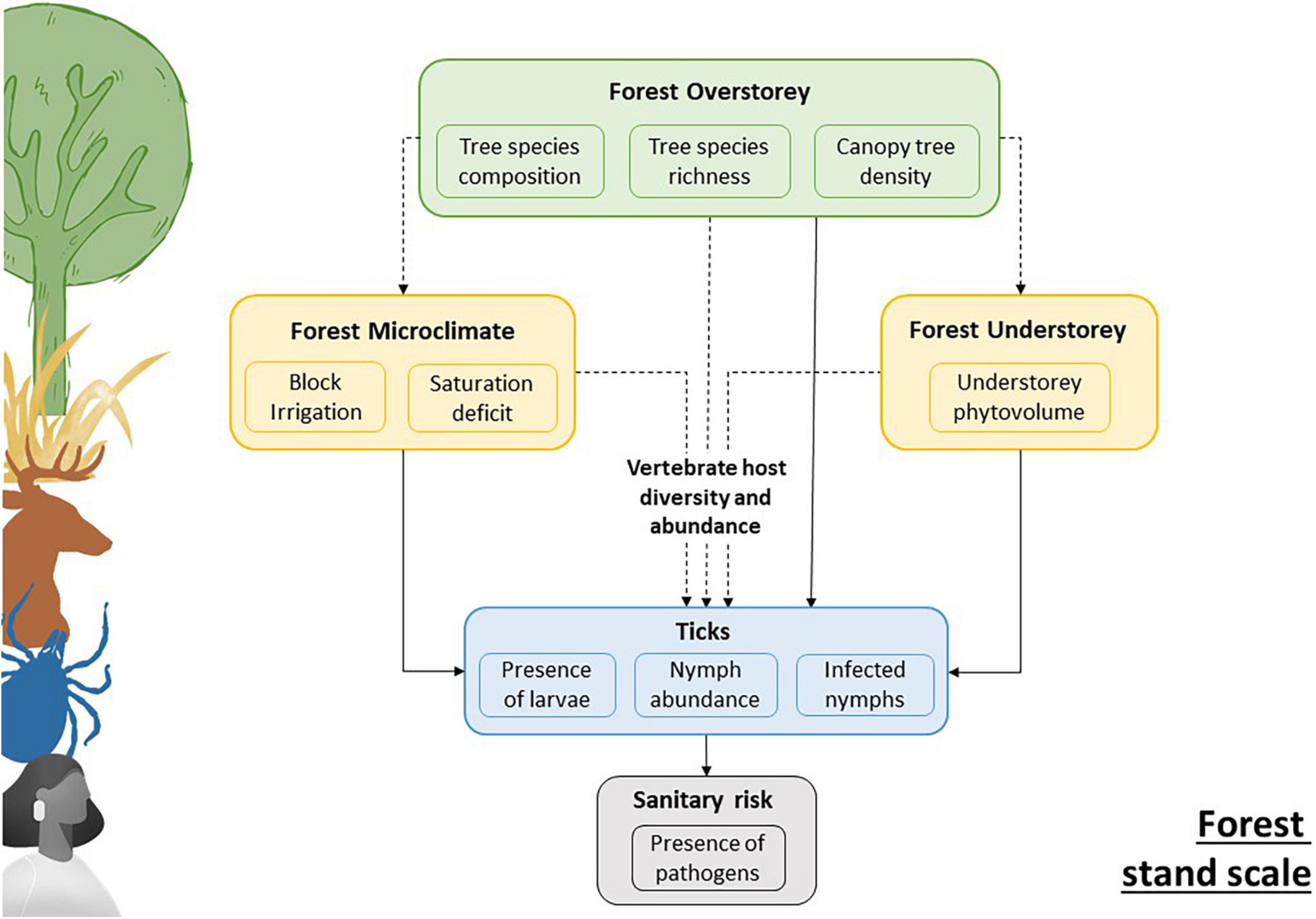
Figure 1. Explanatory diagram showing our study hypotheses at the forest stand level. The biophysical and structural characteristics of the overstorey influence the ticks and associated pathogens through an influence on the within-stand microclimate, the understorey, and the host community in the environment. Diversifying host communities may result in a dilution of competent hosts in the environment and thus affects the prevalence of tick-borne diseases. Dashed arrows represent effects not tested in our study.
Materials and Methods
Study Site
The study was conducted in the tree diversity experiment ORPHEE, located in southwestern France, in the Landes de Gascogne forest. The region is characterized by a mild oceanic climate, with mean annual temperature of 12.5 °C and mean total precipitations of ca. 950 mm. The ORPHEE experiment, which belongs to the global network of tree diversity experiments TreeDivNet (Verheyen et al., 2016; Paquette et al., 2018), was established in 2008 on a 12 ha clearcut of maritime pine stands, on a sandy podzolic soil. It is composed of eight blocks of 32 randomly distributed plots corresponding to all the possible combinations of one to five tree species. Five native tree species were planted in monocultures (European birch: Betula pendula, ROTH; Pedunculate oak: Quercus robur, L.; Pyrenean oak: Quercus pyrenaica, WILLD.; Holm oak: Quercus ilex, L.; and Maritime pine: Pinus pinaster, AIT.) as well as all combinations with two, three, four and five tree species. Each plot is composed of 10 × 10 trees, planted 2 meters apart, resulting in 100 trees per plot, with a plot area of 400 m2. Tree species are planted according to a systematic alternate pattern (see details in the study by Castagneyrol et al., 2013; Damien et al., 2016). Four out of eight blocks are irrigated since 2015. Blocks are sprinkled at night with 42 m3 of water (i.e., 3 mm precipitation, block area of 1.28 ha), daily from May to October, which significantly increases water availability as compared to non-irrigated blocks (Maxwell et al., 2020). The site has been fenced, which may have reduced the presence or passage of large mammal in the plots.
In our study, we focused on 14 different plots with the following four tree species: Q. robur, Q. ilex, P. pinaster, and B. pendula, planted in monocultures (n = 4), two-species mixtures (n = 6), and three-species tree mixtures (n = 4). All plot compositions were replicated eight times, four in irrigated blocks and four in non-irrigated blocks, for a total of 112 plots.
Tick Sampling and Identification
Free-living ticks, being larvae, nymphs or adults, were collected in June and July 2020 on days without rain during the peak of tick activity season, using the dragflag technique (Macleod, 1932; Vassallo et al., 2000). The total number of ticks collected along four parallel 20 meter long transects in each plot (80 m2 sampled area) were denoted N{i,j,k} where i ∈ [1;14] represents the plot composition, j ∈ [1;8] represents the replication of plot composition and k ∈ [1;4] represents the kth parallel long transect. To take into account the effect of different understorey diversity or composition on the sampling rate (Bord et al., 2014), i.e., the proportion of ticks collected by the drag in the questing tick population, each transect was passed four times, corresponding to two return trips, with a 1 m × 1 m white flannel cloth of dragged on the ground at a constant speed (walking pace). Ticks were counted after each successive sampling, stage determined and kept in a 70% ethanol tube at the end of each pass by transect. Abundance of adults, nymphs and larvae was confirmed after binocular examination in the laboratory.
Three plots could not be sampled due to the presence of dense gorse (Ulex europaeus) that would have torn the flannel. A total of 109 plots were therefore sampled, corresponding to 436 transects and 1744 sampling passes. Tick species were determined after binocular examination using the systematic key from Estrada-Peña (2017). Too few adults were collected (n = 35 including 29 I. ricinus). We therefore decided to focus only on I. ricinus nymphs and larvae.
Identification of Tick-Borne Pathogens in I. ricinus Nymphs
All I. ricinus collected nymphs (n = 173) were isolated for pathogen identification. We used a microfluidic PCR technique that provides information on the presence of 35 different pathogens of the following genius: Borrelia, Anaplasma, Rickettsia, Francisella, Bartonella, Babesia and Coxiella potentially present in tick nymphs (developed by Michelet et al., 2014).
Tick nymphs were rinsed twice with distilled water. Prior to extraction, ticks were crushed and processed in a homogeniser at 5,500 rpm for 20 s with 100 μl of elution buffer BE, 40 μl of lysis buffer MG and 10 μl of liquid proteinase K and six steel balls. DNA was extracted using a Nucleospin DNA insect kit (Macherey-Nagel, Germany), and DNA from each sample was stored at −20°C in 50 μl of rehydration solution until next step.
DNA pre-amplification reactions was performed with 2.5 μl of PreAmp Master Mix according to the manufacturer’s instructions (Fluidigm, CA, United States). Prior to experiments, primers targeting all pathogens were pooled in equal volumes (200 nM each). The experiment was performed with 1 μL of PreAmp Master Mix, 1.25 μL of pooled primers mix, 1.5 μL distilled water, and 1.25 μL DNA for 5 μL final volume. The pre-amplifications were performed with one cycle at 95°C for two minutes, 14 cycles at 95°C for 15 s and 60°C for four minutes. Pre-amplified DNA was diluted 1:10 and stored at −20°C. Finally, a high-throughput real-time PCR was performed with the BioMark system (Fluidigm, United States) using the method explained in Michelet et al. (2014).
Pathogens detected with BioMark system were confirmed by conventional PCR or nested PCR on several samples presenting low Ct values. Primers targeting different genes or regions than those of the BioMark system were selected from the literature (listed Supplementary Table 1) and PCR were performed with Phusion High fidelity kit (Thermo scientific, MA, United States). The positive samples after gel migration were sent to sequencing at Eurofins (Germany) and assembled using BioEdit software (Ibis Biosciences). An online BLAST (NCBI; National Center for Biotechnology Information) was used to identify the sequenced organisms.
Response Variables
We analyzed four response variables for I. ricinus: larvae presence, nymph abundance and proportion of infected nymphs by Borrelia burgdorferi s.l. and proportion of infected nymphs by Rickettsia. Nymph abundance indicator was the cumulative number of nymphs collected after the four successive samplings where i ∈ [1;14],j ∈ [1;8],k ∈ [1;4] and l ∈ [1;4] corresponding to the plot composition, the repetition number, the parallel long transect and the successive samplings, respectively. Larvae presence variable is binomial: it is set to 1 when at least one larva was found in the plot, and 0 if none was found. As we performed individual PCR’s, the proportion of nymphs infected by each pathogen was tested in the model as a vector including the number of pathogen-positive nymphs and pathogen-negative nymphs in the plot.
Explanatory Variables
We selected three tree layer variables. First, canopy tree density, corresponding to the percentage of canopy individuals in the plot (here pine and/or birch, since oak trees are much smaller in ORPHEE, still being part of the understorey layer; Damien et al., 2016).
Second, tree composition corresponds to different types of tree mixtures (a total of nine type of mixtures). In order to obtain sufficient statistical power to test the effect of plot composition on ticks variables, individual plot compositions were combined into four composition types: (1) Pure pine (including monocultures of pines and mixtures of pines and oaks, which are still small enough to be considered part of the understorey); (2) Pure birch (including monocultures of birches and mixtures of birches and oaks); (3) Mixtures of pine and birch (including mixtures of pines and birches, and mixtures of pines, birches and oaks); (4) Oaks (including monocultures of oaks and mixtures of only oaks) (Table 1).
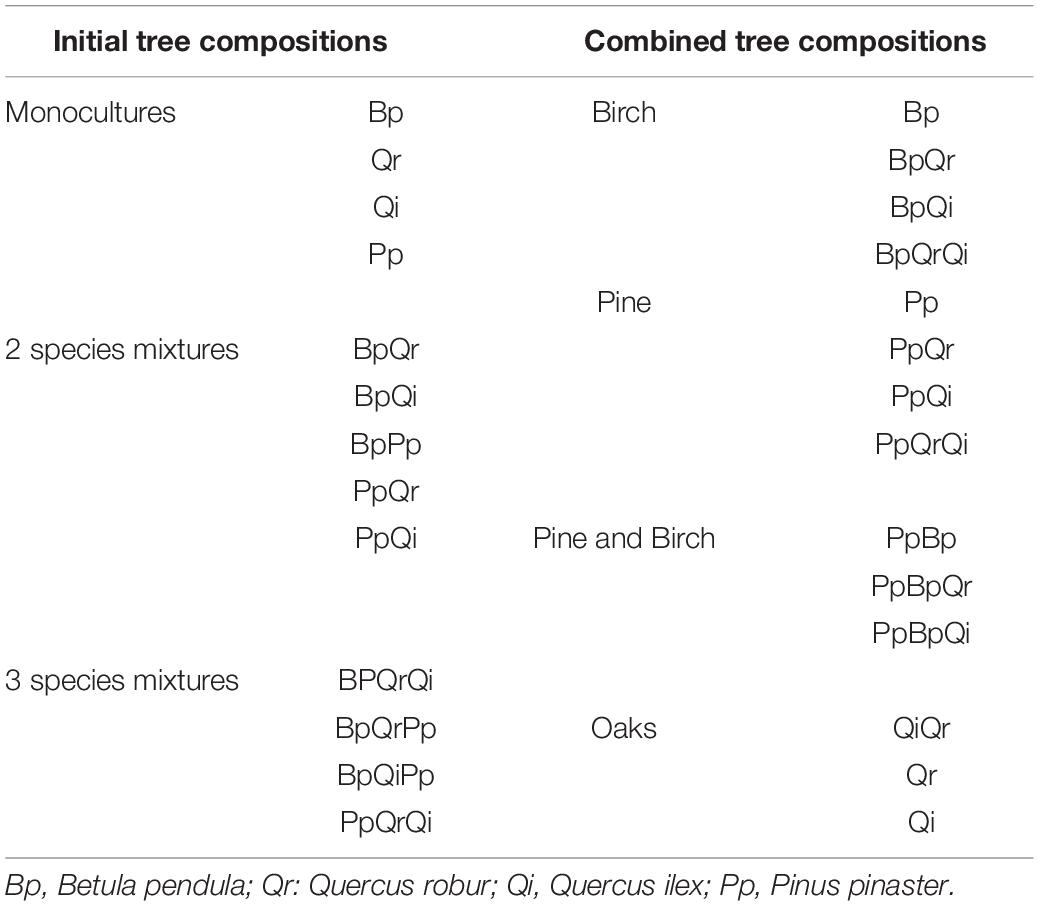
Table 1. Table representing the combination of the different tree compositions chosen for the analyses.
Third, tree species richness corresponds to the number of different tree species in the plot (including the oak species).
Further, we included understorey (understorey phytovolume) and microclimatic variables (presence/absence of irrigation and the saturation deficit of the plot) into the models.
To describe the understorey vegetation, we sampled one 1 m × 1 m quadrat in each transect passed for the ticks (i.e., four quadrats per plot). In each quadrat, we estimated the percentage of bare soil, litter and moss cover, the percentage cover of the main plant types found in ORPHEE (Corcket et al., 2020), i.e., grasses, heath, ferns and shrubs (mainly gorses), and the maximum height of each plant type. We considered not only plants directly rooted in the quadrat but also those whose aerial parts partially or completely covered the surface of the quadrat. The percentage cover of each type was transformed in eight categorical classes: O: 0%; A: 1–5%; B: 6–15%; C: 16–25%; D:26–50%, E:51–75%; F:75–99%; G: 100% [inspired by the Braun-Blanquet approach (Braun-Blanquet, 1964)]. The maximal height of each plant type was measured on the highest individual in the quadrat using a measuring pole. Finally, we aggregated the data at the plot level by averaging across the four quadrats to calculate the understorey phytovolume (cm3) according to equation (1):
Finally, temperature (T) and relative atmospheric humidity (RH) were measured in each plot Ni,j before each sampling (with Kestrel 3500 NV), in order to calculate the Saturation Deficit in millimeters of mercury, according to equation (2) (Vourc’h et al., 2016):
Saturation Deficit, a proxy for tick desiccation potential, is considered a relevant predictor of nymph foraging activity (Gray, 2008).
Statistical Analysis
According to our hypotheses we were interested in testing the effects of tree layer variables, understorey vegetation variables and microclimatic variables on tick response variables. A first sorting of the explanatory variables was done with univariate tests. Variables with a p value < 0.1 were selected as candidates for the full models.
Tick nymphs, larvae and associated pathogens were analyzed separately. For nymph abundance, we built one negative-binomial generalized linear model (glmer.nb) suitable for overdispersed data (Breslow, 1990). We modeled the presence of larvae and proportion of infected nymphs with binomial generalized linear models (glm, binomial family).
Nymph abundance, presence of larvae, and proportion of infected nymphs were modeled as a function of tree composition or canopy tree density, irrigation, tree species richness, understorey phytovolume and their interactions, and saturation deficit as fixed effects. Spatial autocorrelation of plots in the block was accounted for by including Block as random factor.
All continuous predictor variables were scaled and centered prior to modeling to make their coefficients comparable (Schielzeth, 2010). We first built full models fitting the most complex model consistent with the experimental design including all fixed effect factors, interactions and random factors. Then we applied model simplification by sequentially removing one-by-one, the less significant terms to allow a non-singular fit (Barr, 2013). Once optimally simplified, we selected the best fitted model with the lowest AIC. Statistical analyses were performed using the R software version 4.0.5 (R Core Team, 2021).
Results
We collected three tick species in ORPHEE, namely I. ricinus (L., 1758), Ixodes frontalis (PANZER 1795), and Dermacentor reticulatus (FABRICIUS, 1794). However, I. ricinus was by far the most abundant with 1458/1535 larvae and 173/179 nymphs. We therefore focused our analyses on this species. The abundance of I. ricinus nymphs greatly varied between tree composition and the water supply of forest plots (Figure 2).
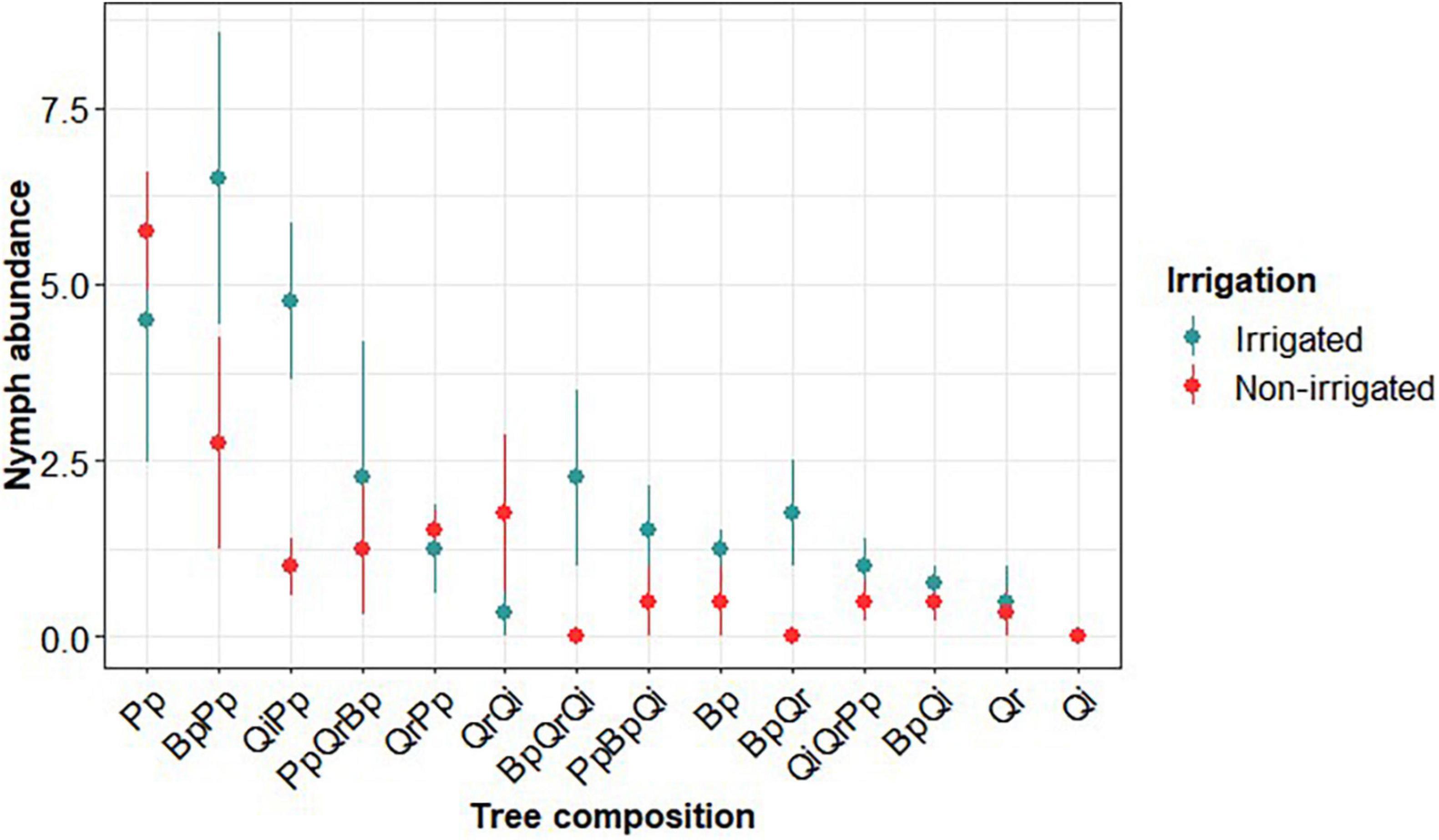
Figure 2. Ixodes ricinus nymph abundance as a function of tree composition in all sampled plots, in irrigated plots (blue) and non-irrigated plots (red). Pp: Pinus pinaster, Bp: Betula pendula, Qr: Quercus robur, Qi: Quercus ilex. Dots and solid lines represents mean ± SE calculated on raw data.
Presence of I. ricinus Larvae Along the Tree Diversity Gradient
Larvae were present in 56 out of the 109 sampled plots and their abundance was on average (±sd, n = 1458) 13.38 ± 0.37 per plot and 26.03 ± 0.46 in the plots where they were present. However, because larval distribution was highly aggregated due to clustering in larval nests, we analyzed only presence/absence data.
The probability of presence of the larvae significantly differed between tree compositions (Figure 3). Presence of larvae was highest in pine plots (pure and mixed with birch), intermediate in birch plots and lowest in oak plots. We also found a significantly positive effect of irrigation, with higher probability of presence in irrigated plots, irrespective of plot composition (i.e., lack of significant interaction) (Table 2 and Figure 3). The probability of larvae presence was significantly higher in irrigated pure and mixed pine plots than in non-irrigated oak plots (Figure 3).
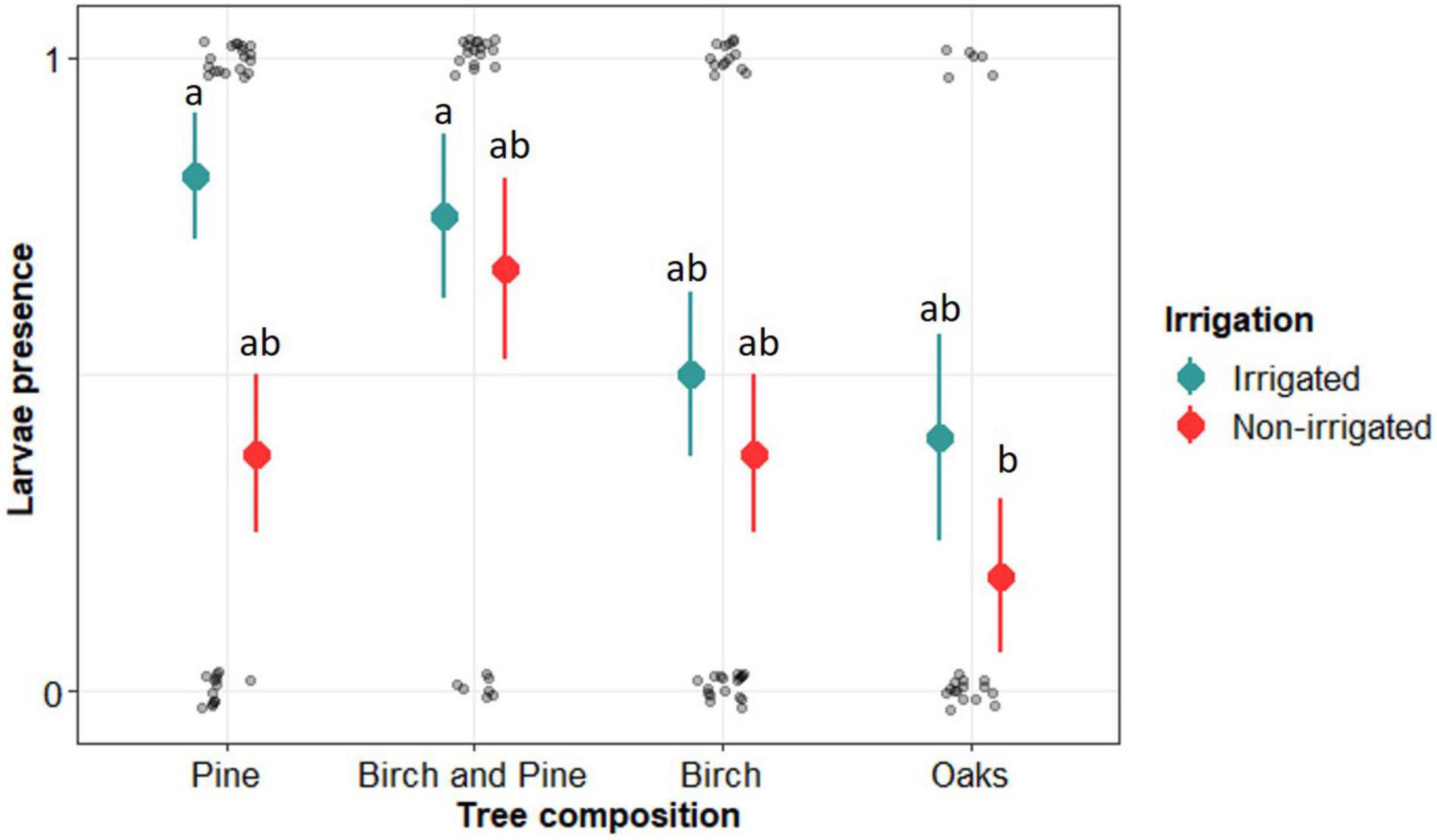
Figure 3. Presence of Ixodes ricinus larva as a function of tree composition and irrigation in the plot. Graph made on raw data. Pine include Pine with or without oaks, Birch includes Birch with or without oaks, Birch and Pine include Birch with pine with or without oaks, and Oaks includes oaks only. Mean values with common letters were not significantly different (Tukey’s post hoc test). Dots and solid lines represents mean ± SE calculated on raw data.
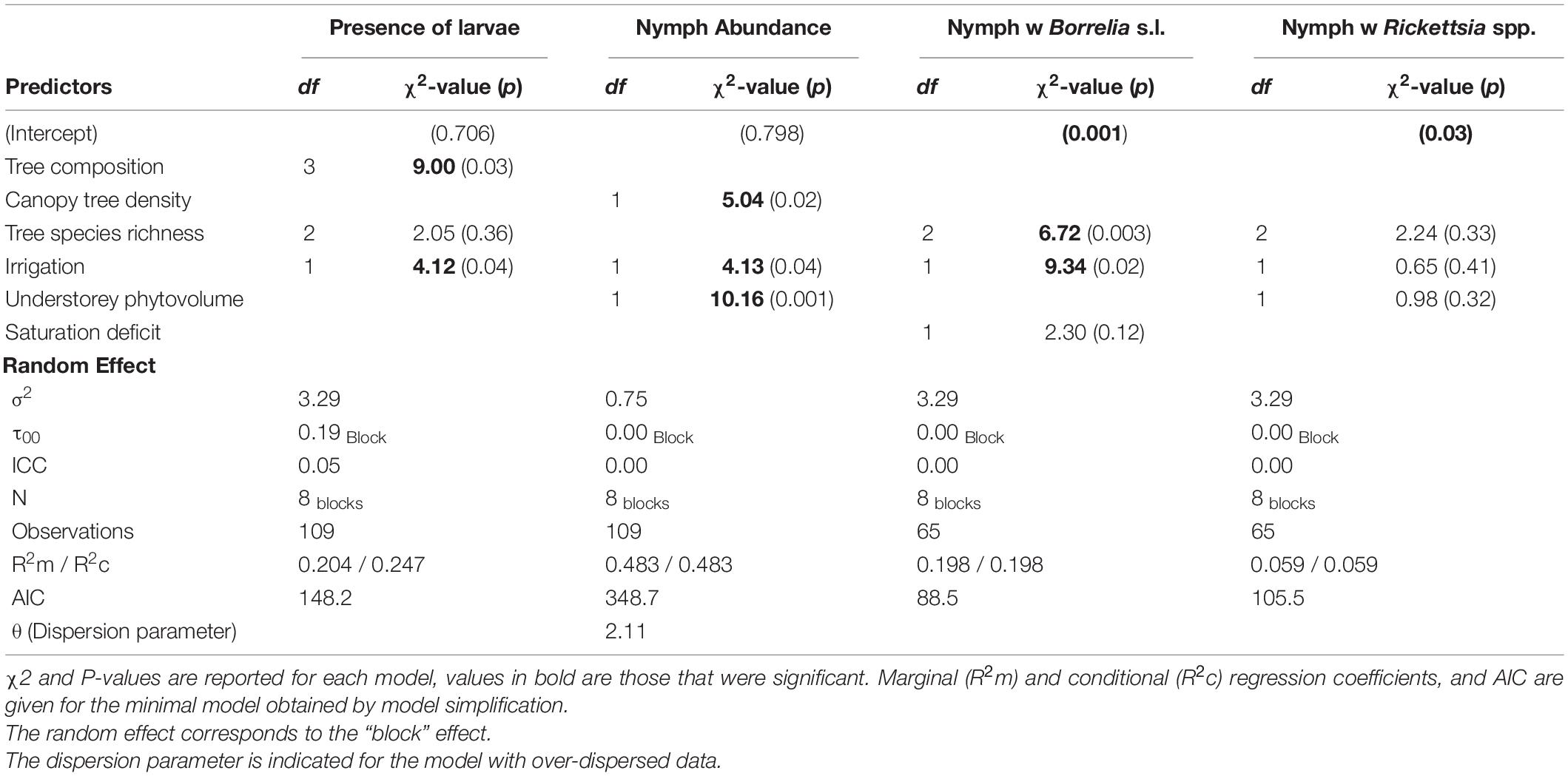
Table 2. Summary of results of final negative binomial GLM models for Ixodes ricinus nymph abundance, binomial GLMMs for presence of larva and binomial logit model for proportion of infected nymph by Borrelia and Rickettsia pathogens.
Abundance of Tick Nymphs
We collect I. ricinus nymphs in 65 out of 109 plots. Nymph abundance was on average (±sd, n = 173) 1.59 ± 0.02, with a median of 1 nymph per plot and 2.66 ± 0.02 in the plots with at least one nymph was present. According to the AIC of compared models, three explanatory variables were retained as significantly influencing the abundance of tick nymphs (Table 2). Nymph abundance increased with the density of canopy trees (Figure 4) and decreased with the phytovolume of understorey vegetation (Figure 5), being significantly higher in irrigated plots, irrespective of their composition (no interaction effect). We did not find any effect of the saturation deficit or tree species richness.
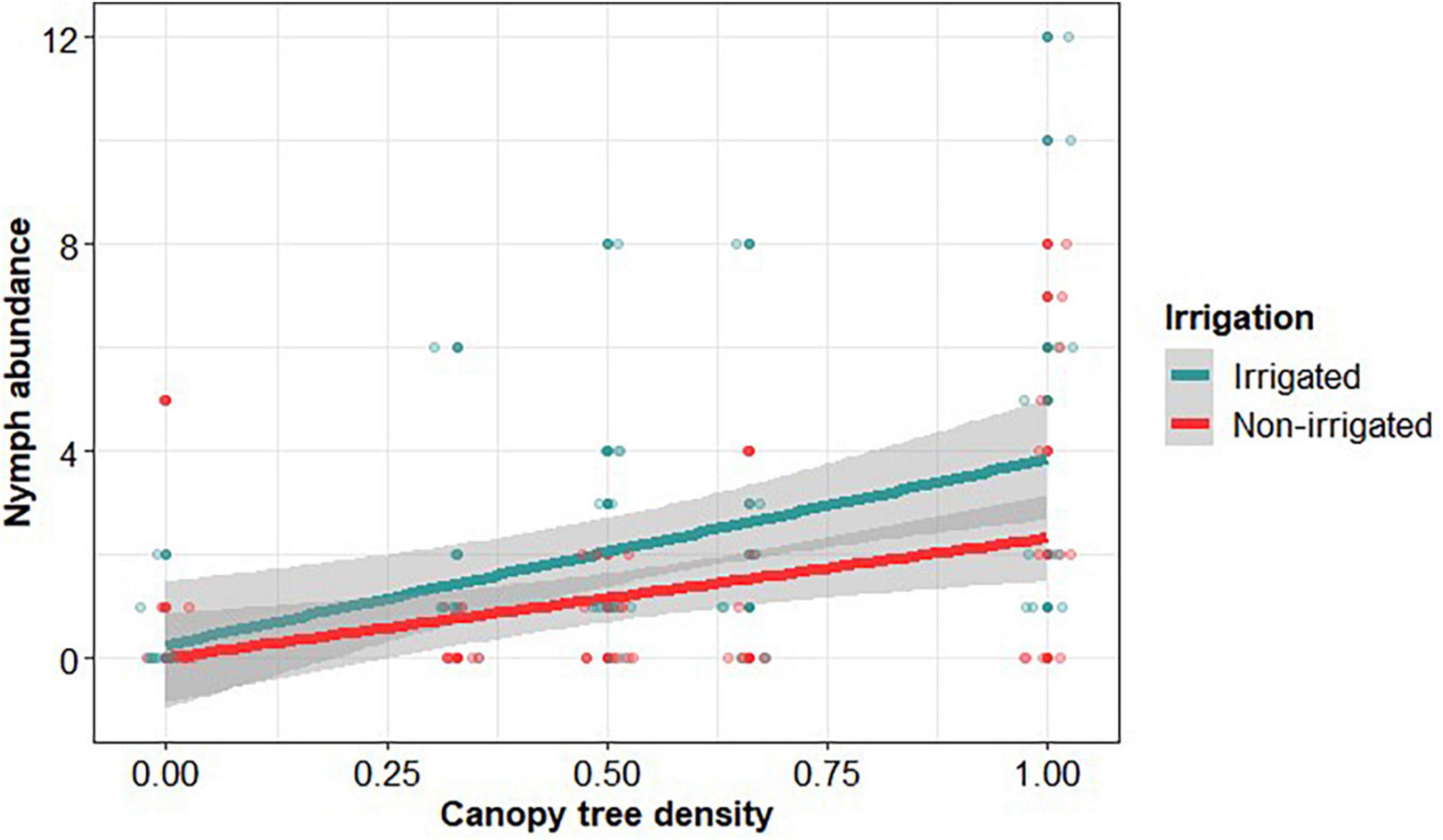
Figure 4. Ixodes ricinus nymph abundance as a function of canopy tree density and irrigation in the plot. Canopy tree density is the ratio of the number of birch and/or pine trees to 100 total trees in the plot. Solid lines and gray shaded area correspond to the regression line and its 95% confidence interval, calculated on raw data.
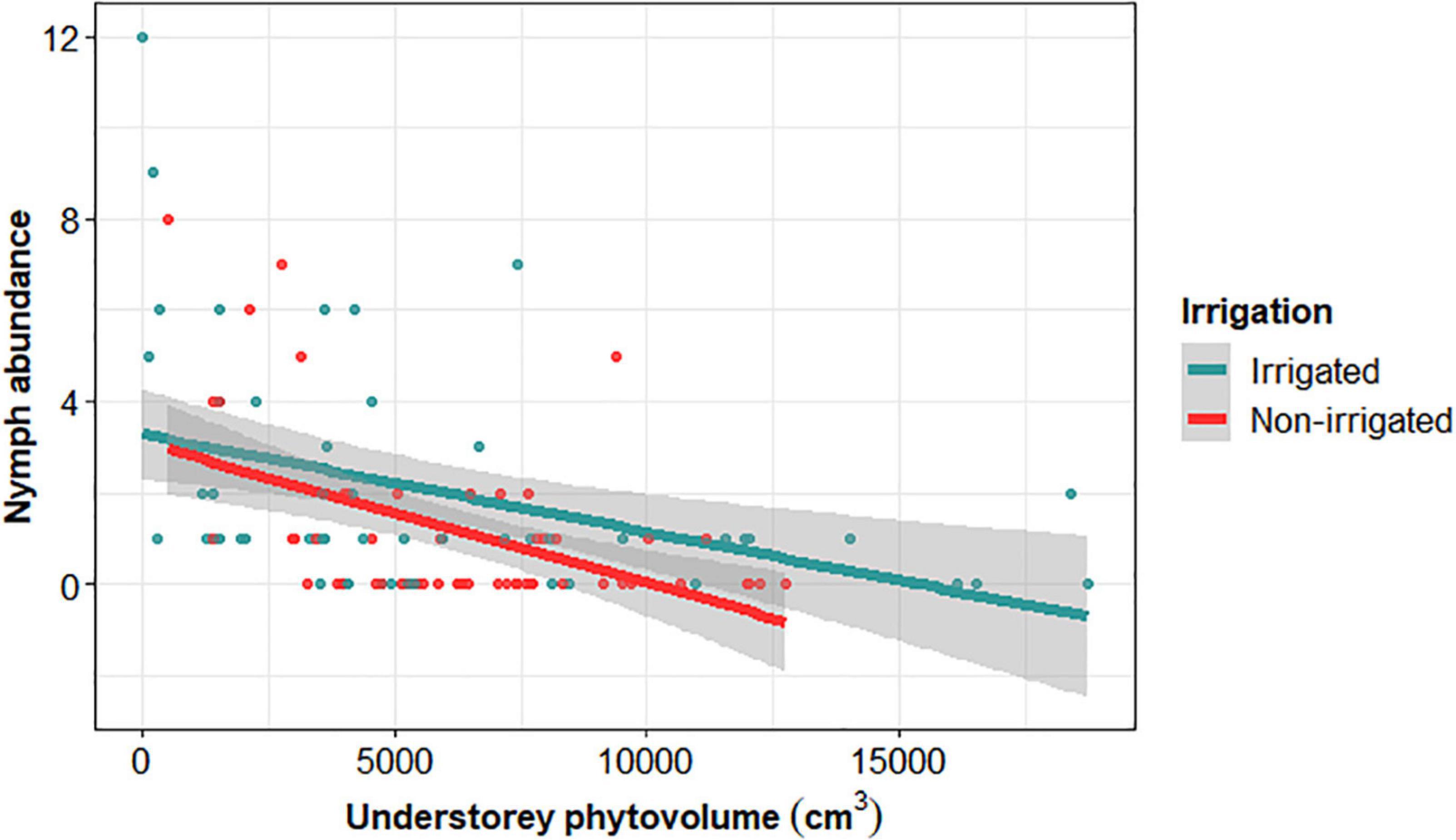
Figure 5. Ixodes ricinus nymph abundance as a function of understorey phytovolume in irrigated (blue) and non-irrigated plots (red). Solid lines and gray shaded area correspond to the regression line and its 95% confidence interval, calculated on raw data.
Presence of Infected Nymphs
The control probe was positive for 172 of 173 collected nymphs, so one DNA extraction failed. Infected nymphs were found in 40 of the 65 plots with nymphs, 72 nymphs were infected with at least one pathogen and 6 nymphs presented a co-infection with several pathogens. Among the 72 infected nymphs, the majority of associated pathogens were from the B. burgdorferi s.l. complex (n = 35), and Rickettsia spp. (n = 32, with 69% of Rickettsia helvetica and 31% of Rickettsia monacensis), the other pathogens were not found frequently enough to test for a significant relationship with covariates.
The Borrelia complex included five different species: 80% of B. lusitaniae, 11% B. afzelii, 3% B. valaisiana, 3% B. garinii and 3% B. burgdorferi s.s. No B. spielmanii, B. bissetti, and no B. miyamotoi (relapsing fever Borrelia) were found. B. lusitaniae, which was the more frequently detected species, is known to be vectored by ticks with reptiles as hosts. Two other reptile-borne pathogens were found: 10 nymphs carried Rickettsia monacensis and one carried Candidatus cryptoplasma. The proportion of nymphs infected by B. burgdorferi s.l. was significantly influenced by the richness of tree species and also by irrigation, with no interaction between these factors (Table 2). The occurrence of nymphs infected by B. burgdorferi s.l. pathogen was lower in irrigated plots and decreased with increasing tree species richness, being highest in non-irrigated pure plots and lowest in irrigated three-species mixtures (Figure 6).
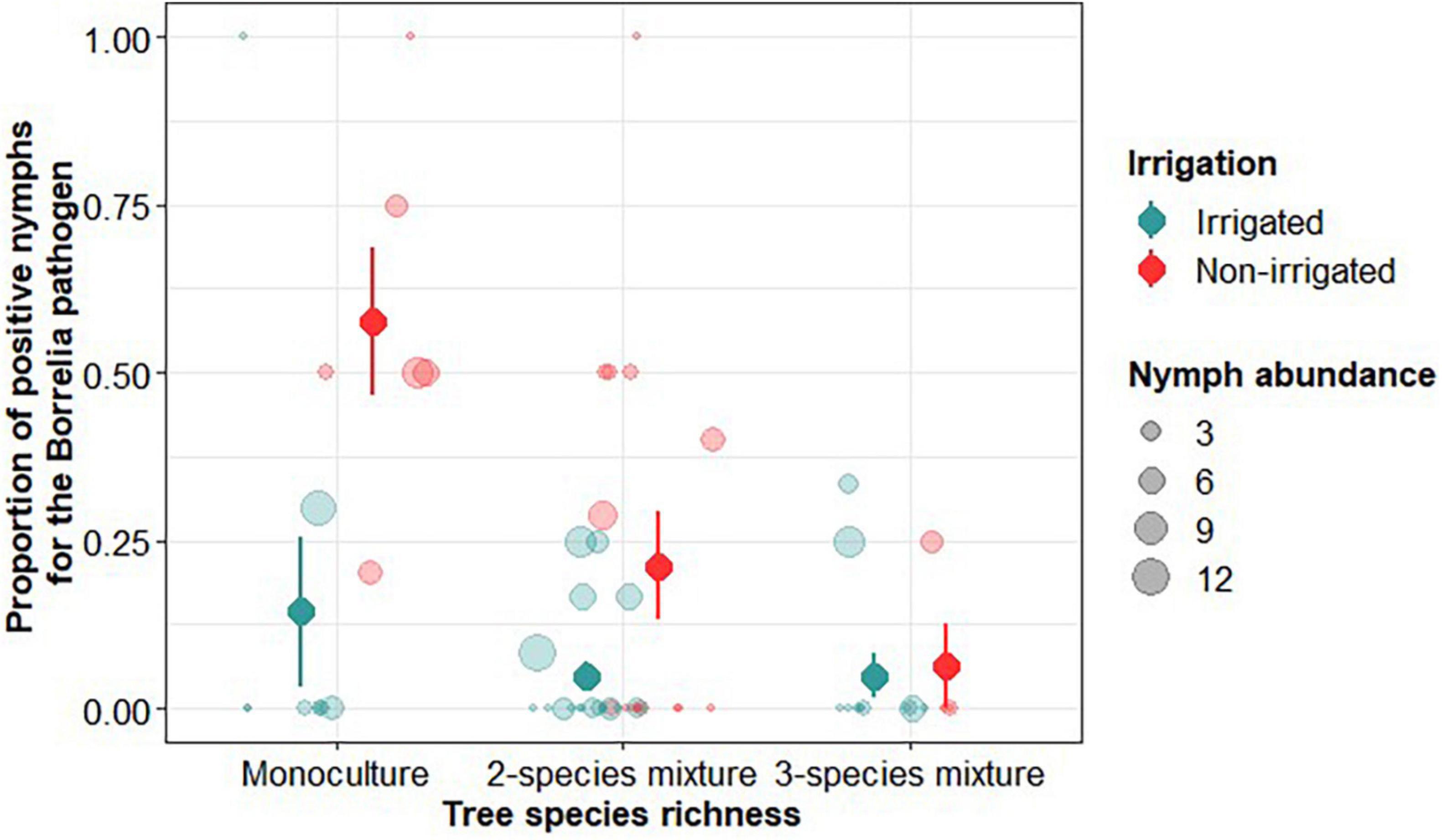
Figure 6. Proportion of positive Ixodes ricinus nymphs for Borrelia burgdorferi s.l. pathogen as a function of tree species richness and irrigation. The size of the dots is proportional to the total number of nymphs on which the proportion of positive nymphs was calculated. Dots and solid lines represents mean ± SE calculated on raw data.
None of our variables were significantly related to the proportion of ticks infected with a Rickettsia pathogen (data not shown).
Discussion
Using a dedicated experiment where both tree diversity and water supply were manipulated, our study demonstrated that the abundance and pathogen load of the most common tick species in Central Europe, Ixodes ricinus, were significantly influenced by tree species composition, canopy density, tree species richness, volume of understorey vegetation, and by irrigation. The main trend was a decrease in presence of larvae, abundance of nymphs and proportion of nymphs carrying Borrelia pathogens in mixed forests where pine trees were substituted with broadleaved trees, irrespective of plot humidity (be it driven by the weather or controlled via irrigation).
Effects of Forest Diversity, Composition and Structure
Our results show that forest diversity and composition can influence tick populations across different stages and their level of infection by pathogens. The probability of presence of larvae was lowest in oak plots, intermediate in birch plots and greatest in pure and mixed pine plots, suggesting a strong tree species identity effect. The abundance of nymphs was significantly affected by canopy tree density, being greater in denser forest plots (e.g., 100% pines, 100% birches or 50% pine–50% birch mixtures), suggesting a stronger effect of forest structure than tree identity. For a tick to be present in an environment, it must (i) have been deposited by a host for nymphal and adult stages or have been laid by a female for the larval stages. (ii) survived in a favorable environment. Overstorey trees can thus have an impact on young tick population by controlling local microclimate (Renaud et al., 2011) and host presence. For example, dense, shaded forests as a result of canopy packing (Jucker et al., 2015; Williams et al., 2017) can be optimal habitats because they provide host animals with food resources while maintaining sufficient moisture for tick survival. A 19-year study in a mature oak-maple mixt forest showed a bottom-up, cascading effect of acorns yield on increasing mouse population levels, resulting in increased abundance of Ixodes scapularis (Say, 1821) nymph and associated Lyme disease risks (Ostfeld et al., 2018), and similar results were recently found with I. ricinus (Bregnard et al., 2020, 2021). High moisture is important for survival of tick larvae and nymphs (Knülle and Devine, 1972; Tukahirwa, 1976; Davey et al., 1991) and Gray (1998) explains that I. ricinus are usually found in environments with 80% atmospheric humidity. The questing activity of tick nymphs occurs higher in the understorey vegetation than that of larvae, so that they can attached to a passing animal, but this presents a greater risk of desiccation during this questing period (Schulze and Jordan, 2005). Denser forests with more trees and with a higher tree canopy packing could therefore limit this risk of desiccation and thus favoring the survival of tick nymphs. This explanation for the positive correlation between nymph abundance and proportion of canopy trees in our experimental plots is also consistent with our result of a positive effect of irrigation.
The presence of larvae and their questing activity are related to the microclimate closer to the ground (Yoder et al., 2006; Ehrmann et al., 2017). Leal et al. (2020) suggest that forest types where the most larvae are found differ between tick species. Indeed, several studies have found greater abundance of I. ricinus larvae in deciduous forests (Walker et al., 2001; Tack et al., 2012; Ruyts et al., 2018). Due to the much slower growth of these species, the plots with only oaks in ORPHEE are currently characterized by small trees of about 2 m height and thus an open canopy. Our results in deciduous plots are therefore to be distinguished from the scientific literature on mature deciduous forests. These conditions on ORPHEE were probably less favorable for the maintenance of suitable humidity in the understorey and for the visit of host animals. In contrast, pine plots characterized by dense and tall trees, may have favored larval survival by providing thick needle litter as a protective barrier against desiccation. Birch plots are in an intermediate position in terms of likelihood of larvae presence, probably because they are composed of high trees but with lower canopy closure and perhaps lower quality litter for ticks.
The proportion of nymphs vectoring Borrelia pathogens significantly decreased with increasing tree species richness, with the highest frequency in pure pine plots and the lowest in mixtures associating pine, birch and oaks. Our results thus support the finding by Ehrmann et al. (2018) that a reduction in the prevalence of tick-borne diseases is associated with greater forest diversity, confirming the beneficial role of tree species mixing for natural risk reduction (Jactel et al., 2017). Ruyts et al. (2016) did not find a tree species diversity effect on the prevalence of tick-borne pathogens but they found that the risk of Lyme borreliosis differed between different forest compositions. They showed that B. afzelii was more often found on ticks in pine plots while B. garinii and B. burgdorferi s.s. were more prevalent in oak plots. A negative effect of tree species richness on infected nymphs could be explained by an increase in ecological niches for host species, resulting in a change in the ratio of competent to incompetent reservoir hosts, with diluting effects on Borrelia (Levi et al., 2016; Ehrmann et al., 2018). Empirical multitaxonomic studies (e.g., Ampoorter et al., 2020) and meta-analyses (e.g., Stemmelen et al., 2021) have indeed shown greater faunal diversity in mixed forests than in pure forests. Additional research is now needed to inventory potential tick host animals in pure and mixed forests and better assess their level of competence for tick-borne pathogens.
Ruyts et al. (2016) suggested that the dilution effect would depend on the species of B. burgdorferi s.l. In our study, 80% of B. burgdorferi s.l. were in fact B. lusitaniae, which appears to be carried and multiplied by lizards in several countries around the Mediterranean rim (Michelis et al., 2000; Younsi et al., 2001; Sarih et al., 2003; Tomassone et al., 2017). As ectothermic animals, lizards often prefer environments that allow them significant sun exposure for active thermoregulation (Smith and Ballinger, 2001). This may explain the negative effect of irrigation on the proportion of nymphs infected by Borrelia burgdorferi s.l. pathogens, either directly by increasing soil humidity or indirectly by increasing the density and thus shading of understorey vegetation (Corcket et al., 2020). We do not have yet direct data from the ORPHEE experiment but surveys in the Aquitaine region reported the presence of three lizard species in forests: the common wall lizard [Podarcis muralis (Laurenti, 1768)], the viviparous lizard [Zootoca vivipara (Lichtentein, 1823)] and the western green lizard (Lacerta bilineata, Daudin, 1802) (Berroneau, 2015). All are known as I. ricinus potential hosts (Scali et al., 2001; Richter and Matuschka, 2006; Wu et al., 2019; Gwiazdowicz et al., 2020), and two of them (common wall and western green lizards) have already been involved in the transmission of B. lusitaniae in I. ricinus populations (Richter and Matuschka, 2006; Mendoza-Roldan et al., 2021). To date, no studies on the dilution effect of lizard hosts have been tested on B. lusitaniae; it would therefore be interesting to inventory them along a gradient of tree diversity and also to classify them according to their level of competence.
Finally, no variable explained the proportion of I. ricinus nymphs infected with Rickettsia spp. pathogens. The ecological drivers of distribution and prevalence of Rickettsia spp. remain poorly understood in the literature and even less the effect of forest composition or diversity.
Effects of Understorey
Our study showed a distinct negative effect of the phytovolume of understorey vegetation on nymph abundance, independently from canopy diversity or composition. Several studies found opposite results with the abundance of nymphs positively correlated with high density of understorey vegetation, a condition considered favorable for visit by potential tick host animals (Tack et al., 2013; Ostfeld et al., 2018). However, other studies found a negative relationship between high density of understorey vegetation and nymph abundance, suggesting on the contrary that these environments would be less visited by hosts or would not be associated with good tick survival rates (Stein et al., 2008). Similarly, a denser understorey vegetation can also dilute tick infection prevalence (Ehrmann et al., 2018). A dense understorey can provide a suitable habitat for ticks but also for small mammals; this increases the likelihood of ticks finding a host, but at the same time decreases the likelihood that many ticks can make contact with the same host species. Thus, a lower average tick load and a lower co-feeding probability on hosts could result in dilution of Borrelia prevalence.
Bord et al. (2014) also highlighted that the dragflag technique, although very effective for sampling free-living ticks in forest, is less effective when the understorey is too dense, thus resulting in underestimation of tick abundance. In our study, the negative effect of understorey phytovolume on nymph abundance is, however, still present at low levels of understorey phytovolume. In addition, the phytovolume does not take into account plant species identity, an important factor for host attraction. In ORPHEE, the main understorey plant species were Molinia caerulae, Ulex europaeus, Pteridium aquilinum and some Ericaceae spp. (Corcket et al., 2020), which are not or only little palatable for many herbivorous hosts and whose density or height may limit the passage of large ones.
Medical Relevance and Practical Perspectives
All Rickettsia and Borrelia pathogens detected in our study are transmissible to humans and of medical importance (Steere et al., 2004; Fournier and Raoult, 2009). R. helvetica and R. monacensis are belonging to the spotted fever group (Merhej and Raoult, 2011) and B. afzelii, B. valaisiana, B. garinii and B. burgdorferi s.s. are bacteria involved in the Lyme disease in humans (Cutler et al., 2017). Although the transmission of B. lusitaniae to humans has been demonstrated, the link with Lyme disease has yet to be investigated (Stanek and Reiter, 2011). Previous studies found B. lusitaniae associated with medical symptoms in humans after a tick bite (Collares-Pereira et al., 2004; Franca et al., 2005; Lopes de Carvalho et al., 2008). In our study, the proportion of infected nymphs did not correlate with nymph abundance, which confirms that it is important to distinguish tick abundance vs. tick-borne pathogen dynamics and to study pathogens at the species level, due to their host and home range specificity.
Conclusion
Our results suggest that various habitat characteristics, including tree overstorey composition, structure and diversity, as well as understorey density, can affect tick abundance and pathogen prevalence. They thus support the notion that habitats can have either “diluting” or “amplifying” characteristics for tick-borne diseases with direct relevance for human health (Ehrmann et al., 2018). However, more research is needed to decipher the underlying mechanisms and to determine the optimal characteristics of heterogeneous forests to inform managers. Still, our results support the generally higher risk to get infected by tick-borne diseases in shady, humid and dense forests during the tick activity period. Nevertheless, evidence is accumulating that “dilution habitats” are characterized by high structural, functional or compositional diversity of the plant community, with propagating effects on host species composition and abundance, and hence on host dilution. If further supported by additional research, this perspective would open possibilities for an active tick-control reducing the survival of ticks and/or decreasing the proportion of infected ticks through forest management that supports the different facets of forest biodiversity.
Data Availability Statement
The original contributions presented in the study are included in the article/Supplementary Material, further inquiries can be directed to the corresponding author/s.
Author Contributions
AB and HJ designed the study, collected the data, and wrote the first draft. SM and CG performed the molecular analysis and formatted the related data. AB computed the data and run the analysis with SB and JD. All authors contributed substantially to subsequent revisions.
Funding
This study was funded by the project BiodivERsA Dr. Forest and by ANR (Grant, ANR–19–EBI3–0002–07).
Conflict of Interest
The authors declare that the research was conducted in the absence of any commercial or financial relationships that could be construed as a potential conflict of interest.
Publisher’s Note
All claims expressed in this article are solely those of the authors and do not necessarily represent those of their affiliated organizations, or those of the publisher, the editors and the reviewers. Any product that may be evaluated in this article, or claim that may be made by its manufacturer, is not guaranteed or endorsed by the publisher.
Acknowledgments
We thank Damien Bouic and Alex Stemmelen for their help in collecting samples.
Supplementary Material
The Supplementary Material for this article can be found online at: https://www.frontiersin.org/articles/10.3389/fevo.2022.891908/full#supplementary-material
References
Allan, B. F., Dutra, H. P., Goessling, L. S., Barnett, K., Chase, J. M., Marquis, R. J., et al. (2010). Invasive honeysuckle eradication reduces tick-borne disease risk by altering host dynamics. PNAS 107, 18523–18527. doi: 10.1073/pnas.1008362107
Ampoorter, E., Baeten, L., Koricheva, J., Vanhellemont, M., and Verheyen, K. (2014). Do diverse overstoreys induce diverse understoreys? Lessons learnt from an experimental–observational platform in Finland. For. Ecol. Manag. 318, 206–215. doi: 10.1016/j.foreco.2014.01.030
Ampoorter, E., Barbaro, L., Jactel, H., Baeten, L., Boberg, J., Carnol, M., et al. (2020). Tree diversity is key for promoting the diversity and abundance of forest-associated taxa in Europe. Oikos 129, 133–146. doi: 10.1111/oik.06290
Barr, D. (2013). Random effects structure for testing interactions in linear mixed-effects models. Front. Psychol. 4:328. doi: 10.3389/fpsyg.2013.00328
Berroneau, M. (2015). Guide des Amphibiens et Reptiles d’Aquitaine, Ed. C. Nature. Le Haillan: Association Cistude Nature, 181.
Bord, S., Druilhet, P., Gasqui, P., Abrial, D., and Vourc’h, G. (2014). Bayesian estimation of abundance based on removal sampling under weak assumption of closed population with catchability depending on environmental conditions. Application to tick abundance. Ecol. Model. 274, 72–79. doi: 10.1016/j.ecolmodel.2013.12.004
Boyard, C., Barnouin, J., Gasqui, P., and Vourc’h, G. (2007). Local environmental factors characterizing Ixodes ricinus nymph abundance in grazed permanent pastures for cattle. Parasitology 134, 987–994. doi: 10.1017/S0031182007002351
Braun-Blanquet, J. (1964). “Gesellschaftsgeschichte (Synchronologie),” in Pflanzensoziologie: Grundzüge der Vegetationskunde, ed. J. Braun-Blanquet (Vienna: Springer), 704–719. doi: 10.1007/978-3-7091-8110-2_7
Bregnard, C., Rais, O., and Voordouw, M. J. (2020). Climate and tree seed production predict the abundance of the European Lyme disease vector over a 15-year period. Parasit. Vect. 13:408. doi: 10.1186/s13071-020-04291-z
Bregnard, C., Rais, O., and Voordouw, M. J. (2021). Masting by beech trees predicts the risk of Lyme disease. Parasit. Vect. 14:168. doi: 10.1186/s13071-021-04646-0
Breslow, N. (1990). Tests of hypotheses in overdispersed poisson regression and other Quasi-Likelihood Models. J. Am. Stat. Assoc. 85, 565–571. doi: 10.1080/01621459.1990.10476236
Brockerhoff, E. G., Barbaro, L., Castagneyrol, B., Forrester, D. I., Gardiner, B., González-Olabarria, J. R., et al. (2017). Forest biodiversity, ecosystem functioning and the provision of ecosystem services. Biodivers. Conserv. 26, 3005–3035. doi: 10.1007/s10531-017-1453-2
Burri, C., Schumann, O., Schumann, C., and Gern, L. (2014). Are Apodemus spp. mice and Myodes glareolus reservoirs for Borrelia miyamotoi, Candidatus Neoehrlichia mikurensis, Rickettsia helvetica, R. monacensis and Anaplasma phagocytophilum? Ticks Tick Borne Dis. 5, 245–251. doi: 10.1016/j.ttbdis.2013.11.007
Castagneyrol, B., Giffard, B., Péré, C., and Jactel, H. (2013). Plant apparency, an overlooked driver of associational resistance to insect herbivory. J. Ecol. 101, 418–429. doi: 10.1111/1365-2745.12055
Collares-Pereira, M., Couceiro, S., Franca, I., Kurtenbach, K., Schäfer, S. M., Vitorino, L., et al. (2004). First isolation of borrelia lusitaniae from a human patient. J. Clin. Microbiol. 42, 1316–1318. doi: 10.1128/JCM.42.3.1316-1318.2004
Corcket, E., Alard, D., van Halder, I., Jactel, H., Garrido Diaz, B., Reuzeau, E., et al. (2020). Canopy composition and drought shape understorey plant assemblages in a young tree diversity experiment. J. Veg. Sci. 31, 803–816. doi: 10.1111/jvs.12903
Cutler, S. J., Ruzic-Sabljic, E., and Potkonjak, A. (2017). Emerging borreliae – Expanding beyond Lyme borreliosis. Mol. Cell. Probes 31, 22–27. doi: 10.1016/j.mcp.2016.08.003
Damien, M., Jactel, H., Meredieu, C., Régolini, M., van Halder, I., and Castagneyrol, B. (2016). Pest damage in mixed forests: disentangling the effects of neighbor identity, host density and host apparency at different spatial scales. For. Ecol. Manag. 378, 103–110. doi: 10.1016/j.foreco.2016.07.025
Davey, R. B., Cooksey, L. M., and Despins, J. L. (1991). Survival of larvae of Boophilus annulatus, Boophilus microplus, and Boophilus hybrids (acari: ixodidae) in different temperature and humidity regimes in the laboratory. Vet. Parasitol. 40, 305–313. doi: 10.1016/0304-4017(91)90110-H
De Frenne, P., Zellweger, F., Rodríguez-Sánchez, F., Scheffers, B. R., Hylander, K., Luoto, M., et al. (2019). Global buffering of temperatures under forest canopies. Nat. Ecol. Evol. 3, 744–749. doi: 10.1038/s41559-019-0842-1
de la Fuente, J. (2008). Overview: ticks as vectors of pathogens that cause disease in humans and animals. Front. Biosci. 13, 6938–6946. doi: 10.2741/3200
Diuk-Wasser, M. A., Hoen, A. G., Cislo, P., Brinkerhoff, R., Hamer, S. A., Rowland, M., et al. (2012). Human Risk of Infection with Borrelia burgdorferi, the Lyme Disease Agent, in Eastern United States. Am. J. Trop. Med. Hyg. 86, 320–327. doi: 10.4269/ajtmh.2012.11-0395
Diuk-Wasser, M. A., VanAcker, M. C., and Fernandez, M. P. (2021). Impact of land use changes and habitat fragmentation on the eco-epidemiology of tick-borne diseases. J. Med. Entomol. 58, 1546–1564. doi: 10.1093/jme/tjaa209
Dunn, R. R. (2010). Global mapping of ecosystem disservices: the unspoken reality that nature sometimes kills us. Biotropica 42, 555–557. doi: 10.1111/j.1744-7429.2010.00698.x
Ehrmann, S., Liira, J., Gärtner, S., Hansen, K., Brunet, J., Cousins, S. A. O., et al. (2017). Environmental drivers of Ixodes ricinus abundance in forest fragments of rural European landscapes. BMC Ecol. 17:31. doi: 10.1186/s12898-017-0141-0
Ehrmann, S., Ruyts, S. C., Scherer-Lorenzen, M., Bauhus, J., Brunet, J., Cousins, S. A. O., et al. (2018). Habitat properties are key drivers of Borrelia burgdorferi (s.l.) prevalence in Ixodes ricinus populations of deciduous forest fragments. Parasites Vect. 11:23. doi: 10.1186/s13071-017-2590-x
Estrada-Peña, A. (2001). Distribution, abundance, and habitat preferences of Ixodes ricinus (Acari: Ixodidae) in Northern Spain. J. Med. Entomol. 38, 361–370. doi: 10.1603/0022-2585-38.3.361
Estrada-Peña, A. (2008). Climate, niche, ticks, and models: what they are and how we should interpret them. Parasitol. Res. 103, 87–95. doi: 10.1007/s00436-008-1056-7
Everard, M., Johnston, P., Santillo, D., and Staddon, C. (2020). The role of ecosystems in mitigation and management of Covid-19 and other zoonoses. Environ. Sci. Policy 111, 7–17. doi: 10.1016/j.envsci.2020.05.017
Fischhoff, I. R., Keesing, F., and Ostfeld, R. S. (2019). Risk factors for bites and diseases associated with black-legged ticks: a meta-analysis. Am. J. Epidemiol. 188, 1742–1750. doi: 10.1093/aje/kwz130
Fournier, P.-E., and Raoult, D. (2009). Current knowledge on phylogeny and taxonomy of Rickettsia spp. Ann. N.Y. Acad. Sci. 1166, 1–11. doi: 10.1111/j.1749-6632.2009.04528.x
Franca, I., Santos, L., Mesquita, T., Collares-Pereira, M., Baptista, S., Vieira, L., et al. (2005). Lyme borreliosis in Portugal caused by Borrelia lusitaniae? Clinical report on the first patient with a positive skin isolate. Wien Klin Wochenschr 117, 429–432. doi: 10.1007/s00508-005-0386-z
Gazol, A., and Ibáñez, R. (2009). Different response to environmental factors and spatial variables of two attributes (cover and diversity) of the understorey layers. For. Ecol. Manag. 258, 1267–1274. doi: 10.1016/j.foreco.2009.06.024
Gray, J. S. (1998). Review the ecology of ticks transmitting Lyme borreliosis. Exp. Appl. Acarol. 22, 249–258. doi: 10.1023/A:1006070416135
Gray, J. S. (2008). Ixodes ricinus seasonal activity: implications of global warming indicated by revisiting tick and weather data. Int. J. Med. Microbiol. 298, 19–24. doi: 10.1016/j.ijmm.2007.09.005
Gwiazdowicz, D. J., Gdula Anna, K., Kurczewski, R., and Zawieja, B. (2020). Factors influencing the level of infestation of Ixodes ricinus (Acari: Ixodidae) on Lacerta agilis and Zootoca vivipara (Squamata: Lacertidae). Acarologia 60, 390–397. doi: 10.24349/acarologia/20204372
Halliday, F. W., Rohr, J. R., and Laine, A.-L. (2020). Biodiversity loss underlies the dilution effect of biodiversity. Ecol. Lett. 23, 1611–1622. doi: 10.1111/ele.13590
Harmon, M. E., Fasth, B., Woodall, C. W., and Sexton, J. (2013). Carbon concentration of standing and downed woody detritus: effects of tree taxa, decay class, position, and tissue type. For. Ecol. Manag. 291, 259–267. doi: 10.1016/j.foreco.2012.11.046
Hofmeester, T. R., Jansen, P. A., Wijnen, H. J., Coipan, E. C., Fonville, M., Prins, H. H. T., et al. (2017). Cascading effects of predator activity on tick-borne disease risk. Proc. R. Soc. B Biol. Sci. 284:20170453. doi: 10.1098/rspb.2017.0453
Jactel, H., Bauhus, J., Boberg, J., Bonal, D., Castagneyrol, B., Gardiner, B., et al. (2017). Tree diversity drives forest stand resistance to natural disturbances. Curr. For. Rep. 3, 223–243. doi: 10.1007/s40725-017-0064-1
Jactel, H., Gritti, E. S., Drössler, L., Forrester, D. I., Mason, W. L., Morin, X., et al. (2018). Positive biodiversity–productivity relationships in forests: climate matters. Biol. Lett. 14:20170747. doi: 10.1098/rsbl.2017.0747
Jactel, H., Moreira, X., and Castagneyrol, B. (2021). Tree diversity and forest resistance to insect pests: patterns. Mechan. Prospects Annu. Rev. Entomol. 66, 277–296. doi: 10.1146/annurev-ento-041720-075234
Jones, B. A., Grace, D., Kock, R., Alonso, S., Rushton, J., Said, M. Y., et al. (2013). Zoonosis emergence linked to agricultural intensification and environmental change. PNAS 110, 8399–8404. doi: 10.1073/pnas.1208059110
Jucker, T., Bouriaud, O., and Coomes, D. A. (2015). Crown plasticity enables trees to optimize canopy packing in mixed-species forests. Funct. Ecol. 29, 1078–1086. doi: 10.1111/1365-2435.12428
Keesing, F., and Ostfeld, R. S. (2021). Impacts of biodiversity and biodiversity loss on zoonotic diseases. Proc. Natl. Acad. Sci. U.S.A. 118:e2023540118. doi: 10.1073/pnas.2023540118
Kilpatrick, A. M., and Randolph, S. E. (2012). Drivers, dynamics, and control of emerging vector-borne zoonotic diseases. Lancet 380, 1946–1955. doi: 10.1016/S0140-6736(12)61151-9
Knülle, W., and Devine, T. L. (1972). Evidence for active and passive components of sorption of atmospheric water vapour by larvae of the tick Dermacentor variabilis. J. Insect Physiol. 18, 1653–1664. doi: 10.1016/0022-1910(72)90095-9
Lange, M., Eisenhauer, N., Sierra, C. A., Bessler, H., Engels, C., Griffiths, R. I., et al. (2015). Plant diversity increases soil microbial activity and soil carbon storage. Nat. Commun. 6:6707. doi: 10.1038/ncomms7707
Leal, B., Zamora, E., Fuentes, A., Thomas, D. B., and Dearth, R. K. (2020). Questing by tick Larvae (Acari: Ixodidae): a review of the influences that affect off-host survival. Ann. Entomol. Soc. Am. 113, 425–438. doi: 10.1093/aesa/saaa013
Levi, T., Keesing, F., Holt, R. D., Barfield, M., and Ostfeld, R. S. (2016). Quantifying dilution and amplification in a community of hosts for tick-borne pathogens. Ecol. Applic. 26, 484–498. doi: 10.1890/15-0122
Linske, M. A., Williams, S. C., Stafford, K. C. III, and Ortega, I. M. (2018). Ixodes scapularis (Acari: Ixodidae) reservoir host diversity and abundance impacts on dilution of Borrelia burgdorferi (Spirochaetales: Spirochaetaceae) in residential and woodland habitats in connecticut, United States. J. Med. Entomol. 55, 681–690. doi: 10.1093/jme/tjx237
LoGiudice, K., Ostfeld, R. S., Schmidt, K. A., and Keesing, F. (2003). The ecology of infectious disease: effects of host diversity and community composition on Lyme disease risk. PNAS 100, 567–571. doi: 10.1073/pnas.0233733100
Lopes de Carvalho, I., Fonseca, J. E., Marques, J. G., Ullmann, A., Hojgaard, A., Zeidner, N., et al. (2008). Vasculitis-like syndrome associated with Borrelia lusitaniae infection. Clin. Rheumatol. 27, 1587–1591. doi: 10.1007/s10067-008-1012-z
Macleod, J. (1932). The bionomics of Ixodes ricinus L., the “sheep tick” of scotland. Parasitology 24, 382–400. doi: 10.1017/S0031182000020795
Mather, T. N., Nicholson, M. C., Donnelly, E. F., and Matyas, B. T. (1996). Entomologic index for human risk of lyme disease. Am. J. Epidemiol. 144, 1066–1069. doi: 10.1093/oxfordjournals.aje.a008879
Maxwell, T. L., Augusto, L., Bon, L., Courbineau, A., Altinalmazis-Kondylis, A., Milin, S., et al. (2020). Effect of a tree mixture and water availability on soil nutrients and extracellular enzyme activities along the soil profile in an experimental forest. Soil Biol. Biochem. 148:107864. doi: 10.1016/j.soilbio.2020.107864
McCoy, K. D., and Boulanger, N. (eds) (2015). Tiques et Maladies à Tiques: Biologie, Écologie Évolutive, Épidémiologie. Wellington: IRD.
Mendoza-Roldan, J. A., Manoj, R. R. S., Latrofa, M. S., Iatta, R., Annoscia, G., Lovreglio, P., et al. (2021). Role of reptiles and associated arthropods in the epidemiology of rickettsioses: a one health paradigm. PLoS Neglect. Trop. Dis. 15:e0009090. doi: 10.1371/journal.pntd.0009090
Merhej, V., and Raoult, D. (2011). Rickettsial evolution in the light of comparative genomics. Biol. Rev. 86, 379–405. doi: 10.1111/j.1469-185X.2010.00151.x
Messier, C., Bauhus, J., Sousa-Silva, R., Auge, H., Baeten, L., Barsoum, N., et al. (2021). For the sake of resilience and multifunctionality, let’s diversify planted forests! Conserv. Lett. 15:e12829. doi: 10.1111/conl.12829
Michelet, L., Delannoy, S., Devillers, E., Umhang, G., Aspan, A., Juremalm, M., et al. (2014). High-throughput screening of tick-borne pathogens in Europe. Front. Cell. Infect. Microbiol. 4:103. doi: 10.3389/fcimb.2014.00103
Michelis, S. D., Sewell, H.-S., Collares-Pereira, M., Santos-Reis, M., Schouls, L. M., Benes, V., et al. (2000). Genetic diversity of Borrelia burgdorferi Sensu Lato in Ticks from Mainland Portugal. J. Clin. Microbiol. 38, 2128–2133. doi: 10.1128/JCM.38.6.2128-2133.2000
Milligan, H. T., and Koricheva, J. (2013). Effects of tree species richness and composition on moose winter browsing damage and foraging selectivity: an experimental study. J. Anim. Ecol. 82, 739–748. doi: 10.1111/1365-2656.12049
Ostfeld, R. S., and Keesing, F. (2012). Effects of host diversity on infectious disease. Annu. Rev. Ecol. Evol. Syst. 43, 157–182. doi: 10.1146/annurev-ecolsys-102710-145022
Ostfeld, R. S., Levi, T., Keesing, F., Oggenfuss, K., and Canham, C. D. (2018). Tick-borne disease risk in a forest food web. Ecology 99, 1562–1573. doi: 10.1002/ecy.2386
Paquette, A., Hector, A., Castagneyrol, B., Vanhellemont, M., Koricheva, J., Scherer-Lorenzen, M., et al. (2018). A million and more trees for science. Nat. Ecol. Evol. 2, 763–766. doi: 10.1038/s41559-018-0544-0
Pepin, K. M., Eisen, R. J., Mead, P. S., Piesman, J., Fish, D., Hoen, A. G., et al. (2012). Geographic variation in the relationship between human lyme disease incidence and density of infected host-seeking Ixodes scapularis Nymphs in the Eastern United States. Am. J. Trop. Med. Hyg. 86, 1062–1071. doi: 10.4269/ajtmh.2012.11-0630
Prinz, M., Fuchs, J., Ehrmann, S., Scherer-Lorenzen, M., Kochs, G., and Panning, M. (2017). Molecular identification of novel phlebovirus sequences in European ticks. Ticks Tick Borne Dis. 8, 795–798. doi: 10.1016/j.ttbdis.2017.06.005
R Core Team (2021). R: A Language and Environment for Statistical Computing. Vienna: R Foundation for Statistical Computing.
Randolph, S. E., and Dobson, A. D. M. (2012). Pangloss revisited: a critique of the dilution effect and the biodiversity-buffers-disease paradigm. Parasitology 139, 847–863. doi: 10.1017/S0031182012000200
Renaud, V., Innes, J. L., Dobbertin, M., and Rebetez, M. (2011). Comparison between open-site and below-canopy climatic conditions in Switzerland for different types of forests over 10 years (1998–2007). Theor. Appl. Climatol. 105, 119–127. doi: 10.1007/s00704-010-0361-0
Richter, D., and Matuschka, F.-R. (2006). Perpetuation of the lyme disease spirochete Borrelia lusitaniae by lizards. Appl. Environ. Microbiol. 72, 4627–4632. doi: 10.1128/AEM.00285-06
Rizzoli, A., Silaghi, C., Obiegala, A., Rudolf, I., Hubálek, Z., Földvári, G., et al. (2014). Ixodes ricinus and its transmitted pathogens in urban and peri-urban areas in europe: new hazards and relevance for public health. Front. Public Health 2:251. doi: 10.3389/fpubh.2014.00251
Ruyts, S. C., Ampoorter, E., Coipan, E. C., Baeten, L., Heylen, D., Sprong, H., et al. (2016). Diversifying forest communities may change Lyme disease risk: extra dimension to the dilution effect in Europe. Parasitology 143, 1310–1319. doi: 10.1017/S0031182016000688
Ruyts, S. C., Landuyt, D., Ampoorter, E., Heylen, D., Ehrmann, S., Coipan, E. C., et al. (2018). Low probability of a dilution effect for Lyme borreliosis in Belgian forests. Ticks Tick Borne Dis. 9, 1143–1152. doi: 10.1016/j.ttbdis.2018.04.016
Sarih, M., Jouda, F., Gern, L., and Postic, D. (2003). First isolation of Borrelia burgdorferi Sensu Lato from Ixodes ricinus Ticks in Morocco. Vect. Borne Zoonot. Dis. 3, 133–139. doi: 10.1089/153036603768395834
Scali, S., Manfredi, M. T., and Guidali, E. (2001). Lacerta bilineata (Reptilia, Lacertidae) as a host of Ixodes ricinus (Acari, Ixodidae) in a protected area of Northern Italy. Parassitologia 43, 165–168.
Schielzeth, H. (2010). Simple means to improve the interpretability of regression coefficients. Methods Ecol. Evol. 1, 103–113. doi: 10.1111/j.2041-210X.2010.00012.x
Schmidt, K. A., and Ostfeld, R. S. (2001). Biodiversity and the dilution effect in disease ecology. Ecology 82, 609–619. doi: 10.1890/0012-9658(2001)082[0609:batdei]2.0.co;2
Schulze, T. L., and Jordan, R. A. (2005). Influence of meso- and microscale habitat structure on focal distribution of sympatric Ixodes scapularis and Amblyomma americanum (Acari: Ixodidae). J. Med. Entomol. 42, 285–294. doi: 10.1093/jmedent/42.3.285
Smith, G. R., and Ballinger, R. E. (2001). The ecological consequences of habitat and microhabitat use in lizards:: a review. Contemp. Herpetol. 1:11957. doi: 10.17161/ch.vi1.11957
Smith, K. F., Goldberg, M., Rosenthal, S., Carlson, L., Chen, J., Chen, C., et al. (2014). Global rise in human infectious disease outbreaks. J. R. Soc. Interf. 11:20140950. doi: 10.1098/rsif.2014.0950
Sprong, H., Wielinga, P. R., Fonville, M., Reusken, C., Brandenburg, A. H., Borgsteede, F., et al. (2009). Ixodes ricinus ticks are reservoir hosts for Rickettsia helvetica and potentially carry flea-borne Rickettsia species. Parasites Vect. 2:41. doi: 10.1186/1756-3305-2-41
Stafford, K. C., Cartter, M. L., Magnarelli, L. A., Ertel, S.-H., and Mshar, P. A. (1998). Temporal correlations between tick abundance and prevalence of ticks infected with borrelia burgdorferi and increasing incidence of lyme disease. J. Clin. Microbiol. 36, 1240–1244. doi: 10.1128/JCM.36.5.1240-1244.1998
Stanek, G., and Reiter, M. (2011). The expanding Lyme Borrelia complex—clinical significance of genomic species? Clin. Microbiol. Infect. 17, 487–493. doi: 10.1111/j.1469-0691.2011.03492.x
Steere, A. C., Coburn, J., and Glickstein, L. (2004). The emergence of Lyme disease. J. Clin. Invest. 113, 1093–1101. doi: 10.1172/JCI21681
Stein, K. J., Waterman, M., and Waldon, J. L. (2008). The effects of vegetation density and habitat disturbance on the spatial distribution of Ixodid ticks (Acari: Ixodidae). Geospatial Health 2, 241–252. doi: 10.4081/gh.2008.247
Stemmelen, A., Jactel, H., Brockerhoff, E. G., and Castagneyrol, B. (2021). Meta-analysis of tree diversity effects on the abundance, diversity and activity of herbivores’ enemies. biorxiv [Preprint]. doi: 10.1101/2021.07.05.451117
Tack, W., Madder, M., Baeten, L., De Frenne, P., and Verheyen, K. (2012). The abundance of Ixodes ricinus ticks depends on tree species composition and shrub cover. Parasitology 139, 1273–1281. doi: 10.1017/S0031182012000625
Tack, W., Madder, M., Baeten, L., Vanhellemont, M., and Verheyen, K. (2013). Shrub clearing adversely affects the abundance of Ixodes ricinus ticks. Exp. Appl. Acarol. 60, 411–420. doi: 10.1007/s10493-013-9655-0
Tomassone, L., Ceballos, L. A., Ragagli, C., Martello, E., De Sousa, R., Stella, M. C., et al. (2017). Importance of common wall lizards in the transmission dynamics of tick-borne pathogens in the Northern Apennine Mountains, Italy. Microb. Ecol. 74, 961–968. doi: 10.1007/s00248-017-0994-y
Tukahirwa, E. M. (1976). The effects of temperature and relative humidity on the development of Rhipicephalus appendiculatus Neumann (Acarina, Ixodidae). Bull. Entomol. Res. 66, 301–312. doi: 10.1017/S0007485300006696
Vassallo, M., Pichon, B., Cabaret, J., Figureau, C., and Pérez-Eid, C. (2000). Methodology for sampling questing nymphs of Ixodes ricinus (Acari: Ixodidae), the principal vector of lyme disease in europe. J. Med. Entomol. 37, 335–339. doi: 10.1093/jmedent/37.3.335
Verheyen, K., Vanhellemont, M., Auge, H., Baeten, L., Baraloto, C., Barsoum, N., et al. (2016). Contributions of a global network of tree diversity experiments to sustainable forest plantations. Ambio 45, 29–41. doi: 10.1007/s13280-015-0685-1
Vourc’h, G., Abrial, D., Bord, S., Jacquot, M., Masséglia, S., Poux, V., et al. (2016). Mapping human risk of infection with Borrelia burgdorferi sensu lato, the agent of Lyme borreliosis, in a periurban forest in France. Ticks Tick Borne Dis. 7, 644–652. doi: 10.1016/j.ttbdis.2016.02.008
Walker, A. R., Alberdi, M. P., Urquhart, K. A., and Rose, H. (2001). Risk factors in habitats of the tick Ixodes ricinus influencing human exposure to Ehrlichia phagocytophila bacteria. Med. Vet. Entomol. 15, 40–49. doi: 10.1046/j.1365-2915.2001.00271.x
Williams, L. J., Paquette, A., Cavender-Bares, J., Messier, C., and Reich, P. B. (2017). Spatial complementarity in tree crowns explains overyielding in species mixtures. Nat. Ecol. Evol. 1:63. doi: 10.1038/s41559-016-0063
Wu, Q., Richard, M., Rutschmann, A., Miles, D. B., and Clobert, J. (2019). Environmental variation mediates the prevalence and co-occurrence of parasites in the common lizard, Zootoca vivipara. BMC Ecol. 19:44. doi: 10.1186/s12898-019-0259-3
Yoder, J. A., Ark, J. T., Benoit, J. B., Rellinger, E. J., and Tank, J. L. (2006). Inability of the lone star tick, Amblyomma americanum (L.), to resist desiccation and maintain water balance following application of the entomopathogenic fungus Metarhizium anisopliae var. anisopliae (Deuteromycota). Int. J. Acarol. 32, 211–218. doi: 10.1080/01647950608684463
Younsi, H., Postic, D., Baranton, G., and Bouattour, A. (2001). High prevalence of Borrelia lusitaniae in Ixodes ricinus ticks in Tunisia. Eur. J. Epidemiol. 17, 53–56. doi: 10.1023/A:1010928731281
Keywords: biodiversity, forest ecosystems, human health, ORPHEE, ticks, tick-borne disease
Citation: Bourdin A, Bord S, Durand J, Galon C, Moutailler S, Scherer-Lorenzen M and Jactel H (2022) Forest Diversity Reduces the Prevalence of Pathogens Transmitted by the Tick Ixodes ricinus. Front. Ecol. Evol. 10:891908. doi: 10.3389/fevo.2022.891908
Received: 08 March 2022; Accepted: 04 April 2022;
Published: 09 May 2022.
Edited by:
Alexandro Guterres, Oswaldo Cruz Foundation (Fiocruz), BrazilReviewed by:
Gerhard Dobler, Bundeswehr Institute of Microbiology, GermanyJulia Geller, National Institute for Health Development, Estonia
Copyright © 2022 Bourdin, Bord, Durand, Galon, Moutailler, Scherer-Lorenzen and Jactel. This is an open-access article distributed under the terms of the Creative Commons Attribution License (CC BY). The use, distribution or reproduction in other forums is permitted, provided the original author(s) and the copyright owner(s) are credited and that the original publication in this journal is cited, in accordance with accepted academic practice. No use, distribution or reproduction is permitted which does not comply with these terms.
*Correspondence: Audrey Bourdin, audrey.bourdin5@gmail.com