Integument carotenoid-based colouration reflects contamination to perfluoroalkyl substances, but not mercury, in arctic black-legged kittiwakes
- 1Unité Physiologie Moléculaire et Adaptation, UMR 7221, Muséum National d’Histoire Naturelle, CNRS, CP32, Paris, France
- 2Centre d’Etudes Biologiques de Chizé (CEBC), UMR 7372 CNRS–La Rochelle Université, Villiers-en-Bois, France
- 3Akvaplan-niva AS, Fram Centre, Tromsø, Norway
- 4Norwegian Institute for Nature Research, Fram Centre, Tromsø, Norway
- 5Norwegian Polar Institute, Fram Centre, Tromsø, Norway
- 6Norwegian Institute for Air Research, Fram Centre, Tromsø, Norway
- 7Laboratory of Evolutionary Ecophysiology, Institute of Biology, University of Neuchâtel, Neuchâtel, Switzerland
- 8Norwegian Institute for Nature Research, Trondheim, Norway
- 9Littoral Environnement et Sociétés (LIENSs), UMR 7266 CNRS–La Rochelle Université, La Rochelle, France
- 10Université de Pau et des Pays de l’Adour, E2S UPPA, INRAE, ECOBIOP, Saint-Pée-sur-Nivelle, France
- 11Institut Universitaire de France (IUF), Paris, France
Anthropogenic activities are introducing multiple chemical contaminants into ecosystems that act as stressors for wildlife. Perfluoroalkyl substances (PFAS) and mercury (Hg) are two relevant contaminants that may cause detrimental effects on the fitness of many aquatic organisms. However, there is a lack of information on their impact on the expression of secondary sexual signals that animals use for mate choice. We have explored the correlations between integument carotenoid-based colourations, blood levels of carotenoids, and blood levels of seven PFAS and of total Hg (THg) in 50 adult male black-legged kittiwakes (Rissa tridactyla) from the Norwegian Arctic during the pre-laying period, while controlling for other colouration influencing variables such as testosterone and body condition. Kittiwakes with elevated blood concentrations of PFAS (PFOSlin, PFNA, PFDcA, PFUnA, or PFDoA) had less chromatic but brighter bills, and brighter gape and tongue; PFOSlin was the pollutant with the strongest association with bill colourations. Conversely, plasma testosterone was the only significant correlate of hue and chroma of both gape and tongue, and of hue of the bill. Kittiwakes with higher concentrations of any PFAS, but not of THg, tended to have significantly higher plasma concentrations of the carotenoids astaxanthin, zeaxanthin, lutein, and cryptoxanthin. Our work provides the first correlative evidence that PFAS exposure might interfere with the carotenoid metabolism and the expression of integument carotenoid-based colourations in a free-living bird species. This outcome may be a direct effect of PFAS exposure or be indirectly caused by components of diet that also correlate with elevated PFAS concentrations (e.g., proteins). It also suggests that there might be no additive effect of THg co-exposure with PFAS on the expression of colourations. These results call for further work on the possible interference of PFAS with the expression of colourations used in mate choice.
Introduction
Carotenoids are lipophilic molecules that birds are unable of synthetizing de novo, so that they have to acquire them through their diet. Many bird species exhibit carotenoid-based colourations in feathers or in bare parts (Zahavi, 1975; Olson and Owens, 2005; Hill and McGraw, 2006a,b). These colourations work as sexual signals of quality; more colourful individuals will have higher mating success than less colourful birds (Zahavi, 1975; Olson and Owens, 2005; Hill and McGraw, 2006a,b). Moreover, birds use carotenoids to sustain several organism functions, such as immune response, protection of cell membranes, and metabolism (Hill and McGraw, 2006a,b). Carotenoids occur in limited supply in foods, so that it is hypothesised the existence of a trade-off between allocation of carotenoids towards the expression of sexual colourations or towards self-maintenance functions (von Schantz et al., 1999; Koch and Hill, 2018).
The condition-dependent nature of carotenoid-based colourations makes them ideally suited as indicators of environmental quality. Indeed, numerous environmental stressors can influence the expression of carotenoid-based colourations (Hill, 1995; Hutton and McGraw, 2016; Lifshitz and St Clair, 2016). Hill (1995) advanced the hypothesis that sexual ornaments act as indicators of environmental quality. Consistently, the majority of published studies on this topic found that animals have reduced expression of colourations, including those that are carotenoid-based, in habitats of poorer quality [reviewed in Hutton and McGraw (2016)]. In recent times, there has been a growing interest in elucidating the mechanisms (e.g., endocrine disruption, interference with lipoprotein activity, oxidative stress) through which chemical pollutants affect body colourations (e.g., Lifshitz and St Clair, 2016; Marasco and Costantini, 2016). Pioneering work by Bortolotti et al. (2003) demonstrated that exposure to an environmentally relevant dose of a mixture of PCBs affected both plasma carotenoids and carotenoid-dependent colourations in the American kestrel (Falco sparverius). Since then, a growing body of research established links between body colourations and contaminant burden (Blévin et al., 2014; Lifshitz and St Clair, 2016; Marasco and Costantini, 2016).
We know comparatively less for organic pollutants of emerging concern, such as perfluoroalkyl substances (PFAS), and for trace metals, such as mercury (Hg). PFAS are synthetically manufactured chemicals, which consist of a perfluorinated alkyl chain with a terminal functional group and that are used as surface-active agents in a multitude of manufactured products (Glüge et al., 2020) including firefighting foams, waterproof clothing, non-stick cookware, coatings, and food packaging. Most PFAS have bioaccumulation and biomagnification potential and are highly persistent in the environment (e.g., Lau et al., 2007; Muir and de Wit, 2010). PFAS might have physiological disrupting properties and negative impacts on reproduction in free-living birds and other vertebrates (e.g., Bossi et al., 2005; Bustnes et al., 2008; Braune et al., 2011; Nøst et al., 2012; Tartu et al., 2014a,b; Blévin et al., 2017a,b; Melnes et al., 2017; Haarr et al., 2018; Costantini et al., 2019; Blévin et al., 2020). Although PFAS may interfere with lipoproteins (i.e., carriers of carotenoids in blood) or lipid metabolism (Wang et al., 2014; Dale et al., 2020), we know little about the potential interference of PFAS with carotenoid metabolism in birds (e.g., Yanai et al., 2008) and to date, there is a lack of information about potential effects of PFAS on plasma carotenoids and carotenoid-based colourations in animals.
On the other hand, Hg (particularly in the form of methylmercury MeHg) is a highly toxic non-essential metal of major concern for wildlife, which urged the adoption of new regulations to limit its impact on human health and the environment (e.g., the Minamata Convention). Once accumulated in the body, Hg may affect fundamental organism traits (e.g., Tartu et al., 2013, 2015, 2016; Soldatini et al., 2020) that may turn into negative effects at the population level (Evers et al., 2008; Goutte et al., 2014a,b). Effects of Hg on body colourations might be particularly ecologically relevant given the role of these colourations in sexual and social interactions. For example, prior work found that feathers of great tits (Parus major) with higher concentrations of Hg were less colourful (Giraudeau et al., 2015) and captive zebra finches (Taeniopygia guttata) experimentally exposed to MeHg had less red bills compared to control birds (Spickler et al., 2020).
In the Norwegian Arctic, birds are exposed to a complex cocktail of chemical contaminants that represent multiple stressors for them (Townhill et al., 2022). Black-legged kittiwakes (Rissa tridactyla) are chronically exposed to a large number of contaminants, including PFAS, OCPs, PCBs, and Hg, all suspected to impact on individual fitness and population dynamics in addition to colouration (e.g., Tartu et al., 2013, 2014a,b,2015, 2016; Blévin et al., 2016, 2017a,b,2018, 2020; Costantini et al., 2019). Both female and male kittiwakes express intense carotenoid-based colourations, including the yellow bill, orange tongue, red-orange gape, and red eye-ring during the breeding season (Leclaire et al., 2011; Doutrelant et al., 2013). Prior work conducted on arctic kittiwakes showed that individuals with higher blood concentrations of PCBs and OCPs had duller carotenoid-based colouration in labile integuments (i.e., gape, eye-ring and tongue) (Blévin et al., 2014). Because kittiwakes are exposed to multiple contaminants, we addressed the impact of single PFAS and of Hg (expressed as total concentration, THg) and their possible additive effects on sexual colourations. We have examined the correlations between three metrics of carotenoid-based colourations of integuments, concentrations of five circulating carotenoids of interest, and blood levels of seven PFAS and of THg in 50 adult male Arctic kittiwakes during the pre-laying period, while controlling for potential confounding factors known to affect the expression of colourations in birds, such as blood testosterone (e.g., Blas et al., 2006) and body condition (e.g., Doutrelant et al., 2013; Blévin et al., 2014). Concentrations of PCBs/OCPs were not determined in the here presented samples.
Materials and methods
Study area and sampling collection
We carried out the fieldwork from 25th May to 6th June 2016 in a colony of black-legged kittiwakes breeding at Kongsfjord, Svalbard (Krykkjefjellet, 78°54′N; 12°13′E). This study was examined and approved by the Norwegian Animal Ethics Committee and the governor of Svalbard, and was conducted under the permissions FOTS ID 8679 and 16/00483-2, respectively.
We caught 50 adult male kittiwakes during the pre-laying period (i.e., courtship and mating period) on their nest using a noose attached to the end of a long telescopic pole. We caught known-sexed kittiwakes after individual identification at a distance using a telescope thanks to a white PVC plastic band engraved with a three-letter code and fixed to the bird’s tarsus. These birds had been molecularly sexed in previous years following Fridolfsson and Ellegren (1999). Within 3 min from capture, we collected 0.5 ml of blood from the brachial vein using a heparinized syringe and a 25-gauge needle. We used this sample of blood for laboratory analyses of physiological metrics. Straightaway, we collected an additional blood sample (∼2 ml) that we allocated to the analyses of contaminants. We stored the tubes containing the blood samples in ice while in the field. Upon returning to the lab, we stored aliquots of whole blood and of both plasma and red blood cells obtained after centrifugation at −20°C until laboratory analyses. Straightaway after the collection of blood, we weighted all the birds to the nearest 5 g using a Pesola spring balance. We also measured the skull length (head + bill) using a sliding calliper with an accuracy of 0.1 mm.
Contaminant analyses
We measured concentrations of PFAS in plasma samples at the Norwegian Institute for Air Research (NILU) in Tromsø, Norway. The protocol to measure PFAS concentrations was already presented in Costantini et al. (2019). Briefly, we extracted a 0.2 ml aliquot of plasma spiked with internal standards applying the method of isotopic dilution (carbon labeled PFAS) with methanol (1 ml) by repeated sonication and vortexing. We cleaned-up the supernatant using ENVICarb graphitized carbon absorbent and glacial acetic acid. Finally, we analysed the extracts by UPLC/MS/MS (ultra performance liquid chromatography–tandem mass spectrometer). Recovery of the internal standards ranged between 86.3 and 120%. We validated the results with procedural blanks and standard reference material (SRM; 1957 human serum from NIST) run every 10 samples. The deviation of the target concentrations in the SRM were within the laboratory’s accepted range (69–119%). Blanks varied between concentrations below the instrument detection limits and 30 pg g–1 and were applied as the LOD in the form of 3 times the average concentration. PFAS below the limit of detection (LOD) were replaced with a value equal to LOD × detection frequency, when the detection frequency (percentage of detection) was >50% (e.g., James et al., 2002). Thereby, PFAS remaining for further statistical evaluation were the perfluoroalkyl carboxylic acids: perfluorononanoic acid (PFNA), perfluorodecanoate (PFDcA), perfluoroundecanoic acid (PFUnA), perfluorododecanoic acid (PFDoA), perfluorotridecanoic acid (PFTriA), perfluorotetradecanoic acid (PFTeA), and one perfluoroalkyl sulfonic acid: linear perfluorooctane sulfonic acid (PFOSlin).
We quantified THg in red blood cells (RBCs) at the Littoral Environment et Sociétés laboratory (LIENSs) in La Rochelle, France. We placed freeze-dried and powdered RBCs in an Advanced Hg analyser Spectrophotometer (AMA254; Altec) as described in Bustamante et al. (2006). We analysed aliquots ranging from 0.92 to 2.09 mg in duplicate (all CVs < 5%). We ran blanks at the beginning of each set of samples and we used certified reference material (CRM; DOLT-5; certified value 0.44 ± 0.18 [SD] μg g–1 dry wt) to validate the accuracy of the analyses. Measured values of the CRM were 0.35 ± 0.04 (SD) μg g–1 dry wt, n = 6. All blanks contained concentrations below the instrument detection limit (0.005 μg g–1 dry wt).
Testosterone assay
We quantified the plasma concentrations of testosterone at the Centre d’Etudes Biologiques de Chizé (CEBC), France, by radioimmunoassay as described in Goutte et al. (2010). Testosterone was extracted by adding 3 ml of diethyl-ether to 50 μl of plasma, vortexing, and centrifuging (5 min at 2000 rpm, at 4°C). The diethyl-ether phase containing testosterone was decanted and poured off after snap freezing the tube. The solvent was then evaporated at 37°C. The dried extract was re-dissolved in phosphate 0.01 M pH 7.4 buffer and testosterone was assayed in duplicate with RIA method. 100 μl of extract were incubated overnight at 4°C with 4000 cpm of H3-testosterone (Perkin Elmer, US) and polyclonal rabbit antiserum provided by Dr. Picaper (CHU La Source, Orléans, France). The bound fraction was then separated from free fraction by addition of dextran-coated charcoal and activity was counted on a tri-carb 2810 TR scintillation counter (Perkin Elmer, US). Inter- and intra-assay variations were, respectively, 7.08 and 7.28%. Testosterone lowest detectable concentration was 0.45 pg ml–1. Cross-reactions of testosterone antiserum were as follows: androsterone (63%), progesterone (1.45%), 17-β-estradiol (0.18%), corticosterone (0.41%), estrone (0.03%), aldosterone (<0.01%), cortisone (<0.01%).
Carotenoid measurements
We quantified the concentration of carotenoids in plasma at the Littoral Environment et Sociétés laboratory (LIENSs) in La Rochelle, France. First, we precipitated the proteins by adding 700 μl of absolute ethanol (VWR chemicals, Ref. 153385E) to 100 μl of plasma and vortexed the tube for 15 s. Afterwards, we added 700 μl of methyl-tert-butyl ether (MTBE; Alfa Aesar, ThermoFisher, Ref. 40477), we vortexed the mixture for 15 s, and we centrifuged the tubes at 12,000 rpm min–1 at 4°C for 15 min. Then, we transferred 1.3 ml of supernatant to glass vials and we evaporated it to dryness under a nitrogen flow. We re-suspended the residuals in 150 μl of methanol (Carol Erba Réactifs, Ref. 525101). Afterwards, we filtered the resulting solutions through 0.22 μm PVDF syringe filters (4 mm Millex-GV filters, Merck) to remove any potentially remaining protein fragments. Before quantification, we diluted 10 μl of sample and 20 μl of internal standard (canthaxantin) in 120 μl of a solution water:methanol, 20:80 (v:v). We carried out the quantification by ultra-high performance liquid chromatography (Acquity UPLC H-class, Waters) coupled to a high-resolution electrospray ionization mass spectrometer (XEVOG2 S Q-TOF, Waters). We injected 5 μl of the samples in the column (Acquity UPLC BEH C18, Waters, 2.1 × 50 mm, 1.7 μm), and we eluted the products at a flow rate of 300 μl min–1 using a gradient composed of solvents A (water:formic acid, 100:0.001 v:v) and B (methanol:formic acid, 100:0.001 v:v), according to the following procedure: 0–1 min, 80–85% B; 1–5 min 85% B; 5–8 min 85–90% B; 8–12 min 90% B; 12–14 min, 90–98% B, 14–22 min 98% B, 22–23 min 98–100% B, 23–27 min 100% B. During the analysis, we maintained the column and the injector at 25 and 7°C, respectively. We calibrated the mass spectrometer before analysis using 0.5 mM of sodium formate and we used leucine enkephalin (M = 555.62 Da, 1 ng μl–1) as a lock-mass. We identified peaks by comparing their retention time and absorbent properties with reference carotenoids (astaxanthin from Cayman Chemicals; β-carotene, β-cryptoxanthin, lutein, and zeaxanthin from Extrasynthese; anhydrolutein from US Biological) that were identified in the integument of kittiwakes (Leclaire et al., 2011, 2015, 2019; Doutrelant et al., 2013). We calculated the concentrations of carotenoids relying on dilution curves constructed from known amounts of references (2.5, 5, 7.5, 10, 25, 50, 75, 100, 250 μg l–1). We also passed three quantification controls (mix of reference carotenoids at concentrations 4, 40, and 80 μg l–1) at the start, middle and end of the sequence of samples to check the temporal stability of the analysis. We corrected the estimated quantities in reference to a canthaxanthin internal standard (50 μg l–1). We analysed each sample in triplicate. Anhydrolutein was not detectable in any sample. For β-cryptoxanthin, 5 values were <LOD and were treated like contaminant data < LOD. Technical repeatability was >0.99 for all carotenoids except β-cryptoxanthin, for which all values were below the limit of quantification, i.e., 2.5 μg l–1 (astaxanthin: R [95% CI] = 0.999 [0.998; 0.999]; β-carotene: 0.993 [0.988; 0.995]; β-cryptoxanthin: 0.963 [0.937; 0.978]; lutein: 0.990 [0.984; 0.994]; zeaxanthin: 0.993 [0.987; 0.995]). We estimated the extraction efficiency by extracting and quantifying known amounts of the reference carotenoids and comparing them with the direct quantification of the solution without extraction. Extraction efficiency was high, except for astaxanthin (anhydrolutein 98.2%; astaxanthin 77.4%; β-carotene 99.3%; β-cryptoxanthin 95.4%; lutein 99.2%; zeaxanthin 98.9%). We estimated the matrix effect by extracting and quantifying the increase in concentration when adding known amounts of the reference carotenoids to a sample, compared to the extraction and quantification of similar amounts of carotenoids without the sample. The decrease in quantified carotenoids due to the biological matrix was low, except for β-carotene and β-cryptoxanthin (anhydrolutein 3.0%; astaxanthin 8.1%; β-carotene 28.7%; β-cryptoxanthin 25.0%; lutein 3.5%; zeaxanthin 2.5%).
Integument colourations
We quantified the colourations (as emitted light) of three body parts (bill, gape, and tongue) considered as reliable signals of individual quality during the breeding season in this species (Leclaire et al., 2011; Doutrelant et al., 2013; Blévin et al., 2014). Although there is no experimental nor correlative evidence that black-legged kittiwakes use these colours for mate choice, prior work showed that males with high gape yellow chroma or higher tongue yellow chroma had higher fledging success than males with lower gape yellow chroma or lower tongue yellow chroma (Leclaire et al., 2011). In contrast, bill, tongue and gape brightness, and bill yellow chroma were not associated with fledging success (Leclaire et al., 2011).
Specifically, we took the colour measurements on the lower mandible of the bill, at the intersection between the upper and lower left mandible of the gape, and at ∼4 mm from the top of the tongue. We did not measure the eye-ring colouration because the light of the spectrophotometer could have damaged the eyes. We quantified the colouration with a reflectance spectrophotometer (Ocean Optics USB4000), a xenon light source, and a 200-μm fiber optic reflectance probe held at 45° to the integument surface. We took colour measurements five times per trait for each individual (repeatability ranging from 0.60 to 0.88), and we used the average value for subsequent statistical analyses. We measured the reflectance using SpectraSuite software (Ocean Optics) that we calibrated with dark reference (black background) and white standard (WS1 ocean optics). We recalibrated the spectrophotometer between each bird. We extracted standard achromatic (brightness) and chromatic (hue and chroma) colouration metrics (Montgomerie, 2006; Doutrelant et al., 2013). We analysed the reflectance spectra using the Avicol software (Gomez, 2006). We computed the brightness as the average reflectance over the total range of bird sensitivity 300–700 nm. Brightness indicates whether a colour is dark (lower value) or light (higher value). We computed the hue as the wavelength at which reflectance was halfway between its minimum and maximum (Lo50 measured); hue distinguishes one colour from another and is described using common names, such as red or yellow; for example, higher values of hue indicate redder colourations and lower more yellow or orange colourations (Doutrelant et al., 2013; Blévin et al., 2014). Finally, we computed the yellow chroma (measure of colour purity) as an index, which is particularly suited to carotenoid-based colours (Andersson and Prager, 2006); higher values of chroma indicate more saturated colourations.
Statistical analyses
Firstly, we reduced the number of colorimetric variables using a Principal Component Analysis (PCA) run on all the colour variables of the three teguments using PAST (Hammer et al., 2001). Selection of PCs was based on the visualization of the scree plot and broken stick model, and of the loadings of single variable on each PC. We extracted the first two PCs, which explained together 61.9% of the variance (35.8% for PC1 and 26.1% for PC2). PC1 (colour1 herein) was associated positively with the three colorimetric metrics of gape and tongue, and with hue of the bill (globally indicating more colourful, i.e., more chromatic gape and tongue, and higher values of hue for gape, tongue and bill; loadings and bivariate correlations in Supplementary Tables 1, 2); PC2 (colour2 herein) was associated positively with the brightness of bill, gape, and tongue, and negatively with hue and chroma of the bill, so globally indicating brighter bill, gape, and tongue, and less coloured bill (Supplementary Tables 1, 2). Secondly, we also used a PCA to reduce the number of circulating carotenoids. We extracted the first two PCs, which explained together 58.7% of the variance (35.2% for PC1 and 23.5% for PC2). PC1 (carot1 herein) was positively correlated with astaxanthin, zeaxanthin, lutein, cryptoxanthin, and β-carotene (loadings and bivariate correlations in Supplementary Tables 3, 4); PC2 (carot2 herein) was positively correlated with astaxanthin and negatively with lutein and β-carotene (Supplementary Tables 3, 4). Thirdly, we performed linear models using the lme4 package (Bates et al., 2015) in RStudio (R Core Team, 2021) to estimate the relationships between colorimetric or carotenoid metrics and contaminants. For the colorimetric variables, we ran eight models for colour1 and colour2, separately, including, as factors, a given contaminant (PFOSlin, PFNA, PFDcA, PFUnA, PFDoA, PFTriA, PFTeA, or THg) together with carot1, carot2, skull length, body mass, and testosterone. For the carotenoid variables, we ran eight models for carot1 and carot2, separately, including, as factors, a given contaminant (PFOSlin, PFNA, PFDcA, PFUnA, PFDoA, PFTriA, PFTeA, or THg) together with skull length, body mass, and testosterone. The variance inflation factor, quantified using the performance package (Lüdecke et al., 2021), was always below 2, indicating that multicollinearity was low in each of the models performed.
Finally, for colorimetric and for carotenoid variables separately, we compared the fitting of full models performed, where a given contaminant was a significant predictor, with that of a model including the significant contaminants together with the additional predictors used in prior lme models. To do so, first, we calculated the AICc values (small-sample size corrected Akaike Information Criterion), then we estimated the weight (wi) of each model because it indicates the probability that the model is the best among the whole set of candidate models. For example, a wi of 0.50 for a model indicates that the model has a 50% chance of being the best among those considered in the set of candidate models (Burnham and Anderson, 2002). We also considered that a difference > 2 between the AICc of the best-fitting model with another model indicated substantial evidence for the best-fitting model, following Burnham and Anderson (2002). If the variance of the carotenoid metric is being better explained by the effect of several contaminants (e.g., in case of additive or synergistic effects), the ΔAIC and wi of the model including several contaminants together should be > 2 and larger than that of models including single contaminants, respectively.
Results
PFAS and THg
Concentrations of pollutants were as follows (mean ± SD): PFOSlin, 13380 ± 6231 pg g–1 ww; PFBA, 1950 ± 863 pg g–1 ww; PFDcA, 2905 ± 1176 pg g–1 ww; PFUnA, 10268 ± 3675 pg g–1 ww; PFDoA, 1689 ± 831 pg g–1 ww; PFTriA, 8551 ± 3139 pg g–1 ww; PFTeA, 1008 ± 854 pg g–1 ww; THg, 2.88 ± 0.54 μg g–1 dw [reported also in Costantini et al. (2019)]. Correlations among PFAS were always significant (r ≥ 0.34, p ≤ 0.015); in contrast, Hg did not correlate with any of the PFAS considered (Supplementary Table 5).
Integument colorations
Models were consistent in showing that kittiwakes with higher testosterone had more chromatic gape and tongue, and higher values of hue for gape, tongue and bill (Table 1). Neither body condition and plasma carotenoids nor any pollutant were linearly associated with colour1 (Table 1).
Kittiwakes with higher blood concentrations of PFOSlin, PFNA, PFDcA, PFUnA, or PFDoA were characterised with an elevated value of colour2, i.e., brighter but less chromatic bill, and slightly brighter gape and tongue while controlling for testosterone and body condition (Table 1). Neither THg nor PFTriA and PFTeA were significantly associated with colour2 (Table 1).
A comparison of models including PFOSlin, PFNA, PFDcA, PFUnA, or PFDoA based on AICc showed that the highest-ranking model included PFOSlin (Table 2 and Figure 1). Importantly, the rank of this model was also higher than that of a model including the four PFCAs (perfluorocarboxylic acids) PFNA, PFDcA, PFUnA, or PFDoA together.
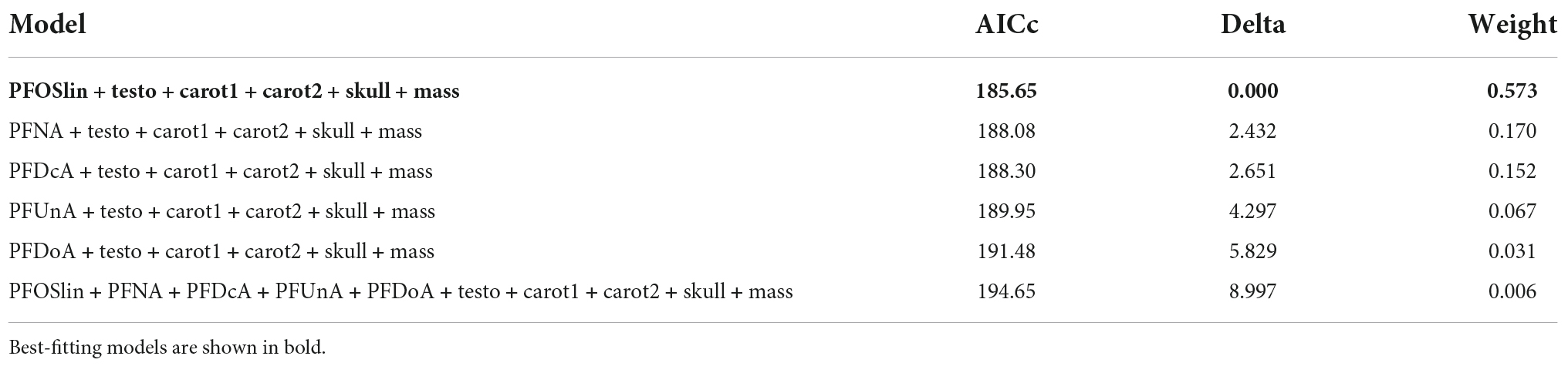
Table 2. Rank of lme models for colorimetric variable colour2 as estimated according to Akaike information criterion.
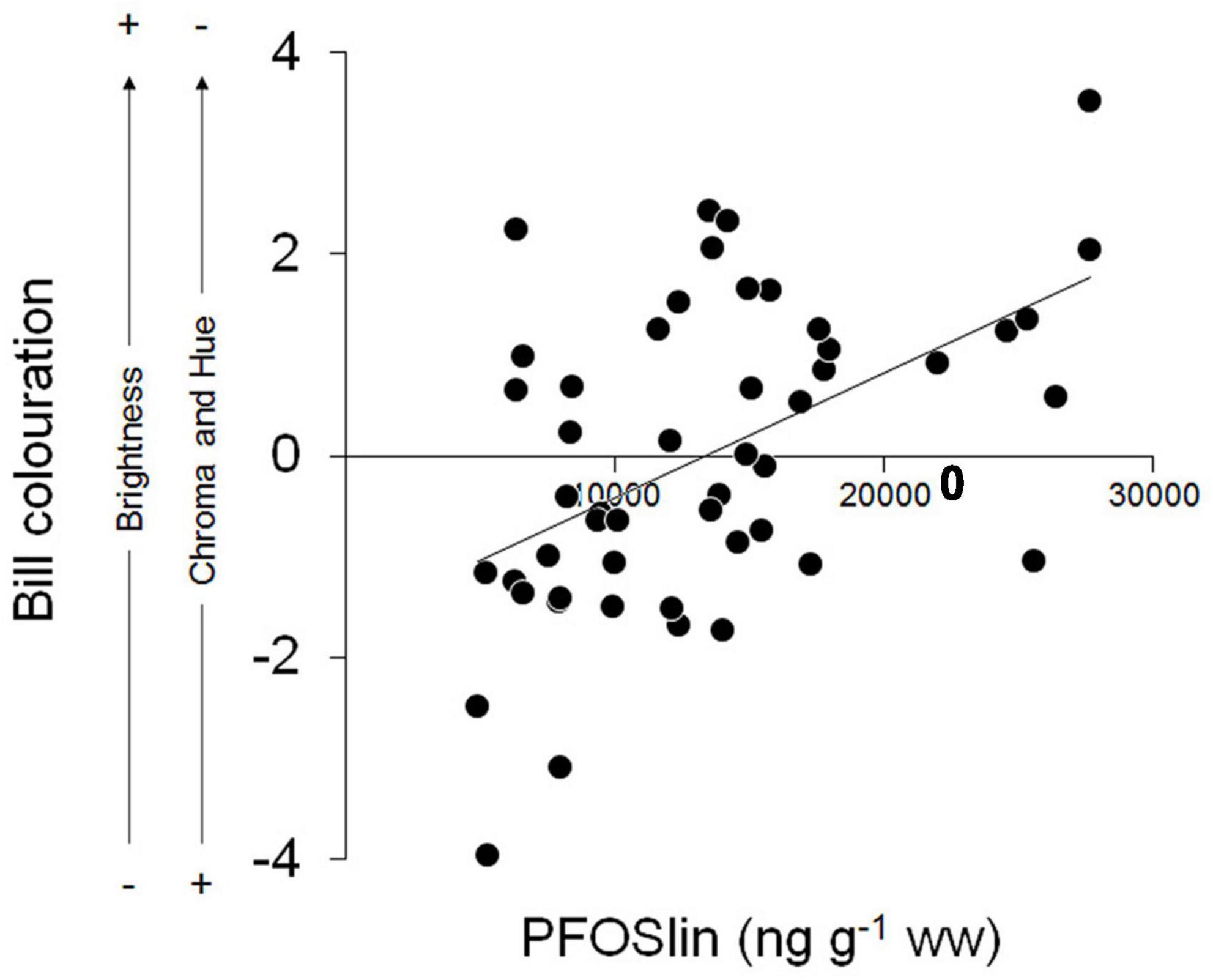
Figure 1. Biplot showing the correlation between plasma concentration of PFOSlin and bill colouration (PC2 from PCA) in 50 Arctic male kittiwakes sampled during the pre-laying period.
Plasma carotenoids
Kittiwakes with higher concentrations of any PFAS had higher values of carot1, indicating higher concentrations of astaxanthin, zeaxanthin, lutein, and cryptoxanthin (Table 3). Neither concentration of THg and none of the other factors were significantly associated with carot1. Carot2 was not linearly associated with any of the factors considered (Table 3). A comparison of models including PFAS congeners based on AICc showed that the highest-ranking models included PFTeA, PFDcA, and PFNA, respectively (Table 4 and Figure 2).
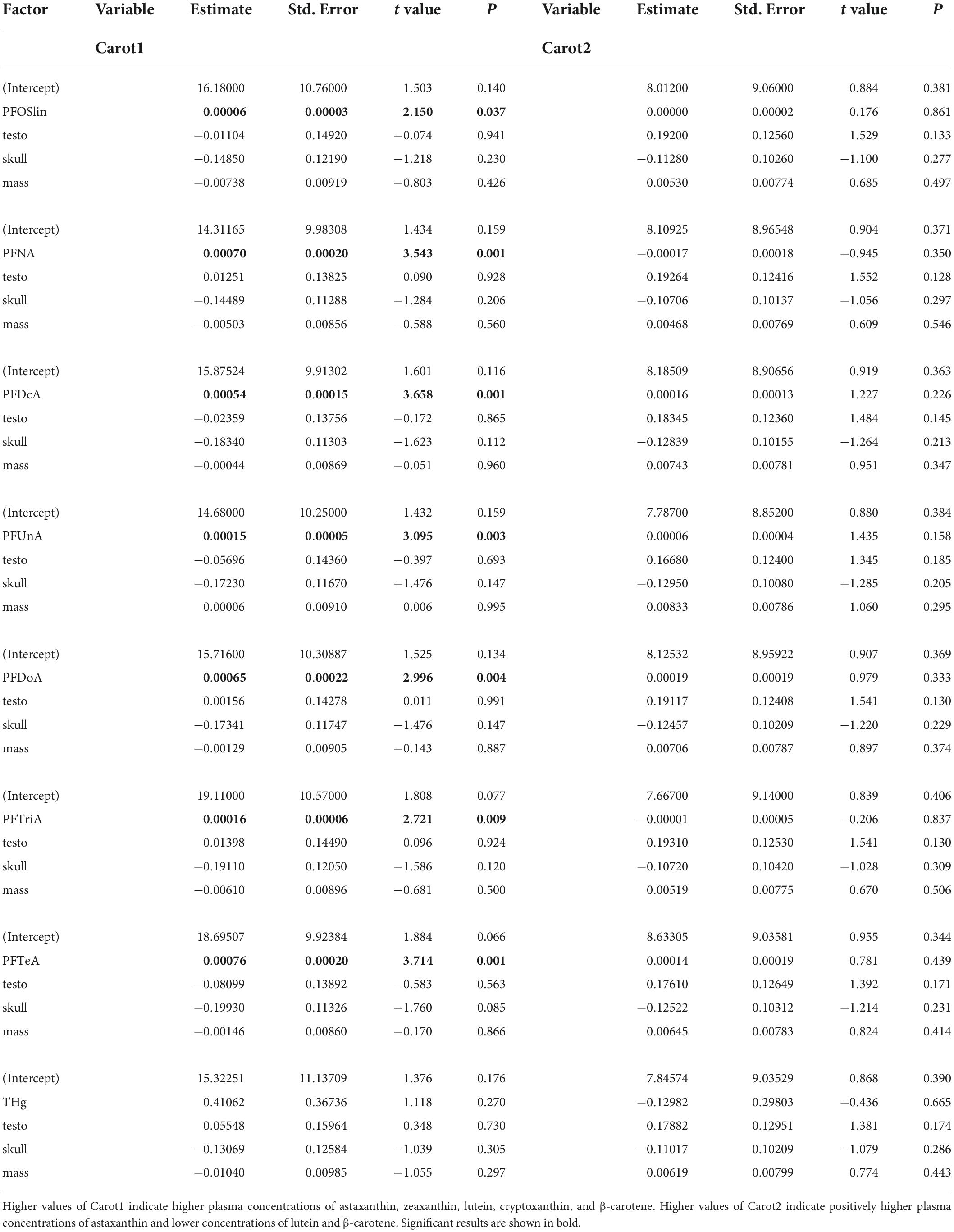
Table 3. Outcomes of lme models testing the effects of contaminants on the concentrations of carotenoids in plasma.
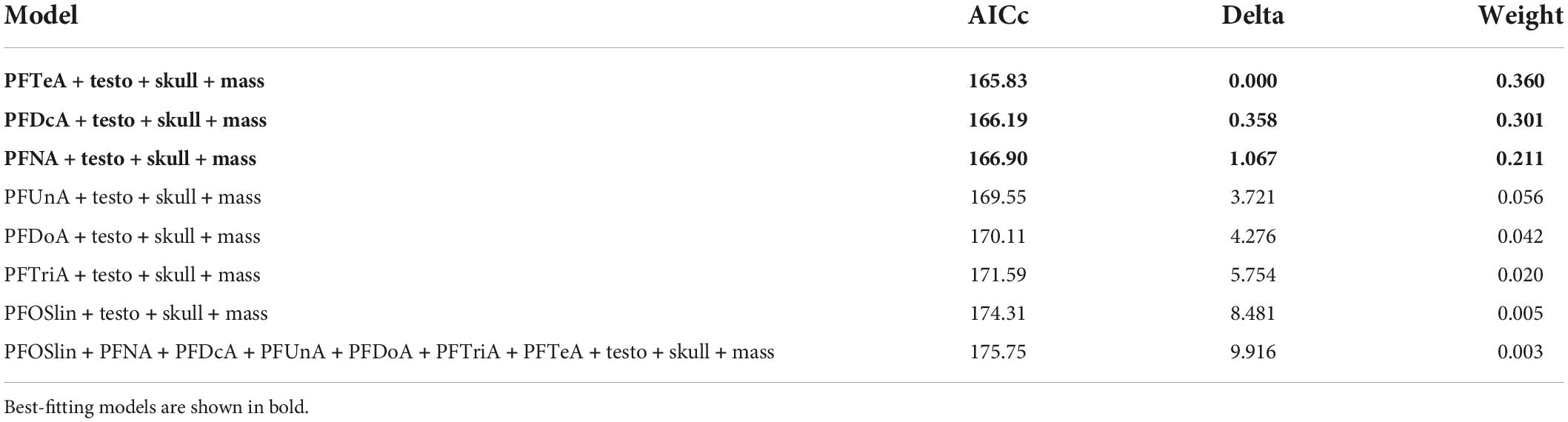
Table 4. Rank of lme models for carot1 (plasma carotenoids) as estimated according to Akaike information criterion.
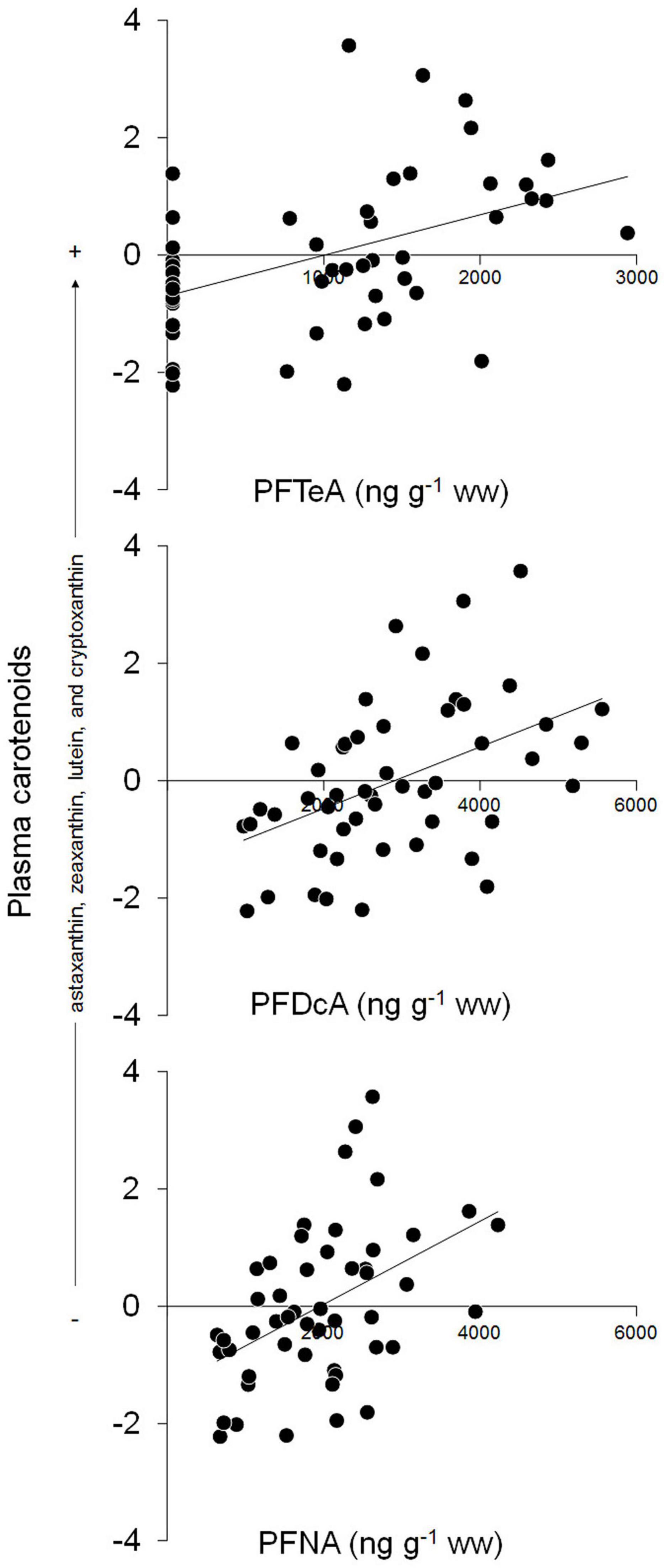
Figure 2. Biplots showing the correlations between plasma concentrations of plasma carotenoids (PC1 from PCA) and PFTeA, PFDcA, or PFNA in 50 Arctic male kittiwakes sampled during the pre-laying period.
Discussion
Our results provide the first evidence in a bird species for a significant correlation between the plasma concentration of some PFAS congeners and metrics of integument carotenoid-based colourations. We found that (i) male kittiwakes with elevated concentrations of PFOSlin, PFNA, PFDcA, PFUnA, or PFDoA tended to have brighter bill, gape and tongue, and lower values of hue and chroma of the bill and (ii) PFOSlin was the congener which best explained the variation in brightness and chroma of the colourations. Neither PFTriA, PFTeA, nor THg were significantly associated with colourations. We also found that concentrations of PFAS, were positively associated with plasma concentrations of the carotenoids astaxanthin, zeaxanthin, lutein, and cryptoxanthin. Finally, we found that plasma testosterone was the only significant correlate of bill, gape, and tongue chromas and hues.
The results of our study suggest that PFAS might have interfered somehow with the allocation of carotenoids to bill colouration and to a lower extent to gape and tongue. Several studies have found positive effects of carotenoid supplementation on traits as diverse as sperm quality (Helfenstein et al., 2010), song (Van Hout et al., 2011), or immune function (Simons et al., 2012), so that birds might strategically mobilize them from tissues or rapidly metabolize them to overcome the harmful effects of environmental stressors (e.g., chemical pollutants) at the expense of coloured sexual signals. Integument carotenoid-based colourations can change dynamically depending on the circumstances (e.g., Casagrande et al., 2007) with carotenoids being diverted from the skin to the circulating system. This might explain to some degree why we found plasma concentrations of carotenoids in kittiwakes to be positively correlated to levels of PFAS. It might also be that PFAS inhibited the activity of molecular carriers of carotenoids (lipoproteins), limiting their deposition into the tissue and determining elevated levels in the blood as a consequence. Prior work documented effects of PFAS on lipoproteins and lipid homeostasis (Wang et al., 2014; Dale et al., 2020; Liu et al., 2020) and found that the apolipoprotein A–I was a key protein for PFOS binding and transport in the plasma of tiger pufferfish (Takifugu rubripes) (Honda et al., 2014). In prior work based on the same dataset, we found that increased oxidative stress might be one consequence of long-chain perfluoroalkyl carboxylates (PFDoA, PFTriA, and PFTeA) exposure (Costantini et al., 2019). Although carotenoids have modest antioxidant effects in birds (Costantini and Møller, 2008), it might be that they are rapidly mobilised from tissues in response to oxidative stress, but they are being bleached by oxidation processes. Thus, less carotenoids would be available for body colourations. More studies are required to identify the molecular mechanisms through which PFAS would impact on carotenoids.
None of the models provided evidence for the conclusion that THg contamination in kittiwakes has significant effects on carotenoids. A previous detailed review on impacts of Hg exposure in birds showed that 72% of field studies and 91% of laboratory studies found evidence of deleterious effects of Hg on some physiological, behavioural or reproductive endpoint (Whitney and Cristol, 2017). This review also showed that studies on body colourations were underrepresented in literature and that carotenoids were largely ignored in favour of melanin-based colourations. For example, White and Cristol (2014) found that THg contamination was positively correlated with brightness of structural and melanin-based plumage colour in belted kingfishers (Megaceryle alcyon) consistent with the hypothesis that Hg slows the production of melanins. Similarly, McCullagh et al. (2015) found that higher tissue Hg accumulation was associated with brighter melanin-based plumage in eastern bluebirds (Sialia sialis). Also, studies on birds found significant associations between Hg and both plumage and integument carotenoid-based colourations (Giraudeau et al., 2015; Spickler et al., 2020). For example, Giraudeau et al. (2015) found a negative correlation between feather THg and carotenoid-based colouration of breast feathers in great tits. Thus, it might be that the range of THg concentrations (1.98–4.80 μg g–1 dw) in kittiwakes was probably too low for this particular species (e.g., more resistant or tolerant as compared to terrestrial species), preventing us to detect any significant sublethal effects. Thus, we believe that further research would be needed to explore the interaction between Hg and carotenoid metabolism.
Conclusion
Our work provides the first evidence in a bird species that PFAS might be directly or indirectly related with carotenoid metabolism and the expression of integument carotenoid-based colourations. It does not support the conclusion that (i) current Hg contamination would interfere with carotenoid usage in Arctic kittiwakes and (ii) the co-exposure to multiple PFAS might generate stronger effects (e.g., additive, synergistic) on the expression of carotenoid-based colourations. Prior work on male kittiwakes showed that the colorimetric traits that we found to be significantly associated with PFAS were poor predictors of reproductive success (Leclaire et al., 2011). However, given the multiple signals that integument carotenoid-based colourations may convey, our results might break new ground on the potential interference of PFAS contamination and correlated variables (e.g., other organic pollutants) with sexual selection.
Data availability statement
The raw data supporting the conclusions of this article will be made available by the authors, without undue reservation.
Ethics statement
The animal study was reviewed and approved by the Norwegian Animal Ethics Committee and the governor of Svalbard.
Author contributions
PBl and OC led the conception and design of the study. PBl, OC, SH-G, and DC collected samples on the field. CP, PBu, CR, and DH performed or supervised lab work. PBl built the database and wrote the method section of the manuscript. VE extracted integument colouration metrics. DC performed data processing and statistical analyses and wrote the first draft of the manuscript. All authors contributed to the manuscript revision, read, and approved the submitted version.
Funding
This project was supported by the Institut Polaire Français (project 330 to OC), the ANR ILETOP (ANR-16-CE34-0005), and the Contrat Plan-Etat Région (ECONAT). PBl was funded by a Ph.D. grant from University of La Rochelle. PBu was supported by the Institut Universitaire de France as a Senior Member. Additional funding was provided by the hazardous substances flagship programme of the Fram Center (Multiple Stressor seabird project to JB) and the Research Council of Norway (COPE project to I.S. Krogseth and D. Herzke #287114). Mercury analyses were funded by the project ILETOP (ANR-16-CE34-0005).
Acknowledgments
We thank the French-German Arctic Research Base AWIPEV and the Norwegian Polar Institute for their logistic help in the field. We thank B. Michaud, S. Nilsen, and I. Gabrielsen for their great help in the field. We would also like to thank all the staff of the NILU for their assistance during PFAS analysis; C. Doutrelant for help with quantification of colourations; M. Brault Favrou, C. Churlaud from the plateforme Analyses Elémentaires of LIENSs and J. Sire from CEBC for Hg analysis; A. Bonnet from LIENSs for carotenoids analysis; C. Trouvé from CEBC for testosterone assay. The IUF (Institut Universitaire de France) is acknowledged for its support to PBu as a Senior Member; two reviewers for providing valuable comments on our manuscript.
Conflict of interest
The authors declare that the research was conducted in the absence of any commercial or financial relationships that could be construed as a potential conflict of interest.
Publisher’s note
All claims expressed in this article are solely those of the authors and do not necessarily represent those of their affiliated organizations, or those of the publisher, the editors and the reviewers. Any product that may be evaluated in this article, or claim that may be made by its manufacturer, is not guaranteed or endorsed by the publisher.
Supplementary material
The Supplementary Material for this article can be found online at: https://www.frontiersin.org/articles/10.3389/fevo.2022.952765/full#supplementary-material
References
Andersson, S., and Prager, M. (2006). “Quantifying colours,” in Bird Coloration. Mechanisms and Measurements, Vol. I, eds G. E. Hill and K. J. McGraw (Cambridge: Harvard University Press), 41–89.
Bates, D., Mächler, M., Bolker, B., and Walker, S. (2015). Fitting linear mixed-effects models using lme4. J. Statist. Software 67, 1–48.
Blas, J., Pérez-Rodríguez, L., Bortolotti, G. R., Vinuela, J., and Marchant, T. A. (2006). Testosterone increases bioavailability of carotenoids: insights into the honesty of sexual signaling. Proc. Natl. Acad. Sci. U S A. 103, 18633–18637.
Blévin, P., Angelier, F., Tartu, S., Bustamante, P., Herzke, D., Moe, B., et al. (2016). Exposure to oxychlordane is associated with shorter telomeres in arctic breeding kittiwakes. Sci. Total Environ. 56, 125–130. doi: 10.1016/j.scitotenv.2016.04.096
Blévin, P., Angelier, F., Tartu, S., Bustamante, P., Herzke, D., Moe, B., et al. (2017a). Perfluorinated substances and telomeres in an Arctic seabird: cross-sectional and longitudinal approaches. Environ. Pollut. 230, 360–367. doi: 10.1016/j.envpol.2017.06.060
Blévin, P., Tartu, S., Ellis, H. I., Chastel, O., Bustamante, P., Parenteau, C., et al. (2017b). Contaminants and energy expenditure in an Arctic seabird: organochlorine pesticides and perfluoroalkyl substances are associated with metabolic rate in a contrasted manner. Environ. Res. 157, 118–126. doi: 10.1016/j.envres.2017.05.022
Blévin, P., Shaffer, S. A., Bustamante, P., Angelier, F., Picard, B., Herzke, D., et al. (2018). Organochlorines, perfluoroalkyl substances, mercury, and egg incubation temperature in an Arctic seabird: insights from data loggers. Environ. Toxicol. Chem. 37, 2881–2894. doi: 10.1002/etc.4250
Blévin, P., Shaffer, S. A., Bustamante, P., Angelier, F., Picard, B., Herzke, D., et al. (2020). Contaminants, prolactin and parental care in an Arctic seabird: contrasted associations of perfluoroalkyl substances and organochlorine compounds with egg-turning behavior. Gen. Comp. Endocrinol. 291:113420. doi: 10.1016/j.ygcen.2020.113420
Blévin, P., Tartu, S., Angelier, F., Leclaire, S., Bustnes, J. O., Moe, B., et al. (2014). Integument colouration in relation to persistent organic pollutants and body condition in arctic breeding black-legged kittiwakes (Rissa tridactyla). Sci. Total Environ. 47, 248–254. doi: 10.1016/j.scitotenv.2013.09.049
Bortolotti, G. R., Fernie, K. Y., and Smiths, J. E. (2003). Carotenoid concentration and coloration of American Kestrels (Falco sparverius) disrupted by experimental exposure to PCBs. Funct. Ecol. 17, 651–657.
Bossi, R., Riget, F. F., Dietz, R., Sonne, C., Fauser, P., Dam, M., et al. (2005). Preliminary screening of perfluoroctane sulfonate (PFOS) and other fluorochemicals in fish, birds and marine mammals from Greenland and the Faroe Islands. Environ. Pollut. 136, 323–329. doi: 10.1016/j.envpol.2004.12.020
Braune, B. M., Trudeau, S., Jeffrey, D. A., and Mallory, M. L. (2011). Biomarker responses associated with halogenated organic contaminants in northern fulmars (Fulmarus glacialis) breeding in the Canadian Arctic. Environ. Pollut. 159, 2891–2898. doi: 10.1016/j.envpol.2011.04.036
Burnham, K. P., and Anderson, D. R. (2002). Model Selection and Multimodel Inference: a Practical Information-theoretic Approach, 2nd Edn. New York, NY: Springer-Verlag.
Bustamante, P., Lahaye, V., Durnez, C., Churlaud, C., and Caurant, F. (2006). Total and organic Hg concentrations in cephalopods from the North Eastern Atlantic waters: influence of geographical origin and feeding ecology. Sci. Total Environ. 368, 585–596. doi: 10.1016/j.scitotenv.2006.01.038
Bustnes, J. O., Erikstad, K. E., Lorentsen, S. H., and Herzke, D. (2008). Perfluorinated and chlorinated pollutants as predictors of demographic parameters in an endangered seabird. Environ. Pollut. 156, 414–424. doi: 10.1016/j.envpol.2008.01.028
Casagrande, S., Costantini, D., Fanfani, A., Tagliavini, J., and Dell’Omo, G. (2007). Patterns of serum carotenoid accumulation and skin colour variation in nestling kestrels in relation to breeding conditions and different terms of carotenoid supplementation. J. Comp. Physiol. B 177, 237–245. doi: 10.1007/s00360-006-0125-4
Costantini, D., Blévin, P., Herzke, D., Moe, B., Gabrielsen, G. W., Bustnes, J., et al. (2019). Higher plasma oxidative damage and lower plasma antioxidant defences in an Arctic seabird exposed to longer perfluoroalkyl acids. Environ. Res. 168, 278–285. doi: 10.1016/j.envres.2018.10.003
Costantini, D., and Møller, A. P. (2008). Carotenoids are minor antioxidants for birds. Funct. Ecol. 22, 367–370.
Dale, K., Yadetie, F., Müller, M. B., Pampanin, D. M., Gilabert, A., Zhang, X., et al. (2020). Proteomics and lipidomics analyses reveal modulation of lipid metabolism by perfluoroalkyl substances in liver of Atlantic cod (Gadus morhua). Aquat. Toxicol. 227:105590. doi: 10.1016/j.aquatox.2020.105590
Doutrelant, C., Grégoire, A., Gomez, D., Staszewski, V., Arnoux, E., Tveraa, T., et al. (2013). Colouration in Atlantic puffins and blacklegged kittiwakes: monochromatism and links to body condition in both sexes. J. Avian Biol. 44, 451–460.
Evers, D. C., Savoy, L. J., DeSorbo, C. R., Yates, D. E., Hanson, W., Taylor, K. M., et al. (2008). Adverse effects from environmental mercury loads on breeding common loons. Ecotoxicology 17, 69–81. doi: 10.1007/s10646-007-0168-7
Fridolfsson, A.-K., and Ellegren, H. (1999). A simple and universal method for molecular sexing of non-ratite birds. J. Avian Biol. 30, 116–121.
Giraudeau, M., Mateos-Gonzalez, F., Cotín, J., Pagani-Núñez, E., Torné-Noguera, A., and Senar, J. C. (2015). Metal exposure influences the melanin and carotenoid-based colorations in Great Tits. Sci. Total Environ. 532, 512–516. doi: 10.1016/j.scitotenv.2015.06.021
Glüge, J., Scheringer, M., Cousins, I. T., DeWitt, J. C., Goldenman, G., Herzke, D., et al. (2020). An overview of the uses of per- and polyfluoroalkyl substances (PFAS). Environ. Sci. Process Impacts 22, 2345–2373. doi: 10.1039/d0em00291g
Gomez, D. (2006). AVICOL, a program to analyse spectrometric data. Available online at: http://sites.google.com/site/avicolprogram/
Goutte, A., Angelier, F., Chastel, C. C., Trouvé, C., Moe, B., Bech, C., et al. (2010). Stress and the timing of breeding: glucocorticoid-luteinizing hormones relationships in an arctic seabird. Gen. Comp. Endocrinol. 169, 108–116. doi: 10.1016/j.ygcen.2010.07.016
Goutte, A., Bustamante, P., Barbraud, C., Delord, K., Weimerskirch, H., and Chastel, O. (2014a). Demographic responses to mercury exposure in two closely-related Antarctic top predators. Ecology 95, 1075–1086. doi: 10.1890/13-1229.1
Goutte, A., Barbraud, C., Meillere, A., Carravieri, A., Bustamante, P., Labadie, P., et al. (2014b). Demographic consequences of heavy metals and persistent organic pollutants in a vulnerable long-lived bird, the wandering albatross. Proc. R. Soc. Lond. B 281:20133313. doi: 10.1098/rspb.2013.3313
Haarr, A., Hylland, K., Eckbo, N., Gabrielsen, G. W., Herzke, D., Bustnes, J. O., et al. (2018). DNA damage in Arctic seabirds: baseline, sensitivity to a genotoxic stressor, and association with organohalogen contaminants. Environ. Toxicol. Chem. 37, 1084–1091. doi: 10.1002/etc.4035
Hammer, Ø, Harper, D. A. T., and Ryan, P. D. (2001). PAST: paleontological statistics software package for education and data analysis. Palaeontol. Electron. 4, 1–9.
Helfenstein, F., Losdat, S., Møller, A. P., Blount, J. D., and Richner, H. (2010). Sperm of colourful males are better protected against oxidative stress. Ecol. Lett. 13, 213–222.
Hill, G. E., and McGraw, K. J. (2006a). Bird Coloration. Mechanisms and Measurements, vol. I. Cambridge: Harvard University Press.
Hill, G. E., and McGraw, K. J. (2006b). Bird Coloration. Function and evolution, vol. II. Cambridge: Harvard University Press.
Honda, M., Muta, A., Akasaka, T., Inoue, Y., Shimasaki, Y., Kannan, K., et al. (2014). Identification of perfluorooctane sulfonate binding protein in the plasma of tiger pufferfish Takifugu rubripes Ecotoxicol. Environ. Saf. 104, 409–413. doi: 10.1016/j.ecoenv.2013.11.010
Hutton, P., and McGraw, K. (2016). Urban impacts on oxidative balance and animal signals. Front. Ecol. Evol. 4:54. doi: 10.3389/fevo.2016.00054
James, R. A., Hertz-Picciotto, I., Willman, E., Keller, J. A., and Judith Charles, M. (2002). Determinants of serum polychlorinated biphenyls and organochlorine pesticides measured in women from the child health and development study cohort, 1963–1967. Environ. Health Perspect. 110, 617–624. doi: 10.1289/ehp.02110617
Koch, R. E., and Hill, G. E. (2018). Do carotenoid-based ornaments entail resource trade-offs? an evaluation of theory and data. Funct. Ecol.? 32, 1908–1920.
Lau, C., Anitole, K., Hodes, C., Lai, D., Pfahles-Hutchens, A., and Seed, J. (2007). Perfluoroalkyl acids: a review of monitoring and toxicological findings. Toxicol. Sci. 99, 366–394.
Leclaire, S., Bourret, V., Blanchard, P., de Franceschi, C., Merkling, T., Hatch, S. A., et al. (2015). Carotenoids increase immunity and sex specifically affect color and redox homeostasis in a monochromatic seabird. Behav. Ecol. Sociobiol. 69, 1–15.
Leclaire, S., Bourret, V., Pineaux, M., Blanchard, P., Danchin, E., and Hatch, S. A. (2019). Red coloration varies with dietary carotenoid access and nutritional condition in kittiwakes. J. Exp. Biol. 222:jeb210237. doi: 10.1242/jeb.210237
Leclaire, S., White, J., Arnoux, E., Faivre, B., Vetter, N., Hatch, S. A., et al. (2011). Integument coloration signals reproductive success, heterozygosity, and antioxidant levels in chick-rearing black-legged kittiwakes. Naturwissenschaften 98, 773–782. doi: 10.1007/s00114-011-0827-7
Lifshitz, N., and St Clair, C. C. (2016). Coloured ornamental traits could be effective and non-invasive indicators of pollution exposure for wildlife. Conserv. Physiol. 4:cow028. doi: 10.1093/conphys/cow028
Liu, G., Zhang, B., Hu, Y., Rood, J., Liang, L., Qi, L., et al. (2020). Associations of perfluoroalkyl substances with blood lipids and apolipoproteins in lipoprotein subspecies: the POUNDS-lost study. Environ. Health 19:5. doi: 10.1186/s12940-020-0561-8
Lüdecke, D., Ben-Shachar, M., Patil, I., Waggoner, P., and Makowski, D. (2021). performance: an R package for assessment, comparison and testing of statistical models. J. Open Source Software 6:3139.
Marasco, V., and Costantini, D. (2016). Signaling in a polluted world: oxidative stress as an overlooked mechanism linking contaminants to animal communication. Front. Ecol. Evol. 4:95. doi: 10.3389/fevo.2016.00095
McCullagh, E. A., Cristol, D. C., and Phillips, J. B. (2015). Plumage color and reproductive output of eastern bluebirds (Sialia sialis) nesting near a mercury-contaminated river. J. Environ. Sci. Health A 50, 1020–1028. doi: 10.1080/10934529.2015.1038168
Melnes, M., Gabrielsen, G. W., Herzke, D., Sagerup, K., and Jenssen, B. M. (2017). Dissimilar effects of organohalogenated compounds on thyroid hormones in glaucous gulls. Environ. Res. 158, 350–357. doi: 10.1016/j.envres.2017.06.007
Montgomerie, R. (2006). “Analyzing colors,” in Bird Coloration. Mechanisms and Measurements, Vol. I, eds G. E. Hill and K. J. McGraw (Cambridge: Harvard University Press), 90–147.
Muir, D. C., and de Wit, C. A. (2010). Trends of legacy and new persistent organic pollutants in the circumpolar arctic: overview, conclusions, and recommendations. Sci. Total Environ. 408, 3044–3051. doi: 10.1016/j.scitotenv.2009.11.032
Nøst, T. H., Helgason, L. B., Harju, M., Heimstad, E. S., Gabrielsen, G. W., and Jenssen, B. M. (2012). Halogenated organic contaminants and their correlations with circulating thyroid hormones in developing Arctic seabirds. Sci. Total Environ. 414, 248–256. doi: 10.1016/j.scitotenv.2011.11.051
Olson, V. A., and Owens, I. P. F. (2005). Interspecific variation in the use of carotenoid-based coloration in birds: diet, life history and phylogeny. J. Evol. Biol. 18, 1534–1546. doi: 10.1111/j.1420-9101.2005.00940.x
R Core Team (2021). R: A Language and Environment for Statistical Computing. Vienna: R Foundation for Statistical Computing.
Simons, M. J. P., Cohen, A. A., and Verhulst, S. (2012). What does carotenoid-dependent coloration tell? plasma carotenoid level signals immunocompetence and oxidative stress state in birds–a meta-analysis. PLoS One 7:e43088. doi: 10.1371/journal.pone.0043088
Soldatini, C., Sebastiano, M., Albores-Barajas, Y. V., AbdElgawadmm, H., Bustamante, P., and Costantini, D. (2020). Mercury exposure in relation to foraging ecology and its impact on the oxidative status of an endangered seabird. Sci. Total Environ. 724:138131. doi: 10.1016/j.scitotenv.2020.138131
Spickler, J. L., Swaddle, J. P., Gilson, R. L., Varian-Ramos, C. W., and Cristol, D. A. (2020). Sexually selected traits as bioindicators: exposure to mercury affects carotenoid-based male bill color in zebra finches. Ecotoxicology 29, 1138–1147. doi: 10.1007/s10646-020-02271-8
Tartu, S., Angelier, F., Wingfield, J. C., Bustamante, P., Labadie, P., Budzinski, H., et al. (2015). Corticosterone, prolactin and egg neglect behavior in relation to mercury and legacy POPs in a long-lived Antarctic bird. Sci. Total Environ. 505, 180–188. doi: 10.1016/j.scitotenv.2014.10.008
Tartu, S., Bustamante, P., Angelier, F., Lendvai, A. Z., Moe, B., Blevin, P., et al. (2016). Mercury exposure, stress and prolactin secretion in an Arctic seabird: an experimental study. Funct. Ecol. 30, 596–604.
Tartu, S., Gabrielsen, G. W., Blévin, P., Ellis, H., Bustnes, J. O., Herzke, D., et al. (2014a). Endocrine and fitness correlates of long-chain perfluorinated carboxylates exposure in Arctic breeding black-legged kittiwakes. Environ. Sci. Technol. 48, 13504–13510. doi: 10.1021/es503297n
Tartu, S., Angelier, F., Herzke, D., Moe, B., Bech, C., Gabrielsen, G. W., et al. (2014b). The stress of being contaminated? adrenocortical function and reproduction in relation to persistent organic pollutants in female black legged kittiwakes. Sci. Total Environ. 47, 553–560. doi: 10.1016/j.scitotenv.2014.01.060
Tartu, S., Goutte, A., Bustamante, P., Angelier, F., Moe, B., Clément-Chastel, C., et al. (2013). To breed or not to breed: endocrine response to mercury contamination by an Arctic seabird. Biol. Lett. 9: 20130317.
Townhill, B. L., Reppas-Chrysovitsinos, E., Sühring, R., Halsall, C. J., Mengo, E., Sanders, T., et al. (2022). Pollution in the Arctic Ocean: an overview of multiple pressures and implications for ecosystem services. Ambio 51, 471–483. doi: 10.1007/s13280-021-01657-0
Van Hout, A. J.-M., Eens, M., and Pinxten, R. (2011). Carotenoid supplementation positively affects the expression of a non-visual sexual signal. PLoS One 6:e16326. doi: 10.1371/journal.pone.0016326
von Schantz, T., Bensch, S., Grahn, M., Hasselquist, D., and Wittzell, H. (1999). Good genes, oxidative stress and condition-dependent sexual signals. Proc. R. Soc. Lond. B 266, 1–12.
Wang, L., Wang, Y., Liang, Y., Li, J., Liu, Y., Zhang, J., et al. (2014). PFOS induced lipid metabolism disturbances in BALB/c mice through inhibition of low density lipoproteins excretion. Sci. Rep. 4:4582. doi: 10.1038/srep04582
White, A. E., and Cristol, D. A. (2014). Plumage coloration in belted kingfishers (Megaceryle alcyon) at a mercury-contaminated river. Waterbirds 37, 144–152.
Whitney, M. C., and Cristol, D. A. (2017). Impacts of sublethal mercury exposure on birds: a detailed review. Rev. Environ. Contam. Toxicol. 244, 113–163. doi: 10.1007/398_2017_4
Yanai, J., Dotan, S., Goz, R., Pinkas, A., Seidler, F. J., Slotkin, T. A., et al. (2008). Exposure of developing chicks to perfluorooctanoic acid induces defects in prehatch and early posthatch development. J. Toxicol. Environ. Health Part A 71, 131–133. doi: 10.1080/15287390701613280
Keywords: carotenoids, mate choice, mercury, PFAS, PFOSlin, seabirds, sexual selection, stressors
Citation: Costantini D, Blévin P, Bustnes JO, Esteve V, Gabrielsen GW, Herzke D, Humann-Guilleminot S, Moe B, Parenteau C, Récapet C, Bustamante P and Chastel O (2022) Integument carotenoid-based colouration reflects contamination to perfluoroalkyl substances, but not mercury, in arctic black-legged kittiwakes. Front. Ecol. Evol. 10:952765. doi: 10.3389/fevo.2022.952765
Received: 25 May 2022; Accepted: 13 September 2022;
Published: 30 September 2022.
Edited by:
Celine Arzel, University of Turku, FinlandReviewed by:
Claire Bottini, Western University, CanadaMorgan Gilmour, United States Geological Survey (USGS), United States
Copyright © 2022 Costantini, Blévin, Bustnes, Esteve, Gabrielsen, Herzke, Humann-Guilleminot, Moe, Parenteau, Récapet, Bustamante and Chastel. This is an open-access article distributed under the terms of the Creative Commons Attribution License (CC BY). The use, distribution or reproduction in other forums is permitted, provided the original author(s) and the copyright owner(s) are credited and that the original publication in this journal is cited, in accordance with accepted academic practice. No use, distribution or reproduction is permitted which does not comply with these terms.
*Correspondence: David Costantini, david.costantini@mnhn.fr