The Life Cycle of the Bacterial-Feeding Nematode Diplolaimella stagnosa and Its Population Growth in Response to Temperature and Food Availability
- College of Life and Environmental Science, Hangzhou Normal University, Hangzhou, China
Diplolaimella is a ubiquitous cosmopolitan genus, but information on the life cycles of its species is limited. Here, we describe the life cycle of a free-living bacterivorous nematode, Diplolaimella stagnosa, and report the effects of temperature and food availability on its population dynamics. Specimens were primarily collected from the intertidal zone of Hangzhou Bay Wetland, China and culture experiments were conducted in nutrient agar media with habitat water at 20°C. The nematode primarily fed on an unidentified bacterium that it carried. Under these conditions, both males and females matured in 16 days. Reproduction was by gamogenesis and gravid females normally carried 7–8 eggs. Embryogenesis was completed in 58 h, and the entire life cycle (egg to adult) was completed in 16–18 days. During juvenile development, body lengths of worms increased linearly up to the 16th day, and then remained constant. Body lengths of males and females were 898.1 ± 6.0 μm and 1039.7 ± 14.7 μm, respectively. Nematodes kept at 25°C had a greater population increase than those at 20°C, and the population growth of nematodes was substantially higher in microcosms with abundant bacteria supplied by leaves of Phragmites australis than in microcosms without plant litter. Based on its life strategy and the influence of food availability and temperature on population growth, D. stagnosa was allocated to group c-p 2, suggesting its potential use as a model organism in toxicological studies.
Introduction
The life history of an organism reflects the previous tradeoffs made between current and future fitness components and has great significance for population dynamics (Beckerman et al., 2002). Ecologists have long recognized the importance of studying life history and population dynamic of organisms along environmental gradients to understand, predict and mediate distribution and growth of species (Hall et al., 1992). Previous studies focused on animals and plants in aboveground ecosystems (Peterman and Semlitsch, 2013). Life history of soil fauna and density effects of the environment have remained largely unstudied (Bardgett and van der Putten, 2014). Soil fauna play important roles in material cycling and energy flow (Hoogen et al., 2019). Therefore, such research is crucial to agriculture, natural ecosystem management and soil bioremediation.
Nematodes occur in every niche that provides an available source of organic carbon, and they are the most diverse and abundant soil metazoan taxa, often reaching densities between 105 and 108 individuals per square meter (Heip et al., 1985; Bongers and Ferris, 1999; Lambshead and Boucher, 2003). Based on their feeding habits, soil nematodes are classified into plant-feeding, fungal-feeding, bacterial-feeding, and omnivorous groups, with bacterial-feeding species dominant in soils (Yeates et al., 1993). Bacterivorous nematodes play significant functional roles in soil ecosystems. For example, they affect the abundance, activity, and community composition of soil microorganisms, and they participate in nutrient decomposition and recycling processes, both directly and indirectly. By feeding on bacteria and facilitating the mineralization of organic matter, nematodes increase the pH of micro-environments (Xiao et al., 2014).
Little information is currently available on the life history and culture methods of free-living bacterivorous nematodes, which limits estimation of energy flows and carrying capacities of ecosystems, and modeling population-level responses to toxicants (Tominaga et al., 2003; Kreuzinger-Janik et al., 2017). Therefore, it is important to obtain more information on the biology of these organisms, particularly their life history. The life-history properties of nematodes are driven by various abiotic factors, such as temperature, food availability, salinity and toxicants. Temperature is fundamental to the physiology of living organisms (e.g., growth and development; Clarke, 2006; Vafeiadou and Moens, 2021). In general, population growth become more rapid and life cycle was shorter with increased temperatures (Tietjen and Lee, 1972; Hopper et al., 1973). In addition, De Meester et al. (2015) investigated the impact of temperature and salinity on the life history of four nematode cryptic species of Litoditis marina (Pm I, Pm II, Pm III, and Pm IV), and the results showed that the life history characters were affected by abiotic factors, with temperature significantly having a stronger effect than salinity. Several life-history traits did not clearly exhibit a food-availability dependence, but population biomass of Pellioditis marina was the highest at the highest food availability (Dos Santos et al., 2008).
Diplolaimella is a cosmopolitan genus inhabiting a wide range of environments, including lotic freshwater, inland saline waters, groundwater, and coastal water systems (Pastor de Ward and Lo Russo, 2009). In addition, Diplolaimella is a dominant genus in the sea and wetlands, and it is an important decomposer in the intertidal zone. Most studies on this genus have concentrated on the associated microbial community and on decomposition processes in the litter. Diplolaimella have often been found that they can stimulate decomposition processes. Diplolaimella chitwoodi increased the carbon mineralization rate of Gracilaria detritus and Spartina detritus by 300 and 50%, respectively (Findlay and Tenore, 1982). Bioturbation activity of the bacterivorous nematode Diplolaimella dievengatensis enhanced Spartina detritus decomposition by increasing oxygen diffusion and carbon dioxide production (Alkemade et al., 1992). Laboratory microcosm experiments have shown that bacterivorous nematodes can have a significant top-down influence on the bacterial community structure growing on the detritus, even at low densities (De Mesel et al., 2004). Diplolaimella stagnosa was key species in litter decomposition of Phragmites australis in salt marshes of the Yangtze River estuary (Chen et al., 2007). However, there were few reports concerning this species, the life histories and population dynamics of this species under field or laboratory conditions need further study.
The aim of the present paper is to study the life cycle of Diplolaimella stagnosa, especially its embryonic development and the population growth in relation to temperature and food availability. The life history of the nematodes was observed continuously under a microscope and photographed daily. We constructed microcosms to study the effects of temperature and food availability on nematode population growth. Therefore, this work is not only important for understanding the life history characteristics of nematode species, but also for estimating whether D. stagnosa has the potential to a critical model organism for further studies.
Materials and Methods
Sampling
Sediment samples for this experiment were collected at a depth of 10 cm using clean plastic scoops during low tide in the intertidal zone of Hangzhou Bay Wetland, China, on July 1, 2020. The samples were placed in specimen bags for transfer to the laboratory, and stored at 10°C before extraction of nematodes. For the litter samples, leaves of P. australis, were removed with scissors and transferred to laboratory, where they were cut into 1 cm long pieces and dried to constant weight.
Nematode Culture
Nematodes were extracted with a Baermann funnel, and D. stagnosa were manually picked out from the filtrate under a dissection microscope based on their buccal morphology and body volume. The nematodes were transferred to 60-mm petri dishes containing nutrient media for culture without addition of bacteria. The culture experiment was conducted in the dark at 20°C. When a high population density was reached, small pieces of medium were cut and transferred to new medium, ensuring that nematodes used in experiments were obtained from established monospecific, agnotobiotic cultures in exponential growth phase, with an unidentified bacterium carried over from the habitat as food (Moens and Vincx, 1998). Nematodes were cultured continuously for several generations prior to the start of the experiments.
Life Cycle
Diplolaimella stagnosa females on culture plates were inspected weekly with a dissection microscope until gravid specimens were found. Pregnant females were then handpicked and placed in new identical petri dishes. A total of 20 numbered petri dishes each containing one gravid female were checked daily under the dissection microscope to determine when eggs were laid. Subsequently, the embryonic development of the nematodes was observed continuously under a microscope (BX41, Olympus Corporation, Tokyo, Japan) until the eggs hatched. High-resolution digital images of each embryonic stage were generated at maximal possible magnification using NIS-Elements imaging system, ver. 2.2.0 (Nikon Instruments, Melville, NY, United States), and measurements of their lengths and widths were carried out daily using Image-Pro Plus 6.0 software (Media Cybernetics, Inc., Rockville, MD, United States).
After the eggs hatched, juveniles were transferred to individual petri dishes with media. They were photographed daily at 100 × magnification, and their lengths determined until they had reached adult size and had laid eggs.
Population Growth
Microcosms were constructed to study the effects of temperature and food availability on nematode population growth. Temperatures were set at 20°C, the temperature at which nematodes are normally reared in the laboratory, and at 25°C for the high-temperature treatment. Two levels of food availability were established: (1) High food availability with abundant microbes provided by the addition of P. australis leaf litter; and (2) low food availability without added P. australis. Accurately weighed 1-g portions of P. australis leaves were placed in labeled culture dishes. Habitat water was percolated through 0.45 μm water filter paper. Then, 20 mL of filtered habitat water was added and the leaves, which were soaked overnight. Ten D. stagnosa were inoculated into each petri dish on the following day. The habitat water was then replenished and made up to 25 mL. The weights of microcosms were recorded before placing them in the artificial climate chamber for culture. Microecological dishes without reed leaves were set up simultaneously for the control treatments. Both groups were incubated at two temperatures (20 and 25°C) in darkness, and each treatment had six parallel samples. Lost water was replenished every 5 days during the experiment. Nematodes in microcosms were counted after 16, 32, 48, and 64 days. To quantify the number of nematodes, the litter was washed to concentrate them onto a counting plate.
Data Analysis
We used the generalized additive model “gamma” in the mgcv package in R version 3.1.1 (R Core Team, 2021) to fit the nematode growth curve, using body length as the response variable and time (0–19 days) as the predictor.
Three-way ANOVA and Tukey’s HSD tests based on general linear models in SPSS 17.0 were performed to inspect effects of temperature (20 vs. 25°C), food availability (with vs. without leaf litter), and sampling time (after 16, 32, 48, and 64 days) on nematode population density. To meet the assumptions of ANOVA, nematode numbers were logarithm transformed. Differences were considered statistically significant when P < 0.05.
Results
Embryogenesis of Diplolaimella stagnosa
The eggs of D. stagnosa were oblong in shape and covered with a thin egg membrane. They were single-celled when laid by pregnant females (Figure 1Aa). Approximately 1 h after spawning, the first cleavage occurred, and the eggs split into two unequal cells. The 2-cell stage (Figure 1Bb) continued for 2 h until one of the blastomeres divided to form the 3-cell stage (Figure 1Cc). Approximately 0.66 h after the 3-cell stage, the embryo was observed to enter the 4-cell stage (Figure 1Dd). Most eggs in this period exhibited the conventional appearance of four-celled diamonds. After a further 2 h, cleavage occurred again, and the embryos entered the 5-cell stage (Figure 1Ee). The speed of cell division increased during this period, and the multicellular stage was reached 1 h later (Figure 1Ff). After another 11 h, the inner cell population (endoderm) within the embryo was surrounded by a layer of transparent cells (ectoderm), and the embryos entered the blastocyst stage (Figure 1Gg). Gastrulation started after approximately 14 h after the blastocyst stage (Figure 1Hh), and 10.5 h after gastrulation, the vermiform stage appeared (Figure 1Ii), at which time continuous motion of the embryo in the shell was clearly observed. Approximately 16 h after the vermiform stage, one end of eggshell broke, and the nematode hatched. Figure 2 illustrates the mean duration of the embryonic developmental stages of D. stagnosa. Embryonic development was competed in approximately 58 h.
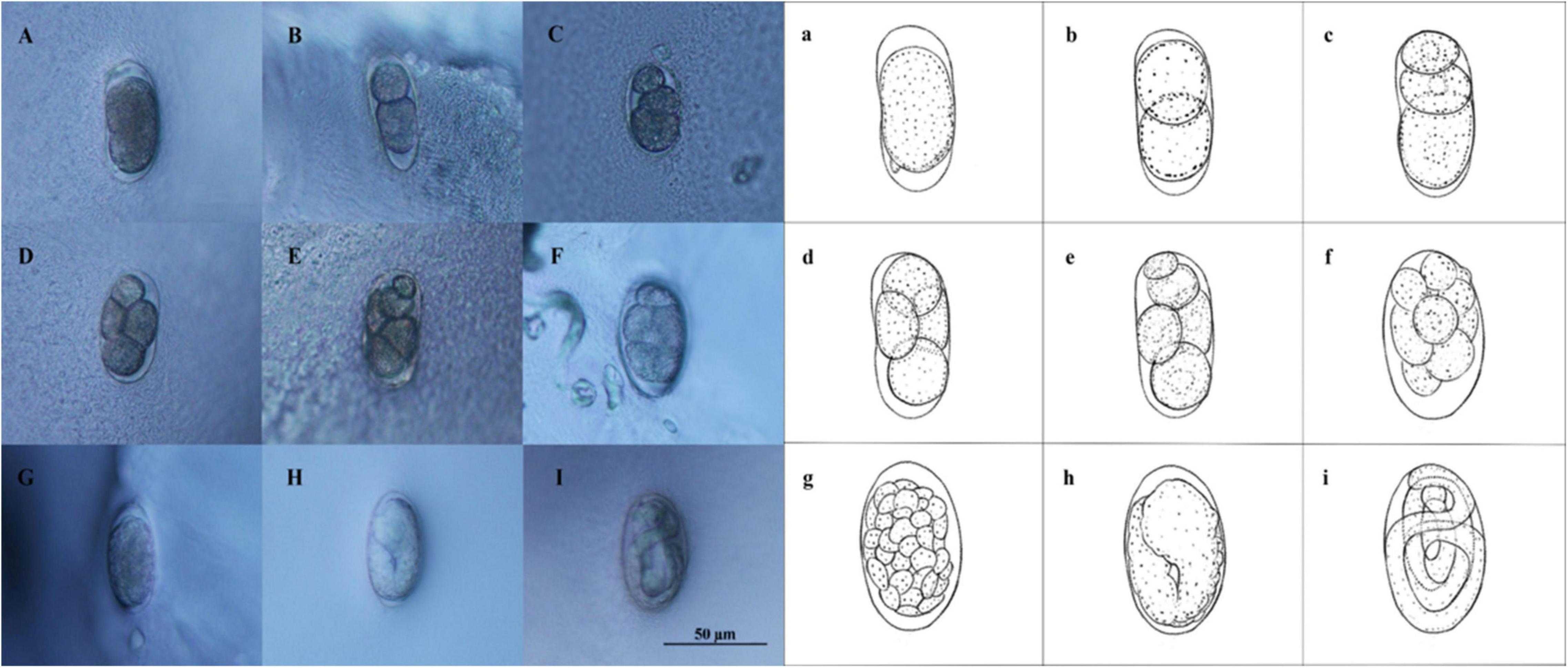
Figure 1. The embryonic development stages of Diplolaimella stagnosa. (Aa) Single cell stage; (Bb), two cells stage; (Cc), three cells stage; (Dd), four cells stage; (Ee), five cells stage; (Ff), multicellular stage; (Gg), blastula stage; (Hh), gastrula stage (tadpole-shaped stage); (Ii), worm stage.
Egg diameter, and the length of each developmental stage, were measured (Table 1). At each stage up to the multicellular stage, the length of the major axis progressively increased, and the width of the minor axis decreased. Thereafter, the minor axis width trended upward, while the major axis continued to increase during the blastocyst and gastrula stages, but decreased somewhat in the worm stage. The shortening of the major axis was associated with the motion of the nematode during the worm phase.
Post Embryonic Development
After hatching from the egg, juveniles are transparent with a mean length of 258.34 ± 9.18 μm. Figure 3 shows the somatic growth curves of D. stagnosa at 20°C. During juvenile development of both males and females, body length increased up to approximately 16 days, and then remained constant.
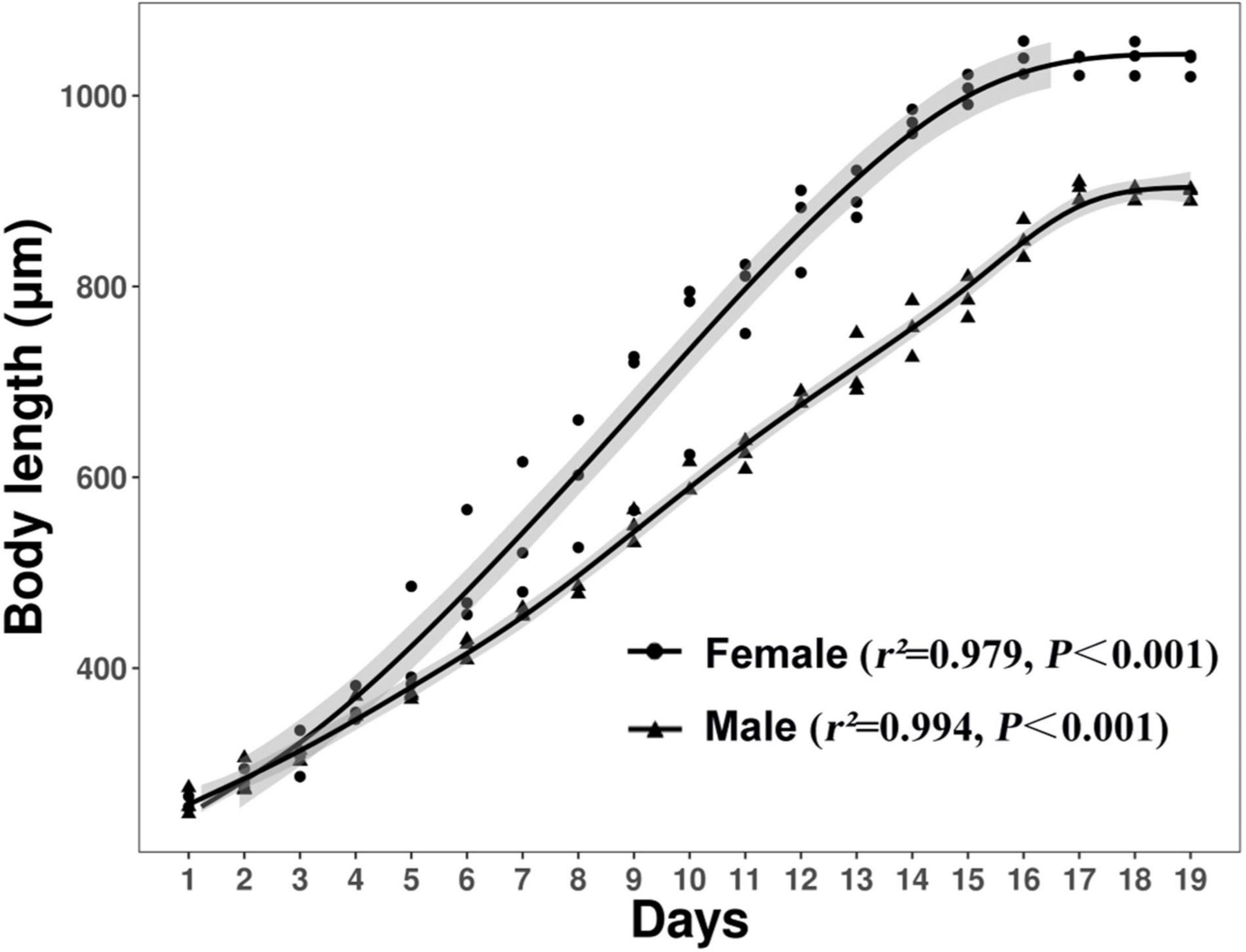
Figure 3. Somatic length-based growth curves of Diplolaimella stagnosa during the life-cycle experiment. Fitted curves (solid lines) of body length vs. time plus corresponding 95% confidence intervals (shading) for male and female are plotted. The approximation coefficient r2 and regression coefficient P are also provided. The growth rate was calculated on a daily basis.
The onset of sexual maturity coincided with the end of the increase in body length. Gravid females generally carried 7–8 eggs. The average length of adult D. stagnosa was 0.7–1.0 mm (Figures 3, 4). However, the mean length of mature females was significantly greater than that of males. Figure 4B shows an entire mature male of D. stagnosa; the arrow indicates the spicule; the mean body length of males was 898.1 ± 6.0 μm. Figure 4C illustrates a female of D. stagnosa; the vulva (arrow) is located anteriorly in the mid-body; the mean length of females was 1039.7 ± 14.7 μm. A double buccal cavity was clearly visible in the head region of the nematode. The spicule is arcuate and robust (Fonseca and Decraemer, 2008), and < 25 μm long. The male of D. stagnosa possesses three pairs of postcloacal papillae of which the posteriormost pair is more prominent than the anterior papillae, in contrast to other Diplolaimella species. Diplolaimella species are characterized by the absence of ocelli (Jacobs et al., 1990).
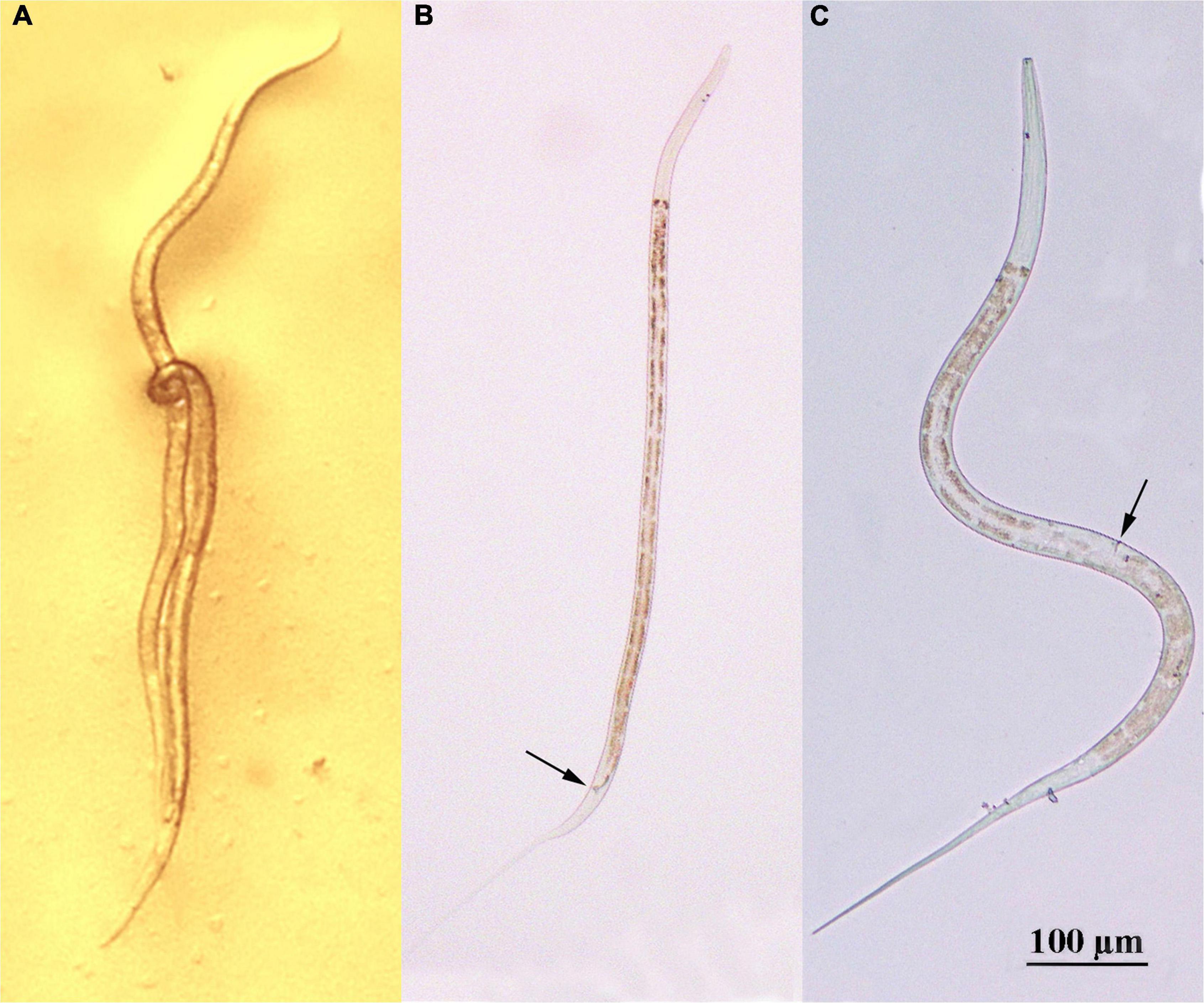
Figure 4. Adults of Diplolaimella stagnosa. (A) Mating worms (the curly one is male, another one is female) male; (B) male; (C) female.
Response to Abiotic Factors
The life history traits of nematodes are differentially affected by abiotic factors. In the present study, the population growth of D. stagnosa varied remarkably between treatments (Table 2). Food availability showed a significant effect on the density of nematodes (P < 0.001; Table 2). Subsequent analysis proved that the treatments with P. australis did not have a significant effect on the density of D. stagnosa compared with the control group on the 16th day (Figure 5). However, on the 32nd day, nematode densities in the treatments with P. australis were 35.1 times higher than the initial number, which in other treatments only 1.55 times. The growth rate of the former (10.97 nematodes per dish per day) was clearly higher than the latter (0.48 nematodes per dish per day). On the 48th day and the 64th day, the presence of litter also caused a significantly higher number of nematodes than the control group and at day 64 there were 176.27 times as many nematodes in the adding litter treatments (2908.5 nematodes per dish) as in the control group (16.5 nematodes per dish). Three-way analysis of variance (ANOVA) showed that temperature did not significantly affect the density of D. stagnosa (Table 2). However, the interactive effect of temperature, food availability and time on the density of D. stagnosa was significant (P < 0.001; Table 2). Subsequent analysis showed no significant differences between the four groups on the 16th day (Figure 5). However, on the 32nd day, the nematode densities at 25°C were significantly higher than that at 20°C regardless of the presence of litter (Figure 5). On the 64th day, densities were also clearly higher at 25°C than that at 20°C in the presence of litter, and in the absence of litter, the result reversed (Figure 5).
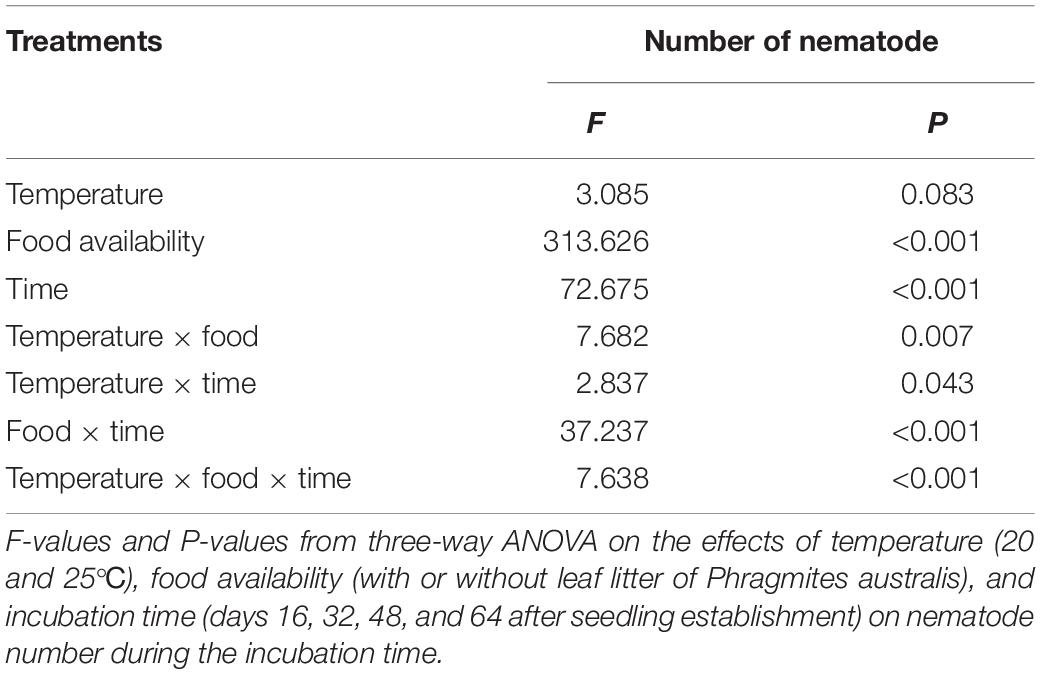
Table 2. Effects of three factors (temperature, food availability, and time) on density of Diplolaimella stagnosa.
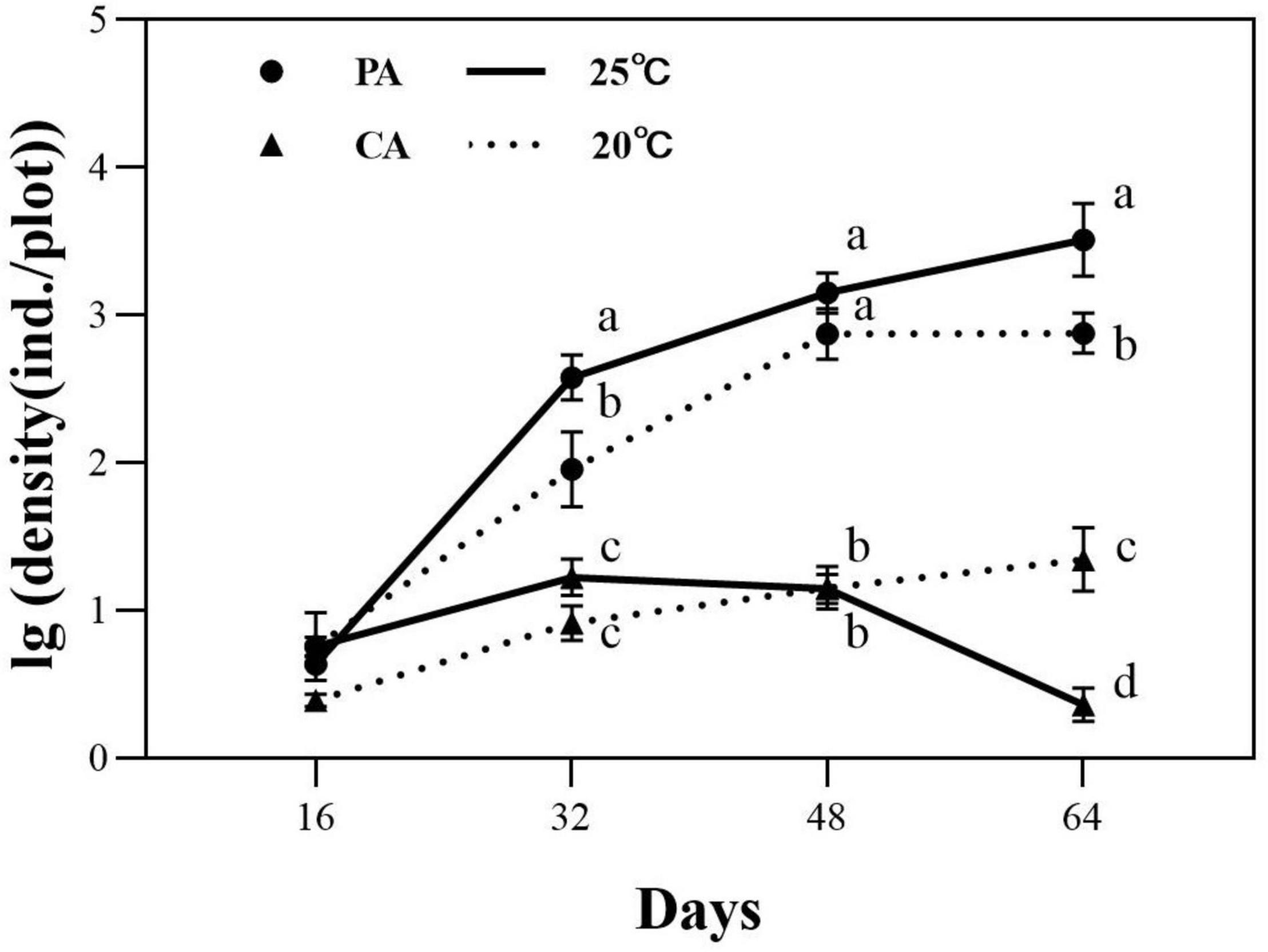
Figure 5. Population growth of nematodes in different treatments. PA, Phragmites australis; CA: without litter. Different lowercase letters (a–d) indicate that there are significant differences among the treatments at each observation time (P < 0.05).
Discussion
The variations in dimensions were probably related to cell division. The egg initially became elongated during cell division but became more rounded after a several divisions and differentiation. Previous studies of nematodes have not noted these changes in the major and minor axes of the egg at different stages while reported only average values of the axes during the whole embryonic developmental process. Our data indicate that the eggs of D. stagnosa are ellipsoids. Tominaga et al. (2003) showed that the zygote of C. elegans is elliptical in outline, whereas the unfertilized egg is globular because of cell polarity. However, no globular eggs were observed in our experiments.
Nematodes grow in size between molts and after the last molt (Muschiol and Traunspurger, 2007). It has not been well established whether juvenile growth of nematodes is generally continuous or intermittent. Muschiol and Traunspurger (2007) showed that growth rates of Panagrolaimus sp. and Poikilolaimus sp. were continuous. The relatively smooth and continuous growth curves of C. elegans imply that the four molts do not represent major growth discontinuities (Byerly et al., 1976). Nonetheless, many species of nematodes have a period of lethargy, with little or no growth, before and during molting (Lee, 2002). The growth rate of Monhystera denticulata showed obvious stagnation at molting (Tietjen and Lee, 1972). It has also been reported that the growth curve of Plectus murrayi was discontinuous at molting (Adhikari et al., 2010). Data in the present study suggest that D. stagnosa growth is a continuous process. Although Wang and Zhang (1994) showed that Diplolaimella sp. undergoes four molts before maturation, no obvious molting was observed for D. stagnosa in our experiment. These findings suggested that each molting event took place relatively quickly and that the observation interval of 24 h was too long to detect slight fluctuations in growth rate (Lee, 2002).
We observed the copulating D. stagnosa and provided the direct evidence of bisexual reproduction. In contrast, a study reported that Diplolaimella sp. was successfully cultured in the laboratory, but no male individuals were found after three successive generations of culture, and the reproduction was by parthenogenesis (Wang and Zhang, 1994). Furthermore, both females and males of D. stagnosa were observed in our experiments, indicating that D. stagnosa is dioecious. Intriguingly, unmated mature females have also been observed with eggs inside their abdomens, but whether parthenogenesis is possible remains determined.
Based on their life strategy, nematodes have been allocated on a continuum from colonizers to persisters (corresponding loosely to r- and K-strategists), with a range of colonizer–persister (c–p) values from 1 to 5 (Bongers, 1990). Diplolaimella species were originally scaled as c-p 1 (Bongers et al., 1991). However, Monhysteridae were transferred to c-p 2 by Bongers and Bongers (1998). It also appropriate to allocate D. stagnosa to c-p 2 because of its short generation time and high reproductive rate. Ferris et al. (2001) assigned nematodes to functional guilds due to their feeding habits and c–p-values and provided weightings of the guilds along their structure and enrichment trajectories. D. stagnosa is a bacterivorous nematode, and the weighting of the guild Ba2 is 0.8 (Ferris et al., 2001).
Our results indicated that, in general, the life history traits of nematodes are influenced by different abiotic factors. Within a suitable temperature range, the life cycles of six species of marine nematodes (Diplolaimella ocellata, Diplolaimelloides sp., Rhabditis marina, Enoplus paralittoralis, Onsholaimus sp., and Haliplectus dorsali) shortened with increasing temperature (Hopper et al., 1973). The effect of temperature and salinity on the life histories of two brackish-water bacterivorous nematode species, Displolaimelloides meyli and P. marina, was investigated; temperature had greater effects than salinity on development times, fecundity, and sex ratio in both nematodes (Moens and Vincx, 2000). Likewise, the life-cycle parameters of three cryptic species of the Halomonhystera disjuncta complex indicated a stronger effect on temperature than on salinity (Jelle et al., 2014; De Meester et al., 2015); lower temperature lengthened the minimum generation time and adult life span (Jelle et al., 2014). By studying the effects of food availability on population growth and different life-history traits in three species of bacterial-feeding nematodes (Diplolaimelloides oschii, Diplolaimelloides meyli, and P. marina), it was found that the two Diplolaimelloides species exhibited the highest population increase at intermediate food availabilities, while the population growth of P. marina peaked at the highest food availability (Dos Santos et al., 2008). The abundances of Panagrolaimus cf. thienemanni and Poikilolaimus cf. regenfussi differed significantly in response to the different food-supply levels, and both reached their maximal abundances when the bacterial densities were the highest (Gansfort et al., 2018). Similarly, in our experiments, the density of D. stagnosa at the high food concentration after 48 days at 25°C was approximately three times that at the low food concentration (Figure 5). Nematodes are highly species-specific in their sensitivity to abiotic factors, such as pollutants, food availability and environmental disturbance, which may relate to different life-history traits (Bongers and Bongers, 1998; Dos Santos et al., 2008).
Because of its short life cycle (3 days at 20°C), C. elegans has been used in many studies as an efficient model for assessment of the toxicity of chemicals at low concentrations (Donkin and Dusenbery, 1993; Tominaga et al., 2003; Dengg and van Meel, 2004). Similarly, Tietjen and Lee (1984) used D. punicea as a bioassay organism for estuarine sediments because of the advantageous characteristics of its life cycle (short generation time, life history and small size). According to Vranken and Heip (1986), exposure of Diplolaimella sp. to toxic levels of copper, lead, and mercury inhibited its development and fecundity. Such suppression was considered reliable criteria for determining cutoff values for environmental safety. Likewise, in less than 3 weeks, D. stagnosa completed its life cycle (from egg hatching to maturity) and was relatively easy to maintain in laboratory culture. Therefore, we suggest that this species would also be suitable for evaluating effects of specific toxicants in natural sediments on populations, rather than on individuals (Tietjen and Lee, 1984). To facilitate wider use of D. stagnosa in pollution assays, further study should investigate whether it spontaneously carries bacteria, attempt to identify and culture the bacteria, and determine their influence on the worm’s growth and development. In addition, research should focus on this nematode’s responses to pollution, its feeding preferences, and the design of an appropriate artificial sea water for the use of this species in subsequent studies. With further research, D. stagnosa has the potential for its use as a model organism of the genus.
Data Availability Statement
The raw data supporting the conclusions of this article will be made available by the authors, without undue reservation.
Author Contributions
JcZ: conceptualization, methodology, investigation, formal analysis, resources, data curation, and writing—original draft, review and editing. JnZ: conceptualization, methodology, data curation, formal analysis, and writing—original draft. HC: conceptualization, methodology, investigation, resources, data curation, supervision, and writing—review and editing. XZ: methodology, investigation, resources, and data curation. BJ: conceptualization, methodology, supervision, and writing—review and editing. All authors contributed to the article and approved the submitted version.
Funding
This study was supported by the Basic and Commonweal Programme of Zhejiang Province (LGN21C140009), the National Natural Science Foundation of China (31770534), and the Students’ Innovation and Entrepreneurship Training Project of China (202010346049).
Conflict of Interest
The authors declare that the research was conducted in the absence of any commercial or financial relationships that could be construed as a potential conflict of interest.
Publisher’s Note
All claims expressed in this article are solely those of the authors and do not necessarily represent those of their affiliated organizations, or those of the publisher, the editors and the reviewers. Any product that may be evaluated in this article, or claim that may be made by its manufacturer, is not guaranteed or endorsed by the publisher.
Acknowledgments
We thank Jiaming Zhang and Haixiang Dai for aid with the start of Diplolaimella stagnosa culture. We thank Fangning Yang and Meifang Jiang for help with quantifying the number of nematodes. We also thank Harry Taylor for polishing the English text of a draft of this manuscript.
References
Adhikari, B. N., Tomasel, C. M., Li, G., Wall, D. H., and Adams, B. J. (2010). The Antarctic nematode Plectus murrayi: an emerging model to study multiple stress survival. Cold Spring Harb. Protoc. 2010:pdbemo142. doi: 10.1101/pdb.emo142
Alkemade, R., Wielemaker, A., Jong, S. A., and Sandee, A. (1992). Experimental evidence for the role of bioturbation by the marine nematode Diplolaimella dievengatensis in stimulating the mineralization of Spartina anglica detritus. Mar. Ecol. Prog. Ser. 90, 149–155. doi: 10.3354/meps090149
Bardgett, R. D., and van der Putten, W. H. (2014). Belowground biodiversity and ecosystem functioning. Nature 515, 505–511. doi: 10.1038/nature13855
Beckerman, A., Benton, T. G., Ranta, E., Kaitala, V., and Lundberg, P. (2002). Population dynamic consequences of delayed life-history effects. Trends Ecol. Evol. 17, 263–269. doi: 10.1016/s0169-5347(02)02469-2
Bongers, A. M. T. (1990). The maturity index: an ecological measure of environmental disturbance based on nematode species composition. Oecologia 83, 14–19. doi: 10.1007/BF00324627
Bongers, A. M. T., Alkemade, R., and Yeates, G. (1991). Interpretation of disturbance-induced maturity decrease in marine nematode assemblages by means of the Maturity Index. Mar. Ecol. Prog. Ser. 76, 135–142. doi: 10.3354/meps076135
Bongers, T., and Bongers, M. (1998). Functional diversity of nematodes. Appl. Soil Ecol. 10, 239–251. doi: 10.1016/S0929-1393(98)00123-1
Bongers, T., and Ferris, H. (1999). Nematode community structure as a bioindicator in environmental monitoring. Trends Ecol. Evol. 14, 224–228. doi: 10.1016/s0169-5347(98)01583-3
Byerly, L., Scherer, S., and Russell, R. (1976). The life cycle of the nematode Caenorhabditis elegans. II. A simplified method for mutant characterization. Dev. Biol. 51, 34–48. doi: 10.1016/0012-1606(76)90119-6
Chen, H., Li, B., Fang, C., Chen, J., and Wu, J. (2007). Exotic plant influences soil nematode communities through litter input. Soil Biol. Biochem. 39, 1782–1793. doi: 10.1016/j.soilbio.2007.02.011
Clarke, A. (2006). Temperature and the metabolic theory of ecology. Funct. Ecol. 20, 405–412. doi: 10.1111/j.1365-2435.2006.01109.x
De Meester, N., Derycke, S., Rigaux, A., and Moens, T. (2015). Temperature and salinity induce differential responses in life histories of cryptic nematode species. J. Exp. Mar. Biol. Ecol. 472, 54–62. doi: 10.1016/j.jembe.2015.07.002
De Mesel, I., Derycke, S., Moens, T., Van der Gucht, K., Vincx, M., and Swings, J. (2004). Top-down impact of bacterivorous nematodes on the bacterial community structure: a microcosm study. Environ. Microbiol. 6, 733–744. doi: 10.1111/j.1462-2920.2004.00610.x
Pastor de Ward, C. T., and Lo Russo, V. (2009). Distribution of Diplolaimella and Diplolaimelloides species from Patagonian lagoons and coastal waters (Nematoda: monhysteridae), Chubut and Santa Cruz provinces (Argentina). J. Mar. Biol. Assoc. U. K. 89, 711–718. doi: 10.1017/s0025315409000198
Dengg, M., and van Meel, J. C. (2004). Caenorhabditis elegans as model system for rapid toxicity assessment of pharmaceutical compounds. J. Pharmacol. Toxicol. Methods 50, 209–214. doi: 10.1016/j.vascn.2004.04.002
Donkin, S. G., and Dusenbery, D. B. (1993). A soil toxicity test using the nematode Caenorhabditis elegans and an effective method of recovery. Arch. Environ. Contam. Toxicol. 25, 145–151. doi: 10.1007/BF00212125
Dos Santos, G. A. P., Derycke, S., Fonsêca-Genevois, V. G., Coelho, L. C. C. B., Correia, M. T. S., and Moens, T. (2008). Differential effects of food availability on population growth and fitness of three species of estuarine, bacterial-feeding nematodes. J. Exp. Mar. Biol. Ecol. 355, 27–40. doi: 10.1016/j.jembe.2007.11.015
Ferris, H., Bongers, T., and de Goede, R. G. M. (2001). A framework for soil food web diagnostics: extension of the nematode faunal analysis concept. Appl. Soil Ecol. 18, 13–29. doi: 10.1016/S0929-1393(01)00152-4
Findlay, S., and Tenore, K. R. (1982). Effect of a Free-Living Marine Nematode (Diplolaimella chitwoodi) on Detrital Carbon Mineralization. Mar. Ecol. Prog. Ser. 8, 161–166. doi: 10.3354/meps008161
Fonseca, G., and Decraemer, W. (2008). State of the art of the free-living marine Monhysteridae (Nematoda). J. Mar. Biol. Assoc. U. K. 88, 1371–1390. doi: 10.1017/s0025315408001719
Gansfort, B., Uthoff, J., and Traunspurger, W. (2018). Interactions among competing nematode species affect population growth rates. Oecologia 187, 75–84. doi: 10.1007/s00442-018-4109-3
Hall, C. A. S., Stanford, J. A., and Hauer, F. R. (1992). The Distribution and Abundance of Organisms as a Consequence of Energy Balances along Multiple Environmental Gradients. Oikos 65, 377–390. doi: 10.2307/3545553
Heip, C. H. R., Vincx, M., and Vranken, G. (1985). The ecology of marine nematodes. Oceanogr. Mar. Biol. 23, 399–489.
Hoogen, J., Geisen, S., Routh, D., Ferris, H., and Crowther, T. W. (2019). Soil nematode abundance and functional group composition at a global scale. Nature 572, 194–198. doi: 10.1038/s41586-019-1418-6
Hopper, B., Fell, J. W., and Cefalu, R. C. (1973). Effect of temperature on life cycles of nematodes associated with the mangrove (Rhizophora mangle) detrital system. Mar. Biol. 23, 293–296. doi: 10.1007/BF00389336
Jacobs, L. J., Van De Velde, M. C., Geraert, E., and Vranken, G. (1990). Description of Diplolaimella Dievengatensis Sp. N. (Nematoda: monhysteridae). Nematology 36, 1–21. doi: 10.1163/002925990X00013
Jelle, V. C., Sofie, D., Tom, M., Ann, V., and Diego, F. (2014). Differences in life-histories refute ecological equivalence of cryptic species and provide clues to the origin of Bathyal Halomonhystera (Nematoda). PLoS One 9:e111889. doi: 10.1371/journal.pone.0111889
Kreuzinger-Janik, B., Brinke, M., Traunspurger, W., and Majdi, N. (2017). Life history traits of the free-living nematode, Plectus acuminatus Bastian, 1865, and responses to cadmium exposure. Nematology 19, 645–654. doi: 10.1163/15685411-00003077
Lambshead, P. J., and Boucher, G. (2003). Marine nematode deep-sea biodiversity - Hyperdiverse or hype? J. Biogeogr. 30, 475–485. doi: 10.1046/j.1365-2699.2003.00843.x
Lee, D. L. (2002). “Cuticle, Moulting and Exsheathment,” in The Biology of Nematodes, ed. D. L. Lee (London, FL: Taylor and Francis), 171–209.
Moens, T., and Vincx, M. (1998). On the cultivation of free-living marine and estuarine nematodes. Helgoland Mar. Res. 52, 115–139. doi: 10.1007/BF02908742
Moens, T., and Vincx, M. (2000). Temperature and salinity constraints on the life cycle of two brackish-water nematode species. J. Exp. Mar. Biol. Ecol. 243, 115–135. doi: 10.1016/S0022-0981(99)00113-6
Muschiol, D., and Traunspurger, W. (2007). Life cycle and calculation of the intrinsic rate of natural increase of two bacterivorous nematodes, Panagrolaimus sp. and Poikilolaimus sp. from chemoautotrophic Movile Cave, Romania. Nematology 9, 271–284. doi: 10.1163/156854107780739117
Peterman, W. E., and Semlitsch, R. D. (2013). Fine-scale habitat associations of a terrestrial salamander: the role of environmental gradients and implications for population dynamics. PLoS One 8:e62184. doi: 10.1371/journal.pone.0062184
R Core Team (2021). R: A Language and Environment for Statistical Computing. R Foundation for Statistical Computing. Available online at: http://www.R-project.org/ (accessed February, 2022).
Tietjen, J. H., and Lee, J. J. (1972). Life cycles of marine nematodes. Oecologia 10, 167–176. doi: 10.1007/BF00347988
Tietjen, J. H., and Lee, J. J. (1984). The use of free-living nematodes as a bioassay for estuarine sediments. Mar. Environ. Res. 11, 233–251. doi: 10.1016/0141-1136(84)90037-0
Tominaga, N., Kohra, S., and Arizono, K. (2003). A Multi-Generation Sublethal Assay of Phenols Using the Nematode Caenorhabditis elegans. Mar. Environ. Res. 49, 459–463. doi: 10.1248/jhs.49.459
Vafeiadou, A. M., and Moens, T. (2021). Effects of temperature and interspecific competition on population fitness of free-living marine nematodes. Ecol. Indic. 120:106958. doi: 10.1016/j.ecolind.2020.106958
Vranken, G., and Heip, C. (1986). Toxicity of copper, mercury and lead to a marine nematode. Mar. Pollut. Bull. 17, 453–457. doi: 10.1016/0025-326X(86)90834-9
Wang, S., and Zhang, Z. (1994). Experimental culture and cife cycle of marine nematodes from prawn cultivation pond. J. Ocean Univ. Qingdao 12, 206–213.
Xiao, H., Li, G., Li, D., Hu, F., and Li, H. (2014). Effect of Different Bacterial-Feeding Nematode Species on Soil Bacterial Numbers, Activity, and Community Composition. Pedosphere 24, 116–124. doi: 10.1016/S1002-0160(13)60086-7
Keywords: bacterivorous, Diplolaimella stagnosa, embryogenesis development, life history, population dynamics
Citation: Zhao J, Zhang J, Zhu X, Lu J, Jin B and Chen H (2022) The Life Cycle of the Bacterial-Feeding Nematode Diplolaimella stagnosa and Its Population Growth in Response to Temperature and Food Availability. Front. Ecol. Evol. 10:953608. doi: 10.3389/fevo.2022.953608
Received: 26 May 2022; Accepted: 20 June 2022;
Published: 30 June 2022.
Edited by:
Lin Zhang, Hubei University of Chinese Medicine, ChinaReviewed by:
Yang Gao, Xi’an University of Technology, ChinaChengyuan Xu, Central Queensland University, Australia
Copyright © 2022 Zhao, Zhang, Zhu, Lu, Jin and Chen. This is an open-access article distributed under the terms of the Creative Commons Attribution License (CC BY). The use, distribution or reproduction in other forums is permitted, provided the original author(s) and the copyright owner(s) are credited and that the original publication in this journal is cited, in accordance with accepted academic practice. No use, distribution or reproduction is permitted which does not comply with these terms.
*Correspondence: Binsong Jin, jin.binsong@gmail.com; Huili Chen, huilichen@hznu.edu.cn