Identification of key areas for ecological restoration of territorial space based on ecological security pattern: a case study of Kangbao County
- 1School of Land Science and Spatial Planning, Hebei GEO University, Shijiazhuang, China
- 2International Science and Technology Cooperation Base of Hebei Province, Hebei International Joint Research Center for Remote Sensing of Agricultural Drought Monitoring, Hebei GEO University, Shijiazhuang, China
- 3Academy of Eco-Civilization Development for Jing-Jin-Ji Megalopolis, Tianjin Normal University, Tianjin, China
- 4Natural Resources Big Data Application Center, Hebei Geographic Information Group Co., Ltd, Shijiazhuang, China
Accurate identification of key areas for ecological restoration is an important foundation for orderly promotion of ecological restoration of territorial space. This study identified the key areas for ecological restoration in Kangbao County according to the research paradigm of “source identification – resistance surface construction – corridor extraction”. First, ecological source sites were identified and screened based on habitat quality modeling, morphological spatial pattern analysis and landscape connectivity analysis. Then, the base resistance surface was constructed using ArcGIS software and nighttime light data. Thereafter, ecological corridors were extracted and ecological pinch points and ecological barrier points identified with the Linkage Mapper plug-in. Forty ecological source sites with a total area of 68.06 km2 were identified in Kangbao County. These sites were dominated by woodland and grassland, and the integrity of these ecological source sites still needed to be improved. The 96 ecological corridors (total length of 743.81 km) were identified, which were densely distributed in the south and east parts of the study area, and there is still much room for improvement of the habitat connectivity. Additionally, 75 ecological pinch points (total area of 31.72 km2) and 69 ecological obstacles (total area of 16.42 km2) were identified. The key areas to be restored were mainly distributed in Yan Yufang, Har Chimega, Tuchengzi, Zhangji, and Danchenghe Townships. This study can lay a firm foundation for the ecological restoration in Kangbao County.
1 Introduction
Territorial space is the material basis for the construction of an ecological civilization, formed by the interaction between the ecosystem and human society (Dai et al., 2021; Li et al., 2021). China’s long-term rough economic development model has led to a series of resource and environmental problems such as soil erosion, desertification, degradation of grassland and forest vegetation, and pollution of soil, water, and air (Di Giulio et al., 2009; Solow, 2017; Wang et al., 2019; Tutak et al., 2021; He et al., 2023). Therefore, the ecological restoration of land space has attracted increasing attention, and it is urgent to carry out ecological restoration (Yuan et al., 2022). The foundation for carrying out ecological restoration of Territorial space is to identify key areas for ecological restoration. In particular, Yu (1996) proposed the theory and method of identifying key areas for ecological restoration by constructing an ecological security pattern. This method comprehensively considers ecological landscape elements, spatial location, and connectivity strength and can effectively enhance the integrity of ecosystem structure and function, which provides an important way to identify key areas for ecological restoration in territorial space.
In recent years, research concerning the ecological security pattern has increasingly focused on ecological restoration of territorial space, and the basic research paradigm of “source identification – resistance surface construction – corridor extraction” has gradually formed (Liu and Chang, 2015; Ma et al., 2019; Peng et al., 2020). There are roughly two methods for identifying ecological sources: the most direct and simplest method is to select green spaces, water bodies, protected areas, mountain areas, and scenic sites as ecological sources (Vergnes et al., 2013; Zhao and Xu, 2015). Another method is to construct a comprehensive evaluation index system to identify ecological sources, where ecological sensitivity, ecological functions, landscape connectivity, ecological risk and habitat importance are generally involved, among which the habitat importance has been widely used (Peng et al., 2018a; Huang et al., 2020; Wang et al., 2022a; Ran et al., 2022). As an ecosystem service evaluation tool, the Integrated Valuation of Ecosystem Services and Trade-offs (InVEST) model can quantitatively assess the habitat quality, carbon storage and fixation, soil conservation and other service functions of ecosystems, identify ecological source areas based on landscape functional attributes (Rahimi et al., 2020; Zhang et al., 2020a; Zhang et al., 2020b; Zawadzka et al., 2021). The MSPA model can accurately distinguish the types and structures of landscapes, and has been widely used in the construction of ecological security patterns in recent years (Wang et al., 2022b; Yang et al., 2022). Based on the comprehensive application of the Integrated Valuation of Ecosystem Services and Trade off model and morphological spatial pattern analysis, this study considers landscape connectivity and identifies ecological sources by combining the functionality, structure, and connectivity of landscapes. Resistance surface construction studies usually select land use types and natural background characteristics as resistance factors, and use nighttime light data, Impervious Surface Index, traffic, and topography to correct the resistance surface to better reflect the impact of anthropogenic interference on the ecosystem (Zhu et al., 2020; Jin et al., 2021; Wang et al., 2022a). The ability of species to migrate and exchange is not only influenced by land types, but also influenced by factors such as human activities. Nighttime light data can accurately reflect the economic development situation, energy consumption, urbanization level, and other human activity factors (Zhang et al., 2016), and therefore the nighttime light data can be used to accurately determine the spatial pattern of human activity intensity levels. Methods for ecological corridor extraction generally include the minimum cumulative resistance, colony model, and circuit theory (Peng et al., 2019; Wang et al., 2020; Dai et al., 2021; Zhang et al., 2021). In particular, the circuit theory can overcome the limitation that the least cumulative resistance model only identifies the least-cost paths. Additionally, advantages of the circuit theory include the ability to explore the width of the corridor and accurate identification of the location of the nodes (McRae and Beier, 2007; McRae et al., 2008; Dilt et al., 2016; Peng et al., 2018b; Dickson et al., 2019; Huang et al., 2022; Zhang et al., 2022; Wu et al., 2023). Due to these characteristics of the circuit theory, studies of ecological security pattern based on the research paradigm of “source identification – resistance surface construction – corridor extraction” have generally included the identification of ecological obstacle points – irreplaceable key areas in the corridor, and ecological “pinch points”, areas in the ecological corridor that impede the flow of organisms (McRae et al., 2008; McRae et al., 2012; Cushman et al., 2013). Ecological pinch points and ecological obstacle points are both key aspects for ecological restoration of territorial space.The findings contributed to forming a more perfect research paradigm of “source identification – resistance surface construction – corridor extraction – key point identification” (Xu et al., 2022; Wu et al., 2023). While many studies have been performed, fewer studies have addressed ecological restoration of territorial space at the county scale based on the framework of “source identification – resistance surface construction – corridor extraction – key point identification”.
Kangbao County is an important part of China’s “Capital Water Cultivation Functional Area and Ecological Environment Support Area”. Kangbao County is also a national key ecological functional area, shouldering the construction of the Beijing-Tianjin-Hebei Ecological Protection Barrier meant to guarantee regional ecological security. In recent years, irrational resource utilization, development, and construction practices have seriously degraded and fragmented the county’s ecosystem. The forest coverage is now <30%, structure of forest stands is unreasonable, overall quality of arable land is poorer, and soil erosion and siltation of reservoirs are more serious. The contradiction between ecological environmental protection and economic development is becoming increasingly prominent, and ecological restoration of the national land space is an urgent priority. From the perspective of ecological security pattern, accurately identifying the key areas of ecological restoration in the country is necessary for ecological conservation in the region.
This study identified key areas for ecological restoration of territorial space in Kangbao County based on the ecological security pattern and the research paradigm of “source identification – resistance surface construction – corridor extraction – key point identification”. This study utilized the habitat quality model, morphological spatial pattern analysis and landscape connectivity analysis to comprehensively identify ecological sources. Then, this study constructed a comprehensive ecological resistance surface based on the minimum cumulative resistance (MCR) model and utilized nighttime light data to correct the basic ecological resistance surface to obtain the integrated ecological resistance surface. This study thereafter extracted the ecological corridors by combining with the circuit theory and finally identified the ecological pinch points and ecological obstacle points. The findings of this study can lay a firm foundation for a proposed restoration strategy for the key areas of ecological restoration in the territorial space. This study can also provide a theoretical basis for holistic and systematic ecological restorations of territorial space in Kangbao County, and reference data for other county scale land space ecological restoration studies.
2 Materials and methods
2.1 Study area
Kangbao County is located in the northwestern part of Hebei Province (114°11′–114°56′ E, 41°25′–42°08′ N) (Figure 1). The county has a total area of 3,365.67 km2. Kangbao County includes seven towns, eight townships, and two township-level units, with a total population of 269,600 in 2021. Located in the southeastern edge of the Inner Mongolia Plateau, the terrain from the northeast to the southwest is gently tilted, with diverse geomorphological types arranged in a well-organized manner from the northeast to the southwest of the ladder-type arrangement of the gradual decline. Kangbao County is in the temperate sub-arid zone of the East Asian continental monsoon climate, with no perennial rivers and rainfall as the main source of water. The whole area has 3169.93 km2 of agricultural and forest land, 88.99 km2 of construction land, and 106.75 km2 of other land (Figure 2). Kangba Nuoer National Wetland Park in Kangbao County is a natural plateau lake wetland with a well-protected ecosystem. The total area of 368.1 hectares includes 220 hectares of marsh wetlands.
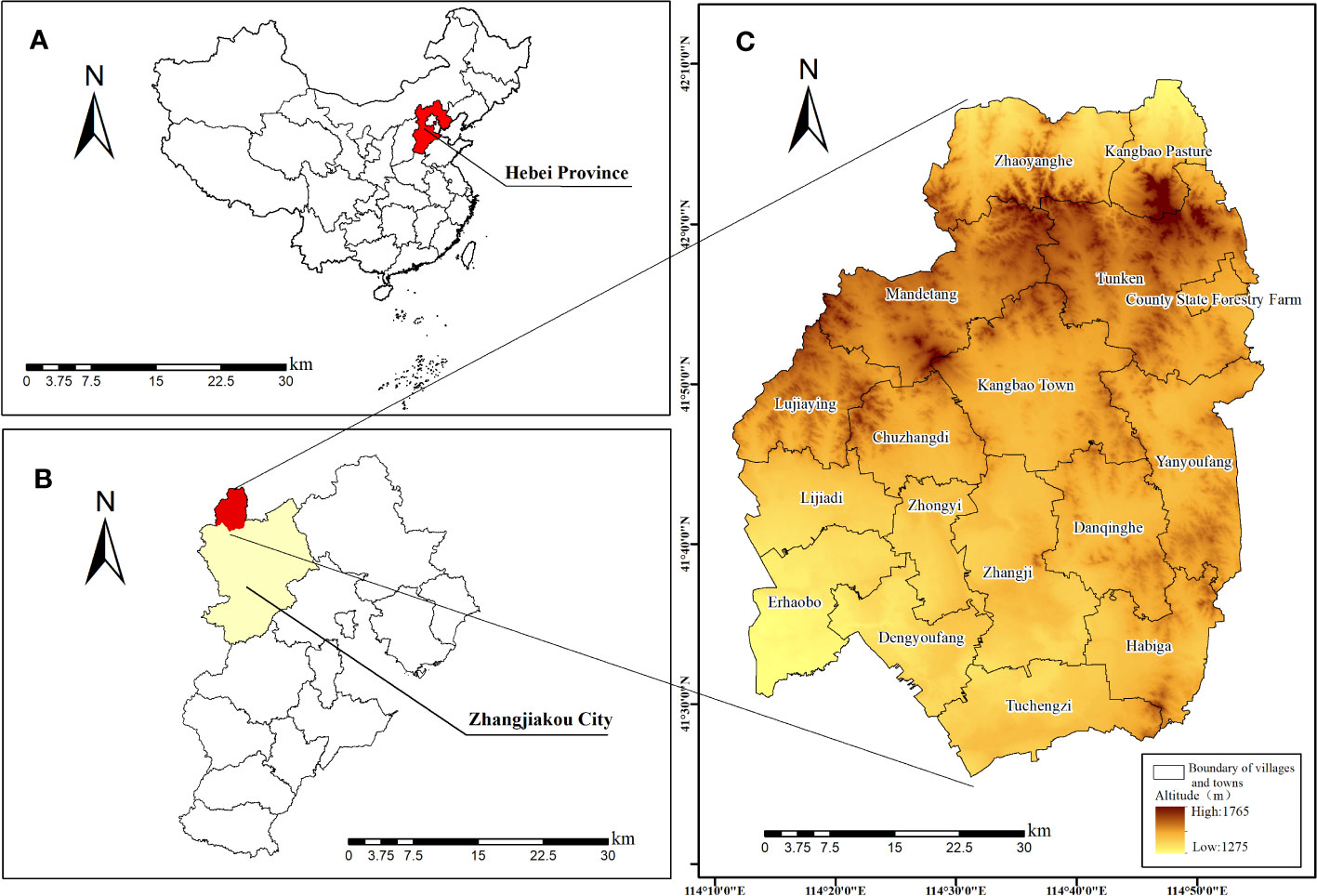
Figure 1 Overview of the study area: (A) geographical location, (B) geographical location, (C) elevation.
2.2 Methods
2.2.1 Research framework
Based on ecological theory, this study utilizes the ecological security pattern to identify key areas for ecological restoration of territorial space at the landscape level. Three methods of habitat quality, morphological spatial pattern analysis, and landscape connectivity analysis were integrated in the ecological source area determination. The habitat quality index (HQI) of Kangbao County was calculated based on the habitat quality module in the InVEST model. Then, the morphological spatial pattern analysis method was used to analyze the landscape type, in which the core area was the potential ecological source area. Finally, the landscape connectivity analysis method was used to calculate the probable connectivity index of the patches and the importance index to realize the screening of the ecological source area patches. In order to simulate the distribution of resistance surfaces more accurately, based on the traditional explicit resistance surfaces based on land use types, implicit resistance surfaces, slope, and undulation factors were introduced to construct the base resistance surfaces. In order to more accurately simulate the distribution of resistance surfaces, on the basis of explicit resistance surfaces which is based on land use types, implicit resistance surfaces, slope, and undulation factors were introduced to construct the base resistance surfaces. The nighttime lighting index, which characterizes human activities, was used to correct the resistance surface. Based on the ecological source and comprehensive ecological resistance surface, the ecological corridor is extracted by combining the MCR model and the Linkage Mapper plug-in in the circuit theory. The ecological “pinch point” area and the ecological “obstacle point” area were identified to complete the construction of the ecological security pattern. Finally, ecological restoration strategies are proposed to address the problems in different regions. The research framework is shown in Figure 3.
2.2.2 Ecological source area identification
2.2.2.1 Habitat quality assessment based on the InVEST model
The HQI reflects the impact of human activities on the ecosystem; the greater the intensity of human activities, the greater the threat to the habitat and the lower its quality. Ecological source areas are places where the structure and function of ecosystems are relatively stable. These areas play an important role in maintaining ecosystem stability and providing high-quality ecosystem services and products (Yu, 1996). Considering the spatial structure of landscape elements and the importance of service functions, this paper comprehensively identifies patches with important ecosystem services, good connectivity, and a certain area as ecological source sites from three aspects: habitat quality, MSPA model and landscape connectivity. Specifically, in this study, based on the distribution of the integrated HQI and the results of the MSPA analysis, we selected the area where the high-quality habitat area intersects with the core area and screened out patches with an area < 0.01 km2, to reduce the negative impacts of the fine patches on the entire ecological source area, and to determine the preliminary ecological source area. In addition, with reference to the relevant literature, forest land, watershed, and grassland were selected as habitats, and transportation, urban villages, industrial and mining land, water conservancy facility land, cultivated land, and facility agricultural land were selected as habitat threat sources. The specific parameter settings are shown in Tables 1, 2 (Zhang and Fang, 2021). In this study, the Habitat Quality module of the InVEST model was used to estimate the HQI for Kangbao County using Equation 1 and Equation 2 (Berta Aneseyee et al., 2020; Zhang et al., 2020; Li et al., 2021).
where Qxj is the habitat quality of grid x in land use type j, and Hxj is the habitat suitability of grid x in landtype j, with land types determined with 2020 Kangbao County Current Land Use data at a spatial resolution of 30 m (data were provided by the Kangbao County Department of Natural Resources and Planning); k is the half-saturation constant, which is half of the highest habitat degradation value; z is the normalization constant, which takes the default value of 2.5; Dxj is the degree of habitat degradation of grid x in land type j; R is the number of threat factors; Yr is the total number of grid cells for the threat factor; Wr is the weight; ry is the number of threat factors on the grid cell; irxy is the influence distance of the threat factor; βx is the reachability level of the grid x; and Sjr is the sensitivity of the land type to the threat factor. In this study, the initial value of the half-saturation parameter was set as 0.5 to obtain the maximum degradation fraction of 0.706. Then, the k value was set to half of the maximum degradation fraction (0.353) for the second adjustment of the parameter. The results of the calculations were classified into five categories using the natural breakpoint method, which finally resulted in the distribution map of the HQI in the study area.
2.2.2.2 Landscape pattern analysis based on MSPA
MSPA was used to accurately identify landscape types that play an important role in maintaining landscape connectivity and do not overlap with each other (Vogt et al., 2009). Based on the current land use status classification standard, the land use types in the study area were classified into seven categories: cropland, forest land, garden land, grassland, watershed, construction land, and unutilized land. The three types of natural landscapes with high ecological service values, including forest land, grassland, and watershed, were taken as the foreground of the MSPA. The rest of the land use categories were taken as the background (Zhu et al., 2020). In order to preserve the small but important landscape elements in the study area, the grid cell size was set to 30 m × 30 m. The grid data were analyzed using Guidos Toolbox software, and the eight-neighborhood analysis was used to obtain seven landscape types that did not overlap with each other, including the core area, isolated island, pore, edge area, bridging area, traffic circle area, and spur (Zhang et al., 2017).
2.2.2.3 Landscape connectivity analysis
Landscape connectivity can reflect the level of connectivity between core patches within a region, which is key to maintaining ecosystem stability and wholeness, and is an important indicator of landscape patterns and ecological processes (An et al., 2021; Xu et al., 2022). In this study, Conefor 2.6 and Conefor Inputs for the ArcGIS plug-in were used to calculate Probability of Connectivity and patch significance index (dPC) for each patch to screen the initially identified ecological source sites. The patch connectivity distance threshold was set to 2500 m and the connectivity probability was set to 0.5. PC and dPC are calculated by Equation 3 and Equation 4 (Saura and Rubio, 2010; Cao et al., 2022).
Where PC is the landscape possible connectivity index; n denotes the total number of ecological patches; ai, aj is the area of ecological patches i and j, respectively; pij is the maximum value of the final connectivity of all paths between patch i and patch j; AL is the total area of the study area; dPC is the value of the importance of the connectivity of the patches, and PCremove is the connectivity index of the landscape after removing the patches.
2.2.3 Integrated ecological resistance surface construction
Explicit resistance surfaces and implicit resistance surfaces were constructed from the perspectives of patch extent and interactions of ecological resistance between patches, respectively. The weights of factors and resistance coefficients were determined with reference to relevant research results (Merrick and Koprowski, 2017; Wang et al., 2020). The results are shown in Table 3. Among these aspects, for explicit resistance surface construction, this study only considered land use types and assigned ecological resistance values to each type of patch. For implicit resistance surface construction, the first step was the extraction of the center of mass of each patch using ArcGIS. Then, ecological resistance values were assigned to the corresponding centers of mass of different land use types. Finally, comprehensive ecological resistance surfaces were constructed by Kriging interpolation. In addition, the slope and topographic relief were calculated for the elevation data using ArcGIS software to represent the topographic conditions, in which the digital elevation model data was SRTM V4.1 data with a spatial resolution of 90 m (the data were provided by the Center for Resource and Environmental Science and Data, Institute of Geoscience and Resources, Chinese Academy of Sciences). Finally, the resistance surfaces constructed by each factor were superimposed and analyzed by a grid calculator to obtain the base ecological resistance surface. The ability of nighttime light data to continuously characterize the intensity of human activity at the surface can reflect the level of internal variability affected by human disturbance (Carroll et al., 2020). Therefore, nighttime light data were utilized to correct the basic ecological resistance surface by Equation 5 (Guo et al., 2019). Finally, the integrated ecological resistance surface is obtained (Guo et al., 2019; Jin et al., 2021).
Where R* is the corrected resistance coefficient of the grid; TLIi is the nighttime light intensity value of the grid i; TLIa is the average nighttime light intensity value of the land type a; and Ro is the base resistance value of the grid i. In this study, we used the 2020 NPP-VIIRS satellite nighttime light remote sensing image data with a spatial resolution of 0.004 degrees provided by the Center for Resource and Environmental Science and Data, Institute of Geoscience and Resources, Chinese Academy of Sciences.
2.2.4 Ecological corridors extraction
The ecological source area and comprehensive ecological resistance surface data of Kangbao County were used as the basis for the analysis. The MCR model and circuit theory were comprehensively applied to construct the core network based on “cost-weighted distance and Euclidean distance” using the Linkage pathway tool of Linkage Mapper plug-in to complete the extraction of ecological corridors (McRae et al., 2012). Ecological corridors are calculated according to Equation 6 (Yu et al., 2018; Li et al., 2021).
Where MCR is the value of MCR; Dij denotes the spatial distance that species need to pass through from the source to the landscape unit; Ri denotes the ecological resistance coefficient of the landscape unit i; and f denotes that there is a positive correlation between the MCR and the ecological process. The maximum length of the corridor was set at 20,000 m.
2.2.5 Identification of ecological “pinch points” and ecological “barrier points” based on circuit theory
The Pinchpoint Mapper tool in the Linkage Mapper plug-in was used to identify ecological “pinch points”, where high current intensity areas are “pinch point” areas. These areas are considered key areas for ecological restoration (McRae et al., 2009). There are two modes in the tool, and related studies have shown that the “pinch point” areas obtained in the “adjacent pair” mode have no significance in maintaining the connectivity of the overall landscape (McRae et al., 2009), and organisms can move between two cores bypassing other cores. Therefore, the “raster centrality” mode was used to identify the ecological “pinch points”. This mode is divided into the pairwise and all-to-one categories. Taking the characteristics of territorial space ecological restoration into account, the “all-to-one” mode under the raster centrality was chosen to calculate the cumulative current density value of each image. Cost-weighted corridor width thresholds set at 1, 1.5, 2, and 2.5 km were set to obtain the ecological network current maps under different width thresholds (Cushman et al., 2013). The current intensity (cumulative current density value) was categorized into five levels using the natural breakpoint method. The higher and high level current intensity areas were selected as ecological “pinch points” in the study area.
Ecological barrier points are areas where species are more impeded in their migration between ecological source sites (McRae et al., 2012; McRae et al., 2012). The Barrier Mapper tool was utilized to identify ecological barrier points. The tool has two modes. One selects the Least Costly Pathway (LCD) Calculate Percentage of Improvement Score option, which identifies areas that are somewhat impeded but not completely impeded. The other mode does not select this option and identifies areas that are completely impeded (Cushman et al., 2013). Two modes were selected in this study for analysis at the same time. The minimum search radius was set as 50 m, maximum search radius was set as 200 m, step size was 50 m, “Maximum” was selected for the iterative operation, and the search and detection was performed using the moving window method. These parameters resulted in the cumulative current restoration value as the basis for the selection of ecological obstacles. Some restoration was carried out for obstacles, thus enhancing the connectivity of the region’s landscapes (Martin, 2017).
3 Results
3.1 Spatial distribution of ecological sources
In this study, habitats were categorized into five groups based on their quality assessment: low quality habitats (0−0.079), lower quality habitats (0.079−0.195), medium quality habitats (0.195−0.280), higher quality habitats (0.280−0.393), and high-quality habitat (0.393−0.722). The overall habitat quality in Kangbao County was low, with >60% of the area having a habitat quality score in the range of 0.079−0.195. There are 3,833 patches with a total area of 206.71 km2 in the high-quality habitat area, which accounted for <6.5% of the total area. The distribution was scattered. Forty-two patches had an area >1 km2 in the high-quality habitat area. Their total area of 97.82 km2 accounts for 47.32% of the area of the high-quality habitat area. Concerning high-quality habitat, the largest patch of (9.31 km2) is located in the southeast of Zhangji Township, the second largest patch (6.67 km2) is located in the southwestern part of Mandetang Township, and the third largest patch (5.83 km2) is located in the southwestern part of the County State Forestry Farm (Figure 4).
Figure 5 shows the spatial pattern of the seven landscape types obtained using the MSPA model. The total area of these landscape types of 1807.37 km2 accounts for 53.70% of the total area of the study area. Among the types, the core area of 1303.78 km2 accounts for 72.13% of the total area of the landscape types, which are mainly distributed in the northern, northwestern and southeastern regions, with scattered distribution in the central part of the country. The isolated island area of 40.38 km2 accounts for 2.23%. The pore area of 55.53 km2 accounts for 3.07%. The fringe area of 250.27 km2 accounts for 13.85%. The traffic circle area is 39.45 km2 (2.18%), the bridging area is 50.26 km2 (2.78%), and the spur area is 67.69 km2 (3.75%). The results show that the core area is the largest among the seven landscape types, accounting for 38.74% of the total area of the study area. The land use types are mainly woodland and grassland.
The preliminary ecological source sites obtained in this study totaled 769 patches with a total area of 156.10 km2 (Figure 6A). Considering the large changes in elevation and topography in Kangbao County, landscape patches with dPC ≥ 1 and area ≥ 0.5 km2 were selected as the final ecological source sites in the study area based on the analysis results (Figure 6B). A total of 40 patches were obtained. These patches played a large role in landscape connectivity, with a total area of 68.06 km2 (Table 4), accounting for 2.02% of the total area of the study area. The results show that the distribution of ecological source land in Kangbao County is relatively decentralized, with a small patch area and a small proportion of the total area. It is mainly located in the county state forest farm, Yanyoufang Township, Habiga Township, Tuchengzi Township, Zhangji Township, Dengyoufang Township, Zhongyi Township, Mandetang Township, Kangbao Township, and Lijiadi Township.
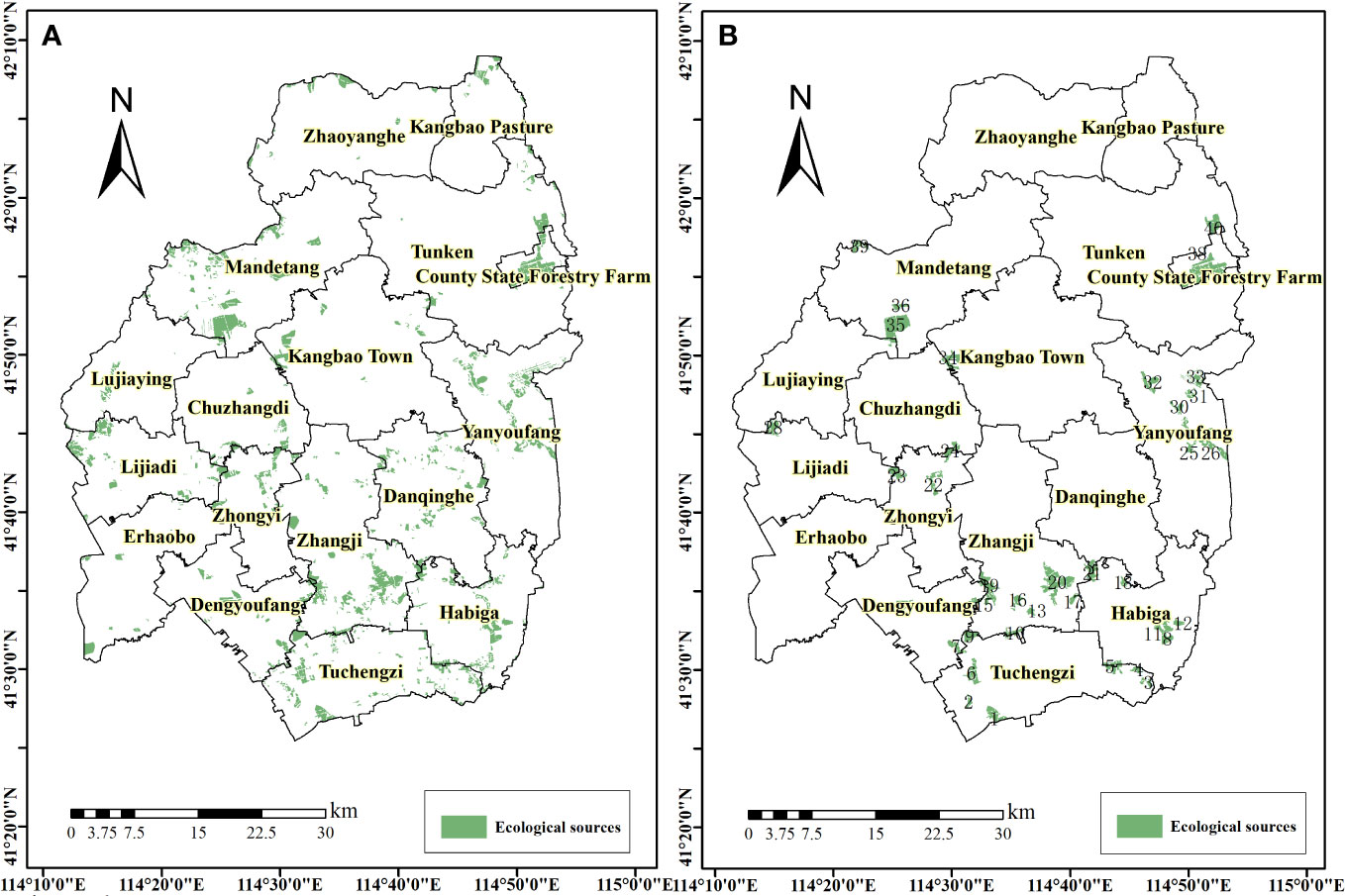
Figure 6 Identification results of ecological source area in Kangbao County: (A) preliminary identification, (B) further screening.
3.2 Resistance surface construction results and distribution
Figure 7 shows that areas with lower resistance values in the explicit resistance surface may have higher resistance values in the implicit resistance surface. The differences are concentrated in the northeastern part of Kangbao County in Kangbao Ranch, Zhaoyanghe Township, Mandetang Township, and Tunchen Township, which usually have a large number of distributed and dispersed settlements. The resistance surfaces of slope factor and undulation factor are significantly affected by topography and geomorphology. The resistance value of the northern area with low mountainous terrain and relatively high elevation is significantly higher than that of the other areas, while the rugged terrain is not conducive to the migratory movement of the species, and plays a weaker role in the connectivity of the landscape. The areas with high resistance values in the base resistance surface are mainly distributed in the center of urban areas and townships in Kangbao County, as well as in the northern areas with large slopes and terrain undulations (Figure 8).
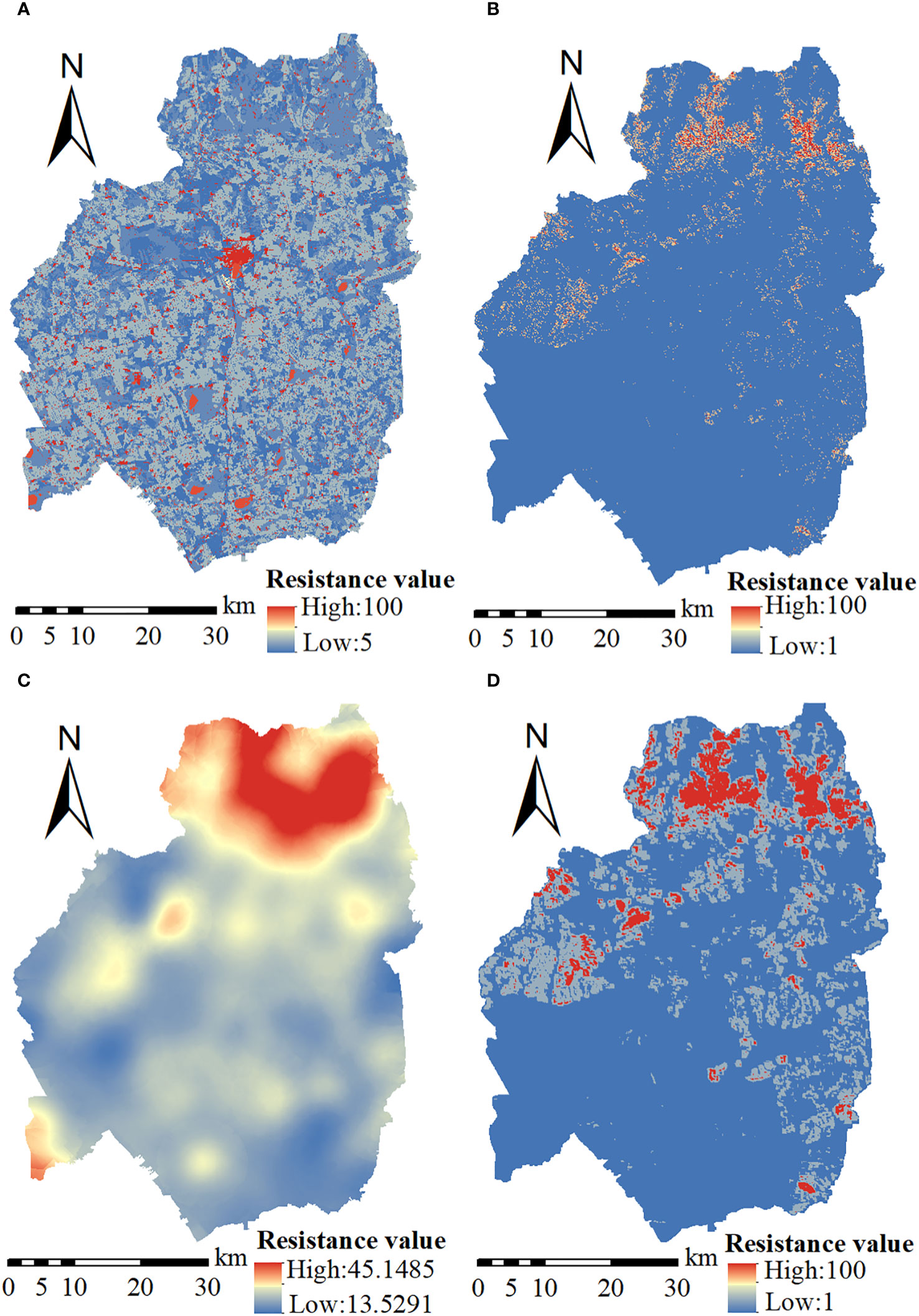
Figure 7 Resistance surface of single-factor: (A) dominance resistance, (B) slope resistance, (C) recessivity resistance, (D) relief resistance.
Figure 9 shows that the spatial difference of the ecological resistance surface within the same land type is more obvious, indicating that the correction based on the nighttime light data has fully considered the impact of human activities on ecological processes. The minimum resistance value was 3.25, maximum resistance value was 775.65, and the average value was 11.13 in Kangbao County. The low resistance value area accounts for >70% of the total and is scattered. The high resistance value area accounts for <10% of the total, and is concentrated in the central urban areas of Kangbao Town, Zhangji Town, and Lijiadi Town, which is mainly affected by the urban construction land use and has frequent human activities.
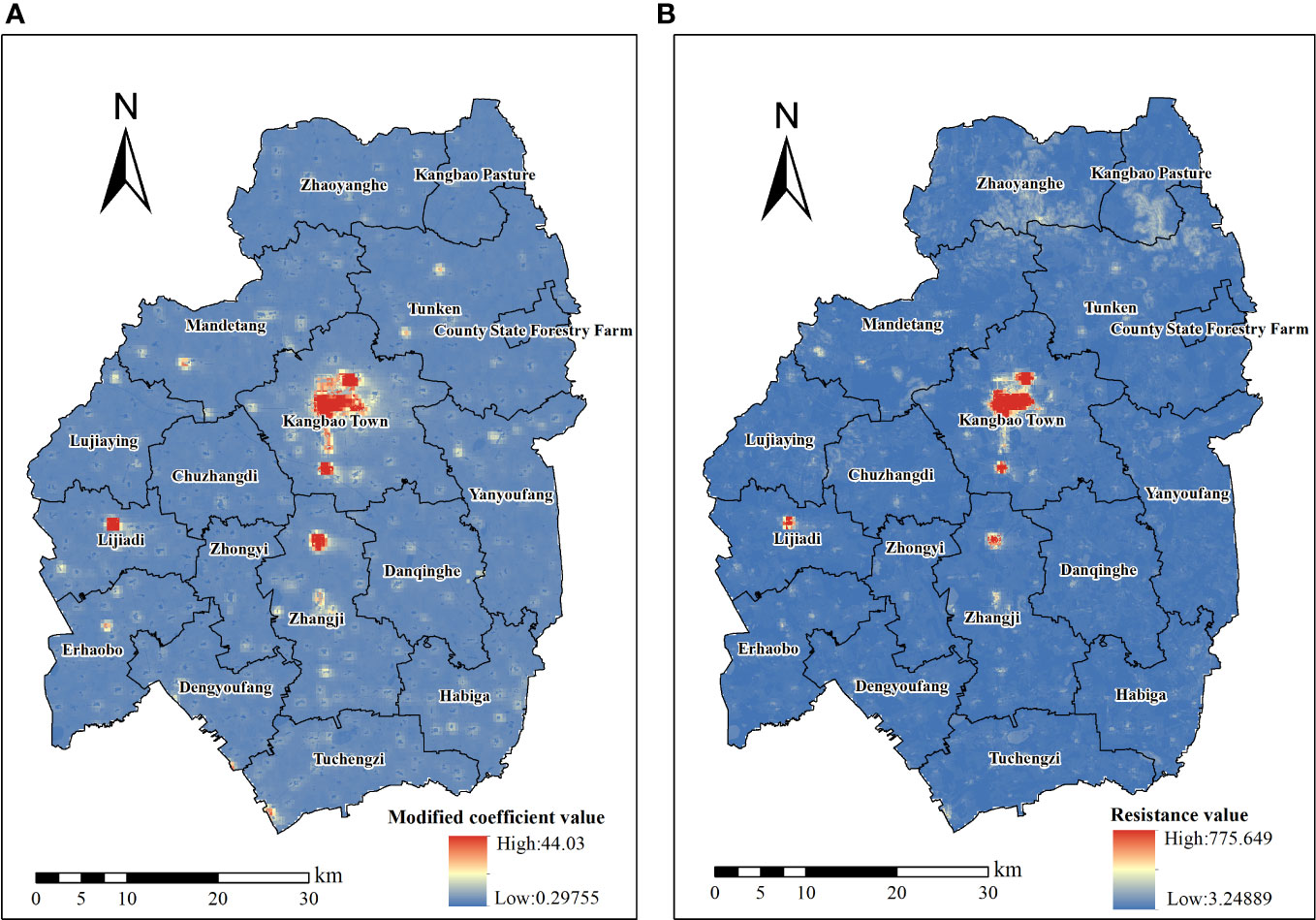
Figure 9 Resistance surface in Kangbao County: (A) modified resistance coefficient of nighttime light data, (B) comprehensive resistance surface.
3.3 Spatial distribution of ecological corridors
A total of 96 ecological corridors were extracted in this study. Their total length was 743.81 km, maximum length was 48.04 km, minimum length was 0.03 km, and average length was 7.75 km. Figure 10 shows that the distribution of ecological corridors in Kangbao County is uneven, with a small number of corridors and a large spatial span in the northern and western regions. In the southern and eastern parts of the study area, due to the fragmentation and scattered distribution of ecological source patches, the density of corridors is larger, mainly distributed in Dengyoufang Township, Tuchengzi Township, Habiga Township, Zhangji Township, Danqinghe Township, and Yanyoufang Township.
3.4 Spatial distribution of key areas for ecological restoration of territorial space
3.4.1 Ecological “pinch point” identification
The cumulative current intensity differed at different thresholds. The increased corridor width threshold resulted in more migration and diffusion choices of organisms, in turn resulting in diversion of the current and leading to the gradual decrease of the cumulative current intensity in the “pinch point” area (Figure 11). The wider the corridor, the larger the area through which the ecological currents flow, the greater the number of ecological flow routes, and the more obvious the degree of ecological “pinch points”. However, the distribution of ecological “pinch points” is always kept within a certain range. The ecological network current map revealed that ecological “pinch points” are roughly distributed at the intersections of neighboring ecological sources, at the borders between ecological sources and ecological corridors, and at the intersections or inflection points between ecological corridors and ecological corridors. In addition, the landscape connectivity of the area is better and the ecological “pinch points” are more prominent when the width threshold is set at 2 km.
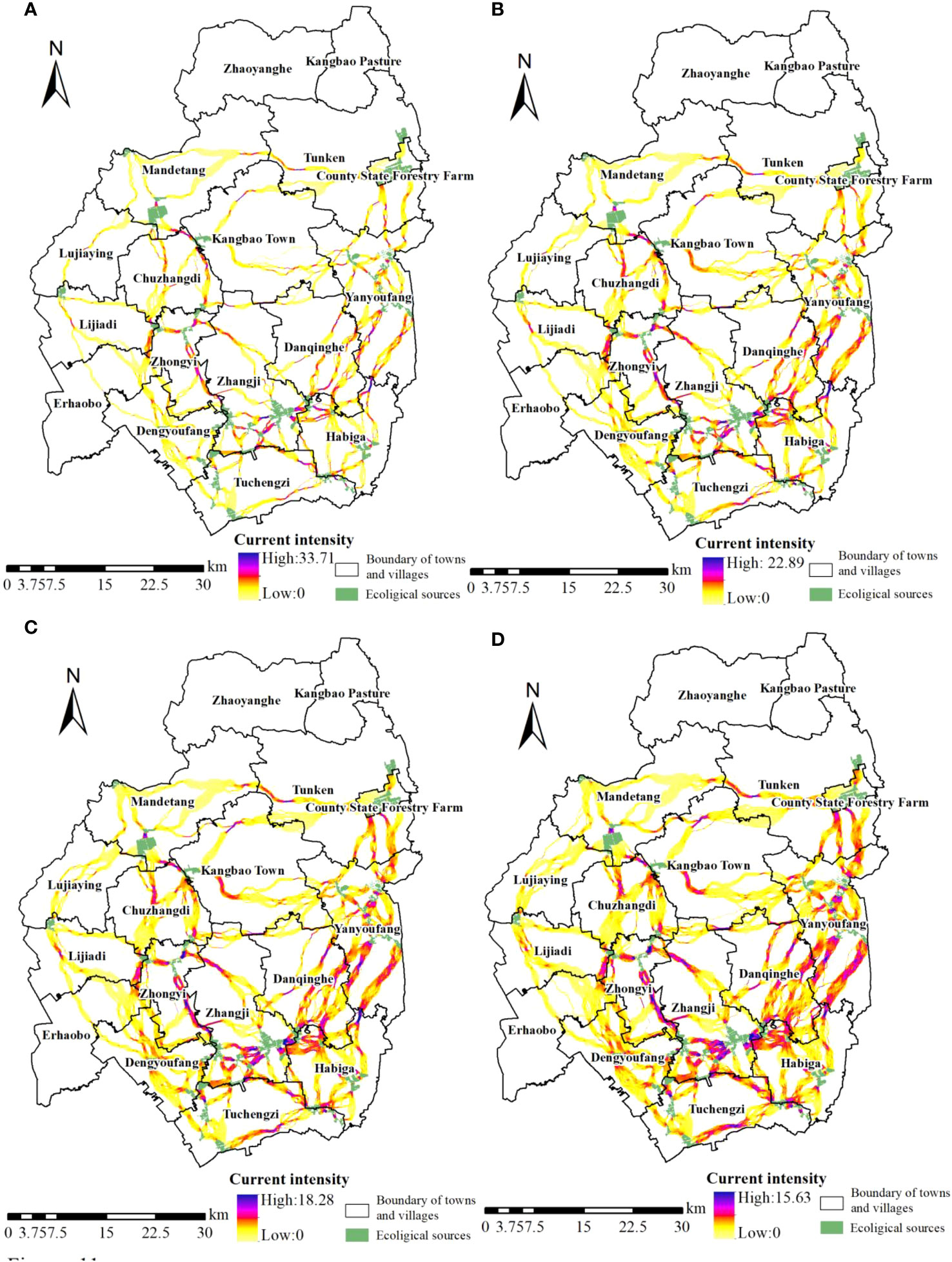
Figure 11 Cumulative current intensity under different thresholds: (A) threshold value of 1 km, (B) threshold value of 1.5 km, (C) threshold value of 2 km, (D) threshold value of 2.5 km.
The current intensity (cumulative current density value) was categorized into five levels by the natural breakpoint method, and the increasingly higher current intensity areas were selected as ecological “pinch points” in the study area. Due to the serious fragmentation of the patches, this study excluded patches of <0.1 km2, and obtained a total of 75 ecological “pinch points” with a total area of 31.72 km2 and an average value of 0.42 km2. These “pinch points” are mainly located in Zhangji Township, Zhongyi Township, Yanyoufang Township, Danqinghe Township, Habiga Township, and Tuchengzi Township (Figure 12). In the northern part of the study area, there are few ecological “pinch points” due to large-scale mining, rugged terrain, and high degree of relief. In the central part of the study area, the distribution of “pinch points” is more dispersed due to the expansion of construction land and roads in the process of urbanization. In the southern part of the study area, the terrain is open and flat, and the distribution of “pinch points” is more concentrated. The ecological “pinch point” area is dominated by forest land, grassland, and cultivated land with high ecosystem quality and rich biological resources. The respective areas of grassland, cultivated land, forest land, construction, and water/garden/bare land are 7.17, 15.28, 0.18 km2, and <0.01 km2 in total.
3.4.2 Ecological barrier points identification
Based on the results of the previous analysis, the 200 m gradient was selected as the most reasonable iteration radius to obtain the spatial distribution of the cumulative current recovery values in the two modes (Figure 13). Comparison of the cumulative current recovery values obtained in the calculated and uncalculated improvement fraction modes, relative to the LCD, showed that the maximum cumulative current recovery values were larger in the former, areas with higher cumulative current recovery values were more widely distributed, and magnitude of the numerical changes in the cumulative current recovery values along the paths connecting the different ecological source was larger. A total of 69 ecological barrier points were identified (Figure 14). They had a total area of 16.42 km2 and a maximum value of 1.51 km2. They are located in the northeast side of the Kangbanol National Wetland Park in the southern part of Kangbao Township. The main distribution and area of the ecological obstacles ranked in descending order of area as follows: Kangbao Township (3.36 km2), Tunken Township (3.00 km2), Yanyoufang Township (2.49 km2), Tuchengzi Township (1.67 km2), Zhangji Township (1.50 km2), Mandetang Township (1.16 km2), Habiga Township (0.88 km2), Danqinghe Township (0.69 km2), and Dengyoufang Township (0.65 km2). The types of land use in the ecological barrier points are mainly grassland and cultivated land, including 10.66 km2 of cultivated land, 3.65 km2 of grassland, 0.99 km2 of forest land, 0.94 km2 of construction land, 0.04 km2 of water area, and 0.03 km2 of bare land.
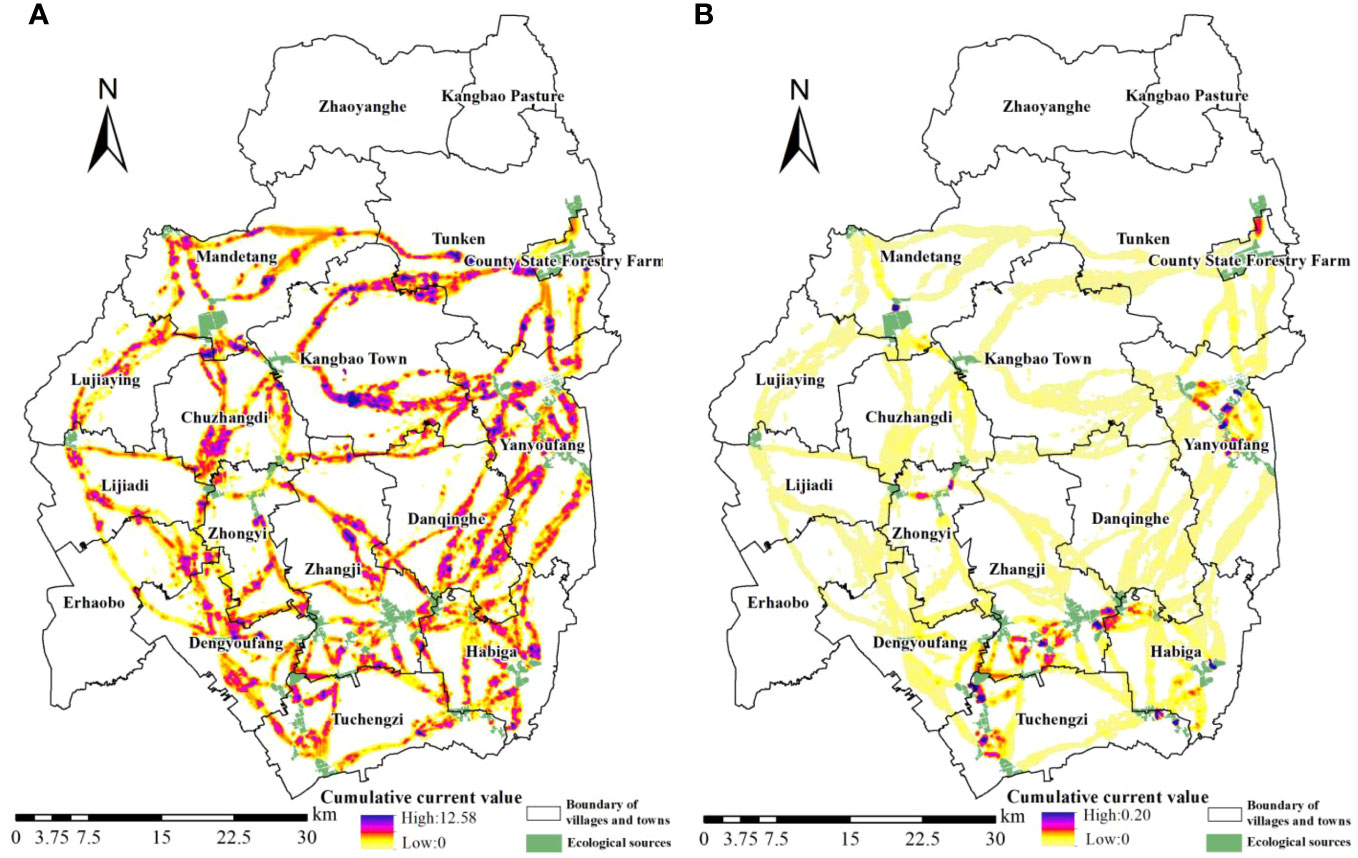
Figure 13 Spatial distribution of cumulative current recovery value in Kangbao County: (A) no calculation of improved score pattern, (B) calculation of improved fractional percentage mode relative to LCD.
4 Discussion
Based on the scale of urban agglomeration (Zhang et al., 2017), metropolitan area (Liu et al., 2023), provincial level (Wang et al., 2022a) and municipal level (Chen et al., 2023), this study determined the research paradigm and theoretical method of ecological security pattern at county level (Jin et al., 2021), and further identified the territorial ecological restoration area of Kangbao County. The study findings are more global, systematic, and complete than the traditional ecological protection and restoration studies targeting a single element. In this study, the theoretical methods of landscape ecology were integrated into the selection of ecological source sites, spatial structure and service function attributes of landscape elements were clarified, and methods of habitat quality assessment, MSPA analysis, and landscape connectivity analysis were comprehensively utilized, so as to practically comply with the characteristics of ecosystem systematicity, completeness and landscape connectivity required for ecological restoration of territorial space. In addition, in constructing the comprehensive ecological resistance surface, explicit resistance factors, implicit resistance factors, slope, and undulation were introduced and supplemented with nighttime light data for correction, which more accurately characterized the natural background conditions and the impact of human activities on the ecosystem. In addition, when extracting ecological corridors, traditional methods generally identify only least-cost paths (Lin et al., 2016), whereas the circuit theory used in this study better captures the stochastic nature of species’ choices for migratory paths. The advantage of the circuit theoretical model is that it can identify all possible connection paths, and is not limited to identifying a single optimal path. Based on circuit theory (Yang et al., 2021), the Linkage Mapper plug-in was utilized to accurately identify ecological “pinch points” and ecological “obstacle points” in the identification of ecological restoration critical areas.
There are still some shortcomings in this study. More in-depth research is needed. (1) At present, the selection of resistance factors, the determination of resistance values and weights have not yet formed a unified standard, especially the determination of resistance values is more subjective. In this study, we used the analytic hierarchy process (AHP) (Liu et al., 2020; Tang et al., 2020), principal component analysis (PCA) and Delphi method to identify land use type, elevation, relief, slope and NDVI as resistance factors, and further determined their resistance values and weights (Guo et al., 2021; Liu et al., 2023). By superimposing the foundation resistance surface with the grid, comparing the difference between the resistance value and the topography, it is found that the distribution of the resistance value is reasonable, and the range of the resistance value modified by the night light data is also in a reasonable range. However, whether the method of index selection, resistance value and weight determination in the construction of foundation resistance surface in this paper is scientific and accurate needs to be further verified. (2) The rationality of the corridor truncation distance threshold involved in the construction of county ecological security pattern in this study remains to be verified. Relevant scholars set the cost-weighted distance threshold as 2km when taking Qingdao as the research object (Qian et al., 2023), and 6000 as the cumulative cut-off threshold when taking Huaibei as the research area (Li et al., 2023). The Linkage Mapper User Guide (McRae and Kavanagh, 2011) specifies a maximum cut-off cost weighted distance threshold of 200,000. It was found that the upper limit of the threshold had little effect on the overall distribution of the ecological corridor, and the habitat quality of large patches and wider corridors was better, which could greatly reduce the land landscape resistance of species migration and diffusion, and improve the survival rate of species during migration. When the scale of the study area is roughly the same, there is no perfect setting of the threshold of the corridor width, so the research results are scientific if the threshold is determined within a reasonable range. In this study, the threshold of corridor truncation distance at county scale (Gao et al., 2022) was determined after comprehensive analysis and reference of other research results. (3) The landscape scene analysis results obtained by the model algorithm in this paper are based on the map spots in the third National land survey data. As the result data of the “third survey” are authentic and authoritative, the ecological pinch points and ecological obstacle points identified and their distribution are scientific and reasonable. The analysis results of ecological clip-points and ecological obstacle points obtained in this paper are verified through the relevant results of ecological restoration planning of territorial space, resource and environment carrying capacity and suitability evaluation of territorial space development, land consolidation planning, and ecological environmental protection planning of the “13th Five-Year Plan”, and the results are roughly the same as those obtained by scientific and technological workers. (4) Finally, further research is needed concerning the improvement of the connectivity of the ecosystem structure after the restoration of these critical areas.
This study identified the key areas for ecological restoration in Kangbao County and proposed classification and restoration suggestions based on the actual situation of the key areas for ecological restoration in territorial space. The ecological “pinch point” area carries important landscape connectivity functions, consistent with the principle of protection as the mainstay and human-made restoration as a supplement. Specifically, this study proposed two categorized restoration strategies. First, if the land use type in the ecological “pinch point” area is arable land, the ecological resistance can be lowered by planting more trees around the arable land, reducing the use of chemical fertilizers and pesticides, and further increasing the improvement of farmland and the surrounding habitats. These actions will improve landscape connectivity and ecological functions. Second, if the land use type of ecological “pinch points” is woodland or grassland, it is necessary to strengthen the cultivation and protection of vegetation, match forest species, and take measures that include such as forest restructuring, forest-farming and agriculture mixed cropping, and forest ecological engineering. These actions have several results. First, the structure of the woodland community is improved and good ecological space for the migration, habitat, and reproduction of species is provided. Second, a hierarchical protection system of woodlands can be implemented, and a full-coverage, three-dimensional system of regulating animal husbandry can be established, to promote the rational development and utilization of woodland. Third, the precise implementation of the system of water retention in the ground, forests, and grasses can be implemented, to achieve the goal of “bringing water to greenery, nourishing water with greenery and protecting the shoreline with forests,” so as to enhance the capacity of the whole region in terms of water containment.
The restoration of the ecological obstacle point area can greatly reduce the resistance in the ecological process, enhance the landscape connectivity, and effectively optimize the overall ecological security pattern of the region. Thus, adoption of restoration-oriented and protection-oriented restoration measures are warranted. Concerning ecological obstacles, six recommendations can be made, taking into account the current land use situation in the study area. First, the key to ecological barrier restoration is to focus on improving the ecological service capacity and quality of arable land. For arable land outside the scope of permanent basic farmland, returning farmland to forests and grasses can be carried out in accordance with the principles of greenness, ecology, and high efficiency. For arable land within the scope of permanent basic farmland, under the premise of ensuring that there is no deterioration in the quality or decrease in the area, the comprehensive improvement of farmland can be performed, and farmland can be developed in the direction of ecological conservation and cultural landscape. The second recommendation is to strength the management and protection of woodlands and grasslands within ecological obstacle sites, expand the area of woodlands and grasslands, and increase the vegetation cover. The foci are on slope to ladder conversion and the construction of ditches and dams, advancing the soil and water conservation management of grassland areas on dams and dams, and preventing and controlling land sands and desertification, while utilizing biological measures to increase vegetation cover and maintain and improve land productivity. The aim is to accelerate the construction of ecological and economic forests, agricultural protection forests, and ecological forests for the public good. The third recommendation involves the small amount of construction land scattered within the ecological barrier points. Comprehensive remediation is being performed on rural collective construction land, low-utility land, and idle land, to urbanize farmers’ residences, effectively increase the arable land area, and scale-up land management. The fourth recommendation concerns mining land, including mines whose resources have been depleted. Priority is given to important ecological function areas, residential living areas, sensitive mountains along transportation routes, and other sites. Comprehensive management measures, where appropriate, such as greening, building construction, and landscaping are taken to actively promote the restoration of the environmental management of mines. For mining land, mines, and other sites that have already been damaged, re-greening of visually exposed mountains is performed to restore the natural landscape to the maximum extent possible, and to avoid wind, sand, and dust from contaminating the surrounding atmosphere. The fifth recommendation concerns regional waters and water conservancy facilities. Further promotion of green water-saving development methods is needed. Other initiatives include promotion of agricultural water-saving technologies, strict control of the area of new high water-consuming cash crop cultivation, and guiding the promotion of “water to drought”. The sixth recommendation concerns bare land. Comprehensive remediation of land sanding should be done and soil sanding should be improved by adopting sand-fixing methods that are mainly based on plant sand fixation and supplemented by engineering measures. As well, comprehensive remediation of soil salinization is needed and reduction of the content of salt in the soil achieved by improved agricultural measures, chemical amelioration, biological amelioration, and other measures.
5 Conclusion
This study identified ecological source areas based on habitat quality assessment, MSPA analysis, and landscape connectivity analysis, corrected the base resistance surface using nighttime light data, extracted ecological corridors by combining the MCR model and circuit theory, completed the construction of the ecological security pattern in Kangbao County, identified the key areas of ecological restoration by using Linkage Mapper plug-in, and proposed restoration strategies in one step. Forty ecological sources were identified in Kangbao County. The total area of 68.06 km2 is dominated by forests and grasslands, with scattered patches. The overall ecological sources still need to be improved. Ninety-six ecological corridors with a total length of 743.81 km were identified. The corridors are more densely distributed in the southern and eastern parts of the county, and there is still much room for improvement in the connectivity of the habitats. Kangbao County has 75 ecological “pinch points” to be restored and 69 ecological barrier points, with a total area of 48.14 km2. The key areas to be restored are centrally located in Yanyoufang, Habiga, Tuchengzi, Zhangji, and Danqinghe Townships. Based on the spatial distribution characteristics of the areas to be restored in Kangbao County, the current types of land, and the ecological characteristics of the study area, it is proposed that the ecological “pinch points” should be mainly protected by nature and restored by humans, while the ecological barrier points should be restored by humans and protected by nature. Specific optimization measures should be taken for the different types of ecological “pinch points” and “barrier points”.
Data availability statement
The raw data supporting the conclusions of this article will be made available by the authors, without undue reservation.
Author contributions
XJ: Conceptualization, Formal Analysis, Funding acquisition, Investigation, Methodology, Visualization, Writing – original draft. ZT: Formal Analysis, Investigation, Methodology, Writing – original draft. HY: Data curation, Funding acquisition, Resources, Software, Writing – original draft. GS: Conceptualization, Funding acquisition, Investigation, Project administration, Resources, Supervision, Writing – review & editing. JM: Data curation, Investigation, Resources, Software, Supervision, Visualization, Writing – review & editing.
Funding
The author(s) declare financial support was received for the research, authorship, and/or publication of this article. This research was financially supported by the China Postdoctoral Science Foundation (2019M650823) and the 333 Talent Project of Hebei Province (C20221028).
Conflict of interest
Author JM was employed by Hebei Geographic Information Group Co., Ltd.
The remaining authors declare that the research was conducted in the absence of any commercial or financial relationships that could be construed as a potential conflict of interest.
Publisher’s note
All claims expressed in this article are solely those of the authors and do not necessarily represent those of their affiliated organizations, or those of the publisher, the editors and the reviewers. Any product that may be evaluated in this article, or claim that may be made by its manufacturer, is not guaranteed or endorsed by the publisher.
References
An Y., Liu S., Sun Y., Shi F., Beazley R. (2021). Construction and optimization of an ecological network based on morphological spatial pattern analysis and circuit theory. Landscape Ecol. 36, 2059–2076. doi: 10.1007/s10980-020-01027-3
Berta Aneseyee A., Noszczyk T., Soromessa T., Elias E. (2020). The inVEST habitat quality model associated with land use/cover changes: A qualitative case study of the Winike watershed in the Omo-Gibe basin, southwest Ethiopia. Remote Sens. 12, 1103. doi: 10.3390/rs12071103
Cao X., Liu Z., Li S., Gao Z. (2022). Integrating the ecological security pattern and the PLUS model to assess the effects of regional ecological restoration: A case study of Hefei City, Anhui province. Int. J. Environ. Res. Publ. Health 19, 6640. doi: 10.3390/ijerph19116640
Carroll K. A., Hansen A. J., Inman R. M., Lawrence R. L., Hoegh A. B. (2020). Testing landscape resistance layers and modeling connectivity for wolverines in the western United States. Global Ecol. Conserv. 23, e01125. doi: 10.1016/j.gecco.2020.e01125
Chen X., Kang B., Li M., Du Z., Zhang L., Li H. (2023). Identification of priority areas for territorial ecological conservation and restoration based on ecological networks: A case study of Tianjin City, China. Ecol. Indic. 146, 109809. doi: 10.1016/j.ecolind.2022.109809
Cushman S. A., McRae B., Adriaensen F., Beier P., Shirley M., Zeller K. (2013). Biological corridors and connectivity. Key topics in conservation biology 2, 384–404.
Dai L., Liu Y., Luo X. (2021). Integrating the MCR and DOI models to construct an ecological security network for the urban agglomeration around Poyang Lake, China. Sci. Total Environ. 754, 141868–141868. doi: 10.1016/j.scitotenv.2020.141868
Dickson B. G., Albano C. M., Anantharaman R., Beier P., Fargione J., Graves T. A., et al. (2019). Circuit-theory applications to connectivity science and conservation. Conserv. Biol. 33, 239–249. doi: 10.1111/cobi.13230
Di Giulio M., Holderegger R., Tobias S. (2009). Effects of habitat and landscape fragmentation on humans and biodiversity in densely populated landscapes. J. Environ. Manage. 90, 2959–2968. doi: 10.1016/j.jenvman.2009.05.002
Dilt T. E., Weisberg P. J., Leitner P., Matocq M. D., Inman R. D., Nussear K. E., et al. (2016). Multiscale connectivity and graph theory highlight critical areas for conservation under climate change. Ecol. Appl. 26, 1223–1237. doi: 10.1890/15-0925
Gao M., Hu Y., Bai Y. (2022). Construction of ecological security pattern in national land space from the perspective of the community of life in mountain, water, forest, field, lake and grass: A case study in Guangxi Hechi, China. Ecol. Indicat. 139, 108867. doi: 10.1016/j.ecolind.2022.108867
Guo J. X., Hu Z. Q., Li H. X., Liu J., Zhang X., Lai X. (2021). Construction of municipal ecological space network based on MCR model. Trans. Chin. Soc. Agric. Machinery 52 (3), 275. doi: 10.6041/j.issn.1000-1298.2021.03.031
Guo R., Wu T., Liu M., Huang M., Stenardo L., Zhang Y. (2019). The construction and optimization of ecological security pattern in the Harbin-Changchun urban agglomeration, China. Int. J. Environ. Res. Publ. Health 16, 1190. doi: 10.3390/ijerph16071190
He W., Zhang K., Kong Y., Yuan L., Peng Q., Mulugeta Degefu D., et al. (2023). Reduction pathways identification of agricultural water pollution in Hubei Province, China. Ecol. Indic. 153, 110464. doi: 10.1016/j.ecolind.2023.110464
Huang J., Hu Y., Zheng F. (2020). Research on recognition and protection of ecological security patterns based on circuit theory: a case study of Jinan City. Environ. Sci. pollut. Res. Int. 27, 12414–12427. doi: 10.1007/s11356-020-07764-x
Huang K., Peng L., Wang X., Deng W. (2022). Integrating circuit theory and landscape pattern index to identify and optimize ecological networks: a case study of the Sichuan Basin, China. Environ. Sci. pollut. Res. Int. 29, 66874–66887. doi: 10.1007/s11356-022-20383-y
Jin X., Wei L., Wang Y., Lu Y. (2021). Construction of ecological security pattern based on the importance of ecosystem service functions and ecological sensitivity assessment: a case study in Fengxian County of Jiangsu Province, China. Environ. Dev. Sustain. 23, 563–590. doi: 10.1007/s10668-020-00596-2
Li J., Song C., Cao L., Zhu F., Meng X., Wu J. (2011). Impacts of landscape structure on surface urban heat islands: A case study of Shanghai, China. Remote Sens. Environ. 115 (12), 3249–3263. doi: 10.1016/j.rse.2011.07.008
Li Q., Wang L., Gul H. N., Li D. (2021). Simulation and optimization of land use pattern to embed ecological suitability in an oasis region: A case study of Ganzhou district, Gansu province, China. J. Environ. Manage. 287, 112321. doi: 10.1016/j.jenvman.2021.112321
Lin Q., Mao J., Wu J., Li W., Yang J. (2016). Ecological security pattern analysis based on InVEST and Least-Cost Path model: a case study of Dongguan Water Village. Sustainability 8 (2), 172. doi: 10.3390/su8020172
Liu D., Chang Q. (2015). Ecological security research progress in China. Acta Ecol. Sin. 35, 111–121. doi: 10.1016/j.chnaes.2015.07.001
Liu X., Su Y., Li Z., Zhang S. (2023). Constructing ecological security patterns based on ecosystem services trade-offs and ecological sensitivity: A case study of Shenzhen metropolitan area, China. Ecol. Indic. 154, 110626. doi: 10.1016/j.ecolind.2023.110626
Liu X., Wei M., Zeng J. (2020). Simulating urban growth scenarios based on ecological security pattern: A case study in Quanzhou, China. Int. J. Environ. Res. Public Health 17 (19), 7282. doi: 10.3390/ijerph17197282
Ma L., Bo J., Li X., Fang F., Cheng W. (2019). Identifying key landscape pattern indices influencing the ecological security of inland river basin: The middle and lower reaches of Shule River Basin as an example. Sci. Total Environ. 674, 424–438. doi: 10.1016/j.scitotenv.2019.04.107
Martin D. M. (2017). Ecological restoration should be redefined for the twenty-first century. Restor. Ecol. 25 (5), 668–673. doi: 10.1111/rec.12554
McRae B. H., Beier P. (2007). Circuit theory predicts gene flow in plant and animal populations. Proc. Natl. Acad. Sci. 104 (50), 19885–19890. doi: 10.1073/pnas.0706568104
McRae B. H., Dickson B. G., Keitt T. H., Shah V. B. (2008). Using circuit theory to model connectivity in ecology, evolution, and conservation. Ecology 89, 2712–2724. doi: 10.1890/07-1861.1
McRae B. H., Hall S. A., Beier P., Theobald D. M. (2012). Where to restore ecological connectivity? Detecting barriers and quantifying restoration benefits. PLoS One 7, e52604. doi: 10.1371/journal.pone.0052604
McRae B. H., Shah V. B., Mohapatra T. K. (2009). Circuitscape user’s guide (Santa Barbara: The University of California).
Merrick M. J., Koprowski J. L. (2017). Circuit theory to estimate natal dispersal routes and functional landscape connectivity for an endangered small mammal. Landscape Ecol. 32, 1163–1179. doi: 10.1007/s10980-017-0521-z
Peng J., Li H. L., Liu Y. X., Hu Y. N., Yang Y. (2018a). Identification and optimization of ecological security pattern in Xiong’an New Area. Acta Geogr. Sin. 73, 701–710. doi: 10.11821/dlxb201804009
Peng J., Lv D., Dong J., Liu Y., Liu Q., Li B. (2020). Processes coupling and spatial integration: Characterizing ecological restoration of territorial space in view of landscape ecology. J. Nat. Resour. 35 (1), 3–13. doi: 10.31497/zrzyxb.20200102
Peng J., Yang Y., Liu Y., Hu Y., Du Y., Meersmans J., et al. (2018b). Linking ecosystem services and circuit theory to identify ecological security patterns. Sci. Total Environ. 644, 781–790. doi: 10.1016/j.scitotenv.2018.06.292
Peng J., Zhao S., Dong J., Liu Y., Meersman J., Li H., et al. (2019). Applying ant colony algorithm to identify ecological security patterns in megacities. Environ. Model. Software 117, 214–222. doi: 10.1016/j.envsoft.2019.03.017
Qian W., Zhao Y., Li X. (2023). Construction of ecological security pattern in coastal urban areas: A case study in Qingdao, China. Ecol. Indic. 154, 110754. doi: 10.1016/j.ecolind.2023.110754
Rahimi L., Malekmohammadi B., Yavari A. R. (2020). Assessing and modeling the impacts of wetland land cover changes on water provision and habitat quality ecosystem services. Nat. Resor. Res. 29, 3701–3718. doi: 10.1007/s11053-020-09667-7
Ran Y., Lei D., Li J., Al E. (2022). Identification of crucial areas of territorial ecological restoration based on Yunnan. Ecol. Ind. 143, 109318. doi: 10.1016/j.ecolind.2022.109318
Saura S., Rubio L. (2010). A common currency for the different ways in which patches and links can contribute to habitat availability and connectivity in the landscape. Ecography 33 (3), 523–537. doi: 10.1111/j.1600-0587.2009.05760.x
Solow A. R. (2017). On detecting ecological impacts of extreme climate events and why it matters. Philos. Trans. R. Soc. B: Biol. Sci. 372 (1723), 20160136. doi: 10.1098/rstb.2016.0136
Tang Y., Gao C., Wu X. (2020). Urban ecological corridor network construction: An integration of the least cost path model and the InVEST model. ISPRS Int. J. Geo-Information 9 (1), 33. doi: 10.3390/ijgi9010033
Tutak M., Brodny J., Bindzár P. (2021). Assessing the level of energy and climate sustainability in the European union countries in the context of the European green deal strategy and agenda 2030. Energies 14, 1767. doi: 10.3390/en14061767
Vergnes A., Kerbiriou C., Clergeau P. (2013). Ecological corridors also operate in an urban matrix: a test case with garden shrews. Urban Ecosyst. 16, 511–525. doi: 10.1007/s11252-013-0289-0
Vogt P., Ferrari J. R., Lookinbill T. R., Gardner R. H., Riitters K. H., Ostapowicz K. (2009). Mapping functional connectivity. Ecol. Ind. 9, 64–71. doi: 10.1016/j.ecolind.2008.01.011
Wang Z., Liu Y., Xie X., Al. E. (2022a). Identifying key areas of green space for ecological restoration based on ecological security patterns in Fujian province, China. Land 11, 1496. doi: 10.3390/land11091496
Wang S., Wu M., Hu M., Xia B. (2022b). Integrating ecosystem services and landscape connectivity into the optimization of ecological security pattern: a case study of the Pearl River Delta, China. Environ. Sci. pollut. Res. Int. 29, 76051–76065. doi: 10.1007/s11356-022-20897-5
Wang C., Yu C., Chen T., Feng Z., Hu Y., Wu K. (2020). Can the establishment of ecological security patterns improve ecological protection? An example of Nanchang, China. Sci. Total Environ. 740, 140051. doi: 10.1016/j.scitotenv.2020.140051
Wang J., Zhai T., Lin Y., Kong X., He T. (2019). Spatial imbalance and changes in supply and demand of ecosystem services in China. Sci. Total Environ. 657, 781–791. doi: 10.1016/j.scitotenv.2018.12.080
Wu Y., Han Z., Meng J., Zhu L. (2023). Circuit theory-based ecological security pattern could promote ecological protection in the Heihe River Basin of China. Environ. Sci. pollut. Res. Int. 30, 27340–27356. doi: 10.1007/s11356-022-24005-5
Xu J., Xu D., Qu C. (2022). Construction of ecological security pattern and identification of ecological restoration zones in the city of Changchun, China. Int. J. Environ. Res. Publ. Health 20, 289. doi: 10.3390/ijerph20010289
Yang R., Bai Z., Shi Z. (2021). Linking morphological spatial pattern analysis and circuit theory to identify ecological security pattern in the Loess Plateau: Taking Shuozhou City as an example. Land 10 (9), 907. doi: 10.3390/land10090907
Yang Y., Chen J., Huang R., Feng Z., Zhou G., You H., et al. (2022). Construction of ecological security pattern based on the importance of ecological protection–A case study of Guangxi, a Karst Region in China. Internat. J. Environ. Res. Publ. Health 19, 5699. doi: 10.3390/ijerph19095699
Yu K. (1996). Security patterns and surface model in landscape ecological planning. Landsc. Urban Plan. 36, 1–17. doi: 10.1016/S0169-2046(96)00331-3
Yu Q., Yue D., Wang Y., Kai S., Fang M., Ma H., et al. (2018). Optimization of ecological node layout and stability analysis of ecological network in desert oasis: a typical case study of ecological fragile zone located at Deng Kou County (Inner Mongolia). Ecol. Ind. 84, 304–318. doi: 10.1016/j.ecolind.2017.09.002
Yuan L., Li R., He W., Wu X., Kong Y., Degefu D. M., et al. (2022). Coordination of the industrial-ecological economy in the Yangtze River Economic Belt, China. Front. Environ. Sci. 10, 451. doi: 10.3389/fenvs.2022.882221
Zawadzka J. E., Harris J. A., Corstanje R. (2021). Assessment of heat mitigation capacity of urban greenspaces with the use of InVEST urban cooling model, verified with day-time land surface temperature data. Landsc. Urban Plan. 214, 104–163. doi: 10.1016/j.landurbplan.2021.104163
Zhang D., Huang Q., He C., Wu J. (2017). Impacts of urban expansion on ecosystem services in the Beijing-Tianjin-Hebei urban agglomeration, China: A scenario analysis based on the Shared Socioeconomic Pathways. Resources, Conservation and Recycling 125, 115–130.
Zhang L., Peng J., Liu Y., Wu J. (2017). Coupling ecosystem services supply and human ecological demand to identify landscape ecological security pattern: A case study in Beijing–Tianjin–Hebei region, China. Urban Ecosystems 20, 701–714.
Zhang C., Fang S. (2021). Identifying and zoning key areas of ecological restoration for territory in resource-based cities: A case study of Huangshi City, China. Sustainability 13 (7), 3931. doi: 10.3390/su13073931
Zhang Y.-Z., Jiang Z.-Y., Li Y.-Y., Yang Z.-G., Wang X.-H., Li X.-B. (2021). Construction and optimization of an urban ecological security pattern based on habitat quality assessment and the minimum cumulative resistance model in Shenzhen City, China. Forests 12, 847. doi: 10.3390/f12070847
Zhang H., Li J., Tian P., Pu R., Cao L. (2022). Construction of ecological security patterns and ecological restoration zones in the city of Ningbo, China. J. Geograph. Sci. 32, 663–681. doi: 10.1007/s11442-022-1966-9
Zhang L., Peng J., Liu Y., Wu J. (2016). Coupling ecosystem services supply and human ecological demand to identify landscape ecological security pattern: A case study in Beijing–Tianjin–Hebei region, China. Urban Ecosys. 20, 1–14. doi: 10.1007/s11252-016-0629-y
Zhang H., Zhang C., Hu T., Zhang M., Ren X., Hou L. (2020a). Exploration of roadway factors and habitat quality using InVEST. Transport. Res. Part D Trans. Environ. 87, 102551. doi: 10.1016/j.trd.2020.102551
Zhang X., Zhou J., Li G., Chen C., Li M., Luo J. (2020b). Spatial pattern reconstruction of regional habitat quality based on the simulation of land use changes from 1975 to 2010. J. Geo. Sci. 30, 601–620. doi: 10.1007/s11442-020-1745-4
Zhao X. Q., Xu X. H. (2015). Research on landscape ecological security pattern in a Eucalyptus introduced region based on biodiversity conservation. Russian J. Ecol. 46, 59–70. doi: 10.1134/S106741361501018X
Keywords: ecological restoration, ecological security, circuit theory, habitat quality, linkage mapper, Kangbao County
Citation: Jiao X, Tian Z, Yang H, Shang G and Ma J (2024) Identification of key areas for ecological restoration of territorial space based on ecological security pattern: a case study of Kangbao County. Front. Ecol. Evol. 11:1301149. doi: 10.3389/fevo.2023.1301149
Received: 24 September 2023; Accepted: 04 December 2023;
Published: 04 January 2024.
Edited by:
Xueru Zhang, Hebei University of Economics and Business, ChinaReviewed by:
Miguel Alfonso Ortega-Huerta, National Autonomous University of Mexico, MexicoLiang Yuan, China Three Gorges University, China
Copyright © 2024 Jiao, Tian, Yang, Shang and Ma. This is an open-access article distributed under the terms of the Creative Commons Attribution License (CC BY). The use, distribution or reproduction in other forums is permitted, provided the original author(s) and the copyright owner(s) are credited and that the original publication in this journal is cited, in accordance with accepted academic practice. No use, distribution or reproduction is permitted which does not comply with these terms.
*Correspondence: Guofei Shang, shangguofei@hgu.edu.cn