Promoting urban ecological resilience through the lens of avian biodiversity
- 1Ecology and Evolutionary Biology Program, Texas A&M University, College Station, TX, United States
- 2Department of Ecology and Conservation Biology, Texas A&M University, College Station, TX, United States
- 3School of Biological Sciences, Louisiana Tech University, Ruston, LA, United States
- 4Department of Integrative Biology, The University of Texas at San Antonio, San Antonio, TX, United States
The significance of urban landscapes in safeguarding biodiversity is often disregarded, even though a considerable amount of conservation focus is directed toward biodiversity hotspots where urban land conversion is happening at the fastest pace. Maintaining biodiversity in urban areas not only benefits the environment, but along with social, economic, and technological factors can increase the stability of urban systems to disturbance, a concept known as “urban resilience”. In this synthesis paper, we explore the ecological dimension of urban resilience and specifically focus on avian biodiversity because birds are easy to observe, relatively abundant, and can serve as an indicator of the overall health of urban environments. We first examine the concept of ecological resilience and discuss the role of environmental stressors associated with urbanization in the ongoing avian biodiversity crisis. We then provide an overview of characteristics of the urban environment that may promote ecological resilience in birds, and associations between social and economic factors and urban ecological resilience. Finally, we provide recommendations on future research regarding strategies to improve urban ecological resilience and thus, urban resilience as a whole, at the intersections of urban ecology, ecosystem ecology, environmental justice, and urban planning. Since 68% of the world’s population is projected to live in urban areas by 2050, it is imperative that scientists, urban planners, civil engineers, architects, and others consider urban ecological resilience as a dimension of both environmental health and the resilience of cities to future natural and anthropogenic stressors.
1 Urban ecological resilience
Fifty-five percent of the world’s population resided in urban areas in 2018 and that number is expected to increase to 68% by 2050 (United Nations, 2019). The importance of these urban areas for conservation of biodiversity is increasingly recognized (Soanes and Lentini, 2019; Kondratyeza et al., 2020). At the same time, biodiversity has a reciprocal influence on urban systems, shaping human health and well-being to enhance the overall resilience of the urban environment (Alberti and Marzluff, 2004; Sharifi, 2023). Here, we define ‘ecological resilience’ as the ability of an ecological system to resist and recover from perturbations (Harrison, 1979; Scheffer et al., 2015; Selwood et al., 2015). Resistance refers to the magnitude of disturbance causing a change in structure, while recovery pertains to the speed at which the system returns to its original structure or a stable state (Tilman and Downing, 1994; Côté and Darling, 2010). While the concept of ecological resilience was first introduced into the scientific literature by C.S. Holling in 1973 (Holling, 1973), the term is an evolving and multidimensional concept (Desjardins et al., 2015).
Measuring ecological resilience directly can be difficult, so researchers often use proxy metrics at different levels, such as species, communities, or ecosystems. A critical component of ecological resilience is the ability of an ecological community to compensate for lost species and/or open niches following a perturbation (McCloy et al., 2022). Thus, many current approaches include species diversity, because it correlates with increased functional redundancy and niche overlap (Biggs et al., 2020). The effectiveness and complexity of these measures vary depending on the scale of analysis (Fischer et al., 2007). The most straightforward methods include species abundance, probability of occupancy, richness, and diversity indices such as Shannon’s Index (Clergeau et al., 1998; Johnson and Winker, 2010; Irizarry et al., 2021). For example, species occupancy data was used in BirdLife Australia’s Atlas Program to estimate resistance and recovery of Australian birds following a 13-year drought (Selwood et al., 2015). These proxies for resilience are easy to calculate and implement, and many authors claim they are adequate for assessing questions pertaining to localized resilience (Johnson and Winker, 2010; Karp et al., 2011; Selwood et al., 2015; Irizarry et al., 2021).
Ecological resilience in urban settings is typically referred to as “urban ecological resilience” and is the ability of an urban ecological system to maintain its structure (i.e., stable state) in response to a disturbance. Urban ecological resilience forms one dimension of urban resilience, along with the social and technological dimensions, and these dimensions are dynamically interconnected (Figure 1; Alberti and Marzluff, 2004; Sharifi, 2023). For example, in Austin, TX, taxpayers have consistently voted to fund open space acquisitions by the city (social dimension) to protect the Edwards Aquifer and for flood mitigation (ecological dimension). In 2020, this resulted in the protection of 28,000 acres of land, predominantly as parks (both social and ecological dimensions). Additionally, Austin’s Watershed Protection Department has implemented a pilot program to reverse damage to the aquifer by incentivizing homeowners (social dimension) to install rain gardens and cisterns (technological dimension). These initiatives involve social support and technological advances to reestablish ecosystem services and create an urban system with more resilience to water stress and flooding. Simultaneously, these measures support greater biodiversity, human health and well-being, and work to reduce social disparities and vulnerability, further stabilizing the ecological and social dimensions and contributing to greater urban resilience (Bixler et al., 2020).
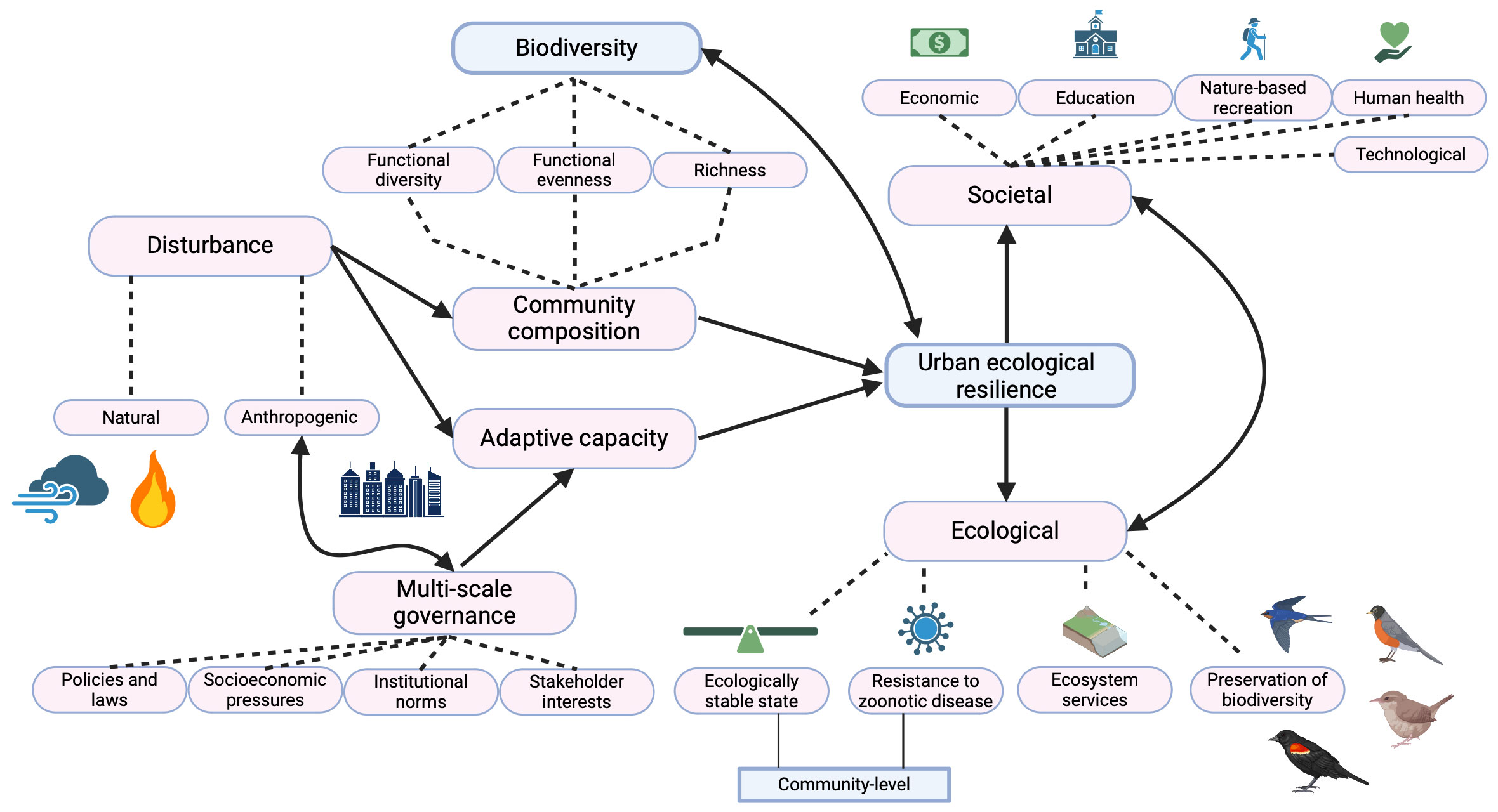
Figure 1 Diagram of the high-level influencing factors of urban ecological resilience, and their implications on societal and ecological levers. Solid arrows represent the directionality of influence, while dotted lines connect subcategories to their parent factor. Created with biorender.com.
Here, we examine the ecological dimension of urban resilience, with a focus on avian biodiversity. We provide a brief overview of typical environmental stressors that impact avian biodiversity in the urban environment and evaluate urban attributes that may mitigate these stressors. We then discuss socioeconomic associations with biodiversity and conclude with recommendations for future research.
1.1 Bird communities as proxies of urban ecological resilience
Birds are often the focus of urban ecological studies (Collins et al., 2021) due to their high visibility, abundance, and ability to be observed across spatial scales, offering valuable insights into how ecosystems respond to urban development (Lepczyk and Warren, 2012). At the same time, bird populations can serve as indicators of the overall health of urban environments (Pollack et al., 2017; Morelli et al., 2021), helping city planners and policymakers make informed decisions for a more sustainable and resilient future. In addition, bird watching is a hugely popular activity, with avian diversity appreciated by human residents (Clergeau et al., 2001) and as a component of total biodiversity can impact human psychological well-being, sense of community, and identity (Horwitz et al., 2001). Therefore, this paper focuses on bird biodiversity (e.g., species and functional diversity) as an indicator of urban ecological resilience (Alberti and Marzluff, 2004; and references therein).
Urbanization tends to result in higher densities of birds than that of surrounding, undeveloped areas. However, the resilience of these urban avian communities, as measured by species richness and species density (i.e., the number of species per unit area), tends to be reduced (Chace and Walsh, 2006; Aronson et al., 2014). Strong evidence indicates that urban avian communities experience biotic homogenization, resulting in global reductions in taxonomic diversity and evolutionary uniqueness (Morelli et al., 2016; Ibáñez-Álamo et al., 2017), although the strength of these effects varies regionally (Ibáñez-Álamo et al., 2017). Urban environments exert especially strong, homogenizing selection on the foraging and nesting habits of birds (reviewed in McCloy et al., 2022). Granivory, omnivory, aerial insectivory, ground foraging, secondary cavity-nesting, and cup-nesting are traits that are typically favored by urban environments, while surface foraging insectivory, carnivory, primary cavity-nesting and ground-nesting traits are selected against (Emlen, 1974; Allen and O’Connor, 2000; Lim and Sodhi, 2004; Blewett and Marzluff, 2005; Chace and Walsh, 2006; Croci et al., 2008; Evans et al., 2011; Guetté et al., 2017; Tomasevic and Marzluff, 2017). Thus, compared to undisturbed habitats, urban environments frequently display both reduced avian biodiversity and ecological resilience.
2 Urban environmental stressors
Four environmental stressors are present in nearly all urban habitats across geographic zones: chemical pollution, noise, artificial light at night (ALAN), and human presence (Isaksson, 2018). Chemical pollution in urban areas is associated with reduced avian survival (Mitra et al., 2011; Kekkonen, 2017), and is an ongoing concern for both ecological and human health in urban areas (Bolund and Hunhammar, 1999; Cristiano et al., 2021). The most common pollutants in urban areas, globally, are those generated by the combustion of fossil fuels by cars (i.e., nitrogen oxides and soot) (Isaksson, 2018). Even in the relatively sparsely populated cities of Sweden, chemical pollution is high enough to cause negative effects in birds and humans (Salmón et al., 2018). Heavy metal pollution is also common in urban areas, although this varies regionally, depending on locations of polluting industries (reviewed in Isaksson, 2018).
Urban ambient noise is also well recognized to reduce bird species richness (Ciach and Fröhlich, 2017; Pena et al., 2017; Barbosa et al., 2020; da Silva et al., 2021). As with many urban characteristics, urban noise can impose niche filtering selection on avian species, favoring habitat generalists whose vocalizations are outside of the range of anthropogenic noise (0-3 kHz; reviewed in Barbosa et al., 2020). Apart from noise, urban birds can be disturbed by the presence of humans. Although urban birds do exhibit some tolerance for human presence (e.g., shorter flight initiation distances than rural conspecifics, reviewed in Evans et al., 2009), negative impacts of human disturbance on bird abundance and species richness persist (Figure 2). For example, human disturbance, including recreational activities, negatively correlates with avian diversity in urban green spaces (Kang et al., 2015), and breeding bird densities tend to be reduced when human disturbance is high (reviewed in Evans et al., 2009).
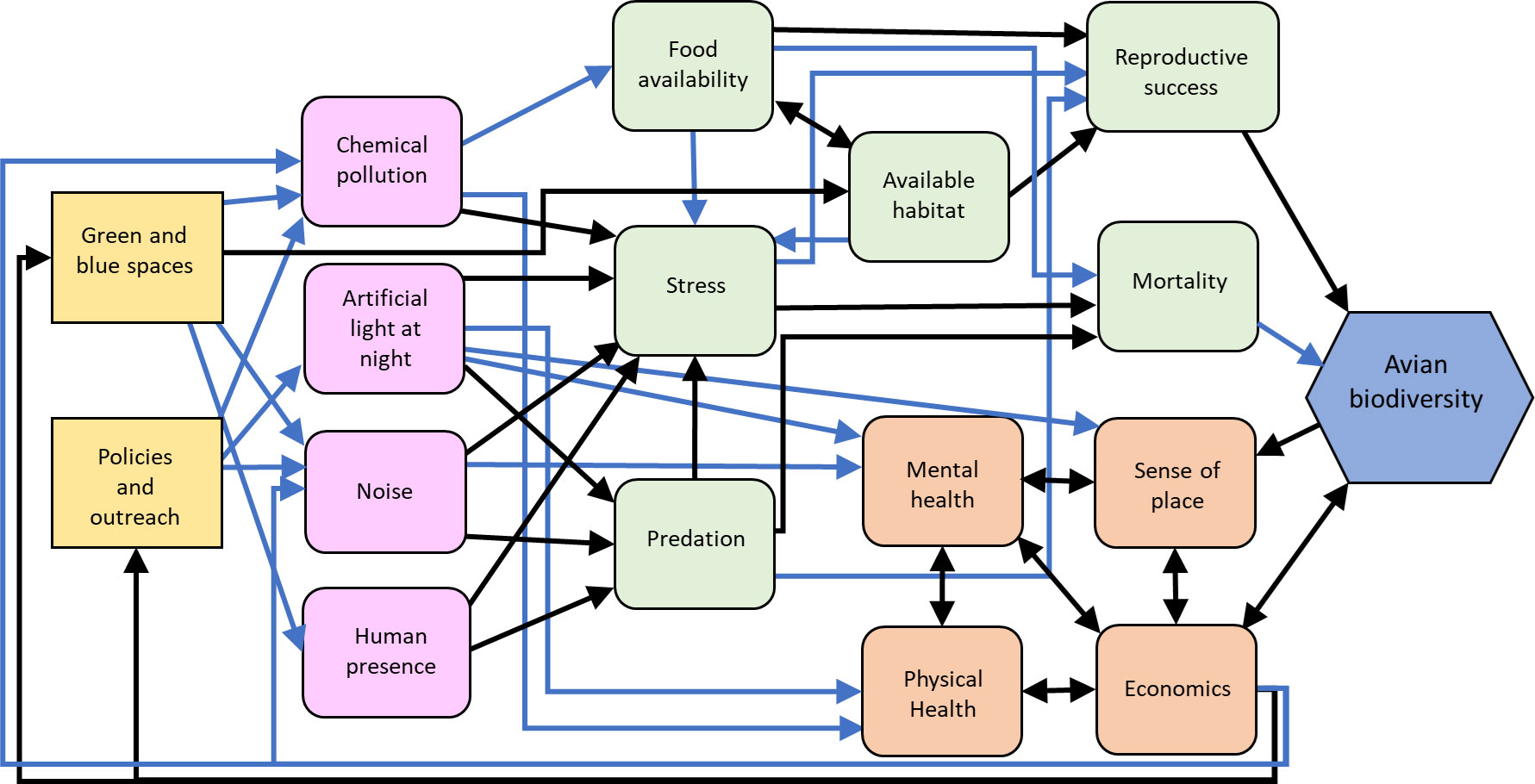
Figure 2 Influence diagram of the relationships between environmental stressor management actions (yellow squares), intermediate stressor (pink squircles), human (orange squircle) and environmental (green squircle) processes, and avian biodiversity (blue hexagon) as a metric of resilience. Black arrows indicate predominantly positive relationships, blue arrows indicate predominantly negative relationships. Created with biorender.com.
ALAN is also of growing concern for human and ecological health (Cupertino et al., 2023), but existing policy structures are poorly designed to address the growth of ALAN or mitigate its impacts (Burt et al., 2023). Researchers have clearly established that ALAN disrupts animal movements and orientation, especially for nocturnally migrating birds of which over a billion individuals are killed annually in collisions with buildings (Burt et al., 2023; Loss et al., 2023). At the individual level, experimental ALAN has been linked to shifts in avian circadian rhythms (de Jong et al., 2016), while also promoting physiological changes linked to decreased brain plasticity (Moaraf et al., 2020, 2021), endocrine dysregulation (Injaian et al., 2021), early reproductive timing (Dominoni et al., 2013), and temporarily increases innate immune activity in birds (Saini et al., 2019). With the widespread use of ALAN in urban habitats, birds are exposed to systemic physiological disruption. which has been shown in humans to negatively affect individual resilience by inflicting a “wear and tear” cost (Oken et al., 2015). At the community level, Morelli et al. (2021) found that light pollution had a significant filtering effect on urban bird communities, leading to taxonomic and functional homogenization in cities across Europe. The homogenizing effect of ALAN on urban bird communities directly correlates to reduced functional and alpha diversity, which in turn, suggests reduced avian community resilience.
Urban areas also modify albedo (reflectivity) and evapotranspiration (the combined water vapor released by plants and evaporation from surfaces), while introducing increased aerosols and anthropogenic heat sources. These factors lead to elevated temperatures, and thus urban environments tend to be warmer than surrounding less developed areas by up to 10°C (Figure 3; Dimoudi et al., 2013; Sharifi and Lejmann, 2015) i.e., the “Urban Heat Island” effect, (reviewed in Aram et al., 2019), an effect that is expected to increase under climate change scenarios (Aram et al., 2019; Leveau et al., 2021). High temperatures associated with urbanization can be a stressor across cities (Cai et al., 2023), but their effect may be especially pronounced in arid environments and those with already high temperatures (e.g., du Plessis et al., 2012), or where species are not adapted to warm temperatures (e.g., Oliver et al., 2017). Conversely, high temperatures may be beneficial to species diversity where cold winter temperature is a limiting factor (Bowler et al., 2018).
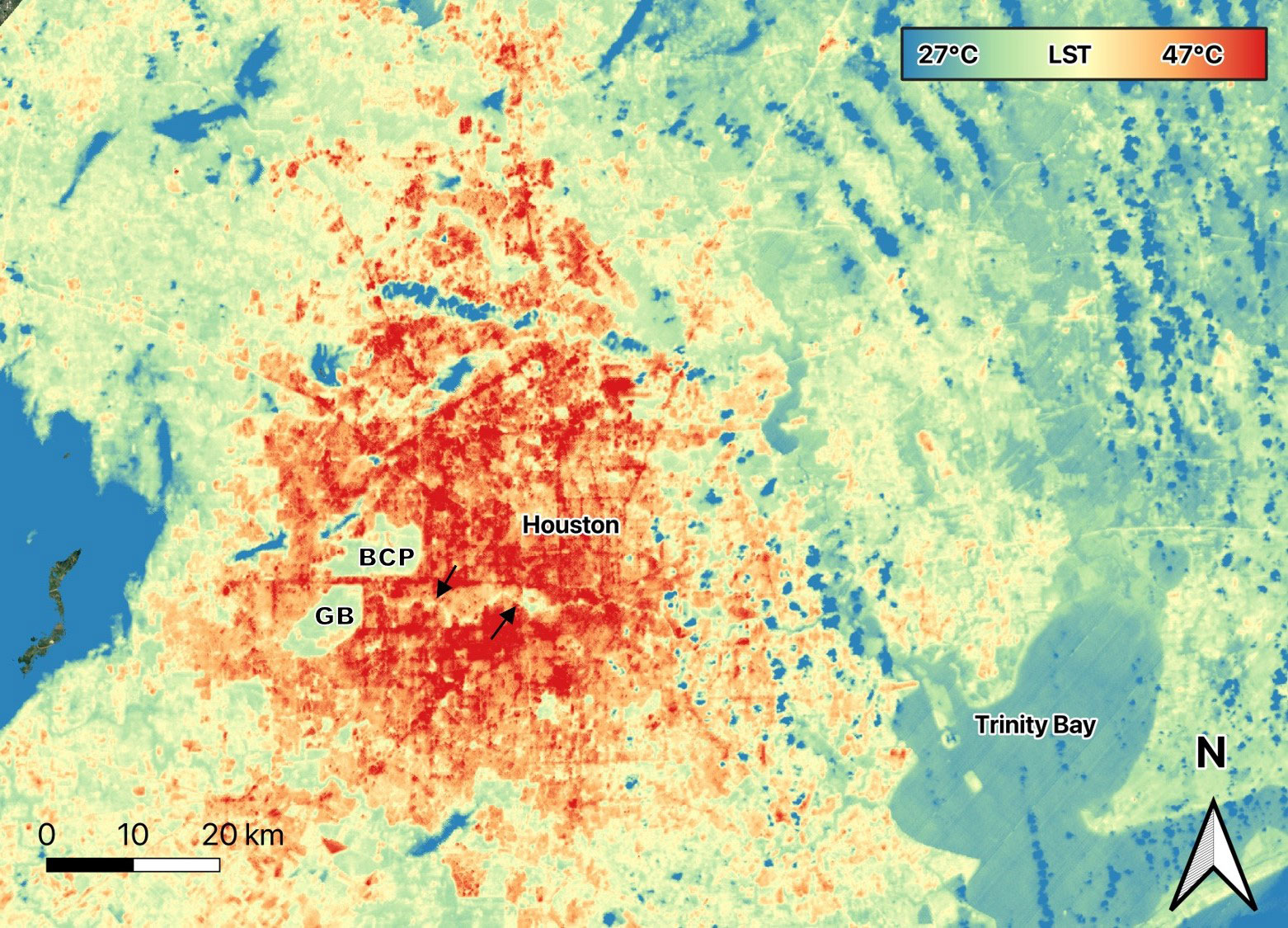
Figure 3 Land Surface Temperature (LST) of Houston, Texas on June 13, 2023 at 17:05 local time. Streets and areas with more buildings are hot spots reaching 47°C. Large parks, Bear Creek Pioneers Park (BCP) and George Bush Park (GB) on the western side of the city are cooler. Green spaces along Buffalo Bayou (arrows) running eastward from these large parks are also cooler (Image from data generated by NASA’s ECOsystem Spaceborne Thermal Radiometer Experiment (ECOSTRESS) mission, Jet Propulsion Laboratory, a division of Caltech in Pasadena, California.
Changes in food abundance, predation, and pathogens relative to adjacent undisturbed areas are additional urban environmental stressors which may affect species diversity and richness of birds (Isaksson, 2018). Anthropogenic food resources in urban areas tend to be patchy, yet relatively predictable. For example, supplementary feeding (i.e., the intentional provision of food by humans to wild birds that supplements their diet) is a widespread phenomenon and regular activity in backyards of developed countries (Reynolds et al., 2017). While our definition frames supplementary feeding as an intentional act, we note that humans unintentionally supplement the diets of birds through discarded waste. Despite increases in anthropogenic food in urban areas via intentional and unintentional supplementary feeding, urbanization may reduce the availability of natural foods (Seress et al., 2018), especially where non-native plants are abundant (Narango et al., 2018). Moreover, reduced diversity of avian food items appears to be a consistent trait of urban environments, regardless of supplementary feeding customs (Isaksson, 2018).
The effect of urbanization on avian predators likely varies with behavioral and physiological traits, and life-histories that predict sensitivity to disturbance (Rodewald and Gehrt, 2014; Boal, 2018). Apex predators have been shown to avoid urban areas (Blecha et al., 2018; Ellington and Gehrt, 2019; Soccorsi and LaPoint, 2023) which may facilitate mesopredator release (Crooks and Soule, 1999). Concomitantly, increased availability of anthropogenic foods may promote small to mid-sized generalist predators (e.g., northern racoons [Procyon lotor] and Virginia opossums [Didelphis marsupialis]), although results are highly variable amongst studies (Rodewald and Gehrt, 2014). Notably, urbanization may also increase densities of free-ranging domesticated cats (Felis catus) (Haskell et al., 2001), although these effects may be mediated by human land use and demographics (Bennett et al., 2021). However, despite increased predation risk from at least some predators, predation rates on birds tend to be higher in rural areas compared to urban areas (Eötvös et al., 2018). The presence of anthropogenic foods in urban areas may underscore this apparent ‘predator paradox’ (Rodewald and Gehrt, 2014).
Finally, although pathogen abundance and diversity is moderated by urban climate and the presence of water bodies, pathogen transmission is generally higher among birds in urban environments (Isaksson, 2018), due to increased bird density (Chace and Walsh, 2006; Aronson et al., 2014) and effects associated with supplementary feeding (Adelman et al., 2015; Galbraith et al., 2017). However, it is worth noting that the prevalence of birds infested with ticks has been shown to be lower in urban areas (Roselli et al., 2022) suggesting that urbanization may reduce the transmission of tick-borne pathogens, but further study is needed to address this idea.
3 Urban management strategies that can promote avian biodiversity and urban ecological resilience
Urban bird species richness and density appear to be more strongly influenced by local factors than regional factors (Evans et al., 2009). This is good news for urban neighborhoods attempting to increase biodiversity, because it suggests that local management has the potential to increase species richness, biodiversity, and functional diversity. These improvements can enhance urban ecological resilience to future disturbance. Management to increase biodiversity in urban environments generally aims to directly or indirectly reduce the strong selective effects of biotic homogenization and niche filtering on wildlife. Below, we discuss several approaches to promoting avian biodiversity, and thus urban ecological resilience. These approaches are not an exhaustive list of management strategies for avian biodiversity in urban habitats, but rather a selection of readily understood and frequently used management frameworks.
3.1 Urban green and blue spaces
Avian biodiversity can be increased through investment in complex urban green (e.g., parks, gardens, and street trees) and blue spaces (e.g., water bodies, streams, rivers, and wetlands) that utilize a diversity of native vegetation. Several studies indicate that urban land cover and the proportion of built infrastructure are negatively associated with bird species density and richness (Aronson et al., 2014; Suárez-Castro et al., 2022), although measures of functional diversity may be more complexly related to urban infrastructure (Suárez-Castro et al., 2022). Urban green and blue spaces can increase bird species density and provide refugia for biodiversity (Figure 4; e.g., Suri et al., 2017; Barbosa et al., 2020) by reducing impervious surfaces and their associated homogenizing effects. In a Neotropical avian community, at least one study indicates that although urban parks exhibit lower resiliency compared to preserved continuous forests, they are important components of urban ecological resilience. This is reflected in reduced species richness, feeding guild richness, and within-scale redundancy, but similar functional richness, functional diversity, functional evenness, and cross-scale redundancy in urban parks as compared to preserved continuous forest (Estevo et al., 2017).
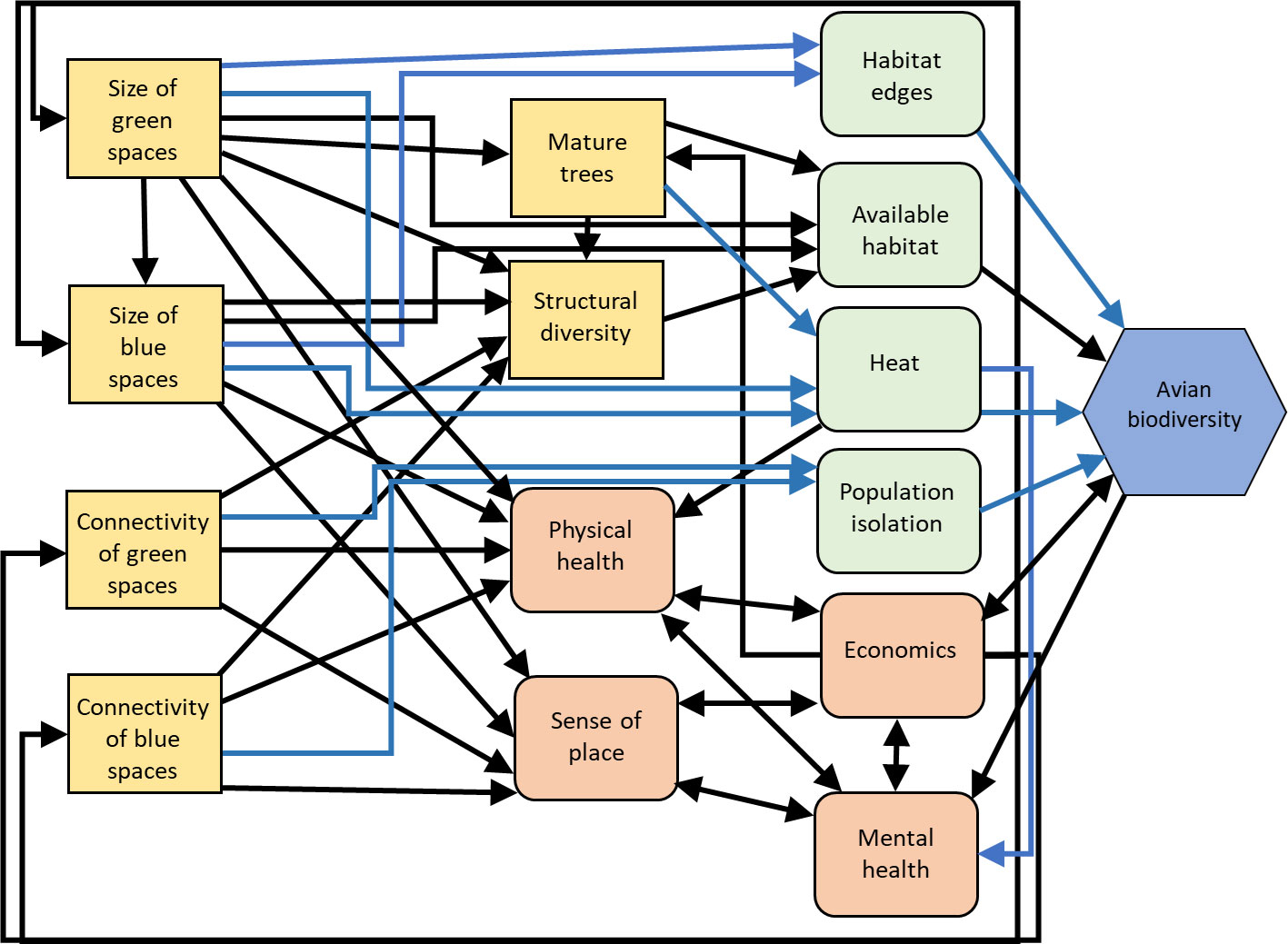
Figure 4 Influence diagram of the relationships between management actions for urban green/blue spaces (yellow squares), intermediate human processes (orange squircle) and environmental processes (green squircle) and avian biodiversity (blue hexagon) as a metric of resilience. Black arrows indicate predominantly positive relationships, blue arrows indicate predominantly negative relationships. Created with biorender.com.
Urban green spaces with diverse, mature native vegetation, and standing dead trees appear to provide the strongest benefits to avian species richness (Campos-Silva and Piratelli, 2021; da Silva et al., 2021). The presence of mature trees in urban green spaces increase both bird taxonomic and functional richness (Morelli et al., 2017), and water bodies or rivers/streams increase both taxonomic richness and functional diversity (Morelli et al., 2017; Suri et al., 2017; Barbosa et al., 2020). Numerous studies provide overwhelming support for the importance of woody vegetation in increasing overall avian biodiversity (Evans et al., 2009; Fontana et al., 2011; Morelli et al., 2017; Kaushik et al., 2022) and the richness and abundance of native resident bird species (Rico-Silva et al., 2021). However, heterogeneous habitat designs have the strongest positive effects on avian biodiversity (Clergeau et al., 2001; Evans et al., 2009). The presence of native vegetation composition and structure, vegetation complexity, and habitat connectivity between urban green and blue spaces are all positively correlated with the number of native bird species and avian biodiversity (Chace and Walsh, 2006; Kang et al., 2015; Jasmani et al., 2017; Isaksson, 2018; Beaugeard et al., 2021), probably because these factors alleviate both the stress of decreased food diversity and changes to predator dynamics, at the same time as providing habitat and corridors in which to travel.
Larger urban green spaces generally provide greater benefits to avian biodiversity (i.e., species richness, Shannon diversity, and functional diversity) than smaller spaces (Evans et al., 2009; Kang et al., 2015; Callaghan et al., 2018; Kaushik et al., 2022). However, small parks can still be important for avian diversity if several are distributed throughout an urban area. Networks composed of these small parks can support higher species richness compared to larger urban parks, in part due to increased use of these networks as stopover sites by migrating species (La Sorte et al., 2023). Large parks likely enhance avian biodiversity more so than isolated small or medium sized parks due to a higher prevalence of mature trees and water bodies, diverse vegetation zones, reduced edge effects, and the capacity to support larger, more stable wildlife populations (Evans et al., 2009; Aram et al., 2019). However, small public urban green spaces are often more feasible to implement, especially in medium and low-income neighborhoods, and thus should also be considered to play an important role in maintaining biodiversity (Jasmani et al., 2017; da Silva et al., 2021). Many of the benefits that large urban green spaces provide can be applied to planning small urban green spaces and gardens, for example: diverse native vegetation, complex vegetation structure, and water bodies (Evans et al., 2009). The negative effects of edge and isolation on biodiversity can be mitigated in small urban green spaces by providing green or blue corridors to connect these spaces (Evans et al., 2009). Where limits to species dispersal and gene flow are identified, “rewilding” or the introduction of native species to urban areas may be a useful management tactic (Lambert and Donihue, 2020). More research is needed on the role of small urban green spaces in avian community richness/diversity, and the costs and benefits of different types of connecting corridors (e.g., power lines, pipe lines, wildlife bridges, etc.) and barriers (e.g., fences, roads, hedges, etc.).
In addition, urban green spaces offer opportunities for recreation, relaxation, and connection with nature, improving the overall well-being and mental health of city dwellers (Akpinar et al., 2016; World Health Organization, 20161). Urban green spaces can also contribute to the cultural identity and sense of place for urban communities (Seeland et al., 2009; Keleg et al., 2021). Blue spaces (including man made surface water) are also associated with improved human well-being and mental health (Smith et al., 2021). Additionally, these urban green and blue spaces can attract tourists, boost property values, and support local economies through recreational activities, eco-tourism, and green businesses (Vance and Hedel, 2007; Brownstone and Golob, 2009; Dodman, 2009), boosting the overall resilience of a city.
Urban green and blue spaces can also provide ecosystem services by reducing noise pollution, and removing chemical pollutants from the air and water, benefiting humans and wildlife, alike. Vegetation can reduce noise pollution by directly acting as a sound barrier, and providing positive benefits to biodiversity that mitigate the negative effects of noise (Pena et al., 2017). Diverse assemblages of mature native trees appear to provide the highest benefit to bird species richness in noisy urban areas, probably due to nesting site preferences and the availability of resources, such as arthropods (Pena et al., 2017). Vegetation in urban green spaces can also reduce air pollution through filtering (Bolund and Hunhammar, 1999). Filtering capacities increase with more leaf area, thus large, coniferous trees have the highest filtering capacities (especially since they are green year round), and bushes and grasslands the lowest (Bolund and Hunhammar, 1999). However, coniferous trees are generally more sensitive to pollutants than deciduous trees, which are also better at absorbing gasses. Thus, a mixture of tree types appears most desirable to filter air pollutants (Bolund and Hunhammar, 1999). Water pollution can be mitigated through the use of urban wetlands to treat urban sewage or bioswales to reduce contaminants in urban run-off (Anderson et al., 2016). Wetlands can absorb large amounts of nutrients and allow particles to settle by slowing the flow of sewage (Bolund and Hunhammar, 1999).
Urban green and blue spaces also provide substantially cooling benefits to mitigate the urban heat island effect (Bolund and Hunhammar, 1999), benefiting human health and well-being while simultaneously providing relief from the homogenizing effects of heat on ecological communities. Urban parks and forests with sizes of greater than 10 hectares appear to provide the largest cooling benefit, reducing temperatures by 1-2°C for up to 350m beyond the park boundary, depending on vegetation cover and tree shade (Aram et al., 2019). Estimates of cooling intensity and distance from urban blue spaces vary widely, depending on the area, shape, depth, movement, and surrounding features of the water body (reviewed in Zhou et al., 2023), but can reach reductions up to 5°C for a 270 m wide section of river in Japan (Murakawa et al., 1991). This cooling benefit is even higher when blue spaces are integrated into urban green spaces (e.g., Figure 2). For example, a 30m green buffer on either side of an urban river can reduce land surface temperatures by 3.1 - 3.6°C compared to the blue space, alone, and 2.7 - 3.7°C compared to the green space, alone (Zhou et al., 2023). Thus, large urban parks with water bodies not only provide diverse habitat for wildlife, but can also reduce heat stress for both wildlife and humans alike.
The benefits provided to avian biodiversity by urban green and blue spaces depend on the recreational use of the green space, and human traffic surrounding the green space. Sports facilities (e.g., soccer fields, baseball fields, football stadiums) have few, if any, mature trees, low percent canopy covers, low vegetation diversity, and higher environmental stressors such as human presence and artificial light. These factors limit their usefulness as wildlife habitat. Traffic of humans and their commensals (e.g., dogs) through greenspaces can also be quite high, with associated disruption to nesting and foraging activities of birds (Banks and Bryant, 2007; but see Forrest and St. Clair, 2006). Increasing cover habitat may decrease disturbance in these instances, by decreasing alert and flight distances of birds (reviewed in Evans et al., 2009).
3.2 Community engagement programs
Community engagement which brings awareness to the stressors faced by urban birds is an important component of many strategies to increase urban biodiversity, and thus ecological resilience. This community engagement can be achieved via outreach programs, citizen science projects partnering professional researchers with members of the public to collect scientific data (Cooper et al., 2007), or community science approaches linked to social action (Cooper et al., 2021). For example, Lights Out Texas! (Audubon Texas, 20232) has worked to spread awareness of the negative effects of ALAN on migrating birds through education. By encouraging community members to turn out lights, the program likely bolsters bird communities by decreasing avian mortality (Lao et al., 2020; Van Doran et al., 2021). Similarly, the American Bird Conservancy’s ‘Cats Indoors’ program provides resources to promote responsible cat ownership behaviors that reduce cat-caused avian mortality (American Bird Conservancy, 20213). Other programs, such as the National Wildlife Federation’s certification program of residential yards with wildlife-friendly native landscaping, aim to actively increase both biodiversity and ecological resilience at a hyper-local level (Widows and Drake, 2014). This benefit of native plants is illustrated by frequently increased richness of local avian communities (Campos-Silva and Piratelli, 2021; da Silva et al., 2021).
Citizen science efforts may also promote public awareness of the biodiversity crisis, engaging community members in conservation research while also contributing to scientific datasets. Data contributed to citizen science programs such as eBird (Sullivan et al., 2009) and iNaturalist (iNaturalist, 20244) can be used to assess biodiversity metrics in urban spaces (Callaghan et al., 2018) and inform natural resource management and conservation plans (McKinley et al., 2017) that have the potential to increase urban ecological resilience. One of the longest running citizen science examples is the National Audubon Society’s Christmas Bird Count, which has been in existence since 1900 (National Audubon Society, 2024b5) and brings together birdwatchers of all experience levels. Knowledge about local biodiversity also promotes conservation ideals amongst the general public and can lead to an enhanced sense of place and responsibility for the natural world (Cosquer et al., 2012) alongside associated mental health benefits (Horwitz et al., 2001) increasing the overall resilience of our towns and cities. Though citizen science programs for biodiversity awareness exist across taxa, they are perhaps known best through their use in the identification of birds.
Recreational birdwatching may also be viewed as a popular form of community engagement, with 45 to 85 million individuals in the United States (US) self-identifying as birdwatchers (Cordell, 2013; Carver, 2009). Birdwatchers are more likely to engage in conservation behaviors that enhance wildlife populations than people who do not engage in wildlife-focused recreation (Cooper et al., 2015). More recently, initiatives such as Black Birders Week (National Audubon Society 2024a6) and the National Park Service’s Outdoor Recreation Legacy Partnership (National Parks Service, 20247) aim to connect underserved urban communities with outdoor recreational opportunities. As community engagement efforts grow across social and economic boundaries with active inclusivity promotion, their importance in enhancing urban ecological resilience is likely increasing. Increasing human awareness of biodiversity is a critical component of a.) fostering successful conservation efforts with a net positive ecological impact, and b.) ensuring that strategies to strengthen and maintain urban ecological resilience persist within a system long-term.
3.3 Supplementary feeding
Providing backyard bird food (hereafter ‘supplementary feeding’) such as seeds, grains, and suet is common practice in urban areas in much of the western world (i.e., countries populated by people originating from Europe), especially in areas that experience relatively cold conditions during winter (e.g., northern Europe, North America; Reynolds et al., 2017). In addition, where nectivorous birds frequent residential areas (e.g., South Africa, New Zealand), people regularly provide sugar water in artificial nectar feeders (Coetzee et al., 2021; Erastova et al., 2021). While supplementary feeding may be unintentional (e.g., through discarded waste), here we focus on the intentional provision of food (e.g., via bird feeders). The scale of supplementary feeding is staggering; in the US alone, 70.5% of people 16 years or older intentionally feed wild birds annually, spending approximately US$4 billion on bird food, and an additional US$10 billion on associated hardware, annually (US Fish & Wildlife Service, 2018). The energy inputs represented by this extensive practice are likely vast, as are the potential number of birds supported; estimates suggest that provisioning levels in the UK alone are sufficient to fully support 196 million backyard birds (Orros and Fellowes, 2015).
Correlational studies suggest that supplementary feeding supports diverse communities of birds in backyards (Plummer et al., 2019), and that feeders may support birds experiencing declines in natural food availability (Figure 5; Chamberlain et al., 2005). Similarly, experimental approaches demonstrate that avian abundance and diversity may increase in response to commonly provided food types including bread and wild bird seed (Galbraith et al., 2015; Lamberson, 2022). However, such results are typically underscored by increases in granivorous and omnivorous species that occur within the urban species pool (Lerman et al., 2021), while the abundance of species infrequently seen at feeders (e.g., insectivores) may decrease, possibly in response to the presence of dominant heterospecifics (Galbraith et al., 2015). Under scenarios where nectar is provided in artificial feeders, the abundance of nectar-specialists may also increase (Sonne et al., 2016; du Plessis et al., 2021). Supplementary feeding has also been shown to increase species-richness and the abundance of mammals (Reed and Bonter, 2018; Hansen et al., 2020), while decreasing the abundance of invertebrates (ground-beetles: Coleoptera: Carabidae), potentially driven by increased avian predation (Orros et al., 2015). Therefore, supplementary feeding may restructure communities rather than increase overall biodiversity, and thus whether it aids in urban ecological resilience is unclear and warrants further investigation.
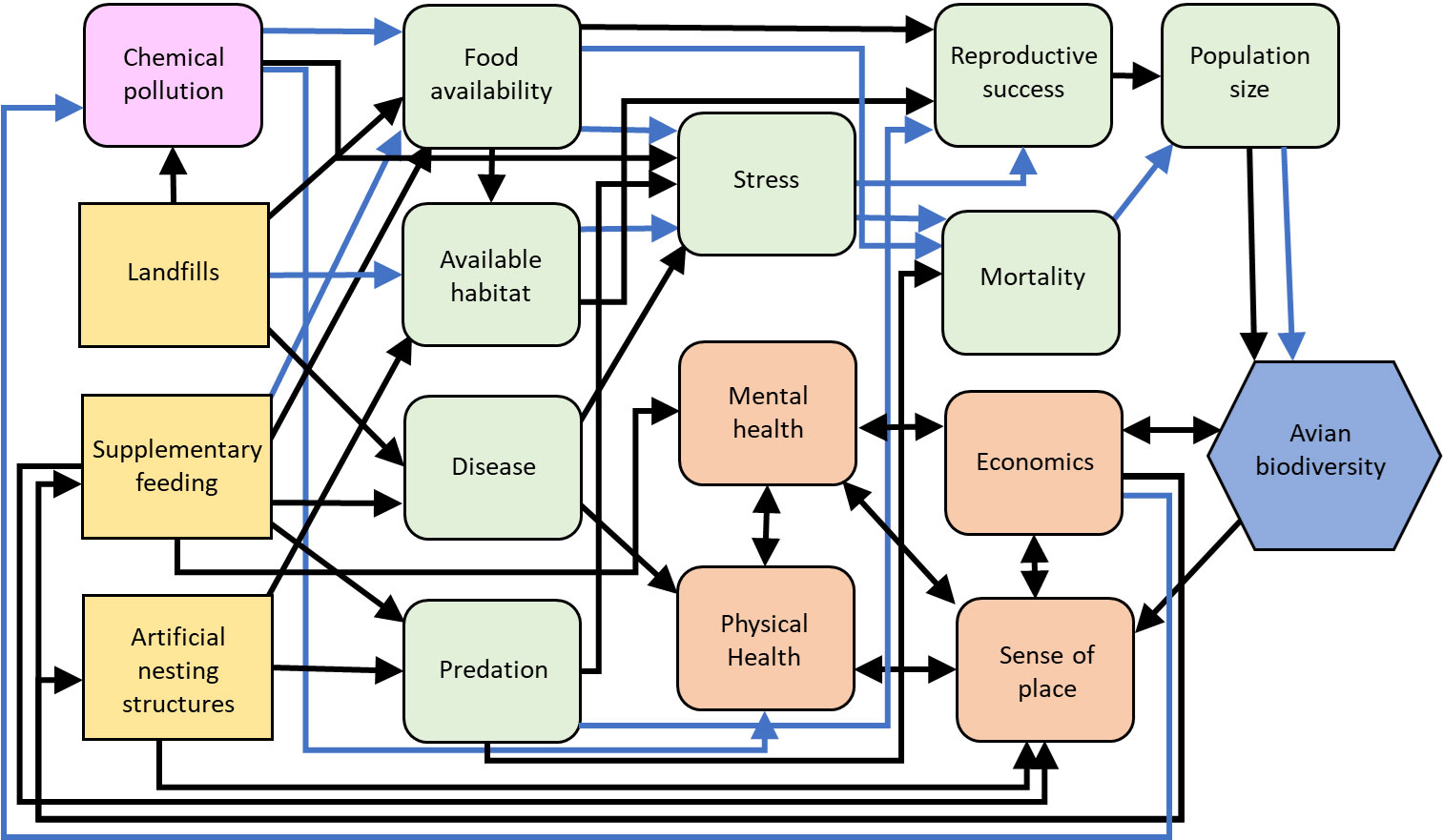
Figure 5 Influence diagram of the relationships between the management actions (yellow squares) from our case studies, intermediate stressor (pink squircles), human (orange squircle) and environmental (green squircle) processes, and avian biodiversity (blue hexagon) as a metric of resilience. Black arrows indicate predominantly positive hypothesized relationships, blue arrows indicate predominantly negative hypothesized relationships. Supplementary feeding can have both positive and negative effects on food availability because it generally increases food availability for granivorous, omnivorous, and nectivorous birds, but food quality and the availability of insects may be reduced. Population size can also have positive and negative relationships with avian biodiversity because large populations of some species can reduce or eliminate those of other species through competition. Created with biorender.com.
While supplementary feeding may appear to bolster biodiversity in some cases, it is important to consider the potential negative effects on birds (reviewed in Shutt and Lees, 2021) in discussions concerning urban ecological resilience. For example, while supplementary feeding may promote metrics of health in wild bird populations (e.g., increased antioxidant levels, reduced stress levels; Wilcoxon et al., 2015), it may facilitate disease transmission (Figure 5) where feeders serve as sites for increased bird interactions and as reservoirs for pathogens (Adelman et al., 2015; Galbraith et al., 2017). Such effects may be amplified where the density of bird feeders is high (Moyers et al., 2018). Concomitantly, while the majority of studies suggest birds experience enhanced breeding productivity in response to supplementary feeding (reviewed in Ruffino et al., 2014), others have demonstrated negative effects in this regard (e.g., reduced clutch size - Harrison et al., 2010; reduced offspring body condition and survival - Plummer et al., 2013; skewed offspring sex ratios - Clout et al., 2002). Furthermore, supplementary feeding may affect predator-prey relationships by increasing the abundance of predators (Hanmer et al., 2017; Malpass et al., 2017) although further research is needed to explore this idea further. Where the provision of nectar in artificial feeders attracts nectivorous birds, visitation rates at flowers may decrease, thus potentially disrupting pollination services provided by birds (du Plessis et al., 2021).
3.4 Urban landfills
The creation of urban-based landfills to store and manage solid waste produced in urban areas removes suitable habitats for many wildlife species. Yet, landfills can provide food subsidies that support scavenging, carnivorous, and omnivorous birds including species of gulls (Burger and Gochfeld, 1983; Belant et al., 1998; Duhem et al., 2008; Ackerman et al., 2018), raptors (Burger and Gochfeld, 1983; Turrin et al., 2015; Al Fazari and McGrady, 2016), corvids (Marasinghe et al., 2018), and wading birds (Burger and Gochfeld, 1983; Singha et al., 2002; Ciach and Kruszyk, 2010; Dorn et al., 2011). Supplementary food from landfills can compensate for reductions in natural food sources (Duhem et al., 2008; Dorn et al., 2011; Ackerman et al., 2018) and support endangered species (Singha et al., 2002; Dementieieva et al., 2023). Certainly, proximity to landfill can be a major determinant in explaining the composition and stability of urban bird communities (Ciach and Fröhlich, 2017).
Landfill design depends on its size, location, and the specific regulations and practices followed in an area. Most contain a drop-off area where garbage is unloaded and several cells or disposal areas where waste is deposited. Once an active disposal site is filled, it is covered with soil, clay, or an impermeable material to prevent leaching. Then, waste deposition shifts to a new cell, and the process continues. Modern landfills are designed to minimize environmental impacts and have leachate collection systems to capture liquid that percolates through the layers of waste and gas collection systems to prevent methane from escaping into the atmosphere. Proper stormwater management systems are established to prevent rainwater from accumulating in the landfill (United States Congress, 1976). Thus, modern landfills can provide a variety of avian habitats (Figure 5) from wetland stormwater collection sites, active dumping sites, closed dumping sites that are planted with grasses, and perimeter areas that are often forested (Marasinghe et al., 2018; Arnold et al., 2021).
Avian abundance tends to be greatest in areas of active dumping, while avian diversity and evenness is greatest at closed dumping areas (Marasinghe et al., 2018). Other features of urban landfills that promote avian diversity are tree cover (Malekian et al., 2021), distance to communal roosts (Turrin et al., 2015), lower human disturbance (Burger and Gochfeld, 1983), and distance to water (Malekian et al., 2021). Once active dumping sites are filled and capped, grasses are planted and maintained to prevent growth of woody plants whose roots could damage the capping system. These grassy areas can support populations of declining grassland specialists (Arnold et al., 2021) and rural surrounding areas can support species of conservation concern (Dementieieva et al., 2023). Thus, urban landfills can play a crucial role in preserving avian diversity and resilience despite posing hazards to wildlife through exposure to plastics, toxic compounds, and pathogens (Figure 5; Plaza and Lambertucci, 2017). Populations of some species of conservation concern, like white storks (Ciconia ciconia) are predicted to decline if urban landfills are closed (López-García et al., 2023). However, closed landfills can be reclaimed as open urban green spaces (Hoefer et al., 2016).
3.5 Artificial nesting structures
Urban environments offer unique challenges and opportunities for nesting birds. In response to urbanization, birds may exploit novel nesting habitats provided by humans, either purposefully or incidentally. Here, we focus on North American breeding species and define artificial nesting structures as man-made structures that support or contain bird nests, even if they were not specifically built for the purpose of providing nesting resources for birds (Mainwaring, 2015). Artificial nesting structures include, but are not limited to, electric pylons, roads, buildings, pipes, rooftops, nest boxes, burrows, and cavities (Mainwaring, 2015). We do not focus on anthropogenic nesting material (i.e, materials incorporated into birds’ nests that are manufactured by humans) because numerous reviews already exist to connect anthropogenic nesting materials to avian reproductive success, and thus resilience (see Jagiello et al., 2019; Reynolds et al., 2019; Jagiello et al., 2023).
In the ever-changing urban landscape, many bird species have demonstrated remarkable plasticity by colonizing novel nesting sites, effectively expanding their ranges as cities continue to grow. For instance, cliff swallows (Petrochelidon pyrrhonota) have exhibited an expansion of their distribution, seizing opportunities presented by the widespread construction of concrete bridges and highway overpasses (Brown et al., 2020). Similarly, ground-nesting birds including common nighthawks (Chordeiles minor), various gull and tern species (Laridae spp.), and killdeer (Charadrius vociferus) have adopted gravel and “green” rooftops in urban areas as novel nesting sites (Fisk, 1978; Brigham, 1989; Cañero and Redondo, 2010; Washburn et al., 2016). Some birds have become highly reliant on the artificial nesting structures provided by humans. Chimney swifts (Chaetura pelagica) and purple martins (Progne subis), for instance, have become almost entirely dependent on human-provided nesting structures for breeding, often expanding into heavily urbanized habitats in pursuit of suitable nest sites (Bridge et al., 2016). Their human “landlords’’ maintain these nesting sites and often report a strong sense of satisfaction and connection with nature through their involvement in avian conservation efforts, which can positively influence mental health and foster a deeper sense of place within urban communities (Figure 5).
Urban habitats can act as substitutes for natural breeding sites (Martínez-Abraín and Jiménez, 2016). For example, peregrine falcons (Falco peregrinus) have exploited anthropogenic structures, including buildings, electric pylons, and buoys which function as cliffs following population recovery after their drastic population declines related to organochlorine pesticides in the 1940s-1970s (US Fish and Wildlife Service, 1999). Similarly, crested caracaras (Caracara plancus), a species typically associated with open or semi-open habitats (Smith et al., 2017; Morrison and Dwyer, 2021) have successfully exploited unconventional nesting sites, such as advertising billboards and electrical pylons, particularly in environments where natural nesting substrates are limited such as in urban areas (Dwyer and Rosa, 2015), and this may be leading to range expansion (Smith and Dwyer, 2024). This underscores the relatively high nesting substrate plasticity that certain bird species exhibit in urban habitats. At the guild level, primary cavity nesting birds (i.e., those that create their own nesting cavity) are often selected against in urban habitats, due to removal of natural structures for nest excavation (Tomasevic and Marzluff, 2017), but secondary cavity nesting species (i.e., those that nest in existing cavities), will readily transition to nesting in cavities in anthropogenic structures (Tomasevic and Marzluff, 2017) where existing natural cavities are limited.
However, the establishment of urban nesting sites may also have negative consequences for urban ecological resilience. In North America, invasive birds often predominate native birds in urban areas (Green and Baker, 2003), and outcompete native species for nesting resources (Charter et al., 2016). House sparrows (Passer domesticus) and European starlings (Sturnus vulgaris) are synanthropic species that exhibit high plasticity in nesting habitat (Clergeau and Quenot, 2007; Sheldon and Griffith, 2017), and will readily nest in artificial nesting structures, often aggressively removing native cavity nesting birds. Provisioning of artificial nesting structures, particularly for cavity nesters, may facilitate these interactions and support non-native species in urban habitats (Clergeau and Quenot, 2007). These non-native species present a dilemma when assessing urban ecological resilience. Incorporating non-native species into community-level biodiversity metrics for resilience is likely to diminish community resilience due to biotic homogenization and the prevalence of non-native species in urban habitats (Green and Baker, 2003). However, when considering adaptive capacity and behavioral or functional plasticity in resilience metrics, highly successful non-native species may be considered more resilient and adaptive than native birds, thus increasing the resilience of ecological communities. This paradoxical relationship highlights the need for more explicit definitions and metrics for ecological resilience, particularly in urban habitats.
It is clear that artificial nesting structures are a tool that can be used to enhance avian biodiversity at various levels, from landowners to government entities (Savard et al., 2000). Management of these structures will likely increase the positive impacts of artificial nesting on the biodiversity of urban habitats, and thus affect resilience metrics. However, broad-scale and effective management strategies for artificial nesting structures in urban environments are lacking. In light of the vital role that urban nesting bird communities play in enhancing urban biodiversity and contributing to the resilience of urban ecosystems, proactive management strategies aimed at optimizing the benefits of artificial nesting structures are needed.
4 Intersections between avian urban ecological resilience, socioeconomic factors, and technology
Urban ecological resilience is dynamically connected to social, economic, and technological factors (Figure 1). Most human residents of cities reside in areas of low biodiversity, representing “biological poverty” (Melles, 2005). Given that over 50% of the human population now resides in cities, it is probable that most of the global human population now lives under this scenario (Turner et al., 2004; Melles, 2005). The majority of those living in biological poverty appear to be located in densely populated low-income urban communities. For example, studies in Vancouver, Canada and Phoenix, Arizona, US conclude that low-income urban neighborhoods tend to have less diverse avian communities and fewer native species of birds than higher income neighborhoods (Kinzig et al., 2005; Melles, 2005), although this is not always the case (e.g., Chicago, Illinois, US; Loss et al., 2009). Urban human residents appear to perceive and appreciate bird diversity more than abundance (Clergeau et al., 2001) and reduced biodiversity can negatively impact human psychological well-being, sense of community, and identity (Horwitz et al., 2001). Within this context, urban ecological resilience can be considered an environmental justice issue.
Unequal social and economic distribution among urban human populations can affect urban biodiversity through multiple processes. First, the density of the urban area directly affects the amount of habitat available for wildlife, with subsequent effects on biodiversity (Figure 3). The majority of human city dwellers reside in densely populated areas characterized by below-average biodiversity (Turner et al., 2004). Second, the distribution of urban greenspaces is typically skewed toward higher-income neighborhoods (Kinzig et al., 2005). These greenspaces are also usually larger and more well-managed than their counterparts in lower-income neighborhoods (Rigolon, 2016). Larger green spaces typically maintain higher levels of avian biodiversity (Kang et al., 2015; Callaghan et al., 2018), especially when they include large mature trees, diverse habitat zones, and water bodies (Morelli et al., 2017; Suri et al., 2017; Aram et al., 2019; Barbosa et al., 2020). These features are also more likely to occur in neighborhoods of higher socioeconomic status (Rigolon, 2016). Of course, there are some exceptions such as large National Wildlife Refuges or other not locally-funded reserves that were created to protect unique and important habitats, irrespective of their relationship with human settlement.
Third, homeowner landscaping choices can affect biodiversity outside of urban greenspaces (Kinzig et al., 2005). Although isolated small urban greenspaces are not as effective at increasing avian biodiversity as large urban greenspaces (Evans et al., 2009; Kang et al., 2015; Callaghan et al., 2018; Kaushik et al., 2022), small urban greenspaces are often more easily implemented and maintained (Jasmani et al., 2017; da Silva et al., 2021), especially in medium- and low-income neighborhoods. Homeowner landscaping choices may be driven by wealth where wealthier individuals are able to invest more in landscaping options that promote biodiversity (i.e., the luxury effect; Hope et al., 2003). Certainly, positive relationships between wealth and bird diversity have been found in numerous studies (Leong et al., 2018), although the strength of this effect may depend on the level of urbanization (Chamberlain et al., 2019), and the aridity of the area, suggesting issues of environmental justice may be amplified as climate change effects (e.g., drought) increase in severity (Chamberlain et al., 2020). Homeowner landscaping choices that promote biodiversity may also depend on other drivers of behavior including social norms and motivations (Peterson et al., 2012; Goddard et al., 2013).
Finally, the extent of supplementary feeding of wild birds is also strongly driven by housing density and socioeconomics where household income predicts bird-feeding activity (Fuller et al., 2013). Under this scenario, the potential for supplementary feeding to affect avian abundance, species richness, and diversity may only be relevant to a relatively wealthy subset of the human population (e.g., luxury effect; Hope et al., 2003). Bird feeding serves to connect people with nature and increase human well-being (Figure 5; Cox and Gaston, 2016), thus inequalities in bird-feeding activities can also produce inequalities in human psychological health and sense of place. These social and economic determinants of biodiversity and ecological health highlight the dynamic interactions between socioeconomic inequalities in human urban populations, ecological resilience, and urban resilience as a whole.
Biodiverse landscapes can also bring economic benefits to a community. Birdwatchers will often travel to view a rare bird species or species they cannot view in their home region, bolstering the tourism and hospitality industries of the hosting community. For example, over one month in winter 2021-2022 thousands of people traveled to see a Steller’s sea-eagle (Haliaeetus pelagicus) on the North American eastern seaboard, generating an estimated $584,373 - $731,809 (Pease et al., 2023). In 2016, over 300,000 birdwatchers visited Alaska, spending an approximately $378 million and supporting an estimated 4,000 jobs (Schwoerer and Dawson, 2022). Canadian birdwatchers prefer areas of high biodiversity, and in Canada are estimated to spend an additional $0.68 for every additional species on a birdwatching trip (Jayalath et al., 2023). Annual birdwatching festivals such as the Grand Isle Migratory Bird Celebration in Grand Isle, Louisiana, US contribute millions of recurring dollars to the local economy (Isaacs, 2010). Thus, biodiversity and socioeconomic factors are dynamically interlinked in the urban system (Figure 6). Investments in biodiversity can improve the economic position and well-being of a community, and, likewise, improvements in the socioeconomic status of a community can bolster biodiversity.
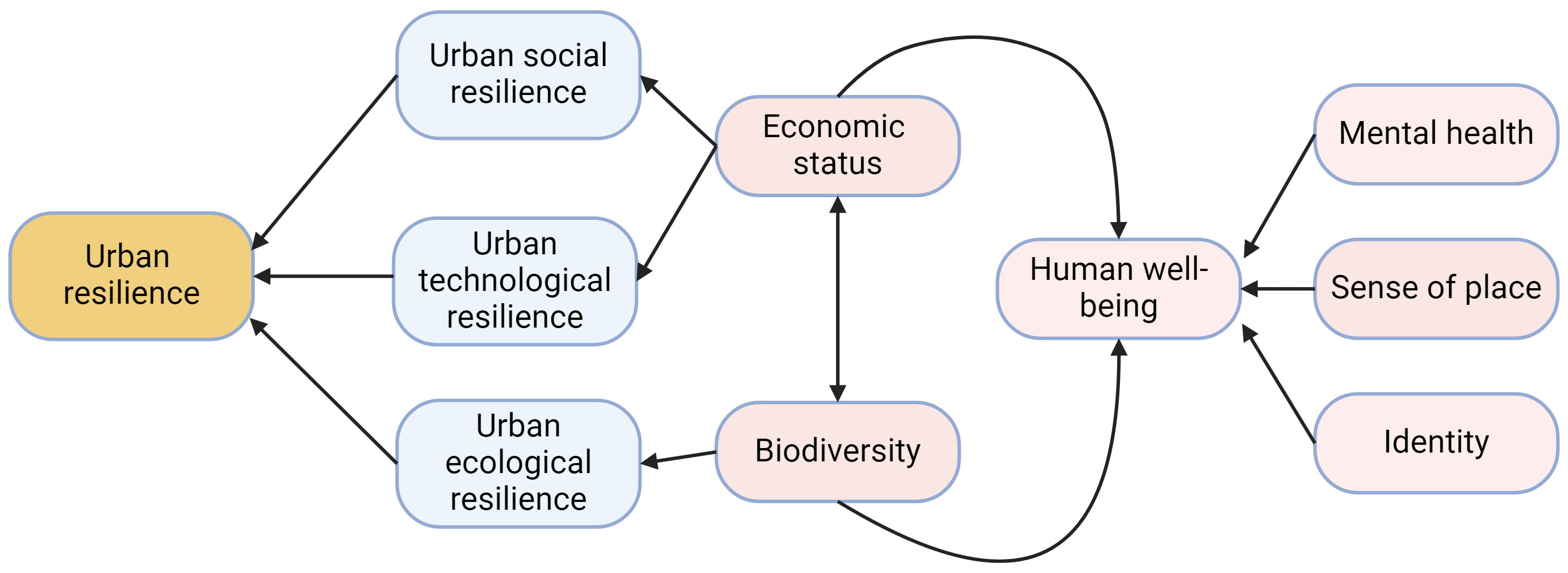
Figure 6 High-level influence diagram of the social, technological, and ecological components (blue squircle) of urban resilience (orange squircle) and how they are influenced by both human well-being (pink squircles) and biodiversity. Black arrows indicate directional relationships, with a two-way interaction between human economic status and biodiversity. Mental health, sense of place, and identity all are core components of human well-being as illustrated in this figure. Created with biorender.com.
5 Conclusions and recommendations
Urban wildlife communities are shaped by selective processes influenced by environmental features, species traits, species interactions, and human socioeconomic factors and behavior, collectively determining which species persist in urban environments. These dynamics highlight the pivotal role of human decision-making in shaping urban bird biodiversity, and thus urban ecological resilience. In this paper we have discussed how urban ecological resilience, using the biodiversity of birds as a proxy, can be altered by characteristics of urbanization, and highlight several local management actions that may promote urban ecological resilience. In particular, there is strong evidence to support the benefits of large green and blue spaces with mature, diverse native vegetation for both avian biodiversity and human physical and mental health.
Synergism between urbanization and climate change may exacerbate their individual negative effects on biodiversity (Travis, 2003; McCloy et al., 2022) and introduce novel dimensions to the selective processes that shape urban avian communities (Pimm, 2009; reviewed in McCloy et al., 2022). For example, Urban Heat Island effects are expected to be amplified as the Earth warms under projected climate change scenarios (Aram et al., 2019; Leveau et al., 2021), leading to the loss of cold-adapted wildlife species (Oliver et al., 2017) and biotic homogenization toward warm-adapted species (Clavero et al., 2011). Urban environments can also create fragmented habitat that restricts the ability of species and communities to track moving climate envelopes (Roberts et al., 2019), as we see with saltmarsh specialist communities (Thorne et al., 2012; Rosencranz et al., 2018).
Despite these negative effects, urban environments can provide relatively stable habitat for species whose ranges are reduced by climate change (reviewed in McCloy et al., 2022), provided the species can adapt to urban environments and/or urban spaces are managed effectively. Urbanization also offers possibilities for effective resource use that can mitigate the effects of climate change, reduce carbon footprints, and increase human well-being and socioeconomic status. Compact urban development with high concentrations of residents and businesses can decrease per capita energy consumption, the distance traveled by vehicles, and carbon emissions (Vance and Hedel, 2007; Brownstone and Golob, 2009; Dodman, 2009), thus reducing climate impacts.
In this paper we use biodiversity (e.g., richness, occupancy, diversity) as a proxy for urban ecological resilience because these measures are straightforward, widely reported, easy to calculate, and frequently used in prior studies to represent ecological resilience (Johnson and Winker, 2010; Karp et al., 2011; Selwood et al., 2015; Irizarry et al., 2021). However, these ‘simple’ methods do not directly consider redundancy or complementarity of species, nor do they account for temporal or spatial heterogeneity in niche space, making them less accurate proxies for resilience (McCloy et al., 2022). To address these issues, ecological resilience can be quantified at the community level through functional diversity indices. These offer a more complex ecological picture (Ricotta et al., 2014; Morelli et al., 2020) by accounting for the components of a species’ phenotype that influence ecosystem functioning, specifically functional traits (Petchey and Gaston, 2006). Therefore, functional diversity indices group members of a community by similarity in ecological functions (Blondel, 2003; Hooper et al., 2005). Since many animal species are either migratory or undertake local seasonal movements, annualized metrics of species and/or functional diversity may commonly be an appropriate choice.
We foresee four primary opportunities for future research to help address the ecological threat of urbanization within the context of climate change:
1.) The influence of land/water management strategies in densely populated urban areas (e.g., green and blue space connectivity, landscaping choices in small green/blue spaces, supplemental resources for birds including food and nest structures/substrates) on ecological resilience and biological poverty.
2.) Understanding the impact of socioecological factors on urban ecological resilience, with a specific focus on birds. Deeper investigations of the influence of social, technological, and economic factors on urban bird communities, and methods to mitigate these effects will provide insight for urban planning. The One Health framework provides a model of how this could be accomplished (Ottinger and Geiselman, 2023).
3.) Investigating urban ecological resilience through functional analyses, such as functional diversity, and multi-taxa studies will offer a more holistic picture of species presence and subsequently provide a comprehensive understanding of the challenges and opportunities for humans and wildlife in urban habitats.
4.) Collaboration with stakeholders at multiple levels of social-ecological-technological resilience, from landowners to government entities, to promote active management of urban landscapes for biodiversity. Engaging varied stakeholders can enhance the effectiveness of gathering data on urban wildlife productivity. This includes improving the creation and maintenance of quality habitat, implementation of monitoring technologies, fostering participation from underrepresented stakeholder groups, and growth of citizen and community science efforts (i.e, eBird, iNaturalist, or community groups dedicated to biodiversity monitoring). A more holistic understanding of urban wildlife ecology will enrich scientific knowledge and provide valuable insights for informed conservation practices and urban planning. Consequently, this will contribute to the development of more resilient and sustainable urban environments.
Urban ecological resilience is at the intersection of climate change, urbanization, technological development, social justice, and human decision-making. To address this, we advocate for interdisciplinary and multiscalar investigations through the lens of urban ecological resilience, the development of proactive urban planning and management strategies, and the promotion of equitable access to greenspaces and resilient urban environments. By comprehensively addressing the complex interplay between humans and avian communities in changing urban environments, we can work toward building more resilient and sustainable urban ecosystems for both humans and wildlife.
Author contributions
MM: Writing – review & editing, Writing – original draft, Visualization, Resources, Project administration, Conceptualization. RA: Writing – review & editing, Writing – original draft, Visualization, Conceptualization. TM: Writing – review & editing, Writing – original draft, Visualization, Conceptualization. JS: Writing – review & editing, Writing – original draft, Visualization, Conceptualization. JG: Writing – review & editing, Writing – original draft, Visualization, Conceptualization.
Funding
The author(s) declare that no financial support was received for the research, authorship, and/or publication of this article.
Acknowledgments
The authors would like to thank the reviewers for their constructive feedback and comments. Additionally, the authors would like to thank their respective academic departments for consistent support and guidance: the Department of Ecology and Conservation Biology at Texas A&M University, the Department of Integrative Biology at The University of Texas at San Antonio, and the School of Biological Sciences at Louisiana Tech University.
Conflict of interest
The authors declare that the research was conducted in the absence of any commercial or financial relationships that could be construed as a potential conflict of interest.
Publisher’s note
All claims expressed in this article are solely those of the authors and do not necessarily represent those of their affiliated organizations, or those of the publisher, the editors and the reviewers. Any product that may be evaluated in this article, or claim that may be made by its manufacturer, is not guaranteed or endorsed by the publisher.
Footnotes
- ^ URL: https://apps.who.int/iris/handle/10665/345751
- ^ URL: https://tx.audubon.org/urbanconservation/lights-out-texas
- ^ URL: https://abcbirds.org/program/cats-indoors/
- ^ URL: https://www.inaturalist.org
- ^ URL: https://www.audubon.org/conservation/science/christmas-bird-count/history-christmas-bird-count
- ^ URL: https://www.audubon.org/black-birders-week
- ^ URL: https://www.nps.gov/subjects/lwcf/outdoor-recreation-legacy-partnership-grants-program.htm
References
Ackerman J. T., Peterson S. H., Tsao D. C., Takekawa J. Y. (2018). California gull (Larus californicus) space use and timing of movements in relation to landfills and breeding colonies. Waterbirds 41, 384–400. doi: 10.1675/063.041.0402
Adelman J. S., Moyers S. C., Farine D. R., Hawley D. M. (2015). Feeder use predicts both acquisition and transmission of a contagious pathogen in a North American songbird. Proc. R. Soc B. 282, 20151429. doi: 10.1098/rspb.2015.1429
Akpinar A., Barbosa-Leiker C., Brooks K. R. (2016). Does green space matter? Exploring relationships between green space type and health indicators. Urban For. Urban Green. 20, 407–418. doi: 10.1016/j.ufug.2016.10.013
Alberti M., Marzluff J. M. (2004). Ecological resilience in urban ecosystems: Linking urban patterns to human and ecological functions. Urban Ecosyst. 7, 241–265. doi: 10.1023/B:UECO.0000044038.90173.c6
Al Fazari W., McGrady M. J. (2016). Counts of Egyptian vultures Neophron percnopterus and other avian scavengers at Muscat’s municipal landfill, Oman, November 2013–March 2015. Sandgrouse 38, 99–105.
Allen A. P., O’Connor R. J. (2000). Hierarchical correlates of bird assemblage structure on northeastern U.S.A. lakes. Environ. Monit. Assess. 62, 15–37. doi: 10.1023/A:1006244932033
American Bird Conservancy (2021) Cats indoors. Available online at: https://abcbirds.org/program/cats-indoors/ (Accessed February 1, 2024).
Anderson B. S., Phillips B. M., Voorhees J. P., Siegler K., Tjeerdema R. (2016). Bioswales reduce contaminants associated with toxicity in urban storm water. Environ. Toxicol. Chem. 35, 3124–3134. doi: 10.1002/etc.3472
Aram F., Higueras García E., Solgi E., Mansournia S., García E. H. (2019). Urban green space cooling effect in cities. Green Space Cooling Effect in Cities. Heliyon 5, 1339. doi: 10.1016/j.heliyon.2019
Arnold Z. J., Wenger S. J., Hall R. J. (2021). Not just trash birds: Quantifying avian diversity at landfills using community science data. PloS One 16, e0255391. doi: 10.1371/journal.pone.0255391
Aronson M. F. J., La Sorte F. A., Nilon C. H., Katti M., Goddard M. A., Lepczyk C. A., et al. (2014). A global analysis of the impacts of urbanization on bird and plant diversity reveals key anthropogenic drivers. Proc. R. Soc B 281, 1780. doi: 10.1098/rspb.2013.3330
Audubon Texas (2023) Lights out, texas!. Available online at: https://tx.audubon.org/urbanconservation/lights-out-texas (Accessed January 29, 2024).
Banks P. B., Bryant J. V. (2007). Four-legged friend or foe? Dog walking displaces native birds from natural areas. Biol. Lett. 3, 611–613. doi: 10.1098/rsbl.2007.0374
Barbosa K. V., Rodewald A. D., Ribeiro M. C., Jahn A. E. (2020). Noise level and water distance drive resident and migratory bird species richness within a Neotropical megacity. Landsc. Urban Plan. 197, 103769. doi: 10.1016/j.landurbplan.2020.103769
Beaugeard E., Brischoux F., Angelier F. (2021). Green infrastructures and ecological corridors shape avian biodiversity in a small French city. Urban Ecosyst. 24, 549–560. doi: 10.1007/s11252-020-01062-7
Belant J. L., Ickes S. K., Seamans T. W. (1998). Importance of landfills to urban-nesting herring and ring-billed gulls. Landsc. Urban Plan. 43, 11–19. doi: 10.1016/S0169-2046(98)00100-5
Bennett K. F. P., Evans B. S., Clark A., Marra P. P. (2021). Domestic cat abundance and activity across a residential land use gradient. Front. Ecol. Evol. 9. doi: 10.3389/fevo.2021.643845
Biggs C. R., Yeager L. A., Bolser D. G., Bonsell C., Dichiera A. M., Hou Z., et al. (2020). Does functional redundancy affect ecological stability and resilience? A review and meta-analysis. Ecosphere 11, e03184. doi: 10.1002/ecs2.3184
Bixler R. P., Lieberknecht K., Atshan S., Zutz C. P., Richter S. M., Belaire J. A. (2020). Reframing urban governance for resilience implementation: The role of network closure and other insights from a network approach. Cities 103, 102726. doi: 10.1016/j.cities.2020.102726
Blecha K. A., Boone R. B., Alldredge M. W. (2018). Hunger mediates apex predator’s risk avoidance response in wildlife-urban interface. J. Anim. Ecol. 87, 609–622. doi: 10.1111/1365-2656.12801
Blewett C. M., Marzluff J. M. (2005). Effects of urban sprawl on snags and the abundance and productivity of cavity-nesting birds. Condor 107, 678–693. doi: 10.1093/condor/107.3.678
Blondel J. (2003). Guilds or functional groups: does it matter? Oikos 100, 223–231. doi: 10.1034/j.1600-0706.2003.12152.x
Boal C. W. (2018). “Urban raptor communities: why some raptors and not others occupy urban environments,” in Urban raptors. Eds. Boal C. W., Dykstra C. R. (Island Press, Washington, DC), 36–50.
Bolund P., Hunhammar S. (1999). Ecosystem services in urban areas. Ecol. Econ. 29, 293–301. doi: 10.1016/S0921-8009(99)00013-0
Bowler D. E., Heldbjerg H., Fox A. D., O’Hara R. B., Böhning-Gaese K. (2018). Disentangling the effects of multiple environmental drivers on population changes within communities. J. Anim. Ecol. 87, 1034–1045. doi: 10.1111/1365-2656.12829
Bridge E. S., Pletschet S. M., Fagin T., Chilson P. B., Horton K. G., Broadfoot K. R., et al. (2016). Persistence and habitat associations of Purple Martin roosts quantified via weather surveillance radar. Landsc. Ecol. 31, 43–53. doi: 10.1007/s10980-015-0279-0
Brigham R. M. (1989). Roost and nest sites of common nighthawks: Are gravel roofs important? Condor 91, 722–724. doi: 10.2307/1368127
Brown C. R., Brown M. B., Pyle P., Patten M. A. (2020). “Cliff swallow (Petrochelidon pyrrhonota),” in Birds of the world. Ed. Rodewald P. G. (Cornell Lab of Ornithology, Ithaca, New York). doi: 10.2173/bow.cliswa.01
Brownstone D., Golob T. F. (2009). The impact of residential density on vehicle usage and energy consumption. J. Urban Econ. 65, 91–98. doi: 10.1016/j.jue.2008.09.002
Burger J., Gochfeld M. (1983). Behavior of nine avian species at a Florida garbage dump. Colonial Waterbirds 6, 54–63. doi: 10.2307/1520967
Burt C. S., Kelly J. F., Fox A. S., Jenkins-Smith H. C., Leon-Corwin M., Khalighifar A. (2023). Can ecological forecasting lead to convergence on sustainable lighting policies? Conserv. Sci. Prac. 5, e12920. doi: 10.1111/csp2.12920
Cai Z., La Sorte F. A., Chen Y., Wu J. (2023). The surface urban heat island effect decreases bird diversity in Chinese cities. Sci. Total Environ. 902, 166200. doi: 10.1016/j.scitotenv.2023.166200
Callaghan C., Major R. E., Lyons M. B., Martin J. M., Kingsford R. T. (2018). The effects of local and landscape habitat attributes on bird diversity in urban greenspaces. Ecosphere 9, e02347. doi: 10.1002/ecs2.2347
Campos-Silva L. A., Piratelli A. J. (2021). Vegetation structure drives taxonomic diversity and functional traits of birds in urban private native forest fragments. Urban Ecosyst. 24, 375–390. doi: 10.1007/s11252-020-01045-8
Carver E. (2009). Birding in the United States: A demographic and economic analysis: addendum to the 2006 national survey of fishing, hunting, and wildlife-associated recreation (Arlington, Virginia, USA: US Fish and Wildlife Service, Division of Economics).
Chace J. F., Walsh J. J. (2006). Urban effects on native avifauna: A review. Landsc. Urban Plan. 74, 46–69. doi: 10.1016/j.landurbplan.2004.08.007
Chamberlain D. E., Henry D. A. W., Reynolds C., Caprio E., Amar A. (2019). The relationship between wealth and biodiversity: A test of the luxury effect on bird species richness in the developing world. Glob. Change Biol. 25, 3045–3055. doi: 10.1111/gcb.14682
Chamberlain D., Reynolds C., Amar A., Henry D., Caprio E., Batáry P. (2020). Wealth, water and wildlife: Landscape aridity intensifies the urban luxury effect. Glob. Ecol. Biogeogr. 29, 1595–1605. doi: 10.1111/geb.13122
Chamberlain D. E., Vickery J. A., Glue D. E., Robinson R. A., Conway G. J., Woodburn R. J. W., et al. (2005). Annual and seasonal trends in the use of garden feeders by birds in winter. Ibis 147, 563–575. doi: 10.1111/j.1474-919x.2005.00430.x
Charter M., Izhaki I., Mocha Y. B., Kark S. (2016). Nest-site competition between invasive and native cavity nesting birds and its implication for conservation. J. Environ. Manage. 181, 129–134. doi: 10.1016/j.jenvman.2016.06.021
Ciach M., Fröhlich A. (2017). Habitat type, food resources, noise and light pollution explain the species composition, abundance and stability of a winter bird assemblage in an urban environment. Urban Ecosyst. 20, 547–559. doi: 10.1007/s11252-016-0613-6
Ciach M., Kruszyk R. (2010). Foraging of white storks Ciconia ciconia on rubbish dumps on non-breeding grounds. Waterbirds 33, 101–104. doi: 10.1675/063.033.0112
Clavero M., Villero D., Brotons L. (2011). Climate change or land use dynamics: Do we know what climate change indicators indicate? PloS One 6, e18581. doi: 10.1371/journal.pone.0018581
Clergeau P., Mennechez G., Sauvage A., Lemoine A. (2001). “Human perception and appreciation of birds: A motivation for wildlife conservation in urban environments of France,” in Avian ecology and conservation in an urbanizing world. Eds. Marzluff J. M., Bowman R., Donnelly R. (Springer, Boston, MA), 69–88.
Clergeau P., Quenot F. (2007). Roost selection flexibility of European starlings aids invasion of urban landscape. Landsc. Urban Plan. 80, 56–62. doi: 10.1016/j.landurbplan.2006.06.002
Clergeau P., Savard J.-P. L., Mennechez G., Falardeau G. (1998). Bird abundance and diversity along an urban-rural gradient: A comparative study between two cities on different continents. Ornithol. Appl. 100, 413–425. doi: 10.2307/1369707
Clout M. N., Elliot G. P., Robertson B. C. (2002). Effects of supplementary feeding on the offspring sex ratio of kakapo: a dilemma for the conservation of a polygynous parrot. Biol. Cons. 107, 13–18. doi: 10.1016/S0006-3207(01)00267-1
Coetzee A., Barnard P., Pauw A. (2021). Reliability and quality of artificial nectar feeders for birds in the Cape Floristic Region. Ostrich 92, 26–31. doi: 10.2989/00306525.2020.1836060
Collins M. K., Mangle S. B., Gallo T. (2021). Global trends in urban wildlife ecology and conservation. Biol. Conserv. 261, 109236. doi: 10.1016/j.biocon.2021.109236
Cooper C. B., Dickinson J., Phillips T., Bonney R. (2007). Citizen science as a tool for conservation in residential ecosystems. Ecol. Soc 12, 11. doi: 10.5751/ES-02197-120211
Cooper C. B., Hawn C. L., Larson L. R., Parrish J. K., Bowser G., Cavalier D., et al. (2021). Inclusion in citizen science: The conundrum of rebranding. Science 372, 1386–1388. doi: 10.1126/science.abi6487
Cooper C. B., Larson L., Dayer A., Stedman R., Decker D. (2015). Are wildlife recreationists conservationists? Linking hunting, birdwatching, and pro-environmental behavior. J. Wildl. Manage. 79, 446–457. doi: 10.1002/jwmg.855
Cordell K. (2013). Birding trends (Athens, Georgia, USA: Athens Research Group, Southern Research Station, USDA Forest Service).
Cosquer A., Raymond R., Prevot-Julliard A.-C. (2012). Observations of everyday biodiversity: a new perspective for conservation? Ecol. Soc. 17. doi: 10.5751/ES-04955-170402
Côté I. M., Darling E. S. (2010). Rethinking ecosystem resilience in the face of climate change. PloS Biol. 8, e1000438. doi: 10.1371/journal.pbio.1000438
Cox D. T. C., Gaston K. J. (2016). Urban bird feeding: connecting people with nature. PloS One 11, e0158717. doi: 10.1371/journal.pone.0158717
Cristiano W., Giacoma C., Carere M., Mancini L. (2021). Chemical pollution as a driver of biodiversity loss and potential deterioration of ecosystem services in Eastern Africa: A critical review. S. Afr. J. Sci. 117, 1–7. doi: 10.17159/sajs.2021/9541
Croci S., Butet A., Clergeau P. (2008). Does urbanization filter birds on the basis of their biological traits? Condor 110, 223–240. doi: 10.1525/cond.2008.8409
Crooks K. W., Soule M. E. (1999). Mesopredator release and avifaunal extinctions in a fragmented system. Nature 400, 563–566. doi: 10.1038/23028
Cupertino M. D. C., Guimarães B. T., Pimenta J. F. G., Almeida L. V. L. D., Santana L. N., Ribeiro T. A., et al. (2023). LIGHT POLLUTION: a systematic review about the impacts of artificial light on human health. Biol. Rhythm. Res. 54, 263–275. doi: 10.1080/09291016.2022.2151763
da Silva B. F., Pena J. C., Viana-Junior A. B., Vergne M., Pizo M. A. (2021). Noise and tree species richness modulate the bird community inhabiting small public urban green spaces of a Neotropical city. Urban Ecosyst. 24, 71–81. doi: 10.1007/s11252-020-01021-2
de Jong M., Jeninga L., Ouyang J. Q., van Oers K., Spoelstra K., Visser M. E. (2016). Dose-dependent responses of avian daily rhythms to artificial light at night. Physiol. Behav. 155, 172–179. doi: 10.1016/j.physbeh.2015.12.012
Dementieieva Y. Y., Chaplygina A. B., Kratenko R. I. (2023). Species composition of bird assemblages on waste landfills in Kharkov Region. Ornis Hungarica 31, 48–61. doi: 10.2478/orhu-2023-0003
Desjardins E., Barker G., Lindo Z., Dieleman C., Dussault A. C. (2015). Promoting resilience. Q. Rev. Biol. 90, 147–165. doi: 10.1086/681439
Dimoudi A., Kantzioura A., Zoras S., Pallas C., Kosmopoulos P. (2013). Investigation of urban microclimate parameters in an urban center. Energy Build. 64, 1–9. doi: 10.1016/j.enbuild.2013.04.014
Dodman D. (2009). Blaming cities for climate change? An analysis of urban greenhouse gas emissions inventories. Environ. Urban. 21, 185–201. doi: 10.1177/0956247809103016
Dominoni D., Quetting M., Partecke J. (2013). Artificial light at night advances avian reproductive physiology. Proc. R. Soc B. 280, 20123017. doi: 10.1098/rspb.2012.3017
Dorn N. J., Cook M. I., Herring G., Boyle R. A., Nelson J., Gawlik D. E. (2011). Aquatic prey switching and urban foraging by the white ibis Eudocimus albus are determined by wetland hydrological conditions. Ibis 153, 323–335. doi: 10.1111/j.1474-919X.2011.01101.x
Duhem C., Roche P., Vidal E., Tatoni T. (2008). Effects of anthropogenic food resources on yellow-legged gull colony size on Mediterranean islands. Popul. Ecol. 50, 91–100. doi: 10.1007/s10144-007-0059-z
du Plessis K. L., Martin R. O., Hockey P. A. R., Cunningham S. J., Ridley A. R. (2012). The costs of keeping cool in a warming world: Implications of high temperatures for foraging, thermoregulation and body condition of an arid-zone bird. Glob. Change Biol. 18, 3063–3070. doi: 10.1111/j.1365-2486.2012.02778.x
du Plessis M., Seymour C. L., Spottiswoode C. N., Coetzee A. (2021). Artificial nectar feeders reduce sunbird abundance and plant visitation in Cape Fynbos adjacent to suburban areas. Glob. Ecol. Conserv. 28, e01706. doi: 10.1016/j.gecco.2021.e01706
Dwyer J. F., Rosa J. P. D. (2015). Use of anthropogenic nest substrates by crested caracaras. Southeast. Nat. 14, N10–N15. doi: 10.1656/058.014.0105
Ellington E. H., Gehrt S. D. (2019). Behavioral responses by an apex predator to urbanization. Behav. Ecol. 30, 821–829. doi: 10.1093/beheco/arz019
Emlen J. T. (1974). An urban bird community in Tucson, Arizona: Derivation, structure, regulation. Condor 76, 184–197. doi: 10.2307/1366729
Eötvös C. B., Magura T., Lövei G. L. (2018). A meta-analysis indicates reduced predation pressure with increasing urbanization. Landsc. Urban Plan. 180, 54–59. doi: 10.1016/j.landurbplan.2018.08.010
Erastova D. A., Galbraith J. A., Cain K. E., van Heezik Y., Filion A., Stanley M. C. (2021). Sugar water feeding practices are associated with bird species composition in urban backyards. J. Urban Ecol. 7, 1–9. doi: 10.1093/jue/juab018
Estevo C. A., Nagy-Reis M. B., Silva W. R. (2017). Urban parks can maintain minimal resilience for Neotropical bird communities. Urban For. Urban Green. 27, 84–89. doi: 10.1016/j.ufug.2017.06.013
Evans K. L., Chamberlain D. E., Hatchwell B. J., Gregory R. D., Gaston K. J. (2011). What makes an urban bird? Glob. Change Biol. 17, 32–44. doi: 10.1111/j.1365-2486.2010.02247.x
Evans K. L., Newson S. E., Gaston K. J. (2009). Habitat influences on urban avian assemblages. Ibis 151, 19–39. doi: 10.1111/j.1474-919X.2008.00898.x
Fernández Cañero R., González Redondo P. (2010). Green roofs as a habitat for birds: a review. J. Anim. Vet. Adv. 9, 2041–2052. doi: 10.3923/javaa.2010.2041.2052
Fischer J., Lindenmayer D. B., Blomberg S. P., Montague-Drake R., Felton A., Stein J. A. (2007). Functional richness and relative resilience of bird communities in regions with different land use intensities. Ecosystems 10, 964–974. doi: 10.1007/s10021-007-9071-6
Fisk E. J. (1978). The growing use of roofs by nesting birds. Bird-Banding 49, 134–141. doi: 10.2307/4512343
Fontana S., Sattler T., Bontadina F., Moretti M. (2011). How to manage the urban green to improve bird diversity and community structure. Landsc. Urban Plan. 101, 278–285. doi: 10.1016/j.landurbplan.2011.02.033
Forrest A., St. Clair C. C. (2006). Effects of dog leash laws and habitat type on avian and small mammal communities in urban parks. Urban Ecosyst. 9, 51–66. doi: 10.1007/s11252-006-7903-3
Fuller R. A., Irvine K. N., Davies Z. G., Armsworth P., Gaston K. J. (2013). “Interactions between people and birds in urban landscapes,” in Urban bird ecology and conservation. Eds. Lepczyk C. A., Warren P. S. (University of California Press, Berkeley, CA), 249–266.
Galbraith J. A., Beggs J. R., Jones D. N., Stanley M. C. (2015). Supplementary feeding restructures urban bird communities. PNAS 112, E2648–E2687. doi: 10.1073/pnas.1501489112
Galbraith J. A., Stanley M. C., Jones D. N., Beggs J. R. (2017). Experimental feeding regime influences urban bird disease dynamics. J. Avian Biol. 48, 700–713. doi: 10.1111/jav.01076
Goddard M. A., Dougill A. J., Benton T. G. (2013). Why garden for wildlife? Social and ecological drivers, motivations and barriers for biodiversity management in residential landscapes. Ecol. Econ. 86, 258–273. doi: 10.1016/j.ecolecon.2012.07.016
Green D. M., Baker M. G. (2003). Urbanization impacts on habitat and bird communities in a Sonoran desert ecosystem. Landsc. Urban Plan. 63, 225–239. doi: 10.1016/S0169-2046(02)00195-0
Guetté A., Gaüzère P., Devictor V., Jiguet F., Godet L. (2017). Measuring the synanthropy of species and communities to monitor the effects of urbanization on biodiversity. Ecol. Indic. 79, 139–154. doi: 10.1016/j.ecolind.2017.04.018
Hanmer H. J., Thomas R. L., Fellowes M. D. E. (2017). Provision of supplementary food for wild birds may increase the risk of local nest predation. Ibis 159, 158–167. doi: 10.1111/ibi.12432
Hansen C. P., Parsons A. W., Keys R., Millspaugh J. J. (2020). Does use of backyard resources explain the abundance of urban wildlife? Front. Ecol. Evol. 8. doi: 10.3389/fevo.2020.570771
Harrison G. W. (1979). Stability under environmental stress: Resistance, resilience, persistence, and variability. Am. Nat. 113, 659–669. doi: 10.1086/283424
Harrison T. J. E., Smith J. A., Martin G. R., Chamberlain D. E., Bearhop S., Robb G. N., et al. (2010). ). Does food supplementation really enhance productivity of breeding birds? Oecologia 164, 311–320. doi: 10.1007/s00442-010-1645-x
Haskell D. G., Knupp A. M., Schneider M. C. (2001). “Nest predator abundance and urbanization,” in Avian ecology and conservation in an urbanizing world. Eds. Marzluff J. M., Bowman R., Donnelly R. (Springer, Boston, MA), 243–258. doi: 10.1007/978-1-4615-1531-9_11
Hoefer W., Gallagher F., Hyslop T., Wibbelt T., Ravit B. (2016). Environmental reviews and case studies: Unique landfill restoration designs increase opportunities to create urban open space. Environ. Pract. 18, 106–115. doi: 10.1017/S1466046616000090
Holling C. S. (1973). Resilience and stability of ecological systems. Ann. Rev. Ecol. Cyst. 4, 1–23. doi: 10.1146/annurev.es.04.110173.000245
Hooper D. U., Chapin F. S., Ewel J. J., Hector A., Inchausti P., Lavorel S., et al. (2005). Effects of biodiversity on ecosystem functioning: A consensus of current knowledge. Ecol. Monogr. 75, 3–35. doi: 10.1890/04-0922
Hope D., Gries C., Zhu W., Fagan W. F., Redman C. L., Grimm N. B., et al. (2003). Socioeconomic drive urban plant diversity. PNAS 100, 8788–8792. doi: 10.1073/pnas.1537557100
Horwitz P., Lindsay M., O’connor M. (2001). Biodiversity, endemism, sense of place, and public health: Inter-relationships for Australian inland aquatic systems. Ecosyst. Health 7, 253–265. doi: 10.1046/j.1526-0992.2001.01044.x
Ibáñez-Álamo J. D., Rubio E., Benedetti Y., Morelli F. (2017). Global loss of avian evolutionary uniqueness in urban areas. Glob. Change Biol. 23, 2990–2998. doi: 10.1111/gcb.13567
iNaturalist (2024). Available online at: https://www.inaturalist.org (Accessed January 31, 2024).
Injaian A. S., Uehling J. J., Taff C. C., Vitousek M. N. (2021). Effects of artificial light at night on avian provisioning, corticosterone, and reproductive success. Integr. Comp. Biol. 61, 1147–1159. doi: 10.1093/icb/icab055
Irizarry A. D., Collazo J. A., Vandermeer J., Perfecto I. (2021). Coffee plantations, hurricanes and avian resiliency: insights from occupancy, and local colonization and extinction rates in Puerto Rico. Glob. Ecol. Conserv. 27, e01579. doi: 10.1016/j.gecco.2021.e01579
Isaacs J. C. (2010). Louisiana bird watchers: A further examination of past research (Baton Rouge, Louisiana, USA: Socioeconomic Research and Development Section, Louisiana Department of Wildlife and Fisheries).
Isaksson C. (2018). “Impact of urbanization on birds,” in Bird Species: How they arise, modify and vanish. Ed. Tietze D. T. (Natural History Museum Basel, Basel, Switzerland), 235–257. doi: 10.1007/978-3-319-91689-7
Jagiello Z., Dylewski Ł., Tobolka M., Aguirre J. I. (2019). Life in a polluted world: A global review of anthropogenic materials in bird nests. Environ. pollut. 251, 717–722. doi: 10.1016/j.envpol.2019.05.028
Jagiello Z., Reynolds S. J., Nagy J., Mainwaring M. C., Ibáñez-Álamo J. D. (2023). Why do some bird species incorporate more anthropogenic materials into their nests than others? Philos. Trans. R. Soc. Lond. B. Biol. Sci. 378, 20220156. doi: 10.1098/rstb.2022.0156
Jasmani Z., Ravn H. P., van den Bosch C. C. K. (2017). The influence of small urban parks characteristics on bird diversity: A case study of Petaling Jaya, Malaysia. Urban Ecosyst. 20, 227–243. doi: 10.1007/s11252-016-0584-7
Jayalath T. A., Lloyd-Smith P., Becker M. (2023). Biodiversity benefits of birdwatching using citizen science data and individualized recreational demand models. Environ. Resource Econ. 86, 83–107. doi: 10.1007/s10640-023-00788-0
Johnson A. B., Winker K. (2010). Short-term hurricane impacts on a neotropical community of marked birds and implications for early- stage community resilience. PloS One 5, e15109. doi: 10.1371/journal.pone.0015109
Kang W., Minor E. S., Park C. R., Lee D. (2015). Effects of habitat structure, human disturbance, and habitat connectivity on urban forest bird communities. Urban Ecosyst. 18, 857–870. doi: 10.1007/s11252-014-0433-5
Karp D. S., Ziv G., Zook J., Ehrlich P. R., Daily G. C. (2011). Resilience and stability in bird guilds across tropical countryside. PNAS 108, 21134–21139. doi: 10.1073/pnas.1118276108
Kaushik M., Tiwari S., Manisha K. (2022). Habitat patch size and tree species richness shape the bird community in urban green spaces of rapidly urbanizing Himalayan foothill region of India. Urban Ecosyst. 25, 423–436. doi: 10.1007/s11252-021-01165-9
Kekkonen J. (2017). “Pollutants in urbanized areas: direct and indirect effects on bird populations,” in Ecology and conservation of birds in urban environments. Eds. Murgui E., Hedblom M. (Springer, Cham, Switzerland), 227–250.
Keleg M., Watson G. B., Salheen M. A. (2021). The path to resilience: Change, landscape aesthetics, and socio-cultural identity in rapidly urbanising contexts. Case Cairo Egypt. Urban For. Urban Green. 65, 127360. doi: 10.1016/j.ufug.2021.127360
Kinzig A. P., Warren P., Martin C., Hope D., Katti M. (2005). The effects of human socioeconomic status and cultural characteristics on urban patterns of biodiversity. Ecol. Soc. 10 (1), 23. doi: 10.5751/ES-01264-100123
Kondratyeza A., Knapp S., Durka W., Kühn I., Vallet J., Machon N., et al. (2020). Urbanization effects on biodiversity revealed by a two-scale analysis of species functional uniqueness vs. redundancy. Front. Ecol. Evol. 8. doi: 10.3389/fevo.2020.00073
Lamberson A. M. (2022). Urban wild bird feeding: Impacts on avian abundance and diversity in Texas. [Thesis]. San Antonio: University of Texas.
Lambert M. R., Donihue C. M. (2020). Urban biodiversity management using evolutionary tools. Nat. Ecol. Evol. 4, 903–910. doi: 10.1038/s41559-020-1193-7
Lao S., Robertson B. A., Anderson A. W., Blair R. B., Eckles J. W., Turner R. J., et al. (2020). The influence of artificial light at night and polarized light on bird-building collisions. Biol. Conserv. 241, 108358. doi: 10.1016/j.biocon.2019.108358
La Sorte F. A., Clark J. A. G., Lepczyk C. A., Arsonson M. F. J. (2023). Collections of small urban parks consistently support higher species richness but not higher phylogenetic or functional diversity. Proc. R. Soc B. 290, 0231424. doi: 10.1098/rspb.2023.1424
Leong M., Dunn R. R., Trautwein M. D. (2018). Biodiversity and socioeconomics in the city: A review of the luxury effect. Biol. Lett. 14, 20180082. doi: 10.1098/rsbl.2018.0082
Lepczyk C. A., Warren P. S. (2012). Urban bird ecology and conservation. (Berkeley, CA: University of California Press).
Lerman S. B., Narango D. L., Andrade R., Warren P. S., Grade A. M., Straley K. (2021). “Wildlife in the city: Human drivers and human consequences,” in Urban ecology: its nature and challenges. Ed. Barbosa P. (CABI, Wallingford, UK), 37–66.
Leveau L. M., Jokimäki J., Kaisanlahti-Jokimäki M., Villalobos F. (2021). Urbanization buffers seasonal change in composition of bird communities: A multi-continental meta-analysis. J. Biogeogr. 48, 2391–2401. doi: 10.1111/jbi.14236
Lim H. C., Sodhi N. S. (2004). Responses of avian guilds to urbanisation in a tropical city. Landsc. Urban Plan 66, 199–215. doi: 10.1016/S0169-2046(03)00111-7
López-García A., Gil-Tapetado D., Aguirre J. I. (2023). Drastic reduction of the population distribution of white storks predicted in absence of landfills. Ornithol. Appl. 125, 1–13. doi: 10.1093/ornithapp/duad021
Loss S. R., Li B. N., Horn L. C., Mesure M. R., Zhu L., Brys T. G., et al. (2023). Citizen science to address the global issue of bird–window collisions. Front. Ecol. Environ. 21, 418–427. doi: 10.1002/fee.2614
Loss S. R., Ruiz M. O., Brawn J. D. (2009). Relationships between avian diversity, neighborhood age, income, and environmental characteristics of an urban landscape. Biol. Conserv. 142, 2578–2585. doi: 10.1016/j.biocon.2009.06.004
Mainwaring M. C. (2015). The use of man-made structures as nesting sites by birds: A review of the costs and benefits. J. Nat. Conserv. 25, 17–22. doi: 10.1016/j.jnc.2015.02.007
Malekian M., Hadi M., Tarkesh M. (2021). Landscape features affecting bird diversity and abundance at an urban landfill site: A case study in Central Iran. Bird Study 68, 21–29. doi: 10.1080/00063657.2021.1960269
Malpass J. S., Rodewald A. D., Matthews S. N. (2017). Species-dependent effects of bird feeders on nest predators and nest survival of urban American robins and northern cardinals. Condor 119, 1–16. doi: 10.1650/CONDOR-16-72.1
Marasinghe S., Perera P., Dayawansa P. (2018). Putrescible waste landfills as bird habitats in urban cities: A case from an urban landfill in the Colombo District of Sri Lanka. J. Trop. For. Environ. 8, 2. doi: 10.31357/jtfe.v8i2.3761
Martínez-Abraín A., Jiménez J. (2016). Anthropogenic areas as incidental substitutes for original habitat. Conserv. Biol. 30, 593–598. doi: 10.1111/cobi.12644
McCloy M. W. D., Andringa R. K., Grace J. K. (2022). Resilience of avian communities to urbanization and climate change: An integrative review. Front. Conserv. Sci. 3. doi: 10.3389/fcosc.2022.918873
McKinley D. C., Miller-Rushing A. J., Ballard H. L., Bonney R., Brown H., Cook-Patton S. C., et al. (2017). Citizen science can improve conservation science, natural resource management, and environmental protection. Biol. Conserv. 208, 15–28. doi: 10.1016/j.biocon.2016.05.015
Melles S. J. (2005). Urban bird diversity as an indicator of human social diversity and economic inequality in Vancouver, British Columbia. Urban Habitats 3, 25–48.
Mitra A., Chatterjee C., Mandal F. (2011). Synthetic chemical pesticides and their effects on birds. Res. J. Environ. Toxicol. 5, 81–96. doi: 10.3923/rjet.2011.81.96
Moaraf S., Heiblum R., Okuliarová M., Hefetz A., Scharf I., Zeman M., et al. (2021). Evidence that artificial light at night induces structure-specific changes in brain plasticity in a diurnal bird. Biomol. 11, 1069. doi: 10.3390/biom11081069
Moaraf S., Vistoropsky Y., Pozner T., Heiblum R., Okuliarová M., Zeman M., et al. (2020). Artificial light at night affects brain plasticity and melatonin in birds. Neurosci. Lett. 716, 134639. doi: 10.1016/j.neulet.2019.134639
Morelli F., Benedetti Y., Ibáñez-Álamo J. D., Jokimäki J., Mänd R., Tryjanowski P., et al. (2016). Evidence of evolutionary homogenization of bird communities in urban environments across Europe. Glob. Ecol. Biogeogr. 25, 1284–1293. doi: 10.1111/geb.12486
Morelli F., Benedetti Y., Ibáñez-Álamo J. D., Tryjanowski P., Jokimaki J., Kaisanlahti-Jokimaki M.-L., et al. (2020). Insurance for the future? Potential avian community resilience in cities across Europe. Clim. Change 159, 195–214. doi: 10.1007/s10584-019-02583-7
Morelli F., Benedetti Y., Su T., Zhou B., Moravec D., Šímová P., et al. (2017). Taxonomic diversity, functional diversity and evolutionary uniqueness in bird communities of Beijing’s urban parks: Effects of land use and vegetation structure. Urban For. Urban Green. 23, 84–92. doi: 10.1016/j.ufug.2017.03.009
Morelli F., Reif J., Díaz M., Tryjanowski P., Ibáñez-Álamo J. D., Suhonen J., et al. (2021). Top ten birds indicators of high environmental quality in European cities. Ecol. Indic. 133, 108397. doi: 10.1016/j.ecolind.2021.108397
Morrison J. L., Dwyer J. F. (2021). “Crested caracara (Caracara plancus),” in Birds of the world. Ed. Poole A. F. (Cornell Lab of Ornithology, Ithaca, New York). doi: 10.2173/bow.y00678.01
Moyers S. C., Adelman J. S., Farine D. R., Thomason C. A., Hawley D. M. (2018). Feeder density enhances house finch disease transmission in experimental epidemics. Philos. Trans. R. Soc B. 373, 1745. doi: 10.1098/rstb.2017.0090
Murakawa S., Sekine T., Narita K.i., Nishina D. (1991). Study of the effects of a river on the thermal environment in an urban area. Energy Build. 16, 993–1001. doi: 10.1016/0378-7788(91)90094-J
Narango D. L., Tallamy D. W., Marra P. P. (2018). Nonnative plants reduce population growth of an insectivorous bird. PNAS 115, 11549–11554. doi: 10.1073/pnas.1809259115
National Audubon Society (2024a) Black birders week. Available online at: https://www.audubon.org/black-birders-week (Accessed February 2, 2024).
National Audubon Society (2024b) History of the christmas bird count. Available online at: https://www.audubon.org/conservation/science/christmas-bird-count/history-christmas-bird-count (Accessed February 2, 2024).
National Parks Service, US Department of the Interior (2024) Land and water conservation fund: outdoor recreation legacy partnership grants program. Available online at: https://www.nps.gov/subjects/lwcf/outdoor-recreation-legacy-partnership-grants-program.htm (Accessed February 2, 2024).
Oken B. S., Chamine I., Wakeland W. (2015). A systems approach to stress, stressors and resilience in humans. Behav. Brain Res. 282, 144–154. doi: 10.1016/j.bbr.2014.12.047
Oliver T. H., Gillings S., Pearce-Higgins J. W., Brereton T., Crick H. Q. P., Duffield S. J., et al. (2017). Large extents of intensive land use limit community reorganization during climate warming. Glob. Change Biol. 23, 2272–2283. doi: 10.1111/gcb.13587
Orros M. E., Fellowes M. D. E. (2015). Wild bird feeding in an urban area: Intensity, economics and numbers of individuals supported. Acta. Ornithol. 50, 43–58. doi: 10.3161/00016454AO2015.50.1.006
Orros M. E., Thomas R. L., Holloway G. J., Fellowes M. D. E. (2015). Supplementary feeding of wild birds indirectly affects ground beetle populations in suburban gardens. Urban Ecosyst. 18, 465–475. doi: 10.1007/s11252-014-0404-x
Ottinger M. A., Geiselman C. (2023). One health meets the exposome: human, wildlife, and ecosystem health. 1st ed (London, UK: Academic Press).
Pease B. S., Gilbert N. A., Casola W. R., Akamani K. (2023). The Steller’s sea-eagle in North America: An economic assessment of birdwatchers traveling to see a vagrant raptor. People Nat. 5, 1937–1947. doi: 10.1002/pan3.10527
Pena J. C. D. C., Martello F., Ribeiro M. C., Armitage R. A., Young R. J., Rodrigues M. (2017). Street trees reduce the negative effects of urbanization on birds. PloS One 12, e0174484. doi: 10.1371/journal.pone.0174484
Petchey O. L., Gaston K. J. (2006). Functional diversity: Back to basics and looking forward. Ecol. Lett. 9, 741–758. doi: 10.1111/j.1461-0248.2006.00924.x
Peterson M. N., Thurmond B., Mchale M., Rodriguez S., Bondell H. D., Cook M. (2012). Predicting native plant landscaping preferences in urban areas. Sustain. Cities Soc 5, 70–76. doi: 10.1016/j.scs.2012.05.007
Pimm S. L. (2009). Climate disruption and biodiversity. Curr. Biol. 19, R595–R601. doi: 10.1016/j.cub.2009.05.055
Plaza P. I., Lambertucci S. A. (2017). How are garbage dumps impacting vertebrate demography, health, and conservation. Glob. Ecol. Conserv. 12, 9–20. doi: 10.1016/j.gecco.2017.08.002
Plummer K. E., Bearhop S., Leech D. I., Chamberlain D. E., Bount J. D. (2013). Winter food provisioning reduces future breeding performance in a wild birds. Sci. Rep. 3, 2002. doi: 10.1038/srep02002
Plummer K. E., Risely K., Toms M. P., Siriwardena G. M. (2019). The composition of British bird communities is associated with long-term garden bird feeding. Nat. Commun. 10, 2088. doi: 10.1038/s41467-019-10111-5
Pollack L., Ondrasek N. R., Calisi R. (2017). Urban health and ecology: The promise of an avian biomonitoring tool. Curr. Zool. 63, 205–212. doi: 10.1093/cz/zox011
Reed J. H., Bonter D. N. (2018). Supplementing non-target taxa: Bird feeding alters the local distribution of mammals. Ecol. Appl. 28, 761–770. doi: 10.1002/eap.1683
Reynolds S. J., Galbraith J. A., Smith J. A., Jones D. N. (2017). Garden bird feeding: Insights and prospects from a north-south comparison of this global urban phenomenon. Front. Ecol. Evol. 5. doi: 10.3389/fevo.2017.00024
Reynolds S. J., Ibáñez-Álamo J. D., Sumasgutner P., Mainwaring M. C. (2019). Urbanisation and nest building in birds: A review of threats and opportunities. J. Ornithol. 160, 841–860. doi: 10.1007/s10336-019-01657-8
Rico-Silva J. F., Cruz-Trujillo E. J., Colorado G. J. (2021). Influence of environmental factors on bird diversity in greenspaces in an Amazonian city. Urban Ecosyst. 24, 365–374. doi: 10.1007/s11252-020-01042-x
Ricotta C., Bacaro G., Moretti M. (2014). A new measure of functional evenness and some of its properties. PloS One 9, e104060. doi: 10.1371/journal.pone.0104060
Rigolon A. (2016). A complex landscape of inequity in access to urban parks: A literature review. Landsc. Urban Plan. 153, 160–169. doi: 10.1016/j.landurbplan.2016.05.017
Roberts C. P., Allen C. R., Angeler D. G., Twidwell D. (2019). Shifting avian spatial regimes in a changing climate. Nat. Clim. Change 9, 562–566. doi: 10.1038/s41558-019-0517-6
Rodewald A. D., Gehrt S. D. (2014). “Wildlife population dynamics in urban landscapes,” in Urban wildlife conservation: theory and practice (Boston, Massachusetts, USA: Springer US), 117–147. doi: 10.1007/978-1-4899-7500-3_8
Roselli M. A., Noden B. H., Loss S. R. (2022). Tick infestation of birds across a gradient of urbanization intensity in the United States Great Plains. Urban Ecosyst. 25, 379–391. doi: 10.1007/s11252-021-01160-0
Rosencranz J. A., Thorne K. M., Buffington K. J., Takekawa J. Y., Hechinger R. F., Stewart T. E., et al. (2018). Sea-level rise, habitat loss, and potential extirpation of a salt marsh specialist bird in urbanized landscapes. Int. J. Bus. Innov. Res. 8, 8115–8125. doi: 10.1002/ece3.4196
Ruffino L., Salo P., Koivisto E., Banks P. B., Korpimaki E. (2014). Reproductive responses of birds to experimental food supplementation: A meta-analysis. Front. Zool. 11, 80. doi: 10.1186/s12983-014-0080-y
Saini C., Hutton P., Gao S., Simpson R. K., Giraudeau M., Sepp T., et al. (2019). Exposure to artificial light at night increases innate immune activity during development in a precocial bird. Comp. Biochem. Physiol. Part A Mol. 233, 84–88. doi: 10.1016/j.cbpa.2019.04.002
Salmón P., Stroh E., Herrera-Dueñas A., von Post M., Isaksson C. (2018). Oxidative stress in birds along a NOx and urbanisation gradient: An interspecific approach. Sci. Total Environ. 622–623, 635–643. doi: 10.1016/j.scitotenv.2017.11.354
Savard J. P. L., Clergeau P., Mennechez G. (2000). Biodiversity concepts and urban ecosystems. Landsc. Urban Plan. 48, 131–142. doi: 10.1016/S0169-2046(00)00037-2
Scheffer M., Carpenter S. R., Dakos V., Van Nes E. H. (2015). Generic indicators of ecological resilience: Inferring the chance of a critical transition. Ann. Rev. Ecol. Evol. Syst. 46, 145–167. doi: 10.1146/annurev-ecolsys-112414-054242
Schwoerer T., Dawson N. G. (2022). Small sight—Big might: Economic impact of bird tourism shows opportunities for rural communities and biodiversity conservation. PloS One 17, e0268594. doi: 10.1371/journal.pone.0268594
Seeland K., Dübendorfer S., Hansmann R. (2009). Making friends in Zurich’s urban forests and parks: The role of public green space for social inclusion of youths from different cultures. For. Policy Econ. 11, 10–17. doi: 10.1016/j.forpol.2008.07.005
Selwood K. E., Thomson J. R., Clarke R. H., Mcgeoch M. A., Mac Nally R. (2015). Resistance and resilience of terrestrial birds in drying climates: Do floodplains provide drought refugia. Glob. Ecol. Biogeogr. 24, 838–848. doi: 10.1111/geb.12305
Seress G., Hammer T., Bókony V., Vincze E., Preiszner B., Pipoly I., et al. (2018). Impact of urbanization on abundance and phenology of caterpillars and consequences for breeding in an insectivorous bird. Ecol. Appl. 28, 1143–1156. doi: 10.1002/eap.1730
Sharifi A. (2023). The resilience of urban social-ecological-technological systems (SETS): a review. Sustain. Cities Soc 99, 104910. doi: 10.1016/j.scs.2023.104910
Sharifi E., Lejmann S. (2015). Correlation analysis of surface temperature of rooftops, streetscapes and urban heat island effect: case study of central Sydney. J. Urban Environ. Eng. 9, 3–11. doi: 10.4090/juee.2015.v9n1.003011
Sheldon E. L., Griffith S. C. (2017). A high incidence of non-cavity nesting in an introduced population of house sparrows suggests that the species should not be constrained by cavity-nest site availability. Avian Res. 8, 29. doi: 10.1186/s40657-017-0087-0
Shutt J. D., Lees A. C. (2021). Killing with kindness: Does widespread generalised provisioning of wildlife help or hinder biodiversity conservation efforts? Biol. Conserv. 261, 109295. doi: 10.1016/j.biocon.2021.109295
Singha H., Rahmani A. R., Coulter M. C., Javed S. (2002). Nesting ecology of the greater adjutant stork in Assam, India. Waterbirds 25, 214–220. doi: 10.1675/1524-4695(2002)025[0214:NEOTGA]2.0.CO;2
Smith J. A., Dwyer J. F. (2024). Assessing range shifts and expansions of crested caracaras over 30 Years in Arizona, Texas, and Florida, USA. J. Raptor Res. doi: 10.3356/JRR-23-27. [Epub ahead of print].
Smith J. A., Dwyer J. F., Fraser J. D., Morrison J. L. (2017). A habitat model to aid the conservation of crested caracaras. J. Wildl. Manage. 81, 712–719. doi: 10.1002/jwmg.21239
Smith N., Georgiou M., King A. C., Tieges Z., Webb S., Chastin S. (2021). Urban blue spaces and human health: A systematic review and meta-analysis of quantitative studies. Cities 119, 103413. doi: 10.1016/j.cities.2021.103413
Soanes K., Lentini P. E. (2019). Why cities are the last chance for saving species. Front. Ecol. Environ. 17, 225–231. doi: 10.1002/fee.2032
Soccorsi A. E., LaPoint S. D. (2023). Assessing spatiotemporal patterns of mesocarnivores along an urban-to-rural gradient. J. Wildl. Manage. 87, e22474. doi: 10.1002/jwmg.22474
Sonne J., Kyvsgaard P., Maruyama P. K., Vizentin-Bugoni J., Ollerton J., Sazima M., et al. (2016). Spatial effects of artificial feeders on hummingbird abundance, floral visitation and pollen deposition. J. Ornithol. 157, 573–581. doi: 10.1007/s10336-015-1287-1
Suárez-Castro A. F., Maron M., Mitchell M. G. E., Rhodes J. R. (2022). Disentangling direct and indirect effects of landscape structure on urban bird richness and functional diversity. Ecol. Appli. 32, e2713. doi: 10.1002/eap.2713
Sullivan B. L., Wood C. L., Iliff M. J., Bonney R. E., Fink D., Kelling S. (2009). eBird: A citizen-based bird observation network in the biological sciences. Biol. Conserv. 142, 2282–2292. doi: 10.1016/j.biocon.2009.05.006
Suri J., Anderson P. M., Charles-Dominique T., Hellard E., Cumming G. S. (2017). More than just a corridor: A suburban river catchment enhances bird functional diversity. Landsc. Urban Plan. 157, 331–342. doi: 10.1016/j.landurbplan.2016.07.013
Thorne K. M., Takekawa J. Y., Elliott-Fisk D. L. (2012). Ecological effects of climate change on Salt Marsh wildlife: A case study from a highly urbanized estuary. J. Coast. Res. 28, 1477–1487. doi: 10.2112/JCOASTRES-D-11-00136.1
Tilman D., Downing J. A. (1994). Biodiversity and stability in grasslands. Nature 367, 363–365. doi: 10.1038/367363a0
Tomasevic J. A., Marzluff J. M. (2017). Cavity nesting birds along an urban-wildland gradient: Is human facilitation structuring the bird community? Urban Ecosyst. 20, 435–448. doi: 10.1007/s11252-016-0605-6
Travis J. M. J. (2003). Climate change and habitat destruction: A deadly anthropogenic cocktail. Proc. R. Soc B. 270, 467–473. doi: 10.1098/rspb.2002.2246
Turner W. R., Nakamura T., Dinetti M. (2004). Global urbanization and the separation of humans from nature. BioScience 54, 585–590. doi: 10.1641/0006-3568(2004)054[0585:GUATSO]2.0.CO;2
Turrin C., Watts B. D., Mojica E. K. (2015). Landfill use by bald eagles in the Chesapeake Bay region. J. Raptor Res. 49, 239–249. doi: 10.3356/JRR-14-50.1
United Nations, Department of Economic and Social Affairs, Population Division (2019). World urbanization prospects: the 2018 revision (ST/ESA/SER.A/420) (New York: United Nations).
US Fish and Wildlife Service. (2018). 2016 National survey of fishing, hunting, and wildlife-associated-recreation. (Washington, DC, USA: US Government Printing Office).
US Congress. (1976). Resource conservation and recovery Act. 90 Stat. 2795. (Washington, DC, USA: US Government Publishing Office).
US Fish and Wildlife Service (1999). Endangered and threatened wildlife and plants; final rule to remove the American peregrine falcon from the federal list of endangered and threatened wildlife, and to remove the similarity of appearance provision for free-flying peregrine in the coterminous United States; Final Rule. Fed Regist. 64, 46541–46558.
Vance C., Hedel R. (2007). The impact of urban form on automobile travel: Disentangling causation from correlation. Transportation 34, 575–588. doi: 10.1007/s11116-007-9128-6
Van Doran B. M., Willard D. E., Hennen M., Winger B. M. (2021). Drivers of fatal bird collisions in an urban center. PNAS 118, e2101666118. doi: 10.1073/pnas.2101666118
Washburn B. E., Swearingin R. M., Pullins C. K., Rice M. E. (2016). Composition and diversity of avian communities using a new urban habitat: Green Roofs. Environ. Manage. 57, 1230–1239. doi: 10.1007/s00267-016-0687-1
Widows S. A., Drake D. (2014). Evaluating the national wildlife federation’s certified wildlife habitat™ program. Landsc. Urban Plan. 129, 32–43. doi: 10.1016/j.landurbplan.2014.05.005
Wilcoxon T. E., Horn D. J., Hogan B. M., Hubble C. N., Huber S. J., Flamm J., et al. (2015). Effects of bird-feeding activities on the health of wild birds. Conserv. Physiol. 3, cov058. doi: 10.1093/conphys/cov058
World Health Organization (2016) Urban green spaces and health. World Health Organization. Regional Office for Europe. Available online at: https://apps.who.int/iris/handle/10665/345751 (Accessed January 16, 2024).
Keywords: bird, biodiversity, conservation, disturbance ecology, urban ecology, urban management
Citation: McCloy MWD, Andringa RK, Maness TJ, Smith JA and Grace JK (2024) Promoting urban ecological resilience through the lens of avian biodiversity. Front. Ecol. Evol. 12:1302002. doi: 10.3389/fevo.2024.1302002
Received: 25 September 2023; Accepted: 29 February 2024;
Published: 14 March 2024.
Edited by:
Tomás Pérez-Contreras, University of Granada, SpainReviewed by:
Ayyoob Sharifi, Hiroshima University, JapanElizabeth Mojica, EDM International, Inc., United States
Yixuan Sun, Shandong Women’s University, China
Copyright © 2024 McCloy, Andringa, Maness, Smith and Grace. This is an open-access article distributed under the terms of the Creative Commons Attribution License (CC BY). The use, distribution or reproduction in other forums is permitted, provided the original author(s) and the copyright owner(s) are credited and that the original publication in this journal is cited, in accordance with accepted academic practice. No use, distribution or reproduction is permitted which does not comply with these terms.
*Correspondence: Jennifer A. Smith, Jennifer.smith@utsa.edu