Non-structural carbohydrate dynamics of Pinus yunnanensis seedlings under drought stress and re-watering
- 1Key Laboratory of Forest Resources Conservation and Utilization in the Southwest Mountains of China Ministry of Education , Southwest Forestry University, Kunming, China
- 2College of Forestry, Southwest Forestry University, Kunming, Yunnan, China
- 3Yunnan Academy of Ecological and Environmental Sciences, Kunming, Yunnan, China
- 4College of Big Data and Intelligence Engineering, Southwest Forestry University, Kunming, China
Non-structural carbohydrates (NSC) are an important “buffer” for maintaining plant physiological functions under drought conditions; however, our understanding of the dynamics of NSC at the organ level during sustained drought of varying intensities and re-watering remains poor. In this study, two-year-old Pinus yunnanensis seedlings were subjected to drought and re-watering trials. Plants were subjected to three drought intensities (light drought, moderate drought, and severe drought) as well as control conditions (suitable moisture) for 51 days, including 30 days of drought followed by 21 days of re-watering for drought-treated seedlings, to study the dynamics of NSC in the leaves, stems, coarse roots, and fine roots. Changes in the distribution of NSC concentrations in the organ of P. yunnanensis seedlings under drought stress varied; in the early drought stages, the drought resistance of P. yunnanensis seedlings was enhanced by increasing soluble sugar concentrations; in later stages of drought, the stored starch in organs, stems, and coarse roots was consumed. Drought inhibited the growth of P. yunnanensis seedlings, but the maximum limit of drought tolerance was not reached under the different drought treatments after 30 days. P. yunnanensis seedlings in all treatment groups resumed growth after re-watering, and the growth of seedlings was actually promoted during re-watering in the moderate drought treatment group, indicating that drought induced the compensatory growth of seedlings. The growth of P. yunnanensis seedlings during re-watering was inhibited in the severe drought treatment group, and NSC continued to be regulated in seedlings in this group. Given that P. yunnanensis seedlings maintain growth through the consumption of coarse root starch in the late stages of drought, seedlings with a larger root-to-shoot ratio should be selected for cultivation in actual production. Based on our findings, exposure to moderate drought stress can enhance the drought tolerance of P. yunnanensis seedlings and promote the compensatory growth of seedlings.
1 Introduction
Water is one of the most common factors affecting the physiological state and growth and development of plants (Kaldenhoff and Fischer, 2006; Abit, 2008). The gradual increase in global temperature in recent years has accelerated the evaporation rates of surface moisture, reduced surface runoff, and decreased the soil water storage capacity, which has altered the intensity, frequency, and seasonality of precipitation in some regions and induced an increase in the frequency of severe and extreme drought events (Qin et al., 2007; Allen et al., 2010; Yu et al., 2014; Mishra, 2019; Li et al., 2021). The effects of drought alone are likely to be greater than those of all other environmental stresses and result in a severe reduction in global forest ecosystem services (Field and Raupach, 2004; Ma et al., 2012). An increase in drought events will also alter the positive feedback regulation of “carbon–climate feedbacks,” producing more severe droughts, leading to the massive loss of vegetation, the conversion of forest carbon sinks to carbon sources, and irreversible ecosystem degradation (Knapp and Smith, 2001; Zhao and Running, 2010; Ma et al., 2012; Ivits et al., 2014; He et al., 2020).
Carbohydrates are the main products of plant photosynthesis and can be further divided into structural carbohydrates (SC) and non-structural carbohydrates (NSC). Structural carbohydrates include lignin and cellulose, etc., and non-structural carbohydrates contain soluble sugars and starches (Hoch et al., 2002). NSC play key roles in plant metabolic activities during the growth and developmental stages of forest trees not only by mitigating the effects of external environmental stress on plants but also by facilitating the reconstruction, repair, and recovery of plant tissues after a disaster (Niinemets, 2010).
Soluble sugars are an important component of NSC and play a role in regulating osmotic pressure; starch is a storage form of NSC, and the conversion of sugars to starch is regulated by changes in environmental factors (Salleo et al., 2009). These changes are regulated by the relationship between carbon use and storage by plants; this is the reason why a balance between plant carbon fixation, assimilation, and consumption needs to be reached (Furze et al., 2019). When plants experience drought stress, cell expansion pressure decreases, and this causes a decline in plant growth before photosynthesis, preventing the consumption of photosynthetic products and resulting in a surplus of NSC stores (Ayub et al., 2011). However, as drought stress persists, plants consume stored NSC when the carbohydrates produced by photosynthesis do not meet the energy required for respiration (McDowell et al., 2011). When the stored NSC can no longer meet the energy requirements of cellular metabolism, “carbon starvation” occurs (McDowell et al., 2008), and this poses a risk to the survival of plants.
Under drought stress, plants increase water uptake and maintain a stable osmotic pressure by regulating the concentration and distribution of NSC. For example, drought stress causes a decrease in the concentrations of NSC in Picea abies roots but does not affect NSC concentrations in the stems and leaves (Hartmann et al., 2013a). After drought stress in Robinia pseudoacacia, NSC concentrations in the stems and coarse roots are significantly reduced, whereas NSC concentrations in the leaves and fine roots do not change significantly (Zhang et al., 2016). The coarse roots have carbon storage and regeneration functions, and stem soluble sugars can be transferred to the coarse roots. When the environment is suitable, the coarse roots can re-sprout using stored energy material. Given that the leaves are organs that provide a source of carbon, drought stress has a weaker effect on the concentration of NSC in the leaves than on the concentration of NSC in other plant organs (Sala et al., 2012).
In general, drought inhibits plant growth, which leads to dwarfing and eventually yield loss (Kamara et al., 2003; Dickin and Wright, 2008) there is also a lag in the growth of plants during water stress, but the same re-watering effect is observed during the physiological recovery of plants after re-watering (Schäffner, 1998). The re-watering effect refers to the phenomenon in which the small supplementation of a resource, which causes an increase in the total amount of resources used by the plant for which it is needed, ultimately leads to an increase in its resource use efficiency and yield (Melchior and Steudle, 1993). Therefore, the final yield of a plant under drought is not only related to its ability to resist and tolerate drought during drought stress but also to the ability of the normal growth of damaged plants to be restored after water conditions improve (Xu et al., 2010). The effects of drought stress and post-drought re-watering on plants are complex, and both stages can directly or indirectly induce a series of adaptive changes in growth metabolism, physiology, and biochemistry; these growth and physiological responses of plants reflect their ability to resist drought and recover after re-watering (Rosenthal et al., 1987; Irigoyen et al., 1992; Pinheiro et al., 2004; Yamada et al., 2005; Zhuang and Chen, 2006).
In a study by Zhang and Kirkham (1994), the regulatory mechanisms and compensatory growth following different levels of water stress and re-watering varied among plants. After re-watering following light water stress, the photosynthetic rate of Cerasus humilis recovered rapidly and was higher than that of the control; optimal values were attained for other photosynthetic physiological indicators (Chu et al., 2008). Gupta et al. (2012) found that all gas exchange parameters of Camellia sinensis were significantly higher after 15 days of re-watering treatment following drought stress, but still less than that in the control treatment. The recovery of plants from water stress depends on its duration and intensity, and the level of water stress should be within the tolerance range of the plant for it to have a growth-promoting effect after re-watering (Mackay and Barber, 1987). The effects of drought on the changes in NSC in trees vary depending on its intensity, and re-watering after different levels of drought may also affect the degree of re-opening of leaf stomata and photosynthesis in leaves, in addition to the recovery of phloem transport functions (He et al., 2020). When Cunninghamia lanceolata was subjected to drought stress, the total NSC concentration in the aboveground part significantly increased or was maintained, and drought reduced the total NSC concentration in the root system of seedlings; after re-watering, the concentration of NSC and its components decreased, and the NSC concentration decreased more significantly in the roots than in the aboveground parts (Yang et al., 2016). Therefore, carbon transport and distribution between different organs of plants under drought and rehydration conditions are interrelated, but the specific mechanisms of their impact are still unclear. This may provide new ideas for the study of the drought resistance mechanism of plants.
Pinus yunnanensis is a native tree species in southwest China, a pioneer tree species for the reforestation of barren mountains in southwest China, and a major forest-building species in Yunnan Province; it covers approximately 52% of the forest area in Yunnan Province (Sun et al., 2008). However, global climate change in recent years has led to an extended dry season in Yunnan, and the prolongation of the dry season has gradually constrained the growth and development of P. yunnanensis communities, especially at the seedling growth stage, where seedling mortality increases exponentially with decreasing rainfall because the seedling root system is still underdeveloped and sensitive to surface soil moisture (Poorter and Hayashida-Oliver, 2000; Marod et al., 2004). Several studies have examined drought stress and NSC, and these studies have examined the effects of drought stress on Robinia pseudoacacia (Yang et al., 2019), Pinus massoniana (Deng et al., 2019), Cunninghamia lanceolata (Yang et al., 2016), Pinus sylvestris, and Picea asperata (Ivanov et al., 2019). Since the 1980s, some scholars have conducted systematic research on P. yunnanensis from the aspects of morphology and growth changes, water physiology and metabolism physiological regulation, osmoregulation, and reactive oxygen species scavenging mechanism (Guo et al., 2016; Sun et al., 2016; Fan et al., 2018). In addition, P. yunnanensis seedlings can adapt to the drought environment by adjusting the interconversion and partitioning of soluble sugars and starch in the body, and at the same time by accumulating growth-inhibiting ABA and decreasing growth-promoting GA, IAA, and ZR, and then adjusting the IAA/ABA, GA/ABA, and ZR/ABA ratios of the needles (Liu et al., 2024). However, no studies have examined the response of the NSC of P. yunnanensis seedlings to rehydration after drought stress. Here, we examined the effects of drought and re-watering on the biomass and NSC of P. yunnanensis seedlings to enhance our understanding of the physiological mechanisms of drought resistance in P. yunnanensis seedlings.
2 Research content and methods
2.1 Experimental materials and research methods
The experimental site was located in the Arboretum of Southwest Forestry University (Kunming, Yunnan, 25°03′N, 102°46′E) in the subtropical plateau monsoon climate zone at an altitude of 1964 m. The climate is mild, with an average annual temperature of 16.5°C, an average annual precipitation of 1035 mm, and an average annual relative humidity of 67%; the soil is classified as a red loam. The temperature inside the shed ranges from 18.5°C to 37.0°C, and the relative humidity of the air ranges from 22.3% to 48.0%.
A potted plant was used to simulate natural early drought conditions. Two-year-old P. yunnanensis seedlings grown in Yiliang Garden Forestry were used in experiments, and the soil was a 3:2 mixture of local red loam and humus; its field water-holding capacity was determined to be 25.94% using the ring-knife method. Seedlings of uniform growth were selected from the available seedlings and transplanted for establishment; each pot had an equal weight of soil and a tray at the bottom. After transplanting, the seedlings were placed in a well-ventilated and well-lit experimental shed lined with ground cover and watered regularly.
When the transplanted seedlings became established and there were no significant differences in height or ground diameter, they were marked for hanging. Four moisture treatments were established: suitable moisture (CK), light drought stress (LD), moderate drought stress (MD), and severe drought stress (SD). The soil moisture content of each treatment was 80% ± 5%, 65% ± 5%, 50% ± 5%, and 35% ± 5% of the field water-holding capacity, and the actual moisture content was maintained at 19.45–22.05%, 15.56–18.16%, 11.67–17.27%, and 7.78–10.38% for the CK, LD, MD, and SD treatments, respectively (Wu et al., 2014; Pan et al., 2014). After initiating the treatments, plants stopped being watered to allow the soil moisture content to fall naturally to a predetermined range, and the soil was weighed and sampled to determine background values. The drought re-watering trial began on 14 March 2021 with 30 days of drought stress, followed by 21 days of re-watering. The actual soil water content was determined using a soil moisture meter and calculated by the weighing method, and all pots were weighed daily and watered according to the target weight.
24 seedlings from each treatment, samples were taken on days 0, 15, and 30 after the drought stress treatment, and on days 7, 14, and 21 of the re-watering treatment, with four plants per treatment and 16 plants at each sampling event. Plants were divided into the leaves, stems, coarse roots greater than 2 mm in diameter, and fine roots less than 2 mm in diameter; they were then placed into envelopes, labeled, and placed into ovens to kill and dry the plant materials. The biomass of each organ was weighed; the plant materials were then crushed, sieved, and stored for the subsequent determination of NSC (soluble sugars and starch). The concentration of soluble sugars and starch in each organ of Pinus sylvestris was determined by the improved phenol-concentrated sulphuric acid method (Buysse and Merckx, 1993; Wang and Huang, 2018), and the sum of the two concentrations was the NSC concentration.
Phenol-concentrated sulphuric acid method:
1) Preparation of Sucrose Standard Solution: Bake pure analytical sucrose in a constant temperature oven at 80 °C until constant amount, weigh 0.1 g of sample (accurate to 0.0001g), add a small amount of distilled water to dissolve and transfer to 100 ml volumetric flasks, and use distilled water to make the concentration of sucrose standard solution of 20, 40, 60, 80, 100 g-L-1, and measure the absorbance value at 485 nm by UV spectrophotometer to make a standard curve. The absorbance value was measured at 485 nm by UV spectrophotometer, and the standard curve was made.
2) Extraction of soluble sugar: Weigh 0.1g of the crushed dry sample, add 10ml of distilled water, extract at 100°C for 30min, cool down and centrifuge at 4000 r-min-1 for 10 min, then pour the centrifuged supernatant into a 100ml volumetric flask; add 10ml of distilled water into the residual precipitate and continue the above steps to obtain the supernatant; after the centrifugation, it can be used for the determination of soluble sugar concentration. The supernatant was then used for the determination of soluble sugar concentration.
3) Extraction of starch: Add 10 ml of distilled water to the above extracted residue, extract at 100°C for 15 min, add 2 ml of 9.2 mol/L perchloric acid while hot, shake well and leave to extract for 15 min, then centrifuge to obtain the supernatant and fix the volume, which can be used for the determination of starch content.
4) Determination of soluble sugar and starch concentration: Take 1 ml of the solution, transfer it to a centrifuge tube, add 1 ml of distilled water, 1 ml of 9% phenol solution, then immediately add 5 ml of concentrated sulphuric acid to the surface of the liquid, shake the centrifuge tube for 1 min, let it stand for 30 min, determine the absorbance value at 485 nm by UV-visible spectrophotometry, and then calculate the concentration of soluble sugar and starch according to the standard curve of sucrose.
2.2 Data processing
All statistical analyseswere performed with SPSS 19.0 (IBM). Values are expressed as mean ± standard deviation, with 4-6 replicates per treatment. The data were tested for normality and homogeneity of variance prior to analysis of variance. Two factor analysis of variance (ANOVA) was used to analyze the effects of drought intensity (CK, LD, MD and SD) and duration of drought (day 0, 15, 30), and time of rehydration treatments (day 7, 14, 21) on the NSC (sugar and starch) content of each tissue. Thedifferences in soluble sugars, starch, NSC content and biomass were examined by one-way ANOVA with different water treatment levels and different treatment times, and Duncan’s method was used for multiple comparisons, at a 0.05 level of significance. The graphs were plotted using Origin (2021 Edition, Origin Lab Company, US), Graphpad prism 8.0.
3 Results
3.1 Effect of drought and re-watering on the biomass of different organs of P. yunnanensis seedlings
During the experiment, the biomass of leaves and stems increased significantly, especially that of the leaves, under all treatments except for the severe drought treatment group (Table 1, P<0.05). At 30 days, the biomass of leaves was significantly smaller under the moderate and severe drought treatments than under the control and light drought treatments; the biomass of the coarse roots was significantly lower under the severe drought treatment than in the light and moderate drought treatments (Table 1, P<0.05). At 51 days, leaf biomass significantly decreased in the light, moderate, and severe drought treatments, and the magnitude of the decrease increased with the drought intensity (Table 1, P<0.05); the total biomass of P. yunnanensis seedlings was significantly lower under the severe drought treatment than under the light, moderate drought, and control treatments (Table 1, P<0.05).
Leaf biomass significantly increased with time on days 0, 30, and 51 under the moderate drought treatment; stem biomass was significantly higher on days 30 and 51 than on day 0 under moderate drought treatment (Table 1, P<0.05). The biomass of the fine roots was significantly higher on day 51 than on days 0 and 30 under moderate and severe drought treatments (Table 1, P<0.05). The biomass of the coarse roots was significantly higher on day 0 than on days 30 and 51 under severe drought treatment (Table 1, P<0.05).
The total biomass of P. yunnanensis seedlings was significantly greater on day 51 than on days 0 and 30 under the light drought treatment; under the moderate drought treatment, the total biomass increased significantly with time on days 0, 30, and 51 (Table 1, P<0.05); and under the severe drought treatment, there was no significant difference in total biomass on days 0, 30, and 51.
3.2 Changes in the NSC concentration in the leaves during drought and re-watering
Leaf soluble sugar concentrations increased and then decreased under light and moderate drought and continued to decrease under severe drought. Soluble sugar concentrations at 15 and 30 days were significantly higher in each drought treatment than in the control; soluble sugar concentrations were significantly higher at day 15 than at day 0 under the light and moderate drought treatments (Figure 1, P<0.05). The leaf starch concentration tended to decrease under each drought treatment during drought; the leaf starch concentration was significantly higher than that of the control under each drought treatment group at 30 days (Figure 1, P<0.05). The NSC concentration of the leaves increased and then decreased in each drought treatment. The leaf NSC concentration was significantly higher at 30 days than in the control under all drought treatment groups and increased significantly with the intensity of drought (Figure 1, P<0.05). The soluble sugar-to-starch ratio at 15 days was significantly higher in the moderate drought treatment than in the other treatment groups; the soluble sugar-to-starch ratio at 30 days was significantly higher in the severe drought treatment than in the light drought and control treatments.
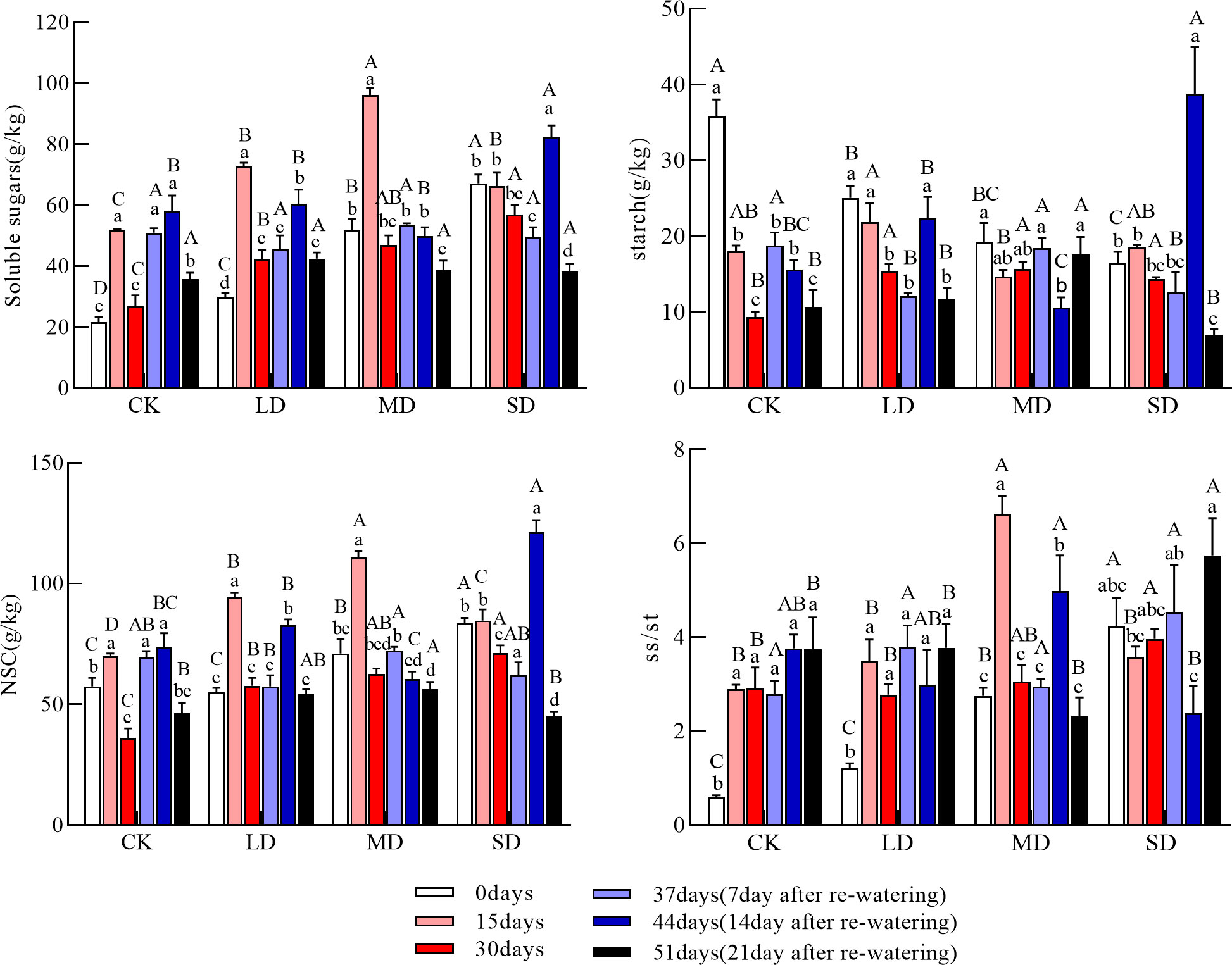
Figure 1 Changes in soluble sugars, starch, NSC (Nonstructural carbohydrates), and ss/st (soluble sugars/starch) in the leaves during drought and re-watering. Values in the graphs are mean ± standard deviation (n=4). Different capital letters indicate significant differences between the four treatments (including the control) on the same sampling day and different lowercase letters indicate significant differences between sampling days under the same treatment; the same applies to the lower graphs.
After re-watering, the soluble sugar concentration of the leaves increased and then decreased under light and severe drought and continued to decrease under moderate drought; at day 44, the soluble sugar concentration of the leaves was significantly higher under the light and severe drought treatments than at day 30. At day 51, there was no significant difference in the soluble sugar concentration between treatments. The starch concentration tended to decrease in all drought treatment groups after re-watering; the leaf starch concentration increased and then decreased under light and severe drought, and then decreased and increased under moderate drought. The leaf starch concentration on day 44 was significantly higher under the light and severe drought treatments than on day 30 and significantly higher in the severe drought treatment than in the other treatment groups; the leaf starch concentration on day 51 was significantly higher in the moderate drought treatment than in the other treatments (Figure 1, P<0.05). The NSC concentration in the leaves after re-watering increased and then decreased under the light and severe drought treatments but continued to decrease under the moderate drought treatment; the leaf NSC concentration at 51 days was significantly higher under the moderate drought treatment than under the severe drought and control treatments (Figure 1, P<0.05). At 51 days, the ratio of soluble sugars to starch in the leaves was significantly higher under the severe drought treatment than under the other treatments (Figure 1, P<0.05).
3.3 Changes in the NSC concentration in the stems during drought and re-watering
The soluble sugar concentration of stems increased and then decreased under each drought treatment group. The soluble sugar concentration of stems at 15 days was significantly higher under moderate and severe drought treatments than under control conditions and at day 0. The soluble sugar concentration was significantly higher in the severe drought treatment than in the light and moderate drought treatments; the soluble sugar concentration of the severe drought treatment at 30 days was significantly higher than that in the light drought and control treatments (Figure 2, P<0.05). The stem starch concentration during drought decreased and then increased under the light and moderate drought treatments; at 30 days, the concentration of starch in the stem was significantly higher than that in the control under each drought treatment (Figure 2, P<0.05). Stem NSC concentrations during drought increased and then decreased under moderate and severe drought treatments but continued to fall under light drought treatment; stem NSC concentrations at 30 days were significantly higher under all drought treatment groups than in the control (Figure 2, P<0.05). The ratio of soluble sugars to starch in stems during drought increased before decreasing in all treatments, and the ratio of soluble sugars to starch in stems at 30 days was significantly lower under the light drought treatment than in the control.
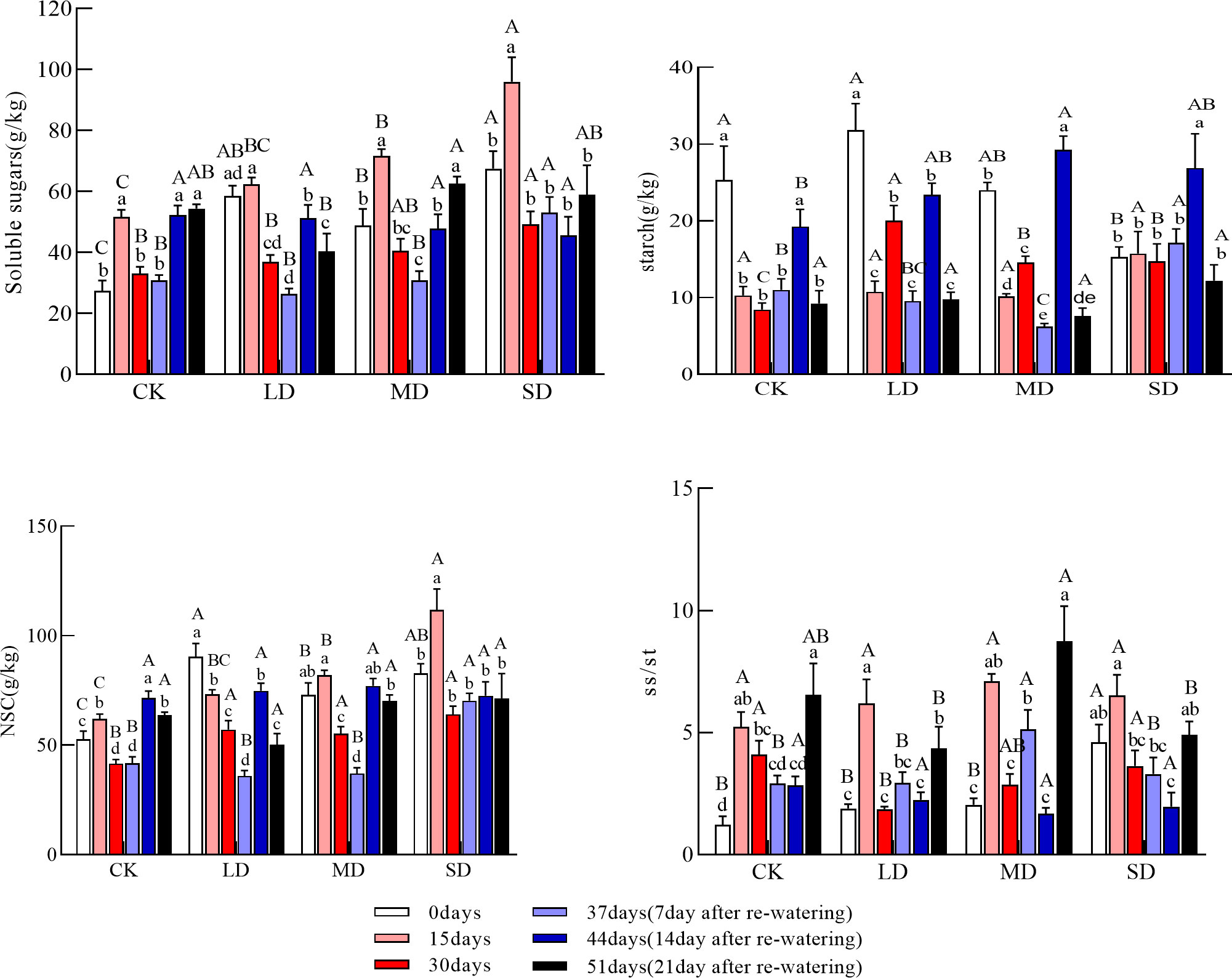
Figure 2 Changes in soluble sugars, starch, NSC (Nonstructural carbohydrates), and ss/st (soluble sugars/starch) in the stems during drought and re-watering. Values in the graph are mean ± standard deviation (n=4). Different capital letters indicate significant differences between the four treatments (including control) on the same sampling day, and different lowercase letters indicate significant differences between sampling days under the same treatment; the same applies to the lower panel.
The soluble sugar concentration of the stems all tended to increase after re-watering compared with the drought period, but the changes were variable; the soluble sugar concentration of the stems tended to increase under the moderate drought treatment but increased then decreased under the light drought treatment and decreased then increased under the severe drought treatment. Soluble sugar concentrations in the stems were significantly higher at day 44 than at day 30 under the light drought treatment (Figure 2, P<0.05); at day 51, there were no significant differences in soluble sugar concentrations in stems across treatments. Stem starch concentrations increased and then decreased in all treatments after re-watering. The stem starch concentration was significantly higher on day 4 under the moderate and severe drought treatments than on day 30, and the concentration of the starch in the stem was significantly higher under the moderate drought treatment than in the control (Figure 2, P<0.05). The stem starch concentration at day 51 did not significantly differ. The NSC concentration of stems increased and then decreased under each drought treatment group after re-watering, and the NSC concentration of stems at 51 days was not significantly different among the treatments. The ratio of soluble sugars to starch in stems decreased and then increased after re-watering, and the ratio of soluble sugars to starch in stems at 51 days was significantly higher under the moderate drought treatment than in the other drought treatments (Figure 2, P<0.05).
3.4 Changes in the NSC concentration in the coarse roots during drought and re-watering
The soluble sugar concentration of the coarse roots continued to decrease in each drought treatment. The soluble sugar concentration of the coarse roots was significantly lower at 15 days in the moderate and severe drought treatments than at day 0, and the soluble sugar concentration of the coarse roots was significantly higher in the severe drought treatment than in the light drought and control treatments; at 30 days, the soluble sugar concentration of the coarse roots was significantly higher in the severe drought treatment than in the light drought treatment (Figure 3, P<0.05). The starch concentration of coarse roots during drought increased and then decreased in each drought treatment group, and the starch concentration of the coarse roots at 30 days did not significantly differ among treatments. The NSC concentration of the coarse roots during drought continuously decreased in each drought treatment, and the NSC concentration of the coarse roots at 30 days was significantly higher under the severe drought treatment than in the other treatments (Figure 3, P<0.05). The ratio of soluble sugars to starch in the coarse roots during drought decreased and then increased in all treatments.
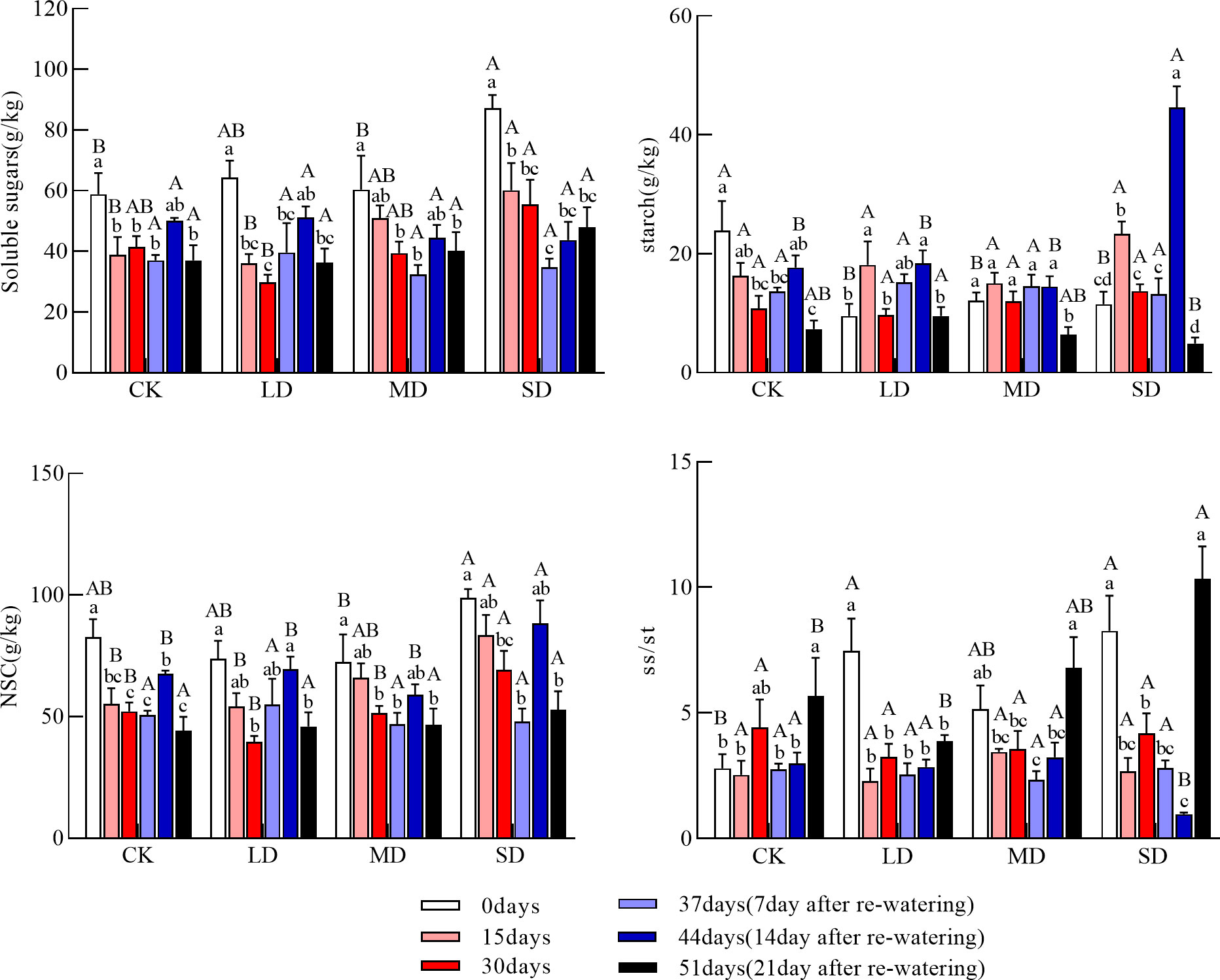
Figure 3 Changes in soluble sugars, starch, NSC (Nonstructural carbohydrates), and ss/st (soluble sugars/starch) in coarse roots during drought re-watering. Values in the graph are mean ± standard deviation (n=4). Different capital letters indicate significant differences between the four treatments (including the control) on the same sampling day, and different lowercase letters indicate significant differences between sampling days under the same treatment; the same applies to the lower panels.
The soluble sugar concentration of the coarse roots after re-watering increased and then decreased under the light and moderate drought treatments but continued to increase under severe drought; the soluble sugar concentration of the coarse roots was significantly higher at day 44 under the light drought treatment than at day 30 (Figure 3, P<0.05); at day 51, the soluble sugar concentration of the coarse roots did not significantly differ among treatments. After re-watering, the starch concentration of the coarse roots increased and then decreased under the light and severe drought treatments. At day 44, the starch concentration of the coarse roots was significantly higher under the light and severe drought treatments than at day 30; the starch concentration of the coarse roots was also significantly higher under the severe drought treatment than in the other treatments. At day 51, the starch concentration of the coarse roots did not significantly differ under the light, moderate drought, and control treatments; the starch concentration was significantly lower under the severe drought treatment than under the light drought treatment (Figure 3, P<0.05). The NSC concentration of the coarse roots after re-watering increased and then decreased under each drought treatment group, and the NSC concentration of the coarse roots at 51 days did not significantly differ among treatments. The ratio of soluble sugars to starch of the coarse roots after re-watering continued to increase under the light and moderate drought treatments, and the ratio of soluble sugars to starch of the coarse roots at 51 days was significantly higher under the severe drought treatment than under the light drought treatment (Figure 3, P<0.05).
3.5 Changes in the NSC concentration in the fine roots during drought and re-watering
The soluble sugar concentration of the fine roots was significantly higher in the light and moderate drought treatments at 15 days than at day 0; the soluble sugar concentration was also significantly higher in the light drought treatment than in the severe drought treatment. At 30 days, the soluble sugar concentration of the fine roots did not significantly differ among treatments. The starch concentration of the fine roots during drought increased and then decreased under the light and severe drought treatments. It continued to increase under the moderate drought treatment; at 30 days, the starch concentration of the fine roots was significantly higher under the moderate drought treatment than under the light, severe drought, and control treatments (Figure 4, P<0.05). The NSC concentration of the fine roots during drought increased and then decreased under the light and moderate drought treatments and continued to decrease under the severe drought treatment; there was no significant difference in the NSC concentration of the fine roots among treatments at 30 days. At 15 days, the ratio of soluble sugars to starch of the fine roots did not significantly differ among treatments; at 30 days, the ratio of soluble sugars to starch of the fine roots was significantly higher under the light and severe drought treatments than under the moderate drought treatment.
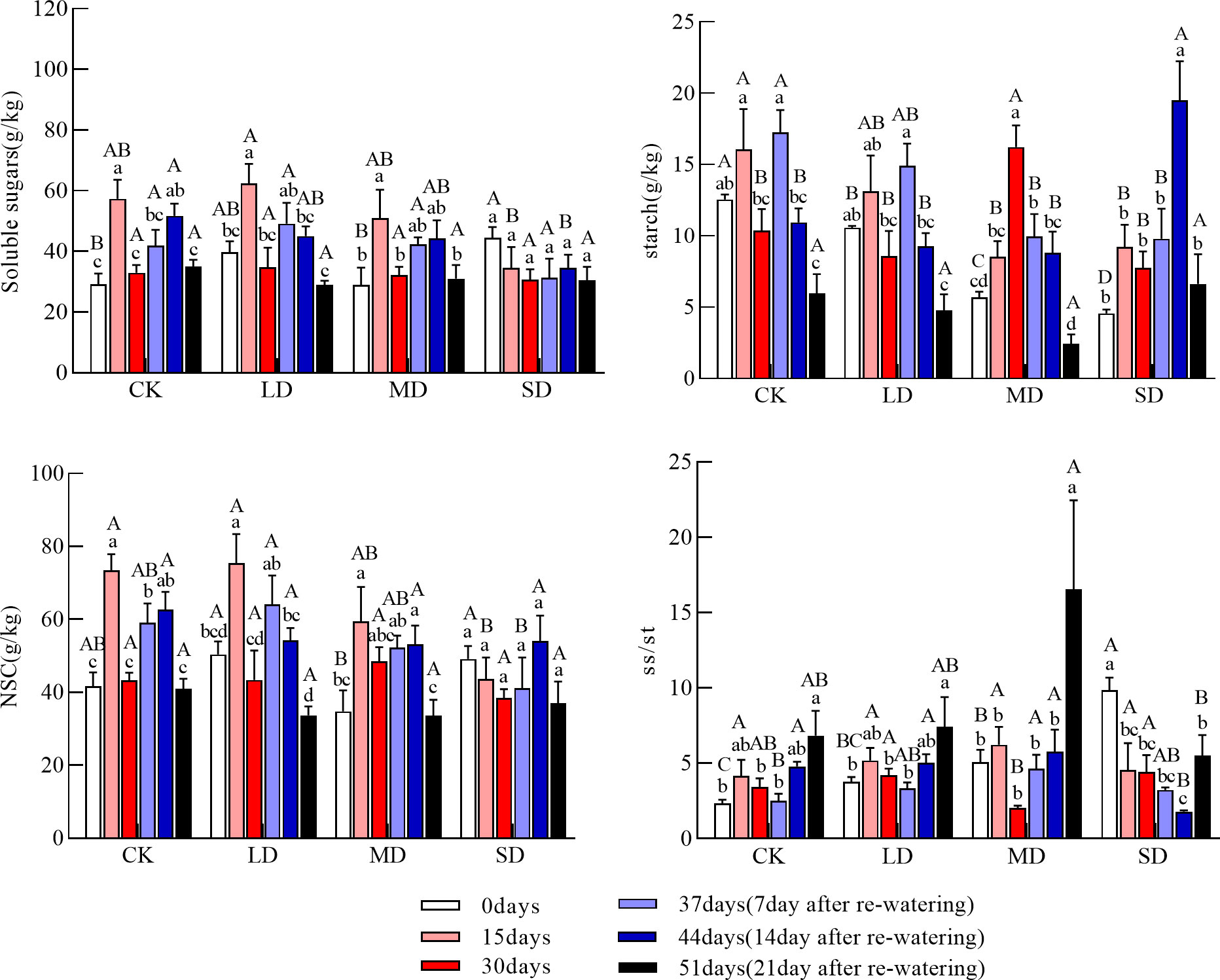
Figure 4 Changes in soluble sugars, starch, NSC (Nonstructural carbohydrates), and ss/st (soluble sugars/starch) in the fine roots during drought re-watering. Values in the graph are mean ± standard deviation (n=4). Different capital letters indicate significant differences between the four treatments (including control) on the same sampling day, and different lowercase letters indicate significant differences between sampling days under the same treatment; the same applies to the lower panels.
The soluble sugar concentration of the fine roots after re-watering increased and then decreased under the moderate and severe drought treatments, but a sustained decrease in the soluble sugar concentration was observed under the light drought treatment. There was no significant difference in the soluble sugar concentration of the fine roots among treatments at 51 days. After re-watering, the starch concentration of the fine roots continued to decrease under the light and moderate drought treatments; it then increased and decreased under the severe drought treatment. At 44 days, the starch concentration of the fine roots was significantly higher under the severe drought treatment than in the other treatment groups. At 51 days, the starch concentration of the fine roots was not significantly different among treatments. The NSC concentration of the fine roots after re-watering increased and then decreased under the moderate and severe drought treatments but continued to decrease under the light drought treatment; the NSC concentration of the fine roots at 51 days did not significantly differ among treatments. The ratio of soluble sugars to starch of the fine roots at 51 days was significantly higher under the moderate drought treatment than under the severe drought treatment (Figure 4, P<0.05).
3.6 NSC distribution patterns
Leaf NSC storage during drought increased continuously under the light drought treatment; it increased and then decreased under the moderate drought treatment, and it decreased and then increased under the severe drought treatment. Leaf NSC storage increased by 23%, 3%, and 11% under the light, moderate, and severe drought treatments, respectively, on day 30 compared with day 0. Stem NSC storage decreased continuously under the light drought treatment and increased continuously under the moderate and severe drought treatments, with a 15% decrease under the light drought treatment and a 7% and 9% increase under the moderate and severe drought treatments, respectively, on day 30 compared with day 0. The NSC storage of the coarse roots mostly decreased, with the exception of the moderate drought treatment, and the NSC storage of the coarse roots was 6%, 9%, and 17% lower on day 30 than on day 0 under the light, moderate, and severe drought treatments, respectively. The NSC storage of the fine roots first increased and then decreased during drought (Figure 5). NSC storage in the leaves decreased by 1% and 11% on day 51 compared with day 30 after re-watering in the light and severe drought treatments; it increased by 1% in the moderate drought treatment. The NSC storage of stems mostly decreased; the NSC storage of the fine and coarse roots mostly decreased after re-watering (Figure 5).
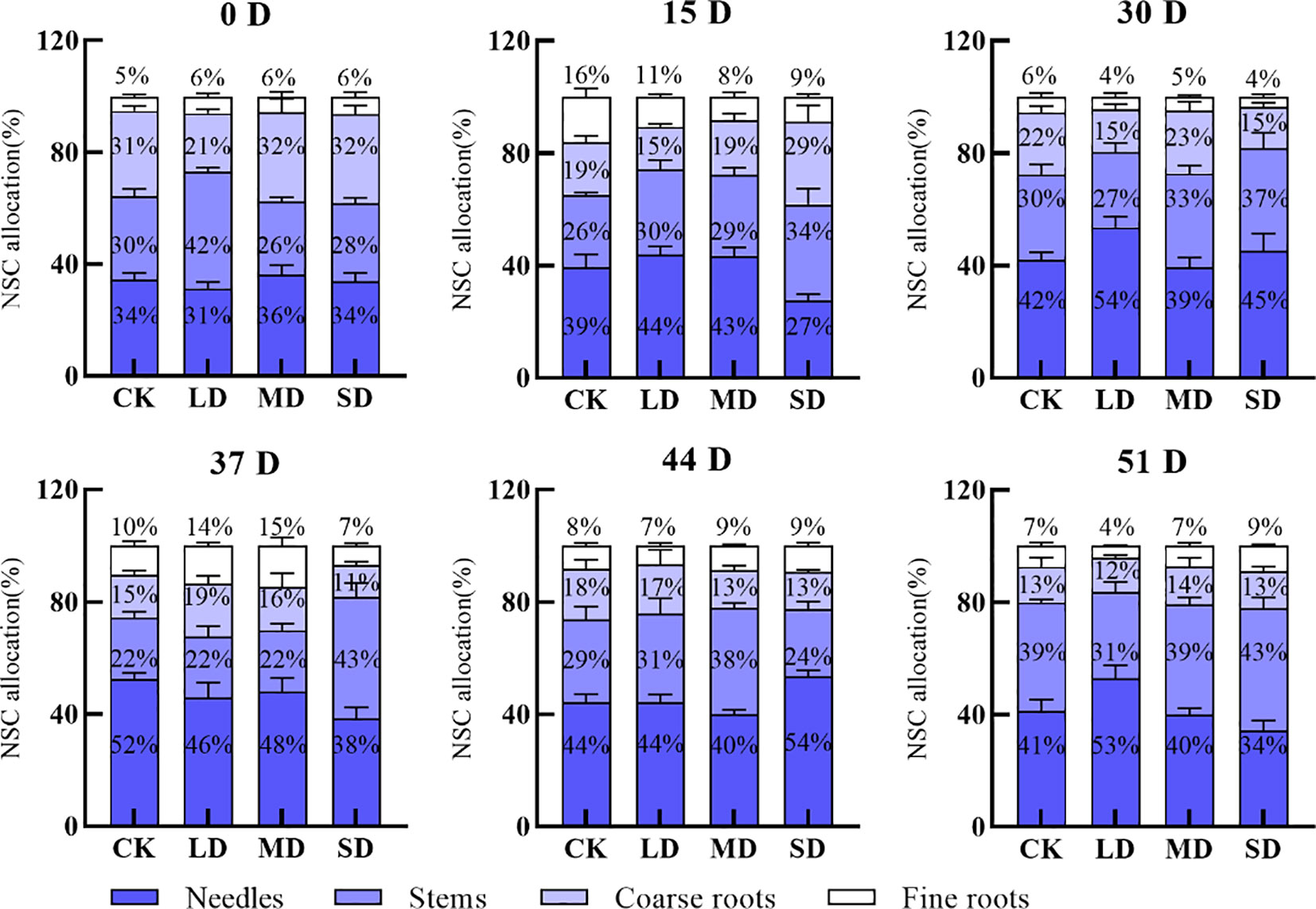
Figure 5 Effect of re-watering after continuous drought with different treatments on the distribution patterns of NSC (Nonstructural carbohydrates) in the leaves, stems, coarse roots, and fine roots of P. yunnanensis.
4 Discussion
4.1 Effect of drought on the biomass and NSC of P. yunnanensis seedlings
During the initial stages of drought, plants stop growing or show growth inhibition once they are stressed (Skirycz et al., 2010). The cessation or suppression of plant growth ultimately results in a reduction in biomass. Therefore, changes in plant biomass can reflect the extent of plant damage. Drought has been shown to significantly reduce root, stem, and leaf biomass as well as total biomass in Populus euphratica (Chen et al., 2014). In this experiment, leaf biomass decreased under water deficit in the moderate and severe drought treatments, and water deficit inhibited the biomass growth of leaves and coarse roots, especially under the severe drought treatment; P. yunnanensis stems had greater biomass under the moderate drought treatment than on day 0 and were not significantly affected by increases in the drought duration (Table 1).
When plants are subjected to drought stress, they often respond by regulating the concentration of NSC to increase the osmotic pressure to enhance water uptake and use. This process often results in an increase in the soluble sugar concentration and a decrease in the starch concentration, which disrupts the stable changes in both soluble sugars and starch (Dietze et al., 2014), induces carbon use and storage in plants, and positively regulates carbon metabolism, the carbon supply, and carbon demand (Furze et al., 2019). During the drought stress phase, NSC concentrations in the leaves and stems of P. yunnanensis seedlings mostly increased, and this was followed by a decrease; only NSC concentrations in the stems continuously decreased under light drought treatment. Soluble sugars in the leaves and stems increased and then decreased, with the exception of the sustained decline in soluble sugars in heavily drought-stricken leaves (Figures 1, 2). The severe water deficit may have caused a hysteresis response (Schäffner, 1998), which caused a brief lag in soluble sugar transport, followed by a slow recovery. The continuous respiratory demand of the root system during drought may make it a powerful carbon reservoir (Hartmann et al., 2013b), and plants may allocate more NSC from the source (i.e., leaves) to the roots (Lemoine et al., 2013). During the drought stress stage, NSC concentrations in the fine and coarse roots of P. yunnanensis mostly decreased, and NSC concentrations in the fine roots increased and then decreased under light and moderate drought treatments, and the NSC concentration of the coarse roots was significantly higher under the severe drought treatment than under the other treatments (Figures 4,5). Drought had little effect on the NSC concentration in the fine roots, and NSC accumulated in the coarse roots under severe drought conditions. Fine and coarse roots can be adjusted to maintain normal growth by regulating NSC as the length and intensity of drought treatment increase.
P. yunnanensis seedlings that had access to more water under the light drought treatment than in the medium or heavy drought treatments might be able to transmit signals, respond to drought, and transport soluble sugars within the plant more rapidly (Feng et al., 2019). At the beginning of drought, all organs generally resist drought or maintain normal growth by regulating the concentration of soluble sugars, and starch tends to be stored in the coarse roots; as the duration of drought increases, the concentration of starch in leaves and stems increases, and the concentration of starch in the coarse and fine roots decreases. Thus, starch might be converted to soluble sugars in the leaves and stems to enhance resistance to drought. P. yunnanensis seedlings did not reach the maximum limit of their drought tolerance after 30 days of different drought treatments.
4.2 Effect of post-drought re-watering on the biomass and NSC of P. yunnanensis seedlings
The biomass of the leaves, stems, and coarse roots of re-watered plants is restored after light drought treatment; under moderate drought treatment, the biomass of the leaves, stems, and coarse roots increases; and under severe drought treatment, the growth in the biomass of the coarse roots is inhibited (Melchior and Steudle, 1993; Zhang and Kirkham, 1994; Li et al., 2024). The leaves and stems of P. yunnanensis seedlings in this experiment were more sensitive to changes in water availability, and these changes were more pronounced after re-watering; the growth in the biomass of the fine roots was normal, and the growth increased under the moderate and severe drought treatments. The biomass of the leaves and stems increased significantly, especially that of the leaves, during re-watering after drought, with the exception of the severe drought treatment group (Table 1). Under the moderate drought treatment, the total biomass increased significantly over time on days 0, 30, and 51 (Table 1). Therefore, the compensatory growth of P. yunnanensis was promoted during re-watering after exposure to moderate drought stress (Melchior and Steudle, 1993).
After drought stress mitigation, seedlings were affected differently by different drought intensities. Under drought conditions, the failure of photosynthesis recovery in P. yunnanensis seedlings consumed a huge amount of NSC, especially in roots (Li et al., 2024). NSC concentrations in needles, stems, coarse roots, and fine roots of P. yunnanensis seedlings roughly showed a trend of increasing and then decreasing after re-watering, but a continuous decrease in NSC appeared in needles with moderate drought and fine roots with mild drought (Figures 1–4). Seedlings that have experienced drought show a strong tendency to maintain and rebuild starch stores in multiple organs during re-watering (Olesinski et al., 2011; Anderegg et al., 2015). Among them, under severe drought conditions, the starch content in needles and coarse roots at 21 days of re-watering was significantly lower than that in other treatment groups; under moderate drought conditions, the starch content in stems and fine roots at 21 days of re-watering was significantly lower than that in other treatment groups.
Using isotope labeling techniques, Galiano et al. (2017) found that plants under drought stress preferentially use most of the newly absorbed carbon for storage and osmotic protection after re-watering, and the newly absorbed carbon is mainly used for the growth of well-watered seedlings. However, the storage of NSC in several organs of P. yunnanensis seedlings was reduced in the post-drought re-watering treatments (Figure 5), and post-drought re-watering might have increased the consumption of NSC (Li et al., 2024); P. yunnanensis seedlings were in better condition after re-watering after they had been exposed to light and moderate drought stress than during drought stress. There was an increase in the NSC concentration in all organs under different drought treatments in the pre-rewatering period (7 and 14 days after re-watering), and the recovery of water may have contributed to seedling NSC production. No significant differences in NSC concentrations were observed between the different drought treatments and the control for all organs at the late re-watering stage (21 days of re-watering), indicating that the increase in NSC ceased and slowly stabilized as water increased during the re-watering phase (Figure 5). Soluble sugar and starch concentrations in all organs were not significantly different from those of the control during the late re-watering period, but starch concentrations in the coarse roots were significantly higher under the light treatment than under the severe drought treatment (Figures 1–4).
P. yunnanensis seedlings may remain in a water deficit state after re-watering following a severe drought, and it is uncertain whether the seedlings will be able to regain growth with increased re-watering time. Drought might also induce irreversible hydraulic damage that severely jeopardizes the transport of sugars between the aboveground organs and the root system, which can result in embolism (Hartmann et al., 2013a; Hartmann et al., 2013b; Yang et al., 2016). Therefore, severe drought may cause P. yunnanensis seedlings to stop growing or delay growth, as the root system can only rely on the available NSC in the plant to carry out its metabolic activities until the root carbon reserves are depleted (Hartmann et al., 2013a; Hartmann et al., 2013b; Yang et al., 2016). Drought damage to the plant phloem and the depletion of root carbon stocks can lead to the death of plants. In our experiment, the growth of P. yunnanensis seedlings that were re-watered after severe drought was inhibited, but no death was observed; the concentration of NSC in the plant body continually changed. During the re-watering phase after severe drought, the ratio of soluble sugars to starch was significantly higher in the severe drought treatment than in the other treatments; thus, leaves during the late stage of re-watering that had been exposed to severe drought treatment might have resumed photosynthesis, began to produce soluble sugars, and altered the concentrations of NSC to maintain normal metabolism and repair damaged tissues, which ensured the survival of P. yunnanensis seedlings.
5 Conclusion
The NSC concentration in the leaves and stems of P. yunnanensis seedlings under drought stress tended to increase and then decrease, and the NSC concentration in the fine and coarse roots tended to decrease. With the prolongation of drought, P. yunnanensis seedlings gradually stopped regulating the concentration of soluble sugars and used starch in the coarse roots to cope with the drought stress. Drought also inhibited the biomass growth of P. yunnanensis seedlings, especially under severe drought conditions. P. yunnanensis seedlings did not die after exposure to different intensities of drought stress for 30 days, which indicates that P. yunnanensis seedlings are drought tolerant. The NSC concentrations in the leaves, stems, coarse roots, and fine roots of P. yunnanensis seedlings tended to increase and then decrease after re-watering. The biomass of the leaves and stems increased significantly after re-watering, especially in the moderate drought treatment group, which indicates that drought induced compensatory growth in P. yunnanensis seedlings. In the severe drought treatment group, the growth of P. yunnanensis seedlings was inhibited after re-watering, but seedlings were not killed. Because photosynthesis was not monitored in this experiment, the changes in soluble sugars and starch could only be used to speculate on how changes in NSC mediate adaptation to drought and re-watering in P. yunnanensis seedlings; if photosynthesis could be monitored, the whole process of carbon production to utilization in P. yunnanensis seedlings could be characterized from the source of carbon. Based on the characteristics of the P. yunnanensis seedlings that maintained their growth through the consumption of thick root starch in the late stages of drought, seedlings with relatively large root-to-shoot ratios should be used for cultivation in actual production; based on the effects of the re-watering phase in this experiment, exposure to an appropriate drought treatment can promote the adaptation of P. yunnanensis seedlings to drought and promote their compensatory growth.
Data availability statement
The raw data supporting the conclusions of this article will be made available by the authors, without undue reservation.
Author contributions
JX: Writing – original draft. ZZ: Writing – original draft. XD: Data curation, Writing – original draft. HH: Investigation, Writing – original draft. YL: Methodology, Writing – review & editing. JS: Methodology, Writing – original draft. XF: Conceptualization, Writing – original draft. JW: Conceptualization, Funding acquisition, Investigation, Methodology, Project administration, Writing – review & editing.
Funding
The author(s) declare financial support was received for the research, authorship, and/or publication of this article. This study was co-supported by the National Natural Science Foundation of China (31960306) and Open Fund for Key Laboratory for Forest Resources Conservation and Utilization in the Southwest Mountain of China (KLESWFU–201902).
Conflict of interest
The authors declare that the research was conducted in the absence of any commercial or financial relationships that could be construed as a potential conflict of interest.
Publisher’s note
All claims expressed in this article are solely those of the authors and do not necessarily represent those of their affiliated organizations, or those of the publisher, the editors and the reviewers. Any product that may be evaluated in this article, or claim that may be made by its manufacturer, is not guaranteed or endorsed by the publisher.
References
Abit P. P. (2008). Ecological and physiological basis for the distribution of woody plants along water availability gradients in the southeastern United States mixed forest. North Carolina State University ProQuest Dissertations Publishing, 3329208.
Allen C. D., Macalady A. K., Chenchouni H., Bachelet D., McDowell N., Vennetier M., et al. (2010). A global overview of drought and heat-induced tree mortality reveals emerging climate change risks for forests. For. Ecol. Manag. 259, 660–684. doi: 10.1016/j.foreco.2009.09.001
Anderegg W. R., Schwalm C., Biondi F., Camarero J. J., Koch G., Litvak M., et al. (2015). Pervasive drought legacies in forest ecosystems and their implications for carbon cycle models. Science 349, 528–532. doi: 10.1126/science.aab1833
Ayub G., Smith R. A., Tissue D. T., Atkin O. K. (2011). Impacts of drought on leaf respiration in darkness and light in Eucalyptus saligna exposed to industrial-age atmospheric CO2 and growth temperature. New Phytol. 190, 1003–1018. doi: 10.1111/j.1469-8137.2011.03673
Buysse J., Merckx R. (1993). An improved colorimetric method to quantify sugar content of plant tissue [J]. J. Exp. Bot. 44, 1627–1629. doi: 10.1093/jxb/44.10.1627
Chen J., Duan B., Wang M., Korpelainen H., Li C. (2014). Intra-and inter-sexual competition of Populus cathayana under different watering regimes. Funct. Ecol. 28, 124–136. doi: 10.1111/1365-2435.12180
Chu J. M., Meng P., Zhang J. S., Gao J. (2008). Effects of soil water stress on the photosynthesis chlorophyll fluorescence param eters of Cerasus humilis seedlings. Forestry. Sci. Res. 21, 295–300. doi: 10.13275/j.cnki.lykxyj
Deng X., Xiao W., Shi Z., Zeng L., Lei L. (2019). Combined effects of drought and shading on growth and non-structural carbohydrates in Pinus massoniana Lamb. Seedlings. Forests 11, 18. doi: 10.3390/f11010018
Dickin E., Wright D. (2008). The effects of winter water logging and summer drought on the growth and yield of winter wheat (Triticum aestivum L.). Eur. J. Agron. 28, 234–244. doi: 10.1016/j.eja.2007.07.010
Dietze M. C., Sala A., Carbone M. S., Czimczik C. I., Mantooth J. A., Richardson A. D., et al. (2014). Nonstructural carbon in woody plants. Annu. Rev. Plant Biol. 65, 667–687. doi: 10.1146/annurev-arplant-050213-040054
Fan Y. K., Lan Q. Y., Hou L. L., Lan Z. Q. (2018). Research progress of seed germination characteristics and seedling drought resistance of Pinus yunnanensis Franch. Seed 37, 47–51. doi: 10.16590/j.cnki.1001-4705.2018.02.047
Feng X., Xu Y., Peng L., Yu X., Zhao Q., Feng S., et al. (2019). TaEXPB7-B, a β-expansin gene involved in low-temperature stress and abscisic acid responses, promotes growth and cold resistance in Arabidopsis thaliana. J. Plant Physiol. 240, 153004. doi: 10.1016/j.jplph.2019.153004
C. B. Field, M. R. Raupach (Eds.) (2004). The global carbon cycle: integrating humans, climate, and the natural world. 62, (Island Press).
Furze M. E., Huggett B. A., Aubrecht D. M., Stolz C. D., Carbone M. S., Richardson A. D. (2019). Whole-tree nonstructural carbohydrate storage and seasonal dynamics in five temperate species. New Phytol. 221, 1466–1477. doi: 10.1111/nph.15462
Galiano L., Timofeeva G., Saurer M., Siegwolf R., Martínez-Vilalta J., Hommel R., et al. (2017). The fate of recently fixed carbon after drought release: towards unravelling C storage regulation in Tilia platyphyllos and Pinus sylvestris. Plant Cell Environ. 40, 1711–1724. doi: 10.1111/pce.12972
Guo L., Wu Y. B., Li L. F., Sun A., Wang W. J., Su N., et al. (2016). Effects of watering control on physiological and biochemical traits of Pinus yunnanensis seedlings. J. Northwest. Forestry. Univ. 31, 78–84. doi: 10.3969/j.issn.1001-7461.2016.05.13
Gupta S., Bharalee R., Bhorali P., Bandyopadhyay T., Gohain B., Agarwal N., et al. (2012). Identification of drought tolerant progenies in tea by gene expression analysis. Funct. Integr. Genomic. 12, 543–563. doi: 10.1007/s10142-012-0277-0
Hartmann H., Ziegler W., Kolle O., Trumbore S. (2013a). Thirst beats hunger-declining hydration during drought prevents carbon starvation in Norway spruce saplings. New Phytol. 200, 340–349. doi: 10.1111/nph.12331
Hartmann H., Ziegler W., Trumbore S. (2013b). Lethal drought leads to reduction in nonstructural carbohydrates in Norway spruce tree roots but not in the canopy. Funct. Ecol. 27, 413–427. doi: 10.1111/1365-2435.12046
He W., Liu H., Qi Y., Liu F., Zhu X. (2020). Patterns in nonstructural carbohydrate contents at the tree organ level in response to drought duration. Global Change Biol. 26, 3627–3638. doi: 10.1111/gcb.15078
Hoch G., Popp M., Körner C. (2002). Altitudinal increase of mobile carbon pools in Pinus cembra suggests sink limitation of growth at the Swiss treeline. Oikos 98, 361–374. doi: 10.1034/j.1600-0706.2002.980301.x
Irigoyen J. J., Einerich D. W., Sánchez-Díaz M. (1992). Water stress induced changes in concentrations of proline and total soluble sugars in nodulated alfalfa (Medicago sativa) plants. Physiol. Plant. 84, 55–60. doi: 10.1111/j.1399-3054.1992.tb08764.x
Ivanov Y. V., Kartashov A. V., Zlobin I. E., Sarvin B., Stavrianidi A. N., Kuznetsov V. V. (2019). Water deficit-dependent changes in non-structural carbohydrate profiles, growth and mortality of pine and spruce seedlings in hydroculture. Environ. Exp. Bot. 157, 151–160. doi: 10.1016/j.envexpbot.2018.10.016
Ivits E., Horion S., Fensholt R., Cherlet M. (2014). Drought footprint on E uropean ecosystems between 1999 and 2010 assessed by remotely sensed vegetation phenology and productivity. Global Change Biol. 20, 581–593. doi: 10.1111/gcb.12393
Kaldenhoff R., Fischer M. (2006). Aquaporins in plants. Acta Physiol. 187, 169–176. doi: 10.1152/physrev.00008.2015
Kamara A. Y., Menkir A., Badu-Apraku B., Ibikunle O. (2003). The influence of drought stress on growth, yield and yield components of selected maize genotypes. J. Agr. Sci-Cambridge. 141, 43–50. doi: 10.1017/S0021859603003423
Knapp A. K., Smith M. D. (2001). Variation among biomes in temporal dynamics of aboveground primary production. Science 291, 481–484. doi: 10.1126/science.291.5503.481
Lemoine R., Camera S. L., Atanassova R., Dédaldéchamp F., Allario T., Pourtau N., et al. (2013). Source-to-sink transport of sugar and regulation by environmental factors. Front. Plant Sci. 4. doi: 10.3389/fpls.2013.00272
Li T. T., Liu Y. J., Ye J. L., Wang S. W., Yin L. N., Deng X. P., et al. (2024). Response and mechanism of sourc-sink-flow caused by the compensation effect of croprehydration after drought. J. Soi. Land. Water Conserv. 38 (2), 1–13. doi: 10.13870/j.cnki.stbcxb.2024.02.002
Li Z., Zhou P., Shi X., Li Y. (2021). Forest effects on runoff under climate change in the Upper Dongjiang River Basin: Insights from annual to intra-annual scales. Environ. Res. Lett. 16, 014032. doi: 10.1088/1748-9326/abd066
Liu Y. X., Wang L. N., Wu J. W., Li S. M., Zhao Z. J., Sun J. L., et al. (2024). Response of non-structural carbohydrates of Pinus yunnanensis seedlings to drought stress and the hormonal regulation mechanism. J. Northwest. A&F. Univ. 01), 1–11. doi: 10.13207/j.cnki.jnwafu.2024.01.007
Ma Z., Peng C., Zhu Q., Chen H., Yu G., Li W., et al. (2012). Regional drought-induced reduction in the biomass carbon sink of Canada’s boreal forests. P. Natl. Acad. Sci. U.S.A. 109, 2423–2427. doi: 10.1073/pnas.1111576109
Mackay A. D., Barber S. A. (1987). Effect of cyclic wetting and drying of a soil on root hair growth of maize roots. Plant Soil 104, 291–293. doi: 10.1007/BF02372544
Marod D., Kutintara U., Tanaka H., Nakashizuka T. (2004). Effects of drought and fire on seedling survival and growth under contrasting light conditions in a seasonal tropical forest. J. Veg. Sci. 15, 691–700. doi: 10.1111/j.1654-1103.2004.tb02311.x
McDowell N. G., Beerling D. J., Breshears D. D., Fisher R. A., Raffa K. F., Stitt M. (2011). The interdependence of mechanisms underlying climate-driven vegetation mortality. Trends Ecol. Evol. 26, 523–532. doi: 10.1016/j.tree.2011.06.003
McDowell N., Pockman W. T., Allen C. D., Breshears D. D., Cobb N., Kolb T., et al. (2008). Mechanisms of plant survival and mortality during drought: why do some plants survive while others succumb to drought? New Phytol. 178, 719–739. doi: 10.1111/j.1469-8137.2008.02436.x
Melchior W., Steudle E. (1993). Water transport in onion (Allium cepa L.) roots (changes of axial and radial hydraulic conductivities during root development). Plant Physiol. 101, 1305–1315. doi: 10.1104/pp.101.4.1305
Mishra A. K. (2019). Quantifying the impact of global warming on precipitation patterns in India. Meteorol. Appl. 26, 153–160. doi: 10.1002/met.1749
Niinemets Ü. (2010). Responses of forest trees to single and multiple environmental stresses from seedlings to mature plants: past stress history, stress interactions, tolerance and acclimation. For. Ecol. Manag. 260, 1623–1639. doi: 10.1016/j.foreco.2010.07.054
Olesinski J., Lavigne M. B., Krasowski M. J. (2011). Effects of soil moisture manipulations on fine root dynamics in a mature balsam fir (Abies balsamea L. Mill.) forest. Tree Physiol. 31, 339–348. doi: 10.1093/treephys/tpr006
Pan X., Qiu Q., Li J. Y., Wang J. H., He Q., Su Y., et al. (2014). Physiological indexes of six plant species from the tibetan plateau under drought stress. Acta Ecol. Sin. 34, 3558–3567. doi: 10.5846/stxb201306031288
Pinheiro C., Passarinho J. A., Ricardo C. P. (2004). Effect of drought and rewatering on the metabolism of Lupinus albus organs. J. Plant Physiol. 161, 1203–1210. doi: 10.1016/j.jplph.2004.01.016
Poorter L., Hayashida-Oliver Y. (2000). Effects of seasonal drought on gap and understorey seedlings in a Bolivian moist forest. J. Trop. Ecol. 16, 481–498. doi: 10.1017/S026646740000153X
Qin D., Chen Z., Averyt K. B., Miller H. L., Solomon S., Manning M. (eds.). (2007). Climate Change 2007: The Physical Science Basis. Contribution of Working Group I to the Fourth Assessment Report of the Intergovernmental Panel on Climate Change. Cambridge University Press.
Rosenthal W. D., Arkin G. F., Shouse P. E., Jordan W. R. (1987). Water deficit effects on transpiration and leaf growth1. Agron. J. 79, 1019–1026. doi: 10.2134/agronj1987.00021962007900060014x
Sala A., Woodruff D. R., Meinzer F. C. (2012). Carbon dynamics in trees: feast or famine? Tree Physiol. 32, 764–775. doi: 10.1093/treephys/tpr143
Salleo S., Trifilò P., Esposito S., Nardini A., Gullo M. A. L. (2009). Starch-to-sugar conversion in wood parenchyma of field-growing Laurus nobilis plants: a component of the signal pathway for embolism repair? Funct. Plant Biol. 36, 815–825. doi: 10.1071/FP09103
Schäffner A. R. (1998). Aquaporin function, structure, and expression: are there more surprises to surface in water relations? Planta 204, 131–139. doi: 10.1007/s004250050239
Skirycz A., De Bodt S., Obata T., De Clercq I., Claeys H., De Rycke R., et al. (2010). Developmental stage specificity and the role of mitochondrial metabolism in the response of Arabidopsis leaves to prolonged mild osmotic stress. Plant Physiol. 152, 226–244. doi: 10.1104/pp.109.148965
Sun Q., Cai N. H., Chen S., Wang D. W., Duan A. A., Xu Y. L. (2016). Drought stress on physiological characteristics of Pinus yunnanensis seedlings. J. SouthwestForestryUniversity. 36, 18–22. doi: 10.11929/j.issn.2095-1914,2016.03.004
Sun H. L., Li S. C., Xiong W. L., Yang Z. R., Cui B. S. (2008). Influence of slope on root system anchorage of Pinus yunnanensis. Ecol. Eng. 32, 60–67. doi: 10.1016/j.ecoleng.2007.09.002
Wang X. G., Huang J. L. (2018). Principles and techniques of plant physiology and biochemistry experiments. 3rd ed (Beijing: Higher Education Press).
Wu J. W., Bai J. J., He Q., Li J Y., Qiu Q., Pan ,. X. (2014). The relationship of leaf water potential and soil water content of five kinds of seedings in south China. J. Fujian. Forestry. Sci. Tech. 41, 16–21. doi: 10.13428/j.cnki.fjlk.2014.03.004
Xu Z., Zhou G., Shimizu H. (2010). Plant responses to drought and rewatering. Plant Signal Behav. 5, 649–654. doi: 10.4161/psb.5.6.11398
Yamada M., Morishita H., Urano K., Shiozaki N., Yamaguchi-Shinozaki K., Shinozaki K., et al. (2005). Effects of free proline accumulation in petunias under drought stress. J. Exp. Bot. 56, 1975–1981. doi: 10.1093/jxb/eri195
Yang B., Peng C., Zhu Q., Zhou X., Liu W., Duan M., et al. (2019). The effects of persistent drought and waterlogging on the dynamics of nonstructural carbohydrates of Robinia pseudoacacia L. seedlings in Northwest China. For. Ecosyst. 6, 1–17. doi: 10.1186/s40663-019-0181-3
Yang Q., Zhang W., Li R., Xu M., Wang S. (2016). Different responses of non-structural carbohydrates in above-ground tissues/organs and root to extreme drought and re-watering in Chinese fir (Cunninghamia lanceolata) saplings. Trees 30, 1863–1871. doi: 10.1007/s00468-016-1419-0
Yu M., Li Q., Hayes M. J., Svoboda M. D., Heim R. R. (2014). Are droughts becoming more frequent or severe in China based on the standardized precipitation evapotranspiration index: 1951–2010? Int. J. Climatol. 34, 545–558. doi: 10.1002/joc.3701
Zhang T., Cao Y., Chen Y. M., Liu G. B. (2016). Effects of drought stress on non-structural carbohydrates of Robinia pseudoacacia saplings at the end of growing season. J. Soil Water Conserv. 30, 297–304. doi: 10.13870/j.cnki.stbcxb.2016.05.049
Zhang J., Kirkham M. B. (1994). Drought-stress-induced changes in activities of superoxide dismutase, catalase, and peroxidase in wheat species. Plant Cell Physiol. 35, 785–791. doi: 10.1093/oxfordjournals.pcp.a078658
Zhao M., Running S. W. (2010). Drought-induced reduction in global terrestrial net primary production from 2000 through 2009. Science 329, 940–943. doi: 10.1126/science.1192666
Keywords: drought and re-watering, organ, non-structural carbohydrates, Pinus yunnanensis, seedlings
Citation: Xiao J, Zhao Z, Deng X, Hu H, Liu Y, Sun J, Fu X and Wu J (2024) Non-structural carbohydrate dynamics of Pinus yunnanensis seedlings under drought stress and re-watering. Front. Ecol. Evol. 12:1343258. doi: 10.3389/fevo.2024.1343258
Received: 23 November 2023; Accepted: 28 February 2024;
Published: 19 March 2024.
Edited by:
Johan Gielis, University of Antwerp, BelgiumReviewed by:
Shuaifeng Li, Chinese Academy of Forestry, ChinaZhiyi Cui, Chinese Academy of Forestry, China
Quan Qiu, South China Agricultural University, China
Copyright © 2024 Xiao, Zhao, Deng, Hu, Liu, Sun, Fu and Wu. This is an open-access article distributed under the terms of the Creative Commons Attribution License (CC BY). The use, distribution or reproduction in other forums is permitted, provided the original author(s) and the copyright owner(s) are credited and that the original publication in this journal is cited, in accordance with accepted academic practice. No use, distribution or reproduction is permitted which does not comply with these terms.
*Correspondence: Xiaoyong Fu, 369755817@qq.com; Junwen Wu, wujunwen@swfu.com
†These authors have contributed equally to this work