Effects of short-term simulated acid rain and nitrogen deposition on soil nutrients and enzyme activities in Cunninghamia lanceolata plantation
- 1Co-Innovation Center for Sustainable Forestry in Southern China, Jiangsu Province Key Laboratory of Soil and Water Conservation and Ecological Restoration, College of Forestry and Grassland, College of Soil and Water Conservation, Nanjing Forestry University, Nanjing, Jiangsu, China
- 2Institute of Plant Ecology, Justus-Liebig University Giessen, Giessen, Germany
- 3Sheyang Management Station, Jiangsu Yancheng National Nature Reserve, Yancheng, Jiangsu, China
Acid rain and nitrogen deposition are emerging as global scale environmental issues due to increasing industrial emissions and agricultural pollutants, which seriously impac t the sustainable development of global ecosystems. However, the specific effects both acid rain and nitrogen deposition interactions on forest soil ecosystems, particularly as relates to the soil nutrient content and enzyme activities, remain unclear. Therefore, we established a simulated sample plot of acid rain (SR, NR) and nitrogen deposition (N) and their interactions (SRN, NRN) in a subtropical Cunninghamia lanceolata (C. lanceolata) plantation in the Yangtze River Delta region of China to investigate the impacts of these factors via correlation analysis and structural equation model (SEM). The results showed that acid rain had a stronger effect on soil pH than nitrogen deposition in C. lanceolata plantation, while the simultaneous addition of acid rain and nitrogen deposition exacerbated soil acidification. Soil available potassium, ammonium nitrogen and nitrate nitrogen in C. lanceolata plantation responded more obviously to acid rain and nitrogen deposition, in which acid rain, nitrogen deposition and their interactions significantly reduced soil available potassium content, while acid rain and nitrogen deposition interactions significantly increased soil ammonium nitrogen and nitrate nitrogen content. Nitric acid rain, nitrogen deposition and their interactions significantly increased soil NAGase activity, but significantly decreased soil urease activity; the single-factor treatment of acid rain and nitrogen deposition significantly increased soil arylsulfatase activity, while the interaction of acid rain and nitrogen deposition significantly decreased soil arylsulfatase activity; in general, the interaction of acid rain and nitrogen deposition had a stronger effect on the soil ecosystem of the C. lanceolata plantation than that of single acid rain or nitrogen deposition, of which nitrogen deposition exacerbated the effects of acid rain on the soil ecosystem of C. lanceolata plantation mainly by changing the soil pH and the content of effective nutrients.
1 Introduction
Acid rain is a global scale issue that has seriously impeded the development of the ecological environment (Grennfelt et al., 2020). Next to North America and Europe, China is the third largest concentrated distribution area of acid deposition in the world, with >40% of the affected land area (Xu et al., 2015a; Yin et al., 2021). Sulfur dioxide (SO2) and nitrogen oxides (NOX) are emitted into the air and react with water to form sulfuric acid rain and nitric acid rain, respectively (Liu et al., 2022). Unlike the acid rain types of North America and Europe, sulfuric acid-based acid rain is the main type in China (Liu X. et al., 2020; Ma et al., 2019). Due to increasing environmental problems, the Chinese government developed a series of measures for the control of SO2 emissions, with the atmospheric content now being decreased by ~50% (Pu et al., 2014; Xin et al., 2018). However, the regulation of NOx emissions was initiated later (Mingxu et al., 2019); since the beginning of the twenty-first century, rapid economic development has led to the increased use of fertilizers, automobiles, and higher industrial emissions and agricultural pollutants (Lu et al., 2012). This has led to an upsurge of ammonia (NH3) and NOx concentrations in the ambient atmosphere, which translates to the generation of nitric acid rain. Meanwhile, NH3 and NOx (NO + NO2) emissions have accelerated nitrogen (N) deposition (Yu et al., 2019; Ma et al., 2023). the annual emissions of reactive N in China have increased by nearly 83.57% (Cui et al., 2013; Deng et al., 2023), which has resulted in the N deposition in China being much higher than that in other countries (Xuejun et al., 2013; Ge et al., 2023). In Southern China, the annual N deposition in the Yangtze River Delta (YRD) has reached 5.0 g·m−2·yr−1, which has exceeded the critical load of some ecosystems in this region (Qi et al., 2020). Excessive N deposition leads to an imbalance in the ecosystem N cycle (Duan et al., 2016); thus, it has emerged as a major environmental challenge.
Acid rain and N deposition can pose major threats to forest ecosystems (Liu et al., 2017b; Zhang et al., 2019). As a critical component of forest ecosystems, soil is most affected by the external environment (Kabatapendias et al., 1955; Xin et al., 2020). It has been shown that acid rain inputs of H+, sulfate (SO42-) and nitrate (NO3-) to the soil will directly lead to soil acidification (Chen et al., 2013; Song et al., 2023) disrupt the soil ion balance (Breemen et al., 1984; Ling et al., 2010), affect the composition of the soil microbial community, and alter soil enzyme activities (Zheng et al., 2022). Among them, the effects of nitrate-type acid rain on forest ecosystems are more complex than those of sulfate-type acid rain (Xin et al., 2018; Liu et al., 2022). Increased nitrogen deposition favours soil nitrogen effectiveness (Wang et al., 2011) and promotes plant and microbial growth, showing a promoting effect (Meunier et al., 2016; Xiao-Rong et al., 2016). However, at the same time, nitrogen deposition exacerbates soil acidification (Beloica et al., 2022) and, like acid rain, damages the soil environment. Numerous studies have shown that acid rain and nitrogen deposition occur simultaneously and interact with each other in atmospheric and soil processes (Liu Z. Q. et al., 2020; McDonnell et al., 2021), and analysing the ecological effects of acid rain or nitrogen deposition separately will affect the accuracy of the results (Zheng et al., 2018; Xiao et al., 2020). However, very few studies have been conducted on the interaction mechanisms of acid rain and nitrogen deposition on forest ecosystems, especially on their effects on degraded plantation forests in acid rain areas.
Cunninghamia lanceolata is an excellent fast-growing conifer tree that is endemic to China and a major afforestation species in the subtropical region of the Yangtze River Delta (Chen et al., 2021). Its planted forest area has reached 990.20 x 104 hm2, accounting for 17.33 per cent of the country’s planted forest area (Liu et al., 2018). However, due to the one-sided pursuit of fast-growing and abundant production, C. lanceolata plantation have suffered from ecological and production issues due to land degradation (Chen et al., 2020). Among them, nitrogen is an important factor limiting the productivity and ecosystem function maintenance of C. lanceolata plantation (Yu et al., 2002; Huang and Spohn, 2015), therefore, the increase of nitrogen deposition may show a promotion and enhancement effect on soil nutrients in C. lanceolata plantation. However, soil acidification due to the interaction of acid rain and nitrogen deposition may inhibit soil enzyme activities in C. lanceolata plantation, thus altering soil nutrient cycling.
Therefore, for this study, taking a Chinese subtropical C. lanceolata plantation as a research model, we formulated different types of experimental acid rain (nitrate and sulfate) and N addition treatments with the same acidity for field simulation experiments. The responses of the effects of simulated acid rain and N deposition on the soil nutrients of the C. lanceolata plantation were quantified by measuring the soil NAG enzymes, sucrase, acid phosphatases, arylsulfatase, urease, β-glucosidase, and hydrogen peroxidase. The purpose of this investigation was to more accurately evaluate the effects of acid rain and N deposition interactions on ecosystems for C. lanceolata plantation, with a view to providing a theoretical basis for the sustainable development of forest ecosystems in regions where acid rain and N deposition are currently severe or may be the future.
2 Materials and methods
2.1 Overview of the study area
The experimental site was established at the Yangtze River Delta Forest Ecosystem Positioning Research Station (32°77′ N, 119°12′ E) at the junction of Nanjing and Zhenjiang City, Jiangsu Province. This area belongs to a northern subtropical monsoon climate zone, with an average annual temperature of 15.2°C, maximum temperature of 39.6°C, minimum temperature of -16.7°C, an average annual relative humidity of 79%, and frost-free period of 233 d. The climate is mild and humid with four distinct seasons and abundant rain and heat resources. The rainy season extends through the summer, from June to August, with an average annual precipitation of 1055.6 mm. It is situated in Jianghuai, where the terrain is comprised of gentle hills and mountains. The average height of the C. lanceolata trees at the site was 10.8 m; the average diameter at breast height was 20.6 cm; the density of the forest stand was 850 plants-hm-2; the tree is 39 years old; the annual leaf area index was from 2.4 to 4.27; the soil type is mainly yellow-brown soil; the thickness of the soil layer is 40-60 cm; the pH of the soil was about 3.85; and the TC, TN,TS, AP, and AK content were about 49.3, 2.1, 3.9, 4.0, and 37.2, respectively.
2.2 Experimental design and sampling
2.2.1 Sample plot setting
This experiment was conducted based on the acid rain and N deposition simulation platform of the Yangtze River Delta Forest Ecosystem Positioning Research Station. (Nanjing, Jiangsu, China). The acid rain and N deposition simulation sample plots were established in February 1, 2021 in a C. lanceolata plantation. The dimensions of the sample plots were 10 m × 10 m, which were partitioned with PVC boards at 1.0 m above each sample plot (Avoid inflow of other treatment analogue solutions).
2.2.2 Acid rain and nitrogen deposition settings
Three acid rain types were set up by combining 0.5 mol-L-1 H2 SO4 and 0.5 mol-L-1 HNO3 at different concentration ratios: Sulfuric acid rain is formulated at a concentration ratio of 5:1, Nitric acid rain is formulated at a concentration ratio of 1:5. This corresponds to the general anion composition of rainfall in Nanjing city (Pu et al., 2014), and set up control and nitrogen deposition simulation plots. Six simulation treatment groups were established, which included control (CK), sulfuric acid-type acid rain (SR) (pH = 2.5), nitric acid-type acid rain (NR) (pH = 2.5), nitrogen addition (N) (15 g-m-2-yr-1), sulfuric acid-type acid rain + N addition (SRN, pH=2.5, 15 g·m-2·yr-1), nitric acid-type acid rain + N addition (NRN, pH=2.5, 15 g-m-2-yr-1).
The N deposition simulation tests were conducted using urea (CO(NH2)2) at a nitrogen addition level of 15 g-m-2-yr-1. The annual urea addition was 3217.50 g for the 10 m x 10 m sample plot, at a monthly application of 268.13 g. This was divided into two sprays per month mixed with a simulated acid rain solution, with the total amount of simulated acid rain being equivalent to the approximate average monthly rainfall.
2.2.3 Simulated rainfall
In an area 5 m distant from the sample plot a rainwater catchment tank (8.33 m2) was installed, which was 1/12 the size of the 10 m × 10 m sample plot. These rainwater catchment tanks were matched with each 10 m × 10 m sample plot, where the collected rainwater penetrating through the C. lanceolata plantation was used to formulate the acid rain simulation solutions (after each rainfall, the mother liquor was mixed with the collected penetrating rain to create the appropriate simulated acid rain solutions, pH 2.5, hydrogen ion input 0.28; the input of hydrogen ions is less than the input of hydrogen ions in severe areas and does not exceed the current most severe acid rain situation), which was then sprayed evenly in the 10 m × 10 m sample plots using a shower (CNL-1201, Zhejiang Chaonongli Intelligent Technology Co., Ltd.). Simultaneously, the collected penetrating rain was sprayed directly onto the control plots. Each simulation treatment involved three 10 m × 10 m sample plots. Further, a set of blank experiments was established that involved three small sample squares without the addition of simulation solutions. These were created to assess the interactive effects of additional moisture inputs. Simulation lasts 18 months (February 1, 2021–July 3, 2022).
2.2.4 Sample collection
Soil samples were collected in July 3, 2022 by randomly sampling a five-point mixture within each sample plot. The sampling was done in quadrats with three replicates per sample plot, for six sample plots (CK, SR, NR, N, SRN, NRN) for a total of 18 soil samples. These were passed through a sieve with a 2 mm pore size, and all leaves, plant roots, and stones were removed. All soil samples were stored in Ziplock bags in a small refrigerator at 4°C pending transfer to the laboratory for analysis.
2.3 Characterization methods
The soil sample properties were determined using methods obtained from the literature (Liu et al., 2017a). The potentiometric method was used to quantify the soil pH; the ammonia fluoride-hydrochloric acid leaching technique was employed to determine the soil available phosphorus (AP); the ammonium acetate-flame photometric process was used to measure the soil available potassium (AK); an elemental analyzer was used to determine the soil carbon (TC), nitrogen (TN), and sulfur (TS), the two-wavelength UV-colorimetric method was utilized to quantify the nitrate nitrogen (Nitrate-Nitrogen) via 2 mol/L KCl leaching. The ammonium N was determined using 2 mol/L KCl extraction and the indophenol blue colorimetric method. The main enzymes involved in the C, N, P, and S cycles were determined spectrophotometrically (Du et al., 2017; Vassanda et al., 2017). These included: sucrase using a 3,5-dinitrosalicylic acid colorimetric assay; urease using a sodium benzoate-sodium hypochlorite colorimetric assay; acid phosphatase using a p-nitrophenyl disodium phosphate colorimetric assay; catalase using a potassium permanganate titration assay; arylsulfataseusing a p-nitrophenyl sulfate method; β-glucosaminidase using a nitro phenol colorimetric assay; and NAG enzymes using the p-nitrophenol method.
2.4 Data analysis
Microsoft Excel 2021 software was used to summarize and collate the raw experimental data. SPSS 22.0 was used to perform one-way analysis of variance (ANOVA, Duncan’s test, significance level 0.05) on the soil nutrient content and soil enzyme activities under the simulated acid rain and nitrogen deposition. R language was used to analyze the correlations between the soil nutrients and enzyme activities (Pearson). The structural equation model (SEM) was used to explain the direct and indirect effects of soil nutrients on enzyme activity, and graphs were drawn using GraphPad Prism 9.0.
3 Results
3.1 One-way analysis of variance (ANOVA) of various indicators of soil in C. lanceolata plantation under different treatments
3.1.1 Changes in soil pH of C. lanceolata plantation under different treatments
The soil pH of the C lanceolata plantation under the CK treatment was ~3.85 (Figure 1). Overall, there was a decrease in the soil pH under both the simulated acid rain and N deposition treatments. In particular, the N and NR treatments decreased it by 0.07 (1.73%) and 0.13 (3.38%), respectively, compared to the CK treatment, with negligible differences (p > 0.05). Further, the SR, SRN, and NRN treatments decreased the pH by 0.20 (5.20%), 0.22 (5.63%), and 0.20 (5.20%), respectively, with significant differences (p < 0.05). In addition, the SRN and NRN treatments reduced the soil pH by 0.15 (39.68%) and 0.14 (36.16%), respectively, compared with the N treatment (Figure 1). The SRN treatment reduced the soil pH by 0.02 (0.46%) compared to the SR treatment, while the NRN treatment reduced it by 0.07 (1.97%) compared to the NR treatment (Figure 1).
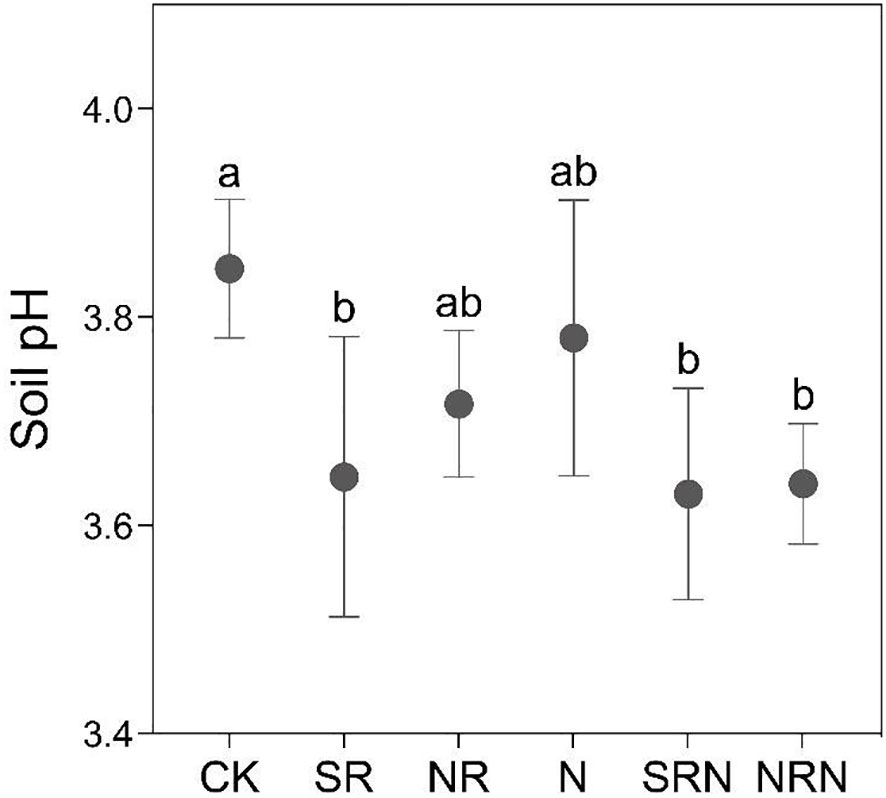
Figure 1 Changes of soil pH in C. lanceolata plantation under different treatments. CK, Control; SR, Sulfuric acid rain; NR, Nitric acid rain; N, Nitrogen deposition treatment; SRN, Sulfuric acid rain plus nitrogen deposition; NRN, Nitric acid rain plus nitrogen deposition; Different letters denote significant differences (p<0.05) between treatments.
3.1.2 Changes in soil nutrient content in C. lanceolata plantation under different treatments
Acid rain and nitrogen deposition reduced the soil total carbon content and soil total sulfur (TS) content of C. lanceolata plantation (except for TS with SRN treatment), but only the changes of NRN treatment was significant (p < 0.05, Figures 2A, C). In addition, NR and N treatments increased soil total nitrogen (TN) content, however, NRN significantly decreased TN content (p < 0.05, Figure 2B). What’s more, no significant difference of soil C/N ratio was found among acid rain and nitrogen deposition treatments (p > 0.05, Figure 2D).
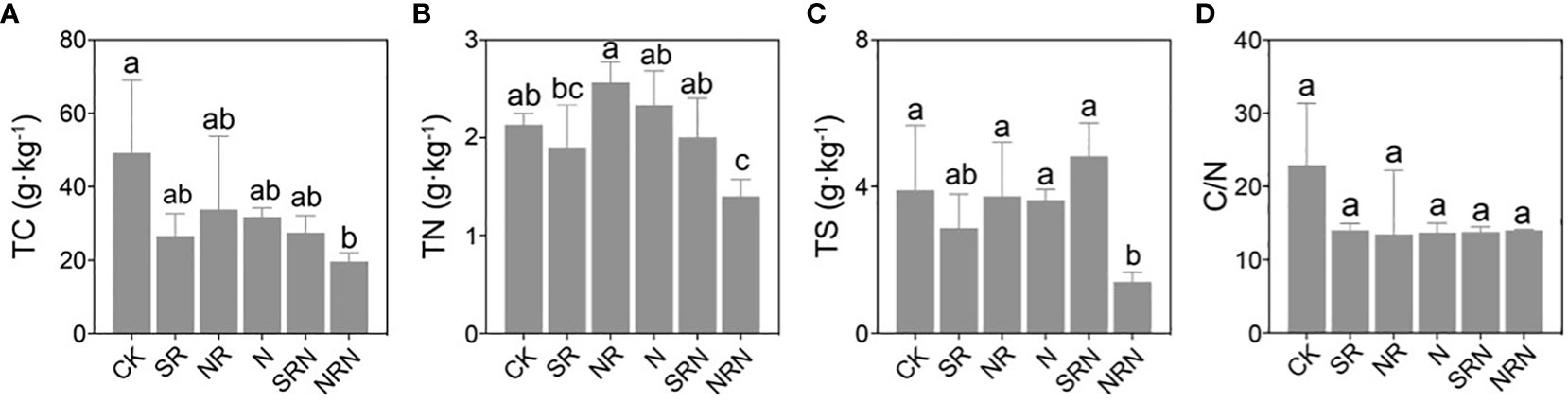
Figure 2 Changes in soil (A) TC, (B) TN, (C) TS, and (D) C/N content in the C. lanceolata plantation under different treatments. CK, Control; SR, Sulfuric acid rain; NR, Nitric acid rain; N, Nitrogen deposition treatment; SRN, Sulfuric acid rain plus nitrogen deposition; NRN, Nitric acid rain plus nitrogen deposition; Different letters denote significant differences (p<0.05) between treatments.
Overall, the available potassium decreased and nitrate N increased (Figures 3B, D), while the ammonium N increased (Figure 3C, except for the N treatment), following the simulated acid rain and N deposition treatments compared to the CK. In particular, acid rain and N deposition significantly decreased the available potassium (Figure 3B) (p < 0.05), while the SRN and NRN treatments increased the ammonium N considerably (Figure 3C) (p < 0.05), and the SR, N, and NRN treatments significantly increased nitrate N (Figure 3D) (p < 0.05). Additionally, both the available potassium and available phosphorus decreased under the SRN and NRN treatments compared to the N treatment (Figures 3A, B), while the ammonium N was increased (Figure 3C).
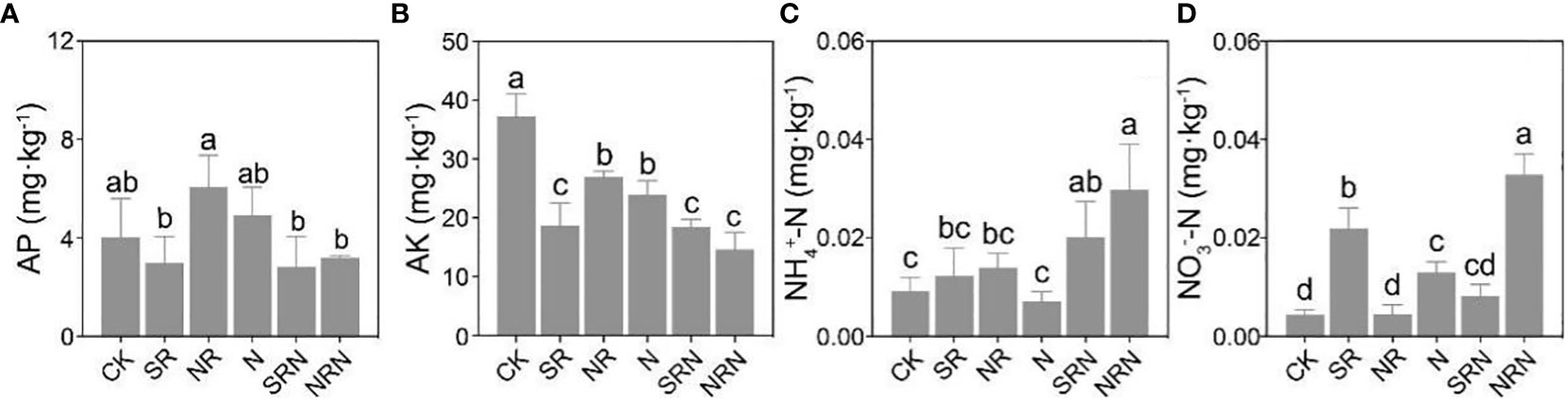
Figure 3 Changes in soil chemical properties of the C. lanceolata plantation under no treatment: (A) AP, (B) AK, (C) NH4+-N, (D) NO3--N. CK, Control; SR, Sulfuric acid rain; NR, Nitric acid rain; N, Nitrogen deposition treatment; SRN, Sulfuric acid rain plus nitrogen deposition; NRN, Nitric acid rain plus nitrogen deposition; Different letters denote significant differences (p<0.05) between treatments.
3.1.3 Changes in soil enzyme activities in C. lanceolata plantation under different treatments
In general, it appeared that NAGase activity increased, and urease activity decreased under the simulated acid rain and N deposition treatments compared to the CK (Figures 4A, G).
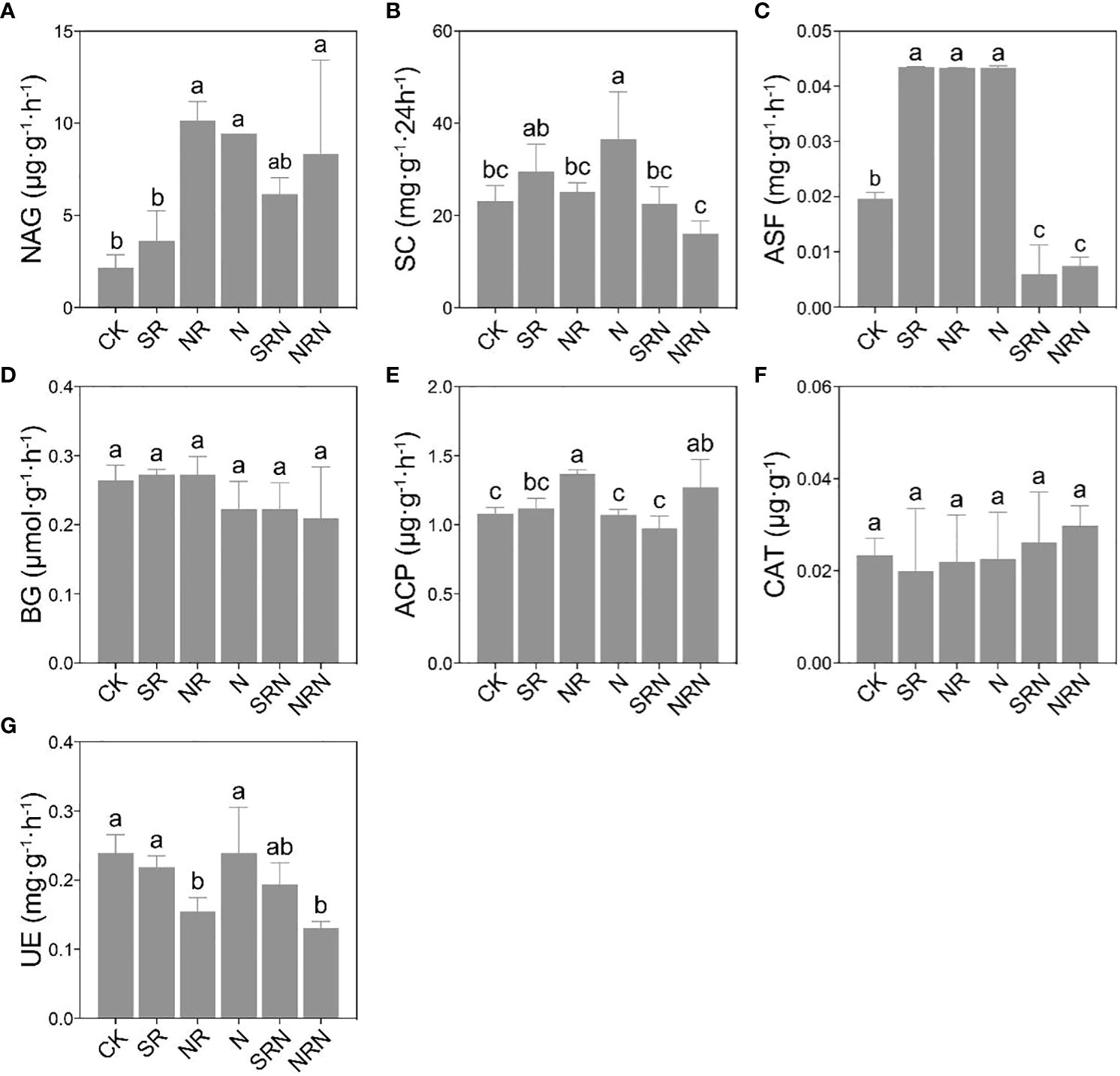
Figure 4 Changes of soil enzyme activities in C. lanceolata plantation under different treatments. (A) N-acetyl-b-D-glucosidase (NAG), (B) sucrase (SC), (C) arylsulfatase (ASF), (D) β‐glucosidase (BG), (E) acid phosphatase (ACP), (F) catalase (CAT), (G) urease (UE). CK, Control; SR, Sulfuric acid rain; NR, Nitric acid rain; N, Nitrogen deposition treatment; SRN, Sulfuric acid rain plus nitrogen deposition; NRN, Nitric acid rain plus nitrogen deposition; Different letters denote significant differences (p<0.05) between treatments.
Particularly, the NAGase activities increased significantly by 334.70%, 367.88%, and 284.47% under the N, NR and NRN treatments, respectively (Figure 4A) (P < 0.05), while the urease activities decreased considerably by 35.47% and 39.98% under the NR and NRN treatments, respectively (Figure 4G) (P < 0.05). The acid phosphatase increased significantly under the NR and NRN treatments (Figure 4E) (P < 0.05); the convertase activities increased considerably under N treatment but decreased significantly under the NRN treatment (Figure 4B) (P < 0.05); and the arylsulfatase activities decreased dramatically under SRN and NRN treatments (Figure 4C) (P < 0.05). Furthermore, the SRN and NRN treatments reduced the activities of convertase, arylsulfatase, β-glucosidase, and urease (Figures 4B–D, G), while the SRN and NRN treatments strongly diminished the arylsulfatase activities (Figure 4C) (P < 0.05), albeit enhanced catalase (Figure 4F) in contrast to the N treatment.
3.2 Relationships between soil nutrients and soil enzyme activities in C. lanceolata plantation under different treatments
From the correlation analysis, it was observed that the soil pH was significantly negatively correlated with ammonium N, while strongly positively correlated with soil catalase (P < 0.05). Total C was significantly (P < 0.05) positively correlated with urease. Total N was significantly (P < 0.05) positively correlated with arylsulfatase and sucrase. Arylsulfatase, however, was significantly (P < 0.05) negatively correlated with ammonium N. In addition, sucrase was significantly positively correlated (P < 0.05) with total N, urease and arylsulfatase.
Based on the results of the correlation analysis a structural equation model (SEM) was developed. Model fitting was the overall fitting of the initial model using the test data. This was based on the standard values of the fit indices of the structural equation model shown in Table 1, to determine whether the model met the parameter requirements.
The chi square degree of freedom ratio (CHISQ/DF) was 1.115 (<5), the chi square test showed a p-value of 0.892 (p>0.05), the goodness of fit index (GFI) value was 0.983 (>0.900), and the root of approximation error (RMSEA) value was 0.000 (<0.08), which indicated that the model met the parameter requirements.
As shown in Figure 5, the results indicated that the direct effects of soil pH on ammonium N(-0.505, p < 0.01)was significant; the direct effects of ammonium N on arylsulfate lyase (-0.925, p < 0.001) and soil sucrase (-0.475, p < 0.05) were significant; the direct effect of sucrase on urease was also significant (0.694, p < 0.001), while the direct effect of urease on acid phosphatase was significant (-0.746, p < 0.001), while the direct effect of acid phosphatase on arylsulfatase was significant (0.312, p < 0.05).the direct effect of sucrase on arylsulfatase was significant (0.380, p < 0.05).
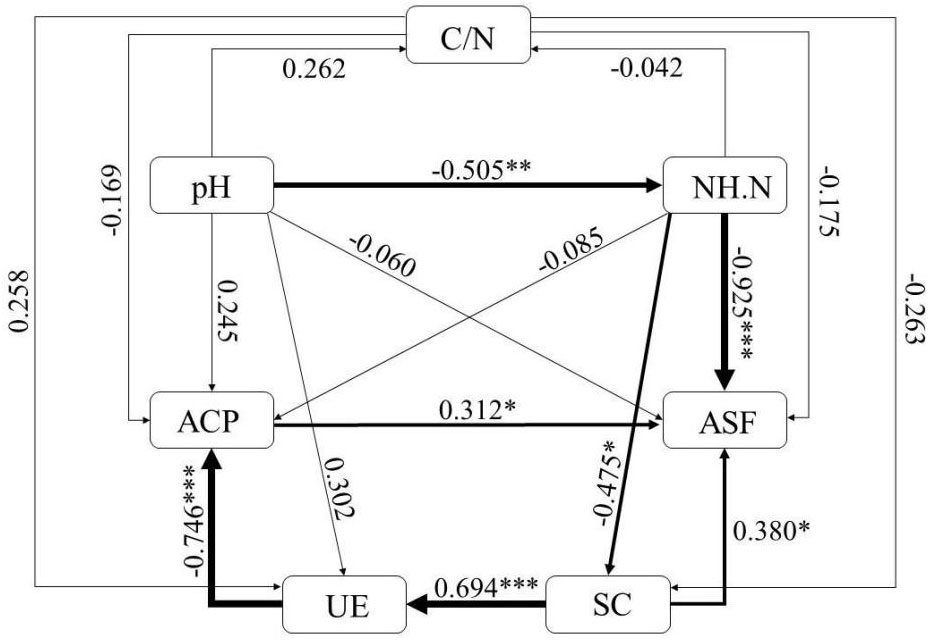
Figure 5 SEM analysis of acid rain and nitrogen deposition on soil nutrients and enzyme activities. Numbers on arrows are standardized path coefficients. The widths of the arrows indicate the strengths of the causal effects. pH: soil pH; C/N: soil carbon ratio; NH.N: ammonium nitrogen; ACP: acid phosphatase; UE: urease; SC: sucrase; ASF: arylsulfatase.
4 Discussion
Soil is the ultimate recipient of acid rain and N deposition, which can alter the chemical properties of the soil (Houlton and Morford, 2015; Sosa Echeverría et al., 2023), as both acid rain and N deposition can lead to soil acidification (Huang et al., 2014). The essence of soil acidification is that the H+ from acid rain is adsorbed to the surface soil particles by exchanging with salt-based ions bound to the surfaces of the soil colloids. The exchanged salt-based ions are leached off, after which the hydrogen ions on the surface of the soil particles react with aluminum on the surface of the mineral lattice to convert it to a form that can participate in the exchange reaction (Dong et al., 2022). N deposition alters the soil N cycle, which can control the hydrogen ion cycle (Zong et al., 2017). It is generally accepted that N deposition accelerates soil acidification (Aber et al., 2003; Patel et al., 2020). The effect of soil acidification is closely related to soil pH; thus, the more severe the soil acidification the lower the soil pH. The results of this study revealed that both acid rain and N deposition induced the soil pH to decrease (Figure 1). Compared with N addition alone, the combined effects of acid rain and N deposition reduced the soil pH by ~0.15 (37.92%) (Figure 1). However, compared with the addition of acid rain alone, the combined effects of acid rain and N deposition reduced the soil pH by only ~0.09 (1.22%) (Figure 1), which suggested that acid rain had a greater impact on the soil pH than did N deposition. Soil acidification through the further application of N can effectively increase the total N, ammonium N, and nitrate N contents of surface soils (Geng et al., 2020; Zhang et al., 2023). In this study, only the total N and nitrate N contents were increased under the N application treatments, while the ammonium N content was decreased (Figure 2B, 3C, D). This may have been due to the rapid loss of available N in a short time period, as well as the acceleration of the further oxidation of ammonium N to nitric acid under the action of microorganisms (Kazuo et al., 2012; Lei et al., 2016). The concentration of ammonium N decreased, its hydrolysis process was weakened and the soil pH increased, in alignment with the results of our correlation analysis, which showed a significantly negative correlation between the soil pH and ammonium N (Figure 6) (P < 0.05). The soil pH exhibited a strong direct effect on ammonium N (Figure 5) (P < 0.05).
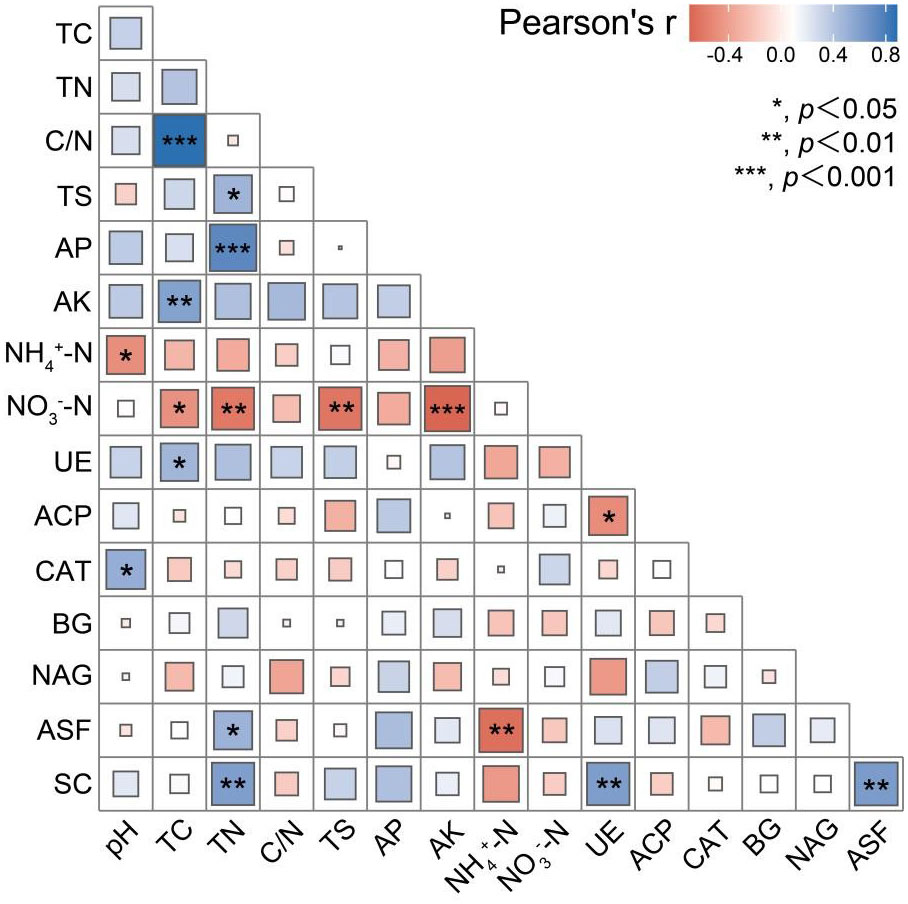
Figure 6 Correlation analysis between soil nutrients and enzymes under different treatments. pH. soil pH; TC, TN, C/N, and TS denote soil total carbon, total nitrogen, carbon to nitrogen ratio, and total sulfur content, respectively; AP and AK denote soil available phosphorus and available potassium content, respectively; NH4+-N and NO3–N denote soil ammonium N and nitrate nitrogen content; UE, ACP, CAT, GC, NAG, ASF, and SC denote soil urease, acid phosphatase, catalase, β-glucosidase, N-acetyl-β-D-glucosidase, arylsulfatase, and sucrase activities, respectively. *** indicates significant correlation at p < 0.001; ** indicates significant correlation at p < 0.01; * indicates significant correlation at p < 0.05.
Furthermore, both the total C and total N were decreased and the ammonium N content increased when the acid rain and N deposition acted together in contrast to the N treatment alone (Figures 2A, B, 3C). This indicated that the acid rain treatments of this study reduced the total C and total N content to some extent. Due to the fact that the soil in this experiment was collected from the surface layer of 0-20 cm and was greatly affected by the input of litter, while acid rain acidified the soil, resulting in a decrease in microbial activity and possibly inhibiting the decomposition of litter, thereby reducing the input of organic matter and affecting the total carbon content. In addition, the soil nitrate N content under the NRN treatment was greater than that under the SRN treatment, which might be explained by the increased inorganic N input under the NRN treatment. This led to an enhancement of the N metabolism of soil microorganisms, which accelerated the decomposition of insoluble N substances in the soil (Li et al., 2018; Liu et al., 2022). Compared with the SR treatment, the SRN treatment resulted in a significant decrease (Figure 3D) (P < 0.05) in soil nitrate nitrogen content, which could be attributed to the fact that the input of nitrogen fertiliser in the SRN treatment enhanced soil microbial acti vity, improved nitrate nitrogen leaching and reduced the nitrate nitrogen content (De Vries and Breeuwsma, 1987; Brown et al., 2021; Li et al., 2023). Currently, there are inconsistent reports as to the effects of N deposition on soil nutrients. Martinsen et al. concluded that N deposition had almost no effect on the soil nutrient content (Martinsen et al., 2012), which may have been due to the fact that N deposition not only promotes the decomposition of soil organic N, but also improves the uptake and utilization of inorganic N by plants (Deluca and Zouhar, 2000; Lu et al., 2021). Nitrogen mineralization affects the bioavailability of N, and earlier studies have shown that N inputs increased the initial N mineralization rate in soils, as increased N bound to organic matter decreased the soil C/N ratio and accelerated the decomposition of soil organic matter and nutrient release processes (Aber et al., 1989; Drewniak and Gonzalez-Meler, 2017). In our results, the soil C/N ratios in C. lanceolata plantation decreased with higher levels of simulated acid rain and N deposition (Figure 2D) (P < 0.05), which was consistent with the results of previous studies (Shuang-Li et al., 2020; Ziqiang et al., 2021).
Further, although the gross mineralization of soil N increased under long-term continuous N application, the net mineralization rate exhibited a decrease from the prior peak and was close to, or lower than, the CK value, with the net mineralization reaching its maximum at moderate N deposition levels (Albert et al., 1998). A field study undertaken by Gundersen et al. found that increased N inputs enhanced the net mineralization rate only in the N-limited areas, whereas the further application of N delayed the net mineralization of soil N in areas with faster N cycling rates (Gundersen et al., 1998). There were two potential explanations for the decreased N mineralization rate. Firstly, increased N levels altered the chemical structure of the soil organic matter and reduced the efficacy of extracellular metabolic enzymes. Secondly, the quantity of humicolytic enzymes generated by soil microorganisms, especially fungi, decreased with the higher soil mineral N content (Aber et al., 1989; Chen et al., 2022).
Acid rain and N deposition led to soil acidification, which altered the soil chemistry and in turn affected soil microorganisms and soil enzyme activities. Soil urease hydrolyses urea to carbonic acid and ammonia, which is a characterization of soil N utilization (Xu et al., 2015b). The results of our correlation analysis revealed a negative correlation between urease and ammonium N (Figure 6), which suggested that enhanced leaching of N from the soil led to an enhanced competition for N between plants and microorganisms and increased the secretion of urease (Wang et al., 2008). Urease is an enzyme associated with the acquisition of N by plants and microorganisms, where an increase in its activity can lead to a faster rate of soil organic N mineralization (Wang et al., 2008; Ren et al., 2014). It is generally believed that an increase in the active soil N content leads to a corresponding increase in the demand for other nutrients (e.g., carbon and phosphorus) by soil microorganisms. Associated enzyme activities are also elevated (e.g., Keeler et al. found that the application of N simultaneously increased soil phosphatase and cellulase activities) (Keeler et al., 2009). In agreement with the results of the present study, there was a significantly positive correlation between the soil sucrase and urease (Figure 6) (P < 0.05), where sucrase enzymes had a significant direct effect on urease (Figure 5) (p < 0.001). In addition, the phosphatase activities under the SRN treatments of this study decreased with higher N application levels, while sucrase activities decreased (Figures 4B, E). This suggested that, in this study, the soil ecosystem of the sample site may have transitioned from N limited to C limited. Overall, in addition to this, there was an increase in NAGase activities following the acid rain and N deposition treatments (Figure 4A). Among them, the activities of NAG enzymes increased significantly only under the N deposition treatments (Figure 4A) (P<0.05), which implied that the NAG enzyme activities in this study may be used as an indicator of the difficulties involved in the microbial acquisition of N due to N deposition.
The change in soil enzyme activity is a complex physicochemical proces, where different enzyme activities in diverse ecosystems respond variably to acid rain and N deposition, which needs to be further explored by combining soil enzyme changes with environmental factors. De Forest et al. (DeForest et al., 2004; Zhou et al., 2018) found that N deposition inhibited the soil peroxidase activity when they investigated the responses of northern broadleaved plantation to N deposition. Li et al. (2022) found that simulated acid rain increased soil catalase activities. Consistent with our findings, the combined effects of acid rain and N deposition increased the soil catalase activities in contrast to the N treatment alone (Figure 4F). This indicated that the soil pH promoted soil catalase activities in the short term (Figure 6) (P < 0.05). Furthermore, Cusack et al. (Pregitzer et al., 2008; Liu et al., 2021) observed that N deposition inhibited the activities of peroxidase in tropical rainforests, albeit promoted those of the enzymes in tropical montane forests. Differences in the effects of N deposition on peroxidase activities may have been due to differences in the background N content and ecosystem N inputs, and those in the structures of soil microbial communities. Kim et al. (Kim and Kang, 2011) and Cusack et al. (Pregitzer et al., 2008; Kazanski et al., 2019) demonstrated that when ecosystems had high background N deposition or soil N contents, this tended to inhibit peroxidase and polyphenoloxidase activities, and conversely tended to increase their activities. In addition, we found that NRN and SRN treatments significantly reduced arylsulfatase activities by ~62.24% and 69.30%, respectively (Figure 4C), which was ~twice that through the N addition alone in an earlier study (Wang et al., 2016; Feyzi et al., 2020). This indicated that arylsulfatase activities were more sensitive to the effects of acid rain than N.
It should be noted that the effects of acid rain and nitrogen deposition on forest soil ecosystems are a very complex process that requires long-term monitoring, and due to the limitations of the observation period, although preliminary results have been obtained in the present study, there are still many issues that need to be further investigated. This study has a relatively short period of time, and there may be some accumulated effects that have not been reflected, and experiments and observations with a longer time span should be carried out in the future. In addition, in the context of acid rain and nitrogen deposition, forest ecosystems are accompanied by problems such as increased CO2 concentration, soil erosion, water environment pollution, deforestation, and climate warming, and changes in these conditions can have profound effects on soil ecosystems. Therefore, future research that integrates other multifactorial changes will help to further reveal the mechanisms of soil ecosystem change in the context of global environmental change.
5 Conclusion
Through a one-year experiment, we analysed the difference between the interaction of acid rain and nitrogen deposition and its single-factor effect on soil properties in C. lanceolata plantation, and the results showed that nitrogen deposition had a lower effect on soil pH than acid rain, but nitrogen deposition exacerbated soil acidification, and that soil effective nutrients and enzyme activities were more easily affected by acid rain and nitrogen deposition, in which the interaction between acid rain and nitrogen deposition significantly increased soil ammonium nitrogen and nitrate nitrogen content and decreased soil arylsulfatase activity. In general, the interaction of acid rain and nitrogen deposition exacerbated soil acidification, changed soil nutrients and enzyme activities, and had a stronger effect on the soil ecosystems of the C. lanceolata plantation than the single-factor effects of acid rain or nitrogen deposition.
Data availability statement
The original contributions presented in the study are included in the article/supplementary material. Further inquiries can be directed to the corresponding author.
Ethics statement
Written informed consent was obtained from the individual(s) for the publication of any potentially identifiable images or data included in this article.
Author contributions
YD: Conceptualization, Data curation, Formal analysis, Investigation, Methodology, Project administration, Resources, Software, Supervision, Validation, Visualization, Writing – original draft, Writing – review & editing. LS: Investigation, Project administration, Resources, Supervision, Visualization, Writing – review & editing. CL: Conceptualization, Formal analysis, Methodology, Software, Supervision, Writing – original draft. MC: Data curation, Investigation, Project administration, Supervision, Writing – review & editing. YZ: Formal analysis, Investigation, Supervision, Visualization, Writing – review & editing. MM: Funding acquisition, Resources, Supervision, Writing – review & editing. ZL: Project administration, Resources, Visualization, Writing – review & editing. JZ: Formal analysis, Funding acquisition, Methodology, Project administration, Resources, Supervision, Visualization, Writing – original draft, Writing – review & editing. XL: Conceptualization, Formal analysis, Methodology, Project administration, Resources, Validation, Visualization, Writing – original draft, Writing – review & editing.
Funding
The author(s) declare financial support was received for the research, authorship, and/or publication of this article. XL and JZ acknowledges funding support from the Innovation and Promotion of Forestry Science and Technology Program of Jiangsu Province (LYKJ[2021]30), Jiangsu Science and Technology Plan Project (BE2022420), Scientific Research Project of Baishanzu National Park (2021ZDLY01), and the Priority Academic Program Development of Jiangsu Higher Education Institutions (PAPD). YD is grateful for the partial financial support from the Postgraduate Research and Practice Innovation Program of Jiangsu Province (KYCX23_1240), Ningxia key research and development plan (No. 2021BEG02010).
Acknowledgments
We would like to thank Xia Shu forestry farm for assistance of field work. Special thanks go to Mr. Gong for his invaluable cooperation and assistance in managing the field experiment during the course of this study.
Conflict of interest
The authors declare that the research was conducted in the absence of any commercial or financial relationships that could be construed as a potential conflict of interest.
Publisher’s note
All claims expressed in this article are solely those of the authors and do not necessarily represent those of their affiliated organizations, or those of the publisher, the editors and the reviewers. Any product that may be evaluated in this article, or claim that may be made by its manufacturer, is not guaranteed or endorsed by the publisher.
References
Aber J. D., Goodale C. L., Ollinger S. V., Marie-Louise S., Magill A. H., Martin M. E., et al. (2003). Is nitrogen deposition altering the nitrogen status of northeastern forests? Bioence 53, 375–389. doi: 10.1641/0006-3568(2003)053[0375:INDATN]2.0.CO;2
Aber J. D., Nadelhoffer K. J., Steudler P., Melillo J. M. (1989). Nitrogen saturation in northern forest ecosystems: Excess nitrogen from fossil-fuel combustion may stress the biosphere. BioScience 39, 378–386. doi: 10.2307/1311067
Albert T., Emmett B. A., Gundersen P., Kjønaas O., Koopmans C. J. (1998). The fate of 15N- labelled nitrogen deposition in coniferous forest ecosystems. For. Ecol. Manage. 101, 19–27. doi: 10.1016/s0378-1127(97)00123-0
Beloica J., Belanovi Simi S., Avlovi D., Kadovi R., Kneevi M., Obratov-Petkovi D., et al. (2022). Soil acidification patterns due to long-term sulphur and nitrogen deposition and how they affect changes in vegetation composition in Eastern Serbia. Adv. Understanding Soil Degradation 21, 737–754. doi: 10.1007/978-3-030-85682-3_34
Breemen N. v., Driscoll C. T., Mulder J. (1984). Acidic deposition and internal proton sources in acidification of soils and waters. Nature 307, 599–604. doi: 10.1038/307599a0
Brown S. P., Brogden M., Cortes C., Tucker A. E., Snyder B. A. (2021). Investigating the effects of nitrogen deposition and substrates on the microbiome and mycobiome of the millipede Cherokia Georgiana Georgiana (Diplopoda: Polydesmida). Soil Biol. Biochem. 159, 108285. doi: 10.1016/j.soilbio.2021.108285
Chen D., Lan Z., Bai X., Grace J. B., Bai Y. (2013). Evidence that acidification-induced declines in plant diversity and productivity are mediated by changes in below-ground communities and soil properties in a semi-arid steppe. J. Ecol. 101, 1322–1334. doi: 10.1111/1365-2745.12119
Chen L. C., Guan X., Wang Q. K., Yang Q. P., Zhang W. D., Wang S. L. (2020). Effects of phenolic acids on soil nitrogen mineralization over successive rotations in Chinese fir plantations. J. Forestry Res. 31, 303–311. doi: 10.1007/s11676-018-0842-z
Chen W., Su F., Nie Y., Zhong B., Zheng Y., Mo J., et al. (2022). Divergent responses of soil microbial functional groups to long-term high nitrogen presence in the tropical forests. Sci. Total Environ. 821, 153251. doi: 10.1016/j.scitotenv.2022.153251
Chen L., Xiang W., Ouyang S., Wu H., Xia Q., Ma J., et al. (2021). Tight coupling of fungal community composition with soil quality in a Chinese fir plantation chronosequence. Land Degradation Dev. 32, 1164–78. doi: 10.1139/CJSS-2019-0142
Cui S., Shi Y., Groffman P. M., Schlesinger W. H., Zhu Y. G. (2013). Centennial-scale analysis of the creation and fate of reactive nitrogen in China, (1910–2010). Proc. Natl. Acad. Sci. United States America 110, 2052–2057. doi: 10.1073/pnas.1221638110
DeForest J. L., Zak D. R., Pregitzer K. S., Burton A. J. (2004). Atmospheric nitrate deposition and the microbial degradation of cellobiose and vanillin in a northern hardwood forest. Soil Biol. Biochem. 36, 965–971. doi: 10.1016/j.soilbio.2004.02.011
Deluca T. H., Zouhar K. L. (2000). Effects of selection harvest and prescribed fire on the soil nitrogen status of ponderosa pine forests. For. Ecol. Manage. 138, 263–271. doi: 10.1016/S0378-1127(00)00401-1
Deng J., Nie W., Huang X., Ding A., Qin B., Fu C. (2023). Atmospheric reactive nitrogen deposition from 2010 to 2021 in Lake Taihu and the effects on phytoplankton. Environ. Sci. Technol. 57, 8075–84. doi: 10.1021/acs.est.2c09434
De Vries W., Breeuwsma A. (1987). The relation between soil acidification and element cycling. Water Air Soil pollut. 35, 293–310. doi: 10.1007/BF00290937
Dong Y., Yang J. L., Zhao X. R., Yang S. H., Mulder J., Drsch P., et al. (2022). Soil acidification and loss of base cations in a subtropical agricultural watershed. Sci. Total Environ. 827, 154338. doi: 10.1016/j.scitotenv.2022.154338
Drewniak B., Gonzalez-Meler M. A. (2017). Earth system model needs for including the interactive representation of nitrogen deposition and drought effects on forested ecosystems. Forests 8, 267. doi: 10.3390/f8080267
Du L., Huang B., Du N., Guo S., Shu S., Sun J. (2017). Effects of garlic/cucumber relay intercropping on soil enzyme activities and the microbial environment in continuous cropping. Hortsci. A Publ. Am. Soc. Hortic. Sci. 52, 78–84. doi: 10.21273/hortsci11442-16
Duan L., Yu Q., Zhang Q., Wang Z., Pan Y., Larssen T., et al. (2016). Acid deposition in Asia: Emissions, deposition, and ecosystem effects. Atmospheric Environ. 146, 55–69. doi: 10.1016/j.atmosenv.2016.07.018
Feyzi H., Chorom M., Bagheri G. (2020). Urease activity and microbial biomass of carbon in hydrocarbon contaminated soils. A case study of cheshmeh-khosh oil field, Iran. Ecotoxicol. Environ. Saf. 199, 110664. doi: 10.1016/j.ecoenv.2020.110664
Ge Z., Wang D., Liu Q., Wang J., Zhang J., Cheng R., et al. (2023). Effects of two-year nitrogen and phosphorus additions on nutrient release and enzyme activity during leaf litter decomposition in Larix principis-rupprechtii plantation. Plant Soil. 487, 521–532. doi: 10.1007/s11104-023-05950-7
Geng J., Fang H., Cheng S., Pei J. (2020). Effects of N deposition on the quality and quantity of soil organic matter in a boreal forest: Contrasting roles of ammonium and nitrate. Catena 198, 104996. doi: 10.1016/j.catena.2020.104996
Grennfelt P., Engleryd A., Forsius M., Hov Ø., Rodhe H., Cowling E. (2020). Acid rain and air pollution: 50 years of progress in environmental science and policy. Ambio 49, 849–864. doi: 10.1007/s13280-019-01244-4
Gundersen P., Emmett B. A., Kjønaas O. J., Koopmans C. J., Tietema A. (1998). Impact of nitrogen deposition on nitrogen cycling in forests: a synthesis of NITREX data. For. Ecol. Manage. 101, 37–55. doi: 10.1016/S0378-1127(97)00124-2
Houlton B. Z., Morford S. L. (2015). A new synthesis for terrestrial nitrogen inputs. SOIL 1, 381–397. doi: 10.5194/soil-1-381-2015
Huang J., Mo J. M., Zhang W., Lu X. K. (2014). Research on acidification in forest soil driven by atmospheric nitrogen deposition. Acta Ecologica Sin. 34, 302–310. doi: 10.1016/j.chnaes.2014.10.002
Huang W., Spohn M. (2015). Effects of long-term litter manipulation on soil carbon, nitrogen, and phosphorus in a temperate deciduous forest. Soil Biol. Biochem. 83, 12–18. doi: 10.1016/j.soilbio.2015.01.011
Kabatapendias A., Pendias H., Kabata P. A., Pendias H., Kabatapendias A., Kabata Pendias A., et al. (1955). Trace elements in soil and plants. Nutr. Rev. 13, 337–339. doi: 10.1111/j.1753-4887.1955.tb03377.x
Kazanski C. E., Riggs C. E., Reich P. B., Hobbie S. E. (2019). Long-term nitrogen addition does not increase soil carbon storage or cycling across eight temperate forest and grassland sites on a sandy outwash plain. Ecosystems 22, 1592–1605. doi: 10.1007/s10021-019-00357-x
Kazuo I., Keisuke K., Yuichi S., Junko I., Yunting F., Muneoki Y., et al. (2012). High abundance of ammonia-oxidizing archaea in acidified subtropical forest soils in southern China after long-term N deposition. FEMS Microbiol. Ecol. 80, 193–203. doi: 10.1111/j.1574-6941.2011.01294.x
Keeler B. L., Hobbie S. E., Kellogg L. E. (2009). Effects of long-term nitrogen addition on microbial enzyme activity in eight forested and grassland sites: implications for litter and soil organic matter decomposition. Ecosystems 12, 1–15. doi: 10.1007/s10021-008-9199-z
Kim H., Kang H. (2011). The impacts of excessive nitrogen additions on enzyme activities and nutrient leaching in two contrasting forest soils. J. Microbiol. 49, 369. doi: 10.1007/s12275-011-0421-x
Lei D., Chen X., Ma X., Zhao B., Larssen T. (2016). Atmospheric S and N deposition relates to increasing riverine transport of S and N in southwest China: Implications for soil acidification. Environ. pollut. (Barking Essex: 1987). 218, 1191–1199. doi: 10.1016/j.envpol.2016.08.075
Li Y., Sun J., Tian D., Wang J., Ha D., Qu Y., et al. (2018). Soil acid cations induced reduction in soil respiration under nitrogen enrichment and soil acidification. Sci. Total Environ. 615, 1535–1546. doi: 10.1016/j.scitotenv.2017.09.131
Li W., Wang W., Sun R., Li M., Liu H., Shi Y., et al. (2023). Influence of nitrogen addition on the functional diversity and biomass of fine roots in warm-temperate and subtropical forests. For. Ecol. Manage. 545, 121309. doi: 10.1016/j.foreco.2023.121309
Li J., Wu B., Luo Z., Lei N., Kuang H., Li Z. (2022). Immobilization of cadmium by mercapto- functionalized palygorskite under stimulated acid rain: Stability performance and micro- ecological response. Environ. pollut. 306, 119400. doi: 10.1016/j.envpol.2022.119400
Ling D. J., Huang Q. C., Ouyang Y. (2010). Impacts of simulated acid rain on soil enzyme activities in a latosol. Ecotoxicol Environ. Saf. 73, 1914–1918. doi: 10.1016/j.ecoenv.2010.07.024
Liu X., Li C., Meng M., Zhai L., Zhang J. (2020). Comparative effects of the recovery from sulfuric and nitric acid rain on the soil enzyme activities and metabolic functions of soil microbial communities. Sci. Total Environ. 714, 136788. doi: 10.1016/j.scitotenv.2020.136788
Liu Z. Q., Li D. F., Zhang J., Saleem M., Zhang Y., Ma R., et al. (2020). Effect of simulated acid rain on soil CO2, CH4 and N2O emissions and microbial communities in an agricultural soil. Geoderma 366, 114222. doi: 10.1016/j.geoderma.2020.114222
Liu X., Ma S., Jia Z., Ramzan M., Meng M., Wang J., et al. (2022). Complex effects of different types of acid rain on root growth of Quercus acutissima and Cunninghamia lanceolata saplings. Ecol. Processes 11, 14. doi: 10.1186/s13717-021-00351-z
Liu Z. Q., Wei H., Shi Z. J., Saleem M., He Y., Zhong J., et al. (2021). Seasonality regulates the effects of acid rain on microbial community in a subtropical agricultural soil of Southern China. Ecotoxicol. Environ. Saf. 224, 112681. doi: 10.1016/j.ecoenv.2021.112681
Liu X., Zhang B., Zhao W., Wang L., Xie D., Huo W., et al. (2017a). Comparative effects of sulfuric and nitric acid rain on litter decomposition and soil microbial community in subtropical plantation of Yangtze River Delta region. Sci. Total Environ. 601-602, 669–678. doi: 10.1016/j.scitotenv.2017.05.151
Liu X., Zhao W., Meng M., Fu Z., Xu L. (2018). Comparative effects of simulated acid rain of different ratios of SO42– to NO3– on fine root in subtropical plantation of China. Sci. Total Environ. 618, 336–346. doi: 10.1016/j.scitotenv.2017.11.073
Liu X., Zhao W., Meng M., Fu Z., Xu L., Zha Y., et al. (2017b). Comparative effects of simulated acid rain of different ratios of SO42 to NO3 on fine root in subtropical plantation of China. Sci. Total Environ. 618, 336–346. doi: 10.1016/j.scitotenv.2017.05.151
Lu M., Cheng S., Fang H., Xu M., Yang Y., Li Y., et al. (2021). Organic nitrogen addition causes decoupling of microbial nitrogen cycles by stimulating gross nitrogen transformation in a temperate forest soil. Geoderma: Int. J. Soil Sci. 385, 114886. doi: 10.1016/j.geoderma.2020.114886
Lu X., Jiang H., Liu J., Zhou G., Zhu Q., Peng C., et al. (2012). Spatial and temporal variability of nitrogen deposition and its impacts on the carbon budget of China. Proc. Environ. Sci. 13, 1997–2030. doi: 10.1016/j.proenv.2012.01.193
Ma Y., Wang B., Zhang R., Gao Y., Zuo Z. (2019). Initial simulated acid rain impacts reactive oxygen species metabolism and photosynthetic abilities in Cinnamonum camphora undergoing high temperature. Ind. Crops Products 135, 352–361. doi: 10.1016/j.indcrop.2019.04.050
Ma M., Zheng B., Xu W., Cao J., Zhou K., Zhao Y. (2023). Trend and interannual variations of reactive nitrogen deposition in China during 2008–2017 and the roles of anthropogenic emissions and meteorological conditions. J. Geophysical Res. D. Atmospheres: JGR. 128, e2022JD037489. doi: 10.1029/2022jd037489
Martinsen V., Mulder J., Austrheim G., Hessen D. O., Mysterud A. (2012). Effects of sheep grazing on availability and leaching of soil nitrogen in low-alpine grasslands. Arctic Antarctic Alpine Res. 44, 67–82. doi: 10.1657/1938-4246-44.1.67
McDonnell T. C., Driscoll C. T., Sullivan T. J., Burns D. A., Baldigo B. P., Shao S., et al. (2021). Regional target loads of atmospheric nitrogen and sulfur deposition for the protection of stream and watershed soil resources of the Adirondack Mountains, USA. Environ. pollut. 281, 117110. doi: 10.1016/j.envpol.2021.117110
Meunier C. L., Gundale M. J., Sanchez I. S., Liess A. (2016). Impact of nitrogen deposition on forest and lake food webs in nitrogen-limited environments. Global Change Biol. 22, 164–179. doi: 10.1111/gcb.12967
Mingxu L., Xin H., Yu S. (2019). Ammonia emission control in China would mitigate haze pollution and nitrogen deposition, but worsen acid rain. Proc. Natl. Acad. Sci. United States America 116, 7760–7765. doi: 10.1073/pnas.1814880116
Patel K. F., Fernandez I. J., Nelson S. J., Malcomb J., Norton S. A. (2020). Contrasting stream nitrate and sulfate response to recovery from experimental watershed acidification. Biogeochemistry 151, 127–138. doi: 10.1007/s10533-020-00711-5
Pregitzer K. S., Burton A. J., Zak D. R., Talhelm A. F. (2008). Simulated chronic nitrogen deposition increases carbon storage in Northern Temperate forests. Global Change Biol. 14, 142–153. doi: 10.1111/j.1365-2486.2007.01465.x
Pu G., Ma X., Du J., Jia Y., Tian X. (2014). Effects of sulfuric, nitric, and mixed acid rain on litter decomposition, soil microbial biomass, and enzyme activities in subtropical forests of China. Appl. Soil Ecol. 79, 1–9. doi: 10.1016/j.apsoil.2013.12.002
Qi X., Mao X., Huang X., Wang D., Yang H. (2020). Tracing the sources of air pollutant emissions embodied in exports in the Yangtze River Delta, China: A four-level perspective. J. Cleaner Production 254, 120155. doi: 10.1016/j.jclepro.2020.120155
Ren X. W., Tang J. Y., Liu J. C., He H. J., Cheng Y. X. (2014). Effects of elevated COand temperature on soil enzymes of seedlings under different nitrogen concentrations. J. Beijing Forestry Univ. 36, 44–53. doi: 10.13332/j.cnki.jbfu.2014.05.016
Shuang-Li H., Hattenschwiler S., Yang J.-J., Sistla S., Wei H.-W., Zhang Z.-W. (2020). Increasing rates of long-term nitrogen deposition consistently increased litter decomposition in a semi-arid grassland. New Phytol. 229, 296–307. doi: 10.1111/nph.16854
Song B., Li Y., Yang L., Shi H., Li L., Bai W., et al. (2023). Soil acidification under long- term N addition decreases the diversity of soil bacteria and fungi and changes their community composition in a semiarid grassland. Microbial Ecol. 85, 221–231. doi: 10.1007/s00248-021-01954-x
Sosa Echeverría R., Alarcón Jiménez A. L., Torres Barrera M., d. C., Sánchez Alvarez P., Granados Hernandez E., et al. (2023). Nitrogen and sulfur compounds in ambient air and in wet atmospheric deposition at Mexico city metropolitan area. Atmospheric Environ. 292, 119411. doi: 10.1016/j.atmosenv.2022.119411
Vassanda C. M., Kundu S., Selladurai R., Saha J. K. (2017). Effect of soil amendments on microbial resilience capacity of acid soil under copper stress. Bull. Environ. Contamination Toxicol. 99, 625–632. doi: 10.1007/s00128-017-2173-8
Wang R. Z., Creamer C. A., Xu Z. W., Jiang Y. (2016). The effects of a 9-year nitrogen and water addition on soil aggregate phosphorus and sulfur availability in a semi-arid grassland. Ecol. Indic 61, 806–814. doi: 10.1016/j.ecolind.2015.10.033
Wang C. Y., Han G. M., Jia Y., Feng X. G., Guo P., Tian X. J. (2011). Response of litter decomposition and related soil enzyme activities to different forms of nitrogen fertilization in a subtropical forest. Ecol. Res. 26, 505–513. doi: 10.1007/s11284-011-0805-8
Wang Q. K., Wang S. L., Liu Y. X. (2008). Responses to N and P fertilization in a young Eucalyptus dunnii plantation: Microbial properties, enzyme activities and dissolved organic matter. Appl. Soil Ecol. 40, 484–490. doi: 10.1016/j.apsoil.2008.07.003
Xiao S., Wang G. G., Tang C., Fang H., Yu X. (2020). Effects of one-year simulated nitrogen and acid deposition on soil respiration in a subtropical plantation in China. Forests 11, 235. doi: 10.3390/f11020235
Xiao-Rong W., Lei P., Hong-Dong P., Jing-Jin Z., Rui-Wen W. (2016). Effects of simulated nitrogen deposition on growth, biomass accumulation and photosynthetic characteristics responses of Quercus seedlings in Mid-subtropics of China. J. Cent. South Univ. Forestry Technol. 36, 79–85. doi: 10.14067/j.cnki.1673-923x.2016.01.01
Xin L., Chong. L., Miaojing M., Bo Z., Jinchi Z. (2020). Comparative effects of the recovery from sulfuric and nitric acid rain on the soil enzyme activities and metabolic functions of soil microbial communities - ScienceDirect. Sci. Total Environ. 714, 136788–136799. doi: 10.1016/j.scitotenv.2020.136788
Xin L., Zhiyuan F., Bo Z., Lu Z., Miaojing M., Jie L., et al. (2018). Effects of sulfuric, nitric, and mixed acid rain on Chinese fir sapling growth in Southern China. Ecotoxicol. Environ. Saf. 160, 154–161. doi: 10.1016/j.ecoenv.2018.04.071
Xu Z., Yu G., Zhang X., Ge J., He N., Wang Q., et al. (2015b). The variations in soil microbial communities, enzyme activities and their relationships with soil organic matter decomposition along the northern slope of Changbai Mountain. Appl. Soil Ecol. 86, 19–29. doi: 10.1016/j.apsoil.2014.09.015
Xu H. Q., Zhang J. E., Ouyang Y., Lin L., Yu J. Y. (2015a). Effects of simulated acid rain on microbial characteristics in a lateritic red soil. Environ. Sci. pollut. Res. Int. 22, 18260–18266. doi: 10.1007/s11356-015-5066-6
Xuejun L., Ying Z., Wenxuan H., Aohan T., Jianlin S. (2013). Enhanced nitrogen deposition over China. Nature 494, 459–459. doi: 10.1038/nature11917
Yin P., Peng Y. J., Song C. C., Liu X. J., Huang J., Lv X. (2021). Effects of simulated acid rain on Growth of Lolium perenne. IOP Conf. Series: Earth Environ. Sci. 714, 22078. doi: 10.1088/1755-1315/714/2/022078
Yu G., Jia Y., He N., Zhu J., Chen Z., Wang Q., et al. (2019). Stabilization of atmospheric nitrogen deposition in China over the past decade. Nat. Geosci. 12, 424–429. doi: 10.1038/s41561-019-0352-4
Yu Z., Zhang Q., Kraus T. E. C., Dahlgren R. A., Anastasio C., Zasoski R. J. (2002). Contribution of amino compounds to dissolved organic nitrogen in forest soils. Biogeochemistry 61, 173–198. doi: 10.1023/A:1020221528515
Zhang T., Song B., Han G., Zhao H., Hu Q., Zhao Y., et al. (2023). Effects of coastal wetland reclamation on soil organic carbon, total nitrogen, and total phosphorus in China: A meta- analysis. Land Degradation And Dev. 34, 3340–3349. doi: 10.1002/ldr.4687
Zhang J., Yang H., Wang J., Tian D., Niu S. (2019). Soil and climate determine differential responses of soil respiration to nitrogen and acid deposition along a forest transect. Eur. J. Soil Biol. 93, 103097–103106. doi: 10.1016/j.ejsobi.2019.103097
Zheng S., Bian H., Quan Q., Xu L., He N. (2018). Effect of nitrogen and acid deposition on soil respiration in a temperate forest in China. Geoderma 329, 82–90. doi: 10.1016/j.geoderma.2018.05.022
Zheng Y., Wang Y., Zheng Y., Li Y. (2022). Effects of simulated acid rain on soil enzyme activity and related chemical indexes in woodlands. Forests 13, 860. doi: 10.3390/f13060860
Zhou F., Cui J., Zhou J., Yang J., Li Y., Leng Q., et al. (2018). Increasing atmospheric deposition nitrogen and ammonium reduced microbial activity and changed the bacterial community composition of red paddy soil. Sci. Total Environ. 633, 776–784. doi: 10.1016/j.scitotenv.2018.03.217
Ziqiang L., Zhaoji S., Hui W., Jiaen Z. (2021). Acid rain reduces soil CO2 emission and promotes soil organic carbon accumulation in association with decreasing the biomass and biological activity of ecosystems: A meta-analysis. Catena 208, 105714. doi: 10.1016/j.catena.2021.105714
Keywords: subtropical forest, sulfuric acid rain, nitric acid rain, nitrogen deposition, soil acidification
Citation: Ding Y, Sun L, Li C, Chen M, Zhou Y, Meng M, Li Z, Zhang J and Liu X (2024) Effects of short-term simulated acid rain and nitrogen deposition on soil nutrients and enzyme activities in Cunninghamia lanceolata plantation. Front. Ecol. Evol. 12:1365954. doi: 10.3389/fevo.2024.1365954
Received: 05 January 2024; Accepted: 21 March 2024;
Published: 11 April 2024.
Edited by:
Ming Jiang, Chinese Academy of Sciences (CAS), ChinaReviewed by:
Aliya Baidourela, Xinjiang Agricultural University, ChinaZiqiang Liu, South China Agricultural University, China
Copyright © 2024 Ding, Sun, Li, Chen, Zhou, Meng, Li, Zhang and Liu. This is an open-access article distributed under the terms of the Creative Commons Attribution License (CC BY). The use, distribution or reproduction in other forums is permitted, provided the original author(s) and the copyright owner(s) are credited and that the original publication in this journal is cited, in accordance with accepted academic practice. No use, distribution or reproduction is permitted which does not comply with these terms.
*Correspondence: Xin Liu, liuxinswc@njfu.edu.cn