Developmental plasticity to desiccation risk in tadpoles of a tropical inselberg specialist
- 1Department of Biological Sciences, Universidad de Los Andes, Bogotá, Colombia
- 2Ecology, Evolution, and Development Group, Doñana Biological Station, Consejo Superior de Investigaciones Cientificas, Seville, Spain
- 3Laboratory of Biodiversity and Cloud Forests Conservancy, Bioconservancy.org, Jardín, Colombia
Developmental plasticity evolves in heterogeneous environmental conditions as long as individuals can accurately perceive them. A paradigmatic example of developmental plasticity is the ability of amphibian larvae to alter growth and development in response to the risk of pond drying. Such responses are often found in amphibian species breeding in highly dynamic water bodies with high desiccation risk. The inselbergs of the Guianan Shield are rocky outcrops with extremely high and fluctuating temperatures and a marked seasonality in precipitation. During the rainy season, eroded depressions form precipitation-dependent pools with a high and variable risk of desiccation within the timeframe of a few days. The frog Leptodactylus lithonaetes specializes in breeding in this extreme environment, and its tadpoles are thus forced to cope with desiccation risk by adjusting their developmental trajectories and physiological performance. We experimentally assessed the effect of different levels of desiccation risk, under controlled temperature conditions, on developmental rate, growth, and temperature-dependent locomotor performance in tadpoles of Leptodactylus lithonaetes. We did not find an effect of desiccation risk on developmental rate, but under simulated drying conditions, tadpoles showed larger body size, greater body mass, and enhanced locomotor performance compared to constant (high or low) water levels. These results suggest that drying pools offer cues that trigger developmental and behavioral changes in these tadpoles, enabling them to enhance growth over a short time span without accelerating development. We discuss the potential compensatory mechanisms behind these responses and highlight the need for further investigations in species with semiterrestrial life histories in extreme environments.
1 Introduction
The ability to cope with changing environmental conditions is pervasive across taxa. Most organisms possess a certain degree of developmental plasticity that allows them to persist over varying conditions by adjusting their morphology, physiology, and behavior (Kingsolver and Huey, 1998; West-Eberhard, 2003). When environmental cues are reliable and phenotypic responses are not limited by costs or constraints, adaptive plasticity is expected to evolve so that the expressed phenotypes will predictably depend on environmental conditions (Schlichting and Pigliucci, 1998; Snell-Rood and Ehlman, 2021). In contrast, less predictable environments or less reliable environmental cues are likely to result in bet-hedging strategies (Schlichting and Pigliucci, 1998). In that case, phenotypic variation will be found within a single environment and associated rather to variation in genotypes, independently of environmental input (Seger and Brockmann, 1987; DeWitt and Langerhans, 2004).
Phenotypic plasticity has been widely documented in larval amphibians with remarkable examples of plastic responses to a wide array of biotic and abiotic environmental factors, including the risk of desiccation (e.g., Newman, 1989; Richter-Boix et al., 2011; Boelter et al., 2020; Lent and Babbitt, 2020). The distribution of larval amphibians among pools with different hydroperiods creates a gradient of desiccation risk (Wellborn et al., 1996; Babbitt et al., 2003), where tadpoles that develop in temporary pools are more vulnerable to desiccation than tadpoles developing in permanent pools (Skelly, 1996). Under these conditions, tadpoles may reveal phenotypic plasticity generally consisting in the shortening of developmental time (Tejedo et al., 2010; Richter-Boix et al., 2011; Gomez-Mestre et al., 2013) and reduced size at metamorphosis (Kulkarni et al., 2011; Richter-Boix et al., 2011). These responses result from alterations in differentiation rate, growth rate, or both (Gomez-Mestre et al., 2010). Accelerated development increases the survival odds of amphibian larvae in the short term by allowing them to escape a deteriorating environment, but the resulting smaller body size reduces the survival odds during the juvenile stage (Gomez-Mestre and Tejedo, 2003; Cabrera-Guzmán et al., 2013).
Responses to desiccation risk in anurans have been recorded primarily in temperate species with aquatic oviposition and development of aquatic larvae, which generally breed in permanent pools or in pools that predictably dry up annually (Richter-Boix et al., 2011). In contrast, tropical anurans present far more diverse reproductive modes (Duellman and Trueb, 1994; Gomez-Mestre et al., 2012; Liedtke et al., 2022), some of which allow them to cope with much less predictable hydroperiods in some water bodies (Goldberg et al., 2022). For example, it is common to find species with entirely terrestrial development, or with tadpoles developing in small phytotelmata, or in foam nests (Nunes-de-Almeida et al., 2021), all of which can represent a risk of desiccation to the larvae. Compared to temperate species, less is known regarding the plastic responses of tropical species with complex oviposition strategies (Schalk, 2016).
Orinoquian Guiana Shield outcrops, also known as inselbergs, are rock formations that rise above the surrounding vegetation (Bremer and Sander, 2000). Located in a region with marked seasonality in precipitation (Fernández et al., 2009), these ecosystems meet the criteria that define an extreme environment. They are characterized by low relative air humidity, which frequently drops below 20% throughout the day (Porembski, 2007), and maximal temperatures that peak over 60°C when measured directly on the rock (Amézquita et al., 2020). During the rainy season, eroded depressions on the outcrops form shallow precipitation-dependent rocky pools that fill with rainfall and become the habitat of larvae of a few frog species (Figure 1A; Lasso and Ceñaris, 2018; Amézquita et al., 2020). Due to their shallowness, exceptionally high temperatures, and high evaporation rate on the rock surface, the pools almost continuously change in size and depth, often drying within hours or days (Jocque et al., 2010; Lasso et al., 2014). The risk of overheating or desiccation depends on the size of the pool, and, therefore, the array of rocky pools, widely variable in size, represents a dynamic mosaic of habitats that expose larvae to high and variable mortality risks (Jocque et al., 2010; Amézquita et al., 2020). Indeed, this system is an ideal natural system for understanding how anuran larvae cope with such heterogeneous habitats through space and time.
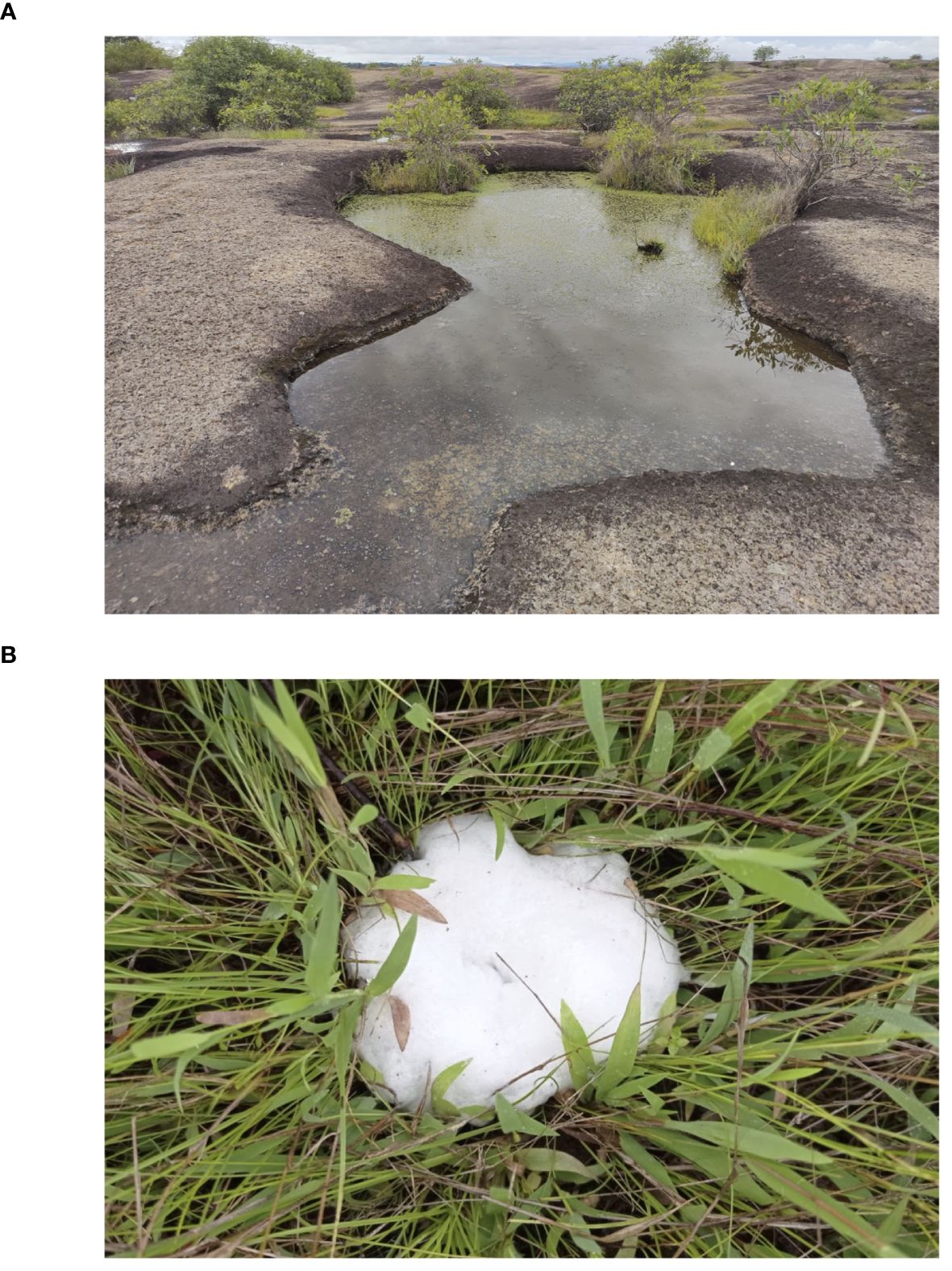
Figure 1 (A) Representative photograph of the shallow rocky pool inhabited by Leptodactylus lithonaetes tadpoles on the inselbergs of the Orinoquian region, Colombia. (B) Representative foam nests from Leptodactylus lithonaetes.
Leptodactylus lithonaetes is an outcrop-adapted tropical anuran (Heyer and Heyer, 2001; De Sá et al., 2014), discontinuously distributed across Guianan inselbergs (Fernández et al., 2009), mainly within the Orinoco River basin. Reproduction in this species is restricted to rocky pools (Figure 1A), where amplecting pairs build foam nests amid the vegetation, in which embryos develop (Figure 1B; De Sá et al., 2014). Tadpoles of L. lithonaetes have semi-terrestrial habits (Heyer, 1995; Heyer and Heyer, 2001): they can move and survive out of the water, crossing over thin layers of water or descending thin trickles of water flowing between nearby pools (Rivero, 1961; pes. obs.) (Figure 2). As inhabitants of this extreme environment, tadpoles of Leptodactylus lithonaetes must cope with desiccating puddles with widely fluctuating water temperatures, often exceeding their critical thermal maxima (Beltrán et al., 2019). Indeed, it has been recorded that following the warmest days, there is a substantial number of dead larvae in these pools (Amézquita et al., 2020).
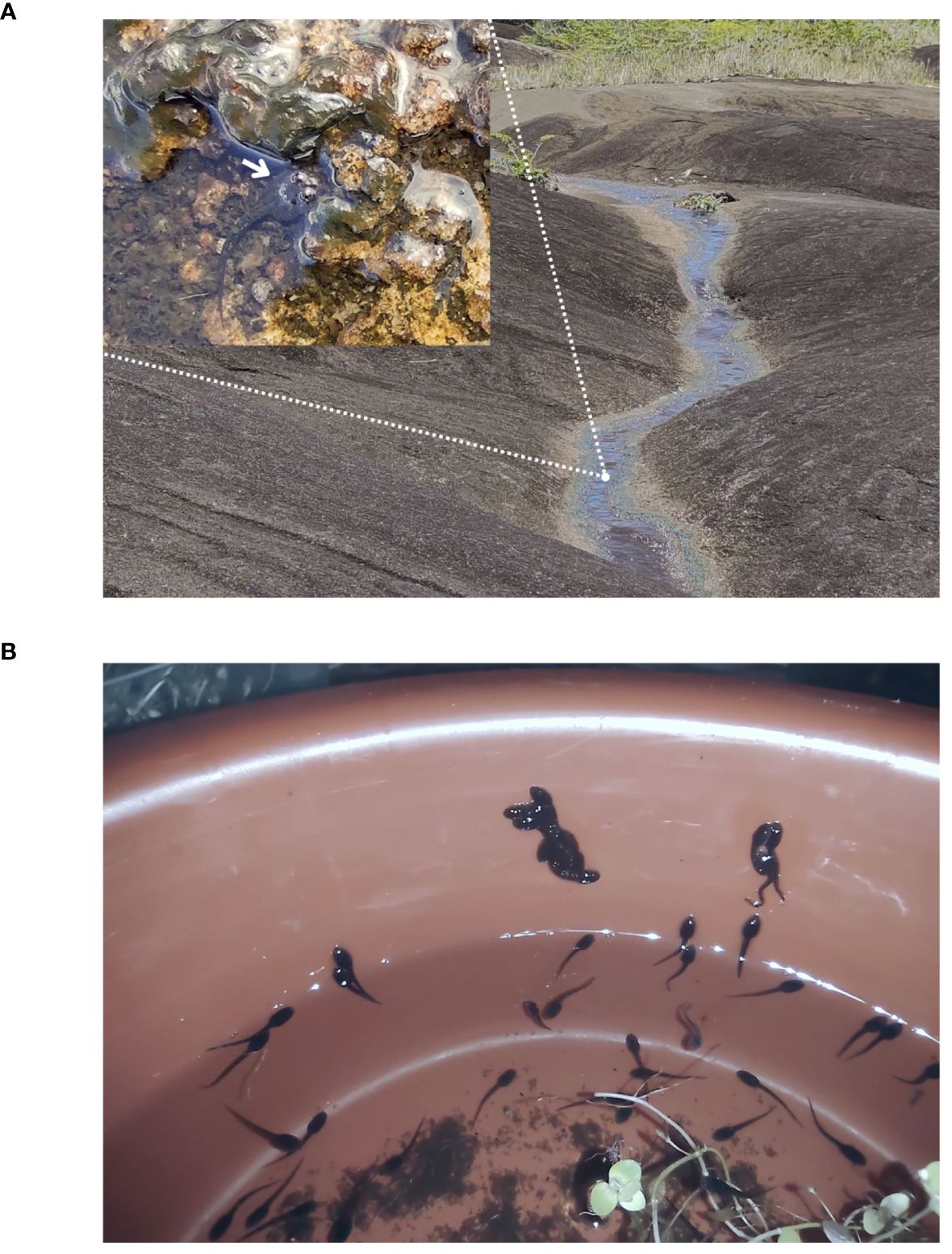
Figure 2 (A) Tadpole of Leptodactylus lithonaetes in a thin trickle of water, with eyes and part of the dorsum outside the water. (B) Tadpoles in laboratory conditions, climbing out of the water in a plastic container.
We thus hypothesized that whether tadpoles of Leptodactylus lithonaetes could respond to the risk of desiccation through plastic responses, accelerating their development at the expense of reduced body size. We expected that tadpoles would accelerate their development both at low and at decreasing water levels, enhancing differentiation rate over growth rate. Similarly, we expected that developmental responses to risk of pond drying could constrain their temperature-dependent locomotor performance, whether through its effect on their body size or physiology. Locomotor performance is known to depend strongly on temperature, although the degree of dependence may decrease in thermally fluctuating environments (Navas, 2006). We expected that tadpoles facing desiccation risk would present poorer locomotor performances, especially at high temperatures. Alternatively, if bet-hedging strategies had evolved in this species due to high unpredictability and lack of reliable cues, we would expect phenotypic variation to vary among clutches with low or no association with environmental conditions. To test these hypotheses, we experimentally exposed L. lithonaetes tadpoles to three pool drying regimes and, after 21 days of exposure, we evaluated tadpole size and their locomotor performance across a wide range of temperatures.
2 Materials and methods
2.1 Study system
We carried out this study at the Bojonawi Nature Reserve, located on the west bank of the Orinoco River, at the western end of the Guianan Shield, about 9.5 km south of Puerto Carreño (Vichada, Colombia) (Figure 3). The reserve protects ancient rocky outcrops of igneometamorphic origin (Bonilla-Pérez et al., 2013), savannas and gallery forests. The climatic conditions in this region align with a tropical savanna climate, characterized by warm and semi-humid conditions. The mean annual temperatures average 28°C, while mean annual precipitation reaches 2300 mm, with a monomodal distribution primarily between May and August, and a marked dry season prevailing from December to March when monthly rainfall drops to ca. 23 mm (Pacheco and León, 2001; Morales-Betancourt and Lasso, 2020).
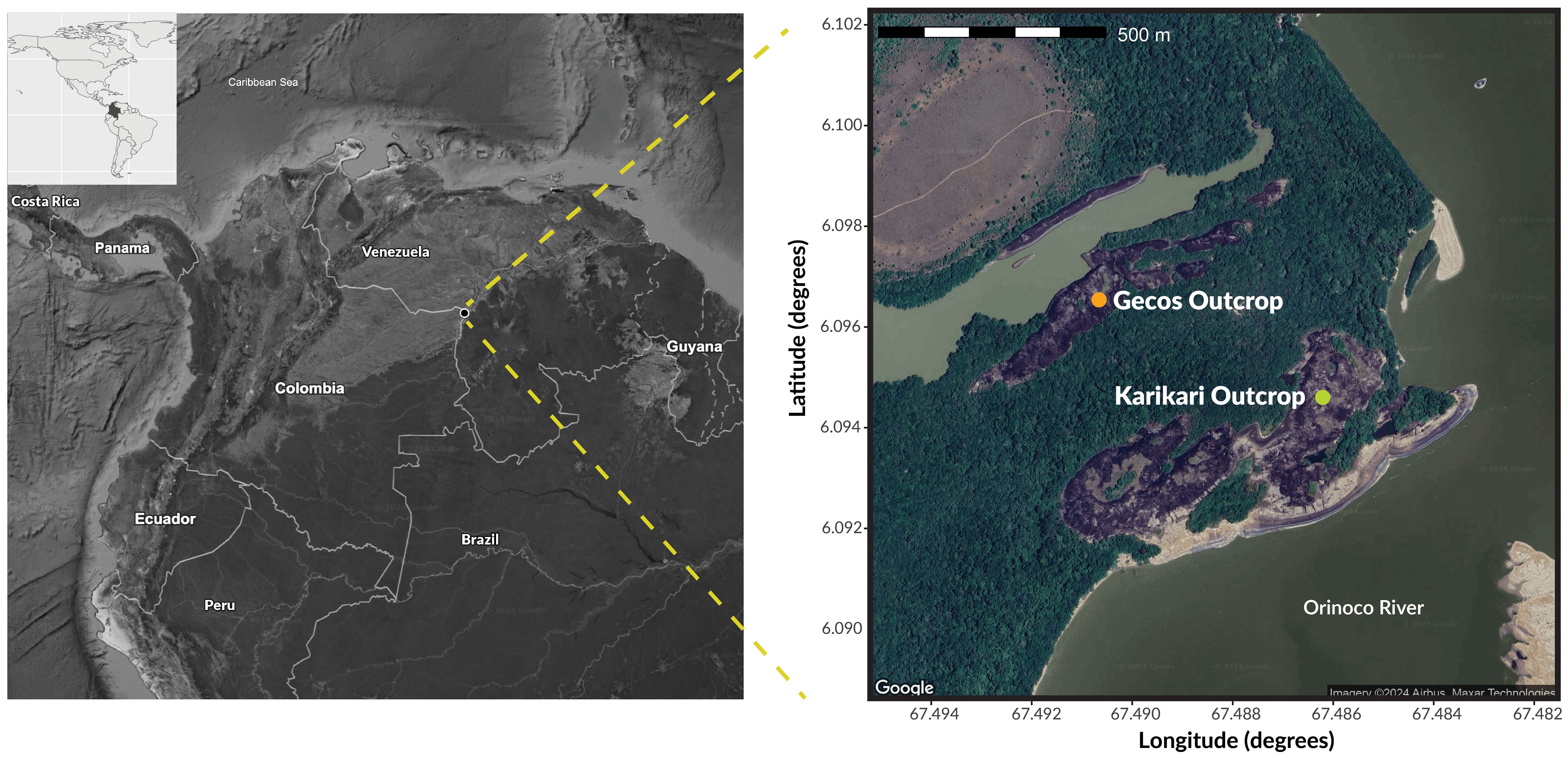
Figure 3 Geographic location of the study area. Right map shows the two rocky outcrops where we obtained the seven clutches used in this study.
During the rainy season, the frog Leptodactylus lithonaetes breeds in water-filled pools created by the weathering and erosion of the rock surface (Amézquita et al., 2020). To characterize these ponds, we conducted a field survey on 50 pools located in two rocky outcrops within Bojonawi Natural Reserve (Figure 3). This survey was carried out between July and August 2019, when ponds were filled. In each pond, we measured the perimeter, vegetation cover, water depth, and daily temperature variation. The perimeter was measured using an odometer encircling the outer edge of the pond. The percentage of vegetation cover in each pond was estimated using two photos taken from opposite locations and at similar distances from the edge of the pond; we calculated the values of vegetation cover in each photo using ImageJ® (Abramoff et al., 2004), and we averaged the values obtained to avoid underestimating the vegetation cover depending on the perspective of the photographs. The water depth was measured in each pond once a day for five days, with a ruler at four equidistant points and at the point of maximum water depth. From these data, we obtained the average depth of the pond and the maximum depth. The temperature was recorded using a data logger (HOBO Pendant® MX Temp) placed in the deepest area within each pool, recording temperature every 15 minutes for 24 hours for eight days.
Pools in these outcrops are shallow, with depths between 0 and 22.6 cm. Although none of the pools dried up entirely during the months we surveyed them, 68% of them reduced their area substantially. Pools also showed considerable variation in their perimeter (between 4.95 and 171.90 m) and vegetation cover (between 6.88 and 92.78%), implying spatial and temporal heterogeneity in the abiotic conditions. We recorded an average temperature of 28.98 ± 3.14°C and a temperature range between 22.78 and 59.97°C. We also found that 40% of the monitored pools reached maximum temperatures above 42.3°C, the critical thermal maximum reported for this species (Beltrán et al., 2019).
Leptodactylus lithonaetes is considered a rocky outcrop specialist (Heyer and Heyer, 2001). Consequently, its distribution is geographically discontinuous, as it only appears associated with the inselbergs in the Colombian departments of Amazonas, Guainía, Vaupés, and Vichada, as well as in the Venezuelan States of Amazonas, Apure, and Bolívar (Heyer, 1995; Fernández et al., 2009). The reproductive period is associated with the rainy season, between May and August (Beltrán et al., 2019). During oviposition, males beat with their hind limbs the oviductal secretions released by females to build foam nests placed amid vegetation (Figure 1B; De Sá et al., 2014). Clutch size varies between 62 and 163 eggs, and the larval period is between 45 and 60 days (ADM personal obs.). As members of the semiterrestrial guild (Altig and Johnston, 1989), tadpoles have flattened and elongated bodies, having almost no veil with low and thin tail fins (Heyer and Heyer, 2001).
2.2 Experimental setup
We collected seven foam nests of Leptodactylus lithonaetes in rocky pools of the Bojonawi Nature Reserve and brought them back to the laboratory inside the Reserve. Each nest was kept in a plastic container until all tadpoles hatched, staying within the foam. Two weeks after the first eggs hatched, we placed 10 tadpoles per clutch (Gosner stage between 25 and 30, Gosner, 1960) in 1.5 L rectangular plastic containers. Larval densities of L. lithonaetes in these rock pools are highly fluctuating, and the density used in this experiment is well within the range observed. Larval density (number of tadpoles per liter) was therefore increased both in the drying treatment and the low water level treatment with respect to the high-water level treatment, as is also the case in natural ponds. Each container was assigned to one of three experimental water level treatments: 1) high and constant water level, in which the water volume was always kept at 1 L; 2) low and constant water level, in which the water volume was always kept at 0.3 L, and 3) drying water treatment, in which the water volume was kept at 1 L for seven days, then lowered to 0.5 L, and seven days later lowered again to 0.3 L, then kept at that volume until the end of the experiment (Figure 4). At the beginning of the experiment, we added to each container 2 cm of substrate and aquatic plants taken from natural pools and aquatic plants, to recreate natural conditions. The containers were filled with water from the Orinoco River to a volume depending on the treatment. During the experiment, the water temperature was 26.54 ± 0.21°C and did not differ significantly among treatments despite the differences in water level (F=4.655, df=2, p=0.07).
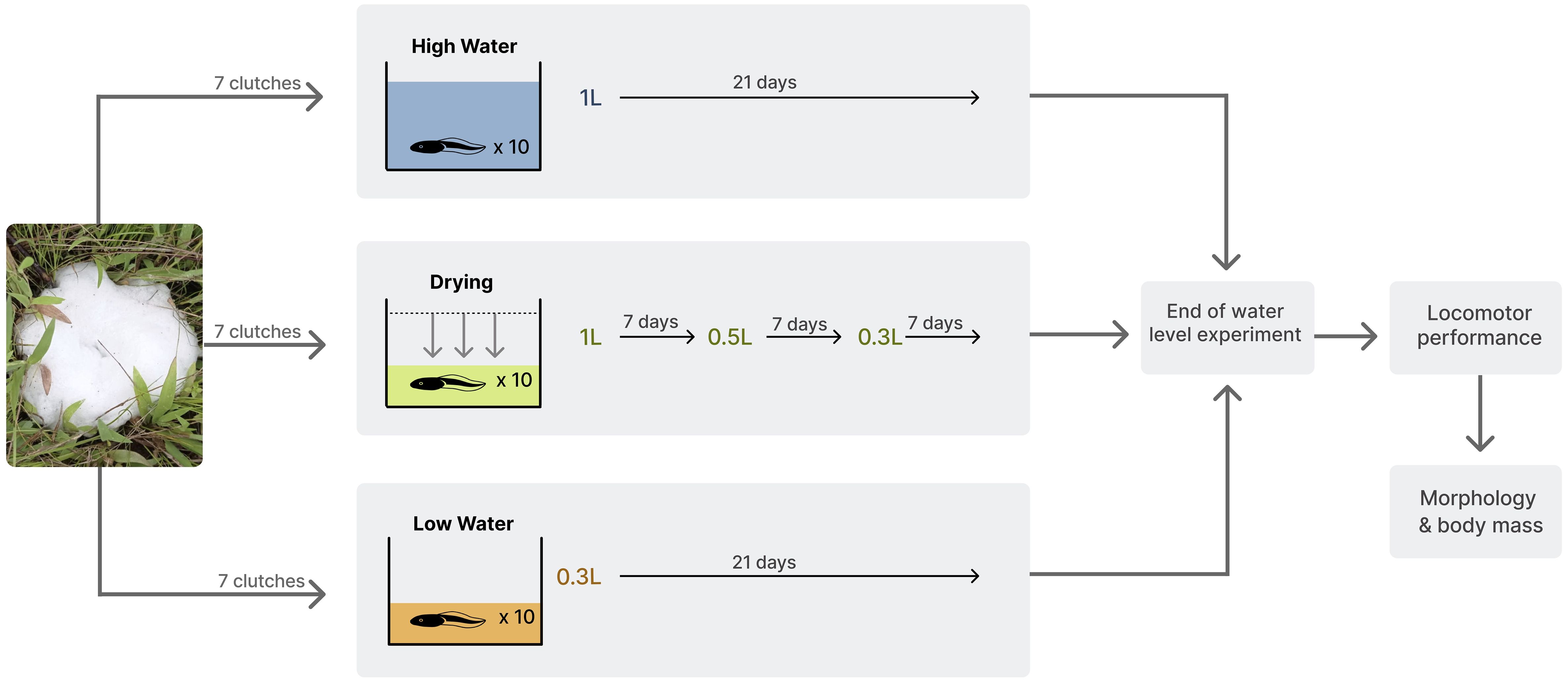
Figure 4 Experimental design and sample distribution. The experiment was carried out with seven clutches. Embryos from each clutch were haphazardly split up into three different treatments: High water, Dry down, and Low water for a total of 21 containers. Each container held ten tadpoles. At the end of the experiments, locomotor performance was assessed on five tadpoles from each container, whereas weight and morphometric measurements were taken on all tadpoles.
The experiment lasted 21 days. Afterwards, we weighed all tadpoles on a high precision scale (digital balance Pesola® PPS200) to the nearest 0.01 g, after gently blotting them dry to remove excess water. To determine Gosner’s developmental stage (Gosner, 1960) and to take morphometric measurements, we placed each tadpole sequentially in a photo booth, i.e. a plexiglass box with a sliding back wall with a millimetric scale to position the tadpoles in an upright position, and hence facilitate taking lateral pictures of the animals without requiring anesthesia. Pictures were taken with a Nikon® Coolpix P600.
2.3 Aquatic locomotor performance tests
We randomly took five tadpoles per container to assess their locomotor performance across a temperature range. At the end of each test, tadpoles were euthanized by immersion in a lethal concentration of tricaine methane-sulfonate (MS-222), following the protocol proposed by the American Association of Veterinary Physicians (Ross and Ross, 2009).
We conducted all locomotor performance assays between 9:00 and 16:00. Each tadpole was evaluated for two minutes at a single temperature: 25, 30, 33, 37, or 40°C. For the assay, each individual was placed in a round plastic aquarium 35 cm in diameter with a length scale at the bottom. To induce tadpoles to swim, we gently prodded them with a fine wire on the anterior part of the head. Every tadpole was filmed until it made at least five attempts to swim rapidly; in all cases, this occurred within the first minute. All trials were recorded from the top of the aquarium with a Nikon® Coolpix P600 video camera fixed to a supporting structure.
We estimated the maximum acceleration performance, a relevant metric of anuran locomotion, correlated with an increased ability to evade predators and linked to fitness (Huey and Stevenson, 1979; Wilson and Franklin, 2000). To estimate the maximum acceleration (m/s2), we tracked the recorded tadpole movements with the software Tracker® (http://www.opensourcephysics.org, Open-Source Physics, Boston, MA, USA). Then, the fastest sequence analyzed for each individual was used as a proxy for maximum performance.
2.4 Size measurements
We obtained morphological measurements from the digital pictures of each tadpole using Image J (Schneider et al., 2012; v.1.52k; https://imagej.nih.gov/ij/). We measured body length, tail length, body height, tail height, and tail muscle perimeter. We measured each tadpole three times to assess repeatability, which was estimated using mixed null models without independent variables. In these models, individual was entered as random factor, and each morphological measurement was a dependent variable. We calculated the intraclass correlation coefficient (ICC) from this model using the performance package (Lüdecke et al., 2021), which allows for determining the stability of the measurement (Finch et al., 2019). For all variables, the ICC values were >0.90, indicating high repeatability between measures. Therefore, we used the median of the three measurements for each trait in subsequent analyses.
2.5 Statistical analyses
We conducted all statistical analyses in R (Version 4.0.3, R Development Core Team, 2020).
Reduction of morphological variables – For each variable, we checked normality using Shapiro tests in the rstatix package (Kassambara, 2020). We also tested for homoscedasticity across treatments using the Levene test in the car package (Fox et al., 2012). All variables were log-transformed to ensure parametric assumptions were met.
To reduce the dimensionality of the five morphological measures, we used a principal component analysis using the Factominer package (Husson et al., 2016). Principal component 1 explained 82.90% of the total variation and positively correlated with all five variables, with correlation coefficients between 0.72 and 0.98. This PC1 was therefore interpreted as “body size”. In order to facilitate the interpretation, the analysis involved a Varimax rotation of the component that was retained. We included this PC1 “body size” for further analyses.
2.5.1 Developmental responses to water level
To determine the effect of water level treatments on the acceleration of development, we ran a mixed ordinal logistic regression model (ordinal package, Christensen, 2018). As a measure of tadpole developmental rate, we used the Gosner staging table (Gosner, 1960) to determine the developmental stage of each individual. In our model, this variable was the dependent variable, and water level (high water, low water, drying) was the independent fixed factor. We added clutch and container as random factors, and then tested the effect of ‘water level’ through a likelihood ratio test with the anova function in the lmerTest package (Kuznetsova et al., 2017).
2.5.2 Body size and weight responses to water level
To determine the effect of water level on tadpole size and weight, we ran two linear mixed models (lme4 package, Bates et al., 2015). In the first model, body size was the dependent variable; in the second model, we included weight as the dependent variable. In both models, we included water level as a fixed factor, adding clutch and container as random factors. We used the anova function in the lmerTest package (Kuznetsova et al., 2017) to obtain associated p-values. Post hoc comparisons among groups were made using the least significant difference (LSD) test in the emmeans package (Lenth, 2022). We calculated the intraclass correlation coefficient and tested the model assumptions of homoscedasticity of the error terms and the normality of the error term’s distribution using the lme4 package (Bates et al., 2015).
2.5.3 Locomotor performance responses to water level
Locomotor performance depends on temperature, and such effect has been shown to be curvilinear in most tested ectotherms. Therefore, we used a generalized mixed additive model (GAM) to determine the effect of water level and temperature on tadpole swimming performance (mgcv package, Wood, 2011). We analyzed variation in tadpole maximum acceleration, testing whether it depended on water level (high water, low water, drying) and temperature, included in the model as fixed factors, and total tadpole length included as a covariate. In this model, clutch was incorporated as a random factor. We tested the significance of the interaction between temperature and treatment, but it was non-significant, and we excluded it from the model (F=0.692, p=0.503, df=2). We calculated LSD posthoc tests to compare among levels of the water level factor with the emmeans package (Lenth, 2022).
2.6 Ethical considerations
Collection of Leptodactylus lithonaetes nests from a natural population was approved by the National Authority for Environmental Licensing (ANLA, Institutional permit no. 1177 9/10/2014 - IDB 0359, Project PR.6.2022.9267). The experimental design and procedures were approved by the Ethical Committee of Los Andes University.
3 Results
3.1 Developmental responses to water level
Tadpole survival was very high throughout the experiment (98.57%), and we found no significant differences across water level treatments (χ2 = 0.147, df=2, p=0.929). The experimental manipulation of water levels did not affect the developmental rate of tadpoles, as the developmental stage at the end of the experiment did not differ among treatments (χ2 = 2.76, df=2, p=0.252, Table 1). The median developmental stage was Gosner stage 29, and the mode stage was 27.

Table 1 Mean ± standard deviation of measurements obtained in tadpoles of Leptodactylus lithonaetes exposed to three water level conditions for 21 days.
3.2 Variation in body size among water levels
The experimental manipulation of water levels affected tadpole size (F=5.921, df=2, p=0.016; Figure 5A, Table 1). Tadpoles subjected to the drying treatment were, on average, 10.51% larger than tadpoles from the low-water treatment (p=0.006), and 7.83% larger than tadpoles from the high-water treatment (p=0.035). We did not find differences in body size between high and low water levels (p=0.352). The model’s total explanatory power was substantial (conditional R2 = 0.469).
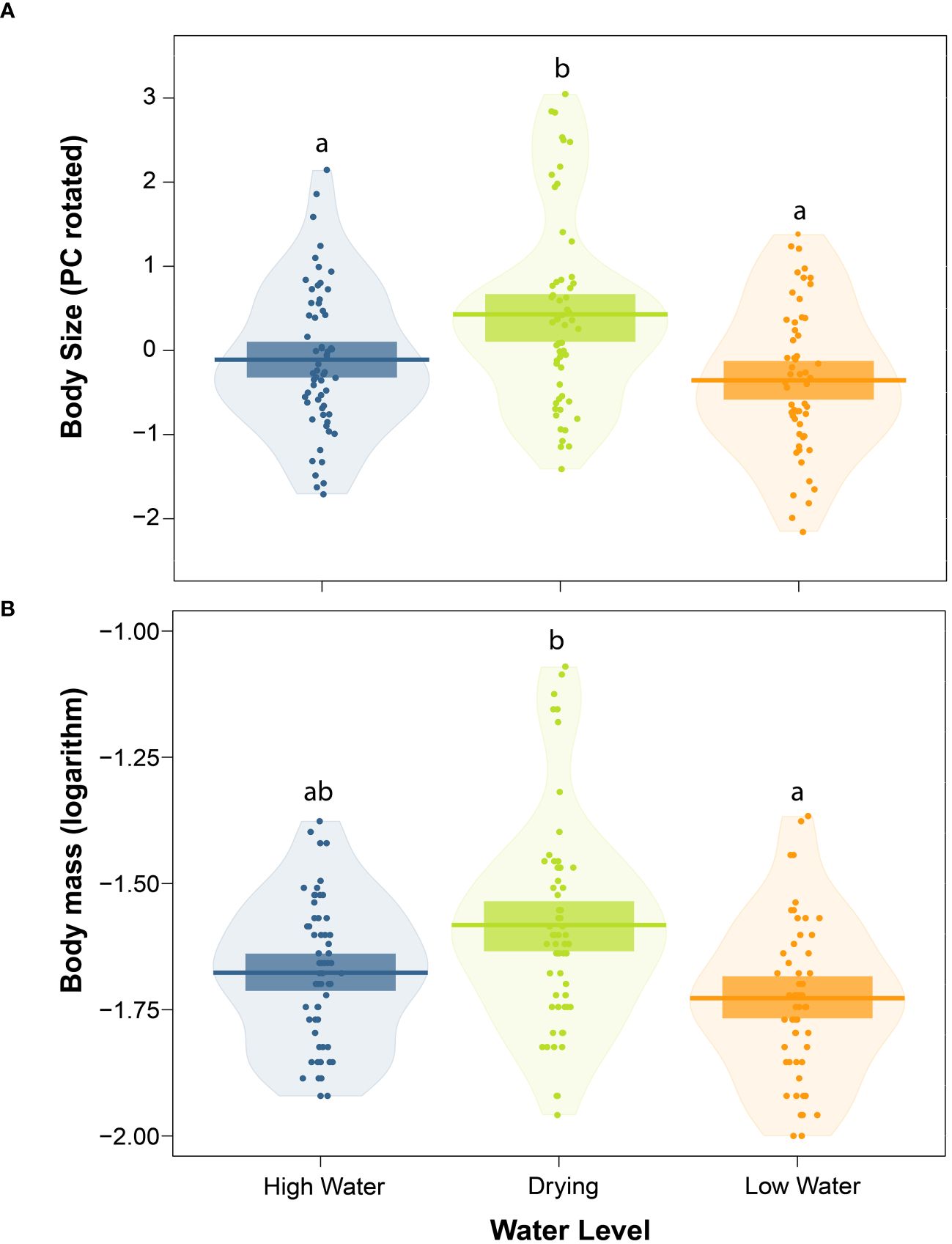
Figure 5 Effect of water level treatment on body size (A) and body mass (B) in Leptodactylus lithonaetes tadpoles. Lines represent the mean for each treatment. Dark boxes show the 95% confidence interval of the mean. In each panel, water level treatments denoted by different letters are statistically different (p < 0.05).
3.3 Variation in tadpole body mass among water levels
The simulated pool-drying treatment also affected body mass in larvae of L. lithonaetes (F=4.243, df=2, p=0.040, Figure 5B, Table 1). Tadpoles subjected to the drying treatment had, on average, 32.54% greater body mass than tadpoles from the low-water treatment (p=0.015) and 24.75% greater body mass than tadpoles from the high-water treatment (p=0.070). We did not find differences in body mass between high and low water levels (p=0.374). The model’s total explanatory power was substantial (conditional R2 = 0.646).
3.4 Effects of water level and temperature on locomotor performance
As expected, water temperature affected maximum acceleration, peaking around 33°C (F=9.177, p<0.001, edf=2.517). Variation in water level significantly affected maximum acceleration too (p=0.023, df=2, F=3.922, Figure 6, Table 1). Tadpoles subjected to simulated pool-drying showed greater maximum acceleration than tadpoles from the other treatments (Figure 6). On average, the maximum swimming acceleration in tadpoles from the drying treatment was 20% higher than in high-water tadpoles (p=0.01) and 17% higher than in low-water ones (p=0.028). We did not find differences in maximum acceleration between high and low water levels (p=0.668). We observed a consistent association between body size and maximum acceleration across all water levels, such that larger tadpoles were capable of greater acceleration (R2 = 0.334, F=7.410, df=1, p=0.008; Table 2).
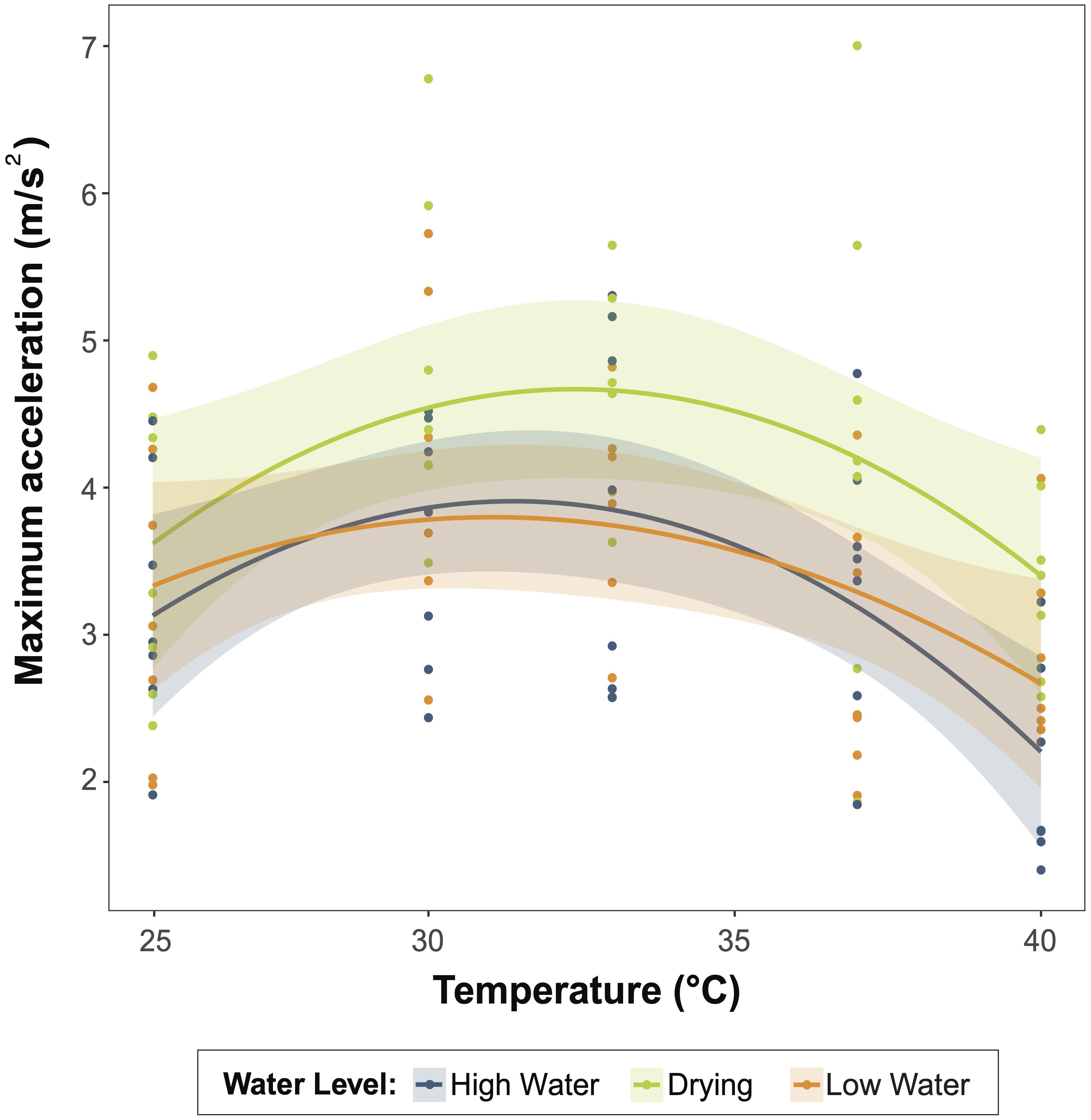
Figure 6 The effect of water level treatment on locomotor performance in Leptodactylus lithonaetes tadpoles. The lines represent the non-linear effects of temperature on maximum acceleration, and the shaded bands denote the 95% confidence interval. The color denotes the experimental treatment.
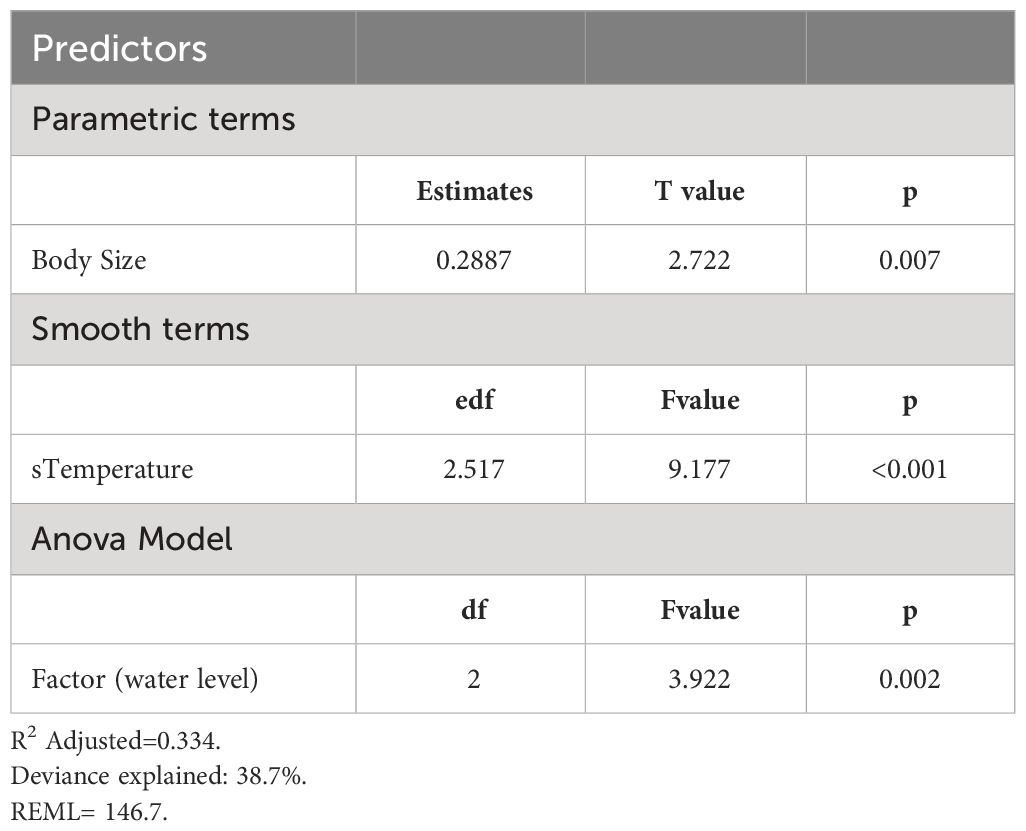
Table 2 Results of the generalized additive mixed model used to test the effect of water level treatment, temperature, and body size on maximum acceleration in tadpoles of Leptodactylus lithonaetes.
4 Discussion
We evaluated possible larval responses to risk of pond drying in tadpoles of the anuran Leptodactylus lithonaetes, a specialist of the Orinoco outcrop rock pools. When exposed to low or decreasing water levels, tadpoles from many amphibian species reduce their growth rate or increase their differentiation rate, hence metamorphosing at a smaller size (Richter-Boix et al., 2011; Gomez-Mestre et al., 2013). Our results with L. lithonaetes contradict this expectation. We also expected that L. lithonaetes tadpoles in low water or drying conditions would show inferior locomotor performance along a range of tested temperatures due to reduced size. Our results also contradicted our initial expectations in this regard. We found that after 21 days of exposure to water level treatments, there were no differences in larval development among treatments, but tadpoles subjected to the drying treatment had reached a bigger size and greater body mass. This effect seems to be directly attributable to the decreased water levels and not to the concomitant increase in larval density in the pond drying treatment, since tadpoles at a similarly high density in the low-water treatment grew similarly to the ones in constant high-water. Additionally, tadpoles from the drying treatment had higher locomotion performance than tadpoles from the constant high and constant low-water treatments in all tested temperatures. Tadpoles in either one of the constant water levels (high or low) grew at a similar rate and showed similar locomotor performances.
In ephemeral pools, most tadpoles exposed to decreasing water levels exhibit a developmental shift, prioritizing metamorphosis over growth to minimize the risk of desiccation (Richter-Boix et al., 2011; Gomez-Mestre et al., 2013). Few species remain unresponsive to varying hydroperiod lengths during larval development (Leips et al., 2000; Cook et al., 2013). Similarly, Goldberg et al. (2022) found that tadpoles of the Yungas Red-belly Toad (Melanophryniscus rubriventris) did not respond to reduced water levels. This species resides in the subtropical montane forests of northwestern Argentina, characterized by short and unpredictable pool durations. The authors argued that this inherent unpredictability contributes to the absence of phenotypic plasticity observed in M. rubriventris. The tadpoles of Leptodactylus lithonaetes inhabit ephemeral pools with low predictability, where pools last from days to months. In contrast to M. rubriventris, tadpoles of L. lithonaetes exhibited phenotypic plasticity in response to decreasing water conditions, indicating that variation in water level of these rock pools is a reliable cue indicating risk of desiccation and hence enabling the evolution of developmental plasticity in this species. Additionally, Schalk (2016) proposed that, in temporary pools, tropical species with terrestrial or semiterrestrial reproductive modes could exhibit phenotypic plasticity as a response to desiccation risk because, at least during part of their early development, constraints of pool hydroperiod are relaxed. This explanation seems plausible for L. lithonaetes, whose eggs are deposited inside rocky pools in foam nests that protect embryos during the first days against unpredictable pool drying (De Sá et al., 2014).
Here we evaluated tadpoles’ size after 21 days of experimental conditions, which is in the middle of the larval phase of the species. Although we couldn’t assess the time or size at metamorphosis, it is clear that tadpoles of L. lithonaetes in the drying treatment were bigger and heavier compared to tadpoles reared in constant low or high-water levels. O’Regan et al. (2014) found similar results: tadpoles of the spadefoot Spea intermontana under desiccation risk grew more at day 31 of development (in the middle of developmental time) than tadpoles in constant water levels. However, at the end of their experiment, O’Regan et al. (2014) found that animals were smaller when metamorphosed. According to the Wilbur-Collins model, a specific size threshold needs to be attained prior to the initiation of metamorphosis (Wilbur and Collins, 1973). Usually, in temporary pools, organisms exhibiting accelerated growth rates will achieve this size threshold more rapidly, enabling them to undergo metamorphosis earlier and preventing death by desiccation (Wilbur and Collins, 1973; Day and Rowe, 2002; Richter-Boix et al., 2011). Our results after 21 days of experiment are consistent with this explanation as we did not find differences in development among treatments. However, to confirm whether tadpoles of L lithonaetes are growing faster to reach the threshold size or if they can compensate for the acceleration in development, it is necessary to evaluate time and size to metamorphosis for this species under different water-level treatments.
Many anuran species that accelerate development under desiccation risk develop faster at the expense of growth and with the cost of being smaller at metamorphosis (Kulkarni et al., 2011; Richter-Boix et al., 2011). Nonetheless, assuming that size at metamorphosis had a significant effect on fitness and that growth rates are modulated as a correlated response, tadpoles could grow faster to compensate for the acceleration in development, thus maximizing size at metamorphosis (Richter-Boix et al., 2011). In several species breeding in permanent pools, compensation could be possible if pools offer enough resources to counteract the energetic cost of growth (Richter-Boix et al., 2011). Previous studies in the Orinoquian outcrops have shown substantial levels of primary production in pools and high concentrations of organic matter (Aristizábal-Botero et al., 2020). Hence, living in ephemeral pools, tadpoles of L lithonaetes could grow faster to compensate for accelerated development. Still, this hypothesis needs to be tested, evaluating if acceleration in development occurs in posterior larval stages and evaluating size and time at metamorphosis.
There is another possibility to explain bigger size and weight in tadpoles under dry down treatment. Being semiterrestrial, instead of having a faster development, tadpoles of L. lithonaetes could escape and move to other pools evading costs associated with accelerating development. This idea is supported by the reports of tadpoles of L. lithonaetes on moss out of body waters (Barrientos et al., 2018) and by our observations in field and laboratory, where we found tadpoles moving out of the water on wet surfaces (Figure 3). Congruent with this idea, our results indicate that tadpoles under drying conditions reached a larger size, greater body mass, and higher locomotion performance, suggesting that these responses could allow tadpoles to be more efficient in escaping from a drying pool, moving through narrow water trickles that at times connect some pools.
In other anuran species, the role of body size in tadpole locomotion has been documented. For instance, in larvae of Limnodynastes peronii, maximum acceleration significantly increased with total length (Wilson and Franklin, 2000), and several other studies in pre-metamorphic tadpoles have also reported an increase in swimming velocity with increases in total length (Huey, 1980; Wassersug and Hoff, 1985; Johnson et al., 2015; Lindgren et al., 2018; Cheron et al., 2021). Nonetheless, enhancing growth during the larval stage necessarily requires increasing foraging rate, and tadpoles would, therefore, incur a greater risk of predation (Skelly, 1994; Touchon et al., 2013). It could thus be that L. lithonaetes tadpoles only enhance growth to evade the pool, and transiently increase their predation risk, when they perceive a reduction in the pool’s water level. This possible interplay between predation and desiccation risks should be explicitly tested.
We expected that tadpoles of Leptodactylus lithonaetes facing desiccation risk would exhibit lower locomotor performances, especially at high temperatures. However, we found that under drying conditions tadpoles reached higher locomotion performance without a significant interaction between temperature and water level treatment. This could be an example of hormesis in that a mild physiological stress could be inducing an adaptive response in tadpoles exposed to risk of pond drying (Costantini, 2014). Temperature and water level have the potential to jointly induce carry-over effects on later life stages. Previous work on Rana sphenocephala showed that pond drying conditions during development affect juvenile thermal performance, reducing jumping performance at high temperatures (Ohmer et al., 2023).
While our experiment explored larval responses within a limited time range during the development of Leptodactylus lithonaetes, our results underscore the need to understand the ecological and developmental mechanisms acting on the responses to desiccation risk in extreme and unpredictable environments and in species with particular life histories. Moreover, ecophysiology of species with semiterrestrial tadpoles has not been studied, and future research is needed to confirm the contrasting results found in our study.
Data availability statement
The raw data supporting the conclusions of this article are deposited in the Dryad Digital Repository. doi: 10.5061/dryad.zcrjdfnmm.
Ethics statement
The animal study was approved by Ethical Committee of Los Andes University. The study was conducted in accordance with the local legislation and institutional requirements.
Author contributions
ADM: Conceptualization, Data curation, Formal analysis, Funding acquisition, Investigation, Methodology, Resources, Validation, Visualization, Writing – original draft, Writing – review & editing. AA: Conceptualization, Formal analysis, Funding acquisition, Methodology, Project administration, Resources, Supervision, Validation, Visualization, Writing – original draft, Writing – review & editing. MAA: Investigation, Methodology, Writing – original draft, Writing – review & editing. CG-A: Funding acquisition, Project administration, Resources, Writing – original draft, Writing – review & editing. IG-M: Conceptualization, Formal analysis, Funding acquisition, Methodology, Project administration, Resources, Supervision, Validation, Visualization, Writing – original draft, Writing – review & editing.
Funding
The author(s) declare financial support was received for the research, authorship, and/or publication of this article. This study was supported by Facultad de Ciencias from Universidad de Los Andes-Colombia (grants to Delgadillo Méndez years 2019, 2022, 2023), National Program for Women in Science UNESCO -L’ORÉAL- MINCIENCIAS-ICETEX, 2021, Colombian Ministry of Science, Technology and Innovation (grant # 80740-201-2019 code 120480863597), Plan Nacional I+D of the Spanish Ministry of Science and Innovation (grant # PID2020-119517GB-I00) and Programa QUALIFICA (Junta de Andalucía, Consejería de Universidad, Investigación e Innovación -project QUAL21 020 EBD). The funder was not involved in the study design, data collection, analysis and interpretation, the writing of this article or the decision to submit it for publication
Acknowledgments
We are deeply grateful to Fernando Trujillo, scientific director of the Fundación Omacha, for allowing us to conduct this research at Bojonawi Nature Reserve. For their help in the field and laboratory, we thank Brayan Marín, Esther Marín, Beiker Castañeda, María Antonia Pulido, Diana Motta, Oscar Cornejo, and Felipe Cruz. For their help with figures, we also thank Paula Martin and Carolina Sarmiento.
Conflict of interest
The authors declare that the research was conducted in the absence of any commercial or financial relationships that could be construed as a potential conflict of interest.
The author(s) declared that they were an editorial board member of Frontiers, at the time of submission. This had no impact on the peer review process and the final decision.
Publisher’s note
All claims expressed in this article are solely those of the authors and do not necessarily represent those of their affiliated organizations, or those of the publisher, the editors and the reviewers. Any product that may be evaluated in this article, or claim that may be made by its manufacturer, is not guaranteed or endorsed by the publisher.
References
Abramoff M. D., Magalhaes P. J., Ram S. J. (2004). Image processing with imageJ. Biophotonics Int. 11, 36–42.
Altig R., Johnston G. F. (1989). Guilds of anuran larvae: relationships among developmental modes, morphologies, and habitats. Herpetological Monogr. 3, 81–109. doi: 10.2307/1466987
Amézquita A., Avellaneda M., Barragan A., Delgadillo A., Pulido M. A., Lasso C. A. (2020). “La vida en las rocas: anfibios y reptiles en ambientes extremos del Escudo Guayanés, ViChada, Colombia,” in VIII. Biodiversidad de la Reserva Natural Bojonawi, ViChada, Colombia: río Orinoco y planicie de inundación. Eds. Trujillo F., Morales-Betancourt M. A. (Instituto de Investigación de Recursos Biológicos Alexander von Humboldt, Bogotá, D. C., Colombia).
Aristizábal-Botero Á., Páez-Pérez D., Realpe E. (2020). “Reconstruyendo procesos ecológicos en hábitats aislados con macroinvertebrados acuáticos de los inselbergs del Escudo Guayanés Colombiano,” in VIII. Biodiversidad de la Reserva Natural Bojonawi, ViChada, Colombia: río Orinoco y planicie de inundación (Instituto de Investigación de Recursos Biológicos Alexander von Humboldt, Bogotá, DC, Colombia), 371–389.
Babbitt K. J., Baber M. J., Tarr T. L. (2003). Patterns of larval amphibian distribution along a wetland hydroperiod gradient. Can. J. Zoology 81, 1539–1552. doi: 10.1139/z03-131
Barrientos L. S., Paz A., Lasso E. (2018). Leptodactylus lithonaetes heyer 1995 Sapo de roca (sapo-rana rugoso occidental). Catálogo anfibios y reptiles Colombia 4, 30–36.
Bates D., Mächler M., Bolker B., Walker S. (2015). Fitting linear mixed-effects models using lme4. J. Stat. Software 67, 1–48. doi: 10.18637/jss.v067.i01
Beltrán I., Ramírez-Castañeda V., Rodríguez-López C., Lasso E., Amézquita A. (2019). Dealing with hot rocky environments: Critical thermal maxima and locomotor performance in Leptodactylus lithonaetes (Anura: Leptodactylidae). Herpetological J. 29, 155–161. doi: 10.33256/hj
Boelter T., dos Santos F. M., Moreira L. F. B., Stenert C., Reichard M., Maltchik L. (2020). Effects of hydroperiod on morphology of tadpoles from highland ponds. Aquat. Ecol. 54, 1145–1153. doi: 10.1007/s10452-020-09799-1
Bonilla-Pérez A., Frantz J. C., Charão-Marques J., Cramer T., Franco-Victoria J. A., Mulocher E., et al. (2013). Petrografía, geoquímica y geocronología del Granito de Parguaza en Colombia. Boletín Geología 35, 83–104.
Bremer H., Sander H. (2000). “Inselbergs: geomorphology and geoecology,” in Inselbergs: biotic diversity of isolated rock outcrops in tropical and temperate regions (Springer Berlin Heidelberg, Berlin, Heidelberg), 7–35.
Cabrera-Guzmán E., Crossland M. R., Brown G. P., Shine R. (2013). Larger body size at metamorphosis enhances survival, growth, and performance of young cane toads (Rhinella marina). PloS One 8, e70121. doi: 10.1371/journal.pone.0070121
Cheron M., Raoelison L., Kato A., Ropert-Coudert Y., Meyer X., MacIntosh A. J., et al. (2021). Ontogenetic changes in activity, locomotion and behavioural complexity in tadpoles. Biol. J. Linn. Soc. 134, 165–176. doi: 10.1093/biolinnean/blab077
Christensen R. H. B. (2018). “ordinal—Regression Models for Ordinal Data.” R package version 2019.12.10. Available at: http://www.cran.r-project.org/package=ordinal/.
Cook M. T., Heppell S. S., Garcia T. S. (2013). Invasive bullfrog larvae lack developmental plasticity to changing hydroperiod. J. Wildlife Manage. 77, 655–662. doi: 10.1002/jwmg.509
Costantini D. (2014). Oxidative stress and hormesis in evolutionary ecology and physiology. A marriage between mechanistic and evolutionary approaches. Berlin: Springer, 362 pp. doi: 10.1007/978-3-642-54663-1
Day T., Rowe L. (2002). Developmental thresholds and the evolution of reaction norms for age and size at life-history transitions. Am. Nat. 159, 338–350. doi: 10.1086/338989
De Sá R. O., Grant T., Camargo A., Heyer W. R., Ponssa M. L., Stanley E. (2014). Systematics of the neotropical genus leptodactylus fitzinger 1826 (Anura: Leptodactylidae): Phylogeny, the relevance of non-molecular evidence, and species accounts. South Am. J. Herpetology 9, 1–128. doi: 10.2994/sajh-d-13-00022.1
DeWitt T. J., Langerhans R. B. (2004). Integrated solutions to environmental heterogeneity: theory of multimoment reaction norms. Phenotypic plasticity: Funct. conceptual approaches DeWitt T. J., Scheiner S. M. (New York: Oxford University Press), 98–111. doi: 10.1093/oso/9780195138962.001.0001
Duellman W. E., Trueb L. (1994). Biology of amphibians. (Baltimore, Maryland: JHU press). doi: 10.56021/9780801847806
Fernández M., Cole D., Heyer W. R., Reichle S., De Sá R. O. (2009). Predicting Leptodactylus (Amphibia, Anura, Leptodactylidae) distributions: broad-ranging versus patchily distributed species using a presence-only environmental niche modeling technique. South Am. J. Herpetology 4, 103–116. doi: 10.2994/057.004.0202
Finch W. H., Bolin J. E., Kelley K. (2019). Multilevel modeling using R (New York: CRC Press). doi: 10.1201/9781351062268
Fox J., Weisberg S., Adler D., Bates D., Baud-Bovy G., Ellison S., et al. (2012). Package’’car” (Vienna: R Foundation for Statistical Computing), 16.
Goldberg J., Quinzio S. I., Vaira M. (2022). Lack of response to pond desiccation by eggs and tadpoles of the Yungas Red-belly Toad (Melanophryniscus rubriventris) in an unpredictable environment. Can. J. Zoology 100, 296–302. doi: 10.1139/cjz-2021-0164
Gomez-Mestre I., Kulkarni S., Buchholz D. R. (2013). Mechanisms and consequences of developmental acceleration in tadpoles responding to pond drying. PloS One 8, e84266. doi: 10.1371/journal.pone.0084266
Gomez-Mestre I., Pyron R. A., Wiens J. J. (2012). Phylogenetic analyses reveal unexpected patterns in the evolution of reproductive modes in frogs. Evolution 66, 3687–3700. doi: 10.1111/evo.2012.66.issue-12
Gomez-Mestre I., Saccoccio V. L., Iijima T., Collins E. M., Rosenthal G. G., Warkentin K. M. (2010). The shape of things to come: linking developmental plasticity to post-metamorphic morphology in anurans. J. Evolutionary Biol. 23, 1364–1373. doi: 10.1111/j.1420-9101.2010.02016.x
Gomez-Mestre I., Tejedo M. (2003). Local adaptation of an anuran amphibian to osmotically stressful environments. Evolution 57, 1889–1899. doi: 10.1111/j.0014-3820.2003.tb00596.x
Gosner K. L. (1960). A simplified table for staging anuran embryos and larvae with notes on identification. Herpetologica 16, 183–190.
Heyer W. R. (1995). South American rocky habitat Leptodactylus (Amphibia: Anura: Leptodactylidae) with description of two new species. Proc. Biol. Soc. Washington 108, 695–716.
Heyer W. R., Heyer M. M. (2001). Leptodactylus lithonaetes. Catalog Am. Amphibians Reptiles 723, 1–3.
Huey R. B. (1980). Sprint velocity of tadpoles (Bufo boreas) through metamorphosis. Copeia 3), 537–540. doi: 10.2307/1444534
Huey R. B., Stevenson R. D. (1979). Integrating thermal physiology and ecology of ectotherms: a discussion of approaches. Am. Zoologist 19, 357–366. doi: 10.1093/icb/19.1.357
Husson F., Josse J., Le S., Mazet J., Husson M. F. (2016). Package “FactoMineR” An R package. Available at: https://cran.r-project.org/web/packages/FactoMineR/index.html.
Jocque M., Vanschoenwinkel B., Brendonck L. (2010). Freshwater rock pools: a review of habitat characteristics, faunal diversity, and conservation value. Freshw. Biol. 55, 1587–1602. doi: 10.1111/j.1365-2427.2010.02402.x
Johnson J. B., Saenz D., Adams C. K., Hibbitts T. J. (2015). Naturally occurring variation in tadpole morphology and performance linked to predator regime. Ecol. Evol. 5, 2991–3002. doi: 10.1002/ece3.1538
Kassambara A. (2020). rstatix: a pipe-friendly framework for basic statistical tests. R package version 0.4. 0. Available online at: https://cran.R-project.org/web/packages/rstatix/index.
Kingsolver J. G., Huey R. B. (1998). Evolutionary analyses of morphological and physiological plasticity in thermally variable environments. Am. Zoologist 38, 545–560. doi: 10.1093/icb/38.3.545
Kulkarni S. S., Gomez-Mestre I., Moskalik C. L., Storz B. L., Buchholz D. R. (2011). Evolutionary reduction of developmental plasticity in desert spadefoot toads. J. Evolutionary Biol. 24, 2445–2455. doi: 10.1111/j.1420-9101.2011.02370.x
Kuznetsova A., Brockhoff P. B., Christensen R. H. (2017). lmerTest package: tests in linear mixed effects models. J. Stat. Software. 82, 1–26. doi: 10.18637/jss.v082.i13
Lasso C. A., Ceñaris C. (Eds.) (2018). VI. Fauna Silvestre del Escudo Guayanés (Colombia - VENEZUELA) (Bogotá, D. C., Colombia: Instituto de Investigación de Recursos Biológicos Alexander von Humboldt)438 pp.
Lasso C. A., Morales-Betancourt M. A., Colonnello G. A., Fernández A., Grande J. (2014). “Charcos temporales en afloramientos rocosos del Escudo Guayanés,” in XI. Humedales de la Orinoquia (Colombia- Venezuela). Eds. Lasso C. A., Rial A., Colonnello G., MaChado-Allison A., Trujillo F. (Instituto de Investigación de Recursos Biológicos Alexander von Humboldt (IAvH, Bogotá, D. C., Colombia) 151–152.
Leips J., McManus M. G., Travis J. (2000). Response of treefrog larvae to drying ponds: comparing temporary and permanent pond breeders. Ecology 81, 2997–3008. doi: 10.1890/0012-9658(2000)081[2997:ROTLTD]2.0.CO;2
Lent E. M., Babbitt K. J. (2020). The effects of hydroperiod and predator density on growth, development, and morphology of wood frogs (Rana sylvatica). Aquat. Ecol. 54, 369–386. doi: 10.1007/s10452-020-09748-y
Lenth R. (2022). emmeans: Estimated Marginal Means, aka Least-Squares Means. R package version 1.8.3. Available online at: https://CRAN.R-project.org/package=emmeans.
Liedtke H. C., Wiens J. J., Gomez-Mestre I. (2022). The evolution of reproductive modes and life cycles in amphibians. Nat. Commun. 13, 7039. doi: 10.1038/s41467-022-34474-4
Lindgren B., Orizaola G., Laurila A. (2018). Interacting effects of predation risk and resource level on escape speed of amphibian larvae along a latitudinal gradient. J. Evolutionary Biol. 31, 1216–1226. doi: 10.1111/jeb.13298
Lüdecke D., Ben-Shachar M., Patil I., Waggoner P., Makowski D. (2021). performance: an R package for assessment, comparison and testing of statistical models. J. Open Source Software 6, 3139. doi: 10.21105/joss.03139
Morales-Betancourt M. A., Lasso C. A. (2020). “La Reserva Natural de la Sociedad Civil Bojonawi, río Orinoco, Vichada, Colombia: aspectos abióticos, socioeconómicos y ecosistemas”. In VIII. Biodiversidad de la Reserva Natural Bojonawi, Vichada, Colombia: Rio Orinoco y planicie de inundación. Eds. Trujillo F., Morales-Betancourt M. A. (D. C., Colombia: Instituto de Investigación de Recursos Biológicos Alexander von Humboldt, Bogotá).
Navas C. A. (2006). Patterns of distribution of anurans in high Andean tropical elevations: insights from integrating biogeography and evolutionary physiology. Integr. Comp. Biol. 46, 82–91. doi: 10.1093/icb/icj001
Newman R. A. (1989). Developmental plasticity of Scaphiopus couchii tadpoles in an unpredictable environment. Ecology 70, 1775–1787. doi: 10.2307/1938111
Nunes-de-Almeida C. H. L., Haddad C. F. B., Toledo L. F. (2021). A revised classification of the amphibian reproductive modes. Salamandra 57, 413–427.
Ohmer M. E., Hammond T. T., Switzer S., Wantman T., Bednark J. G., Paciotta E., et al. (2023). Developmental environment has lasting effects on amphibian post-metamorphic behavior and thermal physiology. J. Exp. Biol. 226, jeb244883. doi: 10.1242/jeb.244883
O’Regan S. M., Palen W. J., Anderson S. C. (2014). Climate warming mediates negative impacts of rapid pond drying for three amphibian species. Ecology 95, 845–855. doi: 10.1890/13-0916.1
Pacheco Y., León G. (2001). Clasificación climática de la Orinoquía Colombiana a partir de los patrones de circulación atmosférica. Meteorología Colombiana 4, 117–120.
Porembski S. (2007). Tropical inselbergs: Habitat types, adaptive strategies, and diversity patterns. Rev. Bras. Botânica 30, 579–586. doi: 10.1590/S0100-84042007000400004
R Core Team. (2020). R: A Language and Environment for Statistical Computing. Vienna, Austria: R Foundation for Statistical Computing. https://www.R-project.org/.
Richter-Boix A., Tejedo M., Rezende E. L. (2011). Evolution and plasticity of anuran larval development in response to desiccation. A comparative analysis. Ecol. Evol. 1, 15–25. doi: 10.1002/ece3.2
Ross L. G., Ross B. (2009). Anesthetic and sedative techniques for aquatic animals. (Oxford: John Wiley Sons).
Schalk C. M. (2016). Predator-induced phenotypic plasticity in an arid-adapted tropical tadpole. Austral Ecol. 41, 409–416. doi: 10.1111/aec.12327
Schlichting C. D., Pigliucci M. (1998). Phenotypic evolution: a reaction norm perspective. (Sunderland: Sinauer associates incorporated).
Schneider C. A., Rasband W. S., Eliceiri K. W. (2012). NIH Image to ImageJ: 25 years of image analysis. Nat. Methods 9, 671–675. doi: 10.1038/nmeth.2089
Skelly D. K. (1994). Activity level and the susceptibility of anuran larvae to predation. Anim. Behav. 4, 465–468. doi: 10.1006/anbe.1994.1063
Skelly D. K. (1996). Pond drying, predators, and the distribution of Pseudacris tadpoles. Copeia 3), 599–605. doi: 10.2307/1447523
Snell-Rood E. C., Ehlman S. M. (2021). “Ecology and evolution of plasticity,” in Phenotypic plasticity evolution: causes, consequences, controversies. Ed. Pfennig D. W.. (Oxford: Taylor Francis) 436.
Tejedo M., Marangoni F., Pertoldi C., Richter-Boix A., Laurila A., Orizaola G., et al. (2010). Contrasting effects of environmental factors during larval stage on morphological plasticity in post-metamorphic frogs. Climate Res. 43, 31–39. doi: 10.3354/cr00878
Touchon J. C., Jiménez R. R., Abinette S. H., Vonesh J. R., Warkentin K. M. (2013). Behavioral plasticity mitigates risk across environments and predators during anuran metamorphosis. Oecologia 173, 801–811. doi: 10.1007/s00442-013-2714-8
Wassersug R. J., Hoff K. V. S. (1985). The kinematics of swimming in anuran larvae. J. Exp. Biol. 119, 1–30. doi: 10.1242/jeb.119.1.1
Wellborn G. A., Skelly D. K., Werner E. E. (1996). Mechanisms creating community structure across a freshwater habitat gradient. Annu. Rev. Ecol. systematics 27, 337–363. doi: 10.1146/annurev.ecolsys.27.1.337
West-Eberhard M. J. (2003). Developmental plasticity and evolution (New York: Oxford University Press). doi: 10.1093/oso/9780195122343.003.0008
Wilbur H. M., Collins J. P. (1973). Ecological Aspects of Amphibian Metamorphosis: Nonnormal distributions of competitive ability reflect selection for facultative metamorphosis. Science 182, 1305–1314. doi: 10.1126/science.182.4119.1305
Wilson R. S., Franklin C. E. (2000). Effect of ontogenetic increases in body size on burst swimming performance in tadpoles of the striped marsh frog, Limnodynastes peronii. Physiol. Biochem. Zoology 73, 142–152. doi: 10.1086/316730
Keywords: inselbergs, desiccation risk, tadpoles, developmental plasticity, body size, locomotor performance, temperature, extreme environments
Citation: Delgadillo Méndez A, Amézquita A, Avellaneda Moreno MA, González-Arango C and Gomez-Mestre I (2024) Developmental plasticity to desiccation risk in tadpoles of a tropical inselberg specialist. Front. Ecol. Evol. 12:1370932. doi: 10.3389/fevo.2024.1370932
Received: 15 January 2024; Accepted: 10 May 2024;
Published: 07 June 2024.
Edited by:
Nathan Wolf, Alaska Pacific University, United StatesReviewed by:
Ryan Robert Kerney, Gettysburg College, United StatesNicholas A. Levis, Indiana University, United States
Copyright © 2024 Delgadillo Méndez, Amézquita, Avellaneda Moreno, González-Arango and Gomez-Mestre. This is an open-access article distributed under the terms of the Creative Commons Attribution License (CC BY). The use, distribution or reproduction in other forums is permitted, provided the original author(s) and the copyright owner(s) are credited and that the original publication in this journal is cited, in accordance with accepted academic practice. No use, distribution or reproduction is permitted which does not comply with these terms.
*Correspondence: Alexandra Delgadillo Méndez, adelgadillo@uniandes.edu.co